- 1Institute of Bee Health, Vetsuisse Faculty, University of Bern, Bern, Switzerland
- 2Agroscope, Swiss Bee Research Centre, Bern, Switzerland
Gut microbiota are known to foster pollen digestion in honey bee workers, Apis mellifera, thereby enhancing longevity and body weight gain. However, it is currently not known how longevity and body weight gain are effected when gut microbiota are reduced in bees with or without access to pollen. Here, using a hoarding cage set-up with freshly emerged summer workers, we manipulated the gut microbiota of half the bees with the antibiotic tetracycline (ABX), and left the other half untreated on a sucrose solution diet. Afterwards, all bees were assigned to either sucrose diets or sucrose plus ad libitum access to pollen (N = 4 treatments, N = 26 bees/treatment, N = 10 replicates/treatment, N = 1,040 total workers). The data confirm that pollen has a positive effect on longevity and body weight in workers with an unmanipulated gut microbiota. Surprisingly, the antibiotics alone also improved the longevity and body weight of the workers fed a strictly sucrose diet, potentially explained by the reduction of harmful bacteria. However, this positive effect was reversed from an observed antagonistic interaction between pollen and antibiotics, underscoring the innate value of natural microbiota on pollen digestion. In conclusion, a combination of adequate pollen supply and an unmanipulated gut microbiota appears crucial to honey bee worker health, calling for respective efforts to ensure both in managed colonies.
Introduction
The Western honey bee (Apis mellifera) is one of the most important insects for agriculture worldwide due to their pollination services (Garibaldi et al., 2013; Hung et al., 2018). In recent years, increasing numbers of honey bee colony losses throughout the northern hemisphere have been reported (Neumann and Carreck, 2010; Gray et al., 2020), likely as a result of the numerous stressors honey bee colonies are exposed to, including pests, parasites, depreciated food resources, and agrochemicals (e.g., Potts et al., 2010; Barron, 2015). Two specific aspects that have the potential to strengthen honey bees to cope with these challenges are honey bee nutrition (e.g., Dolezal and Toth, 2018; Stanimirović et al., 2019) and a functional worker gut microbiota (e.g., Alberoni et al., 2016; Kwong and Moran, 2016; Bonilla-Rosso and Engel, 2018), both of which have recently received increasing attention. Indeed, adequate nutritional supply plays a key role for A. mellifera health (e.g., Haydak, 1970; Brodschneider and Crailsheim, 2010). Pollen collected by foragers in the environment constitutes the unique source of protein and is essential for the supply of macro- and micro-nutrients (i.e., proteins, lipids, vitamins, and minerals) (e.g., Haydak, 1970; Herbert et al., 1978; Wright et al., 2018) that are indispensable for development, tissue building, and growth of the individuals (Winston, 1991; Brodschneider and Crailsheim, 2010). Accordingly, pollen supply translates into a broad array of beneficial health effects including extended longevity (e.g., Wang et al., 2014) higher body weights (e.g., Retschnig et al., 2021) and immune competence (Alaux et al., 2010) as well as an enhanced ability to cope with pesticides (Barascou et al., 2021). On the other hand, pollen may be a source for pathogens (e.g., Nosema ceranae, viruses, Pereira et al., 2019) and has been shown to favor infection levels for certain pathogens, i.e., Nosema ceranae (Porrini et al., 2011).
Pollen digestion is complex (e.g., Nicolson et al., 2018), and honey bee gut bacteria have been reported to play a major role in the degradation of the complex cell wall polysaccharides including hemicellulose as well as pectin, which constitutes an obligatory requirement for the utilization of the nutrients contained in pollen (Lee et al., 2015, 2018; Zheng et al., 2019). Numerous reports assign beneficial health effects to the simple and distinctive microbial community of eusocial bees (Martinson et al., 2011; Kwong and Moran, 2016). Current evidence suggests that a functional gut microbiota contributes to a broad array of health aspects such as the efficient digestion of plant-based food, related body weight gain due to the availability of required nutrients, physiology, endocrine signaling, tolerance to parasites and pesticides, behaviors, and host immunity systems (Anderson et al., 2011; Koch and Schmid-Hempel, 2011; Vásquez et al., 2012; Engel and Moran, 2013; Schwarz et al., 2016; Ricigliano et al., 2017; Zheng et al., 2017; Dosch et al., 2021). Indeed, disturbance of the gut microbiota has been linked to diseases and malnutrition (e.g., Lozupone et al., 2012), including reduced protein digestive efficiency in honey bees (du Rand et al., 2020).
A wide range of xenobiotics can affect size, composition and functional properties of the honey bee gut microbiota (Daisley et al., 2020), as it has been demonstrated for pesticides (i.e., glyphosate, Motta et al., 2018), airborne particular matters (i.e., titanium dioxide, Papa et al., 2021) and antibiotics (e.g., tetracycline, Raymann et al., 2017). In certain countries, including the United States, antibiotics, mainly tetracycline derivates, are applied in apiculture as a preventive or control measure against two common larval diseases, American and European Foulbrood, caused by Paenibacillus larvae and Melissococcus plutonius, respectively (Genersch, 2010; Tian et al., 2012; Daisley et al., 2020). The accumulation and permanent exposure of honey bees to such broad-spectrum antibiotics affect the composition, diversity and functionality of the exposed honey bee's gut microbiota (e.g., Raymann et al., 2017). Indeed, several laboratory experiments have demonstrated that the antibiotic treatment disrupt the beneficial gut bacteria in the treated honey bees. For instance, a significant reduction of the gut bacteria has been reported when workers were treated with tetracycline (450 μg/ml of tetracycline) for 5 days (Raymann et al., 2017) or up to 9 days (Soares et al., 2021), with 500 μg/ml of the same substance for 3 days (Brown et al., 2022) or for the whole duration of the experiment (21 days, Retschnig et al., 2021). More specifically, antibiotic exposure can reduce the abundance of major gut bacteria such as Lactobacillus spp., Bifidobacterium spp., and Snodgrassella alvi (Raymann et al., 2017), and similarly, decrease genetic diversity on a strain level (i.e., Gilliamella apicola, Raymann et al., 2018). Accordingly, exposure of workers to antibiotics is a common method to inactivate gut microbiota (Zheng et al., 2018). Nevertheless, organismic studies addressing the mutualistic host-microbiota relationships in relation to honey bee nutrition remain scarce, even though the role of gut microbiota in protein digestion has been clarified (Lee et al., 2015; Zheng et al., 2019; du Rand et al., 2020). Potential parameters to evaluate the importance of the supply with certain nutrients on honey bee health include longevity and body weight (e.g., Retschnig et al., 2021). Nutritional reserves are associated with honey bee worker longevity (Mattila and Otis, 2006) and worker body weight has been identified as a predictive marker for longevity (Retschnig et al., 2021).
