- 1Department of Agronomy, University of Wisconsin–Madison, Madison, WI, United States
- 2Supply Chain Development Specialist, Forever Green Initiatives, University of Minnesota, St. Paul, MN, United States
- 3Department of Agricultural and Applied Economics, University of Wisconsin–Madison, Madison, WI, United States
Introduction: Kernza intermediate wheatgrass (IWG) is a perennial grain and forage crop. Intercropping IWG with legumes may increase the forage yields and nutritive value but may compromise Kernza grain yields. The interaction between IWG and legumes depends on planting season, row spacing, and legume species. Our aim was to evaluate the effects of those management practices on Kernza grain yield, summer and fall forage yield and nutritive value, weed biomass and, the profitability of the cropping system in Wisconsin, USA.
Methods: In the spring and fall of 2017, we planted eight cropping systems at 38 and 57 cm of row spacing: four IWG monocultures [control without N fertilization or weed removal (IWG), hand weed removal (hand weeded), IWG fertilized with urea at rates of 45 or 90 kg ha−1], and four IWG-legume intercrops (IWG with alfalfa, Berseem clover, Kura clover, or red clover).
Results and discussion: Most of the intercropping systems were similar to IWG monoculture in grain (ranging from 652 to 1,160 kg ha−1) and forage yield (ranging from 2,740 to 5,190 kg ha−1) and improved the forage quality. However, for spring planted IWG, intercropped with red clover or alfalfa, the grain and forage yields were lower than the IWG monoculture (~80 and 450 kg ha−1, respectively). The best performing intercrops in the first year were Kura clover in the spring planting (652 kg Kernza grain ha−1, 4,920 kg IWG forage ha−1 and 825 kg legume forage ha−1) and red clover in the fall planting (857 kg Kernza grain ha−1, 3,800 kg IWG forage ha−1, and 450 kg legume forage ha−1). In the second year, grain yield decreased 84% on average. Overall, the profitability of the IWG legume intercropping was high, encouraging the adoption of dual-purpose perennial crops.
Introduction
Ecosystem disservices from modern agriculture challenge the ability of society to meet current and future needs (Tilman et al., 2002; Power, 2010). The frequent tillage of soils and a lack of vegetation cover for prolonged periods have led to extensive soil erosion, soil carbon loss, and nutrient runoff into groundwater, among other problems, which demands the rethinking of the way humans produce food. Some novel approaches seek to diversify and perennialize cropping systems by reducing soil tillage (Crews and Rumsey, 2017), replacing fallow periods with service crops (Schipanski et al., 2014; Pinto et al., 2017), integrating crop and livestock systems (de Faccio Carvalho et al., 2021; Franco et al., 2021; Picasso et al., 2022), intercropping multifunctional species (Malézieux et al., 2009; Gaba et al., 2015) or including dual-purpose perennial crops in the agricultural rotations (Hunter et al., 2020b; Franco et al., 2021). Recent advances in domestication and breeding of perennial cereals for seed yield offer the opportunity to reintroduce perennial polycultures and regenerate components and processes of natural ecosystems to agroecosystems (Glover et al., 2010; Pimentel et al., 2012; Ryan et al., 2018). Since perennial crops last beyond one season, the disturbance needed for establishment can be compensated throughout multiple production years (Crews et al., 2016). Through their continuous productivity, perennial crops protect soil from erosion (Ryan et al., 2018), compete with weeds (Zimbric et al., 2020), catch nutrients preventing leaching (Culman et al., 2013; Jungers et al., 2019) and improve soil health (Culman et al., 2010; de Oliveira et al., 2020).
Intermediate wheatgrass [IWG, Thinopyrum intermedium (Host) Barkworth and D.R. Dewey)] is among the most promising perennial cereal crops to date (Ryan et al., 2018), due to its synchronous seed maturity, edible grain, moderate shattering, and moderate threshability (Wagoner, 1990). The current grain yield is relatively low relative to annual wheat [i.e., up to ~1,660 kg ha−1 in experimental fields (Franco et al., 2021) and averaging 460 kg ha−1 in the primary production areas (Skelly and Peters, 2021)] but breeders expect IWG to achieve comparable yields in the near future (DeHaan et al., 2018; Bajgain et al., 2020). The grain of IWG is sold as Kernza® to restaurants, bakeries, and other food-related businesses in the United States for use in value-added products (Lubofsky, 2016; Ryan et al., 2018). The forage can be harvested in summer, removing the crop residue or straw, and mixed with higher value forage (e.g., alfalfa hay) to feed beef or dry dairy cows. The forage harvested in spring or fall, as other cool-season grasses commonly grown in the humid climate of the Upper Midwestern US, is suitable for lactating beef cows, dairy cows, and growing heifers (Favre et al., 2019).
Growing legumes with perennial grasses can provide multiple benefits, including providing N inputs by biological fixation (Pinto et al., 2021b), increasing soil organic matter (Lehmann et al., 2020), suppressing weeds (Law et al., 2021), and increasing the total forage harvested and its nutritive value (Favre et al., 2019). Nevertheless, little is known about the agronomic management of IWG-legume intercropping. Furthermore, different legume species could be better or worse companions of IWG to maximize benefits. Some experiences with perennial legumes have shown lower Kernza grain yields in intercropping with alfalfa (Medicago sativa sp.) or red clover (Trifolium pratense L.) than in the IWG monocultures (Tautges et al., 2018; Favre et al., 2019; Mårtensson et al., 2022). However, others showed similar Kernza grain yields in alfalfa, sweet clover, and white clover intercropping (Dick et al., 2018; Reilly et al., 2022). Slow establishing perennial legumes like Kura clover (Trifolium ambiguum M. Bieb) (Sleugh et al., 2000) or annual legumes like Berseem clover (Trifolium alexandrinum L.) could reduce competition and avoid the observed Kernza grain reductions. The interaction between different species involves the co-occurrence of both complementary and competitive relationships (Picasso et al., 2011; Duchene et al., 2017). Usually when grass-legume intercropping systems are compared with grass monocultures, negative and positive effects are confounded (e.g., competition for radiation and soil resources or facilitation processes through the symbiotic association between legumes and N-fixing bacteria). In order to separate the effects of competition for resources from N facilitation, weed removal and N fertilization treatments can be added to intercropping experiments.
Additionally, effective stand establishment is critical for IWG's long-term productivity, and in intercropping systems, it can be influenced by both the planting date and the row spacing. For IWG monocultures of the USA Midwest, late summer and early fall typically achieve successful establishment of Kernza grain production systems (Jungers et al., 2022). Intermediate wheatgrass requires a two-stage induction period with vernalization for flowering (Duchene et al., 2021; Locatelli et al., 2022), thus spring seedings will not produce grain during the first year (Olugbenle et al., 2021; Jungers et al., 2022). For IWG-legume intercropping systems, limited information is available. When IWG was seeded in the fall, the highest intercropped Kernza grain yields were observed when red clover was frost seeded in the spring season (Olugbenle et al., 2021). When red clover was planted in the fall at the same time as IWG, lower Kernza grain yields were likely due to more competition during IWG establishment. The optimal planting season for IWG-legumes should be carefully studied because there is a trade-off between reducing the competition during IWG establishment and promoting the growth of the legumes to optimize benefits related to N fixation (Pinto et al., 2021b). In addition, IWG-legume interactions can be influenced by the distance between IWG rows (i.e., row spacing). In IWG monocultures, wider row spacing has been associated with higher Kernza grain yields than narrower row spacing (Hunter et al., 2020a). However, changes in the available resources such as light, water, and nutrients due to row spacing are likely to vary among different legume species.
The profitability of the IWG cropping systems depends on both grain and forage incomes (Hunter et al., 2020b). Therefore, the lower Kernza grain yields harvested in alfalfa and red clover intercrops (Tautges et al., 2018; Favre et al., 2019; Mårtensson et al., 2022) could be compensated by positive effects on the increased forage yield and nutritive value. Intercropping IWG with red clover has consistently increased the nutritive value of the summer and fall forage and tripled the amount of available forage in the fall (Favre et al., 2019), positively affecting the revenue perceived by the farmers. In fact, it has been seen that higher forage yields achieved by IWG-legume intercropping systems reduce the Kernza grain price required to be profitable (Law et al., 2022). Although Kernza® grain markets are in a price discovery phase, estimating potential net returns could be useful to compare different cropping systems.
Our objectives were to evaluate the effects of IWG planting season, row spacing, and legume species in intercropping on (a) Kernza grain yield, (b) summer and fall forage yield and nutritive value, and (c) the potential profitability of the cropping system in Wisconsin, USA.
Materials and methods
The experiment was conducted at the University of Wisconsin-Madison Arlington Agricultural Research Station, WI (43°18'6.97“ N, 89°21'9.98” W) on a Plano silt loam soil (fine-silty, mixed, superactive, mesic Typic Argiudoll; NRCS-USDA, 2022a). The mean annual temperature is 6.7°C, and the mean annual rainfall is 863 mm (Arguez et al., 2010). A large grain IWG germplasm, a product of four successive breeding cycles at The Land Institute (Salina, KS) was seeded at the rate of 11.2 kg ha−1 in a field previously harvested for soybean grain. At the beginning of the experiment, thirty-two composite soil samples taken at 0–15 cm in the experimental area averaged 3.5% of soil organic matter, 56.5 ppm of P, 244 ppm of K, 5.1 ppm of NO3-N and 21.1 ppm of NH4-N. The experiment was established in 2017 in two different planting seasons (Figure 1): spring (April 12, 2017) and fall (September 21, 2017). In the spring planting season, the plot size was 3 m by 4.8 m, and in fall it was 3 m by 1.5 m. The first Kernza grain harvest for both planting seasons was in 2018 due to IWG vernalization requirements (Locatelli et al., 2022). During the establishment (year 2017), the growing degree days (GDD) and the precipitation accumulated until the first frost were 3,265 GDD and 646 mm, respectively, in the spring planting and, 534 GDD and 114 mm in the fall planting. Precipitation during the growing season until harvest (April to July) was 512 mm in 2018 and 560 mm in 2019, higher than normal (University of Wisconsin, Division of Extension, 2022). Data were collected during two consecutive grain production years (Figure 1).
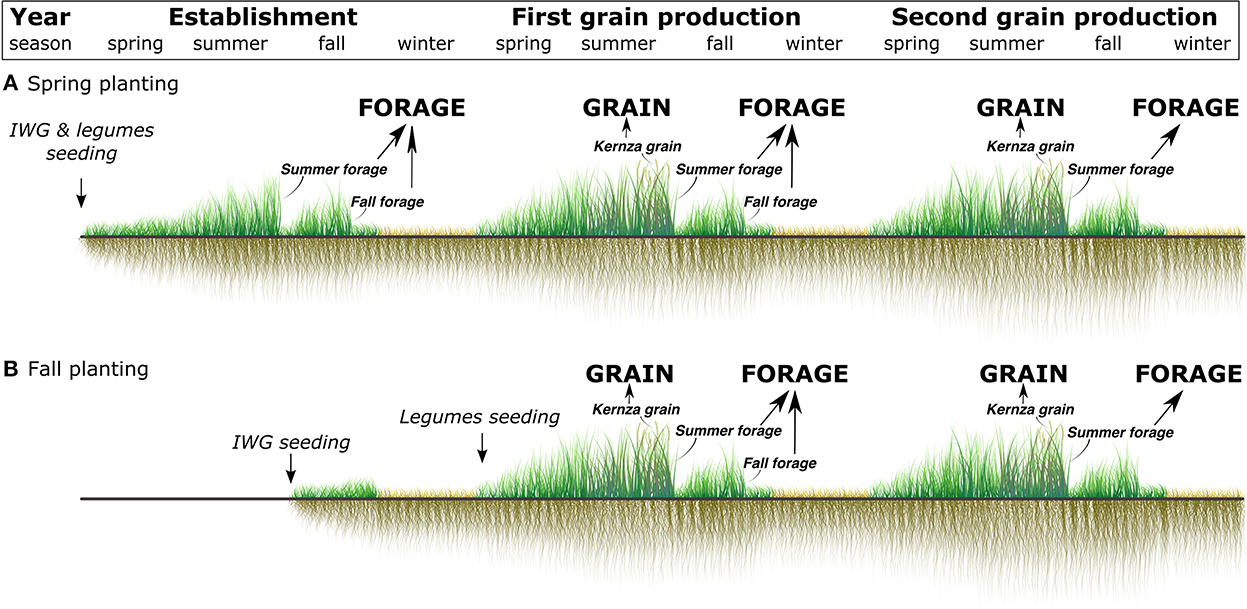
Figure 1. Comparative timeline of establishment and successive grain and forage harvests of IWG and legumes for (A) spring and (B) fall planting seasons.
