- 1The Organic Center, Washington, DC, United States
- 2Department of Environmental Science, Policy, and Management, University of California, Berkeley, Berkeley, CA, United States
- 3Department of Biological Sciences, Simon Fraser University, Burnaby, BC, Canada
- 4Department of Zoology and Biodiversity Research Centre, Institute for Resources, Environment and Sustainability, The University of British Columbia, Vancouver, BC, Canada
Numerous studies show that semi-natural habitats within agricultural landscapes benefit native pollinating insects and increase resultant crop pollination services. More recently, evidence is emerging that agricultural diversification techniques on farms, as well as increased compositional and configurational heterogeneity within the cropped portion of landscapes, enhance pollinator communities. However, to date, only a few studies have investigated how diversifying the crops within the farm field itself (i.e., polyculture) influences wild pollinator communities and crop pollination services. In the Central Coast of California, we investigate how local crop diversification within fields, crossed with the proportion of natural habitat in the surrounding landscape, jointly affect pollinator communities and services to strawberry. On 16 organic farms varying in farm type (monoculture vs. polyculture) and proportion of natural land cover, we find that both factors enhance pollinator abundance and richness, although neither affect honey bee abundance. Further, natural cover has a stronger effect on pollinator richness on monoculture (vs. polyculture) farms. Although strawberry can self-pollinate, we document experimentally that pollinator exclusion doubles the probability of berry malformation, while excluding both pollinators and wind triples malformation, with corresponding effects on berry marketability. Finally, in post-hoc tests, we find that berry malformation is significantly higher with greater visitation by honey bees, and observed a trend that this reduction was mitigated by increased native bee richness. These results suggest that both polyculture and semi-natural habitat cover support more abundant and diverse pollinator communities, and that ambient levels of pollinator visitation to strawberry provide an important crop pollination service by improving berry marketability (i.e., by reducing berry malformation). Although further confirmation would be needed, our work suggests that honey bees alone do not provide sufficient pollination services. Prior work has shown that honey bees tend to visit only the top of the strawberry flower receptacle, while other native bees often crawl around the flower base, leading to more complete pollination of the achenes and, consequently, better formed berries. If honey bee visits reduced native bee visitation in our system, this could explain the unexpected correlation between increased honey bee visits and malformation.
1. Introduction
The security of food production depends on the function of many synergistic ecosystem services such as soil fertility, pest control, pollination, and flood and erosion control (Kremen and Miles, 2012; Tscharntke et al., 2012; Bommarco et al., 2013). Widespread adoption of farming practices that support these services is only feasible when those practices do not inhibit economic sustainability or overextend logistical resources (e.g., time, knowledge, labor) for the farmer. It is, therefore, critical that we determine farming practices that simultaneously benefit ecosystem services, food production, and farmer livelihoods. Practices that increase on-farm vegetative diversity, such as polyculture (growing multiple crops within a field), cover cropping, hedgerow installation, or natural habitat restoration, often improve ecosystem function with neutral or even positive effects on crop yields (Rosa-Schleich et al., 2019; Tamburini et al., 2020). For example, crop diversification has been shown to improve soil health (Beillouin et al., 2021) and natural pest control (Iverson et al., 2014), while hedgerows around fields and natural habitat patches in the surrounding landscape can enhance natural predator and pollinator communities (Kremen and Miles, 2012; Karp et al., 2016; Albrecht et al., 2020; Tamburini et al., 2020).
The importance of maintaining robust pollinator communities for food production is now well-established and recognized by many international government sectors (see http://www.fao.org/pollination/major-initiatives/en/ for a list of global and national pollinator initiatives), including the Pollinator Health Task Force in the US (Pollinator Health Task Force 2015). Reliable pollination services maintain food diversity, marketability, production stability, and even shelf life (Steffan-Dewenter et al., 2005; Klein et al., 2007; Gallai et al., 2009; Potts et al., 2010; Eilers et al., 2011; Tscharntke et al., 2012; Wietzke et al., 2018). Roughly 30% of all foods are dependent on pollination to some degree, and those foods provide the majority of our lipids and important vitamins and minerals (Eilers et al., 2011). Yet, as consolidation and industrialization continues across various agricultural production systems, important pollinator habitat is lost which negatively impacts beneficial native bee communities and increases reliance on the managed honey bee (Apis mellifera) (Kremen et al., 2004; Kremen and Ostfeld, 2005; Potts et al., 2005; Goulson et al., 2008; Kohler et al., 2008; Ricketts et al., 2008; Rundlöf et al., 2008; Dicks et al., 2010; Roulston and Goodell, 2011).
The value of crop pollination by native bees is often underestimated, despite their important contribution to food production (e.g., Kremen et al., 2002; Klein et al., 2007; Winfree et al., 2007; Albano et al., 2009; Brittain et al., 2013b; Garibaldi et al., 2013). While crop pollination is often managed using honey bees in North America, Europe, and other regions, robust and diverse communities of native bees can provide more effective pollination to many crops than honey bees alone (Albano et al., 2009; Klein et al., 2012; Garibaldi et al., 2013). The pollination services provided by a highly abundant single pollinator species, such as the honey bee, could be insufficient when physical, phenological, or behavioral mismatch occur at some or all points in time. In these situations, the crop would benefit from the functional complementarity that a diverse pollinator community may offer; different pollinator species can provide unique, complementary roles that together yield higher function (Chagnon et al., 1993; Blüthgen et al., 2006; Hoehn et al., 2008; Rader et al., 2013a,b). Functional complementarity can occur temporally, when different pollinator species are present at different times of the day, season, or year (Blüthgen and Klein, 2011; Pisanty et al., 2016), or spatially, when different bees forage at different locations on a plant or flower [e.g., outer verses inner flowers, Blüthgen and Klein (2011); Brittain et al. (2013a), different parts of the flower receptacle, Chagnon et al. (1993)]. Thus, promoting diverse pollinator communities that span a range of functionality should result in better quality and more resilient pollination services (Grab et al., 2017). Specifically in strawberry, it has been observed that honey bees tend to visit only the top of the flower receptacle, while other native bees often crawl around the flower base, leading to more complete pollination of the achenes and, consequently, less malformed and heavier berries (Chagnon et al., 1993). Further, honey bee visits have led to smaller berries in strawberry, despite adequate amount of pollen deposition, probably due to their methodical foraging within rows, which reduces cross-pollination among varieties when they are present in a farm field (MacInnis and Forrest, 2019).
