- Department of Human Nutrition and Hospitality Management, The University of Alabama, Tuscaloosa, AL, United States
Cancer is a major health issue and one of the leading causes of death worldwide. Many natural compounds, e.g., lycopene, curcumin, resveratrol, etc., have been shown to inhibit the growth of cancer cells. Similarly, limonene, a major active component in citrus essential oils and widely used flavoring additive, has demonstrated anticarcinogenic effects in both cell and animal studies. This systematic review aimed to evaluate the anticarcinogenic effects of limonene and its potential underlying mechanisms. Eight peer-reviewed articles published in English between 2000 and 2020 were identified after screening using MEDLINE, Academic Search Premier, and CINAHL plus. All 8 studies showed an effect of limonene on reducing tumor burden, resulting in either decreased size, number, weight, or multiplicities of tumors. Limonene treatment extended the latency and survival periods in 2 studies yet did not reduce tumor incidence rate in another study. Limonene was shown to promote cell apoptosis in 4 studies that examined either the apoptosis index or apoptosis related gene/protein expressions. Two studies tried to explain the cancer preventive mechanisms of limonene and found limonene could restore the antioxidant capacity or immune functions that were impaired by cancer. These results supported the potential applicability of limonene on inhibiting cancer development, yet the real-world applicability on human requires more research and evaluation through clinical studies.
Systematic Review Registration: PROSPERO, identifier: CRD42020168387.
Introduction
Cancer remains a major health issue and is one of the leading causes of death worldwide (National Cancer Institute, 2018). In 2018, there were over 9.5 million cancer-related deaths worldwide (International Agency for Research on Cancer, 2018) an increase of 1.3 million from 2012 (National Cancer Institute, 2018). Cancer is defined as uncontrolled growth of abnormal cells, which results from a non-functioning control mechanism of the body (National Cancer Institute, 2015b). Cancer cells involve several characteristics. First, cancer cells respond to the growth signal from other cells with limitless cell division. Secondly, cancer cells also ignore suppressor genes. Thirdly, programmed cell death (PCD) or apoptosis of is interrupted. In addition, angiogenesis and metastasis are unlimited resulting in increased blood supply to tumors and spread of disease, respectively (National Cancer Institute, 2015b). Many factors could contribute to this dysfunctional system, such as inflammation, obesity-derived growth factors and hormones, and exposure to radiation, etc. (National Cancer Institute, 2015a).
Given the increasing prevalence and severity of cancer, tremendous effort has been made to identify the possible methods to protect humans against it. Research on food-based approach for cancer prevention has been encouraging and inspiring, because a great number of foods and nutraceuticals have been shown to possess cancer preventive properties (Stoner, 2020), for instance, lycopene (Mein et al., 2008), curcumin (Shanmugam et al., 2015; Liu and Ho, 2018), and resveratrol (Athar et al., 2007). The mechanisms underlying the inhibitory effects of these compounds on carcinogenesis could involve reactive oxygen species (ROS) scavenging, interference with cell proliferation, inhibition of cell cycle progression, upregulation of immune function, and modulation of signal transduction pathways (Maheshwari et al., 2006; Athar et al., 2007; Mein et al., 2008).
Among bioactive food compounds, limonene has gained increasing attention as a potential natural anti-cancer agent. Limonene, a cyclic monoterpene, is a colorless oily liquid at room temperature (Crowell et al., 1992; Sun, 2007; Ciriminna et al., 2014). The D-isomer of limonene, D-limonene, occurs more commonly in nature compared to the L-isomer and can be extracted from citrus fruit peels. D-limonene is a major component of citrus essential oil (Fahlbusch et al., 2000) and is widely used as a flavoring agent in food, as well as cosmetics, medical products, personal care items, and cleaning products (Gould, 1997), while L-limonene is mainly found in abundance in pine needles (De Simón et al., 2001). The FDA considers limonene to be Generally Recognized as Safe (GRAS) (U.S. Food and Drug Administration, 2019). In addition to these, limonene is also used as an insecticide, due to its insecticidal property (Ibrahim et al., 2001). Importantly, several in vitro studies have found that limonene could inhibit angiogenesis and metastasis in various human cancer cells (Crowell and Gould, 1994). To better understand the anti-carcinogenic properties of limonene, mechanisms, animal models have been utilized and positive effects have been observed. However, no systematic review has been conducted to examine the effects of limonene on cancer development in animal models. Therefore, this systematic review aims to evaluate the effect of limonene treatment on cancer development in rodent models by assessing tumor incidence, tumor burden, expression of apoptosis or autophagy-related factors, and underlying mechanisms. The information obtained from this systemic review will shed light on the potential applicability of limonene in preventing or treating cancers, and provide guidance on future clinical trials using limonene.
Methods
Methods of the review were developed using the Preferred Reporting Items for Systematic Reviews and Meta-Analyses (PRISMA) guidelines (Mcinnes et al., 2018). Prior to the literature search and data extraction, this review was registered and approved with PROSPERO International prospective register of systematic reviews as CRD42020168387.
Literature Search Strategy
Three electronic databases (MEDLINE, Academic Search Premier, and CINAHL plus with Full Text) were searched in May 2020 to identify, screen, and assess primary research reports for inclusion. Search criteria included the following: full-text, peer-reviewed, English language studies published between January 2000 and May 2020. Boolean search logic included the terms “limonene,” “mice or rats or rodents,” and “cancer or tumor or carcinoma or malignancy or neoplasms or tumor.” Searches were snowballed to include bibliographies, citations, and coauthors of relevant publications.
Study Selection
Studies that documented limonene treatment in any format on either mouse or rat model and reported at least one outcome related to the following: (1) latency period, (2) incidence rate, (3) survival period, (4) tumor burden, (5) apoptosis or autophagy-related factors, and (6) underlying molecular mechanisms were eligible and included. Review papers, cell line studies, conference abstracts, short communications, and case studies were excluded. Any disagreement related to inclusion or exclusion of studies was discussed until all authors reach a consensus.
Data Extraction
Data extraction was conducted by the primary researcher and reviewed by the research team. The Cochrane data extraction form was used, which included “participants,” “interventions,” comparison groups, outcomes, and study design (Higgins et al., 2019). The following outcomes were consider for inclusion: (1) latency or survival period or incidence rate, (2) tumor burden, (3) apoptosis or autophagy-related factors, and (4) underlying mechanisms (Tables 2–5). In the identified studies, latency, survival period, and incidence rate were obtained directly through visual observation. Tumor burden was indicated by tumor size, weight, number, or multiplicity. Apoptosis and autophagy-related genes and proteins were measured by different techniques, such as polymerase chain reaction (PCR), immunohistochemical assay, and Western-blot. Underlying mechanisms were determined by examining different cancer-related pathways.