The goal of the present study was to investigate the potential effects of pollen supply on longevity and body weight of honey bee workers (A. mellifera) in the presence and absence of an unmanipulated gut microbiota. In a fully-crossed laboratory hoarding cage trial, workers were either treated with the antibiotic tetracycline (ABX) to inactivate the gut microbiota or allowed the establishment of a functional microbiota and were then assigned to a diet with or without pollen supply. Due to the known importance of protein supply for body weight and longevity (e.g., Haydak, 1970) and the importance of an unmanipulated gut microbiota (Kwong and Moran, 2016), we would expect an enhanced longevity and higher body weights in bees that had access to pollen and had an unmanipulated gut microbiota.
Materials and Methods
Experimental Setup
The experiment was conducted from July 3rd to September 1st 2019 (local summer) at the Institute of Bee Health in Bern, Switzerland. To obtain defined age cohorts of newly emerged workers, capped worker brood frames pre-checked for final stage pupa (dark-eyed, pigmented cuticle pupae) were chosen from four local A. mellifera colonies (N = 4), brushed clean, and incubated following standardized conditions until adult emergence (48 h, 34.5°C, >60% RH; Williams et al., 2013). After 48 h, newly emerged workers from all colonies were randomly mixed to homogenize the impact of genetics, and placed in 100 ml (100 cm3) clear polystyrol hoarding cages (N = 40, 26 bees/cage, N = total workers 1,040; Williams et al., 2013). According to treatments, half of the workers were treated with the antibiotic (ABX) tetracycline for 72 h to reduce gut microbiota and the other half was fed sucrose solution ad libitum (Brown et al., 2022). Subsequently, cages were assigned to a diet with or without pollen (Table 1). All workers were maintained in an incubator at 30°C and >60% RH (Williams et al., 2013) until the last worker died.
Antibiotic and Dietary Treatments
The antibiotic, tetracycline hydrochloride (Sigma-Aldrich), was identified in a pre-trial screening to be the best suitable to inactivate the gut microbiota (i.e., significant reduction of colony forming units in the plated guts of antibiotic-treated vs. control workers) without significantly affecting longevity. Subsequently, a 50% (w/v) tetracycline-sucrose solution (500 μg/mL tetracycline hydrochloride) was prepared and fed ad libitum to the antibiotic groups (groups 1 and 2) for a time period of 72-h. Sucrose solution (50% w/v), made with sterilized tap water and stored at 4°C until use, was prepared freshly on a weekly basis and fed to all experimental workers ad libitum. Two treatment groups (2 and 4) were additionally fed ad libitum non-sterilized corbicula poly-floral pollen from honey bees harvest from an entire season and mixed (Swiss Pollen, Bienen Roth). The pollen was stored frozen at −24°C, and prior to feeding, was thawed, ground, and supplied to workers via 1.5 mL microcentrifuge tubes with a clipped tip. Syringes and pollen tubes were changed with fresh sterilized ones on a bi-weekly basis.
Parameters
Longevity
Worker mortality was recorded daily until the last worker has died. During the cage checks, dead workers were carefully removed and stored at −80°C.
Body Weight
Individual fresh body weights of three live workers per cage were measured on days 7 and 14 using a Mettler AT 400 scale, precise to 10−4 g (N = 30 per treatment and day, total N = 240).
Food Consumption
The workers from the groups 2 and 4 had permanent ad libitum access to pollen. Real pollen consumption was not quantifiable because the workers removed parts of the pollen paste from the tube and distributed it on the floor of the cage. To estimate consumption of sucrose solution per worker per day, the syringes were weighed daily on a Mettler AT 400 scale, and the differences in weight were divided by the number of live workers present in the cage at that time. To account for mechanic loss (i.e., evaporation from the incubator, dripping from syringes), control syringes were filled with sucrose solution and put in cages without workers, incubated, and measured daily, and the average evaporation rates were used to adjust sucrose-solution consumption across all treatments (OECD, 2017).
Pathogen Infection With Nosema spp.
In a subsample of individual experimental workers (1 worker per cage, 9–10 workers/treatment, N total = 39, workers that were collected on Day 14 for body weight measurements) Nosema spores were quantified visually using a standardized method (Cantwell, 1970). To do so, each worker was homogenized in a 2 mL Eppendorf tube using a bead mill homogeniser (MM300 Retsch), one glass bead and 1 mL of distilled water. Each homogenate was further diluted to 2 mL prior to spore quantification, which was done according to Cantwell (1970) using a haemocytometer (Thoma, L.O. Labor Optik) and a light microscope (Laborlux K, Leitz Wetzlar, Germany). Similarly, the pollen fed to the workers in the treatment groups 2 and 4 was checked for Nosema spp. spores using the same method.
Statistical Analyses
Statistical analyses were performed using the program, R, Version 4.1.2 (R Core Team, 2021). For the survival analysis, the packages “survival” (Therneau and Grambsch, 2000; Therneau, 2021) and “surminer” (Kassambara et al., 2021) were used for calculating and plotting Kaplan-Meier survival curves. The Surfdiff function was used to calculate survival curves and log rank testing (rho = 0) as well as to perform a chi squared test. The pairwise_survdiff function was used for multiple comparisons from the survival analysis between all treatment groups, and the resulting p-values were adjusted for multiple comparisons using a Bonferroni method (Bonferroni, 1936). Additionally, a simple linear regression model (lm) for the longevity data was performed, using the mean age per cage as a response variable, with the explanatory variables (i.e., treatments) expressed again as indicator variables (antibiotics = yes/no, pollen = yes/no) to estimate how the treatments, in days, influence life expectancy outcomes.
For the body weight analysis, raw untransformed data was used in a generalized linear mixed effect models (glmer), using the R package “lme4” (Bates et al., 2015) and fitted with a Gamma distribution, while defining “cage” and the time interval “day” as random factors. The model residuals were plotted with qqPlots from the R package “car” (Fox and Weisberg, 2019) to verify model assumptions (Supplementary Figure 1). The fitted (predicted) values were extracted from the model and used in boxplots. Post-hoc multiple comparison testing was done using the “multcomp” package, selecting “Tukey” comparison of means, with “Holm” correction (Holm, 1979). An additional linear mixed effect models (lmer) was carried out on the body weight data, with the explanatory variables (i.e., treatments) expressed as indicator variables (antibiotics = yes/no, pollen = yes/no), with “cage” and “day” defined as random factors, to estimate the effects of the explanatory variables as well as to test for any significant interactions. Sucrose consumption between the treatments was compared using a pairwise t-test with Bonferroni correction.