One of the most effective ways to build or restore robust pollinator communities is to increase vegetative diversity at both landscape and local scales (Ricketts et al., 2008; Winfree et al., 2009; Klein et al., 2012; Kremen and Miles, 2012). At a large scale, farms with greater proportions of natural vegetation in the surrounding landscape have a higher abundance and diversity of native bees (Steffan-Dewenter et al., 2002; Kremen et al., 2004; Klein et al., 2012; Mandelik et al., 2012; Kennedy et al., 2013; Martins et al., 2015). Similarly, studies in Europe and North America have found positive, landscape-scale effects of crop compositional and configurational diversity on pollinator regional species richness (Sirami et al., 2019). At a local scale, leaving areas of undisturbed land on the farm such as field margins or boundaries, drainage ditches, native vegetation remnants, and hedgerows, can provide important habitat to unmanaged pollinators and, in turn, increase pollinator abundance and richness (Hannon and Sisk, 2009; Klein et al., 2012; Kennedy et al., 2013). Recent syntheses comparing farms with more diversified vs. more simplified types of farming found agricultural diversification to have important positive effects on pollinator abundance, richness, and crop pollination (Tamburini et al., 2020; Sánchez et al., 2022). However, while some of these studies looked at aspects of crop diversification, such as crop rotational diversity (planting crops in a sequence over time), agroforestry (including a tree and a shrub or ground layer) or intercropping (growing two crops together in the same row to facilitate inter-species interactions), they did not examine how polycultures (i.e., growing multiple crops in separate blocks of rows within a farm field) can contribute to pollinator abundance, diversity, and function, due to the paucity of such studies.
In California, polyculture is practiced in the Northern and Southern Central Valley and in the Central Coast growing regions of the state, often but not always on organic farms (Chaplin-Kramer and Kremen, 2012; Forrest et al., 2015; Guzman et al., 2019). While organic farming generally supports abundant and diverse insect communities (Holzschuh et al., 2008), variation in farm-scale crop diversity and input use exists across organically managed farms (Tscharntke et al., 2021). Working within a United States Department of Agriculture (USDA) certified organic management system that reduces chemical variables that influence pollinators, we compare field specialization (monoculture) to field diversification (polyculture) to specifically explore whether crop diversity within a field may better support native bee populations and, if so, whether this may lead to higher provisioning of pollination services. We expected that, if crop diversity better supports native pollinators, polyculture farms would increase native bee abundance and diversity, thereby, increasing crop yields. If the benefits of local on-farm crop diversity can substitute for the known benefits of landscape-scale natural habitat, then polyculture farming may provide a pathway to restoring critical services provided by native pollinators, while optimizing the amount of land left in production.
2. Materials and methods
2.1. Study area
We measured pollinator abundance, diversity, and pollination services at each of 16 organic strawberry farm sites located from North of Santa Cruz to South of Salinas in the Central Coast growing region of California (see Supplementary Figure 1 for a map of site locations). Farms were visited two times each across one flowering season in 2012 (except one site was visited only once because the strawberry crop was removed before the second sample round due to complications with soil diseases). This area is one of the largest strawberry producing regions in the US; Monterey and Santa Cruz counties make up nearly 38.6% of CA strawberry production and CA grows 87.6% of the nation's strawberries with a value of over 2.3 Billion USD (California Agricultural Statistics Review CDFA 2018–2019). Monterey county is also the second largest producer of organic produce in California, a state that is responsible for 40% of U.S. organic production (California Department of Food and Agriculture, 2019).
The landscape surrounding the study farms comprises a mosaic of natural habitat and intensive agriculture, which enabled us to examine the effects of large-scale natural habitat separately from local farm crop diversity. Eight study farms were large organic monocultures and eight were organic polycultures (defined below) that included strawberry as one of up to 33 crops (the latter quantity was inferred based on interviews conducted in 2015). Each farm type was surrounded chiefly by intensive agriculture or by natural habitat. Though the extent of field diversification varies in both organic and conventional sectors, we were not able to find sufficiently many diversified conventional farms to allow us to test for consequences of diversification in conventional farms. Therefore, we worked only within the organic sector, which also reduced the potential for chemical factors to depress pollinator populations independently of local or landscape composition (although some organic growers do utilize pesticides allowed by the USDA National Organic Program National Archives and Records Administration, 2018). Farm sites were at least 1, 500 m apart to maintain independence (mean distance between sites was 22 km, and minimum was 1, 600 m).
2.2. Classification of local and landscape vegetative diversity
2.2.1. Local vegetative diversity
We qualified two categories of crop diversity (polyculture and monoculture). We considered farms as “polyculture” if they had five or more crop species or types (e.g., broccoli, lettuce, tomato, herbs, and strawberry) and the majority of polyculture farms had more than 10 and up to 33 crops during the study period. “Monoculture” farms, in contrast, comprised only strawberries in fields of 10+ acres. Field sites ranged from 2 to 80 acres (0.80 to 32 hectares). Non-crop vegetative diversity was also not completely homogenous across sites. Polyculture sites tended to contain floral strips and flowering hedgerows along at least one field margin, though not all polyculture sites contained these features. While flowering hedgerows are less common for monoculture sites, we selected monoculture sites with non-crop vegetative features like those at our monoculture sites, to the best extent possible. Non-flowering perennial wind breaks and floral strips were the most common non-crop vegetation features in monoculture sites. See Supplementary Table 1 for specific details about sites.
2.2.2. Quantifying natural cover in the surrounding landscape
We quantified natural cover in the surrounding landscape using aerial photos available from The National Agriculture Imagery Program (United States Geological Survey, 2022), by summing habitat types across land classification categories “native planted,” “oak woodland,” “oak savannah,” “riparian forest,” “riparian scrub”, and “weedy/sparse scrub”. Specifically, we estimated the amount of natural habitat within a 1km radius around each farm, following many similar studies of pollinators (Steffan-Dewenter et al., 2002; Kremen et al., 2004; Greenleaf et al., 2007; Ricketts et al., 2008; Zurbuchen et al., 2010). We used Geographic Information Systems (ArcGIS, Environmental Systems Research Institute) to calculate the percent area of natural habitat within 1km. Polyculture sites had a mean level of natural habitat equal to 31% (sd = 31%) and monoculture sites 20% (sd = 22%). While the mean level of natural cover is higher at polyculture sites, which might suggest that natural cover and farm type are confounded, natural cover is only weakly correlated with farm type (Pearson's correlation = 0.20). We provide a more complete breakdown of the composition of habitat types surrounding each site in Supplementary Table 2.
