- 1Department of Microbiology and Fermentation Technology, CSIR-Central Food Technological Research Institute, Mysore, Karnataka, India
- 2Academy of Scientific and Innovative Research, CSIR-Central Food Technological Research Institute, Mysore, Karnataka, India
- 3Department of Grain Science and Technology, CSIR-Central Food Technological Research Institute, Mysore, Karnataka, India
The fungus Monascus produces several secondary metabolites of different pigment hues. These pigments have shown various biological activities. In this study, Monascus purpureus pigment extracts were tested (in vitro) against Penicillium expansum MTCC 4900, Rhizopus stolinfer MTCC 10595, and Aspergillus niger MTCC 8652 for antifungal activity. The UV–visible spectrum of M. purpureus fermented rice extracts showed λmax at 395, 425, and 500 nm. This indicated the solubility of yellow, orange, and red pigments in polar-based solvent extraction. The M. purpureus pigment extracts inhibited the radial growth and conidial germination of the test fungi. The fungi treated with pigment extract stained with DiBAC (a vital stain) emitted green fluorescence under a fluorescent microscope. These results indicated that the pigment extracts have affected the membrane potential of the treated fungi. Hence, the fungicidal activity of the pigment extracts is due to the disruption of the cell membrane. The HPLC analysis of the pigment revealed the presence of two major peaks. The UV–visible spectrum corresponding to the HPLC peak at 12-min retention time revealed the presence of orange pigment rubropunctatin. Apparently the rubropunctatin present in the extracts exhibited fungicidal activity. Further studies are warranted to assess the applications of M. purpureus pigments in preventing and treating fungus-related diseases.
Introduction
The fungus Monascus is known to produce various secondary metabolites including pigment, gamma-aminobutyric acid, and statins. The various biological activities of these secondary metabolites were recorded in the Compendium of Materia Medica (Panda et al., 2010). Monascus metabolites have found applications in improving blood circulation and food digestion (Sham et al., 2014). In China, Japan, and other Southeast Asian countries, the Monascus pigments are used in various food products as food coloring agents (Chen et al., 2015a,b). Monascus has received worldwide attention because of its diverse products and abundant beneficial metabolites (Shi and Pan, 2011; Sham et al., 2014; Patel, 2016).
It is reported that more than 50 pigment secondary metabolites are produced by Monascus and have been identified (Feng et al., 2012; Fung et al., 2012). Most of these pigments identified are yellow and red (Mondal et al., 2019). More than 25 yellow and 21 red pigments have been identified and characterized (Kim and Ku, 2018). Even though the major pigment produced by this fungus is yellow, orange, and red, only four orange pigments, in addition to rubropunctatin and monascorubrin, have been identified and characterized (Feng et al., 2012). Several biological functions such as cholesterol level regulation, antimicrobial, anticancer, anti-inflammatory, anti-diabetes, and anti-obesity were reported (Patel, 2016; Kim and Ku, 2018). The health benefit activities of M. purpureus pigments are attributed to the individual or synergistic effect of several other metabolites (Mohankumari et al., 2021). The anti-hyperglycemic and anti-hyperlipidemic functions of Monascus fermented rice and pigment extracts were reported and shown a protective effect against free radical-generated oxidative stress in rats (Mohan-Kumari et al., 2011b; Wang et al., 2014). The in vitro antioxidant studies of Monascus metabolites can find the application in preventing oxidative stress-related disorders such as atherosclerosis, cancer, diabetes, inflammation, and parkinsonism (Valko et al., 2006; Dhale et al., 2007a; Mohan-Kumari et al., 2011a).
The mutant M. purpureus CFR410-11 has produced dehydromonacolin-MV2 and monashin metabolites. The production of these metabolites was due to the effect of mutation on the polyketide biosynthetic pathway (Dhale et al., 2007a,b). Moreover, the genetic analysis of the polyketide pigment biosynthetic pathway (Balakrishnan et al., 2014; Yang et al., 2015) has revealed the synthesis of several pigment molecules, but yet to be isolated and identified from the species of Monascus (Li et al., 2017). The increased research interest in the biosynthetic pathway of pigment production and fermented products of Monascus is due to the identification of several new bioactive molecules (Mondal et al., 2019) and biological activities (Mohankumari et al., 2021). However, the detailed antifungal activity of the Monascus pigments is yet to be reported. Hence, in this study, we report the antifungal activity of the pigment produced by M. purpureus CFR410-11.
Materials and methods
Materials
The culture medium potato dextrose agar (PDA) and Roswell Park Memorial Institute (RPMI) medium were purchased from Hi-Media Laboratory Mumbai, India. Rice (BPT 5204 sona masoori) was purchased from the local market for solid-state fermentation. The analytical-grade hexane and ethyl acetate were used for the extraction of pigments from fermented rice. The HPLC-grade acetonitrile was used for HPLC analysis. Tris (hydroxymethyl) amino-methane, bis-(1,3-dibutylbarbituric acid) trimethine oxonol (DiBAC), and 3-(N-morpholino) propanesulfonic acid (MOPS) were obtained from Sigma Chemicals St. Louis, MO, USA.
Organism and culture condition
M. purpureus CFR410-11, a mutant, was developed earlier in the CSIR-CFTRI laboratory and used for pigment production (Dhale et al., 2007b; Dhale and Mohan-Kumari, 2014). The Penicillium expansum, MTCC 4900 Rhizopus stolinfer MTCC 10595, and Aspergillus niger MTCC 8652 were procured from Microbial-Type Culture Collection (MTCC), Chandigarh, India. The fungi were maintained on a PDA slant at 4°C by sub-culturing every 30 days. In total, 1-week-old slants were used for the preparation of spore suspension in 0.85% NaCl and Tween 20 (0.5%). To cultivate M. purpureus, the solid-state medium was prepared by autoclaving 10 g of rice in 20 ml of distilled water (1:2 w/v) for 20 min at 115°C. A volume of 1 ml of M. purpureus spore suspension (≈2 × 105) was inoculated to rice medium and incubated for 10–12 days at 30°C (Hotcold S, J.P. Selecta, Barcelona, Spain). Intermittent shaking of the culture flasks (manually) allowed the uniform growth of M. purpureus. After fermentation, the red rice was dried at 40–45°C for 24 h.
