- 1Faculty of Land and Food Systems, The University of British Columbia, Unceded xwməθkwəýəm Musqueam Territory, Vancouver, BC, Canada
- 2School of the Environment, Trent University, Peterborough, ON, Canada
- 3Centre for Sustainable Food Systems, The University of British Columbia, Unceded xwməθkwəýəm Musqueam Territory, Vancouver, BC, Canada
- 4Faculty of Forestry, The University of British Columbia, Unceded xwməθkwəýəm Musqueam Territory, Vancouver, BC, Canada
- 5Biodiversity Research Centre, The University of British Columbia, Unceded xwməθkwəýəm Musqueam Territory, Vancouver, BC, Canada
Balancing economic and environmental objectives can present trade-offs for organic farmers maximizing crop yields while maintaining core principles of ecology and health. A primary challenge for achieving this balance is nitrogen (N) and phosphorus (P) management. Meeting crop N requirements with compost can build soil carbon (C) and soil health but often over-applies P and increases soil P and associated environmental risks. Alternatively, high-N organic fertilizers can provide N without surplus P but can be expensive and lack C inputs that composts supply. We evaluated these potential trade-offs in 2-year field trials on 20 mixed vegetable farms across three regions of Southwest British Columbia, Canada, capturing a range of climatic-edaphic conditions and organic amendments. Three nutrient management strategies were evaluated: High Compost: compost applied to meet crop N removal, Low Compost + N: compost applied to meet crop P removal plus an organic fertilizer to meet crop N removal, and Typical: varying combinations of composts and/or organic fertilizers (“typical” nutrient application on the farm). Nutrient strategies were evaluated in terms of yield, input costs, and soil properties [permanganate oxidizable C (labile C responsive to soil management), and post-season available N and P]. Soil P was 21% higher with High Compost than Low Compost + N. In one region characterized by inexpensive but nutrient-rich composts and soils high in P, input costs were lowest with Typical, but in the second year, High Compost outperformed Typical in crop yield. Principal component analysis showed a divergence in post-season between nutrient strategies in relation to compost and soil properties: High Compost using high-N composts increased post-season (0–30 cm), whereas relative yields in High Compost tended to be higher on farms with lower soil C and lower C:N composts, while yields with Typical were higher under opposite conditions but associated with higher post-season . Combining input types (e.g., Low Compost + N) can meet environmental objectives in reducing surplus soil P without short-term yield or cost trade-offs compared to High Compost. However, maintaining soil C needs to be investigated to achieve effective ecological nutrient management in organic vegetable production with improved nutrient balances.
Introduction
Organic agriculture aims to sustain healthy people, soils, and ecosystems through a reliance on ecological processes, biological cycles, and biodiversity (Gomiero et al., 2011). With this set of ambitious social and environmental goals, researchers and policymakers have proposed organic farming systems as a way to achieve sustainable agricultural development (Seufert, 2012). This also calls for enhanced production of regionally-grown, nutritious food that supports the livelihoods of small- and medium-scale farmers. Balancing environmental and economic objectives, however, is a particular challenge for organic farmers as they strive to maximize crop yields while maintaining core organic principles of ecologically based management. While organic agriculture can be beneficial for local economies (Marasteanu and Jaenicke, 2019), nutrient management, especially soil nitrogen (N) availability (Berry et al., 2002; Seufert et al., 2012), is a key challenge to organic farming systems contributing to agricultural productivity goals, and (de Ponti et al., 2012); organic farmers rank nutrient management as a top research priority (Jerkins and Ory, 2016).
Organic amendments (e.g., composts, manures, specialty fertilizers) and cover crops are used both for in-season nutrient supply and to build soil organic matter (SOM) to provide long-term soil fertility (Gomiero et al., 2011). However, these inputs have a range of biochemical properties, and unknown or uncertain nutrient content, that make it difficult to predict nutrient supply and match crop nutrient demand (Gale et al., 2006; Maltais-Landry et al., 2016). Vegetable crops—the focus of this study—require relatively high amounts of soil mineral N [ammonium () and nitrate ()] during the growing season, but excess amounts post-harvest can be lost to the surrounding environment, especially through leaching in regions with high rainfall (Maltais-Landry et al., 2019). For any nutrient management approach, a careful assessment of production, economic, and environmental outcomes that accounts for variation in local conditions is required to reduce potential trade-offs and ensure sustainability goals are met.
Balancing nutrient budgets is a central goal of ecological nutrient management (Drinkwater and Snapp, 2007) but is challenging when composts are the primary nutrient source. Applying these types of amendments to meet crop N demand is common on organic farms, but the high P to plant-available N (PAN) ratio in these amendments relative to crop requirements builds up soil P over time (Watson et al., 2002; Nelson and Janke, 2007; Maltais-Landry et al., 2016; Carr et al., 2019). This problem is exacerbated by low first-year N availability in these amendments, including immobilization of N from the soil mineral pool (i.e., −10% to +54% of total N; Gale et al., 2006), in contrast to greater first-year P availability (i.e., +70% to +100% of total P; Nelson and Janke, 2007). As an alternative, or in combination with composts and manures, farmers can use biological N fixation from leguminous cover crops and/or apply specialty organic fertilizers to meet crop N demands while adding little to no P.
High-N but low-P specialty organic fertilizers (e.g., feather meal, blood meal, alfalfa meal/pellets, fish meal), can help balance N and P budgets (Maltais-Landry et al., 2016; Sullivan and Andrews, 2017), but these inputs can be relatively expensive, especially by comparison in regions with intensive livestock industries where manures are abundant (Spargo et al., 2016; Reid et al., 2019; Svanbäck et al., 2019). Reducing compost applications to not exceed crop P requirements provides much less C than when applied to meet crop N requirements (Eghball, 2002; Maltais-Landry et al., 2019), and at typical rates of application, specialty organic fertilizers are limited sources of C compared to composts (White et al., 2020). The impact of changing nutrient management strategies on soil C is important to assess, but difficult to measure with common indicators such as SOM and total soil organic C (SOC) given their slow rate of change (Gregorich et al., 1994; Bünemann et al., 2018). More responsive soil health indicators such as permanganate oxidizable C (POXC) and polysaccharides are likely to provide better insight into the effects of different amendment combinations on soil health (Bünemann et al., 2018).
With varied topographies, climates, and soil types, the province of British Columbia (BC) provides unique conditions for diverse agricultural crops and types of production systems. One of the main agricultural regions in BC is the lower Fraser Valley, where 29.8% of all farms and 26.4% of certified organic farms in the province are located (Government of British Columbia, 2017). Rising land prices coupled with emerging markets outside of urban centers are opening opportunities for agricultural production in other areas of BC such as Vancouver Island and Pemberton Valley. Those two regions have fewer animal livestock operations than the lower Fraser Valley, and thus less access to manure-based composts. High precipitation in the non-growing season across all regions makes leaching of particular concern. With unique soil types, climatic conditions, and types of available organic amendments among these three agricultural regions, nutrient strategy performance among the regions would most likely be different.
This study evaluated ecological nutrient management practices on working mixed vegetable farms in three agricultural regions of southwest BC (the lower Fraser Valley, Pemberton Valley, and Vancouver Island). This study is aimed at overcoming the constraints of research station studies, which can have limited applicability outside of the climate, soil, and management conditions at one or two study sites (Vanlauwe et al., 2019). Our multi-site study introduces greater heterogeneity in field conditions (i.e., background variability) to better understand how treatments perform under real, but varied, agronomic and economic conditions on working farms (Coe et al., 2019). We compared three treatments that represent common but contrasting nutrient management approaches: High Compost: Compost applied at a rate to target crop N removal, Low Compost + N: Compost applied at a rate to target crop P removal plus an organic fertilizer (feather meal) at a rate to meet crop N removal, and Typical: The nutrient application that the farmer would typically use for the specific crop (varying combinations of organic fertilizers, composts, and manures, or no amendments applied). The specific objectives of this study were to evaluate the effect of these three nutrient strategies on farms across the three regions on crop yield, input costs, and selected soil properties [POXC, and post-season available N ( and ) and available P], and identify the farm site edaphic, environmental, and input quality factors that affect nutrient strategy outcomes, particularly any trade-offs among them.
Materials and methods
Field trials were established in the spring of 2018 on vegetable farms that rely on organic amendments in three regions of southwest BC: the lower Fraser Valley, Pemberton Valley, and Vancouver Island; see Supplementary Figure 1 for a map of farm site locations in the three regions. A total of 20 different farms participated over the 2-year study period, with 19 farms in the first year and 18 in the second year. Sixteen out of the 20 farms were certified organic, while four were using organic nutrient management practices, but were not certified organic. The farms in this study sell directly to customers (e.g., farmers' markets or similar programs). As part of this direct marketing strategy, these farms also grow a large diversity of crops (30–50 different vegetable, herb, or fruit types each year), with the exception of one farm which specializes in growing fewer crops for wholesale markets (e.g., corn, beans, peas, potatoes, barley). All farms in this study are primarily mixed vegetable farms and use intensive tillage. Additional characteristics of the three regions are summarized in Table 1, and additional farm characteristics are provided in Supplementary Table 1.
Experimental design
At each of the farm sites, the following three nutrient management strategies were evaluated:
• High Compost: Compost was applied to meet crop N removal (the amount of N exported from the field with crop harvest);
• Low Compost + N: Compost was applied to meet crop P removal (the amount of P exported from the field with crop harvest) and a feather meal fertilizer was applied to meet crop N removal;
• Typical: Varying combinations of organic fertilizers, composts, and manures, or in some cases, no amendments, were applied. This was the “business as usual” nutrient application that each farmer uses for their farm and was different for each farm. The amendments used for Typical were determined by each farmer for the Typical plot on their farm and we simply quantified these for this study.