2.3. Study crop
We used organic strawberry as our model crop. In this study region, farmers mostly plant day-neutral or everbearing varieties which typically start flowering in February (on average) and start producing fruit in March and plants can be harvested until they are pulled up in October/November. Strawberries are mostly grown as an annual crop, though some farmers will occasionally grow strawberries for a second consecutive year. We considered only sites growing first year strawberries because second year berries accumulate more soil-borne diseases, can harbor overwintering pests, and generally exhibit lower yields. We expect that ground nesting pollinator communities might also differ between first and second year strawberry fields, as the ground is deeply tilled more frequently for first year berries. Once open, individual strawberry flowers stay “fresh” for 1–3 days, but will remain open indefinitely. Although they do not completely depend on insect pollination (Free, 1993), strawberries do not always autonomously self and have been shown to produce larger and more marketable berries when open to external pollen vectors (Wietzke et al., 2018). Berries become malformed when seeds fail to develop and pollination failure is attributed as the most frequent cause (Lopez-Medina et al., 2004). While honey bee hives are not managed for strawberries, this region also grows a lot of cane berries (e.g., raspberries and blackberries) for which, honey bee hives are frequently used. Since honey bees have a large foraging distance, it is common to find high numbers of honey bees in all strawberry fields despite their non-management for this particular crop.
Strawberries provided the most feasible system for this study for three reasons: (1) pollinators enhance fertilization and strawberry fruit production (McGregor, 1976; Lopez-Medina et al., 2004; Albano et al., 2009; Connelly et al., 2015), (2) A diverse range of insects pollinate strawberry (Albano et al., 2009; Connelly et al., 2015) and pollinator diversity has been shown to enhance strawberry pollination through functional complementarity (Chagnon et al., 1993; Klatt et al., 2014), (3) strawberry crops can be found in all four qualifying farm type settings (i.e., polyculture and monoculture, each within largely agricultural vs. largely natural landscapes); no other crop types met this study requirement in this or other farming areas in California at the time of the study.
2.4. Native bee abundance and diversity
To sample pollinator communities, we netted floral visitors of strawberries and set out pan traps, two standard and complementary methods (Roulston et al., 2007). We netted insects visiting strawberry flowers along six transects, each for a 10-min sample period for a total sampling time of 60 min. We placed these walking transects strategically to cover as much of the strawberry field as possible, including both field edges and field interior locations. In small strawberry fields, this sometimes required sampling the same area multiple times. During each 10-min sample period, we surveyed strawberry flowers for any floral visitor, stopping the timer to net and process each specimen. Consequently, total walked transect length would often be shorter at sites with a greater abundance of bees, due to more frequent collections. Because honey bees were overwhelmingly abundant at all sites (even though honey bees colonies are not generally placed at strawberry fields) and, because we were interested in non-honey bee pollinators, we did not stop the timer or collect honey bees that visited strawberry flowers. Instead we tallied honey bee visits for each sample period. While we attempted to count each honey bee visitor only once on a given transect, some may have been counted more than once. Twenty-one pan traps (7 each of blue, white, and yellow plastic Solo bowls filled with a soapy solution) were placed 10 m away from one another along a 210 m transect that typically ran from the field edge to the field interior. Pan traps were placed in each field for a duration of 5 h. We used a 5 h window because, in this microclimate, many days did not have fog-free weather conditions for more than 5 h/day. In large fields the transect started at the field edge and finished in the field interior, whereas in some smaller fields, the transect would run from one field edge to another field edge.
We sampled only under good weather conditions, once in late spring to early summer and once in mid to late summer. Summer weather in the Central Coast of California is variable, though typically foggy, windy, and cold for at least part of the day and, consequently, pollinator surveys were sometimes conducted under weather conditions that would be considered poor in other studies in nearby regions (e.g., M'Gonigle et al., 2015). Specifically, we sampled when the sky was at least partially sunny with temperatures at least 14°C but not higher than 35°C, wind speeds no greater than 5 m per second, and flying insects must have been present. Occasionally weather conditions would change during a sampling period, particularly across the 5-h pan trapping duration, as certain regions were prone to fog in the mornings and afternoons and/or high winds that typically picked up in the late afternoons. Pinned bee specimens were identified by Robbin Thorp (UC Davis) and Jason Gibbs (Lasioglossum specimens only, University of Manitoba) and species names were compiled in a database. Non-bee specimens were pinned but only classified with general identification categories such as butterfly, ant, beetle, etc.
2.5. Berry malformation and marketability
To determine the contribution of insect pollinators to strawberry quality and production, we compared quality of fruit from open-pollinated flowers to those where either insect pollinators were excluded or both pollinators and wind were excluded. Fruit marketability has been used as a sound measurement of strawberry pollination services and has shown to be affected by pollination (McGregor, 1976; Lopez-Medina et al., 2004; Albano et al., 2009). Lopez-Medina et al. qualified strawberry shape into two categories: nicely shaped and misshaped. We used a more quantitative measure to allow us to incorporate the malformation factor into the regression analyses. Our categories of malformation are defined as follows:
0: No malformation, “perfect” berry
1: ≤ 10%, minor blemishes
2: 10−25%, some blemishes
3: ≥25%, major blemishes
We worked with grower participants and industry standards to create these designations of categories. Under conservative market standards such as those for wholesale, berries with scores of 2 and 3 are deemed unmarketable, while some direct market channels can allow more imperfection and scores of 3 are deemed unmarketable. We chose to calculate marketability for more conservative markets, which better represented the typical standards for our study region, thus scoring 0 and 1 berries as “marketable” and 2 and 3 berries as “not marketable.”
In both the spring and summer, we applied three pollination treatments to 35 plants in each strawberry field. On each plant, we marked one flower and left it untreated, so that it remained open to insect and wind pollination (in the case of strawberries, wind will facilitate self-pollination, Chagnon et al., 1993; Żebrowska, 1998). We bagged a second and third flower with fine mesh and cotton, respectively, to exclude pollinators (but not wind) and both pollinators and wind, respectively. We flagged plants and then checked berry ripening beginning after 14 days. At full berry maturity and ripeness (indicated by color and firmness), we weighed each berry and also assigned a score of 0−3 for malformation.
Unpublished field observations, previous greenhouse experiments, and work by others (e.g., Bänsch et al., 2021) has revealed that flower position significantly affects fruit size. That is, primary flowers produce larger fruits than secondary, secondary larger than tertiary, and so on. When possible, we selected secondary flowers on the same crown for these experiments. On a given plant, we selected only flowers of the same position (i.e., all secondary flowers or, if that was not possible on a given plant, all tertiary flowers).
2.6. Analyses
We use linear mixed models and generalized linear mixed models to evaluate how farm type and surrounding natural cover impact (1) pollinator communities and (2) strawberry quality, assessed using berry weight and probability of malformation. To assess pollinator communities, we consider four response variables: native bee abundance, native bee richness, honey bee abundance, and non-bee abundance. In all cases, we consider the regression model:
The linear and quadratic “Julian Date” terms allow abundance and/or richness to peak part way through the field season, as bee abundances in Mediterranean climates typically increase across the spring and early summer season and then decrease as floral resources dry down in late summer. The “Collection method” term allows for differences in response totals between net and trap caught specimens. Note, however, that we have not included any interactive effects between Collection method and landscape-level predictors and, thus, by construction, patterns for nets and pans will resemble one another. We include a random effect of site to account for repeated visits to the same site. For abundance metrics, we use a log response and linear mixed model framework (the former, to correct for large skew in the raw abundance data) and, for native bee richness, we use a generalized linear mixed model with a Poisson family and Log link function. We checked predictors for collinearity using variance inflation factors and found that all variance inflation factors were less than 1.1.
