- 1Department of Entomology, University of Illinois Urbana-Champaign, Urbana, IL, United States
- 2Department of Entomology, Iowa State University, Ames, IA, United States
Beekeepers experience high annual losses of colonies, with environmental stressors like pathogens, reduced forage, and pesticides as contributors. Some factors, like nutritional stress from reduced flower abundance or diversity, are more pronounced in agricultural landscapes where extensive farming limits pollen availability. In addition to affecting other aspects of colony health, quantity and quality of pollen available are important for colony brood production and likely for queen egg laying. While some US beekeepers report >50% of colony loss due to queen failure, the causes of poor-quality queens are poorly understood. Access to resources from native prairie habitat is suggested as a valuable late-season resource for honey bees that can reverse colony growth declines, but it is not clear how prairie forage influences queen egg laying. We hypothesized that the pollen resources present in an extensive Midwestern corn/soybean agroecosystem during the critical late season period affect honey bee queen egg laying and that access to native prairies can increase queen productivity. To test this, we designed a field experiment in Iowa, keeping colonies in either soybean or prairie landscapes during a critical period of forage dearth, and we quantified queen egg laying as well as pollen collection (quantity and species). Then, using pollen collected in the field experiments, we created representative dietary mixtures, which we fed to bees using highly controlled laboratory cages to test how consumption of these diets affected the egg laying of naive queens. In two out of three years, queens in prairies laid more eggs compared to those in soybean fields. Pollen quantity did not vary between the two landscapes, but composition of species did, and was primarily driven by collection of evening primrose (Oenothera biennis). When pollen representative of the two landscapes was fed to caged bees in the laboratory queens fed prairie pollen laid more eggs, suggesting that pollen from this landscape plays an important role in queen productivity. More work is needed to tease apart the drivers of these differences, but understanding how egg laying is regulated is useful for designing landscapes for sustainable pollinator management and can inform feeding regimes for beekeepers.
Introduction
Managed honey bee colonies in the U.S. contribute an estimated $15 billion to the agricultural economy annually (Potts et al., 2010; Calderone, 2012), largely due to pollination services, and are fundamental to the production of many plant products (Klein et al., 2007; Khalifa et al., 2021). However, interacting environmental stresses on honey bees, specifically poor forage nutrition, pesticides, and pathogens (Goulson et al., 2015; Belsky and Joshi, 2019; Neov et al., 2021), continue to drive colony losses (Kulhanek et al., 2017; Bee Informed Team, 2021) and pose challenges for sustainable pollinator management (Hristov et al., 2020), especially in systems dominated by agricultural production. While pressure from Varroa mites (Varroa destructor) and disease are risk factors in most operations (Traynor et al., 2020), honey bees in agroecosystems also face nutritional stress from reduced flower abundance or diversity in farm landscapes (Naug, 2009; Tosi et al., 2017; Dolezal et al., 2019b).
The nutritional landscape surrounding colonies determines pollen and nectar collection (Sande et al., 2009; Donkersley et al., 2014; Dolezal et al., 2016; Zhang et al., 2020) and affects colony health and physiology (Smart et al., 2016; Alaux et al., 2017; Dolezal et al., 2019b; Noordyke et al., 2021). When forage quantity and or quality are low, this stress can reduce colony resilience (Horn et al., 2016; Branchiccela et al., 2019; Crone and Grozinger, 2021). For example, honey bee colonies kept in landscapes dominated by corn and soybean agriculture in the Midwestern US have been shown to successfully accumulate adequate honey and pollen stores for part of the growing season, relying predominantly on food resources derived from crops or the non-native plants growing around fields (i.e., clovers) (Dolezal et al., 2019b). Once soybean and clover in those Midwestern landscapes senesce beginning in August, however, colonies may experience substantial declines in mass and individual worker body quality, indicating this time frame as a critical point in the colony life cycle. In these extensive corn and soybean systems, diversified landscapes, in the form of fruit and vegetable farming, may provide an extra boost to colony growth over that of conventional soybean; however, even in fruit and vegetable farms colonies still experience late season mass declines (St. Clair et al., 2020). A promising landscape for reversing or halting late season declines in colony growth may be native prairie habitat (Dolezal et al., 2019b). Although honey bee colonies do not prefer to forage extensively in native prairie habitat compared to agricultural systems during much of the growing season, it has been shown that, when available, they do make a switch to collection of native prairie resources in the late summer/early fall (Carr-Markell et al., 2020; Zhang et al., 2021).
While these studies have provided important insights into understanding the mechanisms behind colony stresses, most focused on whole colony outcomes or on workers alone. Workers make up most of the colony population and perform nearly all aspects of colony function, such as foraging, brood care, and defense (Caron, 2013). Because they leave the colony, they are also most directly exposed to stressors in the environment (Chmiel et al., 2020). However, all the workers are the offspring of a single, long-lived queen who is solely responsible for colony reproduction (Hölldobler and Wilson, 2009). Long-term colony survival depends on her ability to produce thousands of offspring daily to replace the workforce; her productivity is even more critical under stressful conditions that shorten worker lifespans (Simone-Finstrom et al., 2016; Rueppell et al., 2017). Challenges to queen productivity and health have been observed more in recent years, with some beekeeper survey reports indicating queen failure rates as the cause of >50% of total annual losses (Bee Informed Team, 2021). While informative, these reports are not a rigorous causal link between queen health and colony loss but do highlight the lack of understanding behind what causes poor queen quality. Large-scale commercial beekeeping operations report replacement of over 50% of queens within 6 months (Vanengelsdorp et al., 2013; Sandrock et al., 2014), when historically queens survived for 2–3 years (Harbo and Szabo, 1984; Pettis et al., 1991).
Although queen failure is described as a leading contributor to losses (Amiri et al., 2017; Steinhauer et al., 2018; Bee Informed Team, 2021), the cause of “poor queen quality” is not well understood, partly due to a lack of reliable methods to study queen phenotypes (Lee et al., 2019), and partly because measuring effects on queens requires colony level experiments that are logistically difficult, expensive, and, most importantly, hard to control (i.e., colonies are exposed to a wide variety of environmental variables). In many cases, combined factors, all of which have the potential to disrupt queen health, contribute to poor overall colony function (Maini et al., 2010; Vanengelsdorp and Meixner, 2010; Hristov et al., 2020). For this reason, when a colony fails, there is often no clear explanation as to why. Like worker bees, environmental exposure to inadequate nutrition causes stress on the queen at the larval stage (De Souza et al., 2019). Very little work has focused on how nutrition affects mated adult queens. Evidence suggests that varied nutrition does result in differential honey bee queen egg laying (Fine et al., 2018), and nutrition has a strong impact on the health of queen bumblebees (Woodard et al., 2019; Watrous et al., 2021).
Understanding the causes of queen failure poses further challenges because the division of labor and coordination of tasks buffers the colony from stress and separates queens from direct contact with the outside environment (Hölldobler and Wilson, 2009). Apart from her mating flight, and swarming, the queen does not leave the colony or forage for her own food; this is thought to limit her exposure to external stressors worker bees face directly (Johnson, 2010; Klein et al., 2017). Even inside the colony, the queen will rarely, if ever, feed herself; instead, she is fed mainly royal jelly that is produced by nurse aged workers via their glandular secretions (i.e., mandibular and hypopharyngeal glands) (Simuth, 2001; Wright et al., 2017). The quality of the royal jelly produced by workers may be influenced by the type of pollen consumed by nurse bees (Pattamayutanon et al., 2018). Because of their food sharing relationship, the queen's health relies heavily on her worker retinue, and it is hypothesized that her performance is influenced both by the quantity and quality of the diet she receives (Dolasevic et al., 2020). Thus, her egg-laying is controlled by worker physiology (Avni et al., 2014; Degrandi-Hoffman et al., 2018; Fine et al., 2018) and worker response to the queen is modulated by nutritional stimuli (Walton et al., 2018).
Here, we hypothesized that the nutritional resources, particularly pollen, present in an extensive corn/soybean agroecosystem during the critical late summer period (Dolezal et al., 2019b) affect honey bee queen egg production, an important facet of honey bee health. To test this hypothesis, we performed a large on-farm field experiment, replicated over three separate years, where we tracked queen egg laying in full-sized honey bee colonies kept in a corn/soybean landscape. Then, during the critical late-season period, we manipulated colony locations, providing half of our colonies access to native (restored prairie) foraging habitat. During these experiments, we also quantified pollen collection by the colonies and identified pollen sources. Finally, we used the pollen collected in the field experiments to create representative dietary mixtures, which we then used in laboratory experiments to test how consumption of these diets affected queen egg production under highly controlled and replicated laboratory conditions.
Methods
Colony level field experiments
Colony creation
Colonies used in the field study were started from packaged bees (≈0.91 kg bees and a reproductive Apis mellifera ligustica queen) in May 2017. The bees used to make packages were derived from a local stock (Spring Valley Honey Farm, Perry Iowa), and the queens were sourced from Jackie Park-Burris Queens Inc. Palo Cedro, California, and were marketed as “Italian” queens. Bees were transferred to standard Langstroth 10 frame hive bodies with a mixture of undrawn and fully drawn comb and were managed at the Iowa State University (ISU) Bee and Wasp Research Apiary, Horticultural Research Station, Ames Iowa. After 2 weeks, each colony was weighed and inspected to ensure that the queen was actively laying eggs. Colonies were randomized and distributed to experimental soybean fields (see below) such that each field received an apiary of approximately the same average weight (i.e., equipment, honey, pollen, and bees) (13.36 kg ± 0.51 SEM).