We installed a full factorial experiment of three factors: planting season, row spacing, and cropping systems. The planting season factor had two levels: IWG planted in the spring or in the fall of the year 2017. In the IWG spring planting, all forage legumes were sown drilling the inter-row 1 week after sowing IWG. In the IWG fall planting, forage legumes were sown frost in March 2018, hand seeded in the inter-row, pushing the IWG biomass by hand to improve the seed-soil contact. The IWG row spacing factor had two levels: wide (57 cm) or narrow (38 cm) spacing. The seeding rate (11.2 kg ha−1) was the same for both row spacings, so the wide row spacing had ~50% more seeds per row than the narrow row spacing. The cropping system factor had 8 levels: four IWG monocultures [control without N fertilization or weed removal (IWG), hand weed removal (hand weeded), IWG fertilized with urea at rates of 45 or 90 kg ha−1], and four IWG-legume intercrops (IWG with alfalfa, Berseem clover, Kura clover, or red clover). The hand weed removal was bi-weekly in the years 2017 and 2018, and only in May and June in the year 2019. The urea for the fertilized monocultures was broadcasted in a split application during the spring, half of the rate was applied at green up and the other half at IWG stem elongation. The legume seeding rates for fall were higher than the spring ones following the recommendation from forages in Wisconsin according to the planting method (Table 1). The annual legume (Berseem clover) was re-sown every spring. None of the intercrops were fertilized or hand weeded. The experimental design for each planting season was randomized complete blocks with five replications. The column of plots was also included as a source of variation in the model.
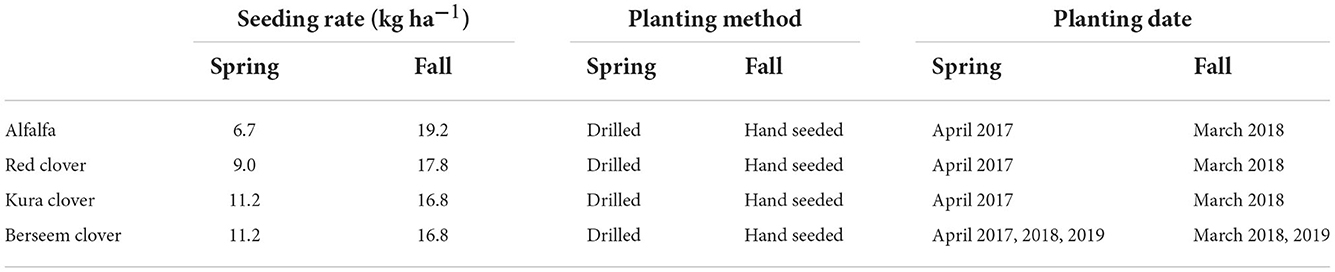
Table 1. Seeding rates and planting methods for legumes intercropped with IWG established in spring and fall at UW Arlington Research Station, Wisconsin, USA.
Data collection
Kernza grain yield and aboveground biomass were sampled approximately at physiological maturity on August 7, 2018, and August 1, 2019, from a 0.25 m2 quadrat randomly placed in each plot. Aboveground biomass was also sampled in fall, on October 27, 2017, and October 24, 2018. Grain yield was determined by cutting the spikes from all tillers within the quadrat. Spikes were dried at 35°C for at least 2 days, threshed with a mechanical seed thresher, and weighed. Aboveground biomass was cut by hand, separated into IWG forage, legume forage, and weeds, dried at 60°C for at least 5 days, and weighed. The quadrat was placed so that one row of IWG would fit inside the quadrat for the wide row spacing, and two rows for the narrow row spacing. Both in wide and narrow row spacing, one legume inter-row of the quadrat was sampled. Kernza grain yield, IWG forage, legume forage, and weed biomass data were adjusted proportionally to the number of rows within the sampled quadrat, to obtain yields in kilograms per hectare. After sampling, grain was harvested with a plot combine and aboveground biomass was removed from the experiment using a mechanical forage harvester (Almaco, FH-88) leaving a stubble height of 10 cm. In the years 2017 and 2019, yield data was collected in all five replications. In the year 2018, yield data was collected in three replications due to labor availability limitations.
Forage nutritive value
Forage samples of IWG, alfalfa, Berseem clover, Kura clover, and red clover were analyzed to characterize the different species and be able to estimate the forage nutritive value in the intercropping systems. Samples of IWG forage were harvested from the different cropping systems in the summer and fall of the establishment and first grain production years. Samples of the different legume species were harvested in the summer and fall of the establishment year. We assumed that all the species had the same forage quality in the first and second production years (Pinto et al., 2021c). Crude protein (CP), neutral detergent fiber (NDF), and acid detergent fibers (ADF) of the forage were analyzed using wet chemistry procedures and reported as a proportion of the dry matter. The selected samples were first ground with a Christy hammer mill (Christy-Turner Ltd, Ipswich, England) to pass a 1-mm screen. Total N was determined according to the Dumas combustion method (Method 990.03-AOAC, 2000) and the analysis was conducted in a LECO FP-528 (LECO Corporation, St-Joseph, MI). Crude protein was calculated as N × 6.25. Neutral detergent fiber and ADF were analyzed sequentially in an Ankom 2,000 Fiber Analyser (Ankom technology, Macedon, NY) according to the procedure of Robertson and Van Soest (1981) and modified by Hintz et al. (1996) to include sodium sulfite during refluxing. For the IWG-legume intercrops, CP, NDF, and ADF concentration of the mixture forage was calculated as the weighted average of intermediate wheatgrass and legumes based on their respective biomass proportion of the total forage accumulation. Relative Feed Value (RFV), an index that relativizes the nutritive value of forages to the fresh full-bloom alfalfa nutritive value, was calculated based on the following equations (Jeranyama and Garcia, 2004): Digestible Dry Matter = 88.9-(0.779 × % acid detergent fiber); Dry Matter Intake (% of body weight) = 120 / (% neutral detergent fiber); Relative Feed Value = (Digestible Dry Matter × Dry Matter Intake) / 1.29.
Economic analysis
The potential profitability to grain and forage production was calculated from current market rates and the estimated cost of production in Wisconsin, USA. Variable incomes of each cropping system were estimated from Kernza grain harvested in 2018 and 2019, and summer and fall forage harvested in 2017 (spring season planting only), 2018, and 2019. Fall forage was not evaluated in the second year (2019), so it was assumed to equal 90% of fall forage in the first year (Hunter et al., 2020b) to complete the potential total incomes per year. Kernza grain yield losses (41%) usually observed in early commercial harvesting were estimated from the difference between average grain observed in our experiment and the last harvest data report from The Land Institute (Skelly and Peters, 2021). Kernza grain prices before cleaning or dehulling were $3.30 kg−1 (Tessa Peters, 2022, pers comm). Forage price was assigned by comparing the RFV (of IWG forage or IWG + legume forage in the intercrops) with the Upper Midwest hay price by quality grade. For each species, the same RFV was used for the first and second grain production year. The prices for hay grade Prime (>151 RFV), Grade 1 (125 to 150 RFV), Grade 2 (103 to 124 RFV), and Grade 3 (87 to 102) were $0.23 kg−1, $0.18 kg−1, $0.13 kg−1, and $ 0.12 kg−1, respectively (Halopka, 2022), and for forage with <87 RFV was $0.10 kg−1. In addition, a payment for actively managing and expanding conservation activities, offered to farmers in Wisconsin by the Conservation Stewardship Program (CSP) from Natural Resources Conservation Service (NRCS) from the US Department of Agriculture (USDA), was included in the establishment year ($391 ha−1, NRCS-USDA, 2022b). Variable costs were estimated considering the different inputs applied for each crop system. Berseem clover and red clover seed price was $7.50 kg−1 (Albert Lea Seed, 2022a,b), alfalfa seed price was $9.92 kg−1 (Albert Lea Seed, 2022c), Kura clover seed price was 26.50 kg−1 (Welter Seed Honey Co., 2022). Licensing and fees were $12.4 ha−1 and 3% of the income. Fixed costs included IWG seed ($123 ha−1), crop establishment (seeding including labor, $137 ha−1), Kernza grain harvest ($64 ha−1), and forage harvest ($54 ha−1) (Tessa Peters, 2022, pers comm). The land cost rent was $329 ha−1 (Wisconsin Agricultural Statistics., 2022). In order to account for the opportunity cost of not using the land for another crop when Kernza is planted in the spring season, we estimated the value of forage harvestable of a 3-years-old Alfalfa pasture as $319 ha−1 and it was included as an income in the fall planting season (Extension Wisconsin., 2022).
Statistical analyses
All variables (i.e., Kernza grain yield, IWG and legumes forage yield, and weed biomass) were tested for normality and homogeneity of variances and transformed using square root to satisfy the assumptions of ANOVA. Different models were used to test specific hypotheses. First, in order to test the effects of the intercropping on the yields, we conducted an analysis of variance on Kernza grain yield and, IWG and legume forage with year (Y) as a repeated measure (covariance structure of compound symmetry); planting season (PS), cropping system (CS, including only the IWG monoculture control and the four intercropping treatments), row spacing (RS), and their interaction as fixed effects and block and column as random effects. Since these analyses showed a RS*PS*Y interaction effect on grain and forage yields, we performed a follow-up analyses of variance by year.
Second, in order to test the effects of the intercropping on the forage quality, we conducted an analysis of variance on the nutritive value metrics (percent CP, NDF, ADF, and RFV) with species, harvest season, and their interaction as fixed effects. Usually, the nutritive value metrics are rather constant over the years (Pinto et al., 2021c) but as IWG is in a vegetative state in the summer of the establishment year and in a reproductive state in the summer of the following years we considered both phenological states. Then, %CP and RFV of IWG-legume intercropping, estimated as the weighted average of IWG and legume forage and their nutritive values, were compared with %CP and RFV of IWG monoculture.
Third, in order to test the effects of the intercropping on the economic results, we conducted an analysis of variance on the annual profit ($ ha−1 year−1) with PS, CS, and their interaction as fixed effect. In this analysis, row spacing was not included because different row spacings have the same costs (i.e., no changes in seeding rate) and similar incomes (i.e., little grain and forage variation) in our experiment. Since the Kernza® grain price is in a discovery phase, we performed analyses of variance by the % of the current Kernza grain yield utilized in the calculation (i.e., 100, 75, or 50% of the current Kernza grain price).
Fourth, in order to test the effect of management practices on IWG monocultures yields only, we conducted an analysis of variance on Kernza grain yield, IWG forage, and weed biomass by year, considering the effects of management (fertilization and weed removal), planting season (PS), row spacing (RS), and their interactions as fixed and block and column as random. Finally, since we found differences in the weed biomass, we compared the IWG monoculture with different weed management and IWG intercrops with different legumes. We conducted an analysis of variance on weed biomass by year, with PS, CS, RS and their interaction as fixed effects and block and column as random effects. All analyses were performed using PROC MIXED procedure in SAS (SAS on Demand, SAS Institute, Cary, North Carolina, USA). Means were compared using the Tukey-Kramer honest significant difference test at α = 0.05. Graphs were created using the ggplot2 (Wickham, 2009) package in RStudio Team. (2020).