To assess berries, we consider two response variables: berry weight and marketability. We used the regression model:
For berry weight we use a linear mixed model and for marketability we use logistic regression to model the probability that a berry is marketable (0 = not marketable, 1 = marketable).
We also included strawberry variety as a fixed effect in the above model and conclusions were unchanged. However, because most berries are the same variety (“Albion”), including variety can cause model convergence problems and, thus, we opted to exclude this variable.
To test whether different visitors were equivalent, we conducted an additional analysis (not planned as part of our original study), where we asked whether our response (berry weight or malformation, for open-pollinated flowers) is affected by native bee richness and/or honey bee abundance. Specifically,
Because abundance and richness of native bees are highly collinear (Pearson's correlation = 0.78), we do not consider the abundance of native bees here. However, results from models that substitute native bee abundance for native bee richness show similar patterns. Note that because we are only considering open pollinated flowers here, we no longer have multiple flowers per plant and, consequently, we no longer include a random effect of plant. Model results are presented in Table 3.
3. Results
We collected/observed 2, 422 honey bees, 1, 699 native bees, and 2, 399 non-bee visitors. Our native bee specimens comprise 41 species. Many of these native species were rare, with 27 of the 41 collected fewer than 5 times. Supplementary Table 3 presents a complete list of collected species and information about how and where they were collected.
We found higher native bee abundance and richness at polyculture farms than monocultures (effect of polyculture on abundance = 0.69, P < 0.01 and richness = 1.82, P < 0.01, Table 1, Figure 1A, and Supplementary Figure 2A). Further, both native bee abundance and richness were higher at farms with more surrounding natural cover (effect of natural cover on abundance = 3.08, P < 0.05 and richness = 1.92, P < 0.001, Table 1) and this effect was stronger at monoculture farms (interaction terms were both negative, Table 1, Figure 1B, and Supplementary Figure 2B; note that the interaction between farm type and natural cover is only statistically significant for native bee richness). In contrast, we found no evidence that farm type or natural cover impacts honey bee or non-bee abundance (Table 1). We found no strong effect of collection method, indicating that, for native bee abundance or richness and for non-bee abundance, both nets and pans collect roughly similar communities in this system. Many fewer honeybees were collected in pans than were counted foraging which is not surprising, given the large number of honeybees foraging at any given time.
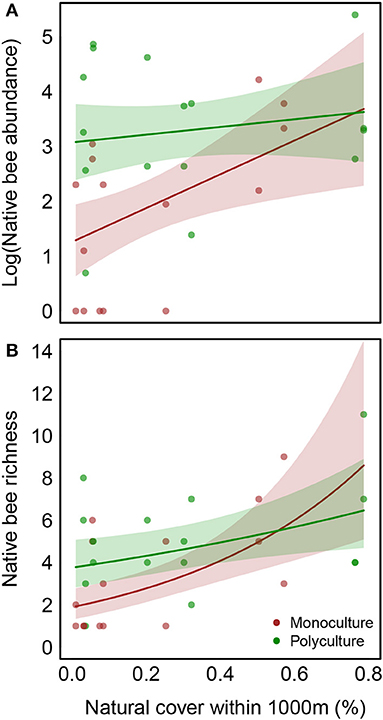
Figure 1. Abundance (A) and richness (B) of net caught native bees is higher in polyculture than in monoculture and increases with increasing natural cover in the surrounding landscape. Further, this increase is more pronounced in monoculture than in polyculture [slopes of brown and green curves differ, but this difference is only statistically significant in (B), P = 0.12 in (A) and P = 0.039 in (B); see Table 3 for parameter estimates]. Shaded regions show 95% confidence intervals, conditioned on mean values for other predictors.
Excluding pollinators led to smaller berries (Table 2) and berries that were more likely to be malformed and non-marketable, while excluding both pollinators and wind intensified these effects (Figure 2 and Table 2). We did not observe a significant effect of farm type or surrounding natural cover on berry malformation, or any evidence of interaction effects between these variables.
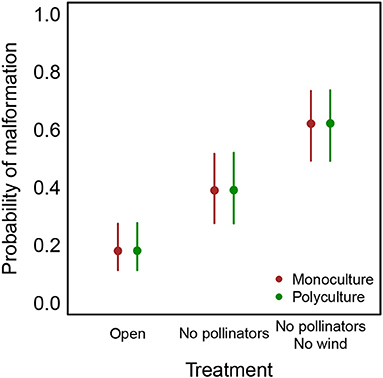
Figure 2. Probability of berry malformation increases when pollinators or pollinators and wind are excluded from flowers. Vertical bars show 95% confidence intervals, conditioned on mean values for other predictors.
Translating the above results into real-world numbers: at both monocultures and polycultures, berry weight decreases from 20 to 18 g per berry when pollinators are excluded and to 16 grams per berry when both pollinators and wind are excluded. The probability that a berry is malformed at a farm at the average level of natural cover more than doubles (from 0.18 to 0.39) when pollinators are excluded and more than triples (from 0.18 to 0.62) when both pollinators and wind are excluded (Figure 2).
Finally, we found that honey bee abundance increases the probability of berry malformation while native bee species richness decreases it (Table 3) but we found no such effects for berry weight.
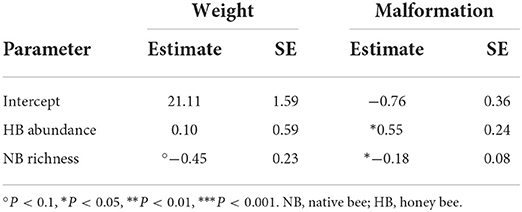
Table 3. Estimated parameters for models examining impacts of the pollinator community on berry weight and malformation.
4. Discussion
For the future of farming to be sustainable, farmers need economically feasible options for practices that maintain or improve natural resources beneficial for farming operations. Our study corroborates the body of literature that shows that vegetative diversity at both the landscape and the field level can indeed enhance the natural resources that provide ecosystem services, in this case pollinators. However, the amount of natural habitat surrounding a farm is largely out of farmer control and, therefore, not usually a feasible diversification option (Morandin and Kremen, 2013). Lack of surrounding natural habitat may be particularly true in agriculturally intensive regions where natural habitat is limited or non-existent. It may also prove impossible for farmers to restore or conserve habitat parcels if they lease rather than own land and have little incentive to invest in long-term diversification, despite the benefits it can provide (Chapman et al., 2022). In either case, farmers may not have access to parcels near natural habitat.