In 2018 and 2019, the same procedure from 2017 was repeated, with the exception that bees to start apiaries in 2018 were purchased as five frame nucleus colonies (containing frames with developing pupae) from a provider in Minnesota, and the bees to start colonies in 2019 were shaken as 0.91 kg packages from surviving overwintering bees from 2018. For both years, queens in colonies were sourced from the same queen breeder as in 2017. In 2018 and 2019, average weight of colonies in apiaries was 26.46 kg ± 0.22 SEM and 19.21 kg ± 0.69.
Site selection
To quantify queen egg laying within colonies in agricultural fields, we selected two soybean fields in central Iowa during the summers of 2017 and 2018, and one soybean field in 2019 (i.e., five total fields) that were on average 37.8 ha (range 27.2–44.8 ha) and were located at least 3.2 km apart (Supplementary Figure S2, Supplementary Table S1). Fields were managed by Iowa State University and were planted with aphid resistant seed variety (IA2010-RA12, Rag1 + Rag2). There were no seed applied insecticidal treatments and no foliar applications of insecticides occurred throughout the season. Pre-emergent weeds in fields were managed with clethodim (Clethodim 2E, Albaugh LLC, Ankeny IA), fomesafen (Flexstar, Syngenta, Wilmington, DE), and post-emergent weeds were managed with spot treatments using a backpack sprayer containing clethodim (Clethodim 2E, Albaugh LLC) and lactofen (Cobra, Valent, Walnut Creek CA).
To minimize any effects of early season agrochemical exposure on our honey bee colonies, each soybean field received an apiary of 16 colonies split across two sub-sites, placed only after the pre- and post-emergence herbicides were applied (2 June 2017; 8 June 2018; 7 June 2019). Sub-sites within a field were at least 150 m from the closest field edge and 300 m from the adjacent sub-site. Colonies remained in soybean fields until the critical timepoint in the second week of August, when we then randomly selected half the colonies from each soybean subsite (n = 4 per subsite; n = 8 per field), and then randomized and relocated them to one of three restored prairie locations in the Chichaqua Bottoms Greenbelt in Polk County, Iowa. Each year this move to prairie occurred on 10 August 2017; 9 August 2018; 12 August 2019. We chose to keep all the colonies in soybean prior to August to ensure that all colonies experienced the same environment prior to our late season assessment of queen egg laying in prairies compared to agricultural sites. The remaining colonies (n = 8 per field) stayed in the soybean fields in their respective sub-site locations. This resulted in prairies with 5–6 colonies present and placed 60 m inside the prairie.
Prairies were on average 35.88 ha (range 20.23–55.85 ha) and located at least 3.2 km from one another (Supplementary Figure S2, Supplementary Table S1). During our study, none of our experimental prairies or prairies bordering experimental prairies were burned or mowed. Because soybean fields in Iowa are rotated annually, new independent soybean fields were located each year, however, the same restored prairies were used in 2017–2019. For logistical considerations, in 2019 the experiment was scaled back such that we only had one soybean field and only moved colonies into two of the three prairies from the prior years.
Colony management
Once colonies were moved into soybean fields, they were inspected every other week following methods from Dolezal et al. (2019b) and St. Clair et al. (2020). At each inspection, we determined presence of the queen by either locating the queen or observing newly laid eggs. If queen presence was absent, the colony was provided a new Apis mellifera ligustica queen within 3 days. Queen excluders, which restrict the queen from accessing additional colony space but allow workers to move freely, were added if more than two hive bodies were necessary, restricting the production of brood to the bottom two hive bodies but allowing for continued storage of honey. Throughout the experiment brood production did not surpass the needs of the two deeps provided. We assessed the Varroa mite levels (Varroa destructor) within colonies via the alcohol wash method monthly (Dietemann et al., 2012). Mite levels remained below treatment threshold (1%) in June and July. In August of each year, colonies were treated with thymol (Apiguard, Dadant and Sons Inc.) regardless of mite levels, to prevent potential late-season mite infestation from confounding the effects of post movement landscape treatment (Dolezal et al., 2016). Specific mite levels across the months and years can are in Supplementary Figure S1.
Queen egg laying
We quantified queen egg laying within colonies by carefully locating the queens inside colonies once per month in June, July, and August. In June and July, all colonies were within soybean fields and assessments provided a baseline for queen egg laying as well as established that the queens in colonies chosen to go to prairie in the late season did not differentially lay eggs compared to soybean prior to their movement. For the August assessment, colonies had been placed in prairies 8 days prior to queen assessment. At each assessment, once queens were located, they were caged to a single empty frame side within her colony. Cages were constructed of queen excluder and sized to fit one frame side of a standard Langstroth deep frame such that queens were unable to escape, but workers could still enter and interact with queens as normal (Figure 1A). If an empty frame was not available within the focal colony, a clean, previously frozen external frame of empty drawn comb was provided. Cages remained in place for 48 h, after which queens were returned to colonies and eggs were estimated by modified methods from Wu-Smart and Spivak (2016). On each frame, we counted eggs in eight parallelograms placed across the frame (Figure 1B). Each parallelogram contained 100 cells resulting in a total of 800 possible eggs counted, from which a proportion of cells filled value can be calculated.
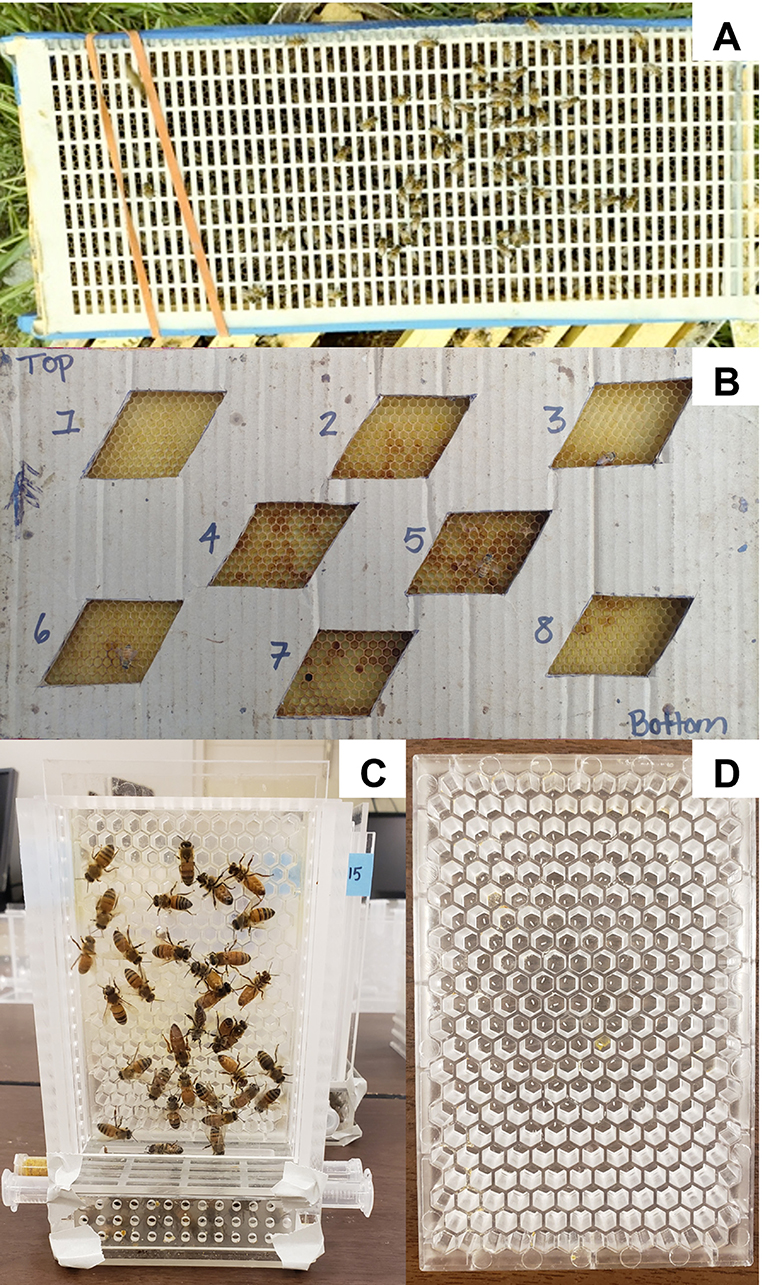
Figure 1. (A) Cage constructed of queen excluder used to contain a queen to a single frame side within the colony. (B) Parallelogram (100 cells per each) used to monitor queen egg laying across the frame. (C) Queen monitoring cage (QMC) used to run laboratory assays and (D) egg laying plates (ELP) that are inserted into QMCs.
Pollen collection and identification
To compare the pollen composition at soybean and prairie sites during the late season, we placed wooden pollen traps over the entrance on eight of the 16 colonies (four per sub-site) in each soybean field. Traps were installed on colonies in June and remained throughout the experiment to allow colonies to adjust to its presence prior to our assessments, but were not engaged (i.e., the bees did not have to pass through the trap restriction and no pollen was removed from them). When we chose colonies to move to the prairie in August, we ensured that four of the eight colonies with pollen traps were randomly selected. Pollen traps were engaged during August over a 24-h period twice monthly in 2017 and 2018, and once in 2019.
Pollen was then brought back to the lab and stored in a −20°C freezer for further analysis. Total pollen collected by each colony at each timepoint was weighed and then sorted by pellet color; each color group was then weighed again. Approximately four pellets from each color category were then crushed in one drop of ethanol, stained, and slide mounted for palynological analysis. In short, pollen was stained using modified methods from Kearns and Inouye (1993) by mixing 7 g unflavored gelatin, 24 ml deionized water, 21 ml Fisherbrand Glycerin, and ≈0.1g of Alfa Aesar crystalline Basic fuchsin which would serve as the base for all our slides. The basic fuchsin jelly was then transferred onto clean slides and the crushed pollen brushed upon the fuchsin jelly and covered with a coverslip. To melt the jelly and stain the pollen grains, a lighter was held 3–6 cm from the underside of the slide. Once the edges melted, the flame was removed, and the coverslip was smoothed to an even consistency.