Results
Longevity
The experimental workers lived between 3 and 61 days (global mean: 18.77 days, SD = 9.9; Table 2). From the Kaplan Meier survival analysis, all four groups showed significant differences in longevity (Kaplan Meier, log rank test, all Ps < 0.05, letters A, B, C, and D, Figure 1; Table 3). Complementing the survival analysis, a simple linear model regression analysis was ran on the longevity data, and the model summary revealed that the regression analysis was significant (Multiple R-squared = 0.45, F-statistic = 9.003, 3 and 36 degrees of freedom, P < 0.0001). In addition, it revealed that workers supplied sucrose combined with antibiotics are estimated to have the longest average lifespan (lm, coefficient 7.84, P < 0.0001; Table 4) followed by workers without antibiotic treatment supplied ad libitum access to pollen (lm, coefficient 4.85, P < 0.004; Table 4), and finally, in sharp contrast, there was a significant negative interaction between a disrupted gut microbiota and ad libitum access to pollen, leading to a significant decrease in average lifespan (lm, coefficient −10.502, P < 0.0001; Table 4).
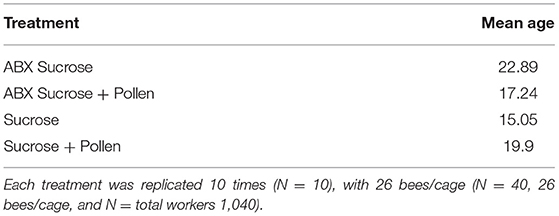
Table 2. Mean age (days) of each treatment group, ABX Sucrose, ABX Sucrose + Pollen, Sucrose, Sucrose + Pollen (N = 4).
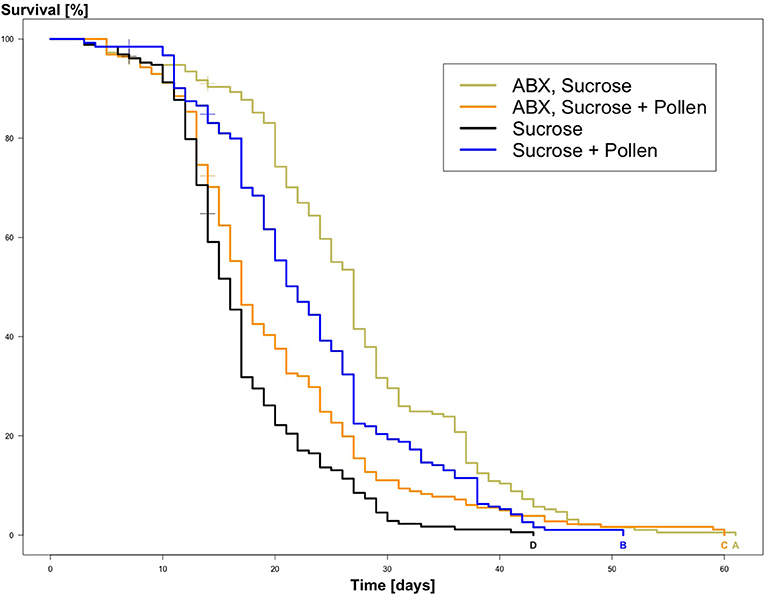
Figure 1. Kaplan Meier Survival curves of experimental adult workers, Apis mellifera, from the four treatment groups: ABX + Sucrose, ABX + Sucrose + Pollen, Sucrose, Sucrose + Pollen (N = 260 workers per group). Significant differences between the survival of the experimental workers are indicated by compact letter display based on log rank tests and Bonferroni p-adjusted values (Ps < 0.05).
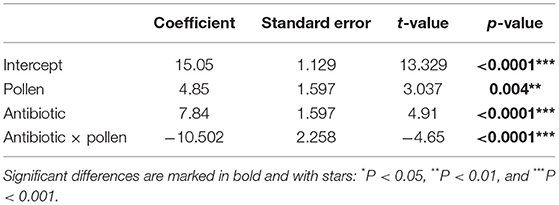
Table 4. Simple linear regression model of average cage lifespan (in days) of adult Apis mellifera workers dependent on pollen and antibiotics summary output.
Body Weight
The fresh body weight of all experimental workers ranged from 0.0776 and 0.1886 g (N = 60 workers per treatment, total N = 240 total workers, mean = 0.13537 g, SD = 0.02235). A generalized linear mixed effect model, with “Gamma” as a defined distribution, proved to be the best fit for the model residuals (Supplementary Figure 1). Post-hoc testing on the fitted (i.e., predicted) values extracted from the glmer (Figure 2) revealed three distinct groups (glmer, Tukey multiple mean comparison, Holm adjusted P-values, all Ps < 0.05). Highest weights were observed in the two groups given ad libitum access to pollen (Figure 2, Letter C), and the lowest body weight was observed in the group with an unmanipulated gut microbiota and sucrose diet (Figure 2, Letter A). Pollen supply was shown to be the main driver for higher body weights in the experimental workers (lmer, coefficient 0.0307, P < 0.0001; Table 5). The antibiotic treatment also had a significantly positive impact when no pollen was supplied (lmer, coefficient 0.0126, P < 0.0001; Table 5) and a negative interaction in workers that had access to pollen (lmer, coefficient −0.0204, P < 0.0001; Table 5).
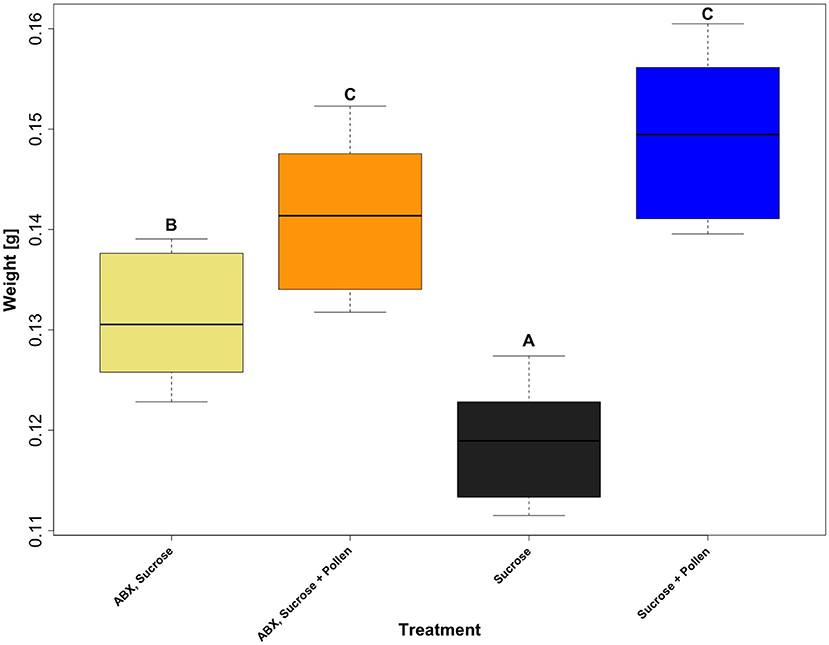
Figure 2. Boxplots of the predicted body weight values of adult workers, Apis mellifera, from four treatment groups: ABX + Sucrose, ABX + Sucrose + Pollen, Sucrose, and Sucrose + Pollen, extracted from a generalized linear mixed effect model (glmer) which was calculated using the weight of the workers, dependent on diet (treatment), and “cage” and “day” as random variables (N = 4 treatments, N = 60 workers per treatment, and total N = 240 total workers). Post-hoc testing from the glmer, comparing group means (Tukey), with Holm correction was used to determine statistical significance. Compact letter display shows indicated groups who vary statistically (P < 0.05).