Results
IWG-legume intercropping
The IWG cropping systems (IWG monoculture control and intercrops) had a high variability in grain and forage yields explained by row spacing, planting season, year, and their interactions (Supplementary Table 1). In the first grain production year, IWG planted at 38 cm of row spacing in the fall planting season yielded more than in the spring planting season (867 and 447 kg ha−1, respectively, p < 0.01, Figure 2A). However, when IWG was planted at wider row spacing (57 cm), there was no difference between planting seasons (800 kg ha−1, p = 0.35, Figure 2A). Overall grain yields decreased 85% in the second year regardless of the planting season or the row spacing (Figure 2A). The IWG forage remained relatively stable between years while that of legumes increased (Figures 2B,C). In the first year IWG forage was higher when it was planted in the fall at 57 cm of row spacing than when it was planted in the spring at 38 cm of row spacing (4,180 and 2,160 kg ha−1, respectively, p < 0.01, Figure 2B). The IWG forage remained rather constant between the first and second grain production year for most cases except for IWG planted in fall at 57 cm where IWG decreased 41% in the second year (Figure 2B). The legume forage was rather constant between different planting seasons or row spacing but consistently increased in the second grain production year (Figure 2C). As a result, whereas the legume forage was 12% of the total summer forage in the summer of the first year, it increased to 47% in the second grain production year (Figures 2B,C).
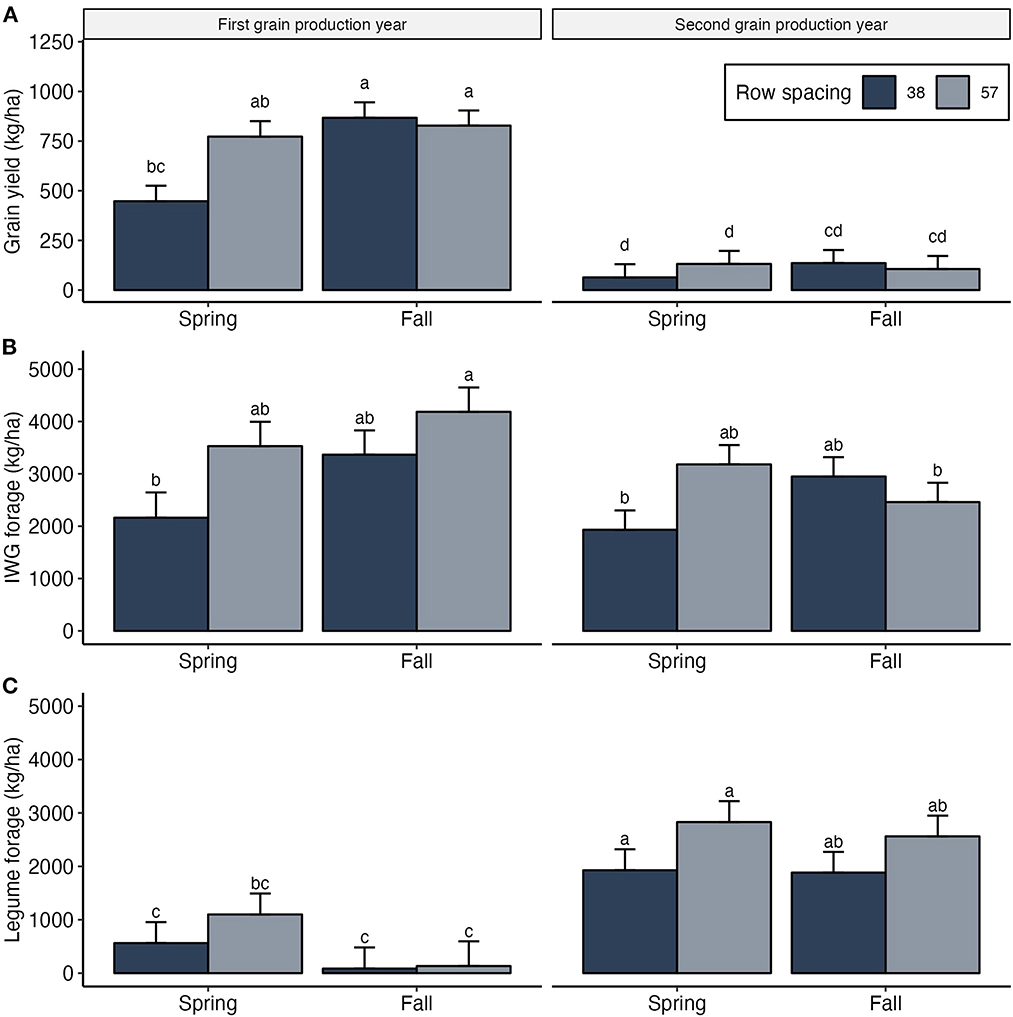
Figure 2. Grain (A), and IWG (B) and legume (C) forage yields (kg ha−1) of the first (2018) and second (2019) grain production year for five IWG cropping systems sown at two row spacings (38 or 57 cm) in two planting seasons (spring or fall 2017), at Arlington, Wisconsin, USA. The cropping systems are IWG monoculture control without N fertilization or weed removal, and four IWG-legume intercrops (IWG with Berseem clover, Kura clover, red clover, or alfalfa). Same letters for each variable indicate no differences at alpha = 0.05.
The planting season and the legume species intercropped with IWG affected the grain and forage yields (Supplementary Table 1). When IWG was planted in the fall, the intercropping systems had similar grain yields to IWG monoculture, regardless of the legume species (Figure 3A). However, when IWG was planted in the spring, grain yields for intercrops with Berseem clover (1,160 kg ha−1) or Kura clover (652 kg ha−1) were similar to IWG monoculture, whereas red clover and alfalfa intercrops had lower grain yields (24 and 136 kg ha−1, respectively, Figure 3A). In the second grain production year, all intercropping systems had similar grain yields to the monoculture in both planting seasons, except for red clover intercrop in the fall, which had lower grain yields (Figure 3B). The row spacing effect was also different depending on the cropping system (p-value row spacing * cropping system * planting season interaction = 0.01). IWG-Kura clover intercrop planted in spring was the only cropping system with higher Kernza grain yield at wider row spacing (1,050 kg ha−1 at wide vs. 256 kg ha−1 at narrow). The rest of the cropping systems had similar Kernza grain at different row spacing independently of the planting season.
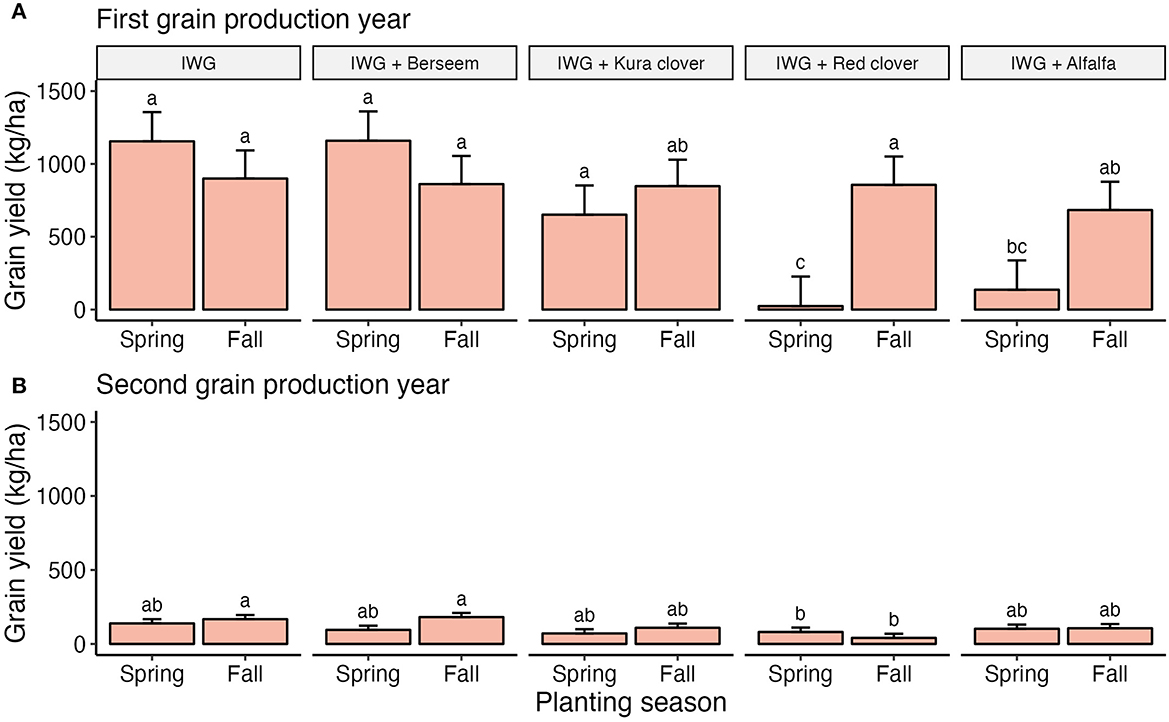
Figure 3. Grain yields (kg ha−1) of the first (2018, A) and second (2019, B) grain production years for IWG monoculture without N fertilization or weed removal (IWG) and four IWG intercrops with annual (Berseem clover) or perennial legumes (Kura clover, red clover, alfalfa) sown in two planting seasons (spring or fall 2017), at Arlington, Wisconsin, USA. Same letters indicate no differences at alpha = 0.05.
The forage yields had a similar response to the grain yield response: they were lower in the IWG planted in the spring intercropped with red clover (Supplementary Table 1). In the establishment year (2017), when forage can be harvested only for IWG planted in the spring, the intercropping with red clover had lower IWG forage yield (422 kg ha−1) than the monoculture and the rest of intercrops (1,300 kg ha−1 on average, Figure 4A). In the first grain production year (2018), the IWG-legume intercrops had similar IWG summer forage yield to the IWG monoculture planted in the fall (3,730 kg ha−1 on average, Figure 4B). However, when planted in the spring, IWG intercropped with red clover or alfalfa had lower IWG summer forage yield (152 and 744 kg ha−1, respectively) than the IWG monoculture or the rest of the intercrops (4,610 kg ha−1 on average). Only red clover and alfalfa had differences on IWG summer forage yield between the spring and fall planting (Figure 4B). Usually, the legume summer forage yield did not compensate for low IWG summer forage yield. Red clover and alfalfa had the lowest total summer forage yield although they produced~1,100 kg ha−1 of legume summer forage (Figure 4B). In contrast, legumes tend to increase total fall forage yield in the first grain year production, although only the Kura clover intercrop had higher total fall forage yield than the IWG monoculture when both were planted in the spring (2,420 vs. 1,140 kg ha−1, respectively, Figure 4C). Finally, in the second grain production year, most of the intercrops had similar IWG summer forage yield to the IWG monoculture except for the red clover intercrop, which had lower yield. The intercropping systems with the least legume biomass accumulation were IWG with Berseem clover and alfalfa in both planting seasons. Their biomass were lower than Kura clover legume biomass in the spring planting and lower than that of red clover in the fall planting (Figure 4D). The summer Kura clover forage yield was more than half of the total forage yield in the second year, resulting in higher total summer forage yield than the IWG monocrop (7,160 vs. 2,930 kg ha−1, respectively when both were planted in the spring, Figure 4D).
