- 1Department of Wildlife, Fish, and Conservation Biology, University of California, Davis, Davis, CA, United States
- 2Department of Biostatistics and Computational Biology, University of Rochester Medical Center, Rochester, NY, United States
- 3Cascade Agroecology, Wenactchee, WA, United States
- 4Escuela de Medicina Veterinaria, Facultad de Ciencias de la Vida, Universidad Andres Bello, Santiago, Chile
- 5School of Nutrition and Food Sciences, Louisiana State University, BatonRouge, LA, United States
- 6CEBAS-CSIC, Spanish National Research Council, Espinardo, Murcia, Spain
- 7Ohio State University Extension and Department of Food Science and Technology, The Ohio State University, Columbus, OH, United States
- 8Department of Fisheries, Animal, and Veterinary Sciences, University of Rhode Island, Kingston, RI, United States
- 9Department of Biology, Birmingham-Southern College, Birmingham, AL, United States
- 10Department of Food Science, Cornell University, Ithaca, NY, United States
- 11Department of Fish and Wildlife Conservation, Virginia Tech, Blacksburg, VA, United States
- 12Food Science and Technology, University of Georgia, Athens, GA, United States
- 13Department of Environmental Biology, College of Environmental Science and Forestry, State University of New York, Syracuse, NY, United States
- 14Agricultural Research Service, U.S. Department of Agriculture, Albany, CA, United States
- 15The Acheson Group, Bigfork, MT, United States
- 16Department of Food Science, The Pennsylvania State University, University Park, PA, United States
- 17Department of Food Science and Technology, Virginia Tech, Blacksburg, VA, United States
- 18Department of Population Medicine and Diagnostic Sciences, Cornell University, Ithaca, NY, United States
- 19Oniris, INRAE, BIOEPAR, Nantes, France
- 20Department of Entomology, Washington State University, Pullman, WA, United States
- 21Department of Population Health and Reproduction, School of Veterinary Medicine, University of California, Davis, Davis, CA, United States
- 22Department of Land, Air and Water Resources, University of California, Davis, Davis, CA, United States
- 23Institute of the Environment, University of California, Davis, Davis, CA, United States
- 24Department of Population Health, University of Georgia, Athens, GA, United States
- 25Ecology, Evolution, and Behavior Program, Michigan State University, East Lansing, MI, United States
- 26International Fresh Produce Association, Washington, DC, United States
Consumption of contaminated produce remains a leading cause of foodborne illness. Increasingly, growers are altering agricultural practices and farm environments to manage food-safety hazards, but these changes often result in substantial economic, social, and environmental costs. Here, we present a comprehensive evidence synthesis evaluating the efficacy of soil, non-crop vegetation, animal, landscape, and irrigation water management strategies aimed at reducing produce-safety risk in North America. We systematically summarized findings from 78 peer-reviewed papers on the effect of 21 management practices on the prevalence, abundance, or survival of four foodborne pathogens (i.e., E. coli, Salmonella spp., Listeria spp., and Campylobacter spp.), resulting in 113 summaries. We then organized a 30-member expert panel, who used these summaries to evaluate the impact of each practice on food-safety outcomes. While more than half of the practices were too understudied to confidently evaluate their impact on food safety, the panel did identify several practices that were associated with reduced preharvest food-safety risks, including not using raw manure, separating crop and livestock production, and choosing low-risk irrigation sources. The panel also identified practices that appear ineffective at reducing food-safety risks, such as the removal of non-crop vegetation. Overall, these findings provide insights into the food-safety impacts of agricultural and land management practices that growers, auditors, and extension personnel can use to co-manage produce preharvest environments for food safety and other aims.
1. Introduction
In the United States, produce-associated outbreaks of foodborne pathogens were linked to 100,939 illnesses, 5,699 hospitalizations, and 145 fatalities between 2009 and 2015 (Dewey-Mattia et al., 2018). Indeed, recent analyses attribute ~40% of Salmonella, 65% of E. coli, and 60% of Listeria illnesses in the U.S. to consumption of fruits or vegetables (Interagency Food Safety Analytics Collaboration, 2021). Foodborne pathogens can contaminate preharvest produce through multiple pathways, including the use of contaminated agricultural water, soil amendments, or farm equipment as well as workers and animal intrusion (LGMA., 2013; FDA, 2015). Food-safety standards, regulations, and voluntary grower agreements have been developed to address these contamination pathways, transforming farm management and growing environments (LGMA., 2013; FDA, 2015). For example, wildlife fences, rodent traps, and bare-ground buffers around fields are commonly implemented to mitigate wildlife intrusion in California (Baur et al., 2016). Growers in California also recently reported using raw manures and animal-based composts less frequently than in the past (Baur et al., 2016).
Changes in farming practices associated with improving food safety have come with socioecological consequences. Especially in California, riparian and other on-farm, natural habitats are often removed, threatening wildlife populations (Gennet et al., 2013; Karp et al., 2015a,b; Baur et al., 2016). On-farm, food-safety management can also exact a heavy economic burden on growers. For instance, leafy greens growers in California reported costs for complying with the California Leafy Greens Marketing Agreement (LGMA) ranging between $7,000 (water testing) to $305,430 (total field audits) for implementing eight different food-safety practices (Calvin et al., 2017). Importantly, food-safety costs benefit from economies of scale, with costs per-acre decreasing as produce acreage increases. As such, the economic impact of food-safety compliance is often most acutely felt by smaller growers (Karp et al., 2015a; Baur et al., 2017; Adalja and Lichtenberg, 2018). Given the public health costs associated with ineffectively managing preharvest food-safety hazards, and the economic/ecological costs associated with implementing food-safety practices, it is essential to ensure that the on-farm agricultural and land management practices effectively mitigate risks. Moreover, increasing evidence from preharvest studies suggest that one-size-fits-all regulations are not universally effective for managing preharvest food safety risks due to the heterogeneity of farm operations (Neher et al., 2019; Sharma et al., 2019; Weller et al., 2020). Instead, “best practices” often appear to be context dependent, varying within and between growing regions, commodities, and farms. Thus, “best practices” are continuously evolving as more data become available (FAO and WHO, 2019).
Further, industry buyers often interpret the Food Safety Modernization Act Produce Safety Rule (FSMA PSR) and/or LGMA regulations as a “floor” rather than a “ceiling,” imposing additional (and often propriety) requirements designed to achieve the least risky growing conditions possible (Karp et al., 2015a; Baur et al., 2016). The efficacy of these practices may or may not be scientifically vetted. For example, as noted, growers may feel pressured to remove non-crop vegetation within and around their fields to discourage wildlife, a practice absent in both FSMA PSR and LGMA regulations (Karp et al., 2015b; Baur et al., 2016; Olimpi et al., 2019; Smith et al., 2020b). Yet, existing studies suggest that, if anything, habitat removal may increase food-safety risks (Karp et al., 2015b) by removing the filtering capacity of natural vegetation (Tate et al., 2006; Glaize et al., 2021) or by shifting ecological communities to favor more competent reservoir hosts (Kilonzo et al., 2013; Smith et al., 2020a, 2022; Olimpi et al., 2022). It is thus unsurprising that growers often report feeling a significant disconnect between the food-safety practices that they are pressured to implement and farming realities (Olimpi et al., 2019).
Discrepancies between grower perceptions and industry recommendations may stem from a lack access to the resources needed to determine which practices have been thoroughly evaluated and shown to be effective and which have not. Different practices are studied to different degrees, causing confidence in their efficacy to vary. Yet the literature needed to evaluate efficacy is hidden behind paywalls, often communicated via inaccessible jargon, and distributed across multiple scientific disciplines (e.g., microbiology, soil science, ecology, hydrology, etc.). As a result, the literature relevant to informing on-farm practices for food safety is often siloed, hindering efforts to find a “one-stop-shop” extension personnel and growers can use to identify best practices for farm management.
Looking forward, a “state-of-the-science” report is needed, whereby the diverse food-safety impacts of multiple agricultural and land management practices are cogently synthesized. Though quantitative (e.g., meta-analyses) and qualitative (e.g., systematic reviews) methods are regularly implemented, “evidence synthesis” or “knowledge synthesis” techniques are particularly well-suited when the impacts of multiple management actions on multiple outcomes (e.g., prevalence and persistence of multiple foodborne pathogens) need to be evaluated simultaneously. In an evidence synthesis framework, existing knowledge is gathered from public databases of scientific studies and then systematically summarized and reviewed by an expert panel (Dicks et al., 2017; Donnelly et al., 2018). The “Delphi Method” (one approach for expert review) offers an iterative and anonymous process for evaluating the synthesized literature while minimizing biases in group decision-making (Mukherjee et al., 2015; Shackelford et al., 2019). A key benefit of this rigorous, objective, and transparent approach is that it enables decision-makers to both quickly understand core conclusions and how these conclusions were reached (James et al., 2016). As such, the Delphi Method has been implemented across fields and decision contexts, including medicine, nursing, social policy, and conservation/environmental science (Sutherland et al., 2004; James et al., 2016; Dicks et al., 2017; Shackelford et al., 2019; Varndell et al., 2021).