Each nutrient strategy treatment was established in one plot per farm site, so each farm site in each year had a total of three plots. Within each farm site and year, all plots were managed the same and only differed by the nutrient strategy applied. Plot size depended on the size of the farm but averaged 29.3 m2 and ranged from 6.3 to 100.0 m2. Overall, the research plots at 11 farm sites received the same nutrient management strategy for 2 years, and at 23 farm sites for 1 year.
Crops grown in the research plots in 2018 included beet (Beta vulgaris L. subsp. Vulgaris), broccoli (Brassica oleracea L. var. botrytis L.), carrot (Daucus carota L. subsp. sativus), cauliflower (Brassica oleracea L. var. botrytis L.), potato (Solanum tuberosum L.), and pickling cucumber (Cucumus sativus L.), and in 2019 included cabbage (Brassica oleracea L. var. capitata), carrot, beet, onion (Allium cepa L. var. cepa), and potato. The distribution of these crops across the farms' research plots is shown in Supplementary Table 1.
Amendment rate calculations
Amendments were applied at rates to target crop-specific N and P removal. Estimates of crop N and P removal in harvests were determined from target (or expected) yields chosen by each farmer for their crop. Nutrient concentrations from local data were used, but if not available then crop-specific recommended nutrient application rates from best-available sources were used as target nutrient application rates instead. Target nutrient application rates used in this study are summarized by general crop groups in Table 2 and data sources and nutrient application rates are listed in Supplementary Table 1.
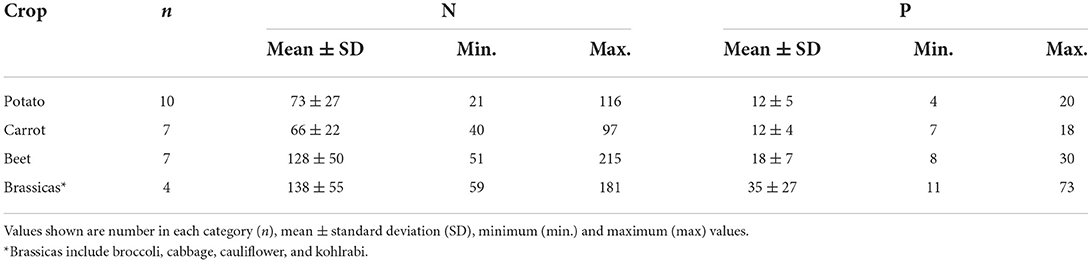
Table 2. Estimated nitrogen (N) and phosphorus (P) crop removal rates (kg ha−1) based on pre-season estimated yields, averaged across major crop categories.
Composts were unique to each farm and were either currently being used by the farmer or we sourced them from regionally-available options. Composts therefore varied widely in their composition due to varied feedstocks and sources and compost C:N ratios ranged from 9.3 to 39.4 (unpublished data). Composts were applied on various spring and summer dates to match when the farm would be planting; see Supplementary Table 2 for the mean and median amendment application rates, application dates, and associated C, N, and P application rates by nutrient strategy and region. All composts and fertilizers were weighed and broadcast by hand, then incorporated into the soil either by hand by us or by the farmer with tractor-mounted equipment.
For High Compost, compost was applied at a rate where the estimated rate of crop removal N was matched with the estimated in-season PAN from the compost. For Low Compost + N, both compost and feather meal were used: compost was applied at a rate where the estimated rate of crop removal P was matched with total P from the compost, and feather meal was applied at a rate to supply PAN to match the difference between PAN applied with the compost and the estimated crop removal N.
Estimated compost PAN was calculated as 15% of the compost organic N plus the compost inorganic N ( and ). A 15% mineralization rate was used based on the literature and a conservative approach to ensure adequate N availability from a variety of composts and manures (Gale et al., 2006). These calculations were made using the following equation:
Two different feather meal products were used in Low Compost + N because of regional availability. A feather meal with reported 11% N (11-0-0, Natures Intent, Pacific Calcium Inc., Tonasket, WA, USA) was used on all farms in the lower Fraser Valley and Pemberton Valley and a feather meal with reported 13% N (13-0-0, Gaia Green, Grand Forks, BC, Canada) was used for all farms on Vancouver Island. For both feather meal products, calculations were based on “guaranteed” total N concentration reported by the manufacturer (on the bag label), and 100% of this N was assumed to become PAN during the growing season, (i.e., 100% mineralization). The C:N ratios of the Gaia Green and Nature's Intent feather meals were 4.2 and 5.3, respectively. Adjustments for moisture content were made in calculations for composts but not for the dried feather meal fertilizer products.
Amendments were weighed and applied by hand to High Compost and Low Compost + N plots using shovels, a 5-gallon bucket, and a field scale. Amendment application rates in Typical were quantified in two ways. If amendments were spread by hand, we weighed and applied them by hand based on instructions from the farmer. Alternatively, if a tractor-mounted compost spreader was used, then we used a tarp and 1 m × 1 m quadrat to measure the application rate. Briefly, we first covered the two research plots (High Compost and Low Compost + N) with a heavy-duty poly tarp held in place with ground staples. The farmer then drove over the tarped area while spreading manure at their typical rate with a tractor-mounted compost spreader (applying amendment directly onto the Typical plot as they went). Next, 51 m2 subsamples of compost were collected from the tarp and the weight of amendment collected from each subsample was recorded. The subsamples were averaged to represent what was spread on the Typical plot (kg amendment m−2).
All farms in this study use cover crops as part of their overall management, but only five farms (two in 2018 and three in 2019) had winter cover crops in the research plots before the growing seasons in which our research took place. Cover crops were observed to be uniform across the three plots at the five farm sites with cover crops; one farm site had fall rye (Secale cereale L.), one farm site had fall rye, hairy vetch (Vicia villosa Roth), and winter peas (Pisum sativum L.), and the other three had fall rye, hairy vetch, and crimson clover (Trifolium incarnatum L.). Given the challenge of coordinating sampling with farmers, and that so few farms had cover crops, N inputs from cover crops were not included in the estimate of N supply from the nutrient strategies.
Soil and compost sampling and analyses
Compost analyses
Compost samples were taken directly from compost piles at each farm during initial farm visits on various dates in the spring of both years of the study. Five subsamples of roughly 0.5 L volume and from 0.5 m depth into the pile were collected from different locations on the pile and mixed thoroughly to make a composite sample. Compost was analyzed at the BC Ministry of Environment and Climate Change Strategy Analytical Laboratory (MOE), Victoria, BC, Canada for and , total C, N, P, and K, pH, EC, and water content.
Within 72 h of sample collection, and were measured using a 2 M potassium chloride (KCl) extraction (Maynard et al., 2008) and analyzed colorimetrically using an A2 Analyzer (Astoria-Pacific, Clackamas, USA) (Weatherburn, 1967; Doane and Horwáth, 2003). Total P and K of composts were determined by microwave-assisted acid digestion using an ultraWave microwave (Milestone, Sorisole, Italy; Karam, 2008) then element concentrations were determined by ICP-OES on a Prodigy Spectrometer (Teledyne Leeman Labs, Mason, OH, USA). Total C and N were measured by combustion on a Flash 2000 Elemental Analyzer (Thermo Fischer Scientific Inc., Waltham, MA, USA; Thermo Fisher, 2010). Electrical conductivity was measured using a 1:4 compost to water ratio with 5 g of compost shaken with deionized water (Hendershot et al., 2008a) and conductivity was read on a conductivity meter and small volume flow-through cell. Varying compost to water ratios were used to measure pH, depending on the compost. First, deionized water was added to 5 g of compost and stirred. After resting for 30 min, the suspension was stirred again, and pH was measured with a pH meter (Hendershot et al., 2008b).
Compost bulk density was measured on farm sites using a 5-gallon bucket (Washington State University, 2020). First, a scale was tared to the weight of an empty 5-gallon bucket, then the bucket was filled 1/3 full of compost taken from a hole dug in the compost pile (not from the dry outer layer). Next, the bucket was dropped ten times from a roughly 0.3 m height onto a hard surface. The bucket was then filled to 2/3 full of compost, dropped ten times again, filled to full, and dropped ten times again. Finally, the bucket was filled to full again and the weight was taken, and the compost bulk density was calculated by dividing by the volume of the bucket. This was repeated three times and the average was used.
Soil analyses
Depending on conditions, soil samples were collected using either a soil auger (5.5 cm inner diameter) or probe (1.9 cm inner diameter). Ten to fifteen subsamples were taken from each plot when using a probe, or five subsamples when using an auger to account for differences in sampling volume. Soil samples were taken three times at all farms in 2018 (pre-season, mid-season, and post-season) and two times for all farms in 2019 (mid-season and post-season), except for at the three new farm sites in 2019 that were not included in the first year of the study, where pre-season samples were also collected in 2019. Pre-season soil samples were analyzed at the same laboratory (MOE) as compost samples and mid- and post-season soil samples were analyzed in our lab.
Pre-season soil samples were analyzed for a variety of properties and were collected at two depths (0–15 and 15–30 cm) prior to applying amendments. Within 72 h of collection, samples were analyzed for and using a 2 M potassium chloride (KCl) extraction using the same methods as described previously for composts. The remaining sample was dried (35°C), ground, and sieved to <2 mm particle size prior to all other analyses. Percent sand, silt, and clay were determined using the hydrometer sedimentation method with water maintained at 25°C and particles were dispersed using Calgon detergent prior to analysis (Kroetsch and Wang, 2008). Total C and N were measured by combustion on a Flash 2000 Elemental Analyzer (Thermo Fischer Scientific Inc., Waltham, MA, USA; Thermo Fisher, 2010) and inorganic C was measured on a Primacs SNC-100 TN/TC Analyzer (Skalar, Breda, the Netherlands; Skalar Analytical, 2019). Available P and potassium were measured from 2.5 g of soil with 25 mL of Mehlich-3 extractant (Ziadi and Sen Tran, 2008). After filtration the element concentrations were determined by ICP-OES on a Prodigy Spectrometer (Teledyne Leeman Labs, Mason, OH, USA). Electrical conductivity was measured using a 1:2 soil water ratio with 10 g of soil shaken with deionized water in a 50 mL centrifuge tube for 1 min then centrifuged for 10 min (Hendershot et al., 2008a). For pH a 1:1 soil water ratio with 10 g of soil was used (Hendershot et al., 2008b).