Pollen was initially identified by light microscopy using the dichotomous key Kapp's guide to pollen and spores (Kapp, 2000). Determinations were then verified using Paldat.org and the Global Pollen project (Martin and Harvey, 2017; PalDat [WWW Document], 2022). As a secondary verification of pollen identifications, reference slides of each species determination were created from fresh pollen collected from flowers identified in the field using Newcomb's wildflower guide where available, and from Illinois Natural History Survey ILLS herbarium specimens (Illinois Natural History Survey Herbarium ILLS; Supplementary Table S2) if unavailable locally. Reference slides were created by touching pre-made slides to the anthers of flowers and staining as above. Lastly, determinations were confirmed as appropriate with geographical area and seasonality. Occasionally, a pellet color contained more than one species of pollen grain, therefore, determinations were the grains that were predominant.
Laboratory queen monitoring cage experiments
Pollen preparation
To assess whether pollen source was a significant contributor to honey bee queen egg laying in our field experiments, we used pollen collected with the traps in the field experiment described above to run highly-controlled laboratory experiments. We chose to replicate the nutritional environment of August 2017 (both collection dates combined), a year we observed significantly higher queen egg laying in prairies compared to soybean (Figure 2). To replicate the nutritional environment, we assessed the composition and proportion of taxa collected in each treatment (Figure 3A, Table 1). We then aimed to create three experimental pollen diets representative of the pollen collected in the field. To do this, from the same source material, we produced a mixture of the diet of colonies kept in soybean and prairie habitats, and we were able to produce ≈150 g of each diet. These diets each contained a mixture of multiple pollen varieties based upon the data from our field experiments, using color sorted pollen collected across all the fields over 2017–2019 and stored in the freezer at −20°C (Table 1). Thus, we labeled these diets as (1) polyfloral soybean and (2) polyfloral prairie mixtures. In 2017, we also observed evening primrose (Oenothera biennis) to be the only pollen which was significantly more abundant in prairies compared to soybean (Figure 3A). To better understand the contribution of evening primrose to increased queen egg laying in prairies we added an additional treatment of 100% evening primrose which was referred to as (3) O. biennis.
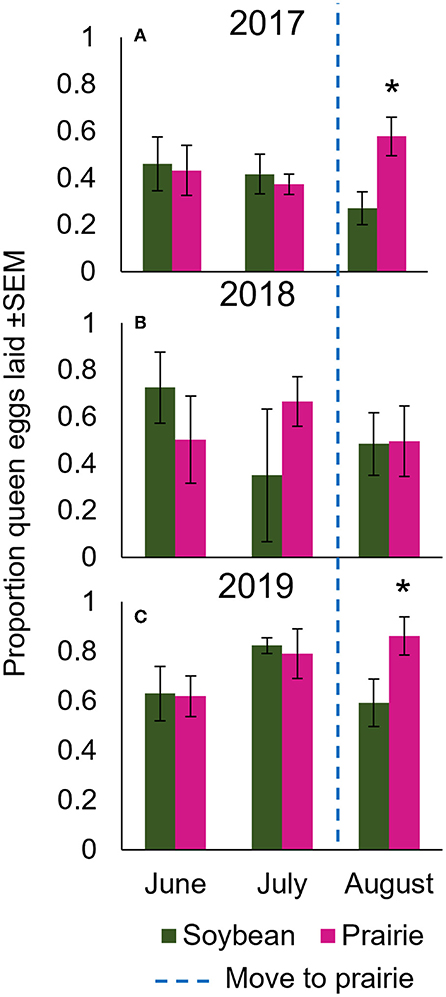
Figure 2. Post-movement (August) egg laying differed significantly in 2017 and 2019. Mean proportion (out of 800 total cells) eggs laid by the queen in colonies kept either in soybean fields or restored tallgrass prairies in central Iowa over the summers of (A) 2017, (B) 2018, and (C) 2019. In June and July, all colonies were kept in soybean fields, however, groups were separated by landscape treatment to demonstrate that there was no significant pre-existing difference in egg laying prior to being introduced to the prairie in August. In August, half the colonies in soybean fields were moved to a restored tall grass prairie as noted by the dotted blue line. Asterisks represent significance between soybean and prairie at specific months; alpha 0.05.
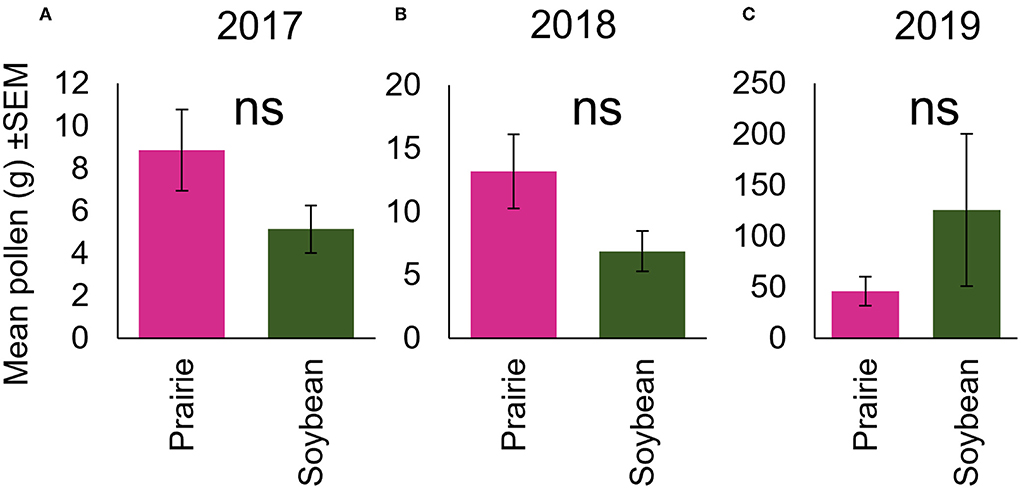
Figure 3. Collection of August pollen mass did not differ by landscape treatment in any year. Average of the total grams of pollen collected by colonies in either the prairie or soybean landscape during the month of August, the time point after colonies had undergone movement treatment in (A) summer 2017, (B) 2018, and (C) 2019.
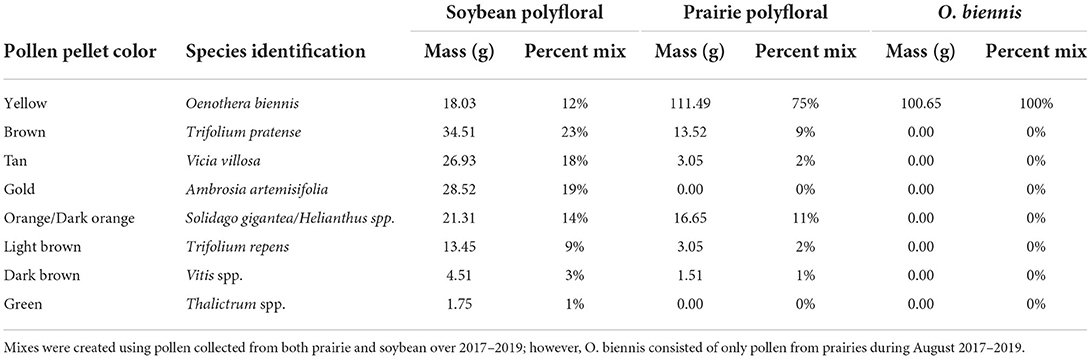
Table 1. Proportion of each pollen type used to create a polyfloral soybean and prairie mix that is representative of pollen collected by honey bee colonies, as well as a representative of the most abundant species (Oenothera biennis) collected in prairies in August 2017.
To prepare the three pollen treatments (polyfloral soybean, polyfloral prairie, and O. biennis) for use in the queen monitoring cages (QMC), we ground pollen in a mechanical coffee grinder and then added 30% by weight of heavy syrup to the pollen and mixed thoroughly. Heavy syrup was created by dissolving 2:1 granular white sugar in sterilized water. Thus, for each diet we produced a homogenized pollen patty paste, from which small, identical aliquots could be obtained for feeding to our experimental bees. The pollen mixtures were then stored at −20°C until use in QMCs.
Bee source and queen monitoring cage setup
Bees used for laboratory experiments were sourced from the Bee Research Facility at the University of Illinois Urbana-Champaign, Urbana Illinois. Naturally mated Italian queens (A. mellifera ligustica) were sourced from Jackie Park-Burris Queens Inc. as in field experiments above. In July 2021, a wax comb frame containing capped worker pupae was obtained from approximately five random colonies maintained according to standard commercial methods. Frames were then placed in an incubation chamber at 33.5°C until adult eclosion. Newly eclosed worker bees (<24 h old) were brushed from frames as they emerged and mixed prior to adding to QMCs (described below) to ensure a random distribution of bees from different colonies throughout the cages.
Queen cages were based on a modified design of Fine et al. (2018). In short, cages were composed of ventilated plexiglass where the queen was excluded from four feeding ports, which each housed a 2 ml microcentrifuge feeding tube, via queen excluder material (Figure 1C). This ensured that any food she received had been transported by workers. She was restricted to the laying arena which consisted of two egg laying plates (ELP; Figure 1D) positioned vertically and served as the inner walls of the cages. These injection-molded, polystyrene plates were patterned with 264 hexagonal wells which mimic the dimensions of the cells in natural honey bee brood comb and do not require beeswax substrates which are often contaminated with agrochemical residues (Mullin et al., 2010; Traynor et al., 2021b). During these experiments, we observed no evidence that workers deposited sucrose solution into the cells, from which the queen could consume food directly.
