- 1Department of Soil Science, Bangladesh Agricultural University, Mymensingh, Bangladesh
- 2Department of Soil Science, Khulna Agricultural University, Khulna, Bangladesh
- 3Natural Resource Management Division, Bangladesh Agriculture Research Council, Dhaka, Bangladesh
- 4Soil and Water Management and Crop Nutrition, Joint FAO/IAEA Division of Nuclear Techniques in Food and Agriculture, Vienna, Austria
- 5Institute of Plant Ecology (IFZ), Justus-Liebig University Giessen, Giessen, Germany
- 6School of Biology and Environmental Science and Earth Institute, University College Dublin, Dublin, Ireland
- 7Liebig Centre for Agroecology and Climate Impact Research, Justus Liebig University Giessen, Giessen, Germany
- 8Centre for Sustainable Farming Systems, Food Futures Institute, Murdoch University, Murdoch, WA, Australia
Ammonia (NH3) emission from rice fields is a dominant nitrogen (N) loss pathway causing negative impacts on farm profitability and the environment. Reducing N fertilizer application to compensate for N inputs in organic amendments was evaluated for effects on N loss via volatilization, rice yields and post-harvest soil properties in an annual irrigated rice (Boro) – pre-monsoon rice (Aus) – monsoon (Aman) rice sequence. That experiment was conducted using the integrated plant nutrition system (IPNS; nutrient contents in organic amendments were subtracted from the full recommended fertilizer dose i.e., RD of chemical fertilizers) where six treatments with four replications were applied in each season: (T1) no fertilizer (control), (T2) RD, (T3) poultry manure biochar (3 t ha−1; pyrolyzed at 450°C) + decreased dose of recommended fertilizer (DRD), (T4) rice husk ash (3 t ha−1) + DRD, (T5) compost (3 t ha−1) + DRD, and (T6) compost (1.5 t ha−1)+ biochar (1.5 t ha−1) + DRD. The N loss via volatilization varied twofold among seasons being 16% in irrigated rice and 29% in the pre-monsoon rice crop. In irrigated rice, T6 had significantly lower NH3 emissions than all other treatments, except the control while in pre-monsoon and monsoon seasons, T6 and T3 were alike. Pooling the three seasons together, biochar (T3) or biochar plus compost (T6) reduced NH3 loss via volatilization by 36-37% while compost alone (T5) reduced NH3 loss by 23% relative to RD. Biochar (T3) and biochar plus compost mixture (T6) reduced yield-scaled NH3 emissions by 40 and 47% relative to the RD of chemical fertilizer (T2). The organic amendments with IPNS reduced the quantity of N fertilizer application by 65, 7, 24, and 45% in T3, T4, T5, and T6 treatments, respectively, while rice yields and soil chemical properties in all seasons were similar to the RD. This study suggests that incorporation of biochar alone or co-applied with compost and decrease of N fertilizer on an IPNS basis in rice-based cropping systems can reduce N application rates and NH3 emissions without harming yield or soil quality.
1. Introduction
More than 90% of rice (Oryza sativa) in the world is grown in Asia, feeding more than 60% of the global population and it supports the livelihood of millions of small and marginal farm families in south Asia (Brolley, 2015). Bangladesh is the third largest rice producing country in the world where rice contributes about 4.5% to the country's gross domestic product. In this country, rice is grown in three seasons i.e., irrigated winter rice called Boro, pre-monsoon rice called Aus and monsoon rice called Aman. In 2020–21, gross annual production of 3-seasons' rice was 36.61 Mt (BBS, 2021). Despite large quantities of nitrogenous fertilizer being applied to maintain rice yields, there is low use efficiency (30–35%) of this fertilizer, and significant gaseous nitrogen (N) loss (Xia and Yan, 2012). In Bangladesh, N is applied at around 150 kg N ha−1 season−1, which is almost double the rate of Japan (80 kg N ha−1 season−1) and a little higher than in the United States (140 kg N ha−1 season−1) (Linquist et al., 2015; Xia et al., 2016). Ammonia (NH3) is one of the most important by-products of applied N in rice field and volatilization of NH3 is the primary source of soil nitrogen loss (Pan et al., 2016; Xu et al., 2019; Kuttippurath et al., 2020; Wang et al., 2021).
In 2021, Bangladesh ranked first globally in air pollution due to elevated concentrations of CH4 and NH3 in the air (IQAir, 2021). Even though NH3 is not a potential greenhouse gas (GHS), its emissions and re-deposition can have negative impacts on the environment (Zhang et al., 2020). Volatilized NH3 is a secondary source of N2O and NO (Mosier et al., 1998), and NH3 volatilization is responsible for around 30% of N deposition (Wolfe and Patz, 2002). Ammonia has a negative impact on regional air quality and human health generating aerosols in the atmosphere, influencing the radiation balance by scattering light and changing the earth's reflectivity (Xu and Penner, 2012; Stokstad, 2014). In Asia, agricultural gaseous N losses including NH3 volatilization may reach 18.8 Tg N yr−1 in 2030 (Zheng et al., 2002; Liu et al., 2021). The global estimate of NH3 emissions from urea-fertilized soils ranges from 10 to 20%, although in warmer zones, it is substantially higher (Cantarella et al., 2018). Because of extensive rice cultivation, the Indo Gangetic Plain has been identified as a hotspot for NH3 fluxes but estimates of the rates of loss are limited (Kuttippurath et al., 2020; Uddin et al., 2021; Jahangir et al., 2022).
Mitigating NH3 emissions from agriculture will not only cut the cost of fertilizer N, but it will also improve air and water quality (Zhao et al., 2017). To limit N losses, various practices are proposed such as use of nitrification inhibitors, urease inhibitors (UI), elemental S, and polymers (He et al., 2018), crop residue removal management (Battaglia et al., 2018, 2021), and organic amendments (Saarnio et al., 2013; Malińska et al., 2014). The role of organic amendments like poultry manure, biochar, compost, etc. in mitigating NH3 fluxes from wetland rice fields is unresolved since some researchers have reported positive effects (Saarnio et al., 2013; Malińska et al., 2014; Ali et al., 2019), while others reported negative effects (Feng et al., 2017; Chu et al., 2019; Rahaman et al., 2020). Ammonia emissions increase with the N fertilizer rate (Uddin et al., 2021; Jahangir et al., 2022) which suggests that with organic amendments the rate of N fertilizer application could be decreased to reduce both economic and environmental costs while maintaining soil quality. Biochar is a carbon-rich substance made from the pyrolysis of organic matter (Lehmann and Joseph, 2009). It has been reported to prevent NH3 loss and improve soil health, crop output, and soil carbon sequestration, while also recycling organic waste (Diatta et al., 2020). Biochar and compost mixture can be utilized as fertilizer sources to increase soil nutrients and reduce nutrient losses (Banik et al., 2021).
Ammonia emissions are estimated by the IPCC Tier 1 method but only a single emission factor is scheduled (Bouwman, 1996). While a large amount of N loss as NH3 can occur, the exact quantity is not known for accurate N balance calculations for many managed agricultural systems including the rice-based cropping patterns of South Asia. Previously, Uddin et al. (2021) evaluated the impact of Conservation Agriculture along with different N fertilization rates on NH3 volatilization in winter rice (Boro rice). They reported that NH3 volatilization accounted for 16–21% of the applied N. However, there is no baseline data of NH3 volatilization in the other two rice growing seasons when temperature is higher (Aus and Aman rice), nor on the impacts of reduced N fertilizer application when co-applied with organic amendments (i.e., integrated plant nutrition system (IPNS) approach) on NH3 volatilization. We hypothesize that co-application of organic fertilizer such as biochar, rice mill ash (RMA) and compost together with inorganic N fertilizers, which together provide the same amount of N as chemical fertilizer alone, would reduce NH3 volatilization loss without changing the soil N status. Thus, the study was conducted to evaluate the effects of rice husk ash, biochar alone or with compost (IPNS basis) on seasonal and annual NH3 emissions, rice yields and soil quality.
2. Materials and methods
2.1. Experimental site description
The study was carried out on the Soil Science Field Laboratory (24° 71.59′ N, 90° 42.50′ E) of Bangladesh Agricultural University (BAU) in Mymensingh, Bangladesh. The experiment was done with an annual irrigated rice– pre-monsoon rice – monsoon rice cropping sequence, which is a common cropping sequence followed by the farmers of this country. The irrigated rice season, pre- monsoon rice season and monsoon rice season were occupied by Boro, Transplanted Aus (T. Aus), and Transplanted Aman (T. Aman) rice growing seasons, respectively. The field site was characterized as a Non-calcareous Dark Gray Floodplain soil (Aeric Haplaquept in US Soil Taxonomy), and belongs to agro-ecological zone-9, Old Brahmaputra Floodplain soil (FAO/UNDP, 1988). The soil is moderately drained with a silt loam texture and near neutral pH (6.5). The region has a sub-tropical monsoon climate with a mean annual temperature of 26°C, average annual rainfall of 1,800 mm, and relative humidity of 85% (Uddin et al., 2021, Supplementary Data 1).