We performed evidence synthesis using the Delphi Method to evaluate the food-safety impacts of 21 agricultural and land management practices that fall into five broad categories: management of soil, non-crop vegetation, agricultural water, domestic/wild animals, and the surrounding landscape (Figure 1). As part of our synthesis, we evaluated which practices were likely to benefit vs. compromise food-safety outcomes, as well as our confidence in each conclusion. By evaluating how well studied each practice was and whether outcomes were consistently reported across farming contexts, we sought to not only identify which practices are likely to decrease, increase, or have no effect on food-safety risks but also pinpoint existing research gaps.
2. Methods
2.1. Literature collection
Our objective was to synthesize findings from peer-reviewed studies conducted in the U.S. or Canada that examined the impact of agricultural and land management practices on preharvest produce-safety outcomes. First, we iteratively identified search terms to return as much relevant literature as possible, while minimizing the number of irrelevant studies returned. Search terms were designed to collect studies conducted in North America and focused on foodborne pathogens, on-farm practices, and produce types covered by FSMA PSR (Table 1). We conducted five searches, focused on retrieving papers within the following management categories: soil management, non-crop vegetation management, animal management, landscape context, and agricultural water management (Table 1). Three public electronic databases were searched [PubMed, Web of Science, and PubMed Central (PMC)] for all literature available between 1945 and November 25, 2019, returning 2,489 papers. Titles and abstracts were independently screened by two people (ND, DW, MJ, or DK). Papers not meeting the following criteria were discarded:
• The study was performed in the US or Canada and written in English
• The study was published in a peer-reviewed journal (gray literature and books were not included);
• The study reported original data (i.e., reviews and modeling papers were excluded);
• The study focused on E. coli (generic or pathogenic), Salmonella spp., Listeria spp. or Campylobacter spp.;
• The study focused on crops covered by the Food Safety Modernization Act's Produce Safety Rule (FSMA PSR);
• The study quantified the effects of management practices or alternative landscape contexts on food-safety outcomes.
2.2. Standardized summaries
Using previously established methods [www.conservationevidence.com; (Shackelford et al., 2019)], we wrote standardized, one-paragraph summaries detailing the methods and results reported in each study (Supplementary Data Sheet 1). If a study considered multiple practices (N = 15), separate summaries were written for each practice. Each paragraph began with a one-sentence synopsis of the study's core finding and ended with a one-line conclusion. Paragraphs noted study type (e.g., observational vs. experimental, controlled or not, replicated or not), study period, growing region, practice of interest, target pathogen(s), farm type, and sample size. All paragraphs were written by ND and then edited by at least one additional person from the core author team (DW, MJ, and/or DK). After finalizing all summaries, summaries were grouped into 21 management practice categories.
2.3. Categorizing practices as beneficial, harmful, or neutral with regards to food-safety outcomes
We used expert elicitation and a modified Delphi technique consisting of two rounds of anonymous evidence evaluation to determine which practices were likely to be beneficial, harmful, or neutral with regards to on-farm, food-safety outcomes (Shackelford et al., 2019). First, we convened an expert panel, that included doctoral-level researchers, doctoral candidates, and extension personnel across several disciplines (e.g., food safety, food microbiology, microbial and disease ecology, agronomy, soil science, agroecology) who were actively conducting research related to preharvest produce-safety and/or received extensive training or education in the preharvest food-safety arena. Of the 53 experts invited to join the review panel, 30 (57%) accepted and agreed to evaluate each practice using only the evidence presented (N = 113 summaries focused on the 21 practices). The experts were provided with detailed guidance documents regarding project goals and evidence scoring procedures (Supplementary Data Sheet 2). Experts were then asked to score each practice, on a scale from 1 to 10, in four separate prompts:
• How beneficial is the practice? Participants were asked to consider how effective the practice was at reducing the survival, prevalence, and/or abundance of foodborne pathogens (STEC, Salmonella spp., Listeria spp., and Campylobacter spp.) or indicator bacteria (fecal indicator bacteria or generic E. coli).
• How harmful is the practice? Participants were asked to consider whether the practice undermines produce safety by bolstering the survival, prevalence, and/or density of foodborne pathogens or indicator bacteria.
• How well studied is the practice? Participants were asked to evaluate whether there have been sufficient high-quality studies to determine whether the practice is beneficial, harmful, or neutral in terms of food-safety outcomes.
• Are the outcomes of the practice reliable across contexts? Participants were asked whether the practice would produce the same (or similar) food-safety outcomes across geographies, climates, soil types, commodities, etc.
In addition, reviewers were asked whether the practices that they scored as “not beneficial” and/or “harmful” for preharvest produce safety outcomes could be implemented in such a way that the practice might reduce food-safety risks. In all cases, reviewers were provided with additional explanation of each area (e.g., examples) and space to report their rationale for the selected scores.
After receiving the completed scoresheets, we calculated median responses to each of the four questions listed above for each practice (Supplementary Table 1). Median responses were used to categorize each practice into one of six evidence scores: harmful, potentially harmful, having a tradeoff between benefits and harms, neutral, potentially beneficial, beneficial, and unknown efficacy (see Supplementary Table 2 for category definitions). To determine whether scores differed substantially between experts, we used generalized linear mixed models (GLMMs) to model evidence scores (outcome) as a function of expert (fixed effect) while controlling for management practice (random effect). Separately, GLMMS were fit without the fixed effect of expert. The GLMMs were fit using the lme4 package (Bates et al., 2015) and gaussian distributions. We compared the GLMMs with and without expert as a fixed effect using likelihood ratio tests. Expert explained significant variation in all cases (P < 0.001). Thus, to determine whether median evidence scores were robust to expert effects, we adjusted scores by model predictions, removing predicted expert effects, and then re-calculated evidence scores (Supplementary Table 1). After correcting for expert, all practices retained their original scores except one (soil management, implement no-till practices), which moved from the “unknown efficacy” to “neutral.” We thus decided to proceed with the original, unadjusted scores.
A separate round of review was then initiated. For each of the 21 management practices, experts were presented with the evidence score assigned to the practices as well as the minimum, maximum, median, and standard deviation in the expert responses to each of the four prompts. Based on these data, experts were asked to “accept” or “reject” the score assigned to each practice. As previously established by Shackelford et al. (2019), we had predetermined that the score would be considered finalized if >66% of reviewers agreed with the assessment; practices that failed to meet this threshold would be subject to another round of review. All practices met the 66% threshold, and another round of assessment was unnecessary.
3. Results
3.1. Literature overview
After screening titles and abstracts, 163 papers were retained. After comprehensively reviewing each article, another 85 articles were excluded and 78 were retained. Across these 78 papers, data were available on the food-safety impacts of 21 different agricultural or land management practices. These 21 practices fell into five broad categories: management of soil, non-crop vegetation, agricultural water, domestic/wild animals, and the surrounding landscape (Table 2).