Extraction of pigments
The dried fermented rice was ground using an electric grinder. Approximately 10 g of fermented rice powder was used to extract the pigment in a semi-automatic solvent extractor (148 series, VELP Scientifica, Italy). The pigments were extracted sequentially twice in hexane, ethyl acetate, and ethanol. The pigment extracts were filtered through Whatman No. 1 filter paper and concentrated using a rotary flash evaporator (Buchi, Switzerland). After flash evaporation, the extract was lyophilized (Labconco, Kansas City) for further analysis.
Antifungal assay
The three different methods for radial growth, germination assay, minimum inhibitory concentration (MIC), and minimum fungicidal concentration (MFC) were used to determine the antifungal activity. The extracted M. purpureus pigments were dissolved appropriately in DMSO, PDA, or RPMI 1640 media for the assay conditions.
Radial growth
The M. purpureus pigment extracts (25 mg) mixed in PDA (130 ml) were sterilized. The aliquot of 20 ml of PDA was poured into the Petri plates and the control plates were maintained without any pigment extracts. These plates were inoculated by point inoculation or with 10 μl (≈1 × 104) of fungal spore suspension on the PDA plates. The plates were incubated aerobically at 30°C. The radial growth (colony diameter) of mycelia on plates was measured after 4–5 days of incubation. The assays were carried out in triplicate. Each datum point is the mean for at least four measurements of a growing colony. The inhibitory activity of the M. purpureus pigment extracts was assayed based on the hyphal radial growth rates of fungi (Rossana et al., 2011). The experiments were carried out in triplicates and the data were expressed as the mean ± standard deviation. The percent radial growth inhibition was calculated from the mean values using the following formula:
Conidial germination
The effect of M. purpureus pigment extracts on the germination of conidia was determined (Magnusson and Schnürer, 2001). The test fungi were grown on the PDA slant for 1 week. The spore suspension was prepared in sterile water containing 0.5% Tween 80. The spores suspension (10 μl) was added to the 500 μl of RPMI medium containing 75 μl of pigment extracts in centrifuge tubes. These tubes were kept at 30°C under gentle shaking, and the test samples were drawn at 8 and 24 h. The germ tube growth was observed under a microscope at 40X magnification.
Determination of MIC and MFC
Monascus pigment extracts were serially diluted in RPMI 1640 to determine the MIC (Rossana et al., 2011). The RPMI 1640 (150 μl) medium was dispensed in each well (flat bottom). The stock solution of pigment extract 25 mg/ml was prepared in DMSO. The pigment extracts, 150 μl, were added to a well and serially diluted (concentrations 150, 75, 37.5, 18.75, 9.38, 4.69, 2.34, and 1.17). To this, 10-μl spore suspension (≈1X104) was inoculated in each well. The controls were maintained without samples to demonstrate the growth of fungal spores. While amphotericin-B (3 mg/ml) was used as a positive control. The plates were incubated at 30°C under mild stirring conditions and observed after 48 and 72 h of incubation. The MIC was defined as the lowest concentration of pigment extract, which inhibited visual fungal growth. All assays for antifungal activity were carried out at least in triplicate. The minimum concentration pigment that showed ≥99.9% reduction of the original inoculums was recorded as the MFC.
Microscopy DiBAC viability staining
To determine whether the Monascus pigment extracts exert fungicidal activity against the test fungal mycelia (Ben-Ami et al., 2010), the viability staining with bis-(1,3- dibutylbarbituric acid) trimethine oxonol (DiBAC) on pigment treated and treated mycelia. The test fungal spores were grown to mycelia in the RPMI 1640 medium in micro-centrifuge tubes at 30°C with shaking for 36–48 h. After incubation, the tubes were centrifuged at 10,000 g for 10 min to remove the RPMI medium. The mycelia were resuspended in 500 μl RPMI containing 75 μl of Monascus pigment extracts. The amphotericin-B and medium without samples were the positive and negative controls, respectively. These tubes were incubated at 37°C for 6 h with gentle shaking. After incubation, the mycelia were washed twice in 3-(N-morpholino) propanesulfonic acid at pH 7 (MOPS7). The stain DiBAC prepared in 100% ethanol (1 mg/ml) was added to the tubes at the final concentration of 2 μg/ml in MOPS7. Tubes were incubated at room temperature in the dark for 1 h with gentle shaking (70–100 rpm). Again the mycelia were washed in MOPS7 and stored on ice until fluorescent microscopic observations. The images were captured under a triple-band fluorescent microscope (Olympus BX-51; Olympus, Melville, NY) using the fluorescein isothiocyanate (FITC) filter and bright field.
Chromatography analysis
A thin-layer chromatography (TLC) analysis was conducted using a Silica gel 60 F254 TLC plate (Merck, Germany) with n-hexane/ethyl acetate/petroleum ether (30:17:5) as the developing solvent (Shi et al., 2017). Furthermore, the extracted pigments were subjected to high-performance liquid chromatography (HPLC). The extracted pigments were dissolved in the mixture of acetonitrile/water (70:30 v/v). The samples were filtered through a 0.45-μm nylon filter and subjected to HPLC (waters liquid chromatograph, PDA detector) in a C18 analytical column a 250 × 4.6 mm i.d., 5 μm (Sigma, Discovery Suppleco, USA) carrying a UV–visible detector. Chromatographic separation was achieved by injecting 20 μl of the sample with isocratic elution of acetonitrile:water (70:30 v/v) at a flow rate of 1 mL min−1. The elution was monitored at 495 nm for 45 min (Dhale et al., 2018). The pigment extracts were dissolved in respective solvents at the appropriate concentration to analyze the qualitative nature of the pigment. The UV–visible spectrum was recorded using a UV-1800 Shimadzu spectrophotometer at room temperature from 300 to 700 nm.
Results
The M. purpureus produced red pigment on the rice medium after incubation for 10–12 days. The pigment has produced three major pigments yellow, orange, and red. The pigment was extracted from the fermented rice and the color characters were observed by UV–visible spectrum (Supplementary Figure 1). The pigment extracted in hexane was yellow, and the UV–visible spectrum analysis showing an absorption peak at 395 nm (λ max) revealed the production of yellow pigment. However, the pigment extracted in ethyl acetate and ethanol has shown an absorption peak at 425 and 500 nm, indicating the presence of orange and red pigments. These pigments were partially purified based on the solubility of the yellow, orange, and red pigments in the different solvents.