2.2. Experimental design and crop management
The experiment was conducted with the same treatment combinations for Boro – T. Aus – T. Aman rice crops in sequence, but with different levels of a nutrient based on the requirements of individual crop and their target yields. That experiment was conducted under integrated plant nutrition system (IPNS; nutrient contents in organic amendments were subtracted from the full recommended fertilizer dose, i.e., RD of chemical fertilizers) where six treatments with four replications were applied in each season. The treatments were: (T1) no fertilizer (control), (T2) RD, (T3) poultry manure biochar + decreased dose of recommended fertilizer (DRD), (T4) rice husk ash + DRD, (T5) compost + DRD, and (T6) compost + biochar + DRD, laid out in a randomized complete block design (RCBD) with four replications. Total plot number was twenty-four for each season and the same plots were used for consecutive rice growing seasons and the unit plot size was 5 m × 4 m, with a 0.75 m inter-plot space, and 1 m inter-block space. The varieties were BRRI dhan28 for Boro, BINA dhan19 for T. Aus and BRRI dhan49 for T. Aman rice, respectively. Boro rice was grown during January–April (winter season), followed by T. Aus rice as a rainfed crop from May to August (pre-monsoon), and then T. Aman rice from August to November (monsoon).
The rate of chemical fertilizer application was based on Fertilizer Recommendation Guide (FRG, 2018) for the test crops. The nutrient contents of used organic amendments are presented in Table 1 while the recommended doses of nutrients for three seasons were presented in Table 2. Urea, triple super phosphate, muriate of potash, gypsum and zinc sulfate were used for N, phosphorus (P), potassium (K), sulfur (S) and zinc (Zn) sources, respectively. Except urea all the nutrients were applied during land preparation. For both Boro and T. Aman rice nitrogenous fertilizer (urea) was applied in equal three splits, followed interval for Boro rice was at 10, 31, and 53 Days After Transplanting (DAT) and for T. Aman that interval was at 9, 24, and 39 DAT. In T. Aus rice two splits of urea application were followed, at 11 and 29 DAT. Compost was collected from Mazim Agro Industries Ltd and rice husk ash from a local rice mill. Biochar was produced using poultry manure by an anaerobic pyrolysis process at 450°C for 4 hr. The organic materials were air dried to 15% moisture content, pulverized and sieved with a 2 mm mesh. In T3, T4, and T5 treatments organic materials were applied at the rate of 3 t ha−1 where T6 was balanced by applying 1.5 t ha−1 compost and 1.5 t ha−1 biochar, the remaining nutrients were applied from chemical fertilizer based on FRG under IPNS approach. Glyphosate (Round up®) was sprayed over the field at a rate of 1.85 kg ha−1 3 days before final land preparation. The field was irrigated to maintain 3 cm standing water throughout rice growing seasons.

Table 1. Chemical properties of organic amendments (poultry manure biochar, cattle compost, rice husk ash) used in three rice growing seasons.
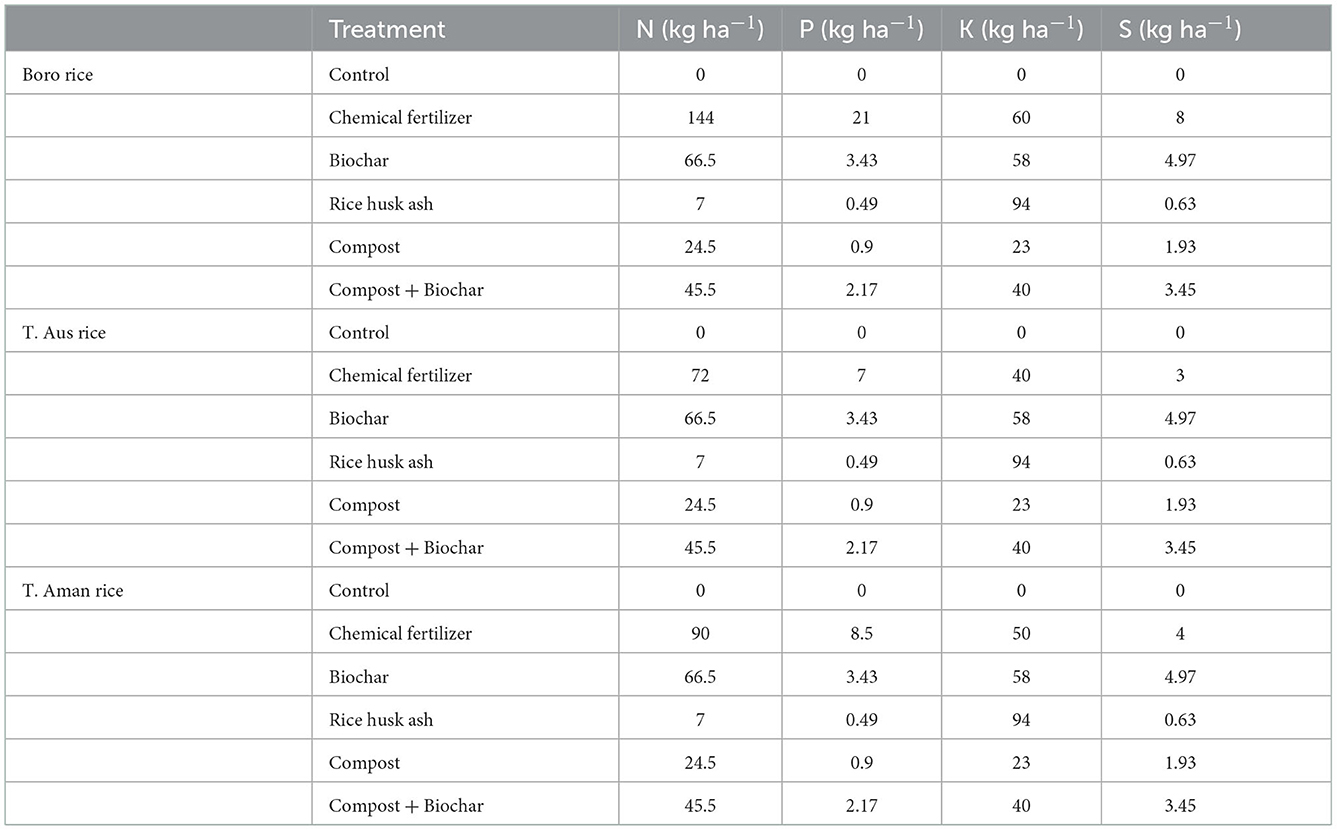
Table 2. Amounts of nutrients added from each source of organic amendments used in three rice growing seasons.
2.3. NH3 gas sampling and analysis
Field measurements of NH3 were conducted during January 2020–November 2021 in the rice field. A low-cost chamber was deployed in field conditions for NH3 volatilization measurements (Nichols et al., 2018) and used for monitoring NH3 fluxes in crop fields (Martins et al., 2021a,b; Zaman et al., 2021). The open chamber method was used to measure NH3 fluxes in the field site on a daily basis. In the laboratory, the amount of NH3 trapped in acid solution was estimated using the Kjeldahl principle (Keeney and Nelson, 1982). Measurements were done on the soil shortly after urea application and it was carried out until the fluxes were below the detection limit in each case.
2.4. NH3 fluxes and emission factor calculation
The NH3 fluxes were calculated following Equation 1.
Where, NH3 flux was measured as mg N m−2 d−1; FBR, Final Burette Reading (ml); IBR, Initial Burette Reading (ml); molecular weight of N = 14.01 g; normality of H2SO4 = 0.01 N; and 1000 = unit conversion factor. The sum of NH3 fluxes on sampling days across the whole sampling period was used to estimate cumulative NH3 fluxes.
We derived EF (%) according to Equation 2 (Mazzetto et al., 2020).
Where EF (%) = Emission Factor, in%; Fluxes FT, Fluxes from fertilizer treatment (in kg N ha−1); Fluxes C, Fluxes from control treatment (in kg N ha−1); Applied Fert, Amount of fertilizer applied (in kg N ha−1).
Yield-scaled NH3 fluxes were determined following the Equation 3.
2.5. Measurement of grain yield
Before the final harvest of each rice growing season, plants from 1 m2 area were collected from each plot, weighed and then oven dried to determine yield and system productivity i.e., pooling together the grain yields of the three rice seasons. For oven drying, 1000 grain samples of each plot were placed in an oven at 65°C until it reached constant weight to determine moisture content. After drying, rice grain samples were weighed and yields were estimated as tonne per hectare.