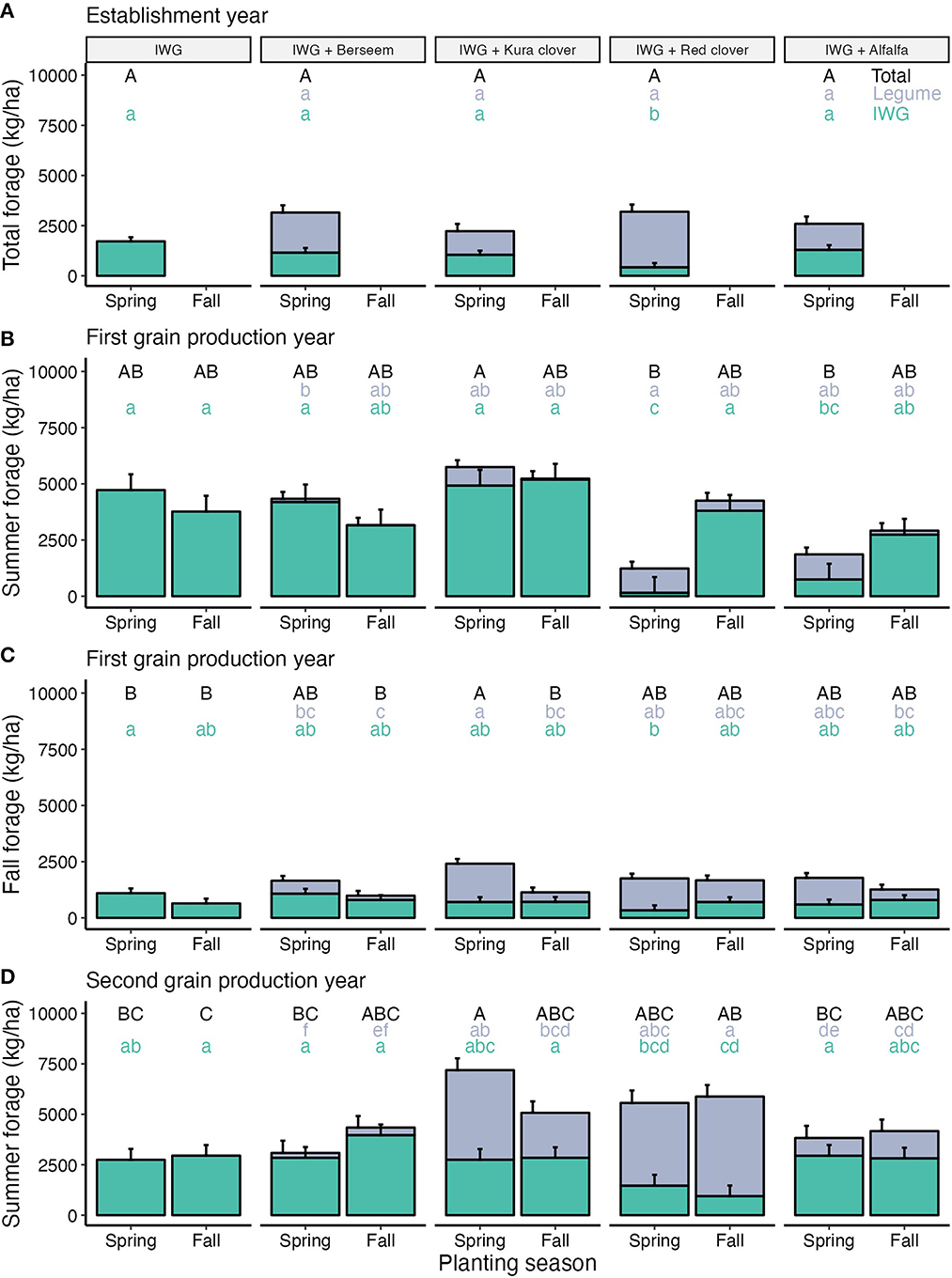
Figure 4. IWG and legume forage (kg ha−1) harvested in the establishment year (A), in the summer (B) or fall (C) of the first grain production year, and in the summer of the second grain production year (D) for IWG monoculture (IWG) and four IWG intercrops with annual (Berseem clover) or perennial legumes (Kura clover, red clover, alfalfa) sown in two planting seasons (spring or fall 2017), at Arlington, Wisconsin, USA. Same letters indicate no differences at alpha = 0.05 in IWG forage (green lowercase letters), legume forage (purple lowercase letters) or total forage (black capital letters).
Overall, the forage yield in the establishment year and in the fall of the first production year was lower than the forage yield in the summer of the first production year but had higher nutritive value. In the summer of the establishment year, IWG and legumes had similar percentages of CP, NDF, ADF, and RFV. In the fall of the establishment year, alfalfa forage had higher CP and RFV than IWG forage, whereas other legumes had intermediate values (Table 2). In the first grain production year, red clover forage harvested both in summer and fall, had similar CP, NDF, ADF, and RFV to legumes in the establishment year. Instead, in the summer of the first grain production year IWG had the lowest percent CP (Table 2). Considering the legume proportion of the total forage harvested in each cropping system and the nutritive values of the IWG and the legumes, we estimated that some intercrops had better nutritive value than IWG monoculture. On the one hand, the fall harvested forage of IWG planted in the spring and intercropped with Kura clover, red clover or alfalfa had higher CP and the RFV than that of IWG monoculture (Figure 5). The fall forage of IWG planted in the fall intercropped with red clover also had higher nutritive value than the IWG monoculture, but the intercrop with other legumes did not. All these CP and RFV increases meant positive changes on the hay quality designation. On the other hand, the IWG monoculture summer forage was classified as “fair” while the intercrop with red clover and alfalfa reached “premium” or “grade 3–4” when IWG and the legumes were planted together in the spring (Figure 5).
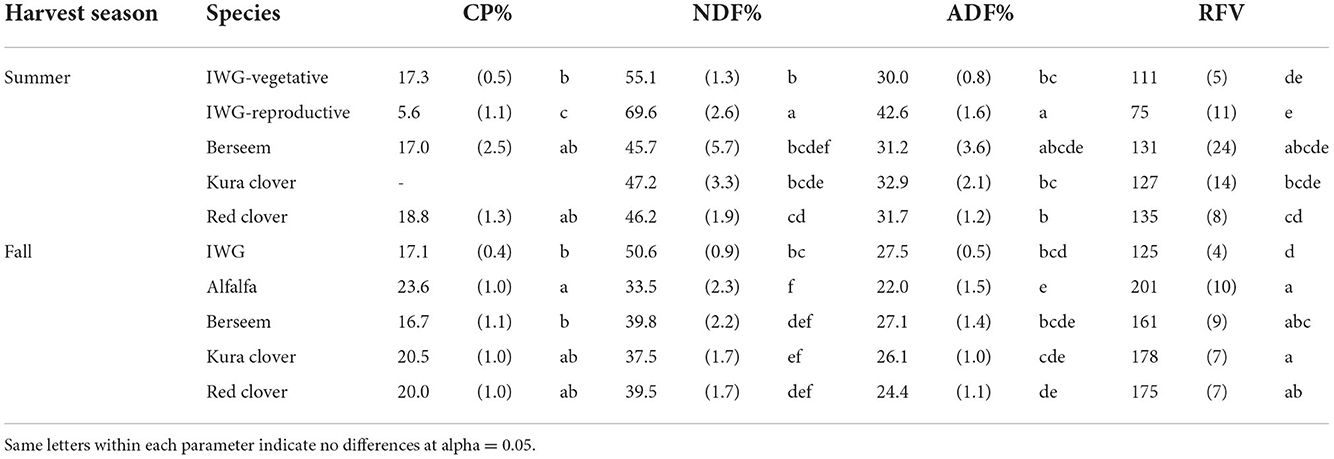
Table 2. Means (standard errors) for crude protein (CP), neutral detergent fiber (NDF), acid detergent fiber (ADF), and the relative feed value (RFV) of intermediate wheatgrass (IWG), alfalfa, Berseem clover, Kura clover and red clover forage harvested in the summer and fall of the establishment and the first Kernza grain production years (IWG-vegetative was harvested in the summer of the establishment year and IWG-reproductive, in the summer of the first grain production year).
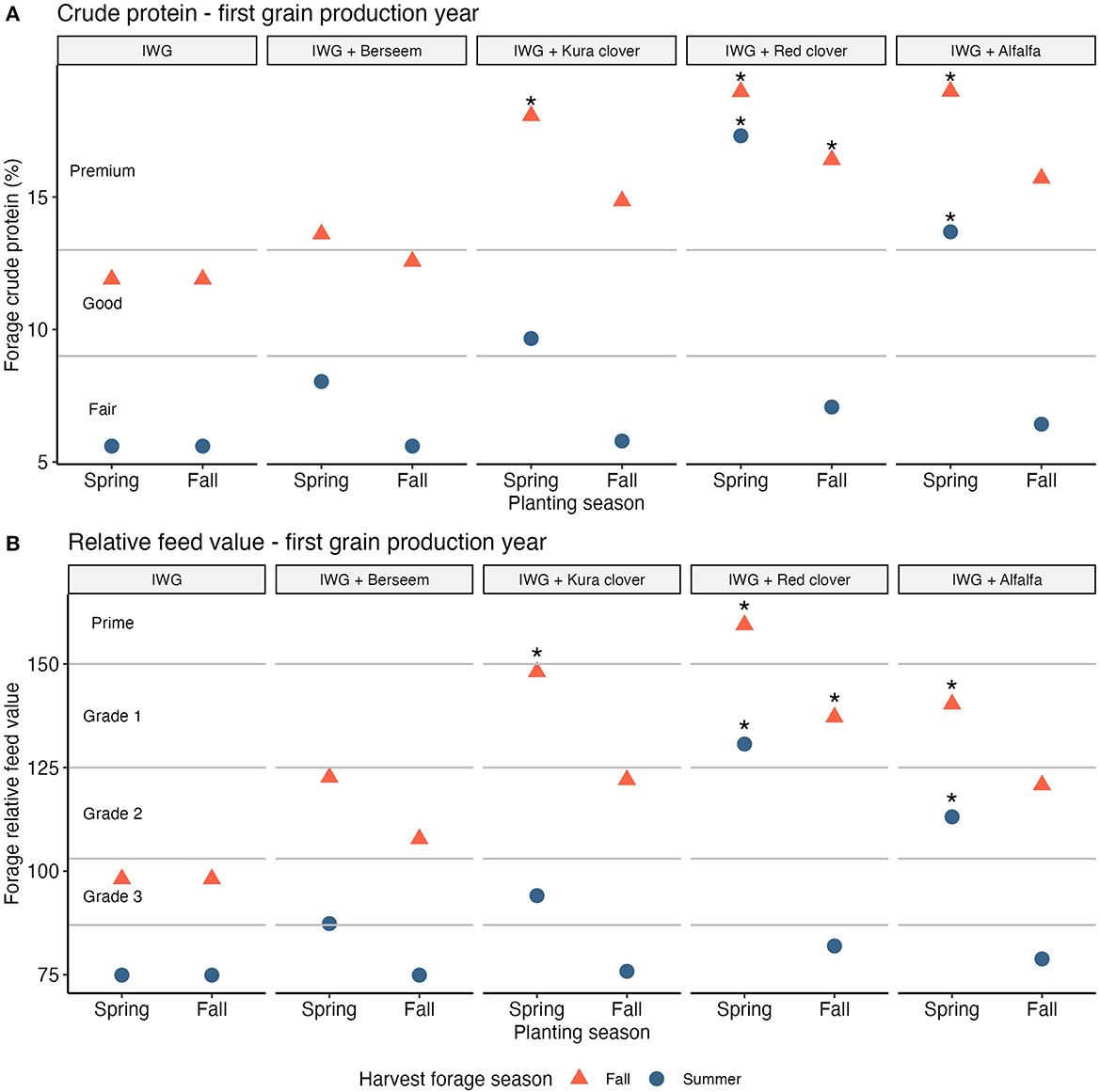
Figure 5. Percent crude protein (A) and relative feed value (B) of the total forage (IWG + legumes) harvested in the summer or fall of the first grain production year (2018), for the control IWG monoculture (i.e., without N fertilization or weed removal), and four IWG intercrops with legumes (Berseem clover, Kura clover, red clover, alfalfa) sown at two planting seasons (spring, fall), at Arlington, Wisconsin, USA. Gray lines show the limits of the forage quality grade according to Halopka (2022) and USDA (2022). The asterisks (*) indicate differences with the minimum value (control IWG monoculture) at alpha = 0.05.
Economic analysis
Mean potential profitability to grain and forage production varied between $260 and $961 ha−1 year−1 (Table 3). Cost and incomes were highly variable among cropping systems between years (Supplementary Table 2). In the establishment year, costs varied between $589 and $994 ha−1 whereas incomes varied between $682 and $976 ha−1 among cropping systems. The subsidy for planting perennial crops represented from 40 to 57% of the income in the establishment year and the net returns were lower than in the grain production years (Supplementary Table 2). The first grain production year had higher profitability than the other years, except when IWG was planted in the spring intercropped with red clover (Supplementary Table 2). The sale of the Kernza grain represented 60% of the total income ha−1 year−1 in the IWG monoculture cropping system and varied between 8 and 55% in the intercropping systems (Table 3). The highest profitability per year was $898 ha−1 year−1 in the IWG intercropped with Kura clover planted in the spring and $961 ha−1 year−1 in the IWG intercropped with red clover planted in the fall. Most of the cropping systems had similar annual profit, except for IWG intercropped with alfalfa planted in the spring ($260 ha−1 year−1, Table 3). The sensitivity analysis indicated that a change in the price of Kernza grain has a little impact in which cropping systems are most profitable, as the pattern of significant differences is largely the same at all three Kernza grain price considered (Table 3). The influence of the Kernza grain price on the annual profit was high but variable among the cropping systems. Assuming a Kernza grain price reduction of 25 and 50%, the annual profit had similar reductions in the IWG-control cropping system (26 and 52%, respectively, Table 3). In contrast, the Kernza grain price reduction tended to impact less on the red clover intercropping system's annual profit (reductions of 10 and 20%, respectively, Table 3) indicating that this cropping system is more highly dependent on forage production than grain.