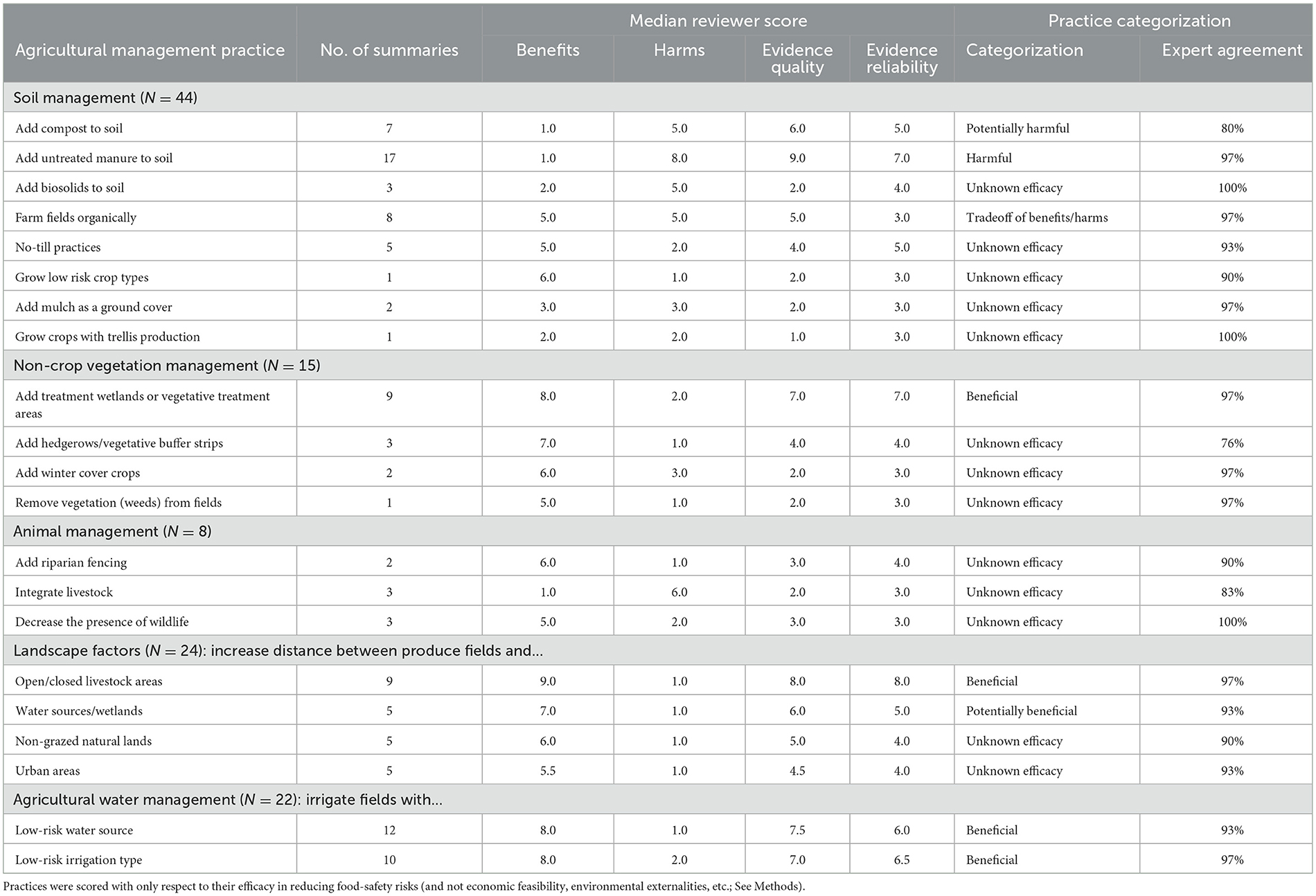
Table 2. Summary of food-safety practices, median expert scores for benefits, harms, quality, and reliability (based on evidence summaries), resulting practice categorization, and expert agreement rates with each categorization.
Sixty-five of the 78 studies considered here were conducted in the United States with 21, 10, 8, 24, and 13 in the West, Midwest, South, Southeast, and Northeast, respectively. Nine studies from the U.S. compared multiple regions. The remaining 13 studies occurred in Canada; specifically, British Columbia (N = 1), the Maritime Provinces (N = 3), Ontario (N = 4), and Quebec (N = 4). Three studies from Canada compared multiple regions. All studies were published between 1998 and 2019. Of the 113 summaries written, an approximately equal number reported on experimental (N = 55) and observational (N = 58) work. While the majority of summaries focused on work conducted in the field (N = 95), a few included greenhouse work (N = 7) or a combination of field, greenhouse, laboratory, and/or growth chamber work (N = 11). While a variety of commodities were studied, the most frequently studied crops were lettuce (N = 25 summaries), spinach (N = 17), and tomatoes (N = 13).
Summaries were unevenly distributed across pathogens and farm management practices. E. coli (generic or pathogenic) was the most studied bacteria (N = 88), followed by Salmonella spp. (N = 46), Listeria spp. (N = 13), and Campylobacter spp. (N = 5). Approximately half of the summaries (N = 60) focused on multiple pathogens, and/or surrogate, index, or indicator organisms whereas the others focused solely on E. coli (N = 47), Salmonella spp. (N = 4), and Listeria spp. (N = 2). In terms of practices, soil management was the most studied (N = 44), followed by landscape (N = 24), agricultural water (N = 22), non-crop vegetation (N = 15), and animal (N = 8) management. Research effort was also unevenly distributed between the 21 practices (Table 2).
3.2. Practice categorization
Expert evaluation of the 21 practices indicated that farming practices varied considerably in their efficacy at reducing produce-safety hazards (Figure 2; Table 2). Five management practices were categorized as beneficial or potentially beneficial for produce safety: (1) adding treatment wetlands/vegetated treatment areas to treat runoff or agricultural water, (2) increasing distances between produce fields and livestock areas, (3) irrigating with low-risk irrigation sources (e.g., ground water instead of surface water), (4) choosing low-risk irrigation types (e.g., drip instead of spray), and (5) increasing distances between fields and water/wetlands. In contrast, amending soils with untreated manure and compost were found to be harmful and potentially harmful, respectively. Organic production exhibited both elevated harm and benefit scores and was thus categorized as having tradeoffs in terms of benefits and harms. The remaining 13 practices were all classified as exhibiting unknown effects on food safety, in part because they were less studied and there was less data on which to base the scores. Specifically, the number of summaries written about each practice ranged from 1 (removing weedy vegetation from produce fields) to 17 (adding untreated manure to soil). Practices that were scored as “unknown efficacy” tended to have fewer summaries written about them (average of 2.8 summaries vs. 9.6 for the other practices).
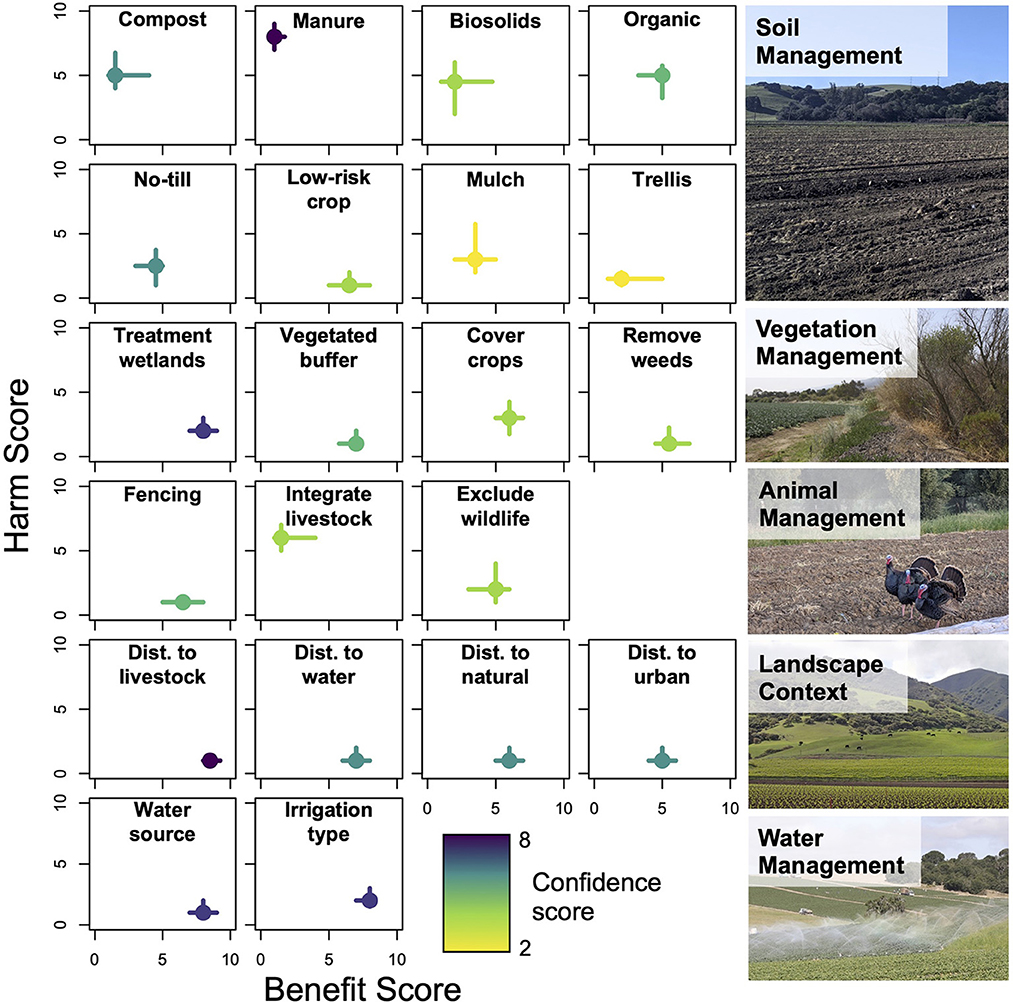
Figure 2. Expert evaluation of food-safety practices. Points represent median food-safety benefit scores (x-axis) and harm scores (y-axis) across the thirty-member expert review panel. Lines represent interquartile ranges. Points and lines are colored by reviewer confidence in each practice, where confidence is defined as the average of median quality and reliability scores. Scores reflect efficacy of practices with respect to food safety only (see Methods).