2.6. Soil sample collection and laboratory analysis
Composite soil samples were collected with an auger at 0–15 cm soil depth from the sites next to each NH3 gas sampling chamber and preserved in sealable plastic bags in a cooler box. The field-moist soil was air-dried for 2 weeks in the shade at room temperature (25°C) and processed (2 mm sieved) for analysis of major soil physico-chemical parameters. During the NH3 loss measurement, the pH of the soil was monitored in the field every seven days using a portable pH meter (HI12923; Hanna Instruments). The Kjeldahl method was used to determine total nitrogen (TN) content in the soil (Fawcett, 1954) and the wet oxidation method (Walkley and Black, 1934) was used for soil organic carbon (SOC) determination. Soil samples were extracted with 2 M KCl (1: 2.5; w/w) and and contents were measured using the method described by Keeney and Nelson (1982).
2.7. Statistical analysis
One-way ANOVA was performed using treatments as fixed factors. The normality test on the NH3 data was checked before analysis. Post-hoc tests were performed to separate differences among the treatments using the Tukey-Kramer multiple comparison Test. All statistical analyses were considered significant at p ≤ 0.05, unless otherwise mentioned. All the statistical analyses were performed on Statistics 10 and Jamovi1.0.0.0 (R Package). Correlation among the parameters studied was tested by using Pearson's correlation coefficient comparison test.
3. Results
3.1. Time course of NH3 fluxes after urea application
Ammonia fluxes reached their peaks at 2–3 days after each split of urea application in all seasons. The highest NH3 fluxes were recorded during the second split application of urea in boro season but that was higher from first split application in both T. Aus and T. Aman seasons (Figure 1). In T. Aman rice, the NH3 fluxes were 1.5–2.0 times higher in the first split compared to the second and third splits, while the latter two results were almost the same. The NH3 flux peaks returned to background level at 7–10 days after each split urea application (Figure 1). The highest peak in all splits at each season ranked in the order of chemical fertilizer > RMA > compost > compost + biochar > biochar > control (Figure 1B). On the peak period at 1st, 2nd, and 3rd splits of urea fertilization the NH3 fluxes from RD treatment were 117, 305, 160 mg N m−2d−1 in Boro season and that were 193, 165 mg N m−2d−1 in T. Aus and 289, 138 and 136 mg N m−2d−1 in T. Aman season, respectively.
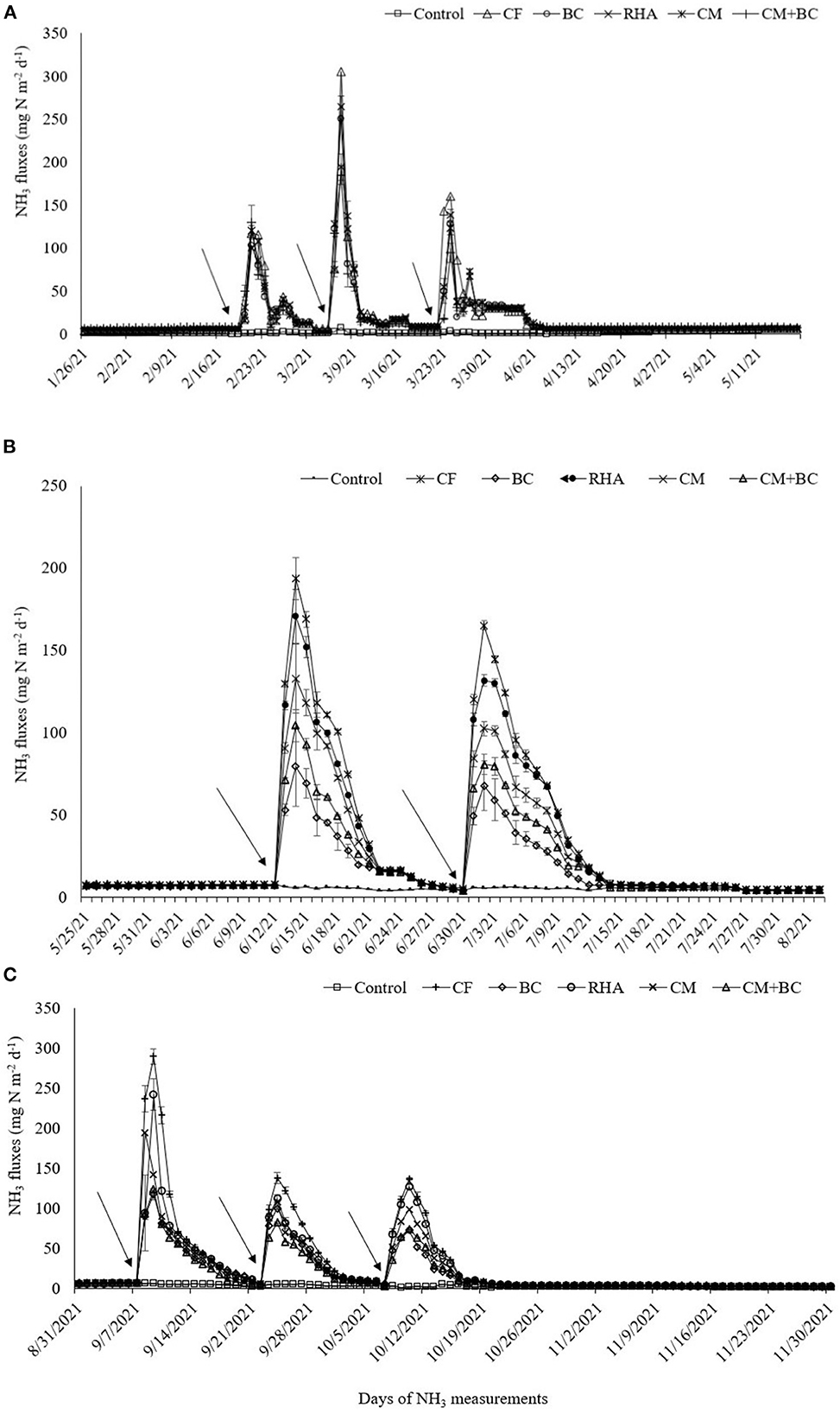
Figure 1. Daily NH3 fluxes (mean ± SE; R = 4) from different plots treated with organic and inorganic fertilizers throughout the year [(A) Boro, (B) T. Aus, (C) T. Aman]. Arrows indicate the day of split urea application.
3.2. Effects on mean and cumulative ammonia fluxes
The effect of organic and inorganic fertilizers on mean and cumulative ammonia fluxes of all three rice crops was significant. In Boro rice, the highest mean and cumulative NH3 fluxes were observed in chemical fertilizer treated plot, which was statistically similar to RMA, and the lowest emission was observed in control (Table 3). Integrated use of organic and inorganic fertilizers reduced NH3 emissions by 6–23% compared to the RD treatment. Either biochar or biochar plus compost reduced N loss via volatilization by 16–23%, while compost alone reduced it by 13%. Likewise, organic and inorganic fertilization also significantly influenced mean and cumulative NH3 fluxes in T. Aus rice. Mean and cumulative NH3 fluxes were higher in RD than in other treatments. Reduction in NH3 fluxes ranged from 10% in rice husk ash to 52% in biochar. Disregarding the control, the highest mean and cumulative NH3 fluxes were measured in chemical fertilizer treated plots, whereas the lowest emissions were measured in compost plus biochar treated plots. Combined application of organic and inorganic fertilizers reduced NH3 volatilization by 20–45% compared to the RD application. Pooling the three rice growing seasons together, treatments comprised of biochar or biochar plus compost under IPNS basis reduced N loss via volatilization by 36–37% while biochar alone reduced it by 23% over sole application of full dose of recommended fertilizer as a treatment.
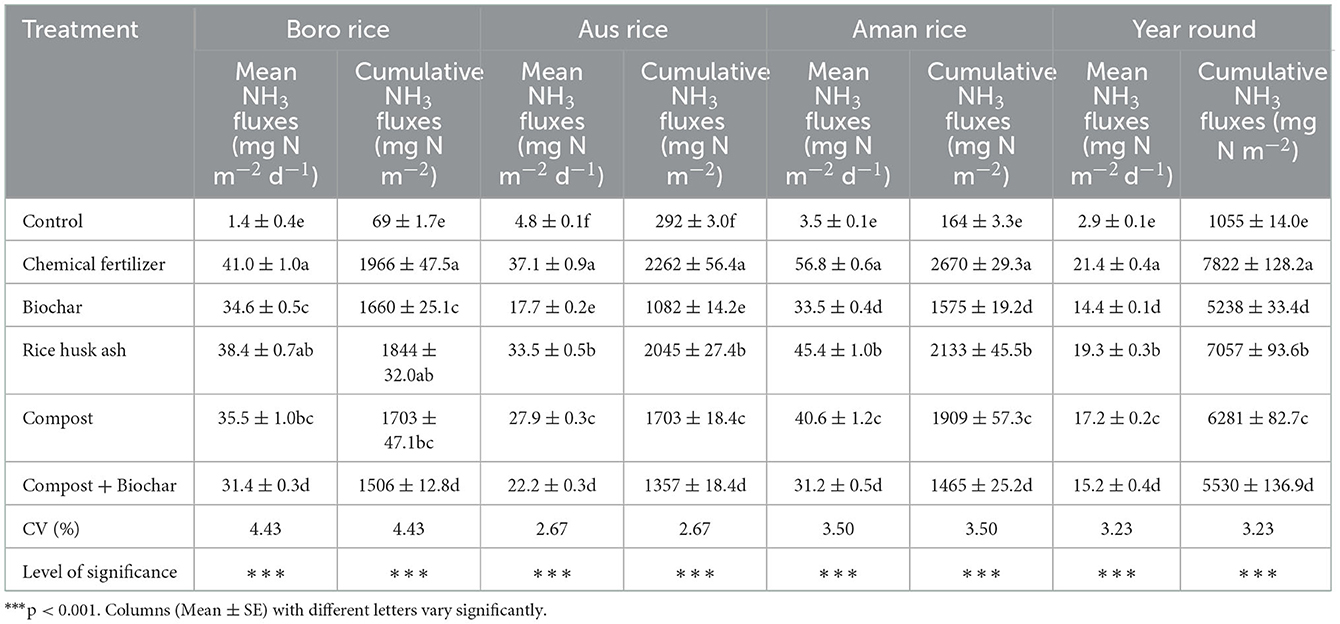
Table 3. Effects of organic and inorganic fertilizers on mean and cumulative ammonia fluxes in the Boro - T. Aus - T. Aman rice cropping pattern.