Quality Assessment
The SYRCLE's Risk of Bias (SYRCLE's RoB) tool was employed to assess the article qualities (Hooijmans et al., 2014). The quality assessment tool includes 10 validity questions for evaluating the research studies. Factors assessed by these validity questions include 6 types of bias: selection bias, performance bias, detection bias, attrition bias, reporting bias and other biases (Table S1 in Supplementary Material), and each of these questions involves several sub-questions. These questions altogether in the checklist address the applicability to practice and scientific validity. For each sub-question, 3 choices are provided: yes, no, and unclear. For the overall quality rating, 3 options are provided as well: positive, neutral, and negative, which is straightforward to tell and conclude. Furthermore, this quality checklist is unique for animal studies.
Therefore, the SYRCLE's RoB tool is a proper and suitable quality assessment tool to be used in this systematic review. Biases and limitations of individual studies are discussed later in this review. Authors conducted quality assessment individually and any disagreement was discussed until a consensus was reached.
Results
A total of 146 articles were identified from database searches. Snowballing of searches yielded an additional 6 articles. After removal of duplicates and screening of titles and abstracts, 35 full-text articles were eligible for full-text review. Studies that did not use cancer models or did not directly perform “intervention” using limonene were excluded. Ultimately, 8 studies were included in this systematic review (Figure 1).
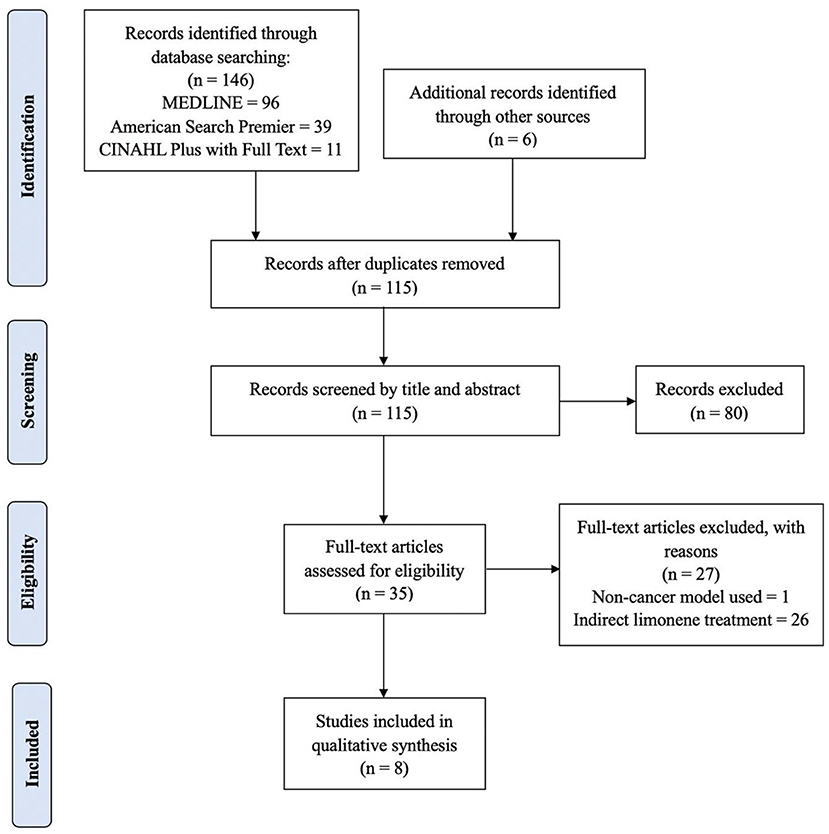
Figure 1. Flow diagram of literature search and selection procedure for research included in the systematic review.
Study Characteristics and Description
The basic characteristics of the included studies are summarized in Table 1. Of the 8 studies, 6 tested the effect of limonene on cancer development using mouse models (Witschi, 2000; Witschi et al., 2000; Lu et al., 2004; Del Toro-Arreola et al., 2005; Chaudhary et al., 2012; Yu et al., 2018) and 2 used rat models (Kaji et al., 2001; Asamoto et al., 2002). In terms of the choices of cancer models, 3 studies used lung cancer (Witschi, 2000; Witschi et al., 2000; Yu et al., 2018), while skin, lymph, liver, stomach, and mammary gland cancer models were used in each of the rest of the studies, respectively. Among the 8 studies, limonene was delivered by mixing into the diet in 5 studies (Witschi, 2000; Witschi et al., 2000; Del Toro-Arreola et al., 2005), while in the other 3 studies, limonene treatments were applied via either oral gavage, or gastric perfusion, or topical administration. Results from the 8 studies were grouped into four categories according to different outcomes evaluated, including: (1) latency or survival period or incidence rate, (2) tumor burden, (3) apoptosis or autophagy-related factors, and (4) underlying mechanisms (Tables 2–5). All of the 8 studies measured tumor burden by different measurements and only 1 study measured all 4 categories of outcomes (Chaudhary et al., 2012).
Effect on Latency or Survival Period or Incidence Rate
Three of the 8 articles reported the effect of limonene treatment on latency period or survival period or tumor incidence during cancer development (Table 2), with latency period indicates the amount of time that elapses between the initial exposure to a carcinogen and the diagnosis of cancer (National Cancer Institute NCI Dictionary of Cancer Terms [Online]); survival period reveals the life span of the test subjects; and tumor incidence rate represents the number of new cancers of a specific site or type occurring (National Cancer Institute Cancer Incidence Rates [Online]).
Chaudhary et al. (2012) induced skin cancer in female Swiss albino mice through repeated application of 12-O-tetradecanoylphorbol 13-acetate (TPA), and by topically applying limonene at 100 mg/kg of body weight (BW), the latency period of tumor induction was delayed by 5 weeks compared to the control group. Del Toro-Arreola et al. (2005) demonstrated that with limonene supplementation in diet (100 g/kg), the life span of lymphoma bearing mice was significantly (p < 0.004) increased from 21 to 25 days. Witschi et al. (2000) examined limonene's effects on tobacco smoke-induced lung cancer by using male A/J mice. However, limonene treatment in diet at 6.3 g/kg did not pose significant effect on tumor incidence rate in mice with lung cancer.
Effect on Tumor Burden
All of the 8 studies looked into the effect of limonene treatment on tumor burden using different methodologies (Table 3). Five of the 8 studies measured the tumor size (Kaji et al., 2001; Asamoto et al., 2002; Del Toro-Arreola et al., 2005; Chaudhary et al., 2012; Yu et al., 2018) and unanimously found that limonene could shrink tumor size, by either observing tumor diameter or volume. Asamoto et al. (2002) treated female Hras128 rats with N-methyl-N-nitrosourea (MNU) to induce mammary tumor. By supplementing limonene at 50 g/kg of diet for 8 weeks, tumor size and volume were significantly reduced from 10.7 ± 7.34 mm to 636.7 ± 1,669.3 mm3 to 8.23 ± 4.88 mm (p < 0.002) and 269.5 ± 735.5 mm3 (p < 0.008), respectively. Tumor number was also reduced but not significantly altered by limonene treatment. Chaudhary et al. (2012) treated the mice bearing skin cancer with limonene at either 50 or 100 mg/kg BW and observed reduction of tumor size in 100 mg/kg BW treatment group. Kaji et al. (2001) induced hepatocarcinogenesis in male Sprague-Dawley rats by providing the animal with drinking water containing N-nitrosomorpholine. By supplementing limonene at either 10 or 30 g/kg of diet, mean tumor size was significantly decreased from 0.26 ± 0.002 mm2 to 0.20 ± 0.01 mm2 (p < 0.05) and 0.18 ± 0.01 mm2 (p < 0.001), respectively. Yu et al. (2018) subcutaneously injected cancer cell (A549) suspension into female BALB/c mice to form lung cancer. The limonene treatment at 400 or 600 mg/kg of BW for 4 weeks significantly reduced the tumor size from 1,251.99 mm3 to 867.44 mm3 (p < 0.05) and 577.08 mm3 (p < 0.001), respectively. Del Toro-Arreola et al. (2005) also studied the effects of limonene on tumor size. However, they only mentioned the significant reduction of tumor size upon limonene supplementation at 100 g/kg of diet without providing statistical results. Among these 5 studies, both Kaji et al. (2001) and Yu et al. (2018) examined and observed dose-dependent pattern.