While we have focussed on landscape level natural habitat, non-crop habitats such as floral strips, flowering hedgerows and windbreaks (which provide nesting habitat) were also present across both monoculture and polyculture sites. Floral strips and flowering hedgerows were typically more abundant at polyculture sites, while non-flowering windbreaks were typically more abundant at monoculture sites (Supplementary Table 1). These semi-natural habitats may impact the observed pollinator communities and it is possible that this may confound the effects of field diversification and abundance of semi-natural habitats in the fields surroundings.
A hopeful outcome of this study is that diversifying crops on a farm, which is largely in control of farmers, can potentially substitute for large scale adjacent natural habitat in supporting abundant native pollinator communities. Farms with greater crop diversity had significantly more native bees visiting strawberry and these visits came from a significantly greater number of species. It is possible that, in polyculture, different crops bloom at different times and this temporal complementarity provides greater phenological coverage for a greater number of pollinator species' flight seasons. In contrast to native bees, honey bees and non-bees were equally abundant across all sites. The former is not surprising given that honey bee numbers in this landscape are largely a function of where hives have been placed to provide pollination for other (non-strawberry) crops. Overall, local crop diversity on farms had an important and positive impact on pollinator communities.
It is notable that the results from this study also highlight the benefits of diversifying crops (within) the organic system. Many studies focus on the comparison of conventional to organic agriculture without recognizing or describing the variation in farm-scale diversification that exists within organic (but see Ponisio et al., 2015; Tscharntke et al., 2021; Lu et al., 2022). Organic food is produced on a range of farm sizes and levels and forms of on-farm diversification, from specialized monocultures to highly diversified systems, both over space and time. While organic management has been shown to support biodiversity better than conventional systems overall (Holzschuh et al., 2008; Kremen and Miles, 2012), our results show that, within organic agriculture, crop-diverse farms will support biodiversity better than specialized monocultures. Since 2017, in the United States, USDA organic certification requires farmers to maintain or improve biodiversity (National Archives and Records Administration, 2018), and this study suggests that adding crop diversity can assist in fulfilling that mandate.
Pollinator exclusion experiments revealed that insect pollination is important for strawberry production, even though most fields hosted only a single variety (Albion) consisting of genetically-identical (clonal) individuals. Pollinator visitation nonetheless can increase the probability of producing marketable fruits by nearly 20%. Fruits resulting from flowers that were open to pollinators were significantly bigger and better shaped. Fruits from flowers where both wind and pollinators were excluded were the smallest and the most likely to be malformed, suggesting that wind may also play an important role in pollination. While strawberries are self compatible, berries from open flowers are often larger and more marketable (Wietzke et al., 2018). That is, the flowers require a vector to move the pollen within the flower to facilitate self-pollination. Our results suggest that wind may move the flowers in a way that allows self pollen to drop onto the receptacle and facilitate selfing. It is also likely that any floral visitor can help facilitate selfing, which is why future work should examine the role of non-bee visitors.
Our post-hoc analyses linking pollinator community metrics to berry weight and marketability revealed that all insect visits are likely not equivalent, with higher native bee richness lowering the likelihood of berry malformation and higher honey bee abundance actually increasing it. Previous studies (Chagnon et al., 1993) and our field observations have found that honey bees tend to visit only the top of the strawberry receptacle, potentially providing incomplete pollination. Because native bees often visit other parts of the receptacle and, in particular, the base of the flower, the native bees may complement honey bees to yield more complete pollination and, ultimately better formed and more marketable berries. In contrast, when honey bees are the dominant strawberry pollinator, complete pollination may not always occur. Further, when multiple varieties are present, honey bee visits may saturate stigmas with self or clonal pollen from the same variety, leading to lower weights or marketability than visits from wild pollinators, which forage more erratically and tend to cross-pollinate among varieties more frequently (MacInnis and Forrest, 2019, 2020). Instead in our study system, pollen transported by bees was most likely from clonally-related plants.
While our results indicate that pollinators (and wind) significantly improve strawberry marketability, we did not find that sites with greater native pollinator abundance or diversity produced more marketable fruits. We also did not find a direct effect of local or landscape diversification on fruit marketability. Our dataset may have been too small or contained too little variation within the open pollinated flowers to detect an effect. The vast majority of flowers that were open to pollination resulted in a marketable fruit, regardless of local or landscape level diversification for each site. It is possible that, while the benefits of pollinators can be demonstrated by exclusion, pollinator limitation does not exist for strawberries at our selected study sites, where both honey bees and native floral visitors were abundant. Larger differences might also have been found if multiple varieties were planted in close proximity, which can lead to increased fruit weight and quality (MacInnis and Forrest, 2019, 2020).
5. Conclusions
Our work suggests that local and landscape diversification support larger and more diverse pollinator communities and those pollinators provide an important service to strawberry production, even in a clonal production system. In this system, field diversification is a promising management practice that provides environmental benefits and is more feasible for farmers than conserving or restoring large swaths of natural habitat that are often not part of the land they manage. Additionally, field diversification is a practice that may be executed by conventional and organic farmers alike (Tscharntke et al., 2021). Field diversification likely improves conditions for pollinators by providing multiple sources of pollen and nectar that support different species, or support the same species at different times (Guzman et al., 2019) and will benefit both. While our findings support the role of diversification in moving toward sustainable food production, moving from a highly specialized farming operation to one with multiple crops will be met with many challenges. Further, many recent syntheses have shown additive or interactive benefits between local diversification and surrounding semi-natural habitat (Kennedy et al., 2013; Siramtext et al., 2019). Future studies should consider multiple and simultaneous ecosystem services to quantify comprehensive benefits of crop diversity against the costs.
Data availability statement
The original contributions presented in the study are included in the article/Supplementary material, further inquiries can be directed to the corresponding author.
Author contributions
AS and CK conceived and designed the study. AS carried out fieldwork. LM'G and AS conducted statistical analyses. All authors wrote and edited the manuscript. All authors contributed to the article and approved the submitted version.
Acknowledgments
We would like to thank the farmers who spent many hours of their time offering site specific information and coordinating site visits for the field team. We thank the many interns who worked tirelessly in the field and lab to catch, pin and identify pollinator specimens, with particular gratitude to Rose Nelson and Bethany Reynolds, who assisted in field sampling and managed the lab team respectively. We acknowledge funding from the U.S. Army Research Office (grant to CK), the CS Fund (grant to CK), USDA NIFA (2015-67019-23147/1005662) (grant to CK), Simon Fraser University (to LM'G), and the Natural Sciences and Engineering Research Council of Canada (RGPIN/05103-2018) (Discovery Grant to LM'G).