To assess whether the pollen treatments influenced queen egg laying, we set up 15 cage replicates for each of the three diet treatments, for a total of 45 QMCs. Throughout the experiment, cages were kept in an environmental chamber at 33.5°C and 50% relative humidity. Treatments were randomly placed within the chamber and rotated daily to avoid temperature and humidity fluctuations or pockets. To each cage we added 7 g of adult, newly eclosed bees, ≈70 individuals. We then used CO2 to anesthetize the queen and added her to the laying arena. Queens were anesthetized for <1 min. Anesthetizing the queens with CO2 ensured they were introduced without harm. Each of the cages received one feeding tube of sterile water and two feeding tubes of 50% sugar solution. Prepared pollen was delivered in a 2 ml microcentrifuge tube with the end removed. Water, sucrose, and pollen were provided ad libitum and replaced fresh daily. We did not assess the quantity of pollen consumption in the cage trials. The experiment was run for 10 days, and each day, queen egg laying was assessed by removing the ELPs, counting the eggs laid, and replacing a fresh ELP within the cage. Queen mortality was monitored and recorded throughout the experiment.
Statistical analysis
Queen egg laying in the field
Because we had independent soybean fields each year, and the pollen composition of those fields could have varied, but prairies were consistent, we chose to assess queen egg laying for each year separately. During June and July, colonies are compared by “landscape” treatment, even though, during those times, they were all located in soybean fields, i.e., these timepoints occurred before the colony movement treatment. Performing this comparison provides a baseline egg laying comparison for the treatment groups before colony movement, which we do not expect to differ significantly prior to their movement to prairies in August.
To compare egg laying, we created a mixed model analysis of variance (PROC GLIMMIX) in SAS 9.4 with proportion eggs laid (out of 800 total cells counted) as the response variable and landscape treatment (soybean and prairie), month (June, July, and August), and their interaction as predictor variables. Colonies nested within sub-site within a field were used as the random factor within the model. We performed a post-hoc comparison of least squared means with a Tukey HSD adjustment to identify significant differences between treatments at each month. Because the objective of the experiment was to assess the effects of diverse prairies on queen egg laying compared to monoculture soybean fields, and because colonies did not arrive in prairies until August, we chose to run an additional analysis assessing the variation in queen egg laying by landscape treatment for the month of August separately.
Pollen collection in the field
Using a similar model as above, we compared the total pollen collected in August by colonies in soybean compared to prairie locations. We used grams of pollen as the response variable, treatment as the predictor variable, and colony nested within sub-site within a field as the random factor. Additionally, we compared the grams of each individual pollen species collected by landscape treatment within a year using the same model.
Queen egg laying in QMCs
To compare queen egg laying in the QMCs we created a repeated measures mixed model ANOVA (PROC GLIMMIX) in SAS 9.4. Total eggs laid over the 10-day experiment was the response variable and pollen treatment (polyfloral soybean, polyfloral prairie, and O. biennis), trial day, and their interaction were the predictor variables. Cage was considered the random factor. If there was a significant interaction of variables, we used post-hoc analysis of least squared means with Tukey HSD adjustment to compare pollen treatments at individual dates.
Results
Honey bee queen egg laying
In our statistical comparison of queen egg laying that included both pre-transportation (June and July) and post-transportation (August) months, we did not observe an overall effect of landscape treatment on queen egg laying in 2017 (F1, 39.64 = 2.34, p = 0.13), and egg laying did not vary by month (F2, 38.24 = 0.10, p = 0.91). This is not surprising, given that this analysis includes data from colonies both before and after the movement treatment; we did not expect to see a difference in “landscape” treatment in June and July because all colonies were in all soybean. There was, however, a significant interaction of landscape and month (F2, 37.98 = 3.48, p = 0.04) where queens laid significantly more eggs in colonies in prairie compared to those in soybean in the post-movement month of August (T39.29 = 3.19, p = 0.003), while egg laying did not differ between landscape treatments in the pre-movement months of June and July (T39.35 = 0.02, p = 0.98, T39.74 = 0.18, p = 0.86 for June and July, respectively). In the analysis examining August only, queens in colonies housed in prairies laid significantly higher quantities of eggs compared to those in soybean fields (F1, 8.22, p = 0.005).
In 2018, we did not observe an overall effect of landscape treatment on queen egg laying (F1, 18.96 = 0.00, p = 0.95), egg laying did not vary by month (F2, 13.61 = 0.19, p = 0.83), and no interactions of treatment and month occurred (F2, 15.11 = 1.65, p = 0.23). At individual months, we did not observe a difference in queen egg laying in colonies housed in prairies compared to soybean (T15.09 = 0.81, p = 0.43; T19 = 1.42, p = 0.18; T15.88 = 0.68, p = 0.51 for June, July, and August, respectively).
In 2019, there were no overall effects of landscape treatment on queen egg laying (F1, 11.89 = 0.82, p = 0.38), egg laying did not vary by month (F2, 19.63 = 1.81, p = 0.19), and there were no interactions of landscape treatment and month (F2, 19.63 = 1.72, p = 0.21). A post-hoc analysis revealed that in August, queens laid marginally significantly more eggs when in prairies compared to soybean (T26.91 = 1.99, p = 0.06), while there were no differences in egg laying in June or July (T26.84 = 0.12, p = 0.91; T26.91 = 0.21, p = 0.83 for June and July, respectively). In the analysis of August only (post-movement), queens in colonies in prairies laid significantly more eggs than those in soybean fields (F1, 7 = 5.40, p = 0.05).
Pollen collection and identification from field experiment
Over the 3-year period we did not observe a significant difference in the total abundance in grams of pollen collected by colonies in the soybean fields compared to those in the prairies in any year (F1, 10.48 = 0.87, p = 0.37; F1, 8 = 2.65, p = 0.14; F1, 4.71 = 2.19, p = 0.20 for 2017, 2018, and 2019, respectively) (Figures 4A–C). In 2017, pollen abundance varied by plant species (F11, 357.6 = 9.27, p = <0.0001), and there was a significant interaction between landscape treatment and pollen species collected (F11, 357.6 = 4.81, p = <0.0001) (Figure 3A). Specifically, evening primrose (Oenothera biennis) was collected in significantly higher amounts in prairie compared to soybean habitats (T202.6 = 6.53, p = <0.0001; Figure 3A, Supplementary Table S3).
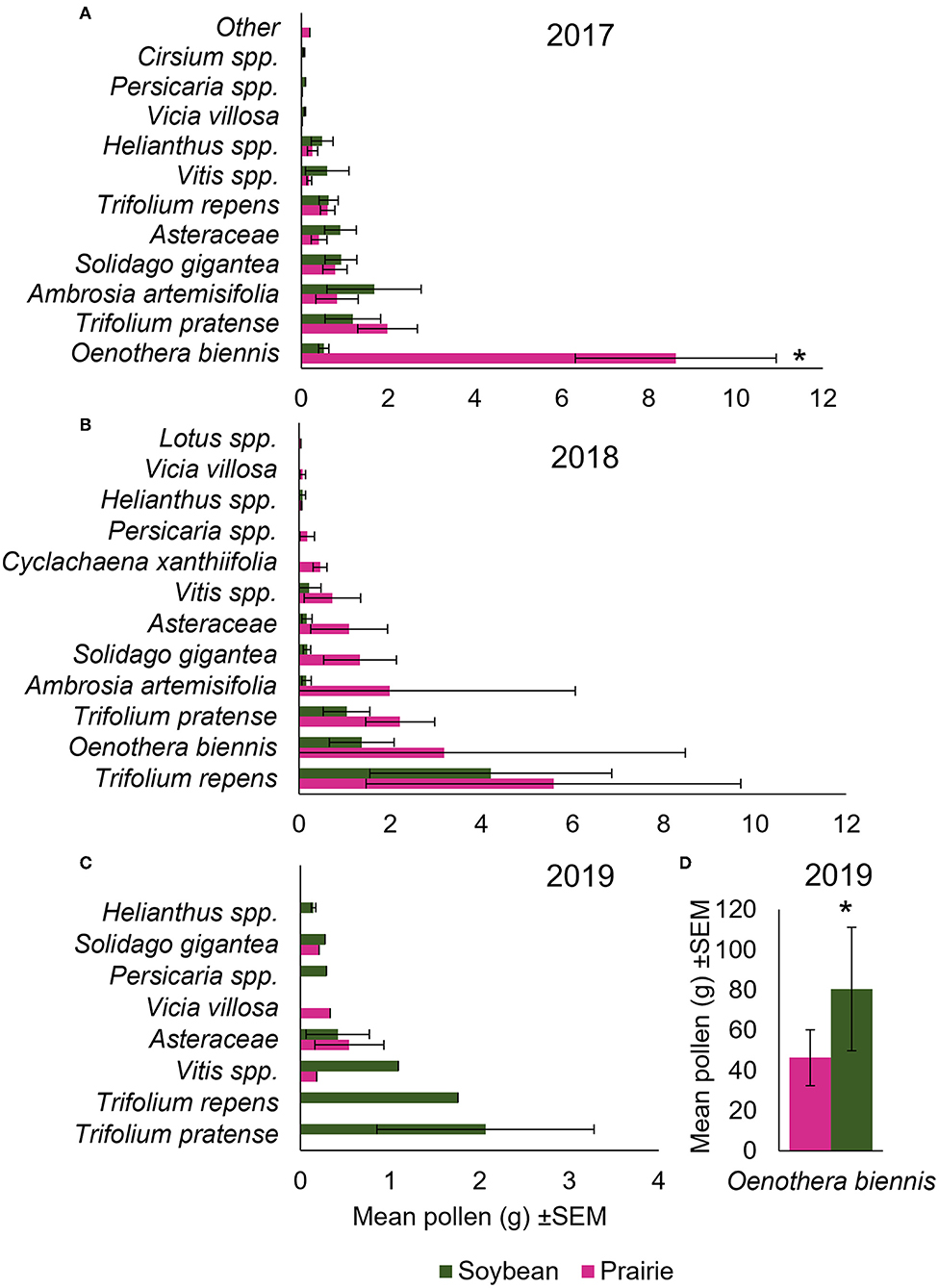
Figure 4. Pollen identity differed due to landscape treatment in 2017 and 2019, but not 2018. Average pollen collected by floral species from colonies in either prairie (pink) or soybean (green) landscapes during the month of August in the summer of (A) 2017, (B) 2018, and (C) 2019, with (D) showing a separate plot of O. biennis, which was collected at very high levels in 2019. Asterisks represent significance in abundance of pollen collected between soybean and prairie; alpha 0.05.