Four studies found that limonene treatment reduced tumor weight (Lu et al., 2004; Del Toro-Arreola et al., 2005; Chaudhary et al., 2012; Yu et al., 2018), and among these 4 studies, Lu et al. (2004), Chaudhary et al. (2012), and Yu et al. (2018) reported significant reductions; only Chaudhary et al. (2012) and Yu et al. (2018) observed dose-dependent relationship. Chaudhary et al. (2012) observed that by comparing with the 48% increase of tumor weight in control group, 50 and 100 mg/kg of BW limonene treatment could reduce such increase to 27% (p < 0.05) and 9% (p < 0.001), respectively. Lu et al. (2004) subcutaneously injected gastric cancer cell (BGC-823) suspension into male BALB/c mice to form gastric cancer, and supplementing limonene at 15 mL/kg of BW via gastric perfusion for 7 weeks could significantly decrease the tumor weight from 2.69 ± 0.32 to 1.41 ± 0.58 g (p < 0.05). Yu et al. (2018) found that limonene treatment at 400 or 600 mg/kg of BW significantly reduced the tumor weight from 0.77 g to 0.48 g (p < 0.05) and 0.31 g (p < 0.001), respectively. Although Del Toro-Arreola et al. (2005) also reported decreased tumor weight upon limonene treatment, the p-value related to the change was not provided.
In addition, 5 studies measured tumor numbers and all observed decreased tumor number upon limonene treatment (Witschi, 2000; Witschi et al., 2000; Kaji et al., 2001; Asamoto et al., 2002; Chaudhary et al., 2012). Among these 5 studies, 2 of them reported significant reduction in a dose-response manner (Kaji et al., 2001; Chaudhary et al., 2012). According to Chaudhary et al. (2012), tumor number could be significantly reduced by limonene treatment at 50 and 100 mg/kg of BW from ~20 to ~15 (p < 0.05) and ~10 (p < 0.005), respectively. Asamoto et al. (2002) also observed a tumor number reduction, but not significant. Kaji et al. (2001) found significant foci number decrease when the rats were treated with limonene at 10 or 30 g/kg of diet, and the foci number was reduced from 141.7 ± 7.8 to 98.6 ± 5.0 (p < 0.001) and 84.4 ± 5.7 (p < 0.001), respectively. Two studies done by Witschi (2000) and Witschi et al. (2000) both investigated limonene's effect on tobacco smoke-induced lung cancer by using A/J mice and observed reduction of lung tumor number upon limonene treatment at 6.3 g/kg of diet. Witschi (2000) observed tumor number reduction from 100 to 60%; Witschi et al. (2000) found that tumor number was decreased from 7.8 ± 0.8 to 13.0 ± 1.2.
Tumor inhibition rate was measured in 2 studies (Lu et al., 2004; Yu et al., 2018), and both found that limonene treatment could largely decrease the tumor growth rate. However, only Yu et al. (2018) observed dose-dependent relationship. They found that tumor inhibition rate could be raised from 0% to 37.08% and 60.03% upon limonene supplementation at 400 or 600 mg/kg of BW, respectively. Lu et al. (2004) found that the limonene treatment at 15 mL/kg of BW could significantly increase the tumor inhibition rate from 0% to 47.58% (p < 0.05).
Effect on Apoptosis or Autophagy-Related Factors
Three studies analyzed apoptosis or autophagy-related factors as affected by limonene treatment (Table 4). Yu et al. (2018) and Chaudhary et al. (2012) tested the expression of apoptosis-related proteins Bax and Bcl2. Bax is a pro-apoptosis protein and plays a crucial role in outer mitochondrial membrane damage (Kim-Campbell et al., 2019). Bcl2 is a negative regulator of apoptosis, which can form a heterodimer complex with Bax and thereby offset the effect of pro-apoptosis (Chaudhary et al., 2012). Both studies found that higher dosage of limonene treatment significantly increased Bax expression and significantly decreased Bcl2 expression. Furthermore, Yu et al. (2018) reported that the expression of apoptosis-related gene bax was significantly increased and that of bcl2 was significantly decreased. In addition, with limonene treatment, the expression of apoptosis-related protein (cleaved-PARP), LC3-II, and Atg5 proteins, and the lc3b and beclin1 gene expressions were all significantly increased. Chaudhary et al. (2012) tested the expression of some proteins that regulate cell division, such as membrane-bond Ras, Raf, and p-Erk1/2. All of these proteins have cell proliferation promoting function (Chang et al., 2003) and their relative expressions were all reduced upon limonene treatment.
Kaji et al. (2001) and Lu et al. (2004) measured the apoptotic index, which is the percentage of apoptotic cells per tumor cell (Potten, 1996). Both studies found that the apoptotic index was significantly increased by limonene treatment compared with the control group, and Kaji et al. (2001) found the effect was in a dose-dependent manner, where 10 and 30 g/kg of diet limonene treatment significantly increased the apoptotic index from 1.3 ± 0.2 to 2.7 ± 0.2 % (p < 0.001) and 3.0 ± 0.2 % (p < 0.001), respectively.
Underlying Mechanisms
The 2 articles that examined the underlying mechanisms of limonene on retarding cancer development are summarized in Table 5. Chaudhary et al. tested both immune function and activity of redox-related enzymes (Chaudhary et al., 2012). They found that the limonene treatment could induce the leukocyte infiltration and increase the reduced redox enzymes' activities, such as glutathione (GSH), glutathione S-transferase (GST), glutathione peroxidase (GPX), etc. In addition, the increased ornithine decarboxylase (ODC) activity that caused by cancer could be significantly reduced by limonene treatment in a dose-dependent manner. After limonene treatment at either 50 or 100 mg/kg of BW, GSH activity was increased from 49 to 62% and 81% (p < 0.01), respectively; GR activity was raised from 50 to 72% (p < 0.01) and 85% (p < 0.001), respectively; GPX activity was leveled up from 63 to 75% (p < 0.05) and 87% (p < 0.001), respectively. Meanwhile, ODC activity was significantly reduced from 833 to 793% (p < 0.001) and 765% (p < 0.001), respectively.