Conflict of interest
The authors declare that the research was conducted in the absence of any commercial or financial relationships that could be construed as a potential conflict of interest.
The reviewer GT declared a past co-authorship with the author CK to the handling editor.
Publisher's note
All claims expressed in this article are solely those of the authors and do not necessarily represent those of their affiliated organizations, or those of the publisher, the editors and the reviewers. Any product that may be evaluated in this article, or claim that may be made by its manufacturer, is not guaranteed or endorsed by the publisher.
Supplementary material
The Supplementary Material for this article can be found online at: https://www.frontiersin.org/articles/10.3389/fsufs.2022.941840/full#supplementary-material
References
Albano, S., Salvado, E., Duarte, S., Mexia, A., and Borges, P. A. (2009). Pollination effectiveness of different strawberry floral visitors in Ribatejo, Portugal: selection of potential pollinators. Part 2. Adv. Hortic. Sci. 23, 246–253.
Albrecht, M., Kleijn, D., Williams, N., Tschumi, M., Blaauw, B. R., Bommarco, R., et al. (2020). The effectiveness of flower strips and hedgerows on pest control, pollination services and crop yield: a quantitative synthesis. Ecol. Lett. 23, 1488–1498. doi: 10.1111/ele.13576
Bänsch, S., Tscharntke, T., Gabriel, D., and Westphal, C. (2021). Crop pollination services: complementary resource use by social vs solitary bees facing crops with contrasting flower supply. J. Appl. Ecol. 58, 476–485. doi: 10.1111/1365-2664.13777
Beillouin, D., Ben-Ari, T., Malézieux, E., Seufert, V., and Makowski, D. (2021). Positive but variable effects of crop diversification on biodiversity and ecosystem services. Glob. Chang. Biol. 27, 4697–4710. doi: 10.1111/gcb.15747
Blüthgen, N., and Klein, A.-M. (2011). Functional complementarity and specialisation: the role of biodiversity in plant-pollinator interactions. Basic Appl. Ecol. 12, 282–291. doi: 10.1016/j.baae.2010.11.001
Blüthgen, N., Menzel, F., and Blüthgen, N. (2006). Measuring specialization in species interaction networks. BMC Ecol. 6, 9. doi: 10.1186/1472-6785-6-9
Bommarco, R., Kleijn, D., and Potts, S. G. (2013). Ecological intensification: harnessing ecosystem services for food security. Trends Ecol. Evol. 28, 230–238. doi: 10.1016/j.tree.2012.10.012
Brittain, C., Kremen, C., and Klein, A. M. (2013a). Biodiversity buffers pollination from changes in environmental conditions. Global Change Biol. 19, 540–547. doi: 10.1111/gcb.12043
Brittain, C., Williams, N., Kremen, C., and Klein, A. M. (2013b). Synergistic effects of non-Apis bees and honey bees for pollination services. Proc. R. Soc. B 280, 20122767. doi: 10.1098/rspb.2012.2767
Chagnon, M., Gingras, J., and DeOliveira, D. (1993). Complementary aspects of strawberry pollination by honey and indigenous bees (Hymenoptera). J. Econ. Entomol. 86, 416–420. doi: 10.1093/jee/86.2.416
Chaplin-Kramer, R., and Kremen, C. (2012). Pest control experiments show benefits of complexity at landscape and local scales. Ecol. Appl. 22, 1936–1948. doi: 10.1890/11-1844.1
Chapman, M. S., Wiltshire, S., Baur, P., Bowles, T., Carlisle, L., Castillo, F., et al. (2022). Social-ecological feedbacks drive tipping points in farming system diversification. One Earth 5, 283–292. doi: 10.1016/j.oneear.2022.02.007
Connelly, H., Poveda, K., and Loeb, G. (2015). Landscape simplification decreases wild bee pollination services to strawberry. Agric. Ecosyst. Environ. 211, 51–56. doi: 10.1016/j.agee.2015.05.004
Dicks, L. V., Showler, D. A., and Sutherland, W. J. (2010). Bee Conservation: Evidence for the Effects of Interventions, Vol. 1. Pelagic Publishing, Exeter, UK. Available online at: https://www.nhbs.com/about
Eilers, E. J., Kremen, C., Greenleaf, S. S., Garber, A. K., and Klein, A. M. (2011). Contribution of pollinator-mediated crops to nutrients in the human food supply. PLoS ONE 6, e21363. doi: 10.1371/journal.pone.0021363
Forrest, J. R. K., Thorp, R. W., Kremen, C., and Willams, N. M. (2015). Contrasting patterns in species and functional-trait diversity of bees in an agricultural landscape. J. Appl. Ecol. 52, 706–715. doi: 10.1111/1365-2664.12433
Gallai, N., Salles, J. M., Settele, J., and Vaissiére, B. E. (2009). Economic valuation of the vulnerability of world agriculture confronted with pollinator decline. Ecol. Econ. 68, 810–821. doi: 10.1016/j.ecolecon.2008.06.014
Garibaldi, L. A., Steffan-Dewenter, I., Winfree, R., Aizen, M. A., Bommarco, R., Cunningham, S. A., et al. (2013). Wild pollinators enhance fruit set of crops regardless of honey bee abundance. Science 339, 1608–1611. doi: 10.1126/science.1230200
Goulson, D., Lye, G. C., and Darvill, B. (2008). Decline and conservation of bumble bees. Annu. Rev. Entomol. 53, 191–208. doi: 10.1146/annurev.ento.53.103106.093454
Grab, H., Blitzer, E. J., Danforth, B., Loeb, G., and Poveda, K. (2017). Temporally dependent pollinator competition and facilitation with mass flowering crops affects yield in co-blooming crops. Sci. Rep. 7, 1–9. doi: 10.1038/srep45296
Greenleaf, S. S., Williams, N. M., Winfree, R., and Kremen, C. (2007). Bee foraging ranges and their relationship to body size. Oecologia 153, 589–596. doi: 10.1007/s00442-007-0752-9
Guzman, A., Chase, M., and Kremen, C. (2019). On-farm diversification in an agriculturally-dominated landscape positively influences specialist pollinators. Front. Sustain. Food Syst. 3, 87. doi: 10.3389/fsufs.2019.00087