3.3. Effects on NH3 emission factor and yield scaled NH3 emissions
The NH3 emission factor (EF) was significantly influenced by the application of organic and inorganic fertilizers. The NH3 EF ranged from 12% in compost + biochar to 16% in chemical fertilizer-treated plots in Boro rice, from 21% in biochar to 29% in sole chemical fertilizer treated plots in T. Aus rice, and from 22% in biochar to 28% in chemical fertilizer-treated plots in T. Aman rice (Table 4). Yield-scaled NH3 emissions in Boro rice varied from 0.17 kg t−1 in control to 2.88 kg t−1 in chemical fertilizer treated plots (Table 4). Except for the control treatment, yield scaled NH3 emissions were similar among treatments in Boro rice. Mixture of biochar and compost reduced the NH3 EF and yield-scaled NH3 emission in all rice fields. Similarly, yield-scaled NH3 emissions in T. Aus rice varied between 1.04 kg t−1 in control and 5.83 kg t−1 in chemical fertilizer treated plots (Table 4). Ignoring the control treatment, the highest yield-scaled NH3 emissions were noted in chemical fertilizer, which was similar to RMA, and the lowest value was in biochar with or without compost treatment. Yield-scaled NH3 emissions in T. Aus rice were 1 to 6 times higher than that in Boro rice. Similarly, yield-scaled NH3 emissions in T. Aman rice ranged from 0.47 kg t−1 in control to 4.43 kg t−1 in chemical fertilizer-treated plots (Table 4). Discounting the control treatment, the highest yield-scaled NH3 emission was recorded in chemical fertilizer, and the lowest value was in biochar with or without compost. Yield-scaled NH3 emissions in T. Aman rice were 1.0 to 2.7 times higher than that in Boro rice, and 0.5 to 1.0 times that of T. Aman rice (Table 4).
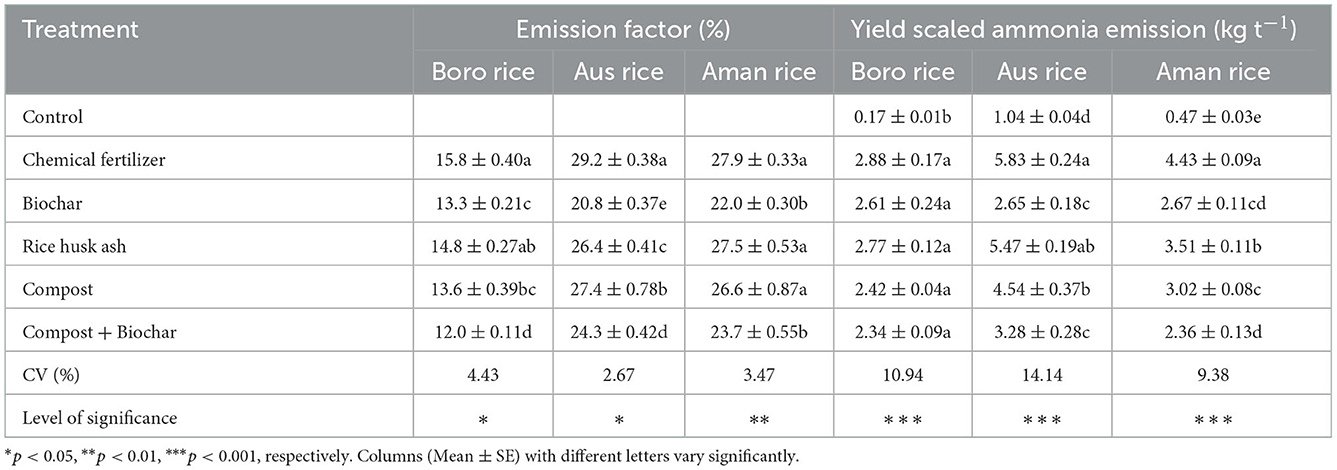
Table 4. Effects of organic and inorganic fertilizers on emission factor and yield-scaled ammonia emissions in rice crops.
3.4. Effects on crop yields and system productivity
Organic and inorganic fertilizers influenced the grain yield of Boro, T. Aus, and T. Aman rice (p < 0.05, Table 5), and system productivity of Boro – T. Aus – T. Aman rice cropping pattern (p < 0.01, Table 5). All the treatments were similar to each other in term of crop yield except T1. Treatments under RD or IPNS had no statistical variation for crop yield and system production. In Boro and T. Aman rice, grain yields were the highest for application of compost and likely the system productivity was the highest for compost application and the lowest for rice husk ash, excluding the control treatment.
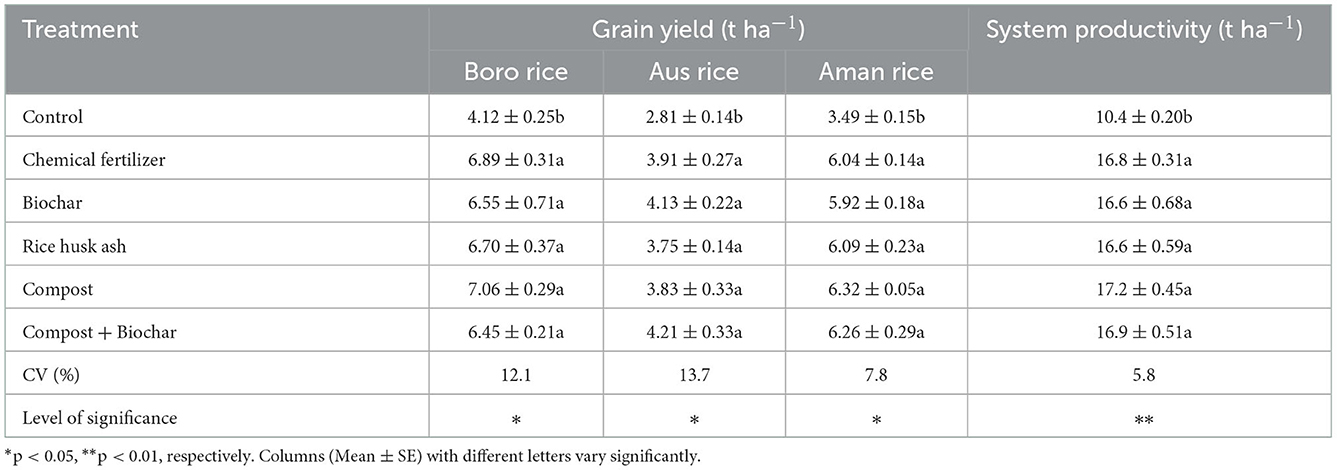
Table 5. Effects of organic and inorganic fertilizers on the grain yield of crops and system productivity in the Boro - T. Aus - T. Aman cropping pattern.
3.5. Effects on soil properties
Organic and inorganic fertilizers had a significant impact on soil organic carbon (SOC) during rice cultivation (p < 0.05, Table 6). Soil organic carbon increased by 6–14% over the control in plots treated with different amendments (Table 6). Biochar and RMA significantly increased soil total nitrogen (TN) content compared to the other treatments including control except in T. Aus season (Table 6). Likewise, organic and inorganic fertilizers significantly influenced soil C:N ratio only in Boro season but not in T. Aus and T. Aman seasons (p < 0.05, Table 6). The highest soil pH was measured in biochar-treated plots, which was similar to other treatments except for compost with biochar and control. Likewise, concentrations in soil were significantly influenced by different organic and inorganic fertilizers (p < 0.001, Table 6). The highest concentrations were found in plots treated with only chemical fertilizer and were lowest in control.