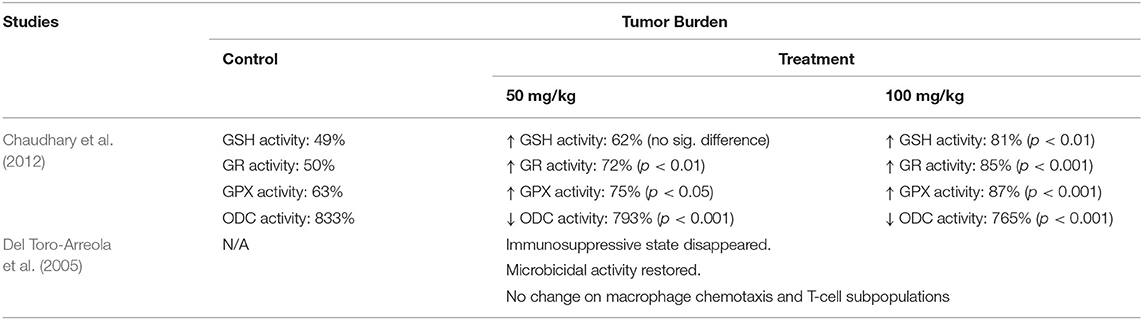
Table 5. Studies investigating underlying mechanisms of the preventative effect of limonene on cancer development.
Del Toro-Arreola et al. (2005) showed that the immunosuppressive state caused by cancer disappeared after supplying the limonene at 100 g/kg in normal diet. Also, the limonene fortified diet could restore the defective phagocytosis and the decreased microbicidal activity. However, the treatment did not affect macrophage chemotaxis and T-cell subpopulations, which means that the ability of eliminating foreign substances and producing antibodies were not affected upon limonene treatment.
Quality of Results
Based on the SYRCLE's RoB tool (Hooijmans et al., 2014), only 1 study (Yu et al., 2018) was rated positive among all eligible studies. All of the studies adequately generated and applied the allocation sequence, and adequately concealed the allocation. In addition, most of the 8 studies described all the possible prognostic factors or animal characteristics to ensure the similarity of control and intervention groups at the start of the experiment, except the studies done by Witschi (2000), Asamoto et al. (2002), and Lu et al. (2004). In addition, all of the studies provided their detailed interventions and comparators. Hence, 5 studies are totally free of selection bias (Witschi et al., 2000; Kaji et al., 2001; Del Toro-Arreola et al., 2005; Chaudhary et al., 2012; Yu et al., 2018). In addition, all of the 8 selected studies randomly housed the animals during the experiment. However, none of the caregivers and/or investigators were blind to prevent introduction of bias. Thus, all of these studies have relatively high risk of performance bias. None of these studies clearly described whether the animals were randomly selected for outcome measurements and none of the outcome assessors in those studies were blinded. These 2 factors together lead to high risk of detection bias. Furthermore, none of these studies adequately addressed the incomplete outcome data, except for 1 study done by Lu et al. (2004), which means that most of these selected studies have medium risk of attrition bias. For the reporting bias, studies done by Kaji et al. (2001), Asamoto et al. (2002), Lu et al. (2004) Chaudhary et al. (2012), and Yu et al. (2018), are free of selective outcome reporting issue. Lastly, only 1 study done by Yu et al. (2018) is free of other risk of bias (Table S1 in Supplementary Material).
Discussion
Main Results
According to the results from the 8 articles, limonene showed potent cancer prevention and control effects (Figure 2). Supplementing limonene topically, or through diet, or via oral gavage could suppress tumor growth by decreasing the size, number, weight, multiplicities of tumors in a dose-dependent manner. Limonene treatment could extend the latency and survival period of carcinoma bearing rodents, however, the effect on controlling tumor incidence rate is inconclusive. These findings agree with many review and primary research articles that demonstrated the chemopreventive and chemotherapeutic activity of limonene on various types of tumors in rodents (Maltzman et al., 1989; Wattenberg et al., 1989; Wattenberg and Coccia, 1991; Crowell et al., 1992; Crowell and Gould, 1994; Giri et al., 1999).
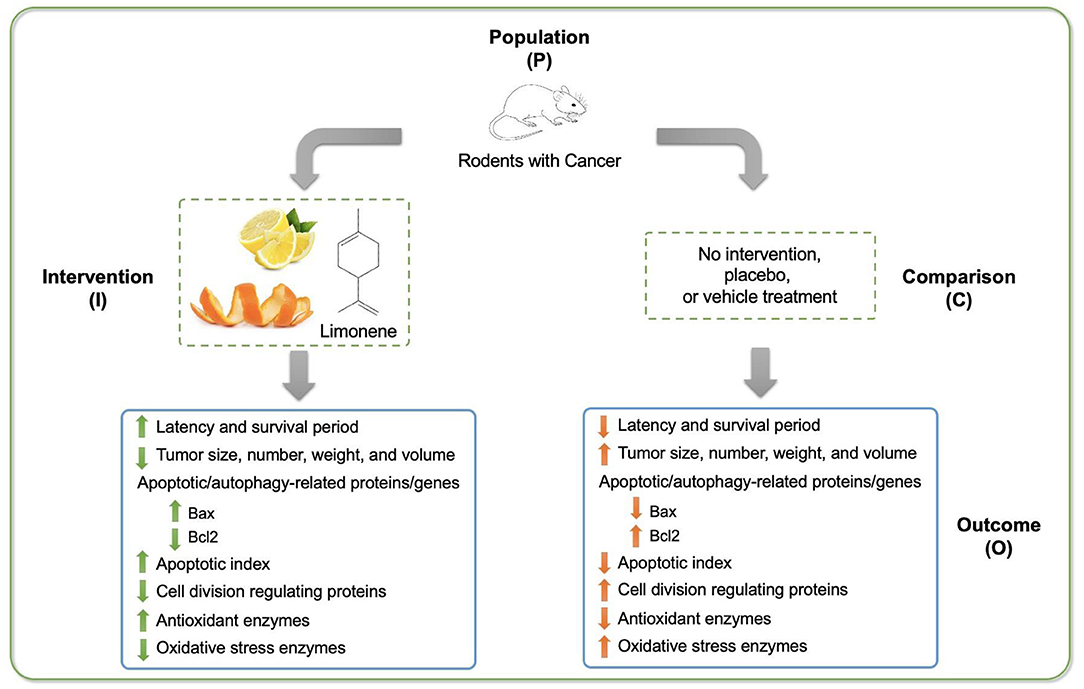
Figure 2. Schematic diagram of major findings from the research evaluating the effect of limonene on cancer development in rodent models.