Hannon, L. E., and Sisk, T. D. (2009). Hedgerows in an agri-natural landscape: potential habitat value for native bees. Biol. Cons. 142, 2140–2154. doi: 10.1016/j.biocon.2009.04.014
Hoehn, P., Tscharntke, T., Tylianakis, J. M., and Steffan-Dewenter, I. (2008). Functional group diversity of bee pollinators increases crop yield. Proc. R. Soc. B 275, 2283–2291. doi: 10.1098/rspb.2008.0405
Holzschuh, A., Steffan-Dewenter, I., and Tscharntke, T. (2008). Agricultural landscapes with organic crops support higher pollinator diversity. Oikos 117, 354–361. doi: 10.1111/j.2007.0030-1299.16303.x
Iverson, A. L., Marín, L. E., Ennis, K. K., Gonthier, D. J., Connor-Barrie, B. T., Remfert, J. L., et al. (2014). Do polycultures promote win-wins or trade-offs in agricultural ecosystem services? A meta-analysis. J. Appl. Ecol. 51, 1593–1602. doi: 10.1111/1365-2664.12334
Karp, D. S., Moses, R., Gennet, S., Jones, M. S., Joseph, S., M'Gonigle, L. K., et al. (2016). Agricultural practices for food safety threaten pest control services for fresh produce. J. Appl. Ecol. 53, 1402–1412. doi: 10.1111/1365-2664.12707
Kennedy, C. M., Lonsdorf, E., Neel, M. C., Williams, N. M., Ricketts, T. H., Winfree, R., et al. (2013). A global quantitative synthesis of local and landscape effects on wild bee pollinators in agroecosystems. Ecol. Lett. 16, 584–599. doi: 10.1111/ele.12082
Klatt, B. K., Holzschuh, A., Westphal, C., Clough, Y., Smit, I., Pawelzik, E., et al. (2014). Bee pollination improves crop quality, shelf life and commercial value. Proc. R. Soc. B 281, 20132440. doi: 10.1098/rspb.2013.2440
Klein, A. M., Brittain, C., Hendrix, S. D., Thorp, R., Williams, N., and Kremen, C. (2012). Wild pollination services to California almond rely on semi-natural habitat. J. Appl. Ecol. 49, 723–732. doi: 10.1111/j.1365-2664.2012.02144.x
Klein, A. M., Vaissiére, B. E., Cane, J. H., Steffan-Dewenter, I., Cunningham, S. A., Kremen, C., et al. (2007). Importance of pollinators in changing landscapes for world crops. Proc. R. Soc. B 274, 303–313. doi: 10.1098/rspb.2006.3721
Kohler, F., Verhulst, J., van Klink, R., and Kleijn, D. (2008). At what spatial scale do high-quality habitats enhance the diversity of forbs and pollinators in intensively farmed landscapes? J. Appl. Ecol. 45, 753–762. doi: 10.1111/j.1365-2664.2007.01394.x
Kremen, C., and Miles, A. (2012). Ecosystem services in biologically diversified versus conventional farming systems: benefits, externalities, and trade-offs. Ecol. Soc. 17, 40. doi: 10.5751/ES-05035-170440
Kremen, C., and Ostfeld, R. S. (2005). A call to ecologists: measuring, analyzing, and managing ecosystem services. Front. Ecol. Environ. 3, 540–548. doi: 10.1890/1540-9295(2005)003[0540:ACTEMA]2.0.CO;2
Kremen, C., Williams, N. M., Bugg, R. L., Fay, J. P., and Thorp, R. W. (2004). The area requirements of an ecosystem service: crop pollination by native bee communities in California. Ecol. Lett. 7, 1109–1119. doi: 10.1111/j.1461-0248.2004.00662.x
Kremen, C., Williams, N. M., and Thorp, R. W. (2002). Crop pollination from native bees at risk from agricultural intensification. Proc. Natl. Acad. Sci. U.S.A. 99, 16812–16816. doi: 10.1073/pnas.262413599
Lopez-Medina, J., Palacio-Villegas, A., and Vazquez-Ortiz, E. (2004). “Misshaped fruit in strawberry, an agronomic evaluation,” in V International Strawberry Symposium, 77–78. doi: 10.17660/ActaHortic.2006.708.8
Lu, A., Gonthier, D. J., Sciligo, A. R., Garcia, K., Chiba, T., Juárez, G., et al. (2022). Changes in arthropod communities mediate the effects of landscape composition and farm management on pest control ecosystem services in organically managed strawberry crops. J. Appl. Ecol. 59, 585–597. doi: 10.1111/1365-2664.14076
MacInnis, G., and Forrest, J. R. K. (2019). Pollination by wild bees yields larger strawberries than pollination by honey bees. J. Appl. Ecol. 56, 824–832. doi: 10.1111/1365-2664.13344
MacInnis, G., and Forrest, J. R. K. (2020). Field design can affect cross-pollination and crop yield in strawberry (Fragaria × ananassa D.). Agric. Ecosyst. Environ. 289, 106738. doi: 10.1016/j.agee.2019.106738
Mandelik, Y., Winfree, R., Neeson, T., and Kremen, C. (2012). Complementary habitat use by wild bees in agro-natural landscapes. Ecol. Appl. 22, 1535–1546. doi: 10.1890/11-1299.1
Martins, K. T., Gonzalez, A., and Lechowicz, M. J. (2015). Pollination services are mediated by bee functional diversity and landscape context. Agric. Ecosyst. Environ. 200, 12–20. doi: 10.1016/j.agee.2014.10.018
McGregor, S. E. (1976). Insect Pollination of Cultivated Crop Plants, Vol. 496. Agricultural Research Service, US Department of Agriculture, Washington, DC.
M'Gonigle, L. K., Ponisio, L. C., Cutler, K., and Kremen, C. (2015). Habitat restoration promotes pollinator persistence and colonization in intensively-managed agriculture. Ecol. Appl. 25, 1557–1565. doi: 10.1890/14-1863.1
Morandin, L. A., and Kremen, C. (2013). Hedgerow restoration promotes pollinator populations and exports native bees to adjacent fields. Ecol. Appl. 23, 829–839. doi: 10.1890/12-1051.1
National Archives Records Administration (2018). Code of Federal Regulations, National Organic Program. Available online at: https://www.ecfr.gov/current/title-7/subtitle-B/chapter-I/subchapter-M/part-205/subpart-G (accessed August, 9, 2022).