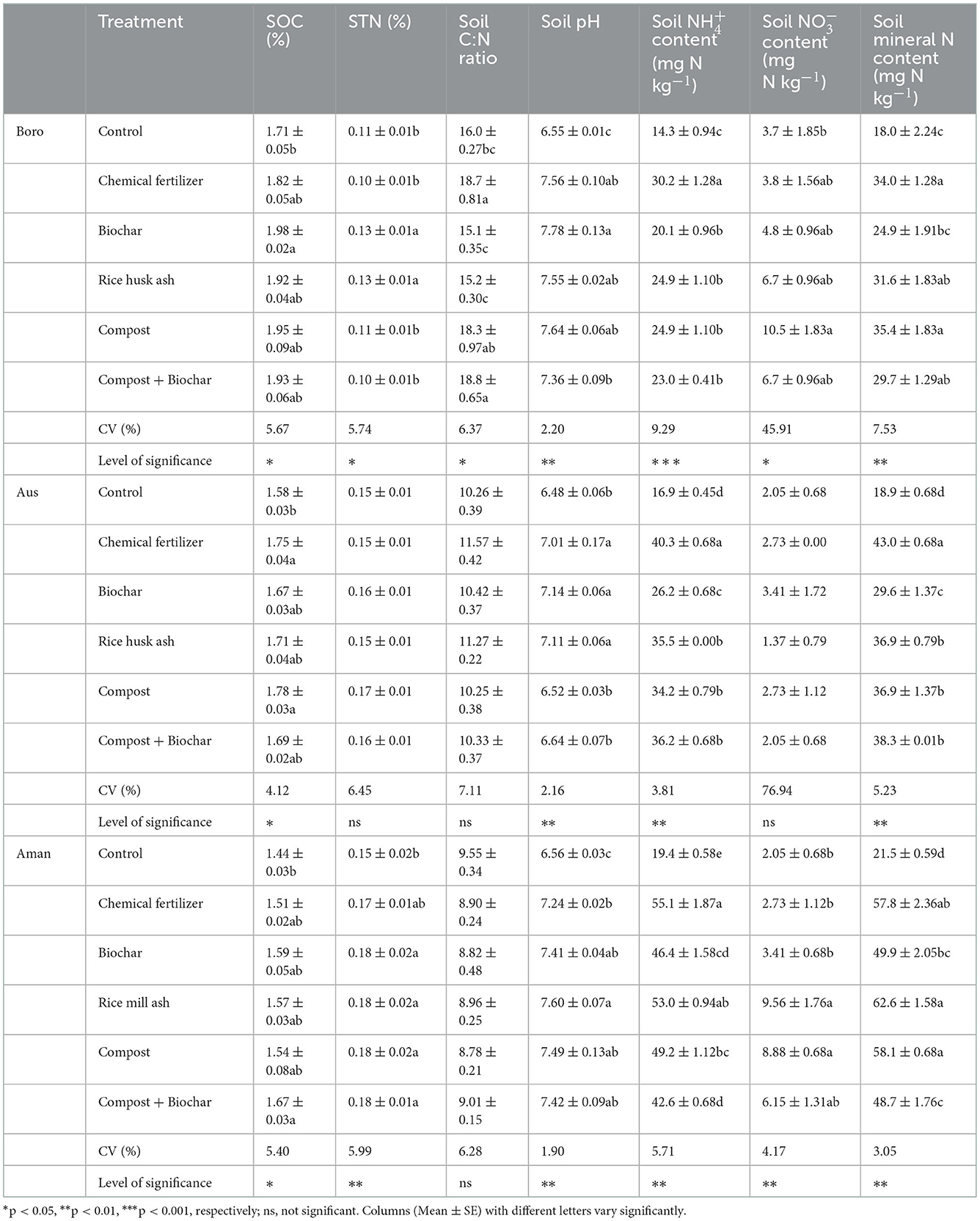
Table 6. Effects of organic and inorganic fertilizer on soil properties after urea application in Boro, T. Aus and T. Aman rice.
The relationship between the NH3 fluxes and soil pH was positive and significant in all rice seasons (Figure 2). NH3 fluxes had a strong correlation with soil pH in Boro rice (R2 = 0.79; p < 0.01). Likewise, NH3 fluxes had a moderate correlation with soil pH in T. Aus rice (R2 = 0.36; p < 0.05) and in T. Aman rice (R2 = 0.50; p < 0.05) (Figure 2). Like soil pH, the relationship between NH3 fluxes and soil content was also positive and significant in all rice seasons (Figure 2). Ammonia fluxes had a strong correlation with soil content in Boro rice (R2 = 0.68; p < 0.01), T. Aus rice (R2 = 0.86; p < 0.001), and T. Aman rice (R2 = 0.91; p < 0.001) (Figure 2).
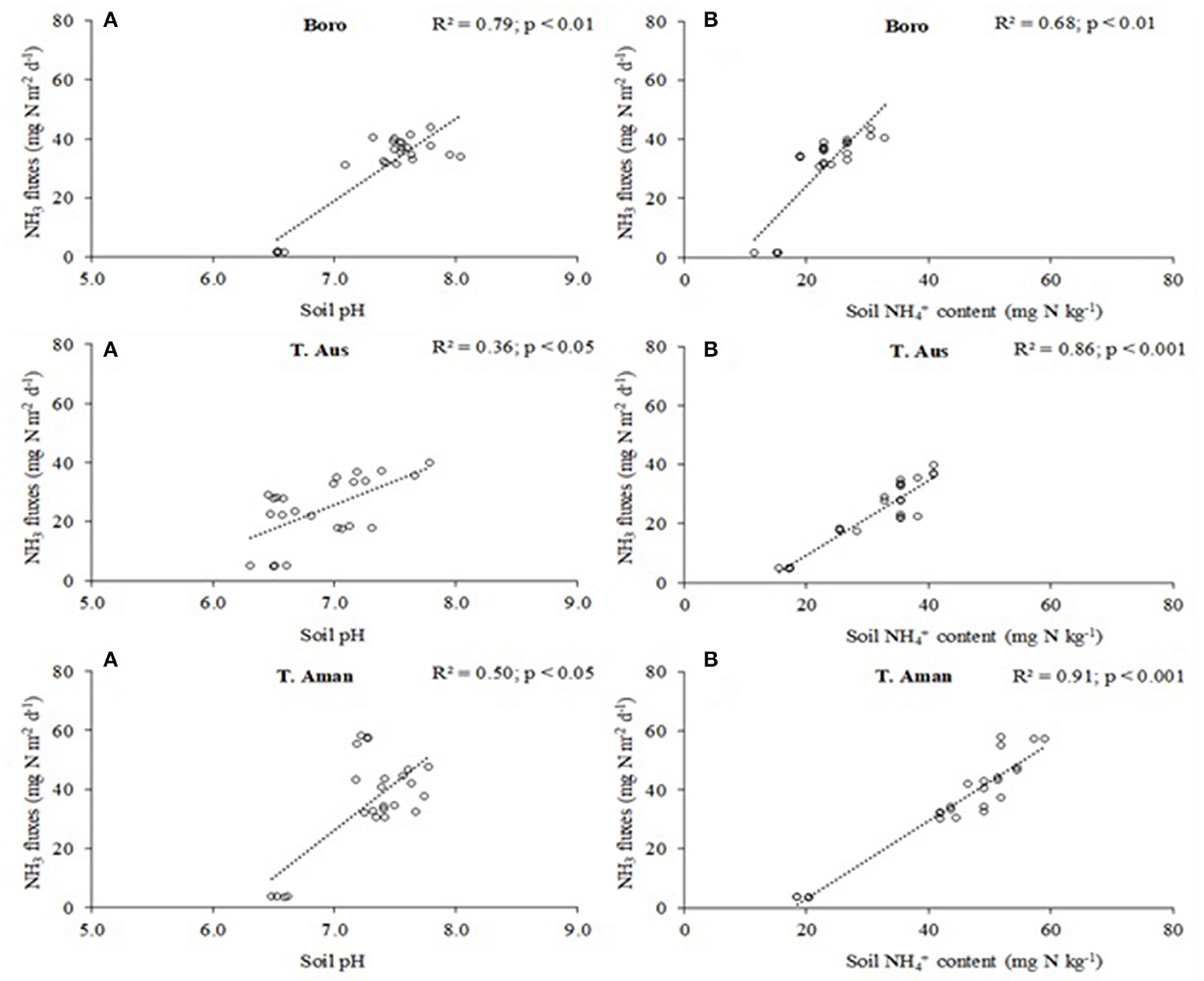
Figure 2. Relationship between NH3 fluxes and soil pH (A) or soil content (B) in the rice crops; n = 24.
4. Discussion
In accord with our hypothesis, the co-application of biochar, RMA and compost together with N fertilizer, while suppling the same amount of N as N fertilizer alone, decreased NH3 volatilization loss by 16–28% without changing the soil N status. In the following discussion, we first examine the dynamics of NH3 fluxes, the NH3 emission factors for treatments and the IPNS treatment co-benefits for soil properties and crop yield.