3.3. Reviewer consistency
Variation in scoring among reviewers was relatively low. For example, interquartile ranges for the benefit and harm scores averaged 2.3 and 1.7 points across the 21 farming practices (on 1–10 point scales). That said, reviewer scores varied more for practices with fewer summaries. For example, the interquartile range of the benefits scores for practices represented by more summaries (and thus more studies) was smaller compared to practices represented by fewer summaries (Pearson's R = −0.68; P < 0.001; Supplementary Figure 1).
The limited variation in experts' scores translated into high levels of agreement in practice scores. On average across all practices, 93% of reviewers agreed with the practice scores (e.g., the classification of practices as beneficial or harmful). No practice fell below the predetermined 66% agreement threshold that would trigger re-evaluation, and only three fell below 90% agreement. Specifically, five experts recommended that the practice of adding hedgerows or vegetative buffer strips around fields (76% agreement) be reclassified as “potentially beneficial” instead of “unknown efficacy.” Four experts argued that the practice of integrating livestock into produce fields (83% agreement) should be reclassified from “unknown efficacy” to “potentially harmful,” and four experts argued amending soil with compost (80% agreement) should be reclassified from “potentially harmful” to “tradeoff of benefits and harms.”
4. Discussion
We sought to use evidence synthesis to evaluate the preharvest produce-safety impacts of 21 agricultural and land management practices across diverse farming contexts. While some practices could be categorized as harmful (e.g., adding untreated manure) or beneficial (e.g., choosing low-risk irrigation types), most were too understudied to confidently evaluate their efficacy. Below, we discuss the five categories of practices evaluated and highlight key research gaps.
4.1. Soil management
Our evidence synthesis supported existing guidance and standards on the use of animal-based soil amendments. Specifically, we found raw manure constituted a food-safety risk, with pathogenic and generic E. coli levels often elevated in raw manure amended soils compared to unamended soils (Park et al., 2014; Sharma et al., 2019) (Supplementary Data Sheet 1). Pathogens could survive for long periods in manure-amended soils; for example, generic E. coli and Salmonella spp. persisted for more than 100-days in soils amended with dairy manure (Ingham et al., 2004; You et al., 2006). Despite these apparent consistencies across studies, there was considerable variability in food-safety outcomes based on when and what manure was applied. For example, multiple studies found that microbial loads were higher, or target microbes survived longer, in soils amended with poultry-derived manures compared to other animal-based soil amendments (Sharma et al., 2016; Gu et al., 2018; Neher et al., 2019) but see (Islam et al., 2004).
Properly treating or composting animal-based soil amendments was associated with improved food-safety outcomes compared to the use of raw manures. This was reflected in the expert panel classifying composts as “potentially harmful” rather than “harmful.” This classification could also be due to the fact that some studies reported elevated food-safety risks associated with compost use (Shah et al., 2019), while other studies failed to find evidence of this (Erickson et al., 2013; Karp et al., 2016). Correspondingly, expert agreement in the “potentially harmful” classification of composts was low relative to other practices (80% agreement), with some reviewers suggesting “tradeoff in benefits and harms” may be a more appropriate designation. Such a designation may be supported by studies not considered in the present synthesis. Specifically, compost is known to bolster soil health by altering soils' physicochemical characteristics (e.g., enhancing micro/macronutrient levels) and by increasing microbial biomass, activity, and diversity (Mehta et al., 2014). Interestingly, several studies (published after November 2019 and thus not included here) suggest that these changes may make soils less hospitable to pathogens. Specifically, higher levels of microbial diversity and biomass in compost-amended soils may limit foodborne pathogen survival in soils, as indigenous bacteria compete with or depredate pathogens (Jones et al., 2019; Baker et al., 2020; Devarajan et al., 2021; Samaddar et al., 2021). Thus, while composts may introduce foodborne pathogens to the farm field environment, they could also help suppress them.
The use of organic instead of conventional practices appeared to be associated with both positive and negative food-safety outcomes, and was thus classified as having “tradeoffs in benefits and harms.” For example, one study found higher prevalence of generic E. coli in conventional compared to organic farms (Pagadala et al., 2015), two studies found higher prevalence in non-certified organic farms compared to certified organic or conventional farms (Mukherjee et al., 2004; Park et al., 2014), and two studies found no effects of organic status (Park et al., 2013; Marine et al., 2015). Such heterogeneity in food-safety outcomes may reflect the fact that organic production systems are not monolithic, and can vary drastically in practices and environmental context (Liebert et al., 2022). Ultimately, studies are needed that compare the preharvest produce-safety impact of specific organic practices to conventional practices.
In comparison to the use of organic practices and amendments of raw manure or composts, the other five soil management practices (tillage, biosolid applications, mulch applications, trellis production systems, and crop choices) were less studied. As a result, all five practices were scored as having “unknown efficacy.” Often the limited data available conflicted in terms of the practice's food-safety impacts. For example, one of the two studies that evaluated mulch applications found that E. coli prevalence was not associated with mulch application, while the second study found that E. coli sometimes survived longer in soils with mulch applied (Micallef et al., 2016; Xu et al., 2016). Though there were more studies that examined the food-safety impact of tillage (N = 5 studies), results were still inconclusive, and most studies reported no effects on foodborne pathogens (Endale et al., 2010; Bezanson et al., 2012; Jenkins et al., 2014; Reed-Jones et al., 2016). As a result, the impacts of tillage, biosolid applications, mulch applications, trellis production systems, and crop choices on food-safety outcomes in preharvest produce environments remains a knowledge gap.
4.2. Non-crop vegetation
Growers report being pressured to remove non-crop vegetation around farm fields to reduce food-safety risks associated with wildlife intrusion (Gennet et al., 2013; Karp et al., 2015a; Baur et al., 2016). We found no evidence to support the efficacy of this practice. Moreover, maintenance of certain types of non-crop vegetation was found to be beneficial. For instance, the nine studies that examined the impact of treatment wetlands on food-safety outcomes reported that treatment wetlands reduced the concentration of pathogens and other microbial targets in irrigation, runoff, and river water (Supplementary Data Sheet 1). One caveat is that elevated concentrations of generic E. coli were observed in treatment reservoirs in one study, potentially resulting from visiting waterfowl (Haverstock et al., 2017). These findings are consistent with the wider scientific literature on ecosystem services provided by wetlands because wetland-mediated removal of various physicochemical and microbial contaminants has been well-documented (e.g., Salimi et al., 2021; Tang et al., 2021).
The synthesis also found that other types of non-crop vegetation may also be beneficial for on-farm food safety. Of the three studies that evaluated hedgerows and other vegetated treatment buffers, one found that Salmonella spp. and Listeria spp. were less likely to be detected in fields with vegetated buffers (Strawn et al., 2013b), and one found that vegetation removal was associated with increased EHEC prevalence (Karp et al., 2015b); the third found no evidence of an association between hedgerows and produce-safety outcomes (Sellers et al., 2018). Thus, although most experts (76%) agreed that the low number of studies meant that hedgerows/vegetated buffers should be categorized as “unknown efficacy,” 23% argued that the practice could be considered potentially beneficial. Regardless, median harm scores associated with treatment wetlands and vegetated buffers were very low (2 and 1, respectively), suggesting that removal of on-farm non-crop vegetation is not an effective strategy for managing preharvest produce-safety risks.
4.3. Animal management
Very few studies evaluated the produce safety impacts of wild and domestic animals, causing all three related practices to be categorized as “unknown efficacy.” Two studies found that fences deterred grazers from entering riparian areas, which may reduce stream contamination (Sunohara et al., 2012; Larson et al., 2016). Similarly, several studies suggested that the presence of domestic or wild animals in fields was positively associated with foodborne pathogen prevalence in said fields (Park et al., 2013; Strawn et al., 2013b). Conversely, a study of an integrated crop/livestock management system found that generic E. coli levels in field soils fell below 1.0 log10 MPN/g 120 days after sheep were allowed to graze down a winter cover crop (Patterson et al., 2018). This may suggest livestock integration is possible with sufficient delays before harvest; however, the limited number of studies that examined this means more research is needed.
4.4. Landscape risk factors
Experts agreed that landscapes surrounding farms can affect on-farm food-safety risks. In particular, increasing the distance between produce fields and livestock operations was considered beneficial, as 8 of the 9 studies evaluated here reported a lower pathogen prevalence on farms further from vs. nearer to livestock operations (Supplementary Data Sheet 1). This was unsurprising since livestock are known sources of foodborne pathogens, and multiple studies have reported high levels of foodborne pathogens within livestock populations (Callaway et al., 2006; Franz and van Bruggen, 2008; Ferens and Hovde, 2011).