In 2018, pollen collection varied significantly by species collected (F11, 88 = 8.06, p = <0.0001) (Figure 3B), but there was not a significant interaction between landscape treatment and pollen species (F11, 88 = 0.62, p = 0.81) (Figure 3B) and no individual species varied in abundance collected between soybean and prairies (Supplementary Table S4). In 2019, pollen collection varied significantly by species collected (F11, 56.65 = 106.98, p = <0.0001) and there was a marginally significant interaction between landscape treatment and pollen species collected (F11, 56.65 = 1.84, p = 0.07) (Figure 3C). Contrary to what we observed in 2017, we found that evening primrose (O. biennis) was collected in significantly higher amounts in soybean compared to prairie (T55.09 = 4.14, p = 0.0001; Figure 3C, Supplementary Table S5)—a significant difference in the opposite direction. While not analyzed statistically, the mass of O. biennis collected in 2019 was ≈10 times higher than in 2017.
Queen egg laying in QMCs
When comparing the total eggs laid over the 10-day in lab queen monitoring assay, there was a significant effect of pollen diet on queen egg laying (F2, 310 = 4.68, p = 0.01; Figure 5A). Queens fed the prairie polyfloral mix laid significantly more eggs than those fed the O. biennis (T310 = 2.70, p = 0.007) and those fed the soybean polyfloral mix (T310 = 2.61, p = 0.01), while the queens fed the O. biennis and soybean polyfloral mixes did not differ significantly in egg laying (T310 = 0.15, p = 0.88) (Figure 5A). Queen egg laying varied significantly across trial days (F9, 310 = 31.44, p = <0.0001) and there was a significant interaction between pollen treatment and trial day (F18, 310 = 1.69, p = 0.04) (Figure 5B). Although the daily effects of trial day were not always consistent, overall, we observed that queens fed O. biennis pollen did not lay as many eggs early on compared to the polyfloral diets (days 2 and 3) (Figure 5B, Supplementary Table S6). However, by day 6, queens fed both prairie diets were laying significantly more eggs than queen fed the soybean sourced diet (Figure 5B, Supplementary Table S6).
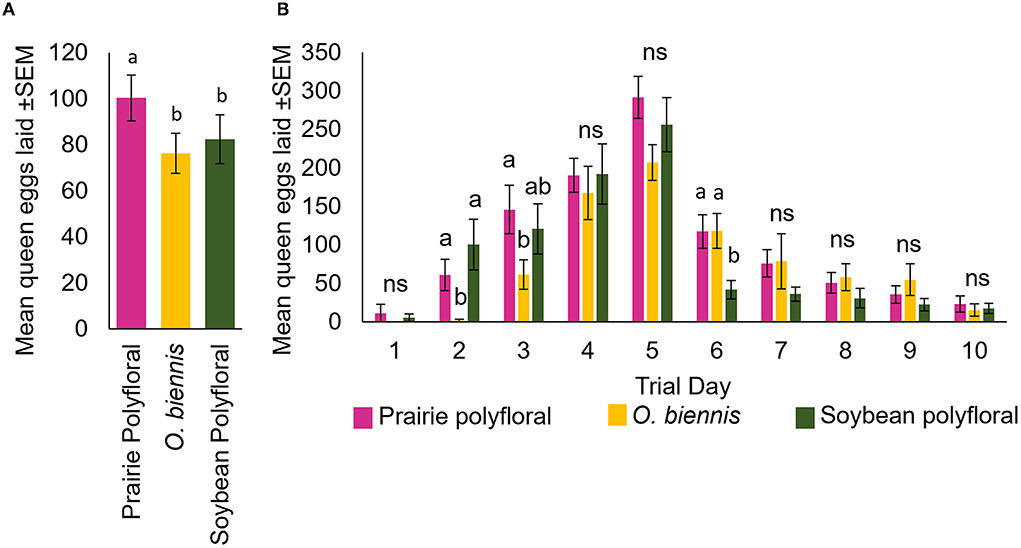
Figure 5. Queens from cages fed the prairie polyfloral diet laid significantly more eggs than other treatments. (A) Mean total queen eggs laid over the 10-day period within each pollen treatment. (B) Mean daily eggs laid by queens in QMCs fed either a polyfloral prairie (pink), O. biennis (yellow), or polyfloral soybean (green) pollen mix. Letters represent the post-hoc comparison of treatments within each trial day, alpha 0.05.
Discussion
Overall, we offer evidence that providing honey bee colonies access to prairie habitat during a critical period of forage dearth in a broadly cultivated corn/soybean agroecosystem can have differential effects on queen egg production. Furthermore, we provide one of the first demonstrations that the pollen derived from those habitats results in similar patterns of egg laying in highly controlled laboratory experiments. Previous work shows that honey bee colonies kept in these landscapes grow through the summer, decline sharply after clover and soybean bloom ceases, but these effects can be rescued by providing them with access to diverse, native vegetation (Dolezal et al., 2019b; St. Clair et al., 2020). While those studies showed effects on colony mass, population, and worker health, they did not evaluate effects on queen productivity. Queens derive their nutrition solely from workers and manipulating the diet of workers in laboratory experiments affects queen egg production (Fine et al., 2018; Fine, 2020). However, there are few, if any, studies that clearly pair field and laboratory experiments as we describe here.
In our field experiments, we found that, after colonies had been moved from solely agricultural areas to those with prairie habitat, colonies in prairie habitats showed greater queen egg production. However, this was observed only in 2017 and 2019, with 2017 producing the most pronounced differences (Figure 2). In 2018, we observed no significant differences in egg laying due to landscape treatment (Figure 2). As is the nature of field experiments, many factors differ between years that could have contributed to the variation in egg laying patterns, including site variation and weather (Zhang et al., 2021). To better understand these trends, we looked at the quantity and source of pollen collected by the colonies. Across all years, the average quantity (mass) of pollen collected by colonies in soybean vs. prairie habitats in August did not differ (Figure 4). An important caveat to note is that different size colonies can collect varied pollen quantities (Beekman et al., 2004). We did not account for colony size in our statistical analysis. However, we attempted to reduce variation in pollen collected across colonies resulting from colony size by carefully controlling the initial mass of colonies and placing them in the same habitat (i.e., soybean) up until movement to prairie and initial collections of pollen.
The types of pollen collected (i.e., plant species) between soybean and prairie were also similar, with the abundance of each not varying, except for evening primrose (O. biennis) (Figure 3). This pollen was collected at different levels in 2017 and 2019, though in opposite directions—it was collected more in prairie habitats in 2017 and soybean habitats in 2019. While we did not analyze the differences statistically, in 2019, colonies in both habitats collected ≈10 times more pollen overall compared to 2017 and 2018, which was driven primarily by great collection of O. biennis. From this we hypothesized that O. biennis may play an important nutritional role in egg production when combined with other polyfloral mixes of native prairie forbs. Based on the differential collection of O. biennis across the years and landscapes, we predicted that there could be a threshold for abundance needed to allow these hypothesized effects. For example, in 2017 colonies collected at least 8 g of O. biennis in prairies but only 2 g in soybean, this threshold may lay somewhere between 2 and 8 g, therefore contributing to significant effects of egg laying in prairie compared to soybean (Figure 3). In 2018, colonies in each site type each site type collected <4 g of O. biennis potentially limiting the added benefit of O. biennis in a polyfloral prairie diet (Figure 3). In 2019, colonies in both landscape types collected well over 8 g of O. biennis, meeting the potential threshold, possibly allowing added benefits to egg laying in the prairielandscape in 2019.
To better understand these trends and test this hypothesis, we performed a set of controlled laboratory experiments using QMCs to test the effects of different pollen diets derived from our field experiments; a polyfloral mix of prairie, a polyfloral mix of soybean, and a monofloral diet of O. biennis. In the lab, we found the same patterns as in the field, with the polyfloral prairie pollen resulting in greater queen egg production compared to the other two groups (Figure 5). Notable is the fact that a diet of O. biennis alone did not result in greater egg production, suggesting that this pollen does not provide the nutritional profile needed to boost queen fecundity. Likely, a polyfloral mixture, with a large proportion of O. biennis, is required to produce the greatest outcome. Polyfloral mixtures have been shown to benefit several other different bee health traits, including responses to several different pathogens (Di Pasquale et al., 2013; Dolezal et al., 2019a; Zhang et al., 2020; Branchiccela et al., 2021), and it is still unclear what dietary components are most likely able to affect different responses (Wright et al., 2017). Understanding the role different nutritive agents play in honey bee diets is challenging, as many phenotypes are complex, and diets can vary widely. For example, pollens that improve response to pathogens can exhibit differences in macronutrients, like lipid and protein content (Di Pasquale et al., 2013) as well as micronutrients like calcium and iron (Dolezal et al., 2019a). Further, pollen from the same plant species can differ in nutritive content depending on its growth environment (Ziska et al., 2016). In the real world, pollen and nectar are also contaminated with highly variable levels of agrochemicals and other pest-control agents (Long and Krupke, 2016; Traynor et al., 2021a) which can also affect workers, how they care for the queen, and ultimately queen egg production (Wu-Smart and Spivak, 2016; Fine, 2020).