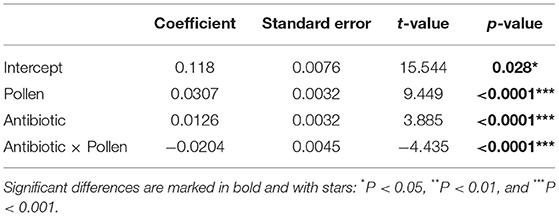
Table 5. Summary output from a linear mixed effect model of weight (in grams) of adult Apis mellifera workers dependent on pollen and antibiotics, with cage and day defined as random factors.
Food Consumption
The sucrose consumption values were pooled for the whole study duration (N = 4 treatments, total N = 233–393 observations/treatment, N = 1,281 total observations) and showed no significant differences between treatments (Pairwise T-test, Bonferroni correction, all Ps > 0.05).
Nosema spp. Infections
No Nosema spp. spores were detected in any of the experimental workers and in the supplied pollen.
Discussion
Our data show that pollen supply had a positive effect on longevity in workers with an unmanipulated gut microbiota. In sharp contrast, an adverse effect of pollen supply was observed in antibiotic-treated workers. Surprisingly, highest longevity was reported in antibiotic-treated workers supplied sucrose only. Pollen had a strong positive impact on worker body weight, and antibiotic treatment also showed a positive effect on body weight in workers without pollen supply. In contrast, the combination of antibiotic treatment and pollen supply showed a negative effect on body weight.
The observed mean longevity in the experimental summer bees was 18.77 days with a maximum lifespan of 61 days, and is in line with previous cage studies (e.g., Di Pasquale et al., 2016; Bernklau et al., 2019; Straub et al., 2019) or even exceeding observed longevities in other studies (e.g. Huang et al., 2014). The positive effect of pollen in workers without antibiotic treatment corresponds to the well-established beneficial impact of pollen supply on worker longevity (e.g., Schmidt et al., 1987; Malone et al., 1999; Di Pasquale et al., 2013; Tritschler et al., 2017; Retschnig et al., 2021). Pollen supplies the workers with the entire range of proteins, lipids and micronutrients (e.g., Haydak, 1970; Herbert et al., 1978) that are key for their development and growth (Winston, 1991; Brodschneider and Crailsheim, 2010). Additionally, pollen supply has been reported to be of importance for numerous further health parameters such as immune function (Alaux et al., 2010) or detoxification (Schmehl et al., 2014; Berenbaum and Johnson, 2015). However, the positive effect of pollen supply turned to the opposite in workers that were previously treated with antibiotics, which may be explained by the complex digestion of pollen (Nicolson et al., 2018) and the functional role of the gut microbiota in this digestion process (Vásquez and Olofsson, 2009; Lee et al., 2015). Pollen digestion is an arduous mechanical and enzymatic task for bees, given its multi-layered cell wall surrounding the nutrient dense center (Keller et al., 2005), and is therefore typically aided by symbiont bee-specific Lactobacillus microbiota (Vásquez and Olofsson, 2009). Further bacteria genera of the honey bee gut microbiota, Bifidobacterium and Gilliamella, have been linked to polysaccharide digestion and are the principal degraders of hemicellulose and pectin (Zheng et al., 2019). Indeed, a recent study showed that antibiotic treatment with oxytetracycline impaired protein digestion in honey bees (du Rand et al., 2020). If the worker gut microbiota loses its function following the exposure to antibiotics, the pollen may cause negative effects, potentially due to indigestible pollen components remaining in the gut or higher energy requirements for pollen digestion in absence of supportive gut bacteria (Klungness and Peng, 1984). Alternatively, pollen may be a source of pathogens or harming substances such as the pathogen Nosema ceranae (Higes et al., 2008), viruses (Singh et al., 2010) or pesticides (Chauzat et al., 2006) that might be harmful for workers, especially when they are weakened by a disrupted gut microbiota (Raymann et al., 2017). As no Nosema spp. spores were detected in the analyzed subsample of workers and the supplied pollen, a potential effect of this specific pathogen can be ruled out in the present study. The present study did not use sterilized pollen, leaving the door open for unknown opportunistic bacteria from the pollen to successfully populate ABX-treated workers. Therefore, food-borne disease resulting from pollen consumption and its potential to harm the health of honey bees with an unmanipulated vs. a compromised gut microbiota may be an interesting aspect to address in future research.
Antibiotic treatment has repeatedly been reported to affect honey bee worker longevity (Raymann et al., 2017; Li et al., 2019; Retschnig et al., 2021). In a recent study, Li et al. (2019) reported a significant decrease in the lifespan of workers when bees were treated with antibiotics. Further, they showed that pollen supply could partially counteract the negative effect of the antibiotic treatment, which is in line with previous reports (Retschnig et al., 2021), but differs considerably from the here obtained data. This dissenting outcome may be due to a different applied antibiotic substance (tetracycline vs. penicillin–streptomycin), dosage (Marceau et al., 2021), or the duration of antibiotic treatment (permanent vs. only 72 h). Further, the study duration may have an impact on the outcome. While Li et al. (2019) analyzed survival data for a 15-day time interval, in the present study, worker mortality was recorded until the last worker has died (61 days). This clearly shows that findings can vary considerably depending on applied methods. Finally, by removing the bees from their frames 48 h post-emergence, the experimental design (by default) disrupted the natural timeline and transmission pathways of microbiota acquisition from real-hive scenarios (Powell et al., 2014) before ABX treatment. Indeed, this entails an implicit issue when considering the non-ABX treated group (sucrose), indicating that they too may have a slightly “modified” microbiota. Nonetheless, we argue this is a necessary step to take in order to fully manipulate the nutritional diets of the bees, given that early-stage nutrition is critically tied to subsequent lifespan of the worker bees (Brodschneider and Crailsheim, 2010), the exact variables we wished to manipulate here.
Surprisingly and contradicting a large body of existing evidence where no negative (du Rand et al., 2020) or significant negative effects on survival (e.g., Raymann et al., 2017; Li et al., 2019; Retschnig et al., 2021) were detected, antibiotic treatment showed a positive effect on worker longevity when workers were fed with sucrose only. This may be explained by an antibiotic-induced reduced potential of pathogens in these workers (e.g., bacteria, viruses; Dutta and Basu, 2011), however, further investigations of possible mechanisms are needed. Additionally, the tetracycline screening pre-trial process was repeated in two separate cage trial experiments to confirm our results with a set 95% confidence level, yet here the ABX Sucrose treatment performed better in both weight and longevity to its direct counterpart “Sucrose only” treatment. Repeatability of experiments is paramount in science, and it is possible we achieved the 1/20th (5%) probability of obtaining a statistically different result than our ABX screening pre-trials. Further cage trials testing the present tetracycline dosing would clarify this important point. Finally, the highly artificial conditions associated with cage studies need to be taken into account for data interpretation (e.g., Retschnig et al., 2015); effects of treatments may be different under field colony conditions, where workers are involved with various in-hive and foraging activities (Winston, 1991).