Radial growth inhibition
The radial growth inhibitory activities of the pigment extracts were tested against several fungi. The pigment extracts incorporated in the PDA medium have inhibited the radial growth of fungi (Figure 1). The growth inhibition was measured on PDA after 4–5 days of incubation at 30°C. The growth of tested fungi against the pigment was variously affected. The pigment extracted in hexane has shown the highest percentage inhibitory activity (48.28 ± 0.48%) against the A. niger MTCC 8652 compared with the pigment extracted in ethyl acetate and ethanol. While P. expansum MTCC 4900 (19.50 ± 2.0%) and R. stolinefer MTCC 10595 (22.02 ± 0.58%) were weakly inhibited by the pigment extracted in hexane. The comparative radial growth inhibition assay of pigment against tested fungi indicated that A. niger MTCC 8652 was more susceptible than P. expansum MTCC 4900 and R. stolinefer MTCC 10595 (Table 1). Whereas, all the pigment extracts have shown a similar growth inhibition of P. expansum MTCC 4900 and R. stolinefer MTCC 10595.
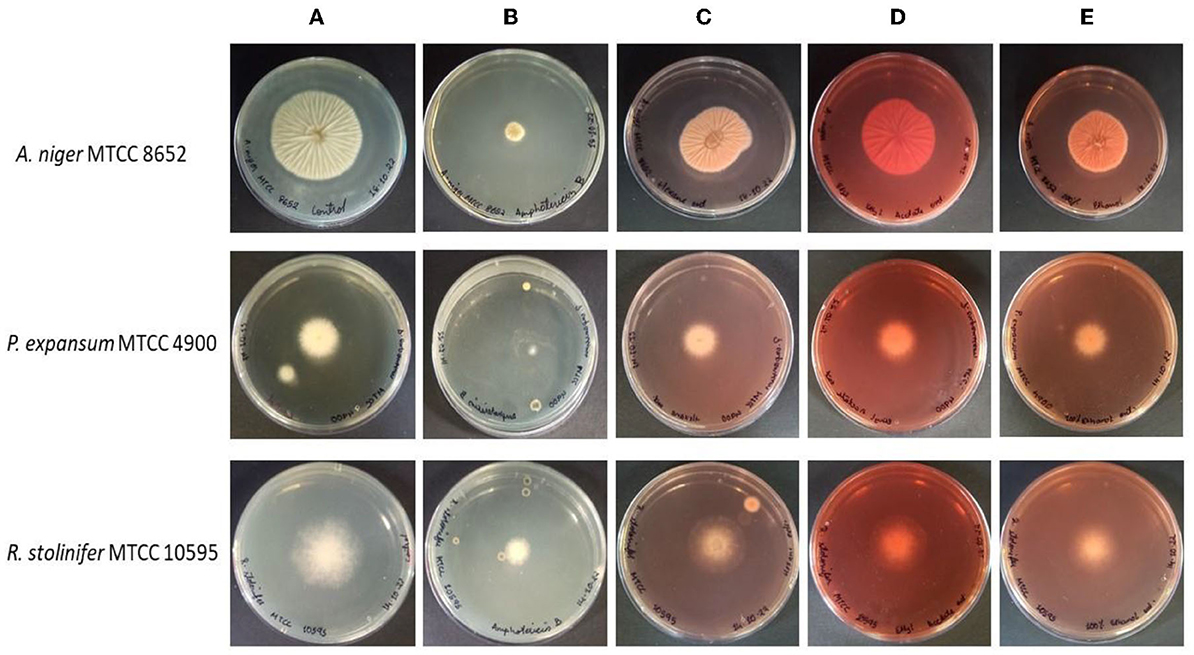
Figure 1. Antifungal activity of M. purpureus pigment extracts [hexane (C), ethyl acetate (D), and ethanol (E)] against the A. niger MTCC 8652, P. expansum MTCC 4900, and R. stolinifer MTCC 10595, as determined by hyphal radial growth inhibition after 4–5 days of incubation at 30°C. The PDA plate without any treatment serves as control (A) and the PDA plate containing amphotericin-B served as a positive control (B).
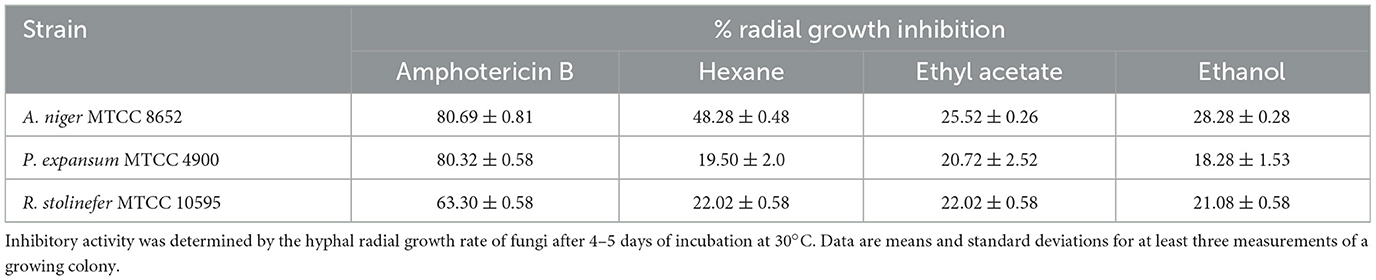
Table 1. Inhibitory activity of M. purpureus pigment extracts on the radial growth of fungi on the PDA medium.
MIC and MFC of pigment extracts
The M. purpureus pigment extracts have shown in vitro antifungal activity against P. expansum MTCC 4900, R. stolinfer MTCC 10595, and A. niger MTCC 8652. Susceptibility testing by broth micro-dilution in RPMI 1640 generally revealed clear endpoints with complete growth inhibition. The MIC of pigment extracted in hexane and ethanol for the tested fungi was 1563.3 μg/ml. The pigment extracted in ethyl acetate showed the MIC at a concentration of 780 μg/ml. The MIC of the standard drug amphotericin-B was 195 μg/ml. While the pigment extracts have shown a fungicidal effect at a concentration of two to three times the MIC. Furthermore, the micro broth dilution assay revealed the MFC at 3126.7 μg/ml (Table 2). In contrast, the amphotericin-B MFCs for all the fungi tested were 390 μg/ml.