Mid-season soil samples were analyzed for POXC. Samples were collected in July both years at one depth (0–15 cm) from within crop rows. Soil samples were air-dried and sieved to 2 mm, then 2.5 g of soil was combined with 18.0 mL of distilled water and 2.0 mL of 0.2 M potassium permanganate (KMnO4) solution adjusted to pH 7.2 (Weil et al., 2003) and analyzed on a 96-well plate on a TECAN Spark® spectrophotometer at 550 nm (TECAN Group Ltd., Mannedorf, Switzerland). For one farm site with high SOM [SOC ~10%; POXC > 2,500 mg kg−1)], 1 g (instead of 2.5 g) of soil was used to avoid full consumption of in the reaction (Wade et al., 2020; Liptzin et al., 2022).
Post-season soil samples were analyzed for available N and P and were collected at two depths (0–15 and 15–30 cm) after crops had been harvested (between September 25 and October 16). Samples were collected prior to the latest date for a valid post-harvest nitrate test (PHNT) according to provincial guidelines (Government of British Columbia, 2019), which account for soil texture and local precipitation. Samples from both depths were analyzed for and by extracting 2.5 g of field-moist soils with 25 mL of 2 M potassium chloride (KCl) (Maynard et al., 2008) and were measured colorimetrically (Weatherburn, 1967; Doane and Horwáth, 2003) using a spectrophotometer (Bio-Rad iMark, Hercules, CA, USA). Samples from only the surface depth (0–15 cm) were air-dried and sieved and were analyzed for Kelowna-extractable P (van Lierop, 1988) by extracting 2.5 g of air-dried soils with 25 mL of a solution of 0.015 M ammonium fluoride (NH4F) and 0.25 M acetic acid (CH3COOH) and were determined on a Varian 725-ES ICP-OES (Agilent Technologies, Mulgrave, Victoria, Australia). To determine soil water content, the weight of a field-moist soil sample was recorded before and after oven drying for 48 h at 105°C.
Crop yield sampling
The number of crop biomass subsamples taken per plot was equal to or >30% of the total crop area in a given plot, minus the area used as buffers on the plot perimeter (between two to ten subsamples, depending on plot size). Subsamples were averaged and recorded as the weight of crop biomass per one bed meter (kg m−1). Harvest dates, plot sizes, and number of subsamples taken per plot are shown in Supplementary Table 1. Plot buffer widths varied between 0.5 and 2.5 m, depending on farm management and type of tillage equipment used. A stratified sampling method was used to choose subsample locations in each plot. Subsamples were taken by placing a 1 m × 1 m quadrat on top of a crop bed, then all marketable crop biomass (e.g., potato tubers but not tops, cabbage heads, beets with tops) between the two ends of the quadrat were harvested and weights were recorded.
Estimating input costs
Input cost data was collected from each farm and includes both the amount paid for the amendment as well as associated shipping or transportation costs. Input costs are reported in Canadian (CAD) dollars per hectare ($ ha−1), as a function of the input costs and their rate of application, extrapolated to 1 hectare. Most farmers within the study have their off-farm amendments delivered to the farm and therefore provided us with shipping costs as part of this calculation. For farms that pick up amendments locally, the farmer's time and vehicle mileage were valued at $20 h−1 and $0.59 km−1, respectively, and were applied to an estimate of round-trip time and mileage, which were provided by the farmer. Any inputs that did not include the two nutrients being studied, N or P (e.g., lime, micronutrients, etc.), were not included in the total cost because they were applied to all three plots and not examined in this study. In the case where the farm was unable to report/estimate shipping costs, the cost was estimated based on nearby farms or estimated mileage costs to the nearest available retailer.
Statistical analyses
We performed all analyses in R (R Core Team, 2019). For various reasons (e.g., farms harvested before our sampling, crop failure, unreported input costs), not every farm site has a complete data set for each year (i.e., all five outcomes). Overall, 63% of farm sites have complete datasets, and sample sizes are reported for each analysis.
We used linear mixed-effects (LME) models to account for the impact of the farm-specific, variable background characteristics on the mean response of the measured outcomes (e.g., yield, POXC, etc.) for each farm site (Crawley, 2013; Krzywinski and Altman, 2014; Webster and Lark, 2018), and to account for autocorrelation of repeated measures where the same plots were sampled from in both years (Krzywinski et al., 2014). We considered each year within one farm as a nested random effect in the model. We performed all analyses with the lme function in the nlme package version 3.1-143 (Pinheiro et al., 2019) using the maximum likelihood (ML) method for model comparisons and the restricted maximum likelihood (REML) method for reporting final model output. As the primary explanatory variable of interest, we included nutrient management strategy as a categorical fixed effect with three levels (High Compost, Low Compost + N, and Typical). We included year (2018 and 2019), region (lower Fraser Valley, Pemberton Valley, and Vancouver Island), and all interactions as fixed effects to investigate if the impact of nutrient strategy on the dependent variables was different between years or regions (i.e., to consider interactions). We tested assumptions of normality and homogeneity of variance using the Shapiro-Wilk test and Bartlett test, respectively, and we transformed data if needed to meet assumptions.
We performed stepwise elimination of terms in the LME models to identify the most parsimonious model based on AIC (Crawley, 2013) and marginal and conditional R2 values (Nakagawa and Schielzeth, 2013); we report output from performing ANOVA for each of the selected LME models. When there were significant interactions between the fixed effect of nutrient strategy and region and/or year, we ran the model separately to assess nutrient strategy within a year and/or region. When the main effect of nutrient strategy was significant in the LME model ANOVA, a post-hoc (Tukey method) test was used to determine significant differences between factor levels using the emmeans function (Lenth, 2019). We determined differences to be significant for p-values < 0.05, and marginally significant for p-values < 0.10. ANOVA F and p-values are reported in Supplementary Tables 3–9. When LME models were run with nutrient strategy and cover crops (presence or absence) as fixed effects, we found that the impact of nutrient strategy on measured outcomes did not vary by the presence or absence of cover crops (nutrient strategy × cover crop interaction was not significant) and, thus, cover crops were not further considered in our analysis.
We used principal component analysis (PCA) using the FactoMineR package (Husson et al., 2020) to assess how the nutrient strategy outcomes (yield and post-season ) covary with each other, farm site pre-season soil properties [soil C and available soil P (0–15 cm)], and farm-specific compost properties (compost total N and P, and compost C:N). We focused on yield and post-season as outcomes in the PCA as they represent a direct trade-off between productivity and environmental impacts. We plotted each outcome as data relativized at each farm site by dividing each observation within a farm site by the farm site average. This allows the outcomes in the PCA to vary by the impact of nutrient strategy rather than between-farm variation. We did not plot pre-season soil or compost variables that are auto-correlated (e.g., soil total N and total organic C). We transformed non-normally distributed data to satisfy conditions of normality for the PCA.
Results
Soil properties: POXC and post-season available N and P
Differences in post-season available N were observed among nutrient strategies in the upper depth (0–15 cm), but not in the lower depth (15–30 cm). Specifically, while post-season (0–15 cm) did not differ among nutrient strategies across region and years (Figure 1A), a region-specific response was observed (nutrient strategy × region interaction, p = 0.010). When analyzed by region, the main effect of nutrient strategy was marginally significant in the lower Fraser Valley and Pemberton Valley (Supplementary Table 7), with trends of greater post-season (0–15 cm) with High Compost and Typical than Low Compost + N in the lower Fraser Valley, and opposite trends in Pemberton Valley (Figure 1B), although none of these differences were significant in the post-hoc test. Across regions and years, Typical and High Compost had higher post-season (0–15 cm) than Low Compost + N (Figure 2A). However, the impact of nutrient strategy on post-season varied among the regions (nutrient strategy × region interaction, p = 0.003), and when analyzed by region, the main effect of nutrient strategy was significant in the lower Fraser Valley and on Vancouver Island, but not in the Pemberton Valley (Figure 2B). On Vancouver Island, post-season (0–15 cm) was lower in Low Compost + N than Typical (Figure 2B). In the lower Fraser Valley, there was a trend of less (0–15 cm) with Low Compost + N than High Compost (Tukey contrast, p = 0.052).
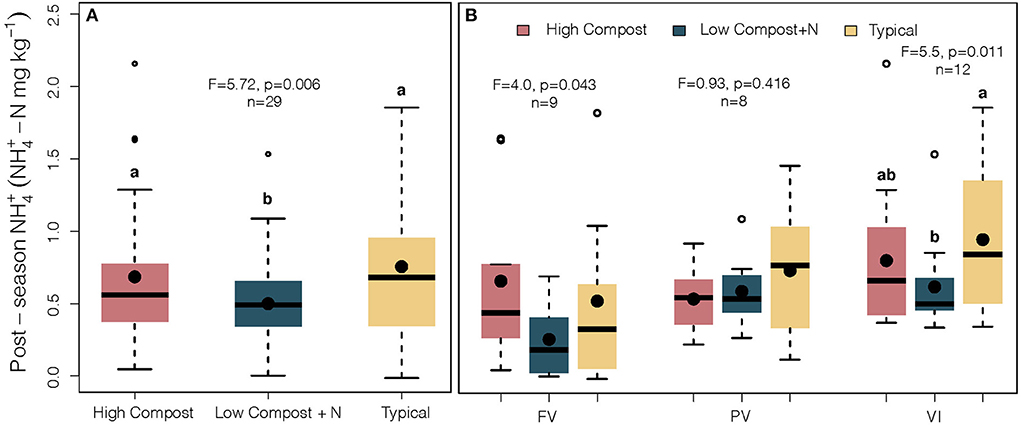
Figure 1. Boxplots of post-season -N (mg kg−1) (0–15 cm). (A) By nutrient management strategy, across regions and years, and (B) by nutrient management strategy within three vegetable production regions of British Columbia [lower Fraser Valley (FV), Pemberton Valley (PV), and Vancouver Island (VI)] over years. ANOVA F and p-value refer to main effect of nutrient strategy. Boxplots with different letters represent significant differences among nutrient strategies using Tukey's post-hoc test at p < 0.05. The center line indicates the median, means are shown as black dots, sample size is indicated by n, and the Tukey-style whiskers extend to a maximum of 1.5 the interquartile range.