Body weight is regularly measured as health parameter (e.g., Pettis et al., 2012; Retschnig et al., 2014; Straub et al., 2019) and has previously been identified as marker for longevity (Retschnig et al., 2021). The body weight results of this study indicate a significant positive signal from ad libitum access to pollen, likely leading to improved growth and body tissue development, which is supported by dated to contemporary publications (Haydak, 1937, 1970; Roulston and Cane, 2000; Tritschler et al., 2017; Retschnig et al., 2021). Previous reports have also shown that pollen can mitigate negative antibiotic-induced effects by increasing observed worker weights (Li et al., 2019), cohering with what was observed in this study. In contrast to pollen, evidence from previously mentioned studies underscore negative impacts of antibiotics on worker body weight in larvae (Duan et al., 2021) and adults (Retschnig et al., 2021) and demonstrate a positive effect of gut bacteria on weight gain in young adult workers (Zheng et al., 2017). Contrary to expectations, yet in line with the longevity data, the tetracycline treatment had a positive impact on worker body weight compared to their non-treated counterpart in the groups that were supplied with sucrose only. As sucrose consumption was consistent between treatment groups, the higher body weight cannot be attributed to altered sucrose intake habits and must be the result of another underlying mechanism. Tetracyclines, in general, are effective broad-spectrum antibiotics (Duggar, 1948), additionally acting as antiviral agents (Dutta and Basu, 2011; Mosquera-Sulbaran and Hernández-Fonseca, 2021). In case the experimental workers of this study harbored unnoticed pathogens and/or viruses, the latter may have been affected by the antibiotics, thereby leading to a beneficial effect on the workers. Investigating if tetracycline also has antiviral effects to common A. mellifera viruses would be of great interest, and if so, if this could further explain the current findings of this paper. Finally, in several areas around the world, tetracyclines are commonplace in agriculture, in part for the known ability to assist in increasing the weight of livestock (Cox, 2016). Although the underlying mechanisms are not fully understood, it is hypothesized that the ABX select for bacteria better at nutrient extraction, thus providing more calories to their host (Cox, 2016). Employing modern micro- and molecular biology techniques to see if this is the case in ABX treated bees would help shed light on accepting or rejecting notion of ABX positively selecting specialized microbes better at deriving nutrients, resulting in weight gain to their host.
In conclusion, this study adds to the evidence of beneficial effects of pollen supply on health parameters in honey bee workers in the presence of a functional gut microbiota. Beyond that, it also revealed a potential of harm of pollen access in antibiotic-treated workers with a compromised gut microbiota. This aspect and underlying mechanisms require further investigation and should be considered when honey bees and honey bee colonies are treated (preventively) with antibiotics against pathogens.
Data Availability Statement
The datasets for this study can be found in the Dryad data repository: https://doi.org/10.5061/dryad.wstqjq2p2.
Author Contributions
AB, VR, PN, and GR designed the experiment. AB, VR, CB, and JP conducted the experiment. AB, PN, and GR analyzed the data and wrote the manuscript. AB, VR, CB, JP, PN, and GR revised and approved the final manuscript. All authors contributed to the article and approved the submitted version.
Funding
The financial support was granted by Ricola Foundation Nature and Culture (GR and PN) and the Vinetum Foundation (PN).
Conflict of Interest
The authors declare that the research was conducted in the absence of any commercial or financial relationships that could be construed as a potential conflict of interest.
Publisher's Note
All claims expressed in this article are solely those of the authors and do not necessarily represent those of their affiliated organizations, or those of the publisher, the editors and the reviewers. Any product that may be evaluated in this article, or claim that may be made by its manufacturer, is not guaranteed or endorsed by the publisher.
Acknowledgments
The authors wish to thank the Ricola Foundation Nature and Culture (GR and PN) and the Vinetum Foundation (PN) for financial support.
Supplementary Material
The Supplementary Material for this article can be found online at: https://www.frontiersin.org/articles/10.3389/fsufs.2022.864741/full#supplementary-material
References
Alaux, C., Ducloz, F., Crauser, D., and Le Conte, Y. (2010). Diet effects on honeybee immunocompetence. Biol. Lett. 6, 562–565. doi: 10.1098/rsbl.2009.0986
Alberoni, D., Gaggìa, F., Baffoni, L., and Di Gioia, D. (2016). Beneficial microorganisms for honey bees: problems and progresses. Appl. Microbiol. Biotechnol. 100, 9469–9482. doi: 10.1007/s00253-016-7870-4
Anderson, K. E., Sheehan, T. H., Eckholm, B. J., Mott, B. M., and DeGrandi-Hoffman, G. (2011). An emerging paradigm of colony health: microbial balance of the honey bee and hive (Apis mellifera). Insect. Soc. 58:431. doi: 10.1007/s00040-011-0194-6
Barascou, L., Sene, D., Barraud, A., Michez, D., Lefebvre, V., Medrzycki, P., et al. (2021). Pollen nutrition fosters honeybee tolerance to pesticides. R. Soc. Open Sci. 8:210818. doi: 10.1098/rsos.210818
Barron, A. B. (2015). Death of the bee hive: understanding the failure of an insect society. Curr. Opin. Insect. Sci. 10, 45–50. doi: 10.1016/j.cois.2015.04.004
Bates, D., Mächler, M., Bolker, B., and Walker, S. (2015). Fitting linear mixed-effects models using lme4. J. Stat. Softw. 67, 1–48. doi: 10.18637/jss.v067.i01
Berenbaum, M. R., and Johnson, R. J. (2015). Xenobiotic detoxification pathways in honey bees. Curr. Opin. Insect Sci. 10, 51–58. doi: 10.1016/j.cois.2015.03.005
Bernklau, E., Bjostad, L., Hogeboom, A., Carlisle, A., and Arathi, H. S. (2019). Dietary phytochemicals, honey bee longevity and pathogen tolerance. Insects 10:14. doi: 10.3390/insects10010014
Bonferroni, C. E. (1936). Statistical theory of classification and calculation of the probability. Pub. R Ist Superiore Sci. Econ. Commerc Firenze 8, 36–62.