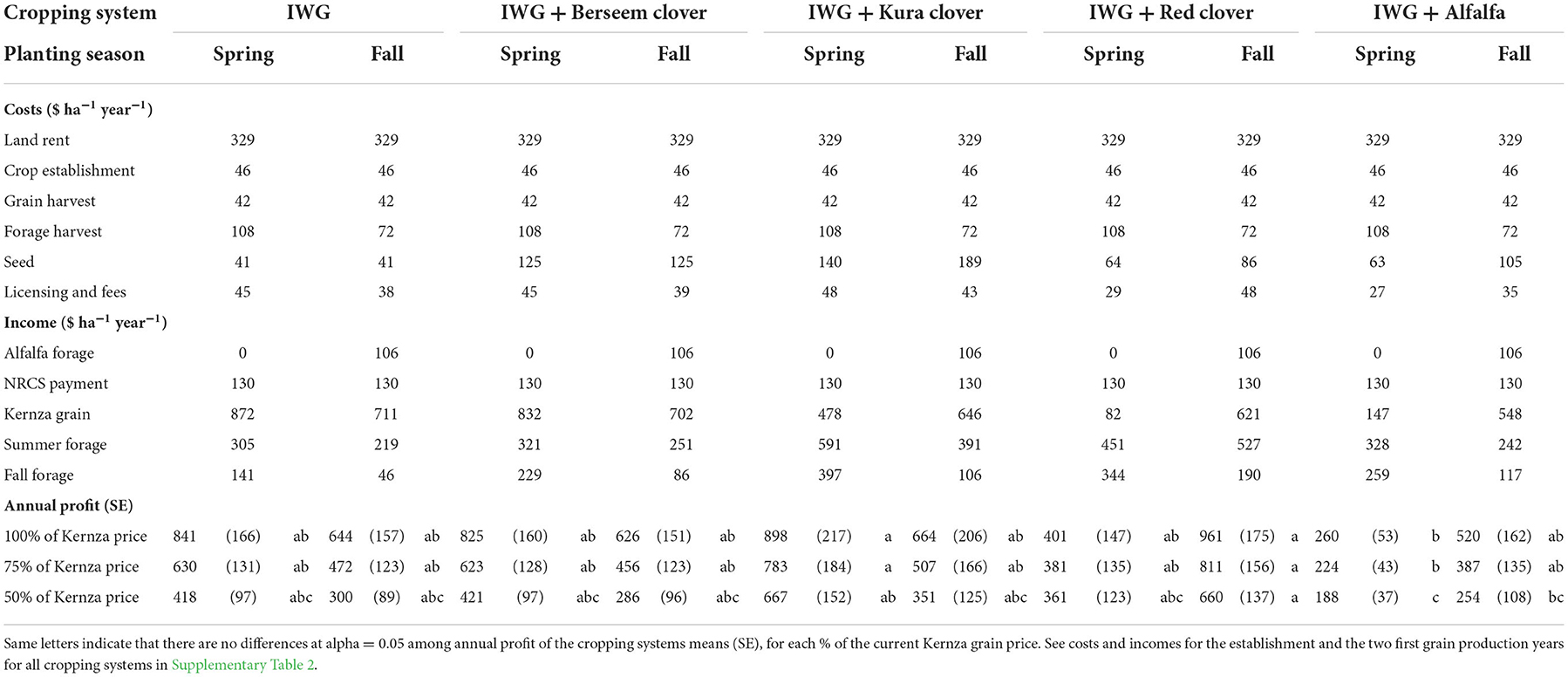
Table 3. Mean costs, income, and annual profit ($ ha−1year−1) of five different cropping systems planted in spring or fall 2017, at both narrow and wide row spacing: IWG monoculture without N fertilization or weed removal (control), and IWG intercropped with Berseem clover, Kura clover, red clover, or alfalfa, for three Kernza grain prices.
IWG management practices on yields and weeds
The IWG monocultures had high variability in grain and forage yields but this was more explained by year than by row spacing, management, or planting season effect. The Kernza monoculture grain yields were 945 ± 73 kg ha−1 in the first grain production year and decreased to 147 ± 10 kg ha−1 (p < 0.01) in the second year. The IWG summer forage (straw) yields were 4,370 ± 308 kg ha−1 in the first grain production year, and 3,490 ± 235 kg ha−1 in the second year (p < 0.01). No response to different N fertilization rates or weed removal (i.e., management practices) was found in grain and IWG forage yields in the first grain production year (Supplementary Table 3). In the second grain production year, spring planting at wide row spacing and fall planting at narrow row spacing had higher grain yields than spring planting at narrow row spacing (173 kg ha−1 on average and 91 kg ha−1, respectively, Supplementary Table 3). The IWG forage yield was higher in both spring and fall planting at wide row spacing than in spring at narrow row spacing (3,740 kg ha−1 on average, and 2,750 kg ha−1, respectively, Supplementary Table 3). In contrast to the first year, the management practices affected the IWG forage yield (Supplementary Table 3): the IWG fertilized with urea at 45 kg ha−1 had higher IWG forage than IWG unfertilized hand weeded (4,280 kg ha−1 and 2,950 kg ha−1, respectively).
As expected, weed summer biomass was lower in the hand weeded plots (203 kg ha−1) than the control (1,900 kg ha−1) in the first year, when the weeds were weekly removed. However, it was similar in the second year when weeds were only removed twice (1,310 kg ha−1, mean of both, Figure 6). In the second year, summer weed biomass was lower at narrow row spacing (1,010 kg ha−1) than at wide row spacing (1,760 kg ha−1) in both spring and fall plantings (Supplementary Table 3). Kura clover and red clover were effective in reducing weed biomass. In the first year, both intercropping systems had similar weed biomass to the hand weeded treatment (Figure 6). In the second year, weed biomass decreased 39% on average among the different cropping systems (from 1,400 kg ha−1 in the first year to 851 kg ha−1 in the second). Weed biomass was lower in the intercrops with red clover and Kura clover than in the hand weeded treatment (87, 317, and 1,166 kg ha−1, respectively, in the second year).
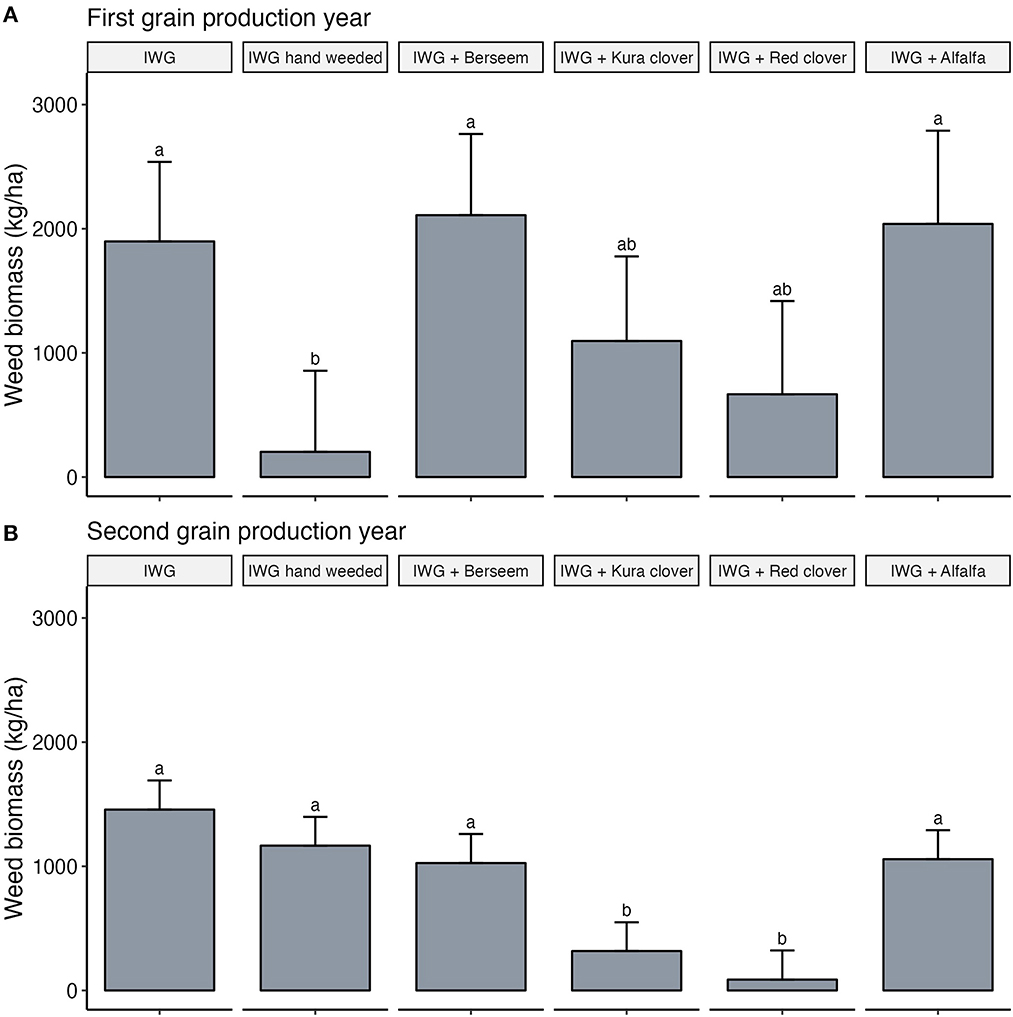
Figure 6. Weed biomass (kg ha−1) of the first (2018, A) and second (2019, B) grain production years for two IWG monocultures (IWG: without N fertilization or weed removal, IWG hand weeded: with hand weed removal) and four IWG intercrops with annual (Berseem clover) or perennial legumes (Kura clover, red clover, alfalfa) sown in two planting seasons (spring or fall 2017), at Arlington, Wisconsin, USA. Same letters indicate no differences at alpha = 0.05.
Discussion
IWG management practices
The N fertilization and the weed removal management in IWG monocultures are useful to understand the potential limitations by N or weed competition in our experiment. The lack of Kernza grain yield response when weeds were removed by hand, suggest that interspecific competition may not be a problem as IWG is well established and accumulated ~4,200 kg ha−1 of IWG aboveground biomass in the summer. However, most of the weeds present at the beginning of the experiment were annuals, and therefore, different results are likely found in fields dominated by perennial weeds (Zimbric et al., 2020). On the other hand, N fertilization usually mitigates the decline in grain yield with stand age (Jungers et al., 2017; Tautges et al., 2018; Fernandez et al., 2020) but no effect was found in grain or IWG forage in our experiment. Likely, both the high initial N soil content and the fact that the previous crop was a legume avoided N limitation in the IWG control cropping system. In the following years, N is likely to become limited because of the N exportation with the harvest of Kernza grain and IWG forage. Without any other N fertilization, the legumes intercropped would need to accumulate approximately 4,500 kg of biomass ha−1 to provide enough N to meet IWG demands considering that 50% of the N uptake by the legumes comes from biological fixation (Pinto et al., 2021b).
The positive response of planting at wider row spacing was lower than we expected. Based on previous experiences, we hypothesized that increasing the distance between rows would help maintain grain allocation over time (Canode, 1968; Hunter et al., 2020a). However, large declines in the allocation to grain were consistently observed in our experiment since grain yield declined whereas forage remained rather constant over time. A possible reason for the limited effect of row spacing is that the different row spacing treatments had the same seeding density per area in our experiment. This means that the wide row spacing treatment has a higher seeding density per row than the narrow, so higher row competition could likely have confounded the effects. Future research should maintain density per row in wide row spacing (i.e., reduce density per hectare) to see if increasing resources per plant in wide row spacing allows yields to be maintained in older stands.