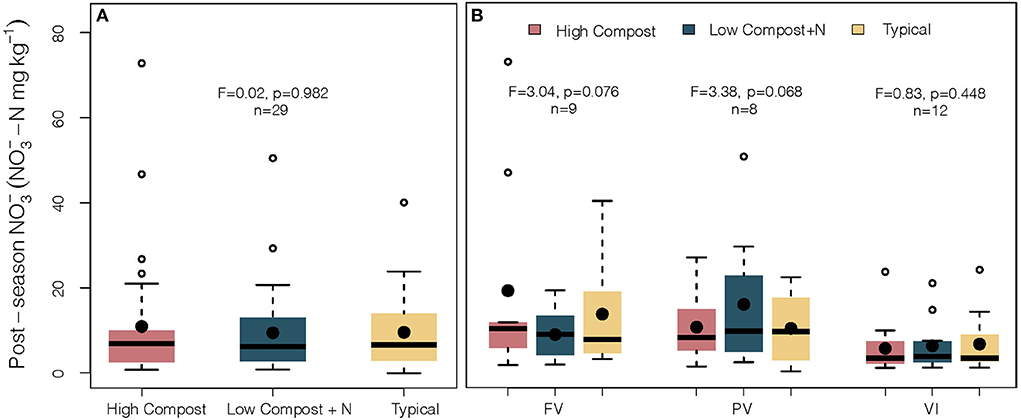
Figure 2. Boxplot of post-season -N (mg kg−1) (0–15 cm). (A) By nutrient management strategy, across regions and years, and (B) by nutrient management strategy within three vegetable production regions of British Columbia [lower Fraser Valley (FV), Pemberton Valley (PV), and Vancouver Island (VI)] over years. ANOVA F and p-value refer to main effect of nutrient strategy. Boxplots with different letters represent significant differences among nutrient strategies using Tukey's post-hoc test at p < 0.05. The center line indicates the median, means are shown as black dots, sample size is indicated by n, and the Tukey-style whiskers extend to a maximum of 1.5 the interquartile range.
The overall trend in post-season available P matched the order of average total P applied with each nutrient strategy, of High Compost > Typical > Low Compost + N (Supplementary Table 2). However, only High Compost and Low Compost + N were significantly different (Figure 3). Permanganate oxidizable C varied widely among farms, and ranged from 248 mg kg soil−1 on a farm transitioning from conventional to organic management to 3,042 mg kg soil−1 on an urban farm with high C inputs. Mean POXC across all treatments, regions, and years was 1,102 mg kg soil−1; POXC was not different among nutrient management strategies.
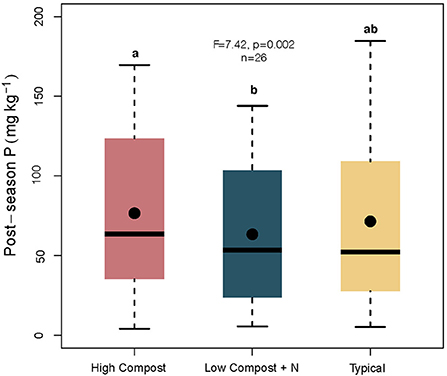
Figure 3. Boxplot of post-season available P (mg kg−1) by nutrient strategy, across years and three vegetable production regions of British Columbia. ANOVA F and p-values refer to main effect of nutrient strategy. Boxplots with different letters represent significant differences among treatments using Tukey's post-hoc test at p < 0.05. The center line indicates the median, means are shown as black dots, sample size is indicated by n, and the Tukey-style whiskers extend to a maximum of 1.5 the interquartile range.
Crop yield
Overall, crop yields did not show consistent differences among nutrient strategies, but a region- and year-specific response was observed (nutrient strategy × region × year interaction; p = 0.044). In the lower Fraser Valley (p = 0.031), yields were higher with High Compost than Typical in 2019 (p = 0.033) but not in 2018 (nutrient strategy × year interaction, p < 0.001; Figure 4).
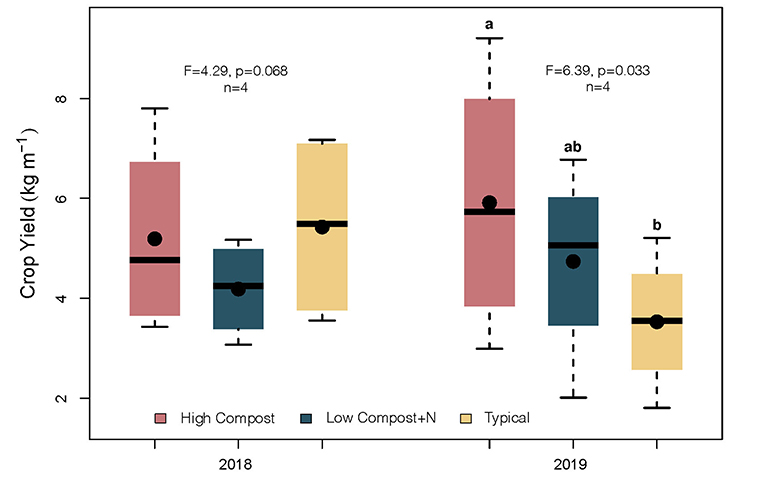
Figure 4. Boxplot of yield (kg m−1) in the lower Fraser Valley of British Columbia by nutrient management strategies within each year of the study. ANOVA F and p-values refer to main effect of nutrient strategy within year. Boxplots with different letters represent significant differences among treatments using Tukey's post-hoc test at p < 0.05. The center line indicates the median, means are shown as black dots, sample size is indicated by n, and the Tukey-style whiskers extend to a maximum of 1.5 the interquartile range.
Input costs
The cost of amendments as determined within the scope of this study was highly variable, especially for Typical, which ranged from $0 with no nutrient application, to $34,977 ha−1 with an expensive compost. Overall, the mean cost of inputs (per nutrient strategy) was $4,959 ha−1. Although we did not find consistent differences in input costs among the nutrient strategies, a region-specific response was found (nutrient strategy × region interaction, p = 0.002). In the lower Fraser Valley, Typical had lower input costs than both High Compost and Low Compost + N (Figure 5).
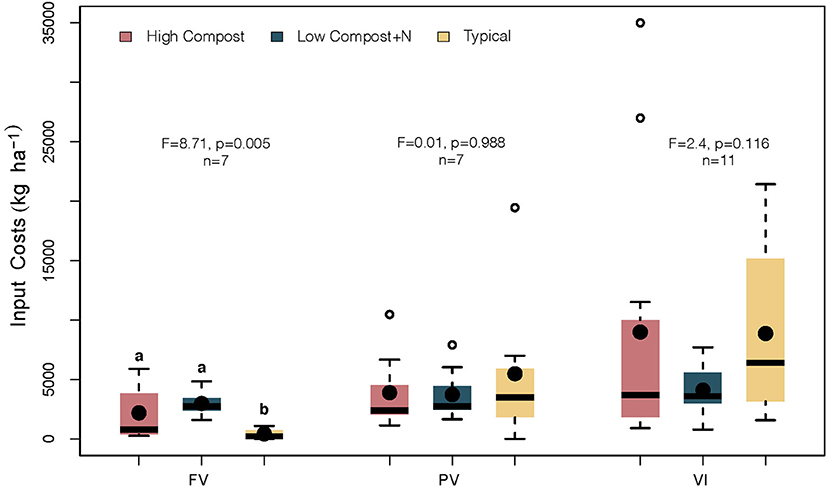
Figure 5. Boxplot of input costs ($ ha−1) by nutrient management strategy across years within three vegetable production regions of British Columbia [lower Fraser Valley (FV), Pemberton Valley (PV), and Vancouver Island (VI)]. ANOVA F and p-values refer to main effect of nutrient strategy within each region. Boxplots with different letters represent significant differences between nutrient strategies using Tukey's post-hoc test at p < 0.05. The center line indicates the median, means are shown as black dots, sample size is indicated by n, and the Tukey-style whiskers extend to a maximum of 1.5 the interquartile range.
Principal component analysis
Covariation in nutrient strategy outcomes and baseline farm site soil and compost properties were well described by the first and second principal component axes (~50% of total covariation), with PC1 and PC2 explaining 28 and 19% of total variation, respectively (Figure 6). Nutrient strategy outcomes differentiated on the first two PCA dimensions, and region had a significant effect on observed covariation described by both PC1 (p = 0.010) and PC2 (p = 0.047). Pre-season soil P, compost total N and P contents, compost C:N, and relative post-season in Low Compost + N and High Compost plots were significantly correlated with PC1 (Supplementary Table 10). On this first axis, farms with higher pre-season soil P that were using composts with higher N and P contents, coordinated with higher relative post-season in High Compost plots characterized by higher PC1 scores, which tended to be on farms in the lower Fraser Valley. In contrast, relative post-season with Low Compost + N coordinated negatively with PC1 and characterized farms with lower pre-season soil P and that used composts with higher C:N; farms on Vancouver Island tended to have lower PC1 axis scores. The second axis described variation in soil C and compost C:N, along with relative post-season in Low Compost + N and Typical, and relative crop yields in High Compost and Typical (Supplementary Table 10). On PC2, relative yields in High Compost tended to be higher on farm sites that had low soil C but also used composts with lower C:N. Relative yields in Typical were higher under the opposite conditions but with a concomitant environmental trade-off of relatively higher post-season . This nutrient strategy-specific relationship between yield and post-season was also found when yield was used as a predictor variable of post-season in the linear mixed effects model to explicitly evaluate for a key production-environment trade-off (nutrient strategy × yield interaction, p = 0.002). Increasing yields were significantly associated with increasing post-season with Typical nutrient strategy, and the opposite was observed with High Compost (Supplementary Tables 11, 12).