Spore germination assay
After incubation for 8 h and 12 h at 30°C in the presence and absence of pigment extracts, the spores of P. expansum MTCC 4900, R. stolinfer MTCC 10595, and A. niger MTCC 8652 were observed under the microscope. The spores grown in the presence of pigment extracts and amphotericin-B did not show the formation of the germ tube, while the formation of germ tubes was observed in the spores grown in the RMPI medium. These results indicated that the M. purpureus pigment extract inhibited the germination of fungal spores. The spores treated with pigment extracts appeared red under the microscope due to the infiltration of the pigment through the destabilized membrane (Figure 2).
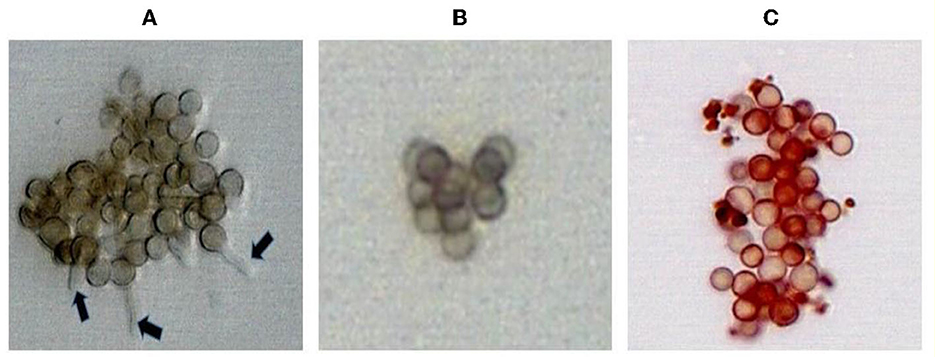
Figure 2. Inhibition of A. niger MTCC 8652 spores germination treated with amphotericin-B (B) and pigment extract of M. purpureus (C). The germination tube formation observed (arrow) in the untreated spores (A) served as a control.
Microscopic DiBAC viability staining
The physiological effects of pigment and amphotericin-B on treated mycelia were studied using a vital stain DiBAC, a fluorescent indicator of cell viability (Liao et al., 1999). The uptake of DiBAC stain was observed in the fungi treated with the pigment and amphotericin-B. The DiBAC stain entered the membrane-compromised cells and exhibited fluorescence (Figure 3). While the untreated mycelia grown in the RPMI medium showed no fluorescence (Figure 3A). These results indicated that amphotericin-B and pigment affected the membrane potential of the fungi. Extensive uptake of DiBAC stain indicated fungicidal activity of the M. purpureus pigment extracts. It was observed that amphotericin-B inhibited the fungi at 195 μg/ml and exerted fungicidal activity at 390 μg/ml, while the MIC was in the range of 780–1563 μg/ml and the MFC of the pigment extracts was 3,126 μg/ml. These results revealed that M. purpureus pigment extracts possess antifungal activity against the mycelia of P. expansum, MTCC 4900, R. stolinfer MTCC 10595, and A. niger MTCC 8652.
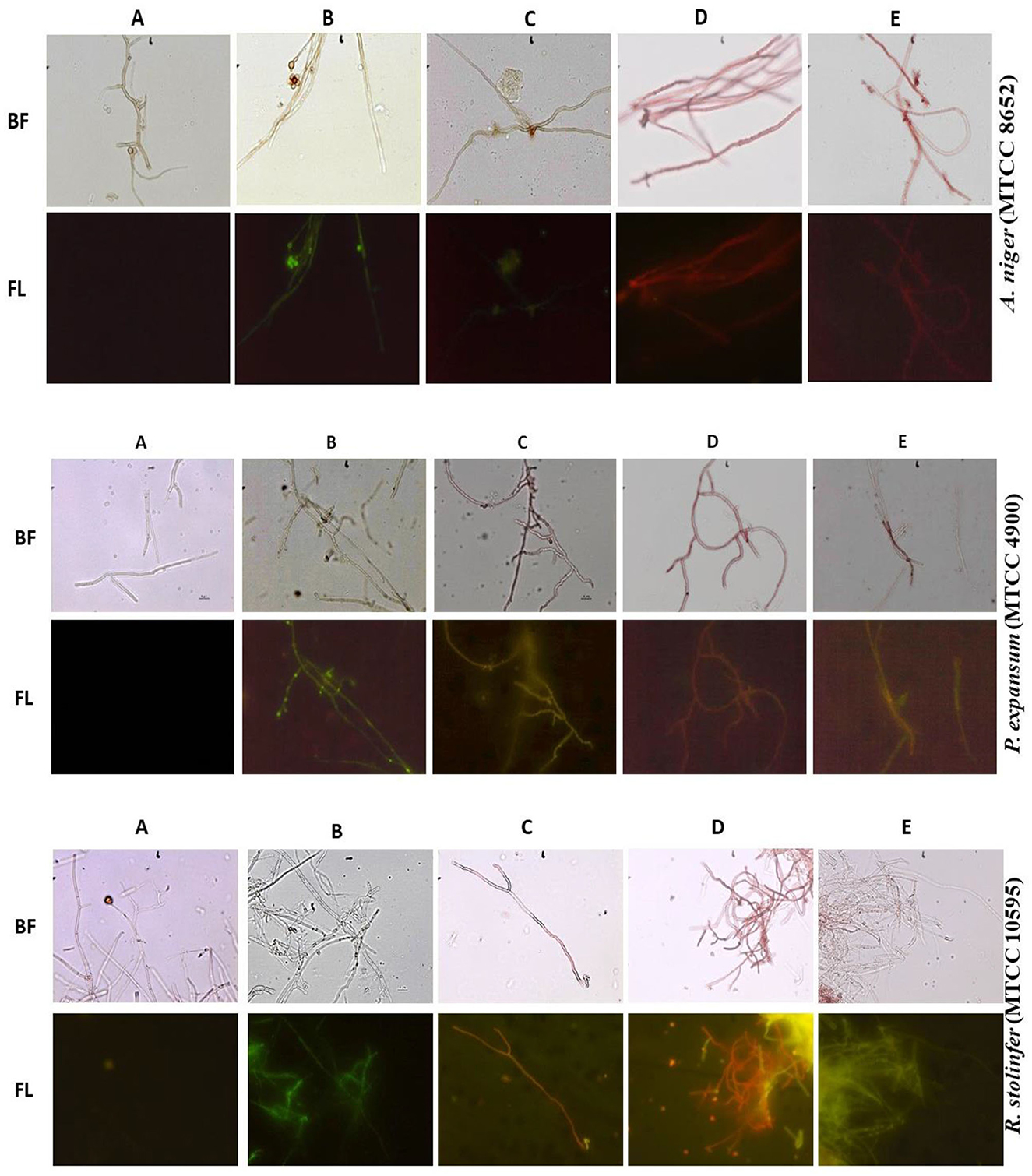
Figure 3. A. niger MTCC 8652, P. expansum MTCC 4900, and R. stolinifer MTCC 10595 mycelia were exposed to M. purpureus pigment extracts extracts [hexane (C), ethyl acetate (D), and ethanol (E)] and amphotericin (B) for 6 h. The untreated mycelia grown in RMPI served as control (A). The treated and untreated mycelia were subsequently stained with DiBAC viability stain and observed with bright field (BF) and fluorescent (FL) microscopy at 400 magnification. The mycelia treated with amphotericin-B and pigment extract have shown enhanced green fluorescence indicating fungicidal activity.