In our field experiment, which involved both data collection and collection of the raw pollen material for the laboratory experiments, we did not directly quantify pesticide residues in our collected pollen. However, we controlled pesticide applications at our focal sites. During June and July, all colonies experienced a similar environment, with the movement to prairies only occurring in August. When in soybean, all colonies experienced identical exposure to herbicides, and no insecticides were used as part of the soybean seed treatment or in foliar applications to the fields. No pesticides were applied at all in the prairies. Therefore, we are confident that our colonies did not experience any direct exposure to insecticides or differential exposure to herbicides due to applications at our apiary sites themselves. However, honey bees forage for long distances, regularly traveling 1–2 km and beyond (Couvillon et al., 2014, 2015; Carr-Markell et al., 2020), and they collect resources from many different locations. Therefore, it is possible that colonies could have experienced different pesticide exposure through foragers traveling offsite and returning with contamination on their bodies or in the pollen and nectar they collected. Honey bee colonies are known to be exposed to a wide variety of agrochemicals in this way, though they are often at very sublethal levels (Traynor et al., 2021a). While honey bee queens appear to be very resilient to some direct agrochemical exposure (McAfee et al., 2021), and pesticide-exposed workers are somehow able to protect the queen by producing mostly contaminant-free royal jelly (Böhme et al., 2018; Milone et al., 2021), pesticide exposure does affect royal jelly nutrient composition (Milone et al., 2021) and colony-level sublethal pesticide treatments can affect egg production (Wu-Smart and Spivak, 2016). Pesticide exposure is likely widespread, though intermittent, and the effects on queens and reproductive physiology are poorly understood. Even carefully quantifying pesticides levels in pollen would only provide a brief snapshot of how a colony is exposed to pesticides and may not explain the effects we observed. At this stage, we only can clearly show that diets from these environments are associated with differences in queen productivity, but we do not yet know the source or mechanism of these differences. Future work will need to combine field and lab experiments to better understand the interplay between nutrition and pesticide exposure and how they combine to affect queens.
From a technical perspective, this is the first study to perform parallel experiments in the field and the QMC system. The QMCs were developed recently as a tool for manipulating queen and worker environments in a completely controlled setting, free from variation in egg laying substrate and background pesticide levels. Other work has used natural comb, which often contains pesticide residues (Calatayud-Vernich et al., 2018), is more difficult to standardize, and is more difficult to procure. Additionally, pesticide residues can result in significant negative effects on both developing workers and queens (Wu et al., 2011; Milone and Tarpy, 2021), which can cloud the potential benefits of nutrition. The QMC tool has proved useful for evaluating the effects of diet (Fine et al., 2018), neonicotinoids (Fine et al., 2017), and insect growth regulator (Fine, 2020) effects on egg production and queen health, but never before has a treatment directly derived from a field study been used. Here, we find that the QMCs appear able to recapitulate the results of the field experiment, showing that a pollen diet derived from field colonies produces the same patterns of egg laying in QMCs as it does in the field.
Interpreting the results of queen egg laying and how it plays into overall colony health likely requires a more nuanced investigation than what we supply here, as queen egg laying is only one aspect of colony reproduction. These results are compelling and provide direct evidence that nutritional environment can influence a queen's fecundity, indicating a change in queen health. A healthy queen's capacity to lay eggs likely far exceeds the colony's ability to raise them, with the normal homeostasis of functioning colonies being that queens lay a surplus of eggs and excess are cannibalized through oophagy. This process results in brood rearing as a colony trait regulated collectively by the workers rather than the queen. In this study we quantified the potential fecundity of the queens but not the actual fecundity of their colonies. Future studies combining full scale colonies or the QMC system should track egg survival for a more accurate representation of colony level fecundity. Rather than a simple conclusion that nutritional diet alters queen fecundity through egg laying, a more accurate inference of our results may be that queens and their colonies are sensitive to their nutritional environment and likely adjust their regulation of brood production accordingly. In other words, here we are unable to distinguish between queens laying more eggs or workers performing less oophagy, both in the field and in the cage trial. Thus, we do not know if our results are due to a direct effect (i.e., colonies or cages of workers fed polyfloral prairie pollen resulted in more queen eggs laid), or an indirect effect (i.e., queens always laid a maximum number of eggs but colonies or cages with better pollen were less constrained nutritionally and thus performed less oophagy). Future work should aim to better tease apart these differences.
In conclusion, our study provides new evidence that the foraging landscape bees encounter is clearly associated with the colony egg production. Previous work showed that landscape composition can affect several colony growth and health metrics (Dolezal et al., 2019b; St. Clair et al., 2020), including bee population. The data we provide here suggests that these differences could be generated very early on, with queens simply laying fewer eggs, or colonies rearing fewer eggs to adulthood. While more work will be needed to tease apart the specific drivers of these differences, understanding how egg production is regulated will be useful for designing landscapes for sustainable pollinator management and can inform feeding regimes for beekeepers.
Data availability statement
The original contributions presented in the study are included in the article/Supplementary materials, further inquiries can be directed to the corresponding author/s.
Author contributions
AS and AD procured funding for, designed the experiment, and wrote the manuscript. AS conducted the field and laboratory trials and conducted all statistical analyses. SS identified pollen species. All authors contributed to manuscript revisions and approved of the final draft.
Funding
This project was made possible through funding from USDA-NIFA grant 2016-07965, USDA-FFAR grant 549025, USDA-WFD 2021-67034-35004, and a student research award through the Eastern Apicultural Society.
Acknowledgments
We would like to thank Iowa State University and the Chichaqua Bottoms Greenbelt for allowing us to conduct research on their grounds. We would also like to thank the many members of the Toth lab at Iowa State University and Dolezal lab at University of Illinois Urbana-Champaign for assisting in field data collection, pollen sorting, and checking of QMC cages in the laboratory. We would like to the reviewers and journal editor for providing thoughtful and useful critiques that improved the manuscript.
Conflict of interest
The authors declare that the research was conducted in the absence of any commercial or financial relationships that could be construed as a potential conflict of interest.
Publisher's note
All claims expressed in this article are solely those of the authors and do not necessarily represent those of their affiliated organizations, or those of the publisher, the editors and the reviewers. Any product that may be evaluated in this article, or claim that may be made by its manufacturer, is not guaranteed or endorsed by the publisher.
Supplementary material
The Supplementary Material for this article can be found online at: https://www.frontiersin.org/articles/10.3389/fsufs.2022.908667/full#supplementary-material
References
Alaux, C., Allier, F., Decourtye, A., Odoux, J.-F., Tamic, T., Chabirand, M., et al. (2017). A “Landscape physiology” approach for assessing bee health highlights the benefits of floral landscape enrichment and semi-natural habitats. Sci. Rep. 7, 40568. doi: 10.1038/srep40568
Amiri, E., Strand, M. K., Rueppell, O., and Tarpy, D. R. (2017). Queen quality and the impact of honey bee diseases on queen health: potential for interactions between two major threats to colony health. Insects 8, 48. doi: 10.3390/insects8020048
Avni, D., Hendriksma, H. P., Dag, A., Uni, Z., and Shafir, S. (2014). Nutritional aspects of honey bee-collected pollen and constraints on colony development in the eastern Mediterranean. J. Insect Physiol. 69, 65–73. doi: 10.1016/j.jinsphys.2014.07.001
Bee Informed Team (2021). United States Honey Bee Colony Losses 2020–2021: Preliminary Results. Bee Informed Partnership. Available online at: https://beeinformed.org/2021/06/21/united-states-honey-bee-colony-losses-2020-2021-preliminary-results/ (accessed July 27, 2021).