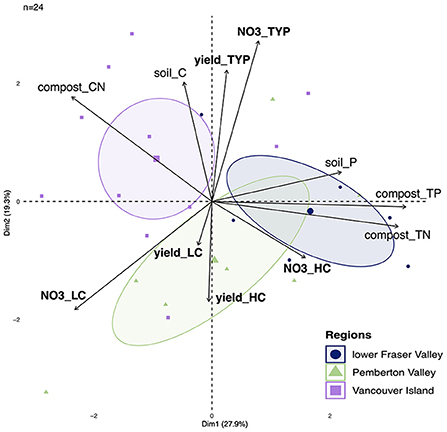
Figure 6. Principal Component Analysis (PCA) biplot of relative post-season (0–30 cm) and relative crop yield following each nutrient management strategy (Typical (TYP), Low Compost + N (LC), and High Compost (HC)). Outcomes by nutrient strategy are plotted with pre-season compost and soil properties including compost total N (compost_TN), compost total P (compost_TP), compost C to N ratio (compost_CN), and pre-season soil total organic C (soil_C), pre-season soil P (soil_P). Pre-season soil samples are averages for the three research plots at each farm. Variables are grouped by each of three vegetable production regions of British Columbia (lower Fraser Valley, Pemberton Valley, and Vancouver Island). The large data points are mean values for each region and the ellipses are 95% confidence intervals. The sample size for each measured outcome within each nutrient strategy is indicated by n.
Discussion
Effects of nutrient strategies on measured outcomes
Identifying nutrient management strategies based on organic amendments that can optimize yields, balance nutrient budgets, and supply C to maintain SOM are fundamental to meeting both economic and environmental goals. However, meeting crop nutrient requirements on mixed vegetable farms can be particularly challenging. Organic systems tend to be N limited, and organic farms are more likely to maximize yields in crop types with greater N use efficiency, such as legumes and perennials (Seufert et al., 2012). Our results of the short-term impacts of three organic nutrient strategies place Low Compost + N as being most likely to meet both environmental and productivity goals of organic vegetable production.
Variable impacts on soil properties
A major difference among nutrient strategies was post-season soil P, which was 21% higher with High Compost than Low Compost + N. On average, High Compost provided over 8× more P than the amount of crop P removal (harvest; Supplementary Table 2). Other studies have also found that large compost applications at ~5× crop P removal (similar to High Compost in this study) and high manure applications at 4× crop P removal, increased soil P in comparison to smaller compost applications (Evanylo et al., 2008; Maltais-Landry et al., 2019). In contrast, Mkhabela and Warman (2005) did not find differences in available P (Mehlich-3 extractable) among corn and potatoe plots receiving 1 or 2 years of compost at 1×, 2×, and 3× crop P removal (much lower than High Compost in this study) in Wisconsin. There was often large quantities of P applied with Typical amendment applications (Supplementary Table 2), which would likely increase soil P on many of the farms in our study if these practices are ongoing. Therefore, switching from Typical to a Low Compost +N strategy would avoid environmental risks of excess soil P.
We did not observe differences in post-season available among nutrient strategies which suggests similar nutrient availability and uptake in crops (with similar yields), regardless of amendment type and/or combination. Post-season leaching has been strongly linked to the quantity of N applied (Drinkwater and Snapp, 2007), and in our study, mean total N applied to High Compost, Typical, and Low Compost + N was 474, 372, and 153 kg N ha−1, respectively. Thus, the very high total N inputs in High Compost could mineralize more than expected and/or asynchronously with crop N uptake, thus increasing environmental risk. However, low levels of post-season in our study (mean = 9.1 mg -N kg−1 soil at 0–30 cm depth) suggests these nutrient strategies were not systematically over-applying N; although there were differences in post-season among the nutrient strategies, values were also generally low. We did, however, find post-season levels in several plots to be greater than the provincial threshold for environmental protection (25 mg kg−1 soil (~ 100 kg -N ha−1); Government of British Columbia, 2019); values above this can require follow-up soil testing and nutrient management planning (depending on location and type of farm). There were four plots (two from High Compost and one each from Low Compost + N and Typical) with post-season above this threshold. These make up only 5% of all plots in our study, yet demonstrate that organic agriculture is not inherently without environmental impacts (Tuomisto et al., 2012). Variable soil properties, precipitation, and sampling times between harvest and post-season sampling (0–2 months) across the different farms in our study likely contributes to some inaccuracy in characterizing post-harvest as a site's potential for N leaching. Future work is needed to develop more systematic methodology of assessing post-harvest and linking with potential for N leaching on diverse organic farms.
The large variability we observed in POXC was mainly attributed to the overall management context of the farm and not the nutrient strategies we tested, and is comparable to similar farming systems elsewhere. POXC ranged from 661 to 1,070 mg kg−1 (measured on 5 g, 2 mm-sieved soil) and from 154 to 983 mg kg−1 (measured on 2.5 g, 2-mm sieved soil) on organic vegetable farms in southwestern Ontario, Canada (Hargreaves et al., 2019) and in New York, USA (Culman et al., 2012; unpublished data cited by Culman et al., 2012), respectively. Despite being regarded as a management-sensitive indicator, POXC did not register the 1 and 2 years of substantially different C inputs among nutrient strategies in our study (see Supplementary Table 2). This potentially reflects the need to perform POXC analysis based on a fixed SOC mass rather than a fixed soil mass (Pulleman et al., 2021) or with an increased number of replicates (Wade et al., 2020) in order to increase the sensitivity, and therefore usefulness, of this indicator.
Economics: Balancing input costs and yields
As expected, yields did not consistently differ by nutrient strategy given they were all designed to meet or exceed crop N and P removal. Similarly, a 3-year study in Virginia, USA found no yield differences in vegetables (pumpkin, bell pepper, and corn) grown using a high compost application (targeting crop N requirements) or a low compost application plus a (conventional) N fertilizer, reportedly due to soil nutrient reserves and adequate nutrient supply from amendments (Evanylo et al., 2008). The only exception where we found yield differences was in the Fraser Valley in the second year of the study, where yields were greater in High Compost than in Typical. It is possible that these yield differences were observed in the lower Fraser Valley, but not in the other two regions, because farms in our study in the lower Fraser Valley are more similar to each other (have more similar farm site characteristics, including high soil P and composts with high N and P content; data not shown), whereas farm site characteristics were more varied amongst farms in the other two regions.
Further, yield differences in the lower Fraser Valley could be due to differences in PAN applications between the treatments. In the Fraser Valley in study year two, there was less estimated PAN applied to Typical than was applied to High Compost on three farms (103 vs. 115, 42 vs. 87, and 0 vs. 46 kg PAN ha−1 applied to Typical vs. High Compost, respectively), whereas on one farm, substantially more PAN was applied to Typical than to High Compost (530 vs. 97 kg PAN ha−1, applied to Typical vs. High Compost, respectively). Similarly, Evanylo et al. (2008) found lower corn yields with smaller compost applications (supplying 20% of crop PAN requirements) compared to larger compost or poultry litter applications (supplying 100% of crop PAN requirements); the same study found a positive correlation between soil and corn earleaf N. Additionally, reduced potato yields from over application of N fertilizer was found by Reiter et al. (2012) when comparing four N fertilizer rates (0, 67, 134, 201, and 268 kg N ha−1) in potato production, where the middle rate (134 kg N ha−1) produced the highest yields. This suggests that amendment application rates based on site-specific but simple nutrient budgets can help prevent under- or over-fertilization and optimize yields. Given that crops on organic farms can be N-limited due to issues with timing, rather than total amounts of N mineralized and available to plants (Berry et al., 2003), additional tools and indicators tailored to ecological nutrient management could build on nutrient budgets to further enhance nutrient use efficiency in these systems (Drinkwater and Snapp, 2007; Bowles et al., 2015).
Although we had expected that meeting crop N requirements with specialty organic fertilizers would cost more than with composts or manures, this was not the case, and instead we found a surprising amount of variation in amendment costs both within and across the regions. The widely varied geography of BC plays an important role with input cost differences across island, mountain, and river valley regions that characterize the agricultural landscapes here. Off-farm and out-of-region fertilizers and composts are subject to additional transportation and distribution costs for Vancouver Island or Pemberton Valley farmers who are separated from the concentration of agricultural suppliers in the lower Fraser Valley. Farmers in the lower Fraser Valley are clearly choosing the most economical nutrient strategy, where costs of Typical were less than both High Compost and Low Compost + N. However, yields were greater in High Compost vs. Typical, which represents a context-specific trade-off in farmers' current nutrient strategies, in terms of input costs and yield gains. Overall, Low Compost + N had the least variability among regions, given that it is less dependent on the highly variable cost of compost.
The costs we estimated in this study were much higher than the $700 ha−1 reported for pelletized poultry manure and pig manure for potatoes in Truro, Nova Scotia, Canada (Lynch et al., 2008), although High Compost and Typical were <$800 ha−1 when using inexpensive poultry manure-based amendments in the lower Fraser Valley. Our estimates were more similar to organic nutrient inputs for vegetable production in California (~$1,561 and $2,247 ha−1 for broccoli and lettuce, respectively; assuming $1 USD ~ $1 CAD in 2011) (Klonsky, 2012). Overall, our observations highlight the range in fertility costs for organic farms in southwest BC, which largely depend on the regional availability of composts, manures, and specialty organic fertilizers.