Chromatography analysis
The pigment extracts were subjected to thin-layer chromatography (TLC) to observe the pigment molecules in the extracts. The TLC results revealed the presence of orange pigment prominently in ethyl acetate and ethanol extracts. While the orange pigment spot in the hexane extract was not observed clearly (Supplementary Figure 2). The pigment extracts subjected to the HPLC analysis showed two prominent peaks in hexane and ethyl acetate extracts. While three prominent peaks were observed in the ethanol extract. The pigments extracted in all the solvents have shown a common peak at a 12-min retention time (Figure 4). The UV–visible spectra analysis of this peak (Rt 12 min) has shown peaks at 248, 285, and 472 nm (Figure 4 inset).
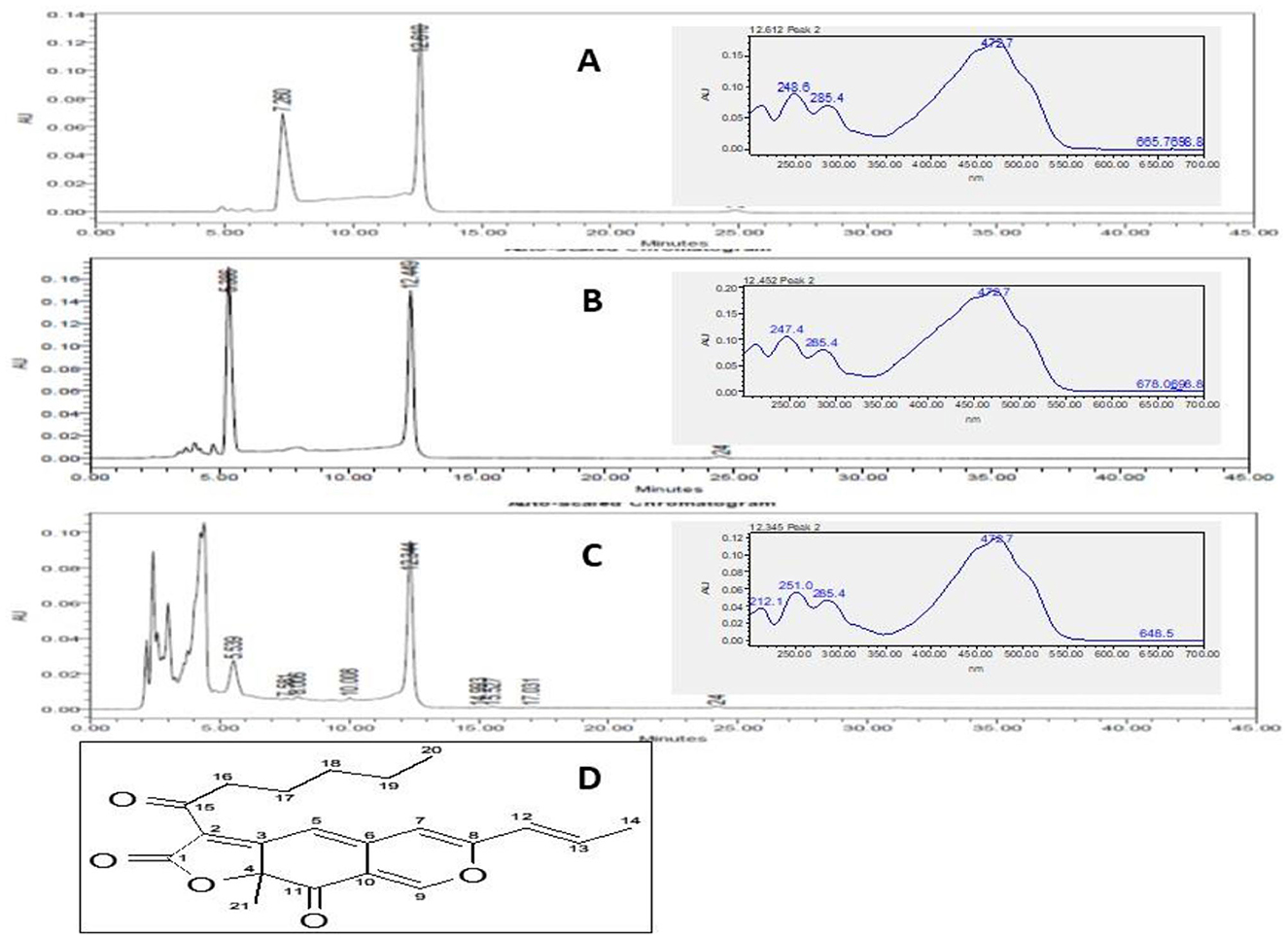
Figure 4. HPLC chromatograph of M. purpureus pigment extracted in hexane (A), ethyl acetate (B), and ethanol (C) showing elution of the pigment fractions. The peak at 12 min was observed in all the extracts. The inset picture shows the UV–visible spectrum of the common major pigment fraction detected at 495 nm. The UV–visible spectrum shows the peak at 248, 285, and 472 nm corresponding to the rubropunctatin (Dhale et al., 2018) pigment molecule (D).