To better understand the underlying mechanisms of limonene's anti-cancer effect, the activities of redox-related enzymes were studied. During cancer development, antioxidant activity is largely damaged, while the activities of reactive oxygen species and oxidative stress enzymes are dramatically increased. Examining the activity of several important antioxidants and oxidative stress enzymes, such as GSH, GR, GPX, and ODC, could clearly demonstrate that limonene restored the antioxidant capacity by elevating antioxidant enzymes and decreasing oxidative stress enzymes. Also, during cancer development, immune system function would be attacked and injured. Limonene treatment was found to be able to restore the immune functions, suggesting that its immune-protective effects conduce to its chemoprotective effects.
Furthermore, during cancer cell growth, cell cycle function is mostly out of control and cell apoptosis and/or autophagy are almost eliminated. Hence, expressions of several apoptotic- and cell division-related genes and proteins, such as Bax (and bax), Bcl2 (and bcl2), Ras, Raf, and p-Erk1/2, were measured to test the limonene's proapoptotic function. The significant increase in Bax (and bax) shows limonene's potent proapoptotic property. On the other hand, the significant decrease of Bcl2 (and bcl2), Ras, Raf, and p-Erk1/2 illustrates limonene's cell division inhibitory function. Thus, the proapoptotic and cell division inhibitory properties of limonene also play a critical role in its anti-cancer function.
Comparing with other bioactive food compounds, e.g., resveratrol, curcumin, and lycopene, there are limited studies on the cancer preventive effect of limonene using animal models and human subjects. Resveratrol is a phytoalexin agent, which is commonly found in red wine and grape skins (Rauf et al., 2018). Aluyen et al. (2012) systematically reviewed 11 in-vitro, in-vivo and pharmacokinetic human studies and observed that the pro-apoptotic, anti-proliferation, and anti-inflammation properties contributed to the anti-cancer effect of resveratrol. Besides, significant inhibitory effect of resveratrol on various types of cancer, including skin cancer, breast cancer, colorectal cancer, and liver cancer, were observed in preclinical animal models (Ko et al., 2017). However, few clinical trials observed beneficial effects of resveratrol in cancer models, e.g., colorectal, myeloma, and prostate cancer (Nguyen et al., 2009; Patel et al., 2010; Howells et al., 2011; Popat et al., 2013; Kjær et al., 2015; Paller et al., 2015). Curcumin, the active ingredient of the Curcuma longa plant, is another well-known bioactive compound that has received great attention over the past decades (Kocaadam and Sanlier, 2017). Many studies demonstrated its antioxidant, anti-inflammatory, and anti-cancer properties (Ravindranath and Chandrasekhara, 1980; Ruby et al., 1995; Divya and Pillai, 2006). It showed promising actions in suppressing cancer cell proliferation in various types of cancer in both cell-line and animal studies, such as colorectal, breast, pancreatic, brain, and prostate cancers (Tomeh et al., 2019). Besides, by reviewing 18 clinical studies, Tomeh et al. (2019) found that the chemoprotective effects of curcumin against cancer were controversial, since not all of the studies observed clear protective effects. Lycopene, a bright red carotenoid pigment found in red colored fruits and vegetables, demonstrates the most potent antioxidant activity among various types of carotenoids (Gajowik and Dobrzynska, 2014). Lycopene could quench singlet oxygen and scavenge free radicals, which may protect against oxidation of proteins, lipids, and DNA (Yaping et al., 2002; Stahl and Sies, 2003). Besides the antioxidant properties, lycopene also possesses regulatory power on gap-junction communication (Zhang et al., 1991, 1992), i.e., suppressing carcinogen-induced phosphorylation of regulatory proteins, such as p53, and stop cell division at the G0–G1 cell cycle phase. Giovannucci (1999) reviewed 72 epidemiological studies and found that higher consumption of tomatoes, tomato-based products, and lycopene (a compound derived predominantly from tomatoes) had prominent effects on regulating prostate, lung, and stomach cancers. Similar to these bioactive food compounds, limonene demonstrated antioxidant properties and thus chemoprotective effect in the rodent models.
Applicability of Limonene Treatment
If definitive protective effects of limonene on cancer could be observed in animal studies, it would be feasible to test and apply limonene treatment in clinical trials. Limonene can be found in many common food items and is listed in the United States Code of Federal Regulations as generally recognized as safe (GRAS) for use as a flavoring additive. According to Kim et al. (2013), the no-observed-adverse-effect level (NOAEL) of limonene was determined to be 250 mg/kg/day. Therefore, limonene treatment dosage can be kept lower than the NOAEL value in order to prevent adverse effect or toxicity. Additionally, the appropriate cancer model should also be taken into consideration. Since limonene is used as a flavor or fragrance additives in food and cosmetic products, it is generally applied on skin or consumed orally. Hence, skin, oral, and gastrointestinal cancers may be easier to target by limonene.
The targeted delivery of limonene can be achieved by certain delivering approaches, e.g., systemic administration and localized delivery by carriers (Rosenblum et al., 2018), which could be liposomes, microparticles, polymeric films, and hydrogels. Encapsulation of drugs in carriers could improve drug's solubility and bioavailability. It can also alter drug's bio-distribution and facilitate entry into the target cell. However, systemic administration approach is hard to achieve due to multiple physiological barriers, such as tumor penetration, tumor heterogeneity, and endosomal escape (Rosenblum et al., 2018), which can all limit the benefits of targeted carriers. By contrast, localized delivery has been found to increase local residence time of the drug and enable controlled release (Rosenblum et al., 2018). This approach can significantly improve pharmacological benefit at the disease site and reduce systemic toxicity compared to systemic administration.
Accordingly, for skin cancer, local drug delivery, such as topical administration of limonene, could be appropriate. This drug delivery method could enhance the pharmacological benefit of the drug at the specific targeted disease site and reduce systemic toxicity compared to systemic administration. However, the systemic absorption through the skin should be minimized in order to maximize the effects of limonene. For oral and gastrointestinal cancer, limonene could be encapsulated for drug delivery as the release of limonene could be controlled by digestive enzymes upon reaching the target tissues or absorption sites. For example, limonene could be incorporated into starch-limonene inclusion complex in chewing gum to target oral cancer (Shi et al., 2019). Upon chewing, limonene could be released from the inclusion complex due to the action of salivary α-amylase. The inclusion complex could also be applied into other foods for ingestion to target other tissues along the gastrointestinal tract.
Strengths and Limitations
To the author's knowledge, this is the first systematic review that examine the effect of limonene on cancer development in animal models. The cancer protective effect of limonene and limonene-related compounds have been previously discussed in several narrative reviews (Crowell and Gould, 1994; Crowell, 1999; Sun, 2007). This review focuses on various aspects of limonene's effects on cancer development by assessing tumor incidence, tumor burden, expression of apoptosis or autophagy-related factors, and underlying mechanisms. Despite some results lacking statistical analysis or significance, the majority of the evidences showed the inhibitory effect of limonene on cancer development in rodents.