Covariation of nutrient strategy performance and farm site characteristics
While we did not find overall differences in post-season , and only minor differences in crop yield among nutrient strategies, there was differentiation between these outcomes when analyzed with farm site characteristics in PCA. Our data show a divergence in post-season between High Compost and Low Compost + N in relation to the nutrient content in composts used and pre-season soil P, highlighting the complex nature of amendment-soil interactions on organic farms. Farm sites using high nutrient composts tended to have high post-season when large quantities of compost were used (High Compost). A meta-analysis by Norris and Congreves (2018), also found that C-based amendments high in N (such as poultry manure) increased risk for leaching. In the lower Fraser Valley, Sullivan and Poon (2012) similarly found more than 2× higher post-season in manured vegetable fields compared to fields that did not receive manure.
Differentiation of yields amongst nutrient strategies was unrelated to coordinated variation in initial soil P levels and the N and P content of composts used at an individual farm site. Notably, high yields with Typical were associated with high post-season ; this relationship was also significant when yield was used as a predictor variable of post-season in the mixed effects model, and follows observations of nutrient saturation reported in intensive annual crop production (Drinkwater and Snapp, 2007). Additionally, these outcomes from Typical did not characterize a particular region but did covary with increased soil C and higher compost C:N. This could reflect an over-application of N fertilizers by farmers aiming to avoid N immobilization with high C:N amendments. Indeed other studies have found that N fertilizers increase vegetable yields when farmers rely on composts with high C:N (e.g., Mkhabela and Warman, 2005; Evanylo et al., 2008), although we did not observe this in our study.
Balancing trade-offs of nutrient management strategies
To illustrate trade-offs among nutrient strategies and across regions, outcomes (yield, input costs, POXC, post-season , post-season P) were plotted in radargraphs (fsmb package; Nakazawa, 2019) and axis limits were set to the highest value among the three nutrient strategies (Figure 7). There were limited and region-specific trade-offs among nutrient management strategies, whereby improvements in the outcomes of one or more productivity and/or environmental metric co-occurred with detrimental or more negative results in other outcomes. Across regions and years, nutrient strategies did not have significant differences in yields, POXC, post-season , or input costs, but High Compost did have higher post-season P than Low Compost + N.
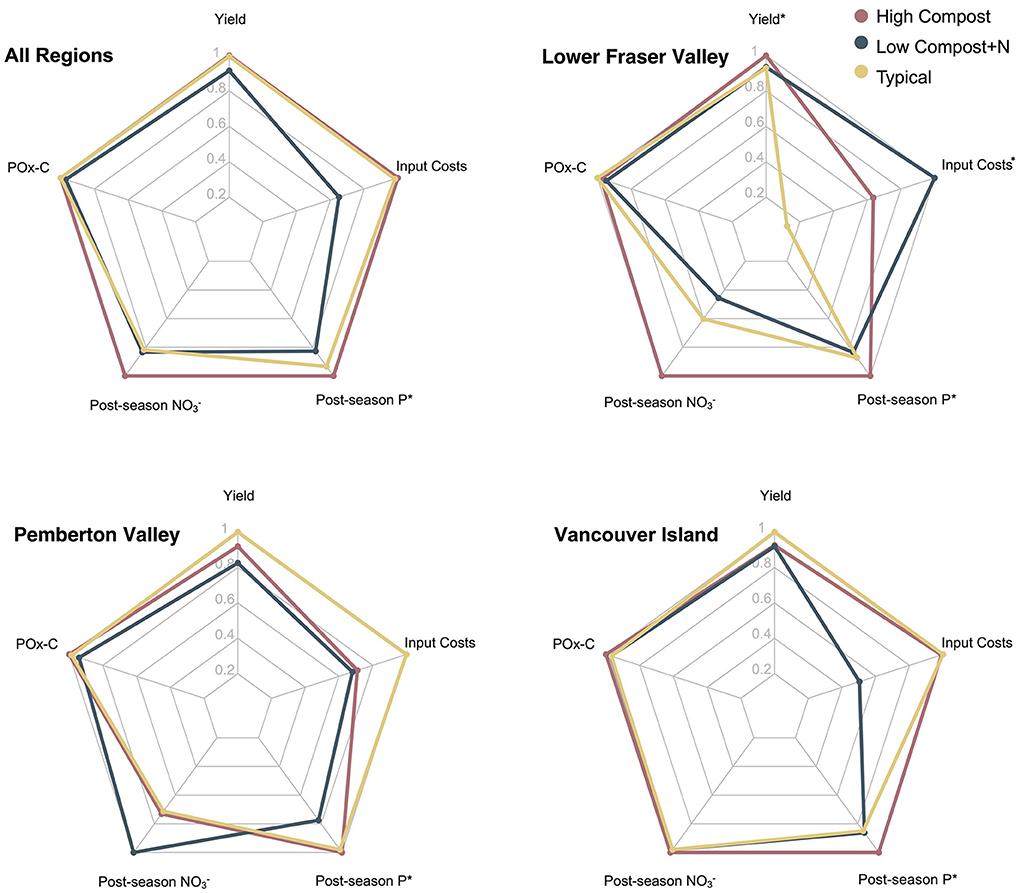
Figure 7. Measured outcomes scaled to the maximum value observed among nutrient strategies, expressed as the mean within each nutrient strategy, averaged across all farms (All Regions) and across all farms in each of three vegetable production regions in British Columbia. Measured outcomes include: yield, input costs, post-season available P (0–15 cm), post-season (0–30 cm), and permanganate oxidizable carbon (POXC). Measured outcomes with significant differences at alpha <0.05 between nutrient strategies are indicated with an asterisk (*).
There were region-specific differences in nutrient strategy performance in the Fraser Valley, with trade-offs in crop yields, costs, and potential environmental impacts. In this region, input costs with Typical were lower than High Compost and Low Compost + N, but High Compost had greater yields than Typical in 1 year in this region, but also greater post-season available P than Low Compost + N. High Compost in this region has a potential trade-off between yield and environmental impacts, yet recommending Low Compost +N over Typical to reduce potential environmental impacts could increase costs for some farmers.
Implications for farm management
Results of this study underscore the contexts where different organic nutrient management strategies can best perform in achieving sustainable agricultural development, as well as the key challenges that farmers face in doing so. In the lower Fraser Valley, High Compost had higher yields than Typical but the PCA also suggests that using High Compost on farms with high soil P and high-N composts (as found in the Fraser Valley) can increase risk for high post-season . Overall, Low Compost + N did not appear to have environmental trade-offs (i.e., high post-season N or P), however, using Low Compost + N will require alternative C inputs to maintain SOC. Cover crops could provide C inputs and potentially capture post-season and provide biological N fixation (from legumes), but will incur additional costs and management complexity. Cover crops are challenging for various reasons, including short shoulder seasons, high land prices, increased management complexity, and grazing by overwinter waterfowl in the lower Fraser Valley (Merkens et al., 2012). Combining cover crops with reduced compost applications is an important area for further research.
As environmental costs are not directly paid for by the farmer, but reduced yield and increased input costs are, it is difficult to reason that farmers using composts as affordable sources of C and N (as in the lower Fraser Valley) should change their practices to decrease soil P and post-season available N from a purely (farm-level) economic standpoint. Farms likely need incentives (e.g., economic rewards, technical support) to balance farm N, P, and C budgets using high-N specialty fertilizers and/or more intensive cover crop use and reduced compost applications. At a global level, policymakers are introducing nutrient management regulations, such as the “Code of Practice for Agricultural Environmental Management” in BC (Government of British Columbia, 2020), the “Vermont Pay-For-Phosphorus Program” (State of Vermont, 2021) in the USA, and various approaches in countries surrounding the Baltic Sea in Europe (Svanbäck et al., 2019).
Farms in regions without easy access to inexpensive, high nutrient composts (e.g., Pemberton Valley and Vancouver Island) may have more economic incentive (without policy interventions) to employ lower compost application rates. These farms can combine cover crops, fertilizers, and compost, depending on how their viability, costs, and availability, respectively fluctuate from season to season. Given that the yield differences we found in the lower Fraser Valley could be due to differences in N management, all farms would benefit from basic annual PAN budgeting to avoid excessive N deficits or surpluses in each season. In contrast, P budgets have greater annual flexibility, where farms with low soil P can over-apply P and farms with high soil P can under-apply P in the short term. In the long term, the Low Compost + N strategy is favorable because it sets the farm field P balance at zero.
Ecological nutrient management can contribute to achieving Sustainable Development Goals (SDG) 2.3 and 2.4 [particularly as these SDGs are clarified by Gil et al. (2019)], which call for advancing both farm productivity and sustainability. However, our study highlights the importance of assessing management practices with multiple, and often competing, end-results, and the need for region- and farm-specific management decisions that can be flexible to system-specific input and soil properties. This study contributes to the emerging literature aimed at policy-makers who are concerned with improved understandings of the contexts where organic agriculture can best perform in terms of meeting sustainable agricultural development goals (Seufert, 2012; Ramankutty et al., 2019). Efforts to optimize farm- or field-level nutrient budgets and build SOC should additionally consider socio-economic factors governing landscape-scale nutrient flows which influence on-farm management practices (e.g., cost and availability of nutrient inputs).
Conclusions
Nutrient management strategies must be evaluated for potential trade-offs that can depend on regional nutrient availability to ensure productivity does not compromise sustainability goals. There were inconsistent trade-offs among the three nutrient strategies compared on 20 working farms across three distinct regions. The typical nutrient management approach used by each of the farmers varied widely, which contributed to challenges in identifying systematic differences between these typical nutrient combinations and our two standardized nutrient strategies. Regardless of regional differences in soils and amendments, post-season P was significantly lower when compost was applied to meet crop P removal instead of crop N removal. Our results show that a nutrient management strategy which combines reduced compost with organic N fertilizer is most likely to meet both environmental and productivity goals. However, long-term research on the impacts to, and strategies to maintain, SOC is required. Given that economics is the key driver for farmer decision making, future research should also include a more substantial economic analysis to thoroughly capture costs and benefits including labor, crop quality, and cover crops.