Bonilla-Rosso, G., and Engel, P. (2018). Functional roles and metabolic niches in the honey bee gut microbiota. Curr. Opin. Microbiol. 43, 69–76. doi: 10.1016/j.mib.2017.12.009
Brodschneider, R., and Crailsheim, K. (2010). Nutrition and health in honey bees. Apidologie 41, 278–294. doi: 10.1051/apido/2010012
Brown, A., Rodriguez, V., Pfister, J., Perreten, V., Neumann, P., and Retschnig, G. (2022). The dose makes the poison: feeding of antibiotic-treated winter honey bees, Apis mellifera, with probiotics and B-vitamins. Apidologie. doi: 10.1007/s13592-022-00927-4
Chauzat, M.-P., Faucon, J.-P., Martel, A.-C., Lachaize, J., Cougoule, N., and Aubert, M. (2006). A survey of pesticide residues in pollen loads collected by honey bees in France. J. Econ. Entomol. 99, 253–262. doi: 10.1093/jee/99.2.253
Cox, L. M. (2016). Antibiotics shape microbiota and weight gain across the animal kingdom. Animal Frontiers 6. doi: 10.2527/af.2016-0028
Daisley, B. A., Chmiel, J. A., Pitek, A. P., Thompson, G. J., and Reid, G. (2020). Missing microbes in bees: how systematic depletion of key symbionts erodes immunity. Trends Microbiol. 28, 1010–1021. doi: 10.1016/j.tim.2020.06.006
Di Pasquale, G., Alaux, C., Le Conte, Y., Odoux, J.-F., Pioz, M., Vaissière, B. E., et al. (2016). Variations in the availability of pollen resources affect honey bee health. PLoS One 11:e0162818. doi: 10.1371/journal.pone.0162818
Di Pasquale, G., Salignon, M., Le Conte, Y., Belzunces, L. P., Decourtye, A., Kretzschmar, A., et al. (2013). Influence of pollen nutrition on honey bee health: do pollen quality and diversity matter? PLoS One 8:e72016. doi: 10.1371/journal.pone.0072016
Dolezal, A. G., and Toth, A. L. (2018). Feedbacks between nutrition and disease in honey bee health. Curr. Opin. Insect. Sci. 26, 114–119. doi: 10.1016/j.cois.2018.02.006
Dosch, C., Manigk, A., Streicher, T., Tehel, A., Paxton, R. J., and Tragust, S. (2021). The gut microbiota can provide viral tolerance in the honey bee. Microorganisms 9:871. doi: 10.3390/microorganisms9040871
du Rand, E. E., Stutzer, C., Human, H., Pirk, C. W. W., and Nicolson, S. W. (2020). Antibiotic treatment impairs protein digestion in the honeybee, Apis mellifera. Apidologie 51, 94–106. doi: 10.1007/s13592-019-00718-4
Duan, X., Zhao, B., Jin, X., Cheng, X., Huang, S., and Li, J. (2021). Antibiotic treatment decrease the fitness of honeybee (Apis mellifera) larvae. Insects 12:301. doi: 10.3390/insects12040301
Duggar, B. M. (1948). Aureomycin: a product of the continuing search for new antibiotics. Ann. N. Y. Acad. Sci. 51, 177–181. doi: 10.1111/j.1749-6632.1948.tb27262.x
Dutta, K., and Basu, A. (2011). Use of minocycline in viral infections. Indian J. Med. Res. 133, 467–470. Available online at: https://pubmed.ncbi.nlm.nih.gov/21623029/
Engel, P., and Moran, N. A. (2013). The gut microbiota of insects - diversity in structure and function. FEMS Microbiol. Rev. 37, 699–735. doi: 10.1111/1574-6976.12025
Fox, J., and Weisberg, S. (2019). An R Companion to Applied Regression, 3rd Edn. Thousand Oaks, CA: Sage. Available online at: https://socialsciences.mcmaster.ca/jfox/Books/Companion/ (accessed January 15, 2022).
Garibaldi, L. A., Steffan-Dewenter, I., Winfree, R., Aizen, M. A., Bommarco, R., Cunningham, S. A., et al. (2013). Wild pollinators enhance fruit set of crops regardless of honey bee abundance. Science 339, 1608–1611. doi: 10.1126/science.1230200
Genersch, E. (2010). American foulbrood in honeybees and its causative agent, paenibacillus larvae. J. Invertebr. Pathol. 103, 10–19. doi: 10.1016/j.jip.2009.06.015
Gray, A., Adjlane, N., Arab, A., Ballis, A., Brusbardis, V., Charrière, J.-D., et al. (2020). Honey bee colony winter loss rates for 35 countries participating in the COLOSS survey for winter 2018-2019, and the effects of a new queen on the risk of colony winter loss. J. Apicult. Res. 59, 744–751. doi: 10.1080/00218839.2020.1797272
Haydak, M. H. (1937). The influence of a pure carbohydrate diet on newly emerged honeybees. Ann. Entomol. Soc. Am. 30, 258–262. doi: 10.1093/aesa/30.2.258
Haydak, M. H. (1970). Honey bee nutrition. Annu. Rev. Entomol. 15, 143–156. doi: 10.1146/annurev.en.15.010170.001043
Herbert, J., Elton, W., and Shimanuki, H. (1978). Chemical composition and nutritive value of bee-collected and bee-stored pollen. Apidologie 9, 33–40. doi: 10.1051/apido:19780103
Higes, M., Martín-Hernández, R., Garrido-Bailon, E., García-Palencia, P., and Meana, A. (2008). Detection of infective nosema ceranae (microsporidia) spores in corbicular pollen of forager honeybees. J. Invertebr. Pathol. 97, 76–78. doi: 10.1016/j.jip.2007.06.002
Huang, S. K., Csaki, T., Doublet, V., Dussaubat, C., Evans, J. D., Gajda, A. M., et al. (2014). Evaluation of cage designs and feeding regimes for honey bee (Hymenoptera: Apidae) laboratory experiments. J. Econom. Entomol. 107, 54–62. doi: 10.1603/EC13213
Hung, K.-L. J., Kingston, J. M., Albrecht, M., Holway, D. A., and Kohn, J. R. (2018). The worldwide importance of honey bees as pollinators in natural habitats. Proc. Royal Soc. B. 285:20172140. doi: 10.1098/rspb.2017.2140
Kassambara, A., Kosinski, M., and Biecek, P. (2021). Survminer: Drawing Survival Curves Using 'ggplot2'. R package version 0.4.9. Available online at: https://CRAN.R-project.org/package=survminer (accessed January 15, 2022).