Beekman, M., Sumpter, D. J. T., Seraphides, N., and Ratnieks, F. L. W. (2004). Comparing foraging behaviour of small and large honey-bee colonies by decoding waggle dances made by foragers. Funct. Ecol. 18, 829–835. doi: 10.1111/j.0269-8463.2004.00924.x
Belsky, J., and Joshi, N. K. (2019). Impact of biotic and abiotic stressors on managed and feral bees. Insects 10, 233. doi: 10.3390/insects10080233
Böhme, F., Bischoff, G., Zebitz, C. P. W., Rosenkranz, P., and Wallner, K. (2018). Pesticide residue survey of pollen loads collected by honeybees (Apis mellifera) in daily intervals at three agricultural sites in South Germany. PLoS ONE 13, e0199995. doi: 10.1371/journal.pone.0199995
Branchiccela, B., Castelli, L., Corona, M., Díaz-Cetti, S., Invernizzi, C., Martínez de la Escalera, G., et al. (2019). Impact of nutritional stress on the honeybee colony health. Sci. Rep. 9, 10156. doi: 10.1038/s41598-019-46453-9
Branchiccela, B., Castelli, L., Díaz-Cetti, S., Invernizzi, C., Mendoza, Y., Santos, E., et al. (2021). Can pollen supplementation mitigate the impact of nutritional stress on honey bee colonies? J. Apic. Res. 0, 1–9. doi: 10.1080/00218839.2021.1888537
Calatayud-Vernich, P., Calatayud, F., Simó, E., and Picó, Y. (2018). Pesticide residues in honey bees, pollen and beeswax: assessing beehive exposure. Environ. Pollut. 241, 106–114. doi: 10.1016/j.envpol.2018.05.062
Calderone, N. W. (2012). Insect pollinated crops, insect pollinators and US agriculture: trend analysis of aggregate data for the period 1992-2009. PLoS ONE 7, e37235. doi: 10.1371/journal.pone.0037235
Carr-Markell, M. K., Demler, C. M., Couvillon, M. J., Schürch, R., and Spivak, M. (2020). Do honey bee (Apis mellifera) foragers recruit their nestmates to native forbs in reconstructed prairie habitats? PLoS ONE 15, e0228169. doi: 10.1371/journal.pone.0228169
Chmiel, J. A., Daisley, B. A., Pitek, A. P., Thompson, G. J., and Reid, G. (2020). Understanding the effects of sublethal pesticide exposure on honey bees: a role for probiotics as mediators of environmental stress. Front. Ecol. Evol. 8, 1–19. doi: 10.3389/fevo.2020.00022
Couvillon, M., Riddell Pearce, F., Accleton, C., Fensome, K., Quah, S., Taylor, E., et al. (2015). Honey bee foraging distance depends on month and forage type. Apidologie 46, 61–70. doi: 10.1007/s13592-014-0302-5
Couvillon, M. J., Schurch, R., and Ratnieks, F. L. W. (2014). Waggle dance distances as integrative indicators of seasonal foraging challenges. PLoS ONE 9, e93495. doi: 10.1371/journal.pone.0093495
Crone, M. K., and Grozinger, C. M. (2021). Pollen protein and lipid content influence resilience to insecticides in honey bees (Apis mellifera). J. Exp. Biol. 224, jeb242040. doi: 10.1242/jeb.242040
De Souza, D. A., Huang, M. H., and Tarpy, D.R. (2019). Experimental improvement of honey bee (Apis mellifera) queen quality through nutritional and hormonal supplementation. Apidologie 50, 14–27. doi: 10.1007/s13592-018-0614-y
Degrandi-Hoffman, G., Gage, S. L., Corby-Harris, V., Carroll, M., Chambers, M., Graham, H., et al. (2018). Connecting the nutrient composition of seasonal pollens with changing nutritional needs of honey bee (Apis mellifera L.) colonies. J. Insect Physiol. 109, 114–124. doi: 10.1016/j.jinsphys.2018.07.002
Di Pasquale, G., Salignon, M., Le Conte, Y., Belzunces, L. P., Decourtye, A., Kretzschmar, A., et al. (2013). Influence of pollen nutrition on honey bee health: do pollen quality and diversity matter? PLoS ONE 8, e72016. doi: 10.1371/journal.pone.0072016
Dietemann, V., Pflugfelder, J., Anderson, D., Charrière, J.-D., Chejanovsky, N., Dainat, B., et al. (2012). Varroa destructor: research avenues towards sustainable control. J. Apic. Res. 51, 125–132. doi: 10.3896/IBRA.1.51.1.15
Dolasevic, S., Stevanovic, J., Aleksic, N., Glavinic, U., Deletic, N., Mladenovic, M., et al. (2020). The effect of diet types on some quality characteristics of artificially reared Apis mellifera queens. J. Apic. Res. 59, 115–123. doi: 10.1080/00218839.2019.1673965
Dolezal, A. G., Carrillo-Tripp, J., Judd, T. M., Allen Miller, W., Bonning, B. C., Toth, A. L., et al. (2019a). Interacting stressors matter: diet quality and virus infection in honeybee health. R. Soc. Open Sci. 6. doi: 10.1098/rsos.181803
Dolezal, A. G., Carrillo-Tripp, J., Miller, W. A., Bonning, B. C., and Toth, A. L. (2016). Intensively cultivated landscape and varroa mite infestation are associated with reduced honey bee nutritional state. PLoS ONE 11, e0153531. doi: 10.1371/journal.pone.0153531
Dolezal, A. G., St Clair, A. L., Zhang, G., Toth, A. L., and O'Neal, M. E. (2019b). Native habitat mitigates feast-famine conditions faced by honey bees in an agricultural landscape. Proc. Natl. Acad. Sci. U. S. A. 116, 25147. doi: 10.1073/pnas.1912801116
Donkersley, P., Rhodes, G., Pickup, R. W., Jones, K. C., and Wilson, K. (2014). Honeybee nutrition is linked to landscape composition. Ecol. Evol. 4, 4195. doi: 10.1002/ece3.1293
Fine, J. D. (2020). Evaluation and comparison of the effects of three insect growth regulators on honey bee queen oviposition and egg eclosion. Ecotoxicol. Environ. Saf. 205, 111142. doi: 10.1016/j.ecoenv.2020.111142
Fine, J. D., Cox-Foster, D. L., and Mullin, C. A. (2017). An inert pesticide adjuvant synergizes viral pathogenicity and mortality in honey bee larvae. Sci. Rep. 7, 40499. doi: 10.1038/srep40499
Fine, J. D., Shpigler, H. Y., Ray, A. M., Beach, N. J., Sankey, A. L., Cash-Ahmed, A., et al. (2018). Quantifying the effects of pollen nutrition on honey bee queen egg laying with a new laboratory system. PLoS ONE 13, e0203444. doi: 10.1371/journal.pone.0203444
Goulson, D., Nicholls, E., Botías, C., and Rotheray, E. L. (2015). Bee declines driven by combined stress from parasites, pesticides, and lack of flowers. Science 347, 1255957. doi: 10.1126/science.1255957
Harbo, J. R., and Szabo, T. I. (1984). A comparison of instrumentally inseminated and naturally mated queens. J. Apic. Res. 23, 31–36. doi: 10.1080/00218839.1984.11100606
Hölldobler, B., and Wilson, E. O. (2009). The Superorganism: The Beauty, Elegance, and Strangeness of Insect Societies. New York, NY: W.W. Norton. p. 3–10.
Horn, J., Becher, M. A., Kennedy, P. J., Osborne, J. L., and Grimm, V. (2016). Multiple stressors: using the honeybee model BEEHAVE to explore how spatial and temporal forage stress affects colony resilience. Oikos 125, 1001. doi: 10.1111/oik.02636
Hristov, P., Shumkova, R., Palova, N., and Neov, B. (2020). Factors associated with honey bee colony losses: a mini-review. Vet. Sci. 7, 166. doi: 10.3390/vetsci7040166
Johnson, B. (2010). Division of labor in honeybees: form, function, and proximate mechanisms. Behav. Ecol. Sociobiol. 64, 305–316. doi: 10.1007/s00265-009-0874-7
Kapp, R. O. (2000). Ronald O. Kapp's Pollen and Spores. Dallas, TX: American Association of Stratigraphic Palynologists Foundation.
Kearns, C. A., and Inouye, D. W. (1993). Techniques for Pollination Biologists. Niwot, CO: University Press of Colorado.
Khalifa, S. A. M., Elshafiey, E. H., Shetaia, A. A., El-Wahed, A. A. A., Algethami, A. F., Musharraf, S. G., et al. (2021). Overview of bee pollination and its economic value for crop production. Insects 12, 688. doi: 10.3390/insects12080688
Klein, A.-M., Vaissière, B. E., Cane, J. H., Steffan-Dewenter, I., Cunningham, S. A., Kremen, C., et al. (2007). Importance of pollinators in changing landscapes for world crops. Proc. R. Soc. B Biol. Sci. 274, 303–313. doi: 10.1098/rspb.2006.3721
Klein, S., Cabirol, A., Devaud, J.-M., Barron, A. B., and Lihoreau, M. (2017). Why bees are so vulnerable to environmental stressors. Trends Ecol. Evol. 32, 268–278. doi: 10.1016/j.tree.2016.12.009
Kulhanek, K., Steinhauer, N., Rennich, K., Caron, D. M., Sagili, R. R., Pettis, J. S., et al. (2017). A national survey of managed honey bee 2015–2016 annual colony losses in the USA. J. Apic. Res. 56, 328–340. doi: 10.1080/00218839.2017.1344496
Lee, K. V., Goblirsch, M., Mcdermott, E., Tarpy, D. R., and Spivak, M. (2019). Is the brood pattern within a honey bee colony a reliable indicator of queen quality? Insects 10, 12. doi: 10.3390/insects10010012
Long, E. Y., and Krupke, C. H. (2016). Non-cultivated plants present a season-long route of pesticide exposure for honey bees. Nat. Commun. 7. doi: 10.1038/ncomms11629
Maini, S., Medrzycki, P., and Porrini, C. (2010). The puzzle of honey bee losses: a brief review. Bull. Insectol. 63, 153–160.