Discussion
M. purpureus produces more than 100 secondary metabolites that include monacolins, polyketide pigments, decalin derivatives, and amino acids. These bioactive molecules play an important role in ameliorating metabolic disorders (Chen et al., 2022). Even though Monascus pigment's antimicrobial activities have been described, most of the studies were correlated to the anti-bacterial activities (Gökmen et al., 2021). Few studies on the antifungal activity of Monascus pigment against Candida albicans and Saccharomyces cerevisiae were reported (Cheng et al., 2011) and multicellular fungi (Kim et al., 2006). The reported antifungal activity of M. purpureus is limited to mostly unicellular fungi/yeast, and in some studies, the antifungal activity of Monascus pigment against Aspergillus niger and Penicillium nigricans was not determined (Gökmen et al., 2021).
The antifungal activity of the M. purpureus pigment on the filamentous fungi was observed in this study. The deviations in the bioactivity of the Monascus pigment are due to the variations among the species and the production of active metabolites. The pigment has inhibited the radial growth of the fungi on PDA plates (Figure 1). Even though the pigment extracts inhibited the radial growth of all the fungi, the percentage of inhibition was varied. This may be due to the susceptibility of the fungi tested against the pigment and the presence of inhibitory molecules in the pigment extracts (Figure 4). The spore germination assay was performed in the presence and absence of pigment extracts to evaluate the mode of radial growth inhibition. It was observed that the pigment extracts inhibited the germination of the fungal spores and eventually inhibited radial growth.
The MIC and MFC values of the pigment extracts are comparatively higher than the amphotericin-B drug. It was also reported that the MIC values of M. purpureus pigments were higher than the MIC values of their derivatives on the filamentous fungi A. niger KCCM 11239, Penicillium citrinum KSSM 11663, Penicillium digitatum KCCM 60140, and C. albicans 10,231 (Kim et al., 2006). Similarly, higher MIC and MFC values of M. purpureus pigment were observed against the fungi P. expansum MTCC 4900, R. stolinfer MTCC 10595, and A. niger MTCC 8652.
The inhibition of spore germination, radial growth inhibition, MIC, and MFC data revealed antifungal activity of pigment extracts against P. expansum MTCC 4900, R. stolinfer MTCC 10595, and A. niger MTCC 8652 spores and mycelia. While the fluorescent microscopic data revealed the fungicidal effect of the pigment. The antifungal effect of M. purpureus pigment extracts was mediated by its disruptive activity on the fungal cytoplasmic membranes (Figure 3). These pigment molecules were able to interact with microbial cell wall-related components selectively. The fungi treated with the pigment extracts appeared red under the bright field microscope (Figures 2, 3). M. purpureus pigments showing antifungal activity are not biosynthesized in animals and should be considered antimicrobial agents (Selvakumar et al., 2006). These results indicated that the pigment permeability through the cell wall of the treated fungi (Figures 2, 3) has caused the fungicidal effect.
The fungicidal activity of M. purpureus pigment extracts was evidenced by staining the pigment-treated mycelia with DiBAC. The DiBAC stain usually enters the membrane-compromised cell and fluoresces green, as observed in amphotericin-B and hexane tread mycelia. While the fluoresces orangish-red mycelia treated with pigment (ethyl acetate and ethanol extract) under fluorescent microscopy is due to the presence of pigment molecules that have entered the membrane-compromised cell (Figure 3). Even though Monascus pigments have shown fluorescent characteristics under UV light (Li et al., 2017), the observation of orangish-red fluorescence characteristic of mycelia treated with pigment is due to the interaction of the pigment and DiBAC stain. The fungicidal activity of caspofungin acetate, which inhibits the synthesis of 1,3-β-D glucan, an essential component of the cell wall of fungi, has been shown (Bowman et al., 2002) by staining with DiBAC. Similarly, the fungicidal activity was evidenced by the DiBAC stain fluorescence in amphotericin-B and pigment-treated mycelia for comparison. The changes in the hyphal morphology of fungi by treating pigment extracts caused the increase in cell permeability, which usually leads to the leakage of small molecular substances and ions, lesions, and discrepancies in cell metabolism (Tang et al., 2018). These results suggested that the M. purpureus pigment extracts have fungicidal activity, and the mechanism of fungi toxic action of M. purpureus pigment extracts against tested fungi is suggested to be through membrane disruption.
The chromatographic and spectrum data (Figure 4) were compared to the earlier reports. The UV–visible spectrum derived for the peak corresponding at 12 Rt showed peaks at 284, 285, and 472 nm. These results confirmed the presence of rubropunctatin in the extracts as compared with the earlier reports (Dhale et al., 2018). Recently, the FDA has approved the three drugs caspofungin, anidulafungin, and micafungin belonging to the class echinocandins for the treatment of invasive fungal infection (Lima et al., 2019). In this study, the orange pigment rubropunctatin exhibited antifungal action against the tested fungi. Moreover, M. purpureus fermented rice and its pigment extract have proven to be safe in animal models (Mohan-Kumari et al., 2009; Mohankumari et al., 2021). Hence, the antifungal activity of M. purpureus pigment extracts suggested the application in the treatment of diseases related to fungal infections.
Conclusion
The radial growth and germination of the fungal spores were inhibited by the treatment of M. purpureus pigment confirming the antifungal activity. The M. purpureus pigment extracts have demonstrated fungicidal activity by disrupting the membrane permeability of the treated fungi. The presence of orange pigment rubropunctatin in the extracts was confirmed by HPLC and UV–visible spectrum data. The antifungal activity is due to the orange pigment rubropunctatin present in the M. purpureus pigment extracts. However, our studies should prompt further preclinical and clinical studies to confirm the antifungal action of rubropunctatin for the treatment and prevention of infections related to fungi.
Data availability statement
The original contributions presented in the study are included in the article/Supplementary material, further inquiries can be directed to the corresponding author.
Author contributions
MH and MD designed the experiment, acquired the funds, analyzed the data, and wrote the manuscript. SM executed the extraction of the pigment and antifungal assays. All the authors read and approved the final manuscript.