Keller, I., Fluri, P., and Imdorf, A. (2005). Pollen nutrition and colony development in honey bees: part 1. Bee World 86, 3–10. doi: 10.1080/0005772X.2005.11099641
Klungness, L. M., and Peng, Y.-S. (1984). A histochemical study of pollen digestion in the alimentary canal of honeybees (Apis mellifera L.). J. Insect Physiol. 30, 511–521. doi: 10.1016/0022-1910(84)90077-5
Koch, H., and Schmid-Hempel, P. (2011). Socially transmitted gut microbiota protect bumble bees against an intestinal parasite. Proc. Natl. Acad. Sci. U. S. A. 108, 19288–19292. doi: 10.1073/pnas.1110474108
Kwong, W. K., and Moran, N. A. (2016). Gut microbial communities of social bees. Nat. Rev. Microbiol. 14, 374–384. doi: 10.1038/nrmicro.2016.43
Lee, F. J., Miller, K. I., McKinlay, J. B., and Newton, I. L. G. (2018). Differential carbohydrate utilization and organic acid production by honey bee symbionts. FEMS Microbiol. Ecol. 94:113. doi: 10.1093/femsec/fiy113
Lee, F. J., Rusch, D. B., Stewart, F. J., Mattila, H. R., and Newton, I. L. G. (2015). Saccharide breakdown and fermentation by the honey bee gut microbiome. Environ. Microbiol. 17, 796–815. doi: 10.1111/1462-2920.12526
Li, J., Heerman, M. C., Evans, J. D., Rose, R., Li, W., Rodríguez-García, C., et al. (2019). Pollen reverses decreased lifespan, altered nutritional metabolism and suppressed immunity in honey bees (Apis mellifera) treated with antibiotics. J. Exp. Biol. 222:jeb202077. doi: 10.1242/jeb.202077
Lozupone, C., Stombaugh, J., Gordon, J., Jansson, J. K., and Knight, R. (2012). Diversity, stability and resilience of the human gut microbiota. Nature 489, 220–230. doi: 10.1038/nature11550
Malone, A. L., Burgess, P. E., and Stefanovic, D. (1999). Effects of a Bacillus thuringiensis toxin, two Bacillus thuringiensis biopesticide formulations, and a soybean trypsin inhibitor on honey bee (Apis mellifera L.) survival and food consumption. Apidologie 30, 465–473. doi: 10.1051/apido:19990601
Marceau, T., Archer, C. R., Bulson, L., and Wilfert, L. (2021). Dose-dependent effects of antibiotic intake on Bombus terrestris (Linnaeus, 1758) dietary intake, survival and parasite infection prevalence. J. Invertebr. Pathol. 182:107580. doi: 10.1016/j.jip.2021.107580
Martinson, V. G., Danforth, B. N., Minckley, R. L., Rueppel, O., Tingek, S., and Moran, N. A. (2011). A simple and distinctive microbiota associated with honey bees and bumble bees. Mol. Ecol. 20, 619–628. doi: 10.1111/j.1365-294X.2010.04959.x
Mattila, H. R., and Otis, G. W. (2006). Effects of pollen availability and nosema infection during the spring on division of labor and survival of worker honey bees (Hymenoptera: Apidae). Environ. Entomol. 35, 708–717. doi: 10.1603/0046-225X-35.3.708
Mosquera-Sulbaran, J. A., and Hernández-Fonseca, H. (2021). Tetracycline and viruses: a possible treatment for COVID-19? Arch. Virol. 166, 1–7. doi: 10.1007/s00705-020-04860-8
Motta, E. V. S., Raymann, K., and Moran, N. A. (2018). Glyphosate perturbs the gut microbiota of honey bees. Proc. Natl. Acad. Sci. U. S. A. 115, 10305–10310. doi: 10.1073/pnas.1803880115
Neumann, P., and Carreck, N. L. (2010). Honey bee colony losses. J. Apicult. Res. 49, 1–6. doi: 10.3896/IBRA.1.49.1.01
Nicolson, S. W., Neves, S. D. S. D., Human, H., and Pirk, C. W. W. (2018). Digestibility and nutritional value of fresh and stored pollen for honey bees (Apis mellifera scutellata). J. Insect. Physiol. 107, 302–308. doi: 10.1016/j.jinsphys.2017.12.008
OECD (2017). Test No. 245: Honey Bee (Apis Mellifera L.), Chronic Oral Toxicity Test (10-Day Feeding). OECD Guidelines for the Testing of Chemicals, Section 2. Paris: OECD Publishing.
Papa, G., Di Prisco, G., Spini, G., Puglisi, E., and Negri, I. (2021). Acute and chronic effects of titanium dioxide (TiO2) PM1 on honey bee gut microbiota under laboratory conditions. Sci. Rep. 11:5946. doi: 10.1038/s41598-021-85153-1
Pereira, K. D. S., Meeus, I., and Smagghe, G. (2019). Honey bee-collected pollen is a potential source of ascosphaera apis infection in managed bumble bees. Sci. Rep. 9:4241. doi: 10.1038/s41598-019-40804-2
Pettis, J. S., Vanengelsdorp, D., Johnson, J., and Dively, G. (2012). Pesticide exposure in honey bees results in increased levels of the gut pathogen nosema. Naturwissenschaften 99, 153–158. doi: 10.1007/s00114-011-0881-1
Porrini, M. P., Sarlo, E. G., Medici, S. K., Garrido, P. M., Porrini, D. P., Damiani, N., et al. (2011). Nosema ceranae development in Apis mellifera: influence of diet and infective inoculum. J. Apicult. Res. 50, 35–41. doi: 10.3896/IBRA.1.50.1.04
Potts, S. G., Biesmeijer, J. C., Kremen, C., Neumann, P., Schweiger, O., and Kunin, W. E. (2010). Global pollinator declines: trends, impacts and drivers. Trends Ecol. Evol. 25, 345–353. doi: 10.1016/j.tree.2010.01.007
Powell, J. E., Martinson, V. G., Urban-Mead, K., and Moran, N. A. (2014). Routes of acquisition of the gut microbiota of the honey bee Apis mellifera. Appl. Environ. Microbiol. 80, 7378–7387. doi: 10.1128/AEM.01861-14
R Core Team (2021). R: A language and environment for statistical computing. R Foundation for Statistical Computing, Vienna, Austria. Available online at: https://www.R-project.org/
Raymann, K., Bobay, L.-M., and Moran, N. A. (2018). Antibiotics reduce genetic diversity of core species in the honeybee gut microbiome. Mol. Ecol. 27, 2057–2066. doi: 10.1111/mec.14434
Raymann, K., Shaffer, Z., and Moran, N. A. (2017). Antibiotic exposure perturbs the gut microbiota and elevates mortality in honeybees. PLoS Biol. 15:e2001861. doi: 10.1371/journal.pbio.2001861
Retschnig, G., Rich, J., Crailsheim, K., Pfister, J., Perreten, V., and Neumann, P. (2021). You are what you eat: relative importance of diet, gut microbiota and nestmates for honey bee, Apis mellifera, worker health. Apidologie 52, 632–646. doi: 10.1007/s13592-021-00851-z
Retschnig, G., Williams, G. R., Mehmann, M. M., Yañez, O., De Miranda, J. R., and Neumann, P. (2014). Sex-specific differences in pathogen susceptibility in honey bees (Apis mellifera). PLoS One 9:e85261. doi: 10.1371/journal.pone.0085261
Retschnig, G., Williams, G. R., Odemer, R., Boltin, J., Di Poto, C., Mehmann, M. M., et al. (2015). Effects, but no interactions, of ubiquitous pesticide and parasite stressors on honey bee (Apis mellifera) lifespan and behaviour in a colony environment. Environ. Microbiol. 17:4322–4331. doi: 10.1111/1462-2920.12825
Ricigliano, V. A., Fitz, W., Copeland, D. C., Mott, B. M., Maes, P., Floyd, A. S., et al. (2017). The impact of pollen consumption on honey bee (Apis mellifera) digestive physiology and carbohydrate metabolism. Arch. Insect Biochem. Physiol. 96:e21406. doi: 10.1002/arch.21406
Roulston, T. H., and Cane, J. H. (2000). “Pollen nutritional content and digestibility for animals,” in Pollen and Pollination, eds. A. Dafni, M. Hesse, and E. Pacini (Vienna: Springer), 187–209.