Martin, A. C., and Harvey, W. J. (2017). The Global Pollen Project: a new tool for pollen identification and the dissemination of physical reference collections. Methods Ecol. Evol. 8, 892–897. doi: 10.1111/2041-210X.12752
McAfee, A., Milone, J. P., Metz, B., McDermott, E., Foster, L. J., Tarpy, D. R., et al. (2021). Honey bee queen health is unaffected by contact exposure to pesticides commonly found in beeswax. Sci. Rep. 11, 15151. doi: 10.1038/s41598-021-94554-1
Milone, J. P., Chakrabarti, P., Sagili, R. R., and Tarpy, D. R. (2021). Colony-level pesticide exposure affects honey bee (Apis mellifera L.) royal jelly production and nutritional composition. Chemosphere 263, 128183. doi: 10.1016/j.chemosphere.2020.128183
Milone, J. P., and Tarpy, D. R. (2021). Effects of developmental exposure to pesticides in wax and pollen on honey bee (Apis mellifera) queen reproductive phenotypes. Sci. Rep. 11, 1020. doi: 10.1038/s41598-020-80446-3
Mullin, C. A., Frazier, M., Frazier, J. L., Ashcraft, S., Simonds, R., vanEngelsdorp, D., et al. (2010). High levels of miticides and agrochemicals in North American apiaries: implications for honey bee health (pesticide exposures to bees). PLoS ONE 5, e9754. doi: 10.1371/journal.pone.0009754
Naug, D. (2009). Nutritional stress due to habitat loss may explain recent honeybee colony collapses. Biol. Conserv. 142, 2369–2372. doi: 10.1016/j.biocon.2009.04.007
Neov, B., Shumkova, R., Palova, N., and Hristov, P. (2021). The health crisis in managed honey bees (Apis mellifera). Which factors are involved in this phenomenon? Biologia 76, 2173–2180. doi: 10.1007/s11756-021-00684-2
Noordyke, E. R., van Santen, E., and Ellis, J. D. (2021). Tracing the fate of pollen substitute patties in western honey bee (Hymenoptera: Apidae) colonies. J. Econ. Entomol. 114, 1421–1430. doi: 10.1093/jee/toab083
PalDat [WWW Document] (2022). Available online at: https://www.paldat.org/info/copyright (accessed March 24, 2022).
Pattamayutanon, P., Peng, C.-C., Sinpoo, C., and Chantawannakul, P. (2018). Effects of pollen feeding on quality of royal jelly. J. Econ. Entomol. 111, 2974–2978. doi: 10.1093/jee/toy251
Pettis, J. S., Wilson, W. T., Shimanuki, H., and Teel, P. D. (1991). Fluvalinate treatment of queen and worker honey bees (Apis mellifera L) and effects on subsequent mortality, queen acceptance and supersedure. Apidologie 22, 1–7. doi: 10.1051/apido:19910101
Potts, S. G., Biesmeijer, J. C., Kremen, C., Neumann, P., Schweiger, O., Kunin, W. E., et al. (2010). Global pollinator declines: trends, impacts and drivers. Trends Ecol. Evol. 25, 345–353. doi: 10.1016/j.tree.2010.01.007
Rueppell, O., Yousefi, B., Collazo, J., and Smith, D. (2017). Early life stress affects mortality rate more than social behavior, gene expression or oxidative damage in honey bee workers. Exp. Gerontol. 90, 19–25. doi: 10.1016/j.exger.2017.01.015
Sande, S. O., Crewe, R. M., Raina, S. K., Nicolson, S. W., and Gordon, I. (2009). Proximity to a forest leads to higher honey yield: another reason to conserve. Biol. Conserv. 142, 2703–2709. doi: 10.1016/j.biocon.2009.06.023
Sandrock, C., Tanadini, M., Tanadini, L. G., Fauser-Misslin, A., Potts, S. G., Neumann, P., et al. (2014). Impact of chronic neonicotinoid exposure on honeybee colony performance and queen supersedure. PLoS ONE 9, e103592. doi: 10.1371/journal.pone.0103592
Simone-Finstrom, M., Li-Byarlay, H., Huang, M. H., Strand, M. K., Rueppell, O., Tarpy, D. R., et al. (2016). Migratory management and environmental conditions affect lifespan and oxidative stress in honey bees. Sci. Rep. 6, 32023. doi: 10.1038/srep32023
Simuth, J. (2001). Some properties of the main protein of honeybee (Apis mellifera) royal jelly. Apidologie 32, 69–80. doi: 10.1051/apido:2001112
Smart, M., Pettis, J., Rice, N., Browning, Z., and Spivak, M. (2016). Linking measures of colony and individual honey bee health to survival among apiaries exposed to varying agricultural land use. PLoS ONE 11, e0152685. doi: 10.1371/journal.pone.0152685
St. Clair, A. L., Dolezal, A. G., Zhang, G., O'Neal, M. E., and Toth, A. L. (2020). Diversified farming in a monoculture landscape: effects on honey bee health and wild bee communities. Environ. Entomol. 49, 743–764. doi: 10.1093/ee/nvaa031
Steinhauer, N., Kulhanek, K., Antúnez, K., Human, H., Chantawannakul, P., Chauzat, M.-P., et al. (2018). Drivers of colony losses. Curr. Opin. Insect Sci. Ecol. Parasites/Parasitoids/Biol. Control 26, 142–148. doi: 10.1016/j.cois.2018.02.004
Tosi, S., Nieh, J. C., Sgolastra, F., Cabbri, R., and Medrzycki, P. (2017). Neonicotinoid pesticides and nutritional stress synergistically reduce survival in honey bees. Proc. R. Soc. B Biol. Sci. 284, 20171711. doi: 10.1098/rspb.2017.1711
Traynor, K. S., Mondet, F., Miranda, d. e., Techer, J. R., Kowallik, M., Oddie, V., et al. (2020). Varroa destructor: a complex parasite, crippling honey bees worldwide. Trends Parasitol. 36, 592–606. doi: 10.1016/j.pt.2020.04.004
Traynor, K. S., Tosi, S., Rennich, K., Steinhauer, N., Forsgren, E., Rose, R., et al. (2021a). Pesticides in honey bee colonies: establishing a baseline for real world exposure over seven years in the USA. Environ. Pollut. 279, 116566. doi: 10.1016/j.envpol.2021.116566
Traynor, K. S., vanEngelsdorp, D., and Lamas, Z. S. (2021b). Social disruption: sublethal pesticides in pollen lead to Apis mellifera queen events and brood loss. Ecotoxicol. Environ. Saf. 214, 112105. doi: 10.1016/j.ecoenv.2021.112105
Vanengelsdorp, D., and Meixner, M. D. (2010). A historical review of managed honey bee populations in Europe and the United States and the factors that may affect them. J. Invertebr. Pathol. 103(Suppl. 1), S80–S95. doi: 10.1016/j.jip.2009.06.011
Vanengelsdorp, D., Tarpy, D. R., Lengerich, E. J., and Pettis, J. S. (2013). Idiopathic brood disease syndrome and queen events as precursors of colony mortality in migratory beekeeping operations in the eastern United States. Prev. Vet. Med. 108, 225–233. doi: 10.1016/j.prevetmed.2012.08.004
Walton, A., Dolezal, A. G., Bakken, M. A., Toth, A. L., and MacMillan, H. (2018). Hungry for the queen: honeybee nutritional environment affects worker pheromone response in a life stage-dependent manner. Funct. Ecol. 32, 2699. doi: 10.1111/1365-2435.13222
Watrous, K. M., Costa, C. P., Diaz, Y. R., and Woodard, S. H. (2021). Flexibility in the critical period of nutrient sequestration in bumble bee queens. Integr. Org. Biol. 3, obab009. doi: 10.1093/iob/obab009
Woodard, S. H., Duennes, M. A., Watrous, K. M., and Jha, S. (2019). Diet and nutritional status during early adult life have immediate and persistent effects on queen bumble bees. Conserv. Physiol. 7, coz048. doi: 10.1093/conphys/coz048
Wright, G. A., Nicolson, S. W., and Shafir, S. (2017). Nutritional physiology and ecology of honey bees. Annu. Rev. Entomol. 63, 327–344. doi: 10.1146/annurev-ento-020117-043423
Wu, J. Y., Anelli, C. M., and Sheppard, W. S. (2011). Sub-lethal effects of pesticide residues in brood comb on worker honey bee (Apis mellifera) development and longevity. PLoS ONE 6, e14720. doi: 10.1371/journal.pone.0014720
Wu-Smart, J., and Spivak, M. (2016). Sub-lethal effects of dietary neonicotinoid insecticide exposure on honey bee queen fecundity and colony development. Sci. Rep. 6, 32108. doi: 10.1038/srep32108
Zhang, G., St. Clair, A. L., Dolezal, A. G., Toth, A. L., and O'Neal, M. E. (2020). Honey bee (Hymenoptera: Apidea) pollen forage in a highly cultivated agroecosystem: limited diet diversity and its relationship to virus resistance. J. Econ. Entomol. 113, toaa055. doi: 10.1093/jee/toaa055
Zhang, G., St. Clair, A. L., Dolezal, A. G., Toth, A. L., and O'Neal, M. E. (2021). Can native plants mitigate climate-related forage dearth for honey bees (Hymenoptera: Apidae)? J. Econ. Entomol. 115, toab202. doi: 10.1093/jee/toab202
Keywords: Apis mellifera, egg laying, bee health, diet quality, nutrition
Citation: St. Clair AL, Suresh S and Dolezal AG (2022) Access to prairie pollen affects honey bee queen fecundity in the field and lab. Front. Sustain. Food Syst. 6:908667. doi: 10.3389/fsufs.2022.908667
Received: 30 March 2022; Accepted: 12 July 2022;
Published: 12 August 2022.
Edited by:
Kimberly Ann Stoner, Connecticut Agricultural Experiment Station, United StatesReviewed by:
David Tarpy, North Carolina State University, United StatesAlison McAfee, North Carolina State University, United States
Ruan Veldtman, South African National Botanical Institute, South Africa
Copyright © 2022 St. Clair, Suresh and Dolezal. This is an open-access article distributed under the terms of the Creative Commons Attribution License (CC BY). The use, distribution or reproduction in other forums is permitted, provided the original author(s) and the copyright owner(s) are credited and that the original publication in this journal is cited, in accordance with accepted academic practice. No use, distribution or reproduction is permitted which does not comply with these terms.
*Correspondence: Ashley L. St. Clair, ashleystclair0@gmail.com
†ORCID: Ashley L. St. Clair https://orcid.org/0000-0002-6002-9759
Adam G. Dolezal https://orcid.org/0000-0001-6164-1344