Data availability statement
The raw data supporting the conclusions of this article will be made available by the authors, without undue reservation.
Author contributions
AN project lead, conducted the experiment, completed data analysis, and wrote the manuscript. DL project concept, technical guidance on experimental setup and data collection, and manuscript revision. KB technical guidance on data collection, analyses, interpretation, and manuscript revision. MK and JC conceptual guidance and manuscript revision. SS project concept, project design, acquired funding, data interpretation, and manuscript revision. All authors contributed to the article and approved the submitted version.
Funding
This research was supported by funding from the Organic Science Cluster 3 under the AgriScience program of Agriculture and Agri-Food Canada and Mitacs Career Connect.
Acknowledgments
The authors thank members of the Sustainable Agricultural Landscapes lab for support in sample collection and analysis, including Hannah Friesen, Conley Keyes, Carson Li, Jessica Mayes, Katie Neufeld, Paula Porto, and Carmen Wong. The authors also wish to thank all of the farmers involved for their active participation and contributions of time, knowledge, and resources. The content of this manuscript has previously appeared online in a thesis.
Conflict of interest
The authors declare that the research was conducted in the absence of any commercial or financial relationships that could be construed as a potential conflict of interest.
Publisher's note
All claims expressed in this article are solely those of the authors and do not necessarily represent those of their affiliated organizations, or those of the publisher, the editors and the reviewers. Any product that may be evaluated in this article, or claim that may be made by its manufacturer, is not guaranteed or endorsed by the publisher.
Supplementary material
The Supplementary Material for this article can be found online at: https://www.frontiersin.org/articles/10.3389/fsufs.2022.706271/full#supplementary-material
References
Berry, P. M., Stockdale, E. A., Sylvester-Bradley, R., Philipps, L., Smith, K. A., Lord, E. I., et al. (2003). N, P and K budgets for crop rotations on nine organic farms in the UK. Soil Use Manag. 19:112–18. doi: 10.1079/sum2003176
Berry, P. M., Sylvester-Bradley, R., Philipps, L., Hatch, D. J., Cuttle, S. P., Rayns, F. W., et al. (2002). Is the productivity of organic farms restricted by the supply of available nitrogen? Soil Use Manag. 18, 248–255. doi: 10.1079/SUM2002129
Bowles, T. M., Hollander, A. D., Steenwerth, K., and Jackson, L. E. (2015). Tightly-coupled plant-soil nitrogen cycling: comparison of organic farms across an agricultural landscape. PLoS ONE 10, 1–25. doi: 10.1371/journal.pone.0131888
Bünemann, E. K., Bongiorno, G., Bai, Z., Creamer, R. E., De Deyn, G., de Goede, R., et al. (2018). Soil quality: a critical review. Soil Biol. Biochem. 120, 105–125. doi: 10.1016/j.soilbio.2018.01.030
Carr, P. M., Cavigelli, M. A., Darby, H., Delate, K., Eberly, J. O., Gramig, G. G., et al. (2019). Nutrient cycling in organic field crops in canada and the united states. Agron. J. 111, 2769–2785. doi: 10.2134/agronj2019.04.0275
Coe, R. I. C., Njoloma, J., and Sinclair, F. (2019). To control or not to contorl: How do we learn more about how agronomic innovations perform on farms? Exp. Agric. 55, 303–309. doi: 10.1017/S0014479717000102
Culman, S. W., Snapp, S. S., Freeman, M. A., Schipanski, M. E., Beniston, J., Lal, R., et al. (2012). Permanganate oxidizable carbon reflects a processed soil fraction that is sensitive to management. Soil Sci. Soc. Am. J. 76, 494–504. doi: 10.2136/sssaj2011.0286
de Ponti, T., Rijk, B., and van Ittersum, M. K. (2012). The crop yield gap between organic and conventional agriculture. Agric. Syst. 108, 1–9. doi: 10.1016/j.agsy.2011.12.004
Doane, T. A., and Horwáth, W. R. (2003). Spectrophotometric determination of nitrate with a single reagent. Anal. Lett. 36, 2713–2722. doi: 10.1081/AL-120024647
Drinkwater, L. E., and Snapp, S. S. (2007). Nutrients in agroecosystems: rethinking the management paradigm. Adv. Agron. 92, 163–186. doi: 10.1016/S0065-2113(04)92003-2
Eghball, B. (2002). Soil properties as influenced by phosphorus- and nitrogen-based manure and compost applications. Agron. J. 94, 128. doi: 10.2134/agronj2002.0128
Evanylo, G., Sherony, C., Spargo, J., Starner, D., Brosius, M., and Haering, K. (2008). Soil and water environmental effects of fertilizer-, manure-, and compost-based fertility practices in an organic vegetable cropping system. Agric. Ecosyst. Environ. 127, 50–58. doi: 10.1016/j.agee.2008.02.014
Gale, E. S., Sullivan, D. M., Cogger, C. G., Bary, A. I., Hemphill, D. D., and Myhre, E. A. (2006). Estimating plant-available nitrogen release from manures, composts, and specialty products. J. Environ. Qual. 35, 2321. doi: 10.2134/jeq2006.0062
Gil, J. D. B., Reidsma, P., Giller, K., Todman, L., Whitmore, A., and van Ittersum, M. (2019). Sustainable development goal 2: improved targets and indicators for agriculture and food security. Ambio 48, 685–698. doi: 10.1007/s13280-018-1101-4
Gomiero, T., Pimentel, D., and Paoletti, M. G. (2011). Environmental impact of different agricultural management practices: conventional vs. organic agriculture. CRC Crit. Rev. Plant Sci. 30, 95–124. doi: 10.1080/07352689.2011.554355
Government of British Columbia (2017). Agriculture in Brief. Available online at: https://www2.gov.bc.ca/assets/gov/farming-natural-resources-and-industry/agriculture-and-seafood/statistics/census/census-2016/aginbrief_2016_all_province_region_regional_districts.pdf (accessed March 25, 2020).
Government of British Columbia (2019). Post-Harvest Nitrate Testing. Available online at: https://www2.gov.bc.ca/gov/content/industry/agriculture-seafood/agricultural-land-and-environment/soil-nutrients/nutrient-management/what-to-apply/soil-nutrient-testing/post-harvest-nitrate-testing#SoilSampling (accessed April 20, 2020).
Government of British Columbia (2020). Agricultural Environmental Management. Available online at: https://www2.gov.bc.ca/gov/content/environment/waste-management/industrial-waste/agriculture (accessed April 2, 2020).
Government of British Columbia. (2018). BC Soil Information Finder Tool. Available online at: https://www2.gov.bc.ca/gov/content/environment/air-land-water/land/soil/soil-information-finder
Government of Canada. (2019). Past Weather and Climate – Historical Data. Available online at: https://climate.weather.gc.ca/historical_data/search_historic_data_e.html
Gregorich, E. G., Carter, M. R., Angers, D. A., Monreal, C. M., and Ellert, B. H. (1994). Towards a minimum data set to assess soil organic matter quality in agricultural soils. Can. J. Soil Sci. 74, 367–385.
Hargreaves, S. K., Dejong, P., Laing, K., McQuail, T., and Van Eerd, L. L. (2019). Management sensitivity, repeatability, and consistency of interpretation of soil health indicators on organic farms in southwestern Ontario. Can. J. Soil Sci. 99, 508–519. doi: 10.1139/cjss-2019-0062
Hendershot, W. H., Lalande, H., and Duquette, M. (2008a). “Electrical conductivity and exchangeable acidity,” in Soil Sampling and Methods of Analysis, eds. M. R. Carter, and E. G. Gregorich (Saskatoon: Canadian Society of Soil Science), 161–172. doi: 10.1201/9781420005271.ch16
Hendershot, W. H., Lalande, H., and Duquette, M. (2008b). “Soil Reaction and Exchangeable Acidity,” in Soil Sampling and Methods of Analysis, eds. M. Carter and E. G. Gregorich (Saskatoon: Canadian Society of Soil Science), 173–178.
Husson, F., Josse, J., Le, S., and Mazet, J. (2020). Package “FactoMineR”. Available online at: http://factominer.free.fr (accessed September 24, 2019).
Jerkins, D., and Ory, J. (2016). National Organic Research Agenda. Santa Cruz, CA: Organic Farming Research Foundation. Available online at: https://ofrf.org/wp-content/uploads/2019/09/NORA_2016_final9_28.pdf (accessed January 17, 2019).
Karam, A. (2008). “Total element analysis (elements other than nitrogen, carbon, oxygen, and hydrogen),” in Soil Sampling and Methods of Analysis, eds. M. R. Carter, and E. G. Gregorich (Saskatoon: Canadian Society of Soil Science), 335–340.