Pisanty, G., Afik, O., Wajnberg, E., and Mandelik, Y. (2016). Watermelon pollinators exhibit complementarity in both visitation rate and single-visit pollination efficiency. J. Appl. Ecol. 53, 360–370. doi: 10.1111/1365-2664.12574
Ponisio, L. C., M'Gonigle, L. K., Mace, K. C., Palomino, J., de Valpine, P., and Kremen, C. (2015). Diversification practices reduce organic to conventional yield gap. Proc. R. Soc. B. 282, 20141396. doi: 10.1098/rspb.2014.1396
Potts, S. G., Biesmeijer, J. C., Kremen, C., Neumann, P., Schweiger, O., and Kunin, W. E. (2010). Global pollinator declines: trends, impacts and drivers. Trends Ecol. Evol. 25, 345–353. doi: 10.1016/j.tree.2010.01.007
Potts, S. G., Vulliamy, B., Roberts, S., O'Toole, C., Dafni, A., Ne'eman, G., et al. (2005). Role of nesting resources in organising diverse bee communities in a Mediterranean landscape. Ecol. Entomol. 30, 78–85. doi: 10.1111/j.0307-6946.2005.00662.x
Rader, R., Edwards, W., Westcott, D. A., Cunningham, S. A., and Howlett, B. G. (2013a). Diurnal effectiveness of pollination by bees and flies in agricultural Brassica rapa: implications for ecosystem resilience. Basic Appl. Ecol. 14, 20–27. doi: 10.1016/j.baae.2012.10.011
Rader, R., Reilly, J., Bartomeus, I., and Winfree, R. (2013b). Native bees buffer the negative impact of climate warming on honey bee pollination of watermelon crops. Glob. Chang. Biol. 19, 3103–3110. doi: 10.1111/gcb.12264
Ricketts, T. H., Regetz, J., Steffan-Dewenter, I., Cunningham, S. A., Kremen, C., Bogdanski, A., et al. (2008). Landscape effects on crop pollination services: are there general patterns? Ecol. Lett. 11, 499–515. doi: 10.1111/j.1461-0248.2008.01157.x
Rosa-Schleich, J., Loos, J., Mußhoff, O., and Tscharntke, T. (2019). Ecological-economic trade-offs of diversified farming systems-a review. Ecol. Econ. 160, 251–263. doi: 10.1016/j.ecolecon.2019.03.002
Roulston, T. H., and Goodell, K. (2011). The role of resources and risks in regulating wild bee populations. Annu. Rev. Entomol. 56, 293–312. doi: 10.1146/annurev-ento-120709-144802
Roulston, T. H., Smith, S. A., and Brewster, A. L. (2007). A comparison of pan trap and intensive net sampling techniques for documenting a bee (Hymenoptera: Apiformes) fauna. J. Kans. Entomol. 80, 179–181. doi: 10.2317/0022-8567(2007)80[179:ACOPTA]2.0.CO;2
Rundlöf, M., Nilsson, H., and Smith, H. G. (2008). Interacting effects of farming practice and landscape context on bumble bees. Biol. Cons. 141, 417–426. doi: 10.1016/j.biocon.2007.10.011
Sánchez, A. C., Jones, S. K., Purvis, A., Estrada-Carmona, N., and De Palma, A. (2022). Landscape and functional groups moderate the effect of diversified farming on biodiversity: a global meta-analysis. Agric. Ecosyst. Environ. 332, 107933. doi: 10.1016/j.agee.2022.107933
Sirami, C., Gross, N., Baillod, A. B., Bertrand, C., Carrié, R., Hass, A., et al. (2019). Increasing crop heterogeneity enhances multitrophic diversity across agricultural regions. Proc. Natl. Acad. Sci. U.S.A. 116, 16442–16447. doi: 10.1073/pnas.1906419116
Steffan-Dewenter, I., Münzenberg, U., Bürger, C., Thies, C., and Tscharntke, T. (2002). Scale-dependent effects of landscape context on three pollinator guilds. Ecology 83, 1421–1432. doi: 10.1890/0012-9658(2002)083[1421:SDEOLC]2.0.CO;2
Steffan-Dewenter, I., Potts, S. G., and Packer, L. (2005). Pollinator diversity and crop pollination services are at risk. Trends Ecol. Evol. 313, 651–652. doi: 10.1016/j.tree.2005.09.004
Tamburini, G., Bommarco, R., Wanger, T. C., Kremen, C., van der Heijden, M. G. A., Liebman, M., et al. (2020). Agricultural diversification promotes multiple ecosystem services without compromising yield. Sci. Adv. 6, eaba1715. doi: 10.1126/sciadv.aba1715
Tscharntke, T., Clough, Y., Wanger, T. C., Jackson, L., Motzke, I., Perfecto, I., et al. (2012). Global food security, biodiversity conservation and the future of agricultural intensification. Biol. Cons. 151, 53–59. doi: 10.1016/j.biocon.2012.01.068
Tscharntke, T., Grass, I., Wanger, T. C., Westphal, C., and Batáry, P. (2021). Beyond organic farming-harnessing biodiversity-friendly landscapes. Trends Ecol. Evol. 36, 919–930. doi: 10.1016/j.tree.2021.06.010
United States Geological Survey (2022). National Agriculture Imagery Program. Available online at: https://www.usgs.gov/centers/eros/science/usgs-eros-archive-aerial-photography-national-agriculture-imagery-program-naip (accessed August, 9, 2022).
Wietzke, A., Westphal, C., Gras, P., Kraft, M., Pfohl, K., Karlovsky, P., et al. (2018). Insect pollination as a key factor for strawberry physiology and marketable fruit quality. Agric. Ecosyst. Environ. 2018, 197–204. doi: 10.1016/j.agee.2018.01.036
Winfree, R., Aguilar, R., Vazquez, D. P., LeBuhn, G., and Aizen, M. A. (2009). A meta-analysis of bees' responses to anthropogenic disturbance. Ecology 90, 2068–2076. doi: 10.1890/08-1245.1
Winfree, R., Williams, N. M., Dushoff, J., and Kremen, C. (2007). Native bees provide insurance against ongoing honey bee losses. Ecol. Lett. 10, 1105–1113. doi: 10.1111/j.1461-0248.2007.01110.x
Żebrowska, J. (1998). Influence of pollination modes on yield components in strawberry (Fragaria × ananassa duch.). Plant Breed. 117, 255–260. doi: 10.1111/j.1439-0523.1998.tb01935.x
Keywords: native bee, pollinators, diversified farming, strawberry pollination, organic, agro-ecosystem, polyculture
Citation: Sciligo AR, M'Gonigle LK and Kremen C (2022) Local diversification enhances pollinator visitation to strawberry and may improve pollination and marketability. Front. Sustain. Food Syst. 6:941840. doi: 10.3389/fsufs.2022.941840
Received: 11 May 2022; Accepted: 03 August 2022;
Published: 22 August 2022.
Edited by:
Patricia Landaverde, Martin Luther University of Halle-Wittenberg, GermanyReviewed by:
Svenja Bänsch, KWS Saat, GermanyGiovanni Tamburini, University of Bari Aldo Moro, Italy
Copyright © 2022 Sciligo, M'Gonigle and Kremen. This is an open-access article distributed under the terms of the Creative Commons Attribution License (CC BY). The use, distribution or reproduction in other forums is permitted, provided the original author(s) and the copyright owner(s) are credited and that the original publication in this journal is cited, in accordance with accepted academic practice. No use, distribution or reproduction is permitted which does not comply with these terms.
*Correspondence: Claire Kremen, Y2xhaXJlLmtyZW1lbkB1YmMuY2E=