Despite the strengths, several limitations must also be acknowledged in this systematic review. First, only studies using rodent models were included in this review, due to the absence of clinical studies evaluating limonene. Although rodent models are commonly used to investigate the actions of anti-cancer drugs, animal experiments cannot fully predict the effect of limonene in human cancers. Since the overall aim of using this systematic review is to plan and conduct clinical trials in the future, the inferior animal studies should be considered as indirectness. Secondly, the heterogeneity of rodent and cancer models make it difficult to draw a conclusion, as the rodent models of these 8 studies had different strains, age, or gender; besides, these 8 studies addressed different types of cancer: 3 studies used lung cancer model, while skin, lymph, liver, stomach, and mammary gland cancer models were used in each of the rest of the studies. Together, the heterogeneity of rodent and cancer models could lead to indirectness and inconsistency. Accordingly, the grade of evidence for all the 4 outcomes discussed in this review should start as very low (Wei et al., 2016). Lastly, the dosage of limonene treatment was based on body weight in some studies, while it was calculated using diet compositions in the other studies. The interconversion of these could be done only through rough estimation, if ever possible.
Conclusions
In conclusion, this systematic review examines the effect of limonene treatment on cancer development in studies using rodent models. In various cancer models, limonene treatment showed beneficial effects against cancer by reducing the size, number, weight, and multiplicities of tumors, extending latency and survival periods, promoting cell apoptosis, and enhancing antioxidant activity and immune functions. Based on the findings, current literature supported the potential application of limonene on inhibiting cancer development. However, more research studies are warranted before a conclusive statement can be reached.
Future research may focus on various types of cancer models, e.g., skin, oral, and gastrointestinal cancer, that are appropriate for limonene treatment, since limonene is a component of essential oils and a food flavoring additive. Proper drug delivery system for limonene may be investigated in order to maximize the bioavailability or efficacy of limonene. In addition, clinical studies are needed to determine the anti-cancer properties of limonene on human.
Data Availability Statement
The original contributions presented in the study are included in the article/Supplementary Material, further inquiries can be directed to the corresponding author.
Author Contributions
JZ: investigation, formal analysis, visualization, and writing. MA: supervision and writing—review and editing. LK: conceptualization, supervision, formal analysis, visualization, and writing—review and editing. All authors contributed to the article and approved the submitted version.
Conflict of Interest
The authors declare that the research was conducted in the absence of any commercial or financial relationships that could be construed as a potential conflict of interest.
Publisher's Note
All claims expressed in this article are solely those of the authors and do not necessarily represent those of their affiliated organizations, or those of the publisher, the editors and the reviewers. Any product that may be evaluated in this article, or claim that may be made by its manufacturer, is not guaranteed or endorsed by the publisher.
Supplementary Material
The Supplementary Material for this article can be found online at: https://www.frontiersin.org/articles/10.3389/fsufs.2021.725077/full#supplementary-material
References
Aluyen, J. K., Ton, Q. N., Tran, T., Yang, A. E., Gottlieb, H. B., and Bellanger, R. A. (2012). Resveratrol: potential as anticancer agent. J. Diet. Suppl. 9, 45–56. doi: 10.3109/19390211.2011.650842
Asamoto, M., Ota, T., Toriyama-Baba, H., Hokaiwado, N., Naito, A., and Tsuda, H. (2002). Mammary carcinomas induced in human c-Ha-ras proto-oncogene transgenic rats are estrogen-independent, but responsive to d-limonene treatment. Japan. J. Cancer Res. 93, 32–35. doi: 10.1111/j.1349-7006.2002.tb01197.x
Athar, M., Back, J. H., Tang, X., Kim, K. H., Kopelovich, L., Bickers, D. R., et al. (2007). Resveratrol: a review of preclinical studies for human cancer prevention. Toxicol. Appl. Pharmacol. 224, 274–283. doi: 10.1016/j.taap.2006.12.025
Chang, F., Steelman, L. S., Shelton, J. G., Lee, J. T., Navolanic, P. M., Blalock, W. L., et al. (2003). Regulation of cell cycle progression and apoptosis by the Ras/Raf/MEK/ERK pathway. Int. J. Oncol. 22, 469–480. doi: 10.3892/ijo.22.3.469
Chaudhary, S., Siddiqui, M., Athar, M., and Alam, M. S. (2012). D-Limonene modulates inflammation, oxidative stress and Ras-ERK pathway to inhibit murine skin tumorigenesis. Human Experim. Toxicol. 31, 798–811. doi: 10.1177/0960327111434948
Ciriminna, R., Lomeli-Rodriguez, M., Cara, P. D., Lopez-Sanchez, J. A., and Pagliaro, M. (2014). Limonene: a versatile chemical of the bioeconomy. Chem. Commun. 50, 15288–15296. doi: 10.1039/C4CC06147K
Crowell, P. L. (1999). Prevention and therapy of cancer by dietary monoterpenes. J. Nutri. 129, 775S−778S. doi: 10.1093/jn/129.3.775S
Crowell, P. L., and Gould, M. N. (1994). Chemoprevention and therapy of cancer by d-limonene. Critic. Reviews™ Oncogen. 5, 1–22. doi: 10.1615/CritRevOncog.v5.i1.10
Crowell, P. L., Kennan, W. S., Haag, J. D., Ahmad, S., Vedejs, E., and Gould, M. N. (1992). Chemoprevention of mammary carcinogenesis by hydroxylated derivatives of d-limonene. Carcinogenesis 13, 1261–1264. doi: 10.1093/carcin/13.7.1261
De Simón, B. F., Vallejo, M. C. G., Cadahía, E., Miguel, C. A., and Martinez, M. C. (2001). Analysis of lipophilic compounds in needles of Pinus pinea L. Ann. For. Sci. 58, 449–454. doi: 10.1051/forest:2001138
Del Toro-Arreola, S., Flores-Torales, E., Torres-Lozano, C., Del Toro-Arreola, A., Tostado-Pelayo, K., Ramirez-Dueñas, M. G., et al. (2005). Effect of D-limonene on immune response in BALB/c mice with lymphoma. Int. Immunopharmacol. 5, 829–838. doi: 10.1016/j.intimp.2004.12.012
Divya, C. S., and Pillai, M. R. (2006). Antitumor action of curcumin in human papillomavirus associated cells involves downregulation of viral oncogenes, prevention of NFkB and AP-1 translocation, and modulation of apoptosis. molecular carcinogenesis: published in cooperation with the University of Texas MD Anderson. Cancer Center 45, 320–332. doi: 10.1002/mc.20170
Fahlbusch, K. G., Hammerschmidt, F. J., Panten, J., Pickenhagen, W., Schatkowski, D., Bauer, K., et al. (2000). Flavors and fragrances. Ullmann's encyclopedia of industrial chemistry.