In general, planting IWG and the legumes together in the spring was no better than planting in the fall. Although it is widely known that IWG does not produce grain in the summer of the establishment year when it is planted in the spring due to lack of vernalization induction (Duchene et al., 2021; Olugbenle et al., 2021; Jungers et al., 2022; Locatelli et al., 2022), we hypothesized that some advantages could be manifested in the first grain production year. However, growing IWG for a longer establishment period (i.e., with more rainfall and GDD accumulated when IWG is planted in the spring) did not lead to higher grain or IWG forage in the first grain production year than planting in the fall. In the IWG-legume intercropping systems, planting IWG and legumes together in the spring tended to favor the growth of legumes. Higher legume biomass accumulation usually implies a higher N contribution by biological N fixation (Pinto et al., 2021b) but in our experiment this potential N contribution was not relevant since no response to N fertilization was seen in the IWG monocultures. In contrast, high biomass accumulated by red clover and alfalfa, which are legumes well adapted to Wisconsin (Sheaffer et al., 2020), compromised the establishment of IWG and its grain and forage yields.
IWG-legume intercropping
Most of the legume species intercropped with Kernza were good companions of IWG since they did not compromise the Kernza grain and IWG forage yields (Figure 7). Overall, Kernza grain yields were highly variable in the first year with differences found only when IWG was not well established. The low Kernza yields achieved by IWG intercropped with red clover or alfalfa in the spring planting suggest that the early IWG biomass accumulation is key. As these legumes' establishment is aggressive (Tautges et al., 2018), legume frost seeded in the spring on IWG planted in the previous fall has been recommended (Law et al., 2021; Olugbenle et al., 2021). The other cropping systems had a wide grain yield range consistent with previously reported yields for 1-year-old stands (Franco et al., 2021). Summer forage yields had a similar response to treatments as Kernza grain yields. Except for IWG intercropped with red clover and alfalfa planted in the spring, the cropping systems had summer forage yields within the range previously reported (Franco et al., 2021). In the second year, summer forage yields decreased but at a slower rate than grain yields. This reduction in grain allocation found in older IWG stands is consistent with previous results (Tautges et al., 2018; Fernandez et al., 2020; Law et al., 2021) although in most of them this was associated with summer forage yield increases.
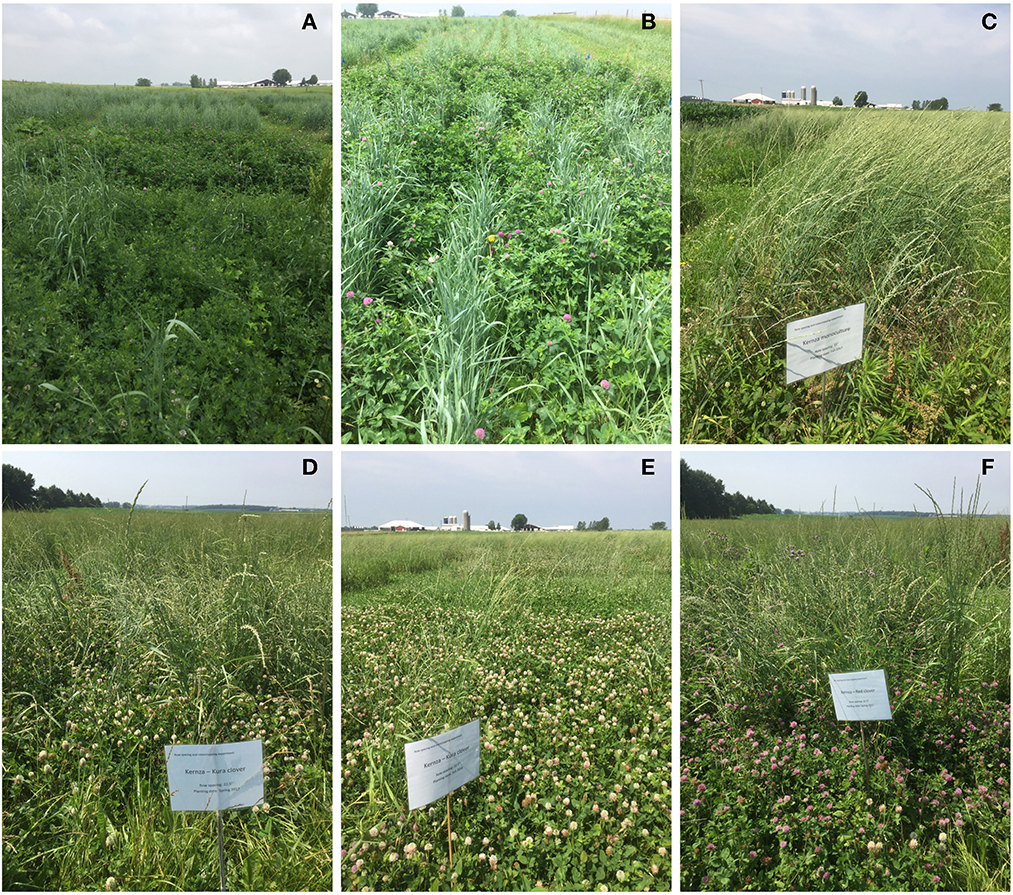
Figure 7. Pictures of intermediate wheatgrass cropping systems in Arlington, Wisconsin, USA: (A) IWG-alfalfa intercropping, spring planting (date picture taken: June 12, 2018); (B) IWG-red clover intercropping, spring planting (June 12, 2018); (C) IWG monoculture control, fall planting (July 9, 2019); (D) IWG-Kura clover intercropping, spring planting (July 9, 2019); (E) IWG-Kura clover intercropping, fall planting (July 9, 2019); (F) IWG-red clover intercropping, spring planting (July 9, 2019).
The benefits of intercropping IWG with legumes were more related to an improvement on nutritive value than on the amount of total forage harvested. Although previous studies had shown increases in the total forage harvested in IWG intercropped with red clover (Favre et al., 2019; Law et al., 2022); in our experiment red clover biomass production did not compensate for the decrease in IWG biomass, resulting in similar total forage yields. The most promising legume to increase the total forage was Kura clover when it was planted with IWG together in the spring even though the greatest differences were seen after the first grain harvest (i.e., fall of the first grain production year and summer of the second grain production year). In contrast, all the perennial legumes improved the forage nutritive value, as seen previously in IWG-red clover intercropping (Favre et al., 2019). Intercropping IWG with red clover, Kura clover, or alfalfa was associated with increases in the hay quality designation, increasing the price per kg of forage (Halopka, 2022; USDA, 2022).
We hypothesized that annual legumes would be better companions than perennial legumes, but scarce biomass was accumulated by annuals in our experiment. Annual legumes could be good alternative companions in the long term since the biomass accumulation by perennial legumes tends to increase as stands get older (e.g., Figure 2, Tautges et al., 2018) and competition with IWG could become limiting. However, in our experiment the biomass accumulated by the annual legume Berseem clover was insufficient to provide increases in the total forage harvested or its nutritive value. Initially we had incorporated soybean (Glycine max (L.) Merr.) as another annual legume companion, but we decided to exclude this treatment from our analysis because the soybean biomass was negligible (data not shown). This treatment tended to yield more Kernza grain than the other intercrops, especially when IWG was planted in the fall. The positive effect was unlikely to be due to changes in N levels but probably because the soil in the inter-row was tilled before soybean planting potentially creating beneficial effects such as those observed under mechanical thinning (Law et al., 2020; Pinto et al., 2021a). Proper management of annual legumes could lead to better intercropping results but the need to plant them every year limits its benefits. Therefore, it seems more promising to learn to regulate the competition between IWG and perennial legumes, for example with forage cuttings, than to intercrop with annual legumes.
Economic analysis
Most of the cropping systems had higher profitability than a 3-years-old Alfalfa pasture ($319 ha−1, Wisconsin Agricultural Statistics., 2022), soybean ($421 ha−1, Economic Research Service., 2022a) or corn ($637 ha−1, Economic Research Service., 2022b) except when IWG was poorly established in the red clover and alfalfa intercrops planted in the spring. However, this high profitability is explained by the high Kernza® grain price and the subsidy for its ecosystem services provision. A 50% reduction in Kernza grain price would make only the best-performing IWG systems competitive with corn and soybean. Kernza® grain markets are in a price discovery phase with prices varying significantly by the management system, year, region, and grain quality. The price included in this paper falls in the middle of the range of observed 2021 farmgate prices for non-organic Kernza grain. Current target and received grain prices reflect significant risks in early commercial Kernza production and marketing. The multiple uncertainties involved with Kernza represent the main disadvantage perceived by the farmers who decide to plant this crop (Lanker et al., 2020). Although Kernza production can be quite profitable where it succeeds, several growers still fail at IWG establishment, experience major weed pressure, do not meet food-grade specifications, and have limited market access. These relatively high risks also explain Kernza's relatively high price (Tessa Peters, 2022, pers comm). Progress in the genetics and management of Kernza IWG will likely lead to less risky scenarios but with lower and more stable prices. That means, the projected net returns given by our relatively strong prices and yields, should be considered optimistic and do not fully encompass the risks of commercial Kernza production and marketing at this time (Tessa Peters, 2022, pers comm).
Our results suggest that red clover and Kura clover are good companions of IWG, given their high profitability at any Kernza grain price considered. Kura clover and IWG can be planted together in the spring, but IWG must be planted in the fall and red clover in the next spring to see the benefits. The legume forage contribution seems to be key to buffer the potential impact of kernza grain price volatility on the overall profitability. Both higher quantity and quality of the forage harvested in the intercropping systems than in the IWG monoculture lead to increases in the income from forages. As a result, the proportion of revenue coming from Kernza grain decreases as well as the impact of Kernza grain price volatility. This means a great potential for intercropping in the future. If Kernza grain yields increase from breeding advances or agronomic management innovations without sacrificing forage yield or quality in these best intercropping systems, it would be a win-win from a production standpoint and increase the crop's economic viability even if grain prices are reduced.
The current ecosystem services value to growers, communities, or society is reflected in the subsidy provided by the Conservation Stewardship Program (NRCS-USDA, 2022b). However, the IWG's key role in preventing nutrient leaching (Culman et al., 2013; Jungers et al., 2019) and improving soil health (Culman et al., 2010; de Oliveira et al., 2020) can be also reflected in the access to new markets in the future, such as water and carbon credits. Ecosystem services, such as water quality, soil health, carbon sequestration, and biodiversity, are appreciated by people, but the incentives for the provision that comes with prices are incipient (Swinton et al., 2007). Understanding how humans perceive and value ecosystem services is key, but a lack of low-cost measurability and valuation currently precludes efficient allocation of many ecosystem services through market-based approaches (Kroeger and Casey, 2007). How to rigorously incorporate these benefits into economic analyses of cropping system performance warrants more research conducted with a transdisciplinary approach.
Limitations and future perspectives
Interpretation of how planting season determined our results is limited because our experiment was not replicated in time or space. This means that the effect of stand age and weather on grain and forage yields cannot be separated. Our experiment was installed in spring and fall of a year wetter than normal (year 2017). In normal or drier years, the interaction between Kernza IWG and legumes could be different and therefore, it should be studied in other environments. For example, in our experiment the intercropping with red clover or alfalfa planted in the spring season seems to be risky because it limited the IWG establishment. However, in other environments or using different management practices (e.g., different seed rate, different cutting regiment, spring forage harvest), legume may have less of a competitive advantage over IWG, resulting in a viable intercrop. Future experiments should consider repeated plantings in consecutive years as recommended to evaluate perennial forage grasses (Casler, 1999). Besides, considering other environments or trying different management strategies for intercropping will help to have more tools to design more diverse cropping systems.