Three New York studies found that Listeria contamination was more likely in produce fields closer to surface water or wetlands (Strawn et al., 2013a; Weller et al., 2015, 2016), leading reviewers to consider increasing the distance between produce fields and water sources to be potentially beneficial. One Colorado/Texas study, however, found no differences in generic E. coli prevalence between spinach fields close (≤10 miles) vs. far from (>10 miles) water sources (Park et al., 2013). This discrepancy could result from the “distance to water” variable being correlated with other landscape (e.g., distance to roads, pasture, and houses) or environmental factors (e.g., soil characteristics). If other factors influence foodborne pathogens, and spatially co-vary with “distance to water,” then divergent effects among studies may arise from some studies failing to account for critical covariates.
Finally, results for proximity to urban regions and non-grazed natural lands were more ambiguous and thus classified as “unknown efficacy.” For example, 3 of 5 studies found higher pathogen prevalence on farms near scrubland or forested areas but the other 2 found no such correlations (Supplementary Data Sheet 1). These conflicting findings could again be due to correlation between landscape factors and other environmental characteristics, or to the heterogeneity unique to farm environments. Indeed, multiple studies have shown factors associated with pathogen contamination of farm environments to be complex and to vary between and within regions (Weller et al., 2020). If anything, studies published after November 2019, when studies were pulled from the electronic databases, further complicate attributing a “beneficial” or “harmful” assignation as many of these report that proximity to non-grazed natural lands was associated with lower pathogen loads in farm environments (Smith et al., 2020a, 2022; Olimpi et al., 2022).
4.5. Agricultural water management
Contaminated agricultural water is a known source of and pathway for pathogen introduction into farm environments and has been identified as the presumptive cause of multiple produce-associated outbreaks (Nygård et al., 2008; Gelting et al., 2011; Mody et al., 2011; FDA, 2019; Bottichio et al., 2020). Our evidence synthesis focused on the effects of different water sources and irrigation strategies, with experts concluding that it was beneficial for growers to choose low risk water sources and irrigation strategies. Specifically, eight studies reported that irrigation water sources affected food-safety risks, with surface water, including standing surface water sources, posing greater risks compared to ground, municipal, and well water (Supplementary Data Sheet 1). One study reported higher prevalence of Salmonella spp.in irrigation water from ponds compared to wells (Gu et al., 2018) and another reported higher generic E. coli levels in surface compared to ground water samples (Pagadala et al., 2015). Similarly, use of sprinkler, spray, and foliar irrigation typically carried higher food-safety risks compared to furrow and drip irrigation systems (Supplementary Data Sheet 1). For example, one study reported higher E. coli levels in lettuce fields irrigated by sprinkler systems compared to furrow and drip irrigation systems (Fonseca et al., 2011). Indeed, splash (e.g., from sprinklers or rain) can transfer pathogens from contamination sources (e.g., soil or feces) to fresh produce (Atwill et al., 2015; Weller et al., 2017, 2019; Lee et al., 2019).
4.6. Limitations
Our study is not without limitations. First, our study seeks to evaluate the food-safety outcomes of agricultural and land management practices but does not consider their feasibility and economic, social, or environmental costs. Any guidance based on the findings reported here should thus take into account the fact that no one-size-fits all guidance will work for all produce operations. Second, we reviewed the impacts of agricultural practices on four bacterial pathogens (E. coli, Salmonella spp., Campylobacter spp., and Listeria spp.), each with varied ecologies and potential sources. However, our sample sizes were not sufficient to disentangle how agricultural practices may differentially affect each pathogen.
Another limitation is that literature searches must balance specificity with completeness. It is therefore inevitable that our search terms resulted in omission of some relevant literature, and of some management practices. For example, the use of time-to-harvest intervals following surface water irrigation, anaerobic soil disinfestation, and no-harvest buffers around in-field feces were not considered but are discussed in the scientific literature (Atwill et al., 2015; Weller et al., 2017, 2019; Jeamsripong et al., 2019; Belias et al., 2020). Similarly, papers published after November 2019 were not included. For example, recent studies have reported that vegetated buffers may mitigate food-safety risks (Glaize et al., 2021; Weller et al., 2022). Had these studies been included, experts may have considered hedgerows/vegetated buffers to be potentially beneficial rather than “unknown efficacy.” Moreover, we did not review gray (unpublished) literature, meaning much of the on-the-ground knowledge held by growers, regulators, and extension personnel was not considered. Additionally, we restricted our literature review to studies conducted in North America, meaning the many studies focused on food-safety impacts of pre-harvest practices outside the United States and Canada were not considered. Similarly, we reviewed the impacts of agricultural practices on four bacterial pathogens—E. coli, Salmonella spp., Campylobacter spp., and Listeria spp.— neglecting other established and emerging pathogens (e.g., Cyclospora spp.).
Finally, the uneven distribution of studies across growing regions, commodities, time, operation type, and growing conditions may have influenced categorizations, as practice efficacy can be context dependent. For example, recent work suggests multiple factors (e.g., soil type, manure source, environmental factors, etc.) mediate the prevalence and survival of foodborne pathogens in soils amended with manures (Neher et al., 2019; Sharma et al., 2019) and in agricultural water (Weller et al., 2020). Similarly, the four bacterial pathogens that we studied have distinct sources and ecologies. Though we scored all practices according to their “reliability,” practices were placed in broad categories that fail to reflect the idea that they may only be effective in certain contexts and only suppress certain pathogens. Reviewing more studies would have been necessary to conclusively identify specific environmental contexts in which each practice would be most effective at suppressing each of the pathogens considered here.
4.7. Conclusion
Our study emphasizes the value of evidence synthesis for managing on-farm, food-safety risks. In many cases, experts reaffirmed accepted best practices; for example, refraining from applying raw manures, growing vulnerable produce far from livestock operations, and choosing low-risk irrigation water sources/types when possible. However, our findings also bucked conventional wisdom that non-crop vegetation may introduce food-safety risks. We found no evidence of increased pathogen prevalences or abundances associated with hedgerows or vegetated buffers and significant benefits associated with installing treatment wetlands. Finally, and perhaps most importantly, our work highlights just how much more work needs to be done, with more than half of our focal practices too understudied for experts to confidently assess their efficacy. Testing these understudied practices, as well as identifying contexts in which better-studied practices are likely to be most effective, will be crucial for making more evidence-based decisions about food-safety management in the pre-harvest growing environment.
Data availability statement
The original contributions presented in the study are included in the article/Supplementary material, further inquiries can be directed to the corresponding author.
Author contributions
DK, DW, MJ, and ND designed the study. DK acquired funding. ND drafted the synopses and led the evidence synthesis. DK, DW, and MJ edited synopses. ND and DK wrote the first draft of the manuscript. All authors served on the expert review panel, edited the final manuscript, contributed to the article, and approved the submitted version.
Funding
Funding for this research was made possible by the Center for Produce Safety (Grant# 2019CPS03), by the U.S. Department of Agriculture's (USDA) Agricultural Marketing Service through grant USDA-AMS-TM-SCBG-G-18-003, and by USDA, Agricultural Research Service, National Program 104: Food Safety (animal and plant products). All opinions, findings, conclusions, and recommendations expressed in this publication are those of the authors and do not necessarily reflect the view of the Center for Produce Safety and/or the United States Department of Agriculture (USDA).
Acknowledgments
We thank the many scientists, growers, and food-safety professionals that have contributed their knowledge, time, labor, and resources to the literature evaluated in this evidence synthesis. We also thank G. Shackleford for early conversations on best practices for conducting evidence synthesis.
Conflict of interest
The authors declare that the research was conducted in the absence of any commercial or financial relationships that could be construed as a potential conflict of interest.
Publisher's note
All claims expressed in this article are solely those of the authors and do not necessarily represent those of their affiliated organizations, or those of the publisher, the editors and the reviewers. Any product that may be evaluated in this article, or claim that may be made by its manufacturer, is not guaranteed or endorsed by the publisher.