Gajowik, A., and Dobrzynska, M. M. (2014). Lycopene-antioxidant with radioprotective and anticancer properties. a review. Roczniki Państwowego Zakładu Higieny 65, 263–271. Available online at: https://agro.icm.edu.pl/agro/element/bwmeta1.element.agro-e15a5315-b6b0-43e0-bccc-beda4a610cfc
Giovannucci, E. (1999). Tomatoes, tomato-based products, lycopene, and cancer: review of the epidemiologic literature. J. Natl. Cancer Inst. 91, 317–331. doi: 10.1093/jnci/91.4.317
Giri, R. K., Parija, T., and Das, B. R. (1999). d-limonene chemoprevention of hepatocarcinogenesis in AKR mice: inhibition of c-jun and c-myc. Oncol. Rep. 6, 1123–1130. doi: 10.3892/or.6.5.1123
Gould, M. N. (1997). Cancer chemoprevention and therapy by monoterpenes. Environ. Health Perspect. 105, 977–979. doi: 10.1289/ehp.97105s4977
Higgins, J., T. J Chandler, J., Cumpston, M., Li, T., Page, M., et al. (2019). Cochrane Handbook for Systematic Reviews of Interventions [Online]. Available online at: https://training.cochrane.org/handbook/current [accessed 04/24/2020].
Hooijmans, C. R., Rovers, M. M., De Vries, R. B., Leenaars, M., Ritskes-Hoitinga, M., and Langendam, M. W. (2014). SYRCLE's risk of bias tool for animal studies. BMC Med. Res. Methodol. 14:43. doi: 10.1186/1471-2288-14-43
Howells, L. M., Berry, D. P., Elliott, P. J., Jacobson, E. W., Hoffmann, E., Hegarty, B., et al. (2011). Phase I randomized, double-blind pilot study of micronized resveratrol (SRT501) in patients with hepatic metastases—safety, pharmacokinetics, and pharmacodynamics. Cancer Prevent. Res. 4, 1419–1425. doi: 10.1158/1940-6207.CAPR-11-0148
Ibrahim, M. A., Kainulainen, P., Aflatuni, A., Tiilikkala, K., and Holopainen, J. K. (2001). Insecticidal, repellent, antimicrobial activity and phytotoxicity of essential oils: with special reference to limonene and its suitability for control of insect pests. Agric. Food Sci. 12:5697. doi: 10.23986/afsci.5697
International Agency for Research on Cancer (2018). Cancer Fact Sheet - All Cancers [Online]. Available online at: https://gco.iarc.fr/today/data/factsheets/cancers/39-All-cancers-fact-sheet.pdf.
Kaji, I., Tatsuta, M., Iishi, H., Baba, M., Inoue, A., and Kasugai, H. (2001). Inhibition by D-limonene of experimental hepatocarcinogenesis in Sprague-Dawley rats does not involve p21ras plasma membrane association. Int. J. Cancer 93, 441–444. doi: 10.1002/ijc.1353
Kim, Y. W., Kim, M. J., Chung, B. Y., Bang, D. Y., Lim, S. K., Choi, S. M., et al. (2013). Safety evaluation and risk assessment of d-limonene. J. Toxicol. Environ. Health, Part B 16, 17–38. doi: 10.1080/10937404.2013.769418
Kim-Campbell, N., Gomez, H., and Bayir, H. (2019). “Cell death pathways: apoptosis and regulated necrosis,” in Critical Care Nephrology (London: Elsevier), 113–121.
Kjær, T. N., Ornstrup, M. J., Poulsen, M. M., Jørgensen, J. O. L., Hougaard, D. M., Cohen, A. S., et al. (2015). Resveratrol reduces the levels of circulating androgen precursors but has no effect on, testosterone, dihydrotestosterone, PSA levels or prostate volume. A 4-month randomised trial in middle-aged men. The Prostate 75, 1255–1263. doi: 10.1002/pros.23006
Ko, J.-H., Sethi, G., Um, J.-Y., Shanmugam, M. K., Arfuso, F., Kumar, A. P., et al. (2017). The role of resveratrol in cancer therapy. Int. J. Mol. Sci. 18:2589. doi: 10.3390/ijms18122589
Kocaadam, B., and Sanlier, N. (2017). Curcumin, an active component of turmeric (Curcuma longa), and its effects on health. Crit. Rev. Food Sci. Nutr. 57, 2889–2895. doi: 10.1080/10408398.2015.1077195
Liu, H.-T., and Ho, Y.-S. (2018). Anticancer effect of curcumin on breast cancer and stem cells. Food Sci. Human Well. 7, 134–137. doi: 10.1016/j.fshw.2018.06.001
Lu, X.-G., Zhan, L.-B., Feng, B.-A., Qu, M.-Y., Yu, L.-H., and Xie, J.-H. (2004). Inhibition of growth and metastasis of human gastric cancer implanted in nude mice by d-limonene. World J. Gastroenterol. WJG 10, 2140–2144. doi: 10.3748/wjg.v10.i14.2140
Maheshwari, R. K., Singh, A. K., Gaddipati, J., and Srimal, R. C. (2006). Multiple biological activities of curcumin: a short review. Life Sci. 78, 2081–2087. doi: 10.1016/j.lfs.2005.12.007
Maltzman, T. H., Hurt, L. M., Elson, C. E., Tanner, M. A., and Gould, M. N. (1989). The prevention of nitrosomethylurea-induced mammary tumors by d-limonene and orange oil. Carcinogenesis 10, 781–783. doi: 10.1093/carcin/10.4.781
Mcinnes, M. D., Moher, D., Thombs, B. D., Mcgrath, T. A., Bossuyt, P. M., Clifford, T., et al. (2018). Preferred reporting items for a systematic review and meta-analysis of diagnostic test accuracy studies: the PRISMA-DTA statement. JAMA 319, 388–396. doi: 10.1001/jama.2017.19163
Mein, J. R., Lian, F., and Wang, X.-D. (2008). Biological activity of lycopene metabolites: implications for cancer prevention. Nutr. Rev. 66, 667–683. doi: 10.1111/j.1753-4887.2008.00120.x
National Cancer Institute (2015a). Risk Factors for Cancer [Online]. Available online at: https://www.cancer.gov/about-cancer/causes-prevention/risk (accessed May 06, 2020).
National Cancer Institute (2015b). What is Cancer? [Online]. Available online at: https://www.cancer.gov/about-cancer/understanding/what-is-cancer (accessed April 16, 2020).
National Cancer Institute (2018). Cancer Statistics [Online]. Available: https://www.cancer.gov/about-cancer/understanding/statistics (accessed April 16, 2020).
National Cancer Institute NCI Dictionary of Cancer Terms [Online], . Available online at: https://www.cancer.gov/publications/dictionaries/cancer-terms/def/latency-period#:~:text=latency%20period%20(LAY%2Dten%2D,a%20virus)%20and%20having%20symptoms (accessed June 15 2020).
National Cancer Institute Cancer Incidence Rates [Online], . Available online at: https://seer.cancer.gov/statistics/types/incidence.html#:~:text=A%20cancer%20incidence%20rate%20is,New%20cancers%20%2F%20Population%20%C3%97%20100%2C000 (accessed June 15 2020).