On the other hand, the lack of some measurements led us to rely on assumptions to interpret our results. In the second grain production year, the fall forage production was not evaluated and we assumed a 10% reduction of the first year forage according to the annual averages published by Hunter et al. (2020b) (3,000 kg/ha in 2015 and 2,700 kg/ha in 2016). This allowed us to estimate the total potential incomes of each year and calculate the annual profit but different intercropping could differently affect forage yields in the second year. In addition, weed biomass was not measured in the fall forage harvest nor considered to determine the forage nutritive value. Both in the summer and fall forage, the nutritive value was determined considering the %CP and RFV of IWG and legumes and their proportion in the mixture. However, the analysis of composite samples of all the forage harvested in the plot could differ due to the impact of the weed biomass. Lastly, taking measurement in the five blocks in the first year would have helped to better characterize the first grain and forage productions.
The design of dual-purpose intercrops is a promising practice that should be carefully evaluated considering multiple dimensions (Duchene et al., 2017; Crews et al., 2018; Law et al., 2021). The possibility to harvest forage twice a year provides an additional source of income and is often beneficial for the Kernza grain yield maintenance. Usually, the high presence of straw and biomass residues in older Kernza IWG stands reduces the resource allocation to grains because reproductive tiller initiation is reduced by shade (Ensign et al., 1983; Chastain, 2003). Therefore, harvesting forage could help to maintain a high harvest index to avoid the grain declines commonly observed (Pugliese et al., 2019; Hunter et al., 2020b; Pinto et al., 2021a). In dual-purpose crops where the forage represents an important proportion of the total income, the mixture with legume helps to improve the forage value (Favre et al., 2019; Halopka, 2022). These advantages make it feasible to include perennial and diverse cropping systems in agricultural rotations to improve their sustainability.
Conclusion
Dual-purpose IWG-legume intercropping systems are promising alternative production systems but both legume species and intercrop management techniques should be carefully chosen to favor the benefits. Most of the intercropping systems achieved similar Kernza grain and forage to IWG monoculture and improve the forage quality. However, our results suggest that when IWG is planted in the spring, intercropped with red clover or alfalfa, the Kernza grain and the IWG summer forage can be reduced by an early high competition. The intercropping with Kura clover or red clover was as effective in weed suppressing as the hand-removal management in the IWG monoculture. In the second year, Kernza grain yields decreased consistently in all cropping systems. In our experiment, planting in a wide row spacing did not prevent the grain yield decline but reducing the seeding rate per hectare in the wide row spacing could lead to different results. Overall, the profitability of the IWG legume intercropping was high mainly due to the current high Kernza grain price and the subsidies provided to farmers in Wisconsin to encourage the adoption of dual-purpose perennial crops.
Data availability statement
The original contributions presented in the study are included in the article/Supplementary material, further inquiries can be directed to the corresponding author.
Author contributions
VP, DS, and PP contributed to conception and design of the study. SC-C and JZ collected and organized the database. PP and SC-C performed the statistical analysis. PP, SC-C, CC, and AS wrote sections of the manuscript. PP wrote the first draft of the manuscript. All authors contributed to manuscript, revision, read, and approved the submitted version.
Funding
This work was supported by Kernza CAP AFRI Sustainable Agricultural Systems Coordinated Agricultural Program (SAS-CAP) grant no. 2020-68012-31934 from the USDA National Institute of Food and Agriculture and USDA Hatch grants to VP and DS.
Acknowledgments
We thank forages and perennial grains technicians N. Leete, H. Ghebrehiwot, E. Bures, and undergraduate and graduate students in the lab.
Conflict of interest
The authors declare that the research was conducted in the absence of any commercial or financial relationships that could be construed as a potential conflict of interest.
Publisher's note
All claims expressed in this article are solely those of the authors and do not necessarily represent those of their affiliated organizations, or those of the publisher, the editors and the reviewers. Any product that may be evaluated in this article, or claim that may be made by its manufacturer, is not guaranteed or endorsed by the publisher.
Supplementary material
The Supplementary Material for this article can be found online at: https://www.frontiersin.org/articles/10.3389/fsufs.2022.977841/full#supplementary-material
References
Albert Lea Seed (2022a). Berseem clover. Available online at: https://alseed.com/product/frosty-berseem-clover/ (accessed March, 2022).
Albert Lea Seed (2022b). Red clover. Available online at: https://alseed.com/product/medium-red-clover/ (accessed March, 2022).
Albert Lea Seed (2022c). Alfalfa. Available online at: https://alseed.com/product/viking-394ap-brand-alfalfa/ (accessed March, 2022).
Arguez, A., Durre, I., Applequist, S., Squires, M., Vose, R., Yin, X., et al. (2010). NOAA's U.S. Climate Normals (1981–2010). NOAA National Centers for Environmental Information.
Bajgain, P., Zhang, X., Jungers, J. M., DeHaan, L. R., Heim, B., Sheaffer, C. C., et al. (2020). ‘MN-Clearwater', the first food-grade intermediate wheatgrass (Kernza perennial grain) cultivar. J. Plant Regist. 14, 288–297. doi: 10.1002/plr2.20042
Canode, C. L. (1968). Influence of row spacing and nitrogen fertilization on grass seed production. Agron. J. 60, 263–267. doi: 10.2134/agronj1968.00021962006000030006x
Casler, M. D. (1999). Repeated measures vs. repeated plantings in perennial forage grass trials: an empirical analysis of precision and accuracy. Euphytica. 105, 33–42. doi: 10.1023/A:1003476313826
Chastain, T. G. (2003). Biological principles of seed production. The art and science of seed production in the Pacific Northwest. p. 12–34.
Crews, T., and Rumsey, B. (2017). What agriculture can learn from native ecosystems in building soil organic matter: a review. Sustainability. 9, 578. doi: 10.3390/su9040578
Crews, T. E., Blesh, J., Culman, S. W., Hayes, R. C., Jensen, E. S., Mack, M. C., et al. (2016). Going where no grains have gone before: from early to mid-succession. Agric. Ecosyst. Environ. 223, 223–238. doi: 10.1016/j.agee.2016.03.012
Crews, T. E., Carton, W., and Olsson, L. (2018). Is the future of agriculture perennial? Imperatives and opportunities to reinvent agriculture by shifting from annual monocultures to perennial polycultures. Glob. Sustain. 1, 1–18. doi: 10.1017/sus.2018.11
Culman, S. W., DuPont, S. T., Glover, J. D., Buckley, D. H., Fick, G. W., Ferris, H., et al. (2010). Long-term impacts of high-input annual cropping and unfertilized perennial grass production on soil properties and belowground food webs in Kansas, USA. Agric. Ecosyst. Environ. 137, 13–24. doi: 10.1016/j.agee.2009.11.008
Culman, S. W., Snapp, S., Ollenburger, M., and Basso, B. (2013). Soil and water quality rapidly responds to the perennial grain Kernza wheatgrass. Agron. J. 105, 735–744. doi: 10.2134/agronj2012.0273
de Faccio Carvalho, C.P., Savian, J. V., Della Chiesa, T., de Souza, W., Terra, J. A., Pinto, P., et al. (2021). Land-use intensification trends in the Rio de la Plata region of South America: Toward specialization or recoupling crop and livestock production. Front. Agric. Sci. Eng. 8, 97–110. doi: 10.15302/J-FASE-2020380
de Oliveira, G., Brunsell, N. A., Crews, T. E., DeHaan, L. R., and Vico, G. (2020). Carbon and water relations in perennial Kernza (Thinopyrum intermedium): an overview. Plant Sci. 295, 107747. doi: 10.1016/j.plantsci.2019.110279
DeHaan, L., Christians, M., Crain, J., and Poland, J. (2018). Development and evolution of an intermediate wheatgrass domestication program. Sustainability. 10, 1499. doi: 10.3390/su10051499
Dick, C., Cattani, D., and Entz, M. H. (2018). Kernza intermediate wheatgrass (Thinopyrum intermedium) grain production as influenced by legume intercropping and residue management. Can. J. Plant Sci. 98, 1376–1379. doi: 10.1139/cjps-2018-0146
Duchene, O., Dumont, B., Cattani, D. J., Fagnant, L., Schlautman, B., DeHaan, L. R., et al. (2021). Process-based analysis of Thinopyrum intermedium phenological development highlights the importance of dual induction for reproductive growth and agronomic performance. Agric. For Meteorol. 301–302, 108341. doi: 10.1016/j.agrformet.2021.108341
Duchene, O., Vian, J. F., and Celette, F. (2017). Intercropping with legume for agroecological cropping systems: complementarity and facilitation processes and the importance of soil microorganisms. a review. Agric. Ecosyst. Environ. 240, 148–161. doi: 10.1016/j.agee.2017.02.019
Economic Research Service. (2022a). USDA-Recent Cost and Return: Soybean (Northern Crescent). Available online at: https://www.ers.usda.gov/data-products/commodity-costs-and-returns/ (accessed September, 2022).
Economic Research Service. (2022b). USDA-Recent Cost and Return: Corn (Northern Crescent). Available online at: https://www.ers.usda.gov/data-products/commodity-costs-and-returns/ (accessed September, 2022).
Ensign, R. D., Hickey, V. G., and Bernardo, M. D. (1983). Effects of sunlight reduction and post-harvest residue accumulations on seed yields of Kentucky bluegrass. Agron. J. 75, 549–551. doi: 10.2134/agronj1983.00021962007500030030x
Extension Wisconsin. (2022). Alfalfa profitability by year. Available online at: https://fyi.extension.wisc.edu/forage/alfalfa/#econ (accessed March, 2022).