4.1. Peak of NH3 fluxes
The NH3 flux peak was within 2–3 days after urea application indicating that NH3 volatilization was a rapid progress that was almost completed within 1 week after each split fertilizer application. The NH3 volatilization flux patterns were consistent among treatments, suggesting that they were primarily driven by the urea applied. The NH3 emission patterns were consistent with previous studies in the same (Uddin et al., 2021) and dissimilar geographical areas (Fan et al., 2006) as our experiment. The NH3 flux from urea hydrolysis usually peaks at 3–7 days after application (Rochette et al., 2009) which is in line with our results but not to Drury et al. (2017) who stated that the peak emissions can take up to 9–15 days if rain occurs after N application. That fluxes were highest from T2 may be attributed to the highest rate of urea applied which rapidly converted into through the ammonification process, which was the first step of ammonia volatilization (Frimpong et al., 2016; Uddin et al., 2021). As NH3 is in a dynamic equilibrium with and H+, urea treatment elevates soil pH through urease hydrolysis (Sommer et al., 2004). Following the peak on day 2–4 after urea application, the NH3 fluxes rapidly declined. While organic amendments did not alter the timing of the peak of NH3 fluxes, they decreased the magnitude of the peak which could be attributed to the lower rate of chemical N-fertilizer based on the IPNS approach. The decrease in soil content and a drop in pH with the organic amendments helps explain the decrease in NH3 volatilization (Adviento-Borbe et al., 2010). Other processes leading to a decrease in NH3 volatilization could be the infiltration of mineral N into the crop rooting zone, and increased nitrification over time (Adviento-Borbe et al., 2010). When the NH3 fluxes for Boro, Aus and Aman seasons were examined in relation to the soil chemical properties, the closest positive correlation was with soil pH followed by soil as found in previous studies (Sommer et al., 2004; Rochette et al., 2013).
4.2. Ammonia fluxes, emission factor, and rice yields
While NH3 volatilization is a major N loss from paddy fields, the rate of N loss is dependent on the fertilization type, time of application, environmental conditions and N application rate (Wang et al., 2016). Pan et al. (2016) stated that about 30% of the applied urea was lost through NH3 fluxes which were consistent with our result that the N loss via volatilization ranged from 16% in Boro to 28% in T. Aman rice season. When N supplied in the urea fertilizer was adjusted based on the N content in the organic amendments, NH3 fluxes were reduced. In this study biochar alone and with compost reduced the NH3 loss during three rice growing seasons. The NH3 fluxes of N fertilizer was higher for Aman rice than for Aus and Boro rice which is most likely due to the seasonal variations in temperature being the lowest in Boro season (15–25°C) and the highest in T. Aman season (25–35°C) while in T. Aus the temperature was moderate (20–30°C). High temperature in standing water in rice fields induces rapid urea hydrolysis and higher ammonia volatilization (Sun et al., 2017). While the Aus season in the Indo-Gangetic plain has high rainfall and moderate temperature, the urea application rate in this season was lower than the other two seasons due to lower yield potential, which may lower volatilization.
Biochar was very effective in reducing NH3 emissions by reducing chemical N input but may also control the N releases. Our results also showed consistency with Sun et al. (2017) and Asada et al. (2002), where their meta-analysis suggested that NH3 fluxes were reduced with the application of biochar pyrolyzed at ~400°C. Ammonia adsorbed onto the biochar surface directly reduces the substrate concentration of NH3 for the volatilization process (Clough et al., 2013). However, the liming effect of alkaline biochar may increase NH3 fluxes (Sun et al., 2017; Sha et al., 2019). The pH increase in soil amended with biochar in the present study was not high enough to enhance NH3 fluxes (Kelly et al., 2015). Among the amended plots biochar required the lowest rate of urea fertilizer to equalize total N input with recommended chemical fertilizer dose, which may explain the lower NH3 fluxes than in compost amended plots. Co-application of biochar with compost has the potential to reduce NH3 emissions due to high surface area to adsorb NH4, high internal porosity to trap ions but this will depend on N mineralization rate and their inherent N content which varies among biochar and compost products.
All the treatments, except control without N fertilizer applied, had the same yield in all three rice seasons even though the urea application rates were different. Moreover, the N uptake was also the same in each treatment (data not presented). Therefore, questions arise of how biochar-treated soils provided similar N for plant uptake in comparison to a full dose of urea. A moderate substitution (<40%) of N fertilizer by manure has been reported to significantly increase N use efficiency by 14 and 25% for upland crops and rice, respectively (Xu et al., 2016; Zhang et al., 2020). In the present study, rice plants were initially paler green in biochar-treated plots suggesting that it decreased initial N mineralization rate. In addition, N from urea in biochar-treated plots may have been used more efficiently due to better synchronization of N supply and demand.
Rice husk ash had less efficiency in retention in all seasons and in controlling NH3 fluxes than biochar, but still decreased N losses relative to the urea fertilizer alone. While ashes are often alkaline, the present RMA did not alter soil pH and was effective in decreasing NH4 content in soil except in Aman season and in decreasing NH3 losses, except in the Boro season. As an abundant biowaste in the Indo-Gangetic Plain, RMA can be used to reduce N fertilizer input and to reduce atmospheric NH3 emissions. However, as the ashing conditions are likely to vary with farm-produced RMA, more study is needed to determine the consistency of the effects reported here.
4.3. Integrated plant nutrition system effects on soil properties
In addition to their effects on NH3 losses, organic amendments had significant effects on some soil properties. In the current research, sole biochar application increased soil pH compared to control treatment, however compost + biochar combination and sole compost application decreased soil pH. Poultry manure biochar may have increased soil pH through its liming effect over the sole compost and chemical fertilizers application but when the mixture of compost and biochar was applied, soil pH decreased relative to the sole biochar application. Herein, soils treated with solitary biochar had the highest pH (7.78) which is not enough to raise NH3 loss, followed by soils treated with both biochar and compost (7.42). Slight increase in soil pH in biochar treated plots could have increased NH3 emissions but the lower contents in soils resulted in lower NH3 emissions.
Application of biochar solely or in combination with compost at a rate of 3 t ha−1 has increased SOC in our study, which is in line with previous research (Liu et al., 2021). Biochar is distinguished from compost by its larger proportion of more stable organic carbon molecules (Mahmoud et al., 2018; Eissa, 2019) making it more efficient in enhancing soil physicochemical parameters (Eissa, 2019). Furthermore, Trupiano et al. (2017) also reported that the application of compost and biochar to soils, either alone or in combination, enhanced soil SOC content compared to un-amended soils, implying that biochar and/or compost is a potential source of soil carbon sequestration.
5. Conclusion
Volatilization loss of N from paddy fields in floodplain soils causes economic losses and is a major concern for air and water quality. Application of biochar alone or in combination with compost on an integrated plant nutrition system basis reduced the rate of N-fertilizer application as well as ammonia volatilization. The NH3 emission factor ranged from 12% in compost plus biochar to 16% in chemical fertilizer-treated plots in Boro rice, from 21% in biochar to 29% in compost treated plots in Aus rice, and from 22% in biochar to 28% in chemical fertilizer-treated plots in Aman rice. Pooling the three rice growing seasons together, either biochar or biochar plus compost mixture reduced N volatilization by 36–37% while compost alone can reduce it by 23%. All the treatments had same crop yield except the control without N fertilizer. Hence, biochar with or without compost mixture has a great potential for mitigating year-round NH3 volatilization in the triple rice cropping system along with a decrease in the rate of applied N-fertilizer in floodplain soils without losing crop yield and system productivity.
Data availability statement
The raw data supporting the conclusions of this article will be made available by the authors, without undue reservation.
Author contributions
MJ and MAH worked on research planning and paper editing. CM worked on research planning, calculation, and paper editing. RB worked on planning and paper editing. MZ contributed in research planning and methodological development. MBH worked on research planning. JF conducted field and laboratory work, data processing, analysis, and paper draft preparation. NM worked in draft preparation. MMJ worked on research planning, data interpretation and paper editing. All authors contributed to the article and approved the submitted version.
Funding
The research was funded by a Krishi Gobeshona Foundation (KGF) project administered by Bangladesh Agriculture Research Council (BARC) in association with the Australian Center for International Agricultural Research (ACIAR: Project LWR 2016/136).
Acknowledgments
We acknowledge the technical support of the Soil and Water Management and Crop Nutrition, Joint FAO/IAEA Division of Nuclear Techniques in Food and Agriculture, Vienna, Austria.
Conflict of interest
The authors declare that the research was conducted in the absence of any commercial or financial relationships that could be construed as a potential conflict of interest.
Publisher's note
All claims expressed in this article are solely those of the authors and do not necessarily represent those of their affiliated organizations, or those of the publisher, the editors and the reviewers. Any product that may be evaluated in this article, or claim that may be made by its manufacturer, is not guaranteed or endorsed by the publisher.
Supplementary material
The Supplementary Material for this article can be found online at: https://www.frontiersin.org/articles/10.3389/fsufs.2022.1067112/full#supplementary-material
Supplementary data 1. Environmental weather data of the experimental site registered during the experimental period (January 2021 to December 2021).