Klonsky, K. (2012). Comparison of production costs and resource use for organic and conventional production systems. Am. J. Agric. Econ. 94, 314–321. doi: 10.1093/ajae/aar102
Kroetsch, D., and Wang, C. (2008). “Partizle size distribution,” in Soil Sampling and Methods of Analysis, eds. M. R. Carter, and E. G. Gregorich (Boca Raton: Taylor and Francis Group), 713–725. doi: 10.1201/9781420005271.ch55
Krzywinski, M., and Altman, N. (2014). Points of significance: analysis of variance and blocking. Nat. Methods 11, 699–700. doi: 10.1038/nmeth.3005
Krzywinski, M., Altman, N., and Blainey, P. (2014). Points of significance: nested designs. Nat. Methods 11, 977–978. doi: 10.1038/nmeth.3137
Lenth, R. (2019). emmeans: Estimated Marginal Means, Aka Least-Squares Means. Available online at: https://cran.r-project.org/web/packages/emmeans/index.html
Liptzin, D., Norris, C. E., Cappellazzi, S. B., Mac Bean, G., Cope, M., Greub, K. L. H., et al. (2022). An evaluation of carbon indicators of soil health in long-term agricultural experiments. Soil Biol. Biochem. 172, 108708. doi: 10.1016/j.soilbio.2022.108708
Lynch, D. H., Zheng, Z., Zebarth, B. J., and Martin, R. C. (2008). Organic amendment effects on tuber yield, plant N uptake and soil mineral N under organic potato production. Renew. Agric. Food Syst. 23, 250–259. doi: 10.1017/S1742170508002330
Maltais-Landry, G., Nesic, Z., Grant, N., Godinez, M., Thompson, B., Hsu, L. Y., et al. (2019). Quantifying trade-offs among on-farm and off-farm fertility sources to make vegetable organic farming systems more sustainable. Agric. Ecosyst. Environ. 286, 106657. doi: 10.1016/j.agee.2019.106657
Maltais-Landry, G., Scow, K., Brennan, E., Torbert, E., and Vitousek, P. (2016). Higher flexibility in input N:P ratios results in more balanced phosphorus budgets in two long-term experimental agroecosystems. Agric. Ecosyst. Environ. 223, 197–210. doi: 10.1016/j.agee.2016.03.007
Marasteanu, J. I., and Jaenicke, E. C. (2019). Economic impact of organic agriculture hotspots in the United States. Renew. Agric. Food Syst. 34, 501–522. doi: 10.1017/S1742170518000066
Maynard, D. G., Kalra, Y. P., and Crumbaugh, J. A. (2008). “Nitrate and exchangeable ammonium nitrogen,” in Soil Sampling and Methods of Analysis, eds. M. R. Carter, and E. G. Gregorich (Saskatoon: Canadian Society of Soil Science), 71–80.
Merkens, M., Bradbeer, D. R., and Bishop, C. A. (2012). Landscape and field characteristics affecting winter waterfowl grazing damage to agricultural perennial forage crops on the lower Fraser River delta, BC, Canada. Crop Prot. 37, 51–58. doi: 10.1016/j.cropro.2012.02.014
Mkhabela, M. S., and Warman, P. R. (2005). The influence of municipal solid waste compost on yield, soil phosphorus availability and uptake by two vegetable crops grown in a Pugwash sandy loam soil in Nova Scotia. Agric. Ecosyst. Environ. 106, 57–67. doi: 10.1016/j.agee.2004.07.014
Nakagawa, S., and Schielzeth, H. (2013). A general and simple method for obtaining R2 from generalized linear mixed-effects models. Methods Ecol. Evol. 4, 133–142. doi: 10.1111/j.2041-210x.2012.00261.x (accessed February 27, 2020).
Nakazawa, M. (2019). Package “fmsb.” Available online at: http://minato.sip21c.org/msb/
Nelson, N. O., and Janke, R. R. (2007). Phosphorus sources and management in organic production systems. Horttechnology 17, 442–454. doi: 10.21273/HORTTECH.17.4.442
Norris, C. E., and Congreves, K. A. (2018). Alternative management practices improve soil health indices in intensive vegetable cropping systems: a review. Front. Environ. Sci. 6, 50. doi: 10.3389/fenvs.2018.00050
Pinheiro, J., Bates, D., DebRoy, S., Sarkar, D., and R Core Team (2019). _nlme: Linear and Nonlinear Mixed Effects Models_R package version 3.1-152. Available online at: https://CRAN.R-project.org/package=nlme (accessed October 12, 2019).
Pulleman, M., Wills, S., Creamer, R., Dick, R., Ferguson, R., Hooper, D., et al. (2021). Soil mass and grind size used for sample homogenization strongly affect permanganate-oxidizable carbon (POXC) values, with implications for its use as a national soil health indicator. Geoderma 383, 114742. doi: 10.1016/j.geoderma.2020.114742
R Core Team (2019). R: A Language and Environment for Statistical Computing. Available online at: https://www.r-project.org/ (accessed September 7, 2019).
Ramankutty, N., Ricciardi, V., Mehrabi, Z., and Seufert, V. (2019). Trade-offs in the performance of alternative farming systems. Agric. Econ. 50, 97–105. doi: 10.1111/agec.12534
Reid, K., Schneider, K., and Joosse, P. (2019). Addressing imbalances in phosphorus accumulation in Canadian agricultural soils. J. Environ. Qual. 48, 1156–1166. doi: 10.2134/jeq2019.05.0205
Reiter, M. S., Rideout, S. L., and Freeman, J. H. (2012). Nitrogen fertilizer and growth regulator impacts on tuber deformity, rot, and yield for russet potatoes. Int. J. Agron. 2012, 1–7. doi: 10.1155/2012/348754
Seufert, V. (2012). Research to Practice Policy Briefs Organic Agriculture as an Opportunity for Sustainable Agricultural Development Funded by the Canadian International Development Agency (CIDA): Executive Summary. Institute for the Study of International Development. Available online at: https://www.mcgill.ca/isid/files/isid/seufert.pb13.pdf (accessed December 29, 2021).
Seufert, V., Ramankutty, N., and Foley, J. A. (2012). Comparing the yields of organic and conventional agriculture. Nature 485, 229–232. doi: 10.1038/nature11069
Spargo, J. T., Cavigelli, M. A., Mirsky, S. B., Meisinger, J. J., and Ackroyd, V. J. (2016). Organic supplemental nitrogen sources for field corn production after a hairy vetch cover crop. Agron. J. 108, 1992–2002. doi: 10.2134/agronj2015.0485
State of Vermont (2021). Vermont Pay-Per-Phosphorus Program. Available online at: https://agriculture.vermont.gov/VPFP (accessed May 1, 2021).
Sullivan, C. S., and Poon, D. (2012). Fraser Valley Soil Nutrient Survey 2012. 21. BC Ministry of Agriculture.
Sullivan, D., and Andrews, N. (2017). Predicting plant-available nitrogen provided by organic fertilizers and cover crops using an online calculator. Sci. Pap. Ser. A Agron. 60, 182–187. https://www.cabdirect.org/cabdirect/FullTextPDF/2019/20193017521.pdf
Svanbäck, A., McCrackin, M. L., Swaney, D. P., Linefur, H., Gustafsson, B. G., Howarth, R. W., et al. (2019). Reducing agricultural nutrient surpluses in a large catchment: links to livestock density. Sci. Total Environ. 648, 1549–1559. doi: 10.1016/j.scitotenv.2018.08.194
Thermo Fisher (2010). Flash 2000 Elemental Analyzer Operating Manual. Waltham, MA: Thermo Fisher Scientific Cambridge: Thermo Fisher Scientific.
Tuomisto, H. L., Hodge, I. D., Riordan, P., and Macdonald, D. W. (2012). Does organic farming reduce environmental impacts? A meta-analysis of European research. J. Environ. Manage. 112, 309–320. doi: 10.1016/j.jenvman.2012.08.018
van Lierop, W. (1988). Determination of available phosphorus in acid and calareous soils with the kelowna multi-element extractant. Soil Sci. 146, 284–291.
Vanlauwe, B., Coe, R. I. C., and Giller, K. E. (2019). Beyond averages: new approaches to understand heterogeneity and risk of technology success or failure in smallholder farming. Exp. Agric. 55, 84–106. doi: 10.1017/S0014479716000193
Wade, J., Maltais-Landry, G., Lucas, D. E., Bongiorno, G., Bowles, T. M., Calderón, F. J., et al. (2020). Assessing the sensitivity and repeatability of permanganate oxidizable carbon as a soil health metric: an interlab comparison across soils. Geoderma 366, 114235. doi: 10.1016/j.geoderma.2020.114235
Washington State University (2020). Calculating Compost Bulk Density. Washington State University. Available online at: https://puyallup.wsu.edu/soils/bulkdensity/ (accessed April 5, 2020).
Watson, C. A., Bengtsson, H., Ebbesvik, M., Løes, A.-K., Myrbeck, A., Salomon, E., et al. (2002). A review of farm-scale nutrient budgets for organic farms as a tool for management of soil fertility. Soil Use Manag. 18, 264–273. doi: 10.1079/SUM2002127
Weatherburn, M. W. (1967). Phenol-hypochlorite reaction for determination of ammonia. Anal. Chem. 39, 971–974. doi: 10.1021/ac60252a045
Webster, R., and Lark, R. M. (2018). Analysis of variance in soil research: let the analysis fit the design. Eur. J. Soil Sci. 69, 126–139. doi: 10.1111/ejss.12511
Weil, R. R., Islam, K. R., Stine, M. A., Gruver, J. B., and Samson-Liebig, S. E. (2003). Estimating active carbon for soil quality assessment: a simplified method for laboratory and field use. Am. J. Altern. Agric. 18, 3–17. doi: 10.1079/AJAA2003003
Keywords: organic agriculture, nitrogen, phosphorus, manure, compost, ecological nutrient cycling, organic fertilizer, organic amendments
Citation: Norgaard AE, Lewis D, Borden KA, Krzic M, Carrillo J and Smukler SM (2022) Trade-offs in organic nutrient management strategies across mixed vegetable farms in Southwest British Columbia. Front. Sustain. Food Syst. 6:706271. doi: 10.3389/fsufs.2022.706271
Received: 07 May 2021; Accepted: 14 September 2022;
Published: 06 October 2022.
Edited by:
Steven J. Vanek, Colorado State University, United StatesReviewed by:
Philippe V. Baret, Université Catholique de Louvain, BelgiumGurbir S. Bhullar, Bern University of Applied Sciences, Switzerland
Copyright © 2022 Norgaard, Lewis, Borden, Krzic, Carrillo and Smukler. This is an open-access article distributed under the terms of the Creative Commons Attribution License (CC BY). The use, distribution or reproduction in other forums is permitted, provided the original author(s) and the copyright owner(s) are credited and that the original publication in this journal is cited, in accordance with accepted academic practice. No use, distribution or reproduction is permitted which does not comply with these terms.
*Correspondence: Amy E. Norgaard, YW15bm9yZ2FAYWx1bS51YmMuY2E=