Favre, J. R., Munoz, T., Combs, D. K., Wattiaux, M. A., and Picasso, V. D. (2019). Forage nutritive value and predicted fiber digestibility of Kernza intermediate wheatgrass in monoculture and in mixture with red clover during the first production year. Anim. Feed Sci. Technol. 258, 114298. doi: 10.1016/j.anifeedsci.2019.114298
Fernandez, C. W., Ehlke, N., Sheaffer, C. C., and Jungers, J. M. (2020). Effects of nitrogen fertilization and planting density on intermediate wheatgrass yield. Agron. J. 112, 4159–4170. doi: 10.1002/agj2.20351
Franco, J. G., Berti, M. T., Grabber, J. H., Hendrickson, J. R., Nieman, C. C., Pinto, P., et al. (2021). Ecological intensification of food production by integrating forages. Agronomy. 11, 1–26. doi: 10.3390/agronomy11122580
Gaba, S., Lescourret, F., Boudsocq, S., Enjalbert, J., Hinsinger, P., Journet, E-P., et al. (2015). Multiple cropping systems as drivers for providing multiple ecosystem services: from concepts to design. Agron. Sustain. Dev. 35, 607–623. doi: 10.1007/s13593-014-0272-z
Glover, J. D., Reganold, J. P., Bell, L. W., Borevitz, J., Brummer, E. C., Buckler, E. S., et al. (2010). Increased food and ecosystem security via perennial grains. Science. 328, 1638–1639. doi: 10.1126/science.1188761
Halopka, R. (2022). Hay Market Demand and Price Report for the Upper Midwest–for August 29, 2022. University of Wisconsin-Madison, Crop and Soil Division of Extension. Available online at: https://cropsandsoils.extension.wisc.edu/hay-market-demand-and-price-report-for-the-upper-midwest-for-august-29-2022/
Hintz, R. W., Mertens, D. R., and Albrecht, K. A. (1996). Effects of sodium sulfite on recovery and composition of detergent fiber and lignin. J. AOAC Int. 79, 16–22. doi: 10.1093/jaoac/79.1.16
Hunter, M. C., Sheaffer, C. C., Culman, S. W., and Jungers, J. M. (2020a). Effects of defoliation and row spacing on intermediate wheatgrass I: grain production. Agron. J. 112, 1748–1763. doi: 10.1002/agj2.20128
Hunter, M. C., Sheaffer, C. C., Culman, S. W., Lazarus, W. F., and Jungers, J. M. (2020b). Effects of defoliation and row spacing on intermediate wheatgrass II: forage yield and economics. Agron. J. 112, 1862–1880. doi: 10.1002/agj2.20124
Jeranyama, P., and Garcia, A. D. (2004). Understanding relative feed value (RFV) and relative forage quality (RFQ). SDSU Extension Extra Archives, 352. Available online at: https://openprairie.sdstate.edu/extension_extra/352
Jungers, J. M., DeHaan, L. H., Mulla, D. J., Sheaffer, C. C., and Wyse, D. L. (2019). Reduced nitrate leaching in a perennial grain crop compared to maize in the Upper Midwest, USA. Agric. Ecosyst. Environ. 272, 63–73. doi: 10.1016/j.agee.2018.11.007
Jungers, J. M., Schiffner, S., Sheaffer, C. C., Ehlke, N. J., Dehaan, L. R., Torrion, J., et al. (2022). Effect of seeding date on grain and biomass yield of Intermediate wheatgrass. Agron. J. 114, 2342–51. doi: 10.1002/agj2.21083
Jungers, J. M., DeHaan, L. R., Betts, K. J., Sheaffer, C. C., and Wyse, D. L. (2017). Intermediate wheatgrass grain and forage yield responses to nitrogen fertilization. Agron. J. 109, 462–472. doi: 10.2134/agronj2016.07.0438
Kroeger, T., and Casey, F. (2007). An assessment of market-based approaches to providing ecosystem services on agricultural lands. Ecol. Econ. 64, 321–332. doi: 10.1016/j.ecolecon.2007.07.021
Lanker, M., Bell, M., and Picasso, V. D. (2020). Farmer perspectives and experiences introducing the novel perennial grain Kernza intermediate wheatgrass in the US Midwest. Renew. Agric. Food Syst. 35, 653–62. doi: 10.1017/S1742170519000310
Law, E. P., Pelzer, C. J., Wayman, S., Ditommaso, A., and Ryan, M. R. (2020). Strip-tillage renovation of intermediate wheatgrass (Thinopyrum intermedium) for maintaining grain yield in mature stands. Renew. Agric. Food Syst. 1–7. doi: 10.1017/S1742170520000368
Law, E. P., Wayman, S., Pelzer, C. J., Culman, S. W., Gómez, M. I., DiTommaso, A., et al. (2022). Multi-criteria assessment of the economic and environmental sustainability characteristics of intermediate wheatgrass grown as a dual-purpose grain and forage crop. Sustainability. 14, 3548. doi: 10.3390/su14063548
Law, E. P., Wayman, S., Pelzer, C. J., DiTommaso, A., and Ryan, M. R. (2021). Intercropping red clover with intermediate wheatgrass suppresses weeds without reducing grain yield. Agron. J. 114, 700–716. doi: 10.1002/agj2.20914
Lehmann, J., Hansel, C. M., Kaiser, C., Kleber, M., Maher, K., Maher, K., et al. (2020). Persistence of soil organic carbon caused by functional complexity. Nat. Geosci. 13, 529–534. doi: 10.1038/s41561-020-0612-3
Locatelli, A., Gutierrez, L., and Picasso, V. D. (2022). Vernalization requirements of Kernza intermediate wheatgrass. Crop Sci. 62, 524–535. doi: 10.1002/csc2.20667
Lubofsky, E. (2016). The promise of perennials: working through the challenges of perennial grain crop development. CSA News. 61, 4–7. doi: 10.2134/csa2016-61-11-1
Mårtensson, L. M. D., Barreiro, A., Li, S., and Jensen, E. S. (2022). Agronomic performance, nitrogen acquisition and water-use efficiency of the perennial grain crop Thinopyrum intermedium in a monoculture and intercropped with alfalfa in Scandinavia. Agron. Sustain. Dev. 21, 1–10. doi: 10.1007/s13593-022-00752-0
Malézieux, E., Crozat, Y., Dupraz, C., Laurans, M., Makowski, D., Ozier-Lafontaine, H., et al. (2009). Mixing plant species in cropping systems: concepts, tools and models. A review. Agron. Sustain. Dev. 29, 43–62. doi: 10.1051/agro:2007057
NRCS-USDA. (2022a). Natural Resources Conservation Service, United States Department of Agriculture. Official Soil Series Descriptions. Available online at: https://www.nrcs.usda.gov/wps/portal/nrcs/detail/soils/scientists/?cid=nrcs142p2_053587 (accessed May, 2022).
NRCS-USDA. (2022b). Conservation Stewardship Program-Wisconsin-E328O. Available online at: https://www.nrcs.usda.gov/wps/PA_NRCSConsumption/download?cid=NRCSEPRD1855304andext=pdf (accessed September, 2022).
Olugbenle, O., Pinto, P., and Picasso, V. D. (2021). Optimal planting date of Kernza intermediate wheatgrass intercropped with red clover. Agronomy. 11, 2227. doi: 10.3390/agronomy11112227
Picasso, D., Brummer, E. C., Liebman, M., and Dixon, P. M. (2011). Diverse perennial crop mixtures sustain higher productivity over time based on ecological complementarity. Renew. Agric. Food Syst. 26, 317–327. doi: 10.1017/S1742170511000135
Picasso, V. D., Berti, M., Cassida, K., Collier, S., Fang, D., Finan, A., et al. (2022). Diverse perennial circular forage systems are needed to foster resilience, ecosystem services, and socioeconomic benefits in agricultural landscapes. Grasslands Research. 1, 123–130. doi: 10.1002/glr2.12020
Pimentel, D., Cerasale, D., Stanley, R. C., Perlman, R., Newman, E. M., Brent, L. C., et al. (2012). Annual vs. perennial grain production. Agric. Ecosyst. Environ. 161, 1–9. doi: 10.1016/j.agee.2012.05.025
Pinto, P., Culman, S., Crews, T. E., DeHaan, L., Jungers, J., Larsen, J., et al. (2021c). “Dual-use Kernza Intermediate wheatgrass seasonal forage yield and nutritional value across North America.” in American Society of Agronomy (ASA), Crop Science Society of America (CSSA), and Soil Science Society of America (SSSA) International Annual Meetings (Salt Lake City, UT), 7–10.
Pinto, P., DeHaan, L., and Picasso, V. (2021a). Post-harvest management practices impact on light penetration and Kernza intermediate wheatgrass yield components. Agronomy. 11, 442. doi: 10.3390/agronomy11030442
Pinto, P., Fernández-Long, M. E., and Piñeiro, G. (2017). Including Cover Crops during fallow periods for increasing ecosystem services: Is it possible in croplands of Southern South America? Agric. Ecosyst. Environ. 248, 48–57. doi: 10.1016/j.agee.2017.07.028
Pinto, P., Rubio, G., Gutiérrez, F., Sawchik, J., Arana, S., Piñeiro, G., et al. (2021b). Variable root : shoot ratios and plant nitrogen concentrations discourage using just aboveground biomass to select legume service crops. Plant Soil. 463, 347–358. doi: 10.1007/s11104-021-04916-x
Power, A. G. (2010). Ecosystem services and agriculture: tradeoffs and synergies. Philosophical transactions of the Royal Society B. 365, 2959–2971. doi: 10.1098/rstb.2010.0143
Pugliese, J. Y., Culman, S. W., and Sprunger, C. D. (2019). Harvesting forage of the perennial grain crop kernza (Thinopyrum intermedium) increases root biomass and soil nitrogen cycling. Plant Soil. 437, 241–254. doi: 10.1007/s11104-019-03974-6
Reilly, E. C., Gutknecht, J. L., Tautges, N. E., Sheaffer, C. C., and Jungers, J. M. (2022). Nitrogen transfer and yield effects of legumes intercropped with the perennial grain crop intermediate wheatgrass. Field Crops Res. 286, 108627. doi: 10.1016/j.fcr.2022.108627
Robertson, J. B., and Van Soest, P. J. (1981). “The detergent system of analysis and its application to human foods.” in The Analysis of Dietary Fiber in Food. Marcel Dekker. Eds W.P. James, O. Theander (New York), 123–158.
RStudio Team. (2020). RStudio: Integrated Development for R. RStudio, PBC, Boston, MA. Available online at: http://www.rstudio.com/
Ryan, M. R., Crews, T. E., Culman, S. W., Dehaan, L. R., Hayes, R. C., Jungers, J. M., et al. (2018). Managing for multifunctionality in perennial grain crops. BioScience. 68, 294–304. doi: 10.1093/biosci/biy014
Schipanski, M. E., Barbercheck, M., Douglas, M. R., Finney, D. M., Haider, K., Kaye, J. P., et al. (2014). A framework for evaluating ecosystem services provided by cover crops in agroecosystems. Agric. Syst. 125, 12–22. doi: 10.1016/j.agsy.2013.11.004
Sheaffer, C. C., Evers, G. W., and Jungers, J. M. (2020). Cool-season legumes for humid areas. In: Forages, Volume 2: The Science of Grassland Agriculture, 7th Edition, Eds K. J. Moore, M. Collins, C. J. Nelson, D. D. Redfearn (Wiley, Chichester, West Sussex, UK), 968.
Skelly, S., and Peters, T. (2021). Kernza® Perennial Grain: 2021. Planting and Harvest data. Salina, Kansas. Available online at: https://kernza.org/wp-content/uploads/Kernza-Growers-2021-Data.pdf
Sleugh, B., Moore, K. J., George, J. R., and Brummer, E. C. (2000). Binary legume–grass mixtures improve forage yield, quality, and seasonal distribution. Agron. J. 92, 24–29. doi: 10.2134/agronj2000.92124x
Swinton, S. M., Lupi, F., Robertson, G. P., and Hamilton, S. K. (2007). Ecosystem services and agriculture: cultivating agricultural ecosystems for diverse benefits. Ecol. Econ. 64, 245–252. doi: 10.1016/j.ecolecon.2007.09.020
Tautges, N. E., Jungers, J. M., Dehaan, L. R., Wyse, D. L., and Sheaffer, C. C. (2018). Maintaining grain yields of the perennial cereal intermediate wheatgrass in monoculture v. bi-culture with alfalfa in the Upper Midwestern USA. J. Agric. Sci. 156, 758–773. doi: 10.1017/S0021859618000680
Tilman, D., Cassman, K. G., Matson, P. A., Naylor, R., and Polasky, S. (2002). Agricultural sustainability and intensive production practices. Nature. 418, 671–677. doi: 10.1038/nature01014
University of Wisconsin Division of Extension. (2022). Current Season Updates-Wisconsin Corn Agronomy. Available online at: http://corn.agronomy.wisc.edu/Season/Default.aspx (accessed May, 2022).
USDA. (2022). Agricultural Marketing Service. Livestock, poultry, and grain market news. Hay quality designation guidelines. Available online at: https://www.ams.usda.gov/sites/default/files/media/HayQualityGuidelines.pdf (accessed September, 2022).
Welter Seed Honey Co. (2022). Kura clover. Available online at: https://welterseed.com/inventory/kura-clover-coated/ (accessed March, 2022).
Wisconsin Agricultural Statistics. (2022). USDA Statistic by state-Wisconsin. Available online at: https://www.nass.usda.gov/Statistics_by_State/Wisconsin/Publications/Annual_Statistical_Bulletin/2021AgStats-WI.pdf (accessed March, 2022).
Keywords: polycultures, row spacing, seeding season, dual-purpose crop, net economic return, Kernza®, forage quality
Citation: Pinto P, Cartoni-Casamitjana S, Cureton C, Stevens AW, Stoltenberg DE, Zimbric J and Picasso VD (2022) Intercropping legumes and intermediate wheatgrass increases forage yield, nutritive value, and profitability without reducing grain yields. Front. Sustain. Food Syst. 6:977841. doi: 10.3389/fsufs.2022.977841
Received: 24 June 2022; Accepted: 14 November 2022;
Published: 01 December 2022.
Edited by:
E. Britt Moore, University of North Carolina Wilmington, United StatesReviewed by:
Eugene Law, University of Delaware, United StatesK. Ann Bybee-Finley, Cornell University, United States
Copyright © 2022 Pinto, Cartoni-Casamitjana, Cureton, Stevens, Stoltenberg, Zimbric and Picasso. This is an open-access article distributed under the terms of the Creative Commons Attribution License (CC BY). The use, distribution or reproduction in other forums is permitted, provided the original author(s) and the copyright owner(s) are credited and that the original publication in this journal is cited, in accordance with accepted academic practice. No use, distribution or reproduction is permitted which does not comply with these terms.
*Correspondence: Priscila Pinto, ppinto@wisc.edu