Valorizing staple Native American food plants as a food resilience resource
- 1Department of Ecosystem Science and Management, College of Agricultural Sciences, The Pennsylvania State University, State College, PA, United States
- 2Department of Plant Science, College of Agricultural Sciences, The Pennsylvania State University, State College, PA, United States
Introduction: Increased risks from global climate change and sun-blocking global catastrophic risks (such as a nuclear winter), warrant the reevaluation of our food systems and their resilience to these climatic changes. Increasing native agrobiodiversity is a simple way of increasing food system resilience, and despite the vast amount of native genetic resources available in North America, the United States has shown little systematic effort to improve its agrobiodiversity.
Methods: We use a systematic literature review of staple Native American food plants (NAFPs) to determine the current state of research interest among them and suggest species of interest for domestication. The nutrient profiles and nutrient adequacy scores for the most researched staple NAFPs were also collected and calculated.
Results: A total of 174 Staple NAFPs were evaluated through systematic review, resulting in a total of 5302 peer-reviewed articles searched. Our findings show that most NAFPs are understudied and are more commonly seen by the agricultural community as weeds. However, there are a few that have current scientific interest in their value as a potential crop.
Discussion: We discuss the 24 most researched NAFPs and their nutritional content. We also discuss potential actions, protocols, and ethical issues regarding future steps in using NAFPs to increase agrobiodiversity and food resilience in the United States through more resilient agroforestry systems.
1. Introduction
Both natural and man-made disasters often drastically affect food and nutrition security. For instance, the Ethiopian famines of the 1970s' and 1980s' proved lethal for many, especially before foreign aid (Crummey, 2018). More recently, droughts in Australia and the COVID-19 pandemic have highlighted the fragility of many food supply chain networks (Singh-Peterson and Lawrence, 2015; Deconinck et al., 2021). These disasters and their effects on food security would pale in comparison to sun-blocking global catastrophic risks (GCRs), such as a nuclear war, asteroid strike, or super volcano eruption. For example, several crop models have clearly predicted that any-scale nuclear war between nations would significantly decrease food production across the globe (Denkenberger and Pearce, 2015; Xia et al., 2015; Jagermeyr et al., 2020). In addition to inevitable supply-chain disruptions after a GCR, severe climate-shifts to a drier, colder, and darker environment would drive crop production decline for many years (Toon et al., 1979, 2019; Coupe et al., 2019). The annual risk of a nuclear war occurring has been estimated as high as 1%, which could certainly continue to be decreased with more strategic diplomacy (Barrett et al., 2013). Concurrently, the world has seen unprecedented and rapid shifts in climate warming, causing more frequent and more severe natural disasters (Garner et al., 2017; Smith et al., 2022). Both the increased risk of natural disasters and higher risk of GCRs mean that creating a more resilient global food system is even more important than ever.
One strategy for food sustainability and resilience, even through disasters, is by increasing and preserving agrobiodiversity. Agrobiodiversity is very broadly defined by the FAO as “the variety and variability of animals, plants and micro-organisms that are used directly or indirectly for food and agriculture, including crops, livestock, forestry, and fisheries. It comprises the diversity of genetic resources (varieties, breeds) and species used for food, fodder, fiber, fuel, and pharmaceuticals. It also includes the diversity of non-harvested species that support production (soil micro-organisms, predators, pollinators), and those in the wider environment that support agro-ecosystems (agricultural, pastoral, forest, and aquatic) as well as the diversity of the agro-ecosystems” (FAO, 1999). Increases in agrobiodiversity mean increased resilience to any changes in the environment and can help dampen the effects from climate change on food production, such as drought (Gonzalez, 2011; Ortiz, 2011; Chivenge et al., 2015). In addition to resiliency from climate change, agrobiodiversity also protects against the spread of crop diseases and buffers yield losses (Gonzalez, 2011). In addition to direct crop-system resilience, increased agrobiodiversity leads to better overall nutrition, boosts in local economies, community independence, and cultural preservation (Birol et al., 2006; Gonzalez, 2011; Dwivedi et al., 2013; Bioversity International., 2019; Mabhaudhi et al., 2019; Odhiambo et al., 2019; Chatzopoulou et al., 2020; Carney, 2021; Tamariz, 2022). If done correctly, agrobiodiversity can also increase native biodiversity by emulating native environments (Gonzalez, 2011; Khumalo et al., 2012).
According to Bioversity International. (2020), the United States scores below average on their agrobiodiversity index compared to other developed nations. Likewise, the US government does not seem to be prioritizing plans for increasing or preserving agrobiodiversity resources for the future, exemplified by their not submitting a National Biodiversity Strategies and Action Plan (NBSAP) to the Convention on Biological Diversity (Jones et al., 2021). To increase the resilience of the US agricultural and food system to these many potential future threats, actions need to be taken to prioritize strategies of increasing and preserving agrobiodiversity resources within US agroecosystems, preferably at a local scale (Paci-Green and Berardi, 2015; Bioversity International., 2019).
North America has no shortage of available plants to use for this purpose, having thousands of seldom-used wild edible plants (WEP)s and hundreds of unique crop varieties (Moerman, 1998; Veteto, 2014). The crops and WEPs once used abundantly by Native Americans are a largely underutilized resource of North America and if developed and valorized could increase the United States' agrobiodiversity, and consequently, food resilience in the face of disaster events and a changing climate (Small, 2013; Bioversity International., 2019). Sadly, much of the knowledge on how to use these species was lost with the extermination and extirpation of many Native American people groups (Moerman, 1998; Veteto, 2014). Even those plant species that were not necessarily domesticated by Native Americans may have still been grown in manipulated ecosystems for the purpose of increased yield and not natural “wild” landscapes (Hladik et al., 1993). For these reasons, we have refrained from using the term WEPs, as the line between wild and semi-domesticated plants is somewhat blurred and cannot be confirmed because some of those who used these plants are no longer living. Throughout this paper we will refer to these plants once used as staple foods by Native American as Native American food plants (NAFPs).