Supplementary material
The Supplementary Material for this article can be found online at: https://www.frontiersin.org/articles/10.3389/fsufs.2023.1101435/full#supplementary-material
References
Adalja, A., and Lichtenberg, E. (2018). Produce growers' cost of complying with the food safety modernization act. Food Policy 74, 23–38. doi: 10.1016/j.foodpol.2017.10.005
Atwill, E. R., Chase, J. A., Oryang, D., Bond, R. F., Koike, S. T., Cahn, M. D., et al. (2015). Transfer of Escherichia coli O157:H7 from simulated wildlife scat onto romaine lettuce during foliar irrigation. J. Food Prot. 78, 240–247. doi: 10.4315/0362-028X.JFP-14-277
Baker, C. A., Lee, S., De, J., Jeong, K. C., and Schneider, K. R. (2020). Survival of Escherichia coli O157 in autoclaved and natural sandy soil mesocosms. PLoS ONE 15:234562. doi: 10.1371/journal.pone.0234562
Bates, D., Maechler, M., Bolker, B., and Walker, S. (2015). Fitting linear mixed-effects models using lme4. J. Stat. Software 67, 1–48. doi: 10.18637/jss.v067.i01
Baur, P., Driscoll, L., Gennet, S., and Karp, D. S. (2016). Inconsistent food safety and conservation pressures put California produce growers on uncertain footing. Calif. Agric. 70, 142–151. doi: 10.3733/ca.2016a0006
Baur, P., Getz, C., and Sowerwine, J. (2017). Contradictions, consequences and the human toll of food safety culture. Agric. Human Values 34, 713–728. doi: 10.1007/s10460-017-9772-1
Belias, A. M., Sbodio, A., Truchado, P., Weller, D., Pinzon, J., Skots, M., et al. (2020). Effect of weather on the die-off of Escherichia coli and attenuated Salmonella enterica serovar typhimurium on preharvest leafy greens following irrigation with contaminated water. Appl. Environ. Microbiol. 86, e00899–e00820. doi: 10.1128/AEM.00899-20
Bezanson, G., Delaquis, P., Bach, S., McKellar, R., Topp, E., Gill, A., et al. (2012). Comparative examination of Escherichia coli O157:H7 survival on romaine lettuce and in soil at two independent experimental sites. J. Food Prot. 75, 480–487. doi: 10.4315/0362-028X.JFP-11-306
Bottichio, L., Keaton, A., Thomas, D., Fulton, T., Tiffany, A., Frick, A., et al. (2020). Shiga toxin-producing escherichia coli infections associated with romaine lettuce-United States, 2018. Clin. Infect. Dis. 71, e323–e330. doi: 10.1093/cid/ciz1182
Callaway, T. R., Edrington, T. S., Brabban, A. D., Keen, J. E., Anderson, R. C., Rossman, M. L., et al. (2006). Fecal Prevalence of Escherichia coli O157, Salmonella, Listeria, and Bacteriophage infecting E. coli O157:H7 in feedlot cattle in the Southern Plains Region of the United States. Foodborne Pathog. Dis. 3, 234–244. doi: 10.1089/fpd.2006.3.234
Calvin, L., Jensen, H., Klonsky, K., and Cook, R. (2017). Food Safety Practices and Costs Under the California Leafy Greens Marketing Agreement. Available online at: https://www.ers.usda.gov/publications/pub-details/?pubid=83770 (accessed March 6, 2023).
Devarajan, N., McGarvey, J. A., Scow, K., Jones, M. S., Lee, S., Samaddar, S., et al. (2021). Cascading effects of composts and cover crops on soil chemistry, bacterial communities and the survival of foodborne pathogens. J. Appl. Microbiol., 131, 1564–1577. doi: 10.1111/jam.15054
Dewey-Mattia, D., Manikonda, K., Hall, A. J., Wise, M. E., and Crowe, S. J. (2018). Surveillance for foodborne disease outbreaks- United States, 2009-2015. MMWR. Surveill. Summ. 67, 1–11. doi: 10.15585/mmwr.ss6710a1
Dicks, L. V., Haddaway, N., Hernández-Morcillo, M., Mattsson, B., Randall, N., Failler, P., et al. (2017). Knowledge Synthesis for Environmental decisions: An Evaluation of Existing Methods, and Guidance for Their Selection, Use and Development- A Report From the EKLIPSE Project. Available online at: https://www.eklipse-mechanism.eu/apps/Eklipse_data/website/EKLIPSE_D3-1-Report_FINAL_WithCovers_V6.pdf (accessed March 6, 2023).
Donnelly, C., Boyd, I., Campbell, P., Craig, C., Vallance, P., Walport, M., et al. (2018). Four principles for synthesizing evidence. Nature 558, 361–364. doi: 10.1038/d41586-018-05414-4
Endale, D. M., Schomberg, H. H., Jenkins, M. B., Franklin, D. H., and Fisher, D. S. (2010). Management implications of conservation tillage and poultry litter use for Southern Piedmont USA cropping systems. Nutr. Cycl. Agroecosystems 88, 299–313. doi: 10.1007/s10705-009-9318-z
Erickson, M. C., Liao, J., Payton, A. S., Webb, C. C., Ma, L., Zhang, G., et al. (2013). Fate of Escherichia coli O157:H7 and Salmonella in soil and lettuce roots as affected by potential home gardening practices. J. Sci. Food Agric. 93, 3841–3849. doi: 10.1002/jsfa.6321
FAO WHO (2019). Safety and Quality of Water Used in Food Production and Processing: Meeting Report. Available online at: https://www.who.int/publications/i/item/9789241516402 (accessed March 6, 2023).
FDA (2015). Standards for the Growing, Harvesting, Packing, and Holding of Produce for Human Consumption. Available online at: https://www.federalregister.gov/documents/2015/11/27/2015-28159/standards-for-the-growing-harvesting-packing-and-holding-of-produce-for-human-consumption (accessed March 6, 2023).
FDA (2019). Factors Potentially Contributing to the Contamination of Romaine Lettuce Implicated in the Three Outbreaks of E. coli O157:H7 During the Fall of 2019. Available online at: https://www.fda.gov/food/outbreaks-foodborne-illness/factors-potentially-contributing-contamination-romaine-lettuce-implicated-three-outbreaks-e-coli (accessed March 6, 2023).
Ferens, W. A., and Hovde, C. J. (2011). Escherichia cole O157:H7: animal reservoir and sources of human infection. Foodborne Pathog. Dis. 8, 465–487. doi: 10.1089/fpd.2010.0673
Fonseca, J. M., Fallon, S. D., Sanchez, C. A., and Nolte, K. D. (2011). Escherichia coli survival in lettuce fields following its introduction through different irrigation systems. J. Appl. Microbiol. 110, 893–902. doi: 10.1111/j.1365-2672.2011.04942.x
Franz, E., and van Bruggen, A. H. C. (2008). Ecology of E. coli O157:H7 and Salmonella enterica in the primary vegetable production chain. Crit. Rev. Microbiol. 34, 143–161. doi: 10.1080/10408410802357432
Gelting, R. J., Baloch, M. A., Zarate-Bermudez, M. A., and Selman, C. (2011). Irrigation water issues potentially related to the 2006 multistate E. coli O157:H7 outbreak associated with spinach. Agric. Water Manag. 98, 1395–1402. doi: 10.1016/j.agwat.2011.04.004
Gennet, S., Howard, J., Langholz, J., Andrews, K. K., Reynolds, M. D., and Morrison, S. A. (2013). Farming practices for food safety: an emerging threat to floodplain and riparian ecosystems. Front. Ecol. Environ. 11, 236–242. doi: 10.1890/120243
Glaize, A., Young, M., Harden, L., Gutierrez-Rodriguez, E., and Thakur, S. (2021). The effect of vegetation barriers at reducing the transmission of Salmonella and Escherichia coli from animal operations to fresh produce. Int. J. Food Microbiol. 347:109196. doi: 10.1016/j.ijfoodmicro.2021.109196
Gu, G., Strawn, L. K., Oryang, D. O., Zheng, J., Reed, E. A., Ottesen, A. R., et al. (2018). Agricultural practices influence Salmonella contamination and survival in pre-harvest tomato production. Front. Microbiol. 9, 1–16. doi: 10.3389/fmicb.2018.02451
Haverstock, M. J., Madani, A., Bald,é, H., VanderZaag, A. C., and Gordon, R. J. (2017). Performance of an agricultural wetland-reservoir-irrigation management system. Water 9, 472. doi: 10.3390/w9070472
Ingham, S. C., Losinski, J. A., Andrews, M. P., Breuer, J. E., Breuer, J. R., Wood, T. M., et al. (2004). Escherichia coli contamination of vegetables grown in soils fertilized with noncomposted bovine manure: garden-scale studies. Appl. Environ. Microbiol. 70, 6420–6427. doi: 10.1128/AEM.70.11.6420-6427.2004
Interagency Food Safety Analytics Collaboration (2021). Foodborne Illness Source Attribution Estimates for 2019 for Salmonella, Escherichia coli O157, Listeria monocytogenes, and Campylobacter Using Multi-Year Outbreak Surveillance Data, United States. Available online at: https://www.cdc.gov/foodsafety/ifsac/pdf/P19-2019-report-TriAgency-508.pdf (accessed March 6, 23).