References
Adviento-Borbe, M. A. A., Kaye, J. P., Bruns, M. V., Mcdaniel, M., McCoy, M., Harkcom, S., et al. (2010). Soil greenhouse gas and ammonia emissions in long-term maize-based cropping systems. Soil Biol. Biochem. 74, 1623–1634. doi: 10.2136/sssaj2009.0446
Ali, M., Haruna, A. O., Majid, N. M. A., Primus, W. C., Asap, A., Maikol, N., et al. (2019). Mitigating ammonia volatilization from waterlogged acids soils using organic amendments. Sustain. Agric. Res. 8, 12–22. doi: 10.5539/sar.v8n3p12
Asada, T., Ishihara, S., Yamane, T., Toba, A., Yamada, A., Oikawa, K., et al. (2002). Science of bamboo charcoal: study on carbonizing temperature of bamboo charcoal and removal capability of harmful gases. Health Sci. 48, 473–479. doi: 10.1248/jhs.48.473
Banik, C., Koziel, J. A., Bonds, D., Singh, A., and Licht, M. (2021). Comparing biochar-swine manure mixture to conventional manure impact on soil nutrient availability and plant uptake - A greenhouse study. Land. 10, 372. doi: 10.3390/land10040372
Battaglia, M., Groover, G., and Tomason, W. (2018). Harvesting and Nutrient Replacement Costs Associated With Corn Stover Removal in Virginia. Virginia: Virginia Cooperative Extension Publication CSES-229NP.
Battaglia, M., Thomason, W., Fike, J. H., Evanylo, G. K., von Cossel, M., Babur, E., et al. (2021). The broad impacts of corn stover and wheat straw removal for biofuel production on crop productivity, soil health and greenhouse gas emissions: a review. GCB Bioen. 13, 45–57. doi: 10.1111/gcbb.12774
Bouwman, A. F. (1996). Direct emission of nitrous oxide from agricultural soils. Nutr. Cycl. Agroecosyst. 46, 53–70. doi: 10.1007/BF00210224
Cantarella, H., Otto, R., Soares, J. R., and de Brito Silva, A. G. (2018). Agronomic efficiency of NBPT as a urease inhibitor: A review. J. Adv. Res. 13, 19–27. doi: 10.1016/j.jare.2018.05.008
Chu, L., Hennayake, H. D., and Sun, H. (2019). Biochar effectively reduces ammonia volatilization from nitrogen-applied soils in tea and bamboo plantations. Phyton. 88, 261–267. doi: 10.32604/phyton.2019.07791
Clough, T. J., Condron, L. M., Kammann, C., and Müller, C. (2013). A review of biochar and soil nitrogen dynamics. Agron. 3, 275–293. doi: 10.3390/agronomy3020275
Diatta, A. A., Fike, J. H., Battaglia, M. L., Galbraith, J. M., and Baig, M. B. (2020). Efects of biochar on soil fertility and crop productivity in arid regions: a review. Arab. J. Geosci. 13, 595. doi: 10.1007/s12517-020-05586-2
Drury, C. F., Yang, X., Reynolds, W. D., Calder, W., Oloya, T. O., Woodley, A. L., et al. (2017). Combining urease and nitrification inhibitors with incorporation reduces ammonia and nitrous oxide emissions and increases corn yields. J. Environ. Qual. 46, 939–949. doi: 10.2134/jeq2017.03.0106
Eissa, M. A. (2019). Effect of compost and biochar on heavy metals phyto stabilization by the halophytic plant old man saltbush [Atriplex nummularia Lindl]. Soil Sed. Contam. Int. J. 28, 135–147. doi: 10.1080/15320383.2018.1551325
Fan, X. H., Song, Y. S., Lin, D. X., Yang, L. Z., and Luo, J. F. (2006). Ammonia volatilization losses and 15N balance from urea applied to rice on a paddy soil. J. Environ. Sci. 18, 299–303.
FAO/UNDP (1988). Land Resources Appraisal of Bangladesh for Agricultural Development, Vol 2, Agroecological Regions of Bangladesh. FAO: Rome, 1–570.
Fawcett, J. K. (1954). The semi-micro Kjeldahl method for the determination of nitrogen. J. Med. Lab. Technol. 12, 1–22.
Feng, Y., Sun, H., Xue, L., Liu, Y., Gao, Q., Lu, K., et al. (2017). Biochar applied at an appropriate rate can avoid increasing NH3 volatilization dramatically in rice paddy soil. Chemosphere. 168, 1277–1284. doi: 10.1016/j.chemosphere.2016.11.151
FRG (2018). Fertilizer Recommendation Guide-2018. Farmgate: Bangladesh Agricultural research Council (BARC).
Frimpong, K. A., Amoakwah, E., Osei, B. A., and Arthur, E. (2016). Changes in soil chemical properties and lettuce yield response following incorporation of biochar and cow dung to highly weathered acidic soils. J. Org. Agric. Environ. 4, 28–39. Available online at: http://hdl.handle.net/123456789/2138
He, T., Yuan, J., Luo, J., Lindsey, S., Xiang, J., Lin, Y., et al. (2018). Effects of application of inhibitors and biochar to fertilizer on gaseous nitrogen emissions from an intensively managed wheat field. Sci. Total Environ. 629, 121–130. doi: 10.1016/j.scitotenv.2018.02.048
IQAir. (2021). Air Quality in Delhi. Staad: IQAir. Available online at: https://www.iqair.com/india/delhi
Jahangir, M. M. R., Rahman, S., Mumu, N. J., Biswas, C., Jahiruddin, M., Mueller, C., et al. (2022). Crop residue interactions with fertilizer rate enhances volatilization loss and reduces nitrogen use efficiency in irrigated maize and potato. Arch. Agron. Soil Sci. 1, 1–13. doi: 10.3389/fenvs.2022.853655
Keeney, D. R., and Nelson, D. W. (1982). “Nitrogen-inorganic forms,” in Methods of Soil Analysis, Part 2. Chemical and Microbiological Propertie, eds. AL Page, RH Miller, DR Keeney (Madison, WI: Soil Science Society of America), 643–698.
Kelly, C. N., Calderon, F. C., Acosta-martinex, V., Mikha, M. M., Benjamin, J., Rutherford, D. W., et al. (2015). Switchgrass biochar effects on plant biomass and microbial dynamics in two soils from different regions. Pedosphere. 25, 329–342. doi: 10.1016/S1002-0160(15)30001-1
Kuttippurath, J., Singh, A., Dash, S. P., Mallick, N., Clerbaux, C., Van Damme, M., et al. (2020). Record high levels of atmospheric ammonia over India: spatial and temporal analyses. Sci. Total Environ. 740, 139986. doi: 10.1016/j.scitotenv.2020.139986
Lehmann, J., and Joseph, S. (2009). “Biochar for environmental management: An introduction,” in Biochar for Environmental Management, Science and Technology, eds J. Lehmann, S. Joseph (London: Earthscan), 1–12.
Linquist, B. A., Anders, M. M., Adviento-Borbe, M. A., Chaney, R. L., Nalley, L. L., Rosa, D. A., et al. (2015). Reducing greenhouse gas emissions, water use, and grain arsenic levels in rice systems. Glob. Change Biol. 21, 407–417. doi: 10.1111/gcb.12701
Liu, D., Ding, Z., Ali, E. F., Kheir, A. M. S., Eissa, M. A., Ibrahim, O. H. M., et al. (2021). Biochar and compost enhance soil quality and growth of roselle (Hibiscus sabdariffa L.) under saline conditions. Sci. Rep. 11, 1–11. doi: 10.1038/s41598-021-88293-6
Mahmoud, E., Ibrahim, M., Ali, N., and Ali, H. (2018). Spectroscopic analyses to study the effect of biochar and compost on dry mass of canola and heavy metal immobilization in soil. Commun. Soil Sci. Plant Anal. 49, 1990–2001. doi: 10.1080/00103624.2018.1492601
Malińska, K., Zabochnicka, M., and Dach, J. (2014). Effects of biochar amendment on ammonia during composting of sewage sludge. Ecol. Eng. 71, 478. doi: 10.1016/j.ecoleng.2014.07.012
Martins, M. R., Sarkis, L. F., Guareschi, R. F., Santos, C. A., Sant'anna, S. A., Zaman, M., et al. (2021a). A simple and easy method to measure ammonia volatilization: accuracy under field conditions. Pedosphere 31, 225–264. doi: 10.1016/S1002-0160(20)60077-7
Martins, M. R., Sarkis, L. F., Sant'Anna, S. A. C., Santos, C. A., Araujo, K. E., Santos, R. C., et al. (2021b). Optimizing the use of open chambers to measure ammonia volatilization in field plots amended with urea. Pedosphere 31, 243–254. doi: 10.1016/S1002-0160(20)60078-9
Mazzetto, A. M., Styles, D., Gibbons, J., Arndt, C., Misselbrook, T., Chadwick, D., et al. (2020). Region-specific emission factors for Brazil increase the estimate of nitrous oxide emissions from nitrogen fertiliser application by 21%. Atmos Environ. 230, 117506. doi: 10.1016/j.atmosenv.2020.117506
Mosier, A., Kroeze, C., Nevison, C., Oenema, O., Seitzinger, S., Cleemput, O., et al. (1998). Closing the global N2O budget: nitrous oxide emissions through the agricultural nitrogen cycle inventory methodology. Nutrient Cycl. Agroecosyst. 52, 225–248. doi: 10.1023/A:1009740530221
Nichols, K. L., Del Grosso, S. J., Derner, J. D., Follett, R. F., Archibeque, S. L., Delgado, J. A., et al. (2018). Nitrous oxide and ammonia emissions from cattle excreta on shortgrass steppe. J. Environ. Qual. 47, 419–426. doi: 10.2134/jeq2017.12.0463
Pan, B., Lam, S. K., Mosier, A., Luo, Y., and Chen, D. (2016). Ammonia volatilization from synthetic fertilizers and its mitigation strategies: a global synthesis. Agric Ecosyst. Environ. 232, 283–289. doi: 10.1016/j.agee.2016.08.019
Rahaman, M. A., Zhan, X., Zhang, Q., Li, S., Long, Y., Zeng, H., et al. (2020). Ammonia volatilization reduced by combined application of biogas slurry and chemical fertilizer in maize–wheat rotation system in North China Plain. Sustainability. 12, 4400. doi: 10.3390/su12114400
Rochette, P., Angers, D. A., Chantigny, M. H., Gasser, M. O., MacDonald, J. D., and Pelster, D. E. (2013), NH3. volatilization, soil NH4+ concentration soil pH following subsurface banding of urea at increasing rates. Can. J. Soil Sci. 93, 261–268. doi: 10.4141/cjss2012-095.