Funding
This study was supported by the Indian Council of Medical Research, Govt of India, New Delhi through financial grants for the project (Reference no. 36/3/2020-Toxi /BMS).
Acknowledgments
The authors thank the Director, CSIR-CFTRI, Mysore, India for encouragement and for providing the facilities.
Conflict of interest
The authors declare that the research was conducted in the absence of any commercial or financial relationships that could be construed as a potential conflict of interest.
Publisher's note
All claims expressed in this article are solely those of the authors and do not necessarily represent those of their affiliated organizations, or those of the publisher, the editors and the reviewers. Any product that may be evaluated in this article, or claim that may be made by its manufacturer, is not guaranteed or endorsed by the publisher.
Supplementary material
The Supplementary Material for this article can be found online at: https://www.frontiersin.org/articles/10.3389/fsufs.2023.1100961/full#supplementary-material
References
Balakrishnan, B., Suh, J. W., Park, S. H., and Kwon, H. J. (2014). Delineating Monascus azaphilone pigment biosynthesis: oxidoreductive modifications determine the ring cyclization pattern in azaphilone biosynthesis. RSC Adv. 103, 59405–59408. doi: 10.1039/C4RA11713A
Ben-Ami, R., Lewis, R. E., Tarrand, J., Leventakos, K., and Kontoyiannis, D. P. (2010). Antifungal activity of colistin against mucorales species in vitro and in a murine model of rhizopus oryzae pulmonary infection. Antimicrobl. Agents Chemoth. 54, 484–490. doi: 10.1128/AAC.00956-09
Bowman, J. C., Scott Hicks, P., Kurtz, M. B., Rosen, H., Schmatz, D. M., Liberator, P. A., et al. (2002). The antifungal echinocandin caspofungin acetate kills growing cells of aspergillus fumigatus in vitro. Antimicrobl. Agents Chemoth. 46, 3001–3012. doi: 10.1128/AAC.46.9.3001-3012.2002
Chen, G., Shi, K., and Song, D. (2015a). The pigment characteristics and productivity shifting in high cell density culture of Monascus anka mycelia. BMC Biotechnol. 15, 72. doi: 10.1186/s12896-015-0183-3
Chen, J., Zheng, Q., Zheng, Z., Li, Y., Liao, H., Zhao, H., et al. (2022). Analysis of the differences in the chemical composition of Monascus rice and highland barley monascus Food Funct. 13, 7000–7019. doi: 10.1039/D2FO00402J
Chen, W., He, Y., Zhou, Y., Shao, Y., Feng, Y., Li, M., et al. (2015b). Edible filamentous fungi from the species monascus: early traditional fermentations, modern molecular biology, and future genomics. Comprehens. Rev. Food Sci. Food Saf. 14, 555–567. doi: 10.1111/1541-4337.12145
Cheng, M.-J., Wua, M.-D., Chen, I.-S., Tseng, M., and Yuan, G.-F, Y. (2011). Chemical constituents from the fungus Monascus purpureus and their antifungal activity. Phytochem. Lett. 4, 372–376. doi: 10.1016/j.phytol.2011.08.003
Dhale, M. A., Divakar, S., Umesh-Kumar, S., and Vijayalakshmi, G. (2007a). Isolation and characterization of dihydromonacolin-MV from Monascus purpureus for antioxidant properties. Appl. Microbiol. Biotechnol. 73, 1197–1202. doi: 10.1007/s00253-006-0578-0
Dhale, M. A., Divakar, S., Umesh-Kumar, S., and Vijayalakshmi, G. (2007b). Characterization of dehydromonacolin-MV2 from Monascus purpureus mutant. J. Appl. Microbiol. 103, 2168–2173. doi: 10.1111/j.1365-2672.2007.03457.x
Dhale, M. A., Manjunath, J., and Mohan-Kumari, H. P. (2018). Protective and antioxidative effect of rubropunctatin against oxidative protein damage induced by metal catalyzed reaction. Int. J. Biol. Macromol. 116, 409–416. doi: 10.1016/j.ijbiomac.2018.04.170
Dhale, M. A., and Mohan-Kumari, H. P. (2014). A comparative rapid and sensitive method to screen L-asparaginase producing fungi. J. Microbiol. Methods 102, 66–68. doi: 10.1016/j.mimet.2014.04.010
Feng, Y., Shao, Y., and Chen, F. (2012). Monascus pigments. Appl. Microbiol. Biotechnol. 96, 1421–1440. doi: 10.1007/s00253-012-4504-3
Fung, W. T., Subramaniam, G., and Lee, J. (2012). Assessment of extracts from red yeast rice for herb-drug interaction by in vitro and in vivo assays. Scientific Report 2, 298. doi: 10.1038/srep00298
Gökmen, G. G., S,ilbir, M. S., Göksungur, Y., and Kis,la, D. (2021). Antimicrobial activity of red pigments derived from Monascus purpureus: a comparison to industrial red pigments. JSFA Reports 1, 5–10. doi: 10.1002/jsf2.20
Kim, C., Jung, H., Kim, Y. O., and Shin, C. S. (2006). Antimicrobial activities of amino acid derivatives of Monascus pigments. FEMS Microbiol. Lett. 264, 117–124. doi: 10.1111/j.1574-6968.2006.00451.x
Kim, D., and Ku, S. (2018). Beneficial effects of Monascus sp. KCCM 10093 pigments and derivatives: a mini review. Molecules 23, 98–113. doi: 10.3390/molecules23010098
Li, M.-N., Li, C.-R., Gao, W., Li, P., and Yang, H. (2017). Highly sensitive strategy for identification of trace chemicals in complex matrix: application to analysis of monacolin analogues in monascus-fermented rice product. Anal. Chim. Acta 982, 156–167. doi: 10.1016/j.aca.2017.05.030
Liao, R. S., Rennie, R. P., and Talbot, J. A. (1999). Assessment of the effect of amphotericin B on the vitality of Candida Albicans. Antimicrobl. Agents Chemoth. 43, 1034–1041. doi: 10.1128/AAC.43.5.1034