Islam, M., Doyle, M. P., Phatak, S. C., Millner, P., and Jiang, X. (2004). Persistence of enterohemorrhagic Escherichia coli O157:H7 in soil and on leaf lettuce and parsley grown in fields treated with contaminated manure composts or irrigation water. J. Food Prot. 67, 1365–1370. doi: 10.4315/0362-028X-67.7.1365
James, K. L., Randall, N. P., and Haddaway, N. R. (2016). A methodology for systematic mapping in environmental sciences. Environ. Evid. 5, 7. doi: 10.1186/s13750-016-0059-6
Jeamsripong, S., Chase, J. A., Jay-Russell, M. T., Buchanan, R. L., and Atwill, E. R. (2019). Experimental in-field transfer and survival of Escherichia coli from animal feces to romaine lettuce in Salinas valley, California. Microorganisms 7, 408. doi: 10.3390/microorganisms7100408
Jenkins, M. B., Truman, C. C., Franklin, D. H., Potter, T. L., Bosch, D. D., Strickland, T. C., et al. (2014). Fecal bacterial losses in runoff from conventional and no-till pearl millet fertilized with broiler litter. Agric. Water Manag. 134, 38–41. doi: 10.1016/j.agwat.2013.11.013
Jones, M. S., Fu, Z., Reganold, J. P., Karp, D. S., Besser, T. E., Tylianakis, J. M., et al. (2019). Organic farming promotes biotic resistance to foodborne human pathogens. J. Appl. Ecol. 56, 1117–1127. doi: 10.1111/1365-2664.13365
Karp, D. S., Baur, P., Atwill, E. R., Master, K. D. E., Gennet, S., Iles, A., et al. (2015a). The unintended ecological and social impacts of food safety regulations in California's Central Coast region. Bioscience 65, 1173–1183. doi: 10.1093/biosci/biv152
Karp, D. S., Gennet, S., Kilonzo, C., Partyka, M., Chaumont, N., Atwill, E. R., et al. (2015b). Comanaging fresh produce for nature conservation and food safety. Proc. Natl. Acad. Sci. 112, 11126–11131. doi: 10.1073/pnas.1508435112
Karp, D. S., Moses, R., Gennet, S., Jones, M., Joseph, S., M'Gonigle, L. K., et al. (2016). Farming practices for food safety threaten pest-control services to fresh produce. J. Appl. Ecol. 53, 1402–1412. doi: 10.1111/1365-2664.12707
Kilonzo, C., Li, X., Vivas, E. J., Jay-Russell, M. T., Fernandez, K. L., and Atwill, E. R. (2013). Fecal shedding of zoonotic food-borne pathogens by wild rodents in a major agricultural region of the Central California Coast. Appl. Environ. Microbiol. 79, 6337–6344. doi: 10.1128/AEM.01503-13
Larson, D. M., Dodds, W. K., Whiles, M. R., Fulgoni, J. N., and Thompson, T. R. (2016). A before-and-after assessment of patch-burn grazing and riparian fencing along headwater streams. J. Appl. Ecol. 53, 1543–1553. doi: 10.1111/1365-2664.12692
Lee, D., Tertuliano, M., Harris, C., Vellidis, G., Levy, K., and Coolong, T. (2019). Salmonella survival in soil and transfer onto produce via splash events. J. Food Prot. 82, 2023–2037. doi: 10.4315/0362-028X.JFP-19-066
LGMA. (2013). Commondity Specific Food Safety Guidelines for the Production and Harvest of Lettuce and Leafy Greens. Sacramento, CA. Available online at: https://lgma-assets.sfo2.digitaloceanspaces.com/downloads/August-2021-CA-LGMA-Metrics_FINAL-v20211208_A11Y.pdf (accessed March 6, 2023).
Liebert, J., Benner, R., Bezner Kerr, R., Björkman, T., De Master, K. T., Gennet, S., et al. (2022). Farm size affects the use of agroecological practices on organic farms in the United States. Nat. Plants 8, 897–905. doi: 10.1038/s41477-022-01191-1
Marine, S. C., Pagadala, S., Wang, F., Pahl, D. M., Melendez, M. V., Kline, W. L., et al. (2015). The growing season, but not the farming system, is a food safety risk determinant for leafy greens in the Mid-Atlantic region of the United States. Appl. Environ. Microbiol. 81, 2395–2407. doi: 10.1128/AEM.00051-15
Mehta, C. M., Palni, U., Franke-Whittle, I. H., and Sharma, A. K. (2014). Compost: its role, mechanism and impact on reducing soil-borne plant diseases. Waste Manag. 34, 607–622. doi: 10.1016/j.wasman.2013.11.012
Micallef, S. A., Callahan, M. T., and Pagadala, S. (2016). Occurrence and dispersal of indicator bacteria on cucumbers grown horizontally or vertically on various mulch types. J. Food Prot. 79, 1663–1672. doi: 10.4315/0362-028X.JFP-16-106
Mody, R. K., Greene, S. A., Gaul, L., Sever, A., Pichette, S., Zambrana, I., et al. (2011). National outbreak of Salmonella serotype saintpaul infections: importance of Texas restaurant investigations in implicating jalapeño peppers. PLoS ONE 6:e16579. doi: 10.1371/journal.pone.0016579
Mukherjee, A., Speh, D., Dyck, E., and Diez-Gonzalez, F. (2004). Preharvest evaluation of coliforms, Escherichia coli, Salmonella, and Escherichia coli O157:H7 in organic and conventional produce grown by Minnesota farmers. J. Food Prot. 67, 894–900. doi: 10.4315/0362-028X-67.5.894
Mukherjee, N., Hug,é, J., Sutherland, W. J., Mcneill, J., Van Opstal, M., Dahdouh-Guebas, F., et al. (2015). The Delphi technique in ecology and biological conservation: applications and guidelines. Methods Ecol. Evol. 6, 1097–1109. doi: 10.1111/2041-210X.12387
Neher, D. A., Cutler, A. J., Weicht, T. R., Sharma, M., and Millner, P. D. (2019). Composts of poultry litter or dairy manure differentially affect survival of enteric bacteria in fields with spinach. J. Appl. Microbiol. 126, 1910–1922. doi: 10.1111/jam.14268
Nygård, K., Lassen, J., Vold, L., Andersson, Y., Fisher, I., Löfdahl, S., et al. (2008). Outbreak of Salmonella Thompson infections linked to imported rucola lettuce. Foodborne Pathog. Dis. 5, 165–173. doi: 10.1089/fpd.2007.0053
Olimpi, E. M., Baur, P., Echeverri, A., Gonthier, D., Karp, D. S., Kremen, C., et al. (2019). Evolving food safety pressures in California's central coast region. Front. Sustain. Food Syst. 3, 1–24. doi: 10.3389/fsufs.2019.00102
Olimpi, E. M., Garcia, K., Gonthier, D. J., Kremen, C., Snyder, W. E., Wilson-Rankin, E. E., et al. (2022). Semi-natural habitat surrounding farms promotes multifunctionality in avian ecosystem services. J. Appl. Ecol. 59, 898–908. doi: 10.1111/1365-2664.14124
Pagadala, S., Marine, S. C., Micallef, S. A., Wang, F., Pahl, D. M., Melendez, M. V., et al. (2015). Assessment of region, farming system, irrigation source and sampling time as food safety risk factors for tomatoes. Int. J. Food Microbiol. 196, 98–108. doi: 10.1016/j.ijfoodmicro.2014.12.005
Park, S., Navratil, S., Gregory, A., Bauer, A., Srinath, I., Jun, M., et al. (2013). Generic Escherichia coli contamination of spinach at the preharvest stage: effects of farm management and environmental factors. Appl. Environ. Microbiol. 79, 4347–4358. doi: 10.1128/AEM.00474-13
Park, S., Navratil, S., Gregory, A., Bauer, A., Srinath, I., Szonyi, B., et al. (2014). Farm management, environment, and weather factors jointly affect the probability of spinach contamination by generic Escherichia coli at the preharvest stage. Appl. Environ. Microbiol. 80, 2504–2515. doi: 10.1128/AEM.03643-13
Patterson, L., Navarro-Gonzalez, N., Jay-Russell, M. T., Aminabadi, P., Antaki-Zukoski, E., and Pires, A. F. A. (2018). Persistence of Escherichia coli in the soil of an organic mixed crop-livestock farm that integrates sheep grazing within vegetable fields. Zoonoses Public Health 65, 887–896. doi: 10.1111/zph.12503
Reed-Jones, N. L., Marine, S. C., Everts, K. L., and Micallef, S. A. (2016). Effects of cover crop species and season on population dynamics of Escherichia coli and Listeria innocua in soil. Appl. Environ. Microbiol. 82, 1767–1777. doi: 10.1128/AEM.03712-15
Salimi, S., Almuktar, S. A. A. A. N., and Scholz, M. (2021). Impact of climate change on wetland ecosystems: a critical review of experimental wetlands. J. Environ. Manage. 286:112160. doi: 10.1016/j.jenvman.2021.112160
Samaddar, S., Karp, D. S., Schmidt, R., Devarajan, N., McGarvey, J. A., Pires, A. F. A., et al. (2021). Role of soil in the regulation of human and plant pathogens: soils' contributions to people. Philos. Trans. R. Soc. B Biol. Sci. 376:20200179. doi: 10.1098/rstb.2020.0179