Rochette, P., Angers, D. A., Chantigny, M. H., MacDonald, J. D., Bissonnette, N., Bertrand, N., et al. (2009). Ammonia volatilization following surface application of urea to tilled and no-till soils: a laboratory comparison. Soil Till. Res. 103, 310–315. doi: 10.1016/j.still.2008.10.028
Saarnio, S., Heimonen, K., and Kettunen, R. (2013). Biochar addition indirectly affects N2O emissions via soil moisture and plant N uptake. Soil Biol. Biochem. 58, 99–106. doi: 10.1016/j.soilbio.2012.10.035
Sha, Z., Li, Q., Lv, T., Misselbrook, T., and Liu, X. (2019). Response of ammonia volatilization to biochar addition: A meta-analysis. Sci. Total Environ. 655, 1387–1396. doi: 10.1016/j.scitotenv.2018.11.316
Sommer, S. G., Schjoerring, J. K., and Denmead, O. T. (2004). Ammonia emission from mineral fertilizers and fertilized crops. Adv. Agron. 82, 557–622. doi: 10.1016/S0065-2113(03)82008-4
Stokstad, E. (2014). Ammonia pollution from farming may exact hefty health costs. Science. 343, 238. doi: 10.1126/science.343.6168.238
Sun, H., Lu, H., Chu, L., Shao, H., and Shi, W. (2017). Biochar applied with appropriate rates can reduce N leaching, keep N retention and not increase NH3 volatilization in a coastal saline soil. Sci. Total Environ. 575, 820–825. doi: 10.1016/j.scitotenv.2016.09.137
Trupiano, D., Cocozza, C., Baronti, S., Amendola, C., Vaccari, F. P., Lustrato, G., et al. (2017). The effects of biochar and its combination with compost on lettuce (Lactuca sativa L.) growth, soil properties, and soil microbial activity and abundance. Hindawi Int. J. Agron. 12, 3158207. doi: 10.1155/2017/3158207
Uddin, S., Nitu, T. T., Milu, U. M., Nasreen, S. S., Hossenuzzaman, M., Haque, M. E., et al. (2021). Ammonia fluxes and emission factors under an intensively managed wetland rice ecosystem. Environ. Sci. Proc Impacts. 23, 132–143. doi: 10.1039/D0EM00374C
Walkley, A., and Black, I. A. (1934). An examination of Degtjareff method for determining soil organic matter and a proposed modification of the chromic acid titration method. Soil Sci. 37, 29–37. doi: 10.1097/00010694-193401000-00003
Wang, C., Sun, H., Zhang, J., Zhang, X., Lu, L., Shi, L., et al. (2021). Effects of different fertilization methods on ammonia volatilization from rice paddies. J. Cleaner Prod. 295, 126299. doi: 10.1016/j.jclepro.2021.126299
Wang, H., Zhang, Y., Han, Q., Xu, Y., Hu, G., Xing, H., et al. (2016). The inflammatory injury of heart caused by ammonia is realized by oxidative stress and abnormal energy metabolism activating inflammatory pathway. Sci Total Environ. 742, 140532. doi: 10.1016/j.scitotenv.2020.140532
Wolfe, A. H., and Patz, J. A. (2002). Reactive Nitrogen and human health: acute and long-term implications. Ambio. 31, 120–125. doi: 10.1579/0044-7447-31.2.120
Xia, L., Ti, C., Li, B., Xia, Y., and Yan, X. (2016). Greenhouse gas emissions and reactive nitrogen releases during the lifecycles of staple food production in China and their mitigation potential. Sci. Total Environ. 556, 116–125. doi: 10.1016/j.scitotenv.2016.02.204
Xia, Y., and Yan, X. (2012). Ecologically optimal nitrogen application rates for rice cropping in the Taihu Lake region of China. Sustain. Sci. 7, 33–44. doi: 10.1007/s11625-011-0144-2
Xu, L., and Penner, J. E. (2012). Global simulations of nitrate and ammonium aerosols and their radiative effects. Atmos. Chem. Phys. 12, 9479–9504. doi: 10.5194/acp-12-9479-2012
Xu, N., Tan, G., Wang, H., and Gai, X. (2016). Effect of biochar additions to soil on nitrogen leaching, microbial biomass and bacterial community structure. Soil Biol. 74, 1–8. doi: 10.1016/j.ejsobi.2016.02.004
Xu, R. T., Tian, H., Pan, S., Prior, S. A., Feng, Y., Batchelor, W. D., et al. (2019). Global ammonia emissions from synthetic nitrogen fertilizer applications in agricultural systems: Empirical and process-based estimates and uncertainty. Global Change Biol. 25, 314–326. doi: 10.1111/gcb.14499
Zaman, M., Kleineidam, K., Bakken, L., Berendt, J., Bracken, C., Butterbach-Bahl, K., et al. (2021). “Methodology for measuring greenhouse gas emissions from agricultural soils using non-isotopic techniques,” in Measuring Emission of Agricultural Greenhouse Gases and Developing Mitigation Options Using Nuclear and Related Techniques, eds M Zaman, L Heng, C Müller (Cham: Springer), 82–89.
Zhang, X., Fang, Q., Zhang, T., Ma, W., Velthof, G. L., Hou, Y., et al. (2020). Benefits and trade-offs of replacing synthetic fertilizers by animal manures in crop production in China: a meta-analysis. Global Change Biol. 26, 888–900. doi: 10.1111/gcb.14826
Zhao, Z. Q., Bai, Z. H., Winiwarter, W., Kiesewetter, G., Heyes, C., Ma, L., et al. (2017). Mitigating ammonia emission from agriculture reduces PM2.5 pollution in the Hai River Basin in China. Sci. Total Environ. 609, 1152–1160. doi: 10.1016/j.scitotenv.2017.07.240
Keywords: emission factor, NH3 emissions, yield- rice yield, soil quality, scaled NH3 emissions, ammonia emissions
Citation: Ferdous J, Mumu NJ, Hossain MB, Hoque MA, Zaman M, Müller C, Jahiruddin M, Bell RW and Jahangir MMR (2023) Co-application of biochar and compost with decreased N fertilizer reduced annual ammonia emissions in wetland rice. Front. Sustain. Food Syst. 6:1067112. doi: 10.3389/fsufs.2022.1067112
Received: 11 October 2022; Accepted: 07 December 2022;
Published: 12 January 2023.
Edited by:
Sudhakar Srivastava, Banaras Hindu University, IndiaReviewed by:
María Sánchez-García, Spanish National Research Council (CSIC), SpainRishikesh Singh, Panjab University, India
Copyright © 2023 Ferdous, Mumu, Hossain, Hoque, Zaman, Müller, Jahiruddin, Bell and Jahangir. This is an open-access article distributed under the terms of the Creative Commons Attribution License (CC BY). The use, distribution or reproduction in other forums is permitted, provided the original author(s) and the copyright owner(s) are credited and that the original publication in this journal is cited, in accordance with accepted academic practice. No use, distribution or reproduction is permitted which does not comply with these terms.
*Correspondence: M. M. R. Jahangir, bW1yamFoYW5naXJAYmF1LmVkdS5iZA==