Schmehl, D. R., Teal, P. E. A., Frazier, J. L., and Grozinger, C. M. (2014). Genomic analysis of the interaction between pesticide exposure and nutrition in honey bees (Apis mellifera). J. Insect. Physiol. 71, 177–190. doi: 10.1016/j.jinsphys.2014.10.002
Schmidt, J. O., Thoenes, S. C., and Levin, M. D. (1987). Survival of honey bees, Apis mellifera (Hymenoptera: Apidae), fed various pollen sources. Ann. Entomol. Soc. Am. 80, 176–183. doi: 10.1093/aesa/80.2.176
Schwarz, R. S., Moran, N. A., and Evans, J. D. (2016). Early gut colonizers shape parasite susceptibility and microbiota composition in honey bee workers. Proc. Natl. Acad. Sci. U. S. A. 113, 9345–9350. doi: 10.1073/pnas.1606631113
Singh, R., Levitt, A. L., Rajotte, E. G., Holmes, E. C., Ostiguy, N., Vanengelsdorp, D., et al. (2010). RNA viruses in hymenopteran pollinators: evidence of inter-taxa virus transmission via pollen and potential impact on non-apis hymenopteran species. PLoS One 5:e14357. doi: 10.1371/journal.pone.0014357
Soares, K. O., de Oliveira, C. J. B., Rodrigues, A. E., Vasconcelos, P. C., Silva, N. M., Octavio Filho, G. C., et al. (2021). Tetracycline exposure alters key gut microbiota in Africanized honey bees (Apis mellifera scutellata × spp.). Front. Ecol. Evol. 9:716660. doi: 10.3389/fevo.2021.716660
Stanimirović, Z., Glavini,ć, U., Ristani,ć, M., Aleksić, N., Jovanovi,ć, N., Vejnovi,ć, B., et al. (2019). Looking for the causes of and solutions to the issue of honey bee colony losses. Acta Vet. 69, 1–31. doi: 10.2478/acve-2019-0001
Straub, L., Williams, G. R., Vidondo, B., Khongphinitbunjong, K., Retschnig, G., Schneeberger, A., et al. (2019). Neonicotinoids and ectoparasitic mites synergistically impact honeybees. Sci. Rep. 9:8159. doi: 10.1038/s41598-019-44207-1
Therneau, T. M., and Grambsch, P. M. (2000). “The Cox model,” in Modeling Survival Data: Extending the Cox Model (New York: Springer), 39–77.
Tian, B., Fadhil, N. H., Powell, J. E., Kwong, W. K., and Moran, N. A. (2012). Long-term exposure to antibiotics has caused accumulation of resistance determinants in the gut microbiota of honeybees. MBio 3, e00377–e00312. doi: 10.1128/mBio.00377-12
Tritschler, M., Vollmann, J. J., Yañez, O., Chejanovsky, N., Crailsheim, K., and Neumann, P. (2017). Protein nutrition governs within-host race of honey bee pathogens. Sci. Rep. 7:14988. doi: 10.1038/s41598-017-15358-w
Vásquez, A., Forsgren, E., Fries, I., Paxton, R. J., Flaberg, E., Szekely, L., et al. (2012). Symbionts as major modulators of insect health: lactic acid bacteria and honeybees. PLoS One 7:e33188. doi: 10.1371/journal.pone.0033188
Vásquez, A., and Olofsson, T. C. (2009). The lactic acid bacteria involved in the production of bee pollen and bee bread. J. Apicult. Res. 48, 189–195. doi: 10.3896/IBRA.1.48.3.07
Wang, H., Zhang, S. W., Zeng, Z. J., and Yan, W. Y. (2014). Nutrition affects longevity and gene expression in honey bee (Apis mellifera) workers. Apidologie 45, 618–625. doi: 10.1007/s13592-014-0276-3
Williams, G. R., Alaux, C., Costa, C., Csáki, T., Doublet, V., Eisenhardt, D., et al. (2013). Standard methods for maintaining adult Apis mellifera in cages under in vitro laboratory conditions. J. Apic. Res. 52, 1–36. doi: 10.3896/IBRA.1.52.1.04
Winston, M. L. (1991). The Biology of the Honey Bee. Harvard University Press, Cambridge, Massachusetts.
Wright, G. A., Nicolson, S. W., and Shafir, S. (2018). Nutritional physiology and ecology of honey bees. Annu. Rev. Entomol. 63, 327–344. doi: 10.1146/annurev-ento-020117-043423
Zheng, H., Perreau, J., Powell, J. E., Han, B., Zhang, Z., Kwong, W. K., et al. (2019). Division of labor in honey bee gut microbiota for plant polysaccharide digestion. Proc. Natl. Acad. Sci. U. S. A. 116, 25909–25916. doi: 10.1073/pnas.1916224116
Zheng, H., Powell, J. E., Steele, M. I., Dietrich, C., and Moran, N. A. (2017). Honeybee gut microbiota promotes host weight gain via bacterial metabolism and hormonal signaling. Proc. Natl. Acad. Sci. U. S. A. 114, 4775–4780. doi: 10.1073/pnas.1701819114
Keywords: Apis mellifera, honey bee, gut microbiota, nutrition, pollen
Citation: Brown AF, Rodriguez V, Brzoska C, Pfister J, Neumann P and Retschnig G (2022) Dream Team for Honey Bee Health: Pollen and Unmanipulated Gut Microbiota Promote Worker Longevity and Body Weight. Front. Sustain. Food Syst. 6:864741. doi: 10.3389/fsufs.2022.864741
Received: 28 January 2022; Accepted: 20 March 2022;
Published: 03 May 2022.
Edited by:
Kimberly Ann Stoner, Connecticut Agricultural Experiment Station, United StatesReviewed by:
Shota Suenami, National Institute of Advanced Industrial Science and Technology (AIST), JapanDaniele Alberoni, University of Bologna, Italy
Copyright © 2022 Brown, Rodriguez, Brzoska, Pfister, Neumann and Retschnig. This is an open-access article distributed under the terms of the Creative Commons Attribution License (CC BY). The use, distribution or reproduction in other forums is permitted, provided the original author(s) and the copyright owner(s) are credited and that the original publication in this journal is cited, in accordance with accepted academic practice. No use, distribution or reproduction is permitted which does not comply with these terms.
*Correspondence: Andrew F. Brown, YW5kcmV3LmYuYnJvd25Ab3V0bG9vay5jb20=