Sellers, L. A., Long, R. F., Jay-Russell, M. T., Li, X., Atwill, E. R., Engeman, R. M., et al. (2018). Impact of field-edge habitat on mammalian wildlife abundance, distribution, and vectored foodborne pathogens in adjacent crops. Crop Prot. 108, 1–11. doi: 10.1016/j.cropro.2018.02.005
Shackelford, G. E., Kelsey, R., Sutherland, W. J., Kennedy, C. M., Wood, S. A., Gennet, S., et al. (2019). Evidence synthesis as the basis for decision analysis: a method of selecting the best agricultural practices for Multiple ecosystem services. Front. Sustain. Food Syst. 3, 1–13. doi: 10.3389/fsufs.2019.00083
Shah, M. K., Bradshaw, R., Nyarko, E., Handy, E. T., East, C., Millner, P. D., et al. (2019). Salmonella enterica in soils amended with heat-treated poultry pellets survived longer than bacteria in unamended soils and more readily transferred to and persisted on spinach. Appl. Environ. Microbiol. 85, e00334–e00319. doi: 10.1128/AEM.00334-19
Sharma, M., Millner, P. D., Hashem, F., Camp, M., Whyte, C., Graham, L., et al. (2016). Survival and persistence of nonpathogenic Escherichia coli and attenuated Escherichia coli O157:H7 in soils amended with animal manure in a greenhouse environment. J. Food Prot. 79, 913–921. doi: 10.4315/0362-028X.JFP-15-421
Sharma, M., Millner, P. D., Hashem, F., Vinyard, B. T., East, C. L., Handy, E. T., et al. (2019). Survival of Escherichia coli in manure-amended soils is affected by spatiotemporal, agricultural, and weather factors in the mid-atlantic United States. Appl. Environ. Microbiol. 85, e02392–e02318. doi: 10.1128/AEM.02392-18
Smith, O. M., Edworthy, A. E., Taylor, J. M., Jones, M. S., Tormanen, A., Kennedy, C. M., et al. (2020a). Agricultural intensification heightens food safety risk associated with wild birds. J. Appl. Ecol. 57, 2246–2257. doi: 10.1111/1365-2664.13723
Smith, O. M., Olimpi, E. M., Navarro-Gonzalez, N., Cornell, K. A., Frishkoff, L. O., Northfield, T. D., et al. (2022). A trait-based framework for predicting foodborne pathogen risk from wild birds. Ecol. Appl. 32, e2523. doi: 10.1002/eap.2523
Smith, O. M., Snyder, W. E., and Owen, J. P. (2020b). Are we overestimating risk of enteric pathogen spillover from wild birds to humans? Biol. Rev. 95, 652–679. doi: 10.1111/brv.12581
Strawn, L. K., Fortes, E. D., Bihn, E. A., Nightingale, K. K., Gröhn, Y. T., and Worobo, R. W. (2013a). Landscape and meteorological factors affecting prevalence of three food-borne pathogens in fruit and vegetable farms. Appl. Environ. Microbiol. 79, 588–600. doi: 10.1128/AEM.02491-12
Strawn, L. K., Grohn, Y. T., Warchocki, S., Worobo, R. W., Bihn, E. A., Weidmann, M., et al. (2013b). Risk factors associated with Salmonella and Listeria monocytigenes contamination of produce fields. Appl. Environ. Microbiol. 79, 7618–7627. doi: 10.1128/AEM.02831-13
Sunohara, M. D., Topp, E., Wilkes, G., Gottschall, N., Neumann, N., Ruecker, N., et al. (2012). Impact of riparian zone protection from cattle on nutrient, bacteria, F-coliphage, Cryptosporidium, and Giardia loading of an intermittent stream. J. Environ. Qual. 41, 1301–1314. doi: 10.2134/jeq2011.0407
Sutherland, W. J., Pullin, A. S., Dolman, P. M., and Knight, T. M. (2004). The need for evidence-based conservation. Trends Ecol. Evol. 19, 305–308. doi: 10.1016/j.tree.2004.03.018
Tang, Z., Wood, J., Smith, D., Thapa, A., and Aryal, N. (2021). A review on constructed treatment wetlands for removal of pollutants in the agricultural runoff. Sustainability 13, 13578. doi: 10.3390/su132413578
Tate, K. W., Atwill, E. R., Bartolome, J. W., and Nader, G. (2006). Significant Escherichia coli attenuation by vegetative buffers on annual grasslands. J. Environ. Qual. 35, 795–805. doi: 10.2134/jeq2005.0141
Varndell, W., Fry, M., Lutze, M., and Elliott, D. (2021). Use of the Delphi method to generate guidance in emergency nursing practice: a systematic review. Int. Emerg. Nurs. 56, 100867. doi: 10.1016/j.ienj.2020.100867
Weller, D., Brassill, N., Rock, C., Ivanek, R., Mudrak, E., Roof, S., et al. (2020). Complex interactions between weather, and microbial and physicochemical water quality impact the likelihood of detecting foodborne pathogens in agricultural water. Front. Microbiol. 11, 134. doi: 10.3389/fmicb.2020.00134
Weller, D., Shiwakoti, S., Bergholz, P., Grohn, Y., Wiedmann, M., and Strawn, L. K. (2016). Validation of a previously developed geospatial model that predicts the prevalence of Listeria monocytogenes in New York State produce. Appl. Environ. Microbiol. 82, 797–807. doi: 10.1128/AEM.03088-15
Weller, D., Wiedmann, M., and Strawn, K. (2015). Spatial and temporal factors associated with an increased prevalence of Listeria monocytogenes in spinach fields in New York State. Appl. Environ. Microbiol. 81, 6059–6069. doi: 10.1128/AEM.01286-15
Weller, D. L., Kovac, J., Kent, D. J., Roof, S., Tokman, J. I., Mudrak, E., et al. (2017). Escherichia coli transfer from simulated wildlife feces to lettuce during foliar irrigation: a field study in the Northeastern United States. Food Microbiol. 68, 24–33. doi: 10.1016/j.fm.2017.06.009
Weller, D. L., Kovac, J., Kent, D. J., Roof, S., Tokman, J. I., Mudrak, E., et al. (2019). A conceptual framework for developing recommendations for no-harvest buffers around in-field feces. J. Food Prot. 82, 1052–1060. doi: 10.4315/0362-028X.JFP-18-414
Weller, D. L., Love, T. M. T., Weller, D. E., Murphy, C. M., Rahm, B. G., and Wiedmann, M. (2022). Structural equation models suggest that on-farm non-crop vegetation removal is not associated with improved food safety outcomes but is linked to impaired water quality. Appl. Environ. Microbiol. 88, e01600–e01622. doi: 10.1101/2022.09.19.508625
Xu, A., Buchanan, R. L., and Micallef, S. A. (2016). Impact of mulches and growing season on indicator bacteria survival during lettuce cultivation. Int. J. Food Microbiol. Microbiol. 224, 28–39. doi: 10.1016/j.ijfoodmicro.2016.02.013
Keywords: evidence synthesis, farming practice, foodborne pathogen, food safety, fresh produce
Citation: Devarajan N, Weller DL, Jones M, Adell AD, Adhikari A, Allende A, Arnold NL, Baur P, Beno SM, Clements D, Olimpi EM, Critzer F, Green H, Gorski L, Ferelli Gruber A, Kovac J, McGarvey J, Murphy CM, Murphy SI, Navarro-Gonzalez N, Owen JP, Pires AFA, Richard N, Samaddar S, Schmidt R, Scow K, Shariat NW, Smith OM, Spence AR, Stoeckel D, Tran TDH, Wall G and Karp DS (2023) Evidence for the efficacy of pre-harvest agricultural practices in mitigating food-safety risks to fresh produce in North America. Front. Sustain. Food Syst. 7:1101435. doi: 10.3389/fsufs.2023.1101435
Received: 17 November 2022; Accepted: 10 April 2023;
Published: 05 May 2023.
Edited by:
Vasco Augusto Pilão Cadavez, Agricultural College of Bragança, PortugalReviewed by:
Diane G. Newell, University of Surrey, United KingdomAgnes Kilonzo-Nthenge, Tennessee State University, United States
Copyright © 2023 Devarajan, Weller, Jones, Adell, Adhikari, Allende, Arnold, Baur, Beno, Clements, Olimpi, Critzer, Green, Gorski, Ferelli Gruber, Kovac, McGarvey, Murphy, Murphy, Navarro-Gonzalez, Owen, Pires, Richard, Samaddar, Schmidt, Scow, Shariat, Smith, Spence, Stoeckel, Tran, Wall and Karp. This is an open-access article distributed under the terms of the Creative Commons Attribution License (CC BY). The use, distribution or reproduction in other forums is permitted, provided the original author(s) and the copyright owner(s) are credited and that the original publication in this journal is cited, in accordance with accepted academic practice. No use, distribution or reproduction is permitted which does not comply with these terms.
*Correspondence: Naresh Devarajan, bmRldmFyYWphbjAxQGdtYWlsLmNvbQ==