Nguyen, A. V., Martinez, M., Stamos, M. J., Moyer, M. P., Planutis, K., Hope, C., et al. (2009). Results of a phase I pilot clinical trial examining the effect of plant-derived resveratrol and grape powder on Wnt pathway target gene expression in colonic mucosa and colon cancer. Cancer Manag. Res. 1:25. doi: 10.2147/CMAR.S4544
Paller, C. J., Rudek, M. A., Zhou, X. C., Wagner, W. D., Hudson, T. S., Anders, N., et al. (2015). A phase I study of muscadine grape skin extract in men with biochemically recurrent prostate cancer: safety, tolerability, and dose determination. Prostate 75, 1518–1525. doi: 10.1002/pros.23024
Patel, K. R., Brown, V. A., Jones, D. J., Britton, R. G., Hemingway, D., Miller, A. S., et al. (2010). Clinical pharmacology of resveratrol and its metabolites in colorectal cancer patients. Cancer Res. 70, 7392–7399. doi: 10.1158/0008-5472.CAN-10-2027
Popat, R., Plesner, T., Davies, F., Cook, G., Cook, M., Elliott, P., et al. (2013). A phase 2 study of SRT 501 (resveratrol) with bortezomib for patients with relapsed and or refractory multiple myeloma. Br. J. Haematol. 160, 714–717. doi: 10.1111/bjh.12154
Potten, C. S. (1996). What is an apoptotic index measuring? a commentary. Br. J. Cancer 74, 1743–1748. doi: 10.1038/bjc.1996.624
Rauf, A., Imran, M., Butt, M. S., Nadeem, M., Peters, D. G., and Mubarak, M. S. (2018). Resveratrol as an anti-cancer agent: a review. Crit. Rev. Food Sci. Nutr. 58, 1428–1447. doi: 10.1080/10408398.2016.1263597
Ravindranath, V., and Chandrasekhara, N. (1980). Absorption and tissue distribution of curcumin in rats. Toxicology 16, 259–265. doi: 10.1016/0300-483X(80)90122-5
Rosenblum, D., Joshi, N., Tao, W., Karp, J. M., and Peer, D. (2018). Progress and challenges towards targeted delivery of cancer therapeutics. Nat. Commun. 9, 1–12. doi: 10.1038/s41467-018-03705-y
Ruby, A. J., Kuttan, G., Babu, K. D., Rajasekharan, K., and Kuttan, R. (1995). Anti-tumour and antioxidant activity of natural curcuminoids. Cancer Lett. 94, 79–83. doi: 10.1016/0304-3835(95)03827-J
Shanmugam, M. K., Rane, G., Kanchi, M. M., Arfuso, F., Chinnathambi, A., Zayed, M., et al. (2015). The multifaceted role of curcumin in cancer prevention and treatment. Molecules 20, 2728–2769. doi: 10.3390/molecules20022728
Shi, L., Hopfer, H., Ziegler, G. R., and Kong, L. (2019). Starch-menthol inclusion complex: structure and release kinetics. Food Hydrocoll. 97:105183. doi: 10.1016/j.foodhyd.2019.105183
Stahl, W., and Sies, H. (2003). Antioxidant activity of carotenoids. Mol. Aspects Med. 24, 345–351. doi: 10.1016/S0098-2997(03)00030-X
Stoner, G. D. (2020). Food-based approach to cancer prevention. Food Front. 1:fft2.3. doi: 10.1002/fft2.3
Sun, J. (2007). D-Limonene: safety and clinical applications. Alternat. Med. Rev. 12:259. Available online at: http://mail.encognitive.com/files/D-Limonene%20cancer%20treatment.pdf
Tomeh, M. A., Hadianamrei, R., and Zhao, X. (2019). A review of curcumin and its derivatives as anticancer agents. Int. J. Mol. Sci. 20, 1033. doi: 10.3390/ijms,20051033
U.S. Food and Drug Administration (2019). CFR—Code of Federal Regulations Title 21 [Online]. Available online at: https://www.accessdata.fda.gov/scripts/cdrh/cfdocs/cfcfr/CFRSearch.cfm?fr=182.60 (accessed April 27, 2020).
Wattenberg, L. W., and Coccia, J. B. (1991). Inhibition of 4-(methylnitrosamino)-1-(3-pyridyl)-1-butanone carcinogenesis in mice by D-limonene and citrus fruit oils. Carcinogenesis 12, 115–117. doi: 10.1093/carcin/12.1.115
Wattenberg, L. W., Sparnins, V. L., and Barany, G. (1989). Inhibition of N-nitrosodiethylamine carcinogenesis in mice by naturally occurring organosulfur compounds and monoterpenes. Cancer Res. 49, 2689–2692.
Wei, D., Tang, K., Wang, Q., Estill, J., Yao, L., Wang, X., et al. (2016). The use of GRADE approach in systematic reviews of animal studies. J. Evid. Med. 9, 98–104. doi: 10.1111/jebm.12198
Witschi, H. (2000). Successful and not so successful chemoprevention of tobacco smoke-induced lung tumors. Exp. Lung Res. 26, 743–755. doi: 10.1080/01902140150216792
Witschi, H., Uyeminami, D., Moran, D., and Espiritu, I. (2000). Chemoprevention of tobacco-smoke lung carcinogenesis in mice after cessation of smoke exposure. Carcinogenesis 21, 977–982. doi: 10.1093/carcin/21.5.977
Yaping, Z., Suping, Q., Wenli, Y., Zheng, X., Hong, S., Side, Y., et al. (2002). Antioxidant activity of lycopene extracted from tomato paste towards trichloromethyl peroxyl radical CCl3O2·. Food Chem. 77, 209–212. doi: 10.1016/S0308-8146(01)00339-9
Yu, X., Lin, H., Wang, Y., Lv, W., Zhang, S., Qian, Y., et al. (2018). D-limonene exhibits antitumor activity by inducing autophagy and apoptosis in lung cancer. Onco. Targets. Ther. 11, 1833–1847. doi: 10.2147/OTT.S155716
Zhang, L.-X., Cooney, R. V., and Bertram, J. S. (1991). Carotenoids enhance gap junctional communication and inhibit lipid peroxidation in C3H/10T1/2 cells: relationship to their cancer chemopreventive action. Carcinogenesis 12, 2109–2114. doi: 10.1093/carcin/12.11.2109
Keywords: limonene, cancer, rodent, tumor, apoptosis, redox enzyme
Citation: Zhou J, Azrad M and Kong L (2021) Effect of Limonene on Cancer Development in Rodent Models: A Systematic Review. Front. Sustain. Food Syst. 5:725077. doi: 10.3389/fsufs.2021.725077
Received: 14 June 2021; Accepted: 01 October 2021;
Published: 01 November 2021.
Edited by:
Kathleen L. Hefferon, Cornell University, United StatesReviewed by:
Anshoo Malhotra, Post Graduate Institute of Medical Education and Research (PGIMER), IndiaGaurisankar Sa, Bose Institute, India
Copyright © 2021 Zhou, Azrad and Kong. This is an open-access article distributed under the terms of the Creative Commons Attribution License (CC BY). The use, distribution or reproduction in other forums is permitted, provided the original author(s) and the copyright owner(s) are credited and that the original publication in this journal is cited, in accordance with accepted academic practice. No use, distribution or reproduction is permitted which does not comply with these terms.
*Correspondence: Lingyan Kong, bGtvbmdAY2hlcy51YS5lZHU=