Lima, S. L., Colombo, A. L., and de Almeida Junior, J. N. (2019). Fungal cell wall: emerging antifungals and drug resistance. Front. Microbiol. 10, 2573. doi: 10.3389/fmicb.2019.02573
Magnusson, J., and Schnürer, J. (2001). Lactobacillus coryniformis subsp. coryniformis strain Si3 produces a broad-spectrum proteinaceous antifungal compound. Appl. Environ. Microbiol. 67, 1–5. doi: 10.1128/AEM.67.1.1-5.2001
Mohan-Kumari, H. P., Dhale, M. A., Gaonkar, V., and Keni, S. (2011a). Statins: 3-hydroxy-3-methylglutaryl-CoA (HMG-CoA) reductase inhibitors demonstrate anti-atherosclerotic character due to their antioxidant capacity. Appl. Biochem. Biotechnol. 163, 215–222. doi: 10.1007/s12010-010-9031-z
Mohan-Kumari, H. P., Naidu, K. A., Dhale, M. A., and Vijayalakshmi, G. (2011b). Antioxidant effect of red mould rice (RMR) in hypercholesterolemic Wistar male rats. Cell Biochem. Funct. 29, 597–602. doi: 10.1002/cbf.1793
Mohankumari, H. P., Naidu, K. A., Narasimhamurthy, K., and Vijayalakshmi, G. (2021). Bioactive pigments of monascus purpureus attributed to antioxidant, HMG-CoA reductase inhibition and anti-atherogenic functions. Front. Sustain. Food Syst. 5, 590427. doi: 10.3389/fsufs.2021.590427
Mohan-Kumari, H. P., Naidu, K. A., Vishwanatha, S., Narasimhamurthy, K., and Vijayalakshmi, G. (2009). Safety evaluation of Monascus purpureus red mould rice in albino rats. Food and Chemical Toxicology 47, 1739–1746. doi: 10.1016/j.fct.2009.04.038
Mondal, S., Pandit, S. G., Mohankumari, H. P., Nanishankar, H. V., and Dhale, M. A. (2019). Structural and functional characterization of new pigment molecule Monashin from Monascus purpureus CFR410-11. Process Biochemistry 82, 173–178. doi: 10.1016/j.procbio.2019.04.004
Panda, B. P., Javed, S., and Ali, M. (2010). Production of angkak through co-culture of Monascus purpureus and Monascus ruber. Braz. J. Microbiol. 41, 757–764. doi: 10.1590/S1517-83822010000300028
Patel, S. (2016). Functional food red yeast rice (RYR) for metabolic syndrome amelioration: a review on pros and cons. W. J. Microbiol. Biotechnol. 32, 87–99. doi: 10.1007/s11274-016-2035-2
Rossana, C., Angela, C., Carlo, G. R., Luana, N., Gianluigi, C., and Marco, G. (2011). Antifungal activity of wickerhamomyces anomalus and lactobacillus plantarum during sourdough fermentation: identification of novel compounds and long-term effect during storage of wheat bread. Appl. Environ. Microbiol. 77, 3484–3492. doi: 10.1128/AEM.02669-10
Selvakumar, D., Miyamoto, M., Furuichi, Y., and Komiyama, T. (2006). Inhibition of fungal β-1,3-glucan synthase and cell growth by HM-1 killer toxin single-chain anti-idiotypic antibodies. Antimicrobl. Agents Chemoth. 50, 3090–3097. doi: 10.1128/AAC.01435-05
Sham, T., Chan, C., Wang, Y., Yang, J., Mok, D. K., and Chan, S. (2014). A review on the traditional Chinese medicinal herbs and formulae with hypolipidemic effect. Biomedical Research International 10, 1153–1166. doi: 10.1155/2014/925302
Shi, K., Tang, R., Huang, T., Wang, L., and Wu, Z. (2017). Pigment fingerprint profile during extractive fermentation with Monascus anka GIM 3.592. BMC Biotechnol. 17, 46. doi: 10.1186/s12896-017-0366-1
Shi, Y.-C., and Pan, T.-M. (2011). Beneficial effects of Monascus purpureus NTU 568-fermented products: a review. Appl. Microbiol. Biotechnol. 90, 1207–1217. doi: 10.1007/s00253-011-3202-x
Tang, X., Ouyang, Q., Jing, G., Shao, X., and Tao, N. (2018). Antifungal mechanism of sodium dehydroacetate against Geotrichum citri-aurantii. W. J. Microbiol. Biotechnol. 34, 29. doi: 10.1007/s11274-018-2413-z
Valko, M., Rhodes, C. J., Moncola, J., Izakovic, M., and Mazura, M. (2006). Free radicals, metals and antioxidants in oxidative stress-induced cancer. Chem. Biol. Interact. 160, 1–40. doi: 10.1016/j.cbi.2005.12.009
Wang, J., Jiang, W., and Zhong, Y. (2014). Xuezhikang attenuated the functional and morphological impairment of pancreatic islets in diabetic mice via the inhibition of oxidative stress. J. Cardiovasc. Pharmacol. 63, 282–289. doi: 10.1097/FJC.0000000000000047
Keywords: Monascus purpureus, pigment, antifungal, spore germination, rubropunctatin
Citation: Majhi S, Dhale MA and Honganoor Puttananjaiah M (2023) Inhibitory effect of Monascus purpureus pigment extracts against fungi and mechanism of action. Front. Sustain. Food Syst. 7:1100961. doi: 10.3389/fsufs.2023.1100961
Received: 17 November 2022; Accepted: 24 January 2023;
Published: 17 February 2023.
Edited by:
Lourdes Morales-Oyervides, Autonomous University of Coahuila, MexicoReviewed by:
Zhilong Wang, Shanghai Jiao Tong University, ChinaJasneet Grewal, University of Warsaw, Poland
Copyright © 2023 Majhi, Dhale and Honganoor Puttananjaiah. This is an open-access article distributed under the terms of the Creative Commons Attribution License (CC BY). The use, distribution or reproduction in other forums is permitted, provided the original author(s) and the copyright owner(s) are credited and that the original publication in this journal is cited, in accordance with accepted academic practice. No use, distribution or reproduction is permitted which does not comply with these terms.
*Correspondence: Mohan Appasaheb Dhale, bW9oYW5hQGNmdHJpLnJlcy5pbg==; Mohankumari Honganoor Puttananjaiah,
bW9oYW5rdW1hcmlAY2Z0cmkucmVzLmlu