The purpose of this study is to better direct the attention of researchers and industry leaders toward NAFPs that would increase nutritional, economic, and ecological resilience of the United States. The NAFPs with the most available research are likely the most useful options as an abundant foundation of knowledge and focused attention is needed for the efficient use and scaling up and profitability of any new crop and plant resource (Cerón-Souza et al., 2021). In this study we propose a list of several plant species and genetic resources of interest, that would increase America's agrobiodiversity and food resilience if preserved and valorized.
2. Methods
To create this list of species and cultivars we have done a literature review on species listed as a “Staple” food in Daniel Moerman's book and database Consejo Nacional del Ambiente (2001) created using primary records ethnographic studies of 291 native people groups in North America. The top NAFPs in this literature review will be highlighted with their nutritional content and possible yields if available in the literature.
A total of 225 NAFPs are listed in Daniel Moerman's book as being used as “Staple” foods by one or more native nations of North America. Of those plants, 196 are native to North America. Of those, 7 do not have species level identification listed and 11 are duplicated as different subspecies. Of the remaining 178 plants, 4 were excluded from the literature search as they have become common mainstream domesticated crops. Those species were cayenne pepper (Capsicum annuum), field pumpkin (Cucurbita pepo), wild strawberry (Fragaria vesca), and common sunflower (Helianthus annuus). The remaining 174 plants were used in the systematic literature review.
The systematic literature review was conducted with the following criteria using Penn States available 940 databases accessed between 03/23/2022 - 11/20/2022: Literature published between 03/23/1982 – 03/23/2022, only Peer-Reviewed articles, only articles in English, only search titles of articles (Figure 1). The purpose of limiting the keyword search to only within the title is to only focus on research that is prioritizing or valorizing specific NAFPs and not merely mentioning them. The genus and species of each NAFP (i.e., “Genus species”) was then searched using the criteria and all available literature was then categorized into one or more subjects with the definitions in Table 1. All duplicates or articles in other languages other than English were not included in total numbers. In addition to the literature review, the regionality, botanical family, habit, ecological status (Either IUCN or NatureServe if IUCN not available), part(s) used, and duration (annual, perennial, or biannual) for each NAFP were collected. Plant region data were gathered from the USDA Plant Database and regionality assigned to plants depending on the states and provinces they were native to Supplementary material.
The micro- and macronutrient profiles of these plants were compiled using available literature and their nutritional density scores, and powerhouse fruit and vegetable (PFV) scores were calculated if enough nutrient value information was available using the methods from di Noia (2014). These scores were calculated using the following equations, where “nutrienti” is one of 17 nutrients chosen by di Noia (2014) for evaluation:
The top ten NAFPs with the most total peer-reviewed literature available, top ten NAFPs with the most nutritional articles, and top ten annual NAFPs with the most total peer-reviewed literature available were compiled into a list (excluding duplicates) (Table 2). Among the top 24 selected plants, only Zizania spp. and Amaranthus spp. had all data available to calculate a nutrient adequacy score, therefore if more than half of the nutrient data was available, a nutrient adequacy score was calculated.
3. Results
A total of 5,302 peer-reviewed articles were categorized and codified for 127 of the staple NAFPs. Yet, 47 of the reviewed NAFPs from Moerman's database did not have any peer-reviewed literature available with their species name in the title over the past 40 years among the databases available to Penn State University Libraries. Only 32 NAFPs had any nutritional literature available about them in the last 40 years.
Although there was more information available on some of these NAFPs' nutrition, only data on the raw whole portion of plant used as a staple food was considered when evaluating the 24 NAFPs' micro- and macronutrients (Table 3) (Darmon et al., 2005; di Noia, 2014). Even still some plants only had genus specific nutritional data which we have labeled accordingly. These nutritional values and nutrition adequacy scores for species are compared with yellow corn grain in Table 4. Among the NAFPs examined, the genus Zizania had the highest nutrient adequacy (22.69) and nutrient density score (6.36), followed by the genus Amaranthus (16.38 and 4.4, respectively). These values were higher than the yellow corn nutrient adequacy score (13.61) and nutrient density score (3.73). All the other NAFPs considered had nutrient adequacy and density scores similar to or lower than yellow corn grain, with Vaccinium myrtillus having the lowest nutrient adequacy score.
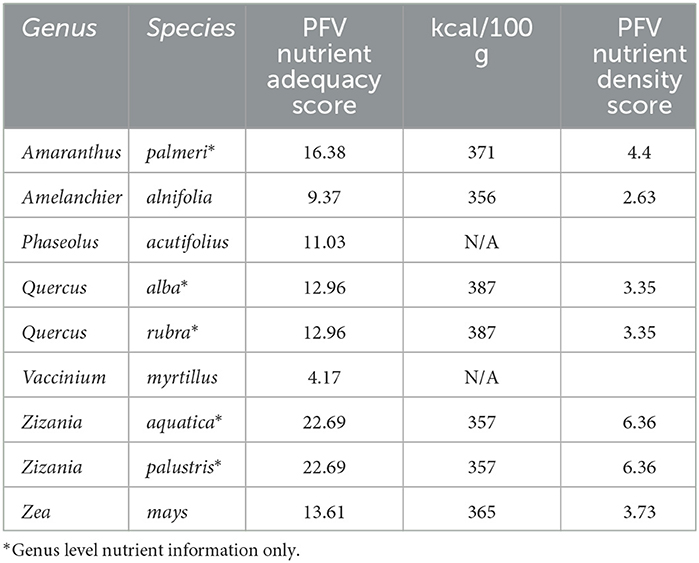
Table 4. Powerhouse fruit and vegetable (PFV) Scores calculated from di Noia (2014) methodology for NAFPs compared to maize (Z. mays).
Currently, the most researched NAFPs for their nutrition and domestication are Mojave Yucca (Yucca schidigera), Whortleberry (Vaccinium myrtillus), Saskatoon serviceberry (Amelanchier alnifolia), and tepary bean (Phaseolus acutifolius). However, most literature for Mojave yucca was directed toward its use as a feedstock for animals rather than the nutritional value of its fruits, which is the plant part listed as a staple for Native American nations. The NAFPs with the most available nutritional values were amaranth (Amaranthus spp.), black walnut (Juglans nigra), wild rice (Zizania spp.), Saskatoon serviceberry (Amelanchier alnifolia), and acorns (Quercus spp.). Although these top five have the most nutritional information available, three of them are only to genus level, and most values for black walnut are for the dried nut and not raw. This leaves Saskatoon serviceberry with the most available nutritional values and one of the plants with the most literature available making it a good candidate for domestication and use.
Most plants reviewed could be found in the Western US (88.5% of NAFPs), or Southwest US (70.7% of NAFPs). The least represented areas were the central, Atlantic, and northern Canadian regions. Only 14.9% of staple NAFPs had ecological statuses that were not a G5 NatureServe rating or Least Concern IUCN rating. There were 45 botanical families represented among the listed staple NAFPs. The five most commonly represented botanical families among the staple NAFPs were the oak and beech family (Fagaceae: 19), sunflower and daisy family (Asteraceae: 16), grass family (Poaceae: 15), cactus family (Cactaceae: 14), and bean family (Fabaceae: 11).
4. Discussion
Our systematic review exposes the lack of literature and ongoing development of NAFPs and their domestication. The lack of substantial nutritional research for most of the listed staple NAFPs shows the lack of interest, funding, and attention to these food crops and likewise, the lack of attention to Native American voices and cultures. Similarly, more literature on NAFPs had been focused on decreasing their growth and prevalence as weeds rather than on how to increase their growth and prevalence through horticultural methods. More than half of the available literature on these staple NAFPs fell under the ecological or organismal study categories, with the next most interest being their pharmaceutical value.
Only 18.4% of staple NAFPs have any literature concerning their nutritional value, and only eight NAFPs have more than five articles on their nutritional value. This shows the overall lack of attention on this group of plants as an agricultural resource. The staple NAFPs regionality follow similar geographic trends to plant diversity geographical distribution in North America, where there is more diversity the farther south in latitude and west in longitude on the North American continent (Qian, 1999).
Wild rice, amaranth seeds, and saskatoon serviceberry all have nutrient adequacy scores and nutrient density scores higher than yellow corn grain. Although there is not much species-specific nutritional information for the species listed in this review, it is important to note that many are congeners with other food plants. It has been shown that plants of the same genus usually have similar nutritional profiles, meaning that many of the plants listed, although understudied, have high nutritional potential given their relation to other nutritious plants (Cantwell-Jones et al., 2022).
4.1. Actions for the future
In order to increase the agrobiodiversity and resilience of North American agriculture, attention must be redirected effectively to these staple NAFPs. Other research involving the incorporation of underutilized plants into modern agriculture has listed government incentives and further nutritional research as key components of success (Hunter et al., 2019; Tharmabalan, 2021). Previous research has also emphasized the importance of cataloging a priority list of underutilized crop plants and gathering more information about them as an essential step in this process (Cerón-Souza et al., 2021). This paper intends to establish what the state of current research is in this area in North America, and which NAFPs have the best foundation of knowledge to use. Likewise, incentives to incorporate some of these foods into local school systems would not only increase awareness of these food resources and their cultural significance, but also provide support for local farmers, healthier school diets, and shorter supply chains (Bioversity International., 2019; Hunter et al., 2019; Mabhaudhi et al., 2019).
Currently, there are no federal protections for wild crop relatives (WCR) in the United States. Both WCRs and NAFPs, are overlapping categories of native edible plants and can both be considered agricultural genetic resources (AGR). The only distinction between WCR and NAFP being genetic relatedness to mainstream agricultural crops. Additionally, there are no protections for WCRs at any state level, and 17 states do not even have any protections in place for endangered plants on state land (Greene et al., 2018). The lack of policies to protect AGR within the United States at both the national and state levels needs to change. Our recommendations as first steps are to identify and define AGR as a clear group of protected plants which should be recognized by federal and state policymakers. Secondly, we recommend that AGR be granted similar protections to endangered and threatened plant species, with possible allowances for harvest by Native American peoples, plant breeding, and controlled use by local farmers.
A possible framework for protection and policy making is the multi-stakeholder, multi-disciplinary, and multi-sector participatory methodology (3M) put forward by Wale et al. in 2009. In this framework, policies are created with a more holistic view with multiple stakeholders interests in mind. Using this framework, protection and use policies are more quickly implemented with more widespread approval (Wale et al., 2009). These policies may include incentives for local farmers to take part in in situ conservation strategies which benefit them and their local communities (Gepts, 2006). Likewise, policies could be implemented that are focused on in situ protection of AGR located in state and federal lands that are naturally rich in AGR. These policies should also include incentives to research and identify public land that is rich in AGR (Ford-Lloyd et al., 2011).
4.2. Domestication
Domestication can be defined as “the selection process exerted –consciously or unconsciously – by humans to adapt wild plants and animals to cultivation and herding, respectively” (Gepts and Papa, 2003). To accomplish these next steps, domestication of NAFPs must take place to allow for these foods to be available to those who need them and to preserve their genetic resources. It is important to note that the domestication of any plant should be looked at in a holistic manner as it requires not only changing plant characteristics, but also creating infrastructure and demand to meet production demands (van Tassel et al., 2020). Domestication in the past has occurred by time-intensive selective breeding which usually results in loss of undesirable traits. These same results can quickly be achieved through the use of gene editing and if applied to NAFPs, it would be possible to domesticate these plants within 5 years (Østerberg et al., 2017). Studies suggest that increasing the yield of edible plant portion is one of the most important traits when domesticating a plant (Luo et al., 2022).
To accomplish new plant domestications in an easy and timely manner, domestication pipelines need to be established using university and government combined resources. Domestication pipelines for grain crops have already been theorized and partially implemented with species like Zizania palustris, one of our highlighted staple NAFPs (DeHaan et al., 2016). However, the partial domestication of Z. palustris has already brought several ethical and cultural questions to light which we will discuss in the next section of this paper. Additionally, depending on the mode of domestication, there may be major push back by mainstream culture due to possible legal GMO statuses of newly domesticated species due to gene editing or even mutation breeding even though these methods preserve more genetic diversity than traditional breeding methods (Østerberg et al., 2017; Luo et al., 2022).
4.3. Biopiracy and importance of collaboration
Because all of these NAFPs are interconnected with the culture, land, and intellectual property of many Native American groups and nations, the use and development of any crops from NAFPs must be fully discussed with the appropriate Native American nations. As previously mentioned, the partial domestication of northern wild rice (Zizania palustris) is a good example of the complexity and importance of this issue. The University of Minnesota was asked to domesticate Z. palustris by non-native farmers and successfully did so; however, none of this was discussed with the local Anishinaabe nations from which the germplasm was retrieved (DeHaan et al., 2016). The neglect of communication and initial plan for proper collaboration resulted in the disrespecting and devaluing of Anishinaabe culture and has been viewed as biopiracy and colonialism. In the future, any research on the development of NAFPs into products must have consent and collaboration from the appropriate native nations from which the NAFP is found and had been used (DeHaan et al., 2016).
Communication and clear regulations on the development of NAFPs into crops or products are needed to avoid biopiracy. Any agreements must ensure that all parties are clearly and equally represented and benefited from said crops and products, and any actions are in collaboration with Native American cultures and preferences (Love and Spaner, 2007; MacKey and Liang, 2012; Cottrell, 2022). Relationships among Native American Nations within the United States, although having an effect on possible policy creation and implementation, is complex and is outside the scope of this paper.
4.4. Disasters and catastrophe food resilience
Because of the lack of information available for these species, it is currently difficult to predict which plants would best be used to improve resilience to climate disasters and global catastrophes. Increasing knowledge on the basic growing requirements for these plants will better direct the scientific community in choosing which of these plants to pursue for domestication. Although there is a lack of information, it can be inferred that plant genera and families with more drought resistant and heat resistant attributes would be profitable for resilience to a warming climate, while others that are drought tolerant, cool tolerant, and shade tolerant and grow quickly would be the most desirable plants in a global sun-blocking catastrophe. One of these plant groups that may do well in a sun-blocking catastrophe are ferns (Polypodiopsida), given their fast-growing nature, short harvest time, cool tolerance, and shade tolerance. For example, the widespread range of bracken fern, and its multiple edible parts would make it ideal for domestication as a disaster food and indoor crop, however it has been proven to have toxic and carcinogenic properties (Maroyi, 2014; Ugochukwu, 2019; Kreshchenok et al., 2021; Sareen et al., 2021; Singla et al., 2022).
Turning our attention to the current warming climate, finding crops that do better in warmer and drier conditions may be necessary. The tepary bean's ability to grow in hot and dry conditions makes it an ideal candidate for growth in the current warming climate (Porch et al., 2017). Likewise, many amaranth species are also good examples of plants that could grow better in a warming climate and produce micro- and macronutrient rich seeds (Gregory et al., 2019; Aderibigbe et al., 2022; Winstead and Jacobson, 2022).
Several of the foods listed are nuts and fruits from trees. This would require the adoption of agroforestry techniques and growing more perennial crops rather than annual monocultures. Native trees like black walnut, American chestnut, ponderosa pine, honey mesquite, white and red oaks, and serviceberries, would offer very nutritious and high yielding food products if incorporated into agroforestry systems with other shaded crop interplanting. This method of agroforestry using the NAFPs suggested would not only increase the agrobiodiversity and crop resilience of American agriculture, but it would also provide ecological services as emulated natural habitat for native species within a variety of different climates (Nair et al., 2017). Lovell et al. (2018) conceptualized a multifunctional woody polyculture (MWP) agroforestry system for temperate areas using native trees and shrubs which emulates natural structure of a forest, increasing resilience, efficiency, and sustainability (Lovell et al., 2018). A follow up study showed that these MWP systems, although of high interest to farmers, could not be implemented because of lack of equipment and access to processing facilities (Stanek et al., 2019). These studies show how there is not only many benefits to agroforestry systems, but there is also interest if the right infrastructure were to be created through programs like government subsidies.
4.5. Comparison to other countries
We can look to other countries that scored higher than the US on the agrobiodiversity index for ideas on how to best proceed with using this information. The top three countries were Italy, Peru, and Australia. Looking at these three countries' National Biodiversity Strategies and Action Plans (NBSAPs), several commonalities stand out (DEST, 1996; Consejo Nacional del Ambiente, 2001; Ministero Dell'ambiente, 2010). For example, there is an emphasis in each about ensuring that the benefits from any domesticated or monetized biological resources have equitable benefits for all who provided knowledge, land, or material of the resource. These countries also advocated for the increased focus on scientific research and funding into research of biological resources and the analysis of current knowledge gaps and research on these resources, like the present review. Likewise, there is emphasis in each on creating some form of government branches to monitor and implement changes regarding their nations' biodiversity. Italy's NBSAP emphasizes that economic, social, and cultural factors need to be considered in addition to merely considering conservation as a driving argument for increasing and protecting biodiversity (Ministero Dell'ambiente, 2010). This holistic approach, with the integration of public policy and private intervention has the potential to lead to new sustainable territorial development especially in marginal areas (Bocci and Bertacchini, 2009; Scaramuzzi et al., 2021). In this perspective, it is important to highlight that in Italy and other Mediterranean countries the use of agrobiodiversity resources, including wild edible plants, has a long history and is part of the Mediterranean diet (Chatzopoulou et al., 2020). Over the last decades, Italy and other European countries have done large investments in research aimed at characterizing the agronomic and nutritional properties of neglected genetic resources (Łuczaj et al., 2012; Pinela et al., 2017), and the scientific community in synergy with local rural communities, chefs, and small farms greatly contributed to the valorization of such neglected genetic resources (Petropoulos et al., 2019, 2020; Conversa et al., 2020; Corrêa et al., 2020a,b). Over time, these initiatives led to the domestication of several wild edible species and the creation of new market opportunities especially for smallholder farms that often have become the custodians of agrobiodiversity resources (Hadjichambis et al., 2008; FAO, 2019). With this approach, in situ conservation of agrobiodiversity resources can complement ex situ conservation efforts (Love and Spaner, 2007; Engels and Ebert, 2021).
4.6. Limitations
This review is not intended to provide an exhaustive list of staple crops used by Native Americans or provide a list of all the possible plants that could be domesticated or used for food native to North America. This review is designed to highlight the most researched NAFPs, and which have relatively more interest and information available than others. This review is not intended to show exactly how much literature is available, but rather the systematic literature search was used as a tool to quantify relative current interest in the scientific community. Because our systematic literature review was for the purpose of determining current interest and limited to the past 40 years and articles, it is possible that there could have been more research interest in the past than there is now.
5. Conclusion
Love and Spaner (2007) suggest that “conservation of agrobiodiversity is a prerequisite for the development of sustainable agricultural systems.” The state of research of NAFPs in North America leaves much to be desired. Although there is current research on some of these staple NAFPs, most of this research has no focus on their nutritional value or potential domestication. We have discussed how the incorporation of these staple NAFPs into the U.S. agricultural system, would increase the resilience of the U.S. food system. The partnership and approval of Native American nations toward domestication pipelines for staple NAFPs with clear delineations on GMO statuses is imperative. As the risk and likelihood of catastrophes and one-off disaster occurrences increase, so also should the U.S. agricultural system grow in its resilience to anticipate these increased risks. We have shown that the limited literature available for the nutritional information of NAFPs is promising by their high Powerhouse Fruit and Vegetable value scores. Future research should focus on the incorporation of these NAFPs into government incentive programs and school food systems, as well as the domestication of the most well-known and highly nutritious NAFPs. Although the U.S. agricultural system is currently mostly monoculture agronomy systems, proper government incentive programs and accessibility to post-harvest preparation would facilitate NAFP agroforestry as there is already farmer interest. Not only do these changes have direct agricultural benefits, but the valorization of NAFPs may lead to a valorization of the voice and culture of many Native American nations and the conservation of our native biodiversity.
Data availability statement
The original contributions presented in the study are included in the article/Supplementary material, further inquiries can be directed to the corresponding author.
Author contributions
DW and FD developed the idea. DW, FD, and MJ contributed to conception and design of the work. DW conducted the literature search, organized the database, conducted the analysis, and with the support of FD and MJ wrote the first draft of the manuscript. All authors contributed to manuscript revision, read, and approved the submitted version.
Funding
This research was funded by the Food Resilience in the Face of Catastrophic Global Events grant funded by Open Philanthropy and FD contribution was supported by the USDA National Institute of Food and Agriculture and Hatch Appropriations under Project #PEN04723 and Accession #1020664.
Conflict of interest
The authors declare that the research was conducted in the absence of any commercial or financial relationships that could be construed as a potential conflict of interest.
Publisher's note
All claims expressed in this article are solely those of the authors and do not necessarily represent those of their affiliated organizations, or those of the publisher, the editors and the reviewers. Any product that may be evaluated in this article, or claim that may be made by its manufacturer, is not guaranteed or endorsed by the publisher.
Supplementary material
The Supplementary Material for this article can be found online at: https://www.frontiersin.org/articles/10.3389/fsufs.2023.1117805/full#supplementary-material
References
Aderibigbe, O. R., Ezekiel, O. O., Owolade, S. O., Korese, J. K., Sturm, B., Hensel, O., et al. (2022). Exploring the potentials of underutilized grain amaranth (Amaranthus spp.) along the value chain for food and nutrition security: a review. Crit. Rev. Food Sci. Nutr. 62, 656–669. doi: 10.1080/10408398.2020.1825323
Antora, S. A., Ho, K., van, Lin, C. H., Thomas, A. L., Lovell, S. T., and Krishnaswamy, K. (2022). Quantification of vitamins, minerals, and amino acids in black walnut (Juglans nigra). Front. Nutr. 9, 936189. doi: 10.3389/fnut.2022.936189
Barrett, A. M., Baum, S. D., and Hostetler, K. (2013). Analyzing and reducing the risks of inadvertent nuclear war between the United States and Russia. Sci. Global Security 21, 106–133. doi: 10.1080/08929882.2013.798984
Bioversity International. (2019). Agrobiodiversity Index Report 2019: Risk and Resilience. Rome, Italy: Bioversity International.
Bioversity International. (2020). Agrobiodiversity, School Gardens and Healthy Diets: Promoting Biodiversity, Food and Sustainable Nutrition. Oxford: Routledge.
Birol, E., Smale, M., and Gyovai, A. (2006). Using a choice experiment to estimate farmers' valuation of agrobiodiversity on hungarian small farms. Environ. Resour. Econ. 34, 439–469. doi: 10.1007/s10640-006-0009-9
Bocci, R., Chiari, T., Bertacchini, E., Melozzi, L., Naziri, D., and Nonne, M. (2009). The Sustainable Use of Agrobiodiversity in Italy-Report on Case Studies on Article 6 of the International Treaty on Plant Genetic Resources for Food and Agriculture. Istituto Agronomico per l'Oltremare. doi: 10.13140/RG.2.1.3375.5365
Cantwell-Jones, A., Ball, J., Collar, D., Diazgranados, M., Douglas, R., Forest, F., et al. (2022). Global plant diversity as a reservoir of micronutrients for humanity. Nat. Plants 8, 225–232. doi: 10.1038/s41477-022-01100-6
Carney, J. A. (2021). Subsistence in the Plantationocene: dooryard gardens, agrobiodiversity, and the subaltern economies of slavery. J. Peasant Studies 48, 1075–1099. doi: 10.1080/03066150.2020.1725488
Cerón-Souza, I., Galeano, C. H., Tehelen, K., Jiménez, H. R., and González, C. (2021). Opportunities and challenges to improve a public research program in plant breeding and enhance underutilized plant genetic resources in the tropics. Genes. 12, 1584. doi: 10.3390/genes12101584
Chatzopoulou, E., Carocho, M., Gioia, D., and Petropoulos, F. (2020). The beneficial health effects of vegetables and wild edible greens: the case of the Mediterranean diet and its sustainability. Appl. Sci. 10, 1–27. doi: 10.3390/app10249144
Chivenge, P., Mabhaudhi, T., Modi, A. T., and Mafongoya, P. (2015). The potential role of neglected and underutilised crop species as future crops under water scarce conditions in Sub-Saharan Africa. Int. J. Environ. Res. Public Health 12, 5685–5711. doi: 10.3390/ijerph120605685
Consejo Nacional del Ambiente (2001). Perú: Estrategia Nacional Sobre Diversidad Biológica. Peru: CONAM.
Conversa, G., Lazzizera, C., Bonasia, A., Cifarelli, S., Losavio, F., Sonnante, G., et al. (2020). Exploring on-farm agro-biodiversity: a study case of vegetable landraces from Puglia region (Italy). Biodivers. Conserv. 29, 747–770. doi: 10.1007/s10531-019-01908-3
Corrêa, R. C. G., di Gioia, F., Ferreira, I., and Petropoulos, S. A. (2020b). Halophytes for future horticulture: the case of small-scale farming in the mediterranean basin. Halophytes Future Hortic. 10, 1–28. doi: 10.1007/978-3-030-17854-3_121-1
Corrêa, R. C. G., di GIoia, F., Ferreira, I. C. F. R., and Petropoulos, S. A. (2020a). “Wild greens used in the Mediterranean Diet,” in The Mediterranean Diet: An Evidence-Based Approach, eds. V. Preedy and R. Watson (London: Academic Press), 209–228.
Cottrell, C. (2022). Avoiding a new era in biopiracy: including indigenous and local knowledge in nature-based solutions to climate change. Environ. Sci. Policy 135, 162–168. doi: 10.1016/j.envsci.2022.05.003
Coupe, J., Bardeen, C. G., Robock, A., and Toon, O. B. (2019). Nuclear winter responses to nuclear war between the united states and russia in the whole atmosphere community climate model version 4 and the goddard institute for space studies ModelE. J. Geophys. Res. 124, 8522–8543. doi: 10.1029/2019JD030509
Crummey, D. E. (2018). “Famine in Ethiopia, 1973-1974 and 1984-1985,” in Farming and Famine: Landscape Vulnerability in Northeast Ethiopia, ed. J. C. Russell (Madison: University of Wisconsin Press), 34–55.
Darmon, N., Darmon, M., Maillot, M., and Drewnowski, A. (2005). A nutrient density standard for vegetables and fruits: Nutrients per calorie and nutrients per unit cost. J. Am. Diet. Assoc. 105, 1881–1887. doi: 10.1016/j.jada.2005.09.005
Davis, S. C. (2022). Agave americana: characteristics and potential breeding priorities. Plants 11, 305. doi: 10.3390/plants11172305
Deconinck, K., Avery, E., and Jackson, L. A. (2021). Food supply chains and covid-19: impacts and policy lessons. EuroChoices 19, 34–39. doi: 10.1111/1746-692X.12297
DeHaan, L. R., van Tassel, D. L., Anderson, J. A., Asselin, S. R., Barnes, R., Baute, G. J., et al. (2016). A pipeline strategy for grain crop domestication. Crop Sci. 56, 917–930. doi: 10.2135/cropsci2015.06.0356
Denkenberger, D., and Pearce, J. (2015). Feeding everyone: solving the food crisis in event of global catastrophes that kill crops or obscure the sun. Futures 72, 57–68. doi: 10.1016/j.futures.2014.11.008
DEST (1996). National Strategy for the Conservation of Australia's Biological Diversity. Canberra: Australian Government. Available online at: www.environment.gov.au/biodiversity/publications/strategy/index.html (accessed December 5, 2022).
di Noia, J. (2014). Defining powerhouse fruits and vegetables: a nutrient density approach. Prev. Chronic Dis. 11, 130390. doi: 10.5888/pcd11.130390
Dwivedi, S., Sahrawat, K., Upadhyaya, H., and Ortiz, R. (2013). Food, Nutrition and Agrobiodiversity Under Global Climate Change Advances in Agronomy. Amsterdam: Elsevier, 1–128.
Engels, J. M. M., and Ebert, A. W. (2021). A critical review of the current global ex situ conservation system for plant agrobiodiversity. I. history of the development of the global system in the context of the political/legal framework and its major conservation components. Plants 10, 557. doi: 10.3390/plants10081557
FAO (1999). Agricultural Biodiversity. in FAO Multifunctional Character of Agriculture and Land: Conference Background Paper No. 1 (Maastricht). Rome: FAO.
Ford-Lloyd, B. V., Schmidt, M., Armstrong, S. J., Barazani, O., Engels, J., Hadas, R., et al. (2011). Crop wild relatives - Undervalued, underutilized and under threat? Bioscience 61, 559–565. doi: 10.1525/bio.2011.61.7.10
Garner, A. J., Mann, M. E., Emanuel, K. A., Kopp, R. E., Lin, N., Alley, R. B., et al. (2017). Impact of climate change on New York City's coastal flood hazard: increasing flood heights from the preindustrial to 2300 CE. Proc. Nat. Acad. Sci. 114, 11861–11866. doi: 10.1073/pnas.1703568114
Gepts, P. (2006). Plant genetic resources conservation and utilization: the accomplishments and future of a societal insurance policy. Crop Sci. 46, 2278–2279. doi: 10.2135/cropsci2006.03.0169gas
Gepts, P., and Papa, R. (2003). Evolution During Domestication Encyclopeia of Life Sciences. London: Wiley.
Golubkina, N. A., Kekina, H. G., Engalichev, M. R., Antoshkina, M. S., and Caruso, G. (2018). Genotypic effect on fruit production and quality, antioxidant content and elemental composition of organically grown Physalis angulata L. and Physalis pubescens L. Folia Hortic. 30, 367–374. doi: 10.2478/fhort-2018-0032
Gonzalez, C. G. (2011). Climate change, food security, and agrobiodiversity: toward a just, resilient, and sustainable food system. Fordham Environ. Law Rev. 22, 493–522. Available online at: https://ssrn.com/abstract=1756914
Greene, S. L., Williams, K. A., Khoury, C. K., Kantar, M. B., and Marek, L. F. (2018). North American Crop Wild Relatives, Volume 1: Conservation Strategies. Cham: Springer.
Gregory, P. J., Mayes, S., Hui, C. H., Jahanshiri, E., Julkifle, A., Kuppusamy, G., et al. (2019). Crops for the future (CFF): an overview of research efforts in the adoption of underutilised species. Planta 250, 979–988. doi: 10.1007/s00425-019-03179-2
Grimes, S. J., Capezzone, F., Nkebiwe, P. M., and Graeff-Hönninger, S. (2020). Characterization and evaluation of salvia hispanica l and salvia columbariae benth. varieties for their cultivation in southwestern germany. Agronomy 10, 2012. doi: 10.3390/agronomy10122012
Hadjichambis, A. C. H., Paraskeva-Hadjichambi, D., Della, A., Elena Giusti, M., Pasquale, d. e., Lenzarini, C. C., et al. (2008). Wild and semi-domesticated food plant consumption in seven circum-Mediterranean areas. Int. J. Food Sci. Nutr. 59, 383–414. doi: 10.1080/09637480701566495
Harden, M. L., and Zolfaghari, R. (1988). Nutritive composition of green and ripe pods of honey mesquite (Prosopis glandulosa, Fabaceae). Econ. Bot. 42, 522–532. doi: 10.1007/BF02862796
Hladik, C. M., Linares, O. F., Hladik, A., Pagezy, H., and Semple, A. (1993). “Tropical forests, people and food: an overview,” in Tropical Forests, People and Food, eds. C. M. Hladik, A. Hladik, O. F. Linares, H. Pagezy, A. Semple, and M. Hadley (New York, NY: The Parthenon Publishing Group), 3–14.
Hunter, D., Borelli, T., Beltrame, D. M. O., Oliveira, C. N. S., Coradin, L., Wasike, V. W., et al. (2019). The potential of neglected and underutilized species for improving diets and nutrition. Planta 250, 709–729. doi: 10.1007/s00425-019-03169-4
Idouraine, A., Tinsley, A. M., and Weber, C. W. (1989). Nutritional quality and sensory acceptability of akara prepared from germinated tepary beans. J. Food Sci. 54, 114–117. doi: 10.1111/j.1365-2621.1989.tb08580.x
Jagermeyr, J., Robock, A., Elliott, J., Muller, C., Xia, L., Khabarov, N., et al. (2020). A regional nuclear conflict would compromise global food security. Proc. Natl. Acad. Sci. U S A 117, 7071–7081. doi: 10.1073/pnas.1919049117
Jones, S. K., Estrada-Carmona, N., Juventia, S. D., Dulloo, M. E., Laporte, M. A., Villani, C., et al. (2021). Agrobiodiversity Index scores show agrobiodiversity is underutilized in national food systems. Nat. Food 2, 712–723. doi: 10.1038/s43016-021-00344-3
Khumalo, S., Chirwa, P. W., Moyo, B. H., and Syampungani, S. (2012). The status of agrobiodiversity management and conservation in major agroecosystems of Southern Africa. Agric. Ecosyst. Environ. 157, 17–23. doi: 10.1016/j.agee.2012.01.028
Kreshchenok, I., Lesik, E., Toushkin, A., and Toushkina, A. (2021). Edible Ferns of the Amur Region and Their Rational Use. IOP Conference Series: Earth and Environmental Science. London: IOP Publishing Ltd.
Love, B., and Spaner, D. (2007). Agrobiodiversity: Its value, measurement, and conservation in the context of sustainable agriculture. J. Sust. Agric. 31, 53–82. doi: 10.1300/J064v31n02_05
Lovell, S. T., Dupraz, C., Gold, M., Jose, S., Revord, R., Stanek, E., et al. (2018). Temperate agroforestry research: considering multifunctional woody polycultures and the design of long-term field trials. Agroforestry Syst. 92, 1397–1415. doi: 10.1007/s10457-017-0087-4
Łuczaj, Ł., Pieroni, A., Tardío, J., Pardo-De-Santayana, M., Sõukand, R., Svanberg, I., et al. (2012). Wild food plant use in 21st century Europe: the disappearance of old traditions and the search for new cuisines involving wild edibles. Acta Soc. Bot. Poloniae 81, 359–370. doi: 10.5586/asbp.2012.031
Luo, G., Najafi, J., Correia, P. M. P., Trinh, M. D. L., Chapman, E. A., Østerberg, J. T., et al. (2022). Accelerated domestication of new crops: yield is key. Plant Cell Physiol. 18, 1–17. doi: 10.1093/pcp/pcac065
Mabhaudhi, T., Chibarabada, T. P., Chimonyo, V. G. P., Murugani, V. G., Pereira, L. M., Sobratee, N., et al. (2019). Mainstreaming underutilized indigenous and traditional crops into food systems: a south African perspective. Sustainability 11, 172. doi: 10.3390/su11010172
MacKey, T. K., and Liang, B. A. (2012). Integrating biodiversity management and indigenous biopiracy protection to promote environmental justice and global health. Am. J. Public Health 102, 1091–1095. doi: 10.2105/AJPH.2011.300408
Maroyi, A. (2014). Not just minor wild edible forest products: Consumption of pteridophytes in sub-Saharan Africa. J. Ethnobiol. Ethnomed. 10, 1–15. doi: 10.1186/1746-4269-10-78
Marrs, R. H., and Watt, A. S. (2006). Biological flora of the British Isles: Pteridium aquilinum (L.) Kuhn. J. Ecol. 94, 1272–1321. doi: 10.1111/j.1365-2745.2006.01177.x
Mazza, G. (2006). Compositional and functional properties of Saskatoon berry and blueberry. Int. J. Fruit Sci. 5, 101–120. doi: 10.1300/J492v05n03_10
Ministero Dell'ambiente (2010). Italian National Biodiversity Strategy. Available online at: www.minambiente.it (accessed March 11, 2023).
Nair, P. K. R., Viswanath, S., and Lubina, P. A. (2017). Cinderella agroforestry systems. Agroforestry Syst. 91, 901–917. doi: 10.1007/s10457-016-9966-3
Odhiambo, F., Id, O., Boedecker, J., and Kennedy, G. (2019). Exploring agrobiodiversity for nutrition: Household on-farm agrobiodiversity is associated with improved quality of diet of young children in Vihiga, Kenya. PLoS ONE 14, e0219680. doi: 10.1371/journal.pone.0219680
Ortiz, R. (2011). “Agrobiodiversity Management for Climate Change,” in Agrobiodiversity Management for Food Security: A Critical Review, eds. J. M. Lenn'e and D. Wood (Wallingford: CABI), 189–211.
Østerberg, J. T., Xiang, W., Olsen, L. I., Edenbrandt, A. K., Vedel, S. E., Christiansen, A., et al. (2017). Accelerating the domestication of new crops: feasibility and approaches. Trends Plant Sci. 22, 373–384. doi: 10.1016/j.tplants.2017.01.004
Paci-Green, R., and Berardi, G. (2015). Do global food systems have an Achilles heel? The potential for regional food systems to support resilience in regional disasters. J. Environ. Studies Sci. 5, 685–698. doi: 10.1007/s13412-015-0342-9
Petropoulos, S. A., Fernandes, Â., Dias, M. I., Pereira, C., Calhelha, R., di Gioia, F., et al. (2020). Wild and cultivated Centaurea raphanina subsp. mixta: a valuable source of bioactive compounds. Antioxidants 9, 314. doi: 10.3390/antiox9040314
Petropoulos, S. A., Fernandes, Â., Tzortzakis, N., Sokovic, M., Ciric, A., Barros, L., et al. (2019). Bioactive compounds content and antimicrobial activities of wild edible Asteraceae species of the Mediterranean flora under commercial cultivation conditions. Food Res. Int. 119, 859–868. doi: 10.1016/j.foodres.2018.10.069
Pinela, J., Carvalho, A. M., and Ferreira, I. C. F. R. (2017). Wild edible plants: nutritional and toxicological characteristics, retrieval strategies and importance for today's society. Food Chem. Toxicol. 110, 165–188. doi: 10.1016/j.fct.2017.10.020
Porch, T. G., Cichy, K., Wang, W., Brick, M., Beaver, J. S., Santana-Morant, D., et al. (2017). Nutritional composition and cooking characteristics of tepary bean (Phaseolus acutifolius Gray) in comparison with common bean (Phaseolus vulgaris L.). Genet. Resour. Crop Evol. 64, 935–953. doi: 10.1007/s10722-016-0413-0
Qian, H. (1999). Spatial pattern of vascular plant diversity in north america north of mexico and its floristic relationship with Eurasia. Ann. Bot0 83, 271–283. doi: 10.1006/anbo.1998.0816
Sareen, B., Bhattacharya, A., and Srivatsan, V. (2021). Nutritional characterization and chemical composition of Diplazium maximum (D. Don) C. Chr. J. Food Sci. Technol. 58, 844–854. doi: 10.1007/s13197-020-04598-w
Scaramuzzi, S., Gabellini, S., Belletti, G., and Marescotti, A. (2021). Agrobiodiversity-oriented food systems between public policies and private action: A socio-ecological model for sustainable territorial development. Sustainability 13, 192. doi: 10.3390/su132112192
Singh-Peterson, L., and Lawrence, G. (2015). Insights into community vulnerability and resilience following natural disasters: perspectives with food retailers in Northern NSW, Australia. Local Environ. 20, 782–795. doi: 10.1080/13549839.2013.873396
Singla, S., Rana, R., Kumar, S., Thakur, R., and Goyal, S. (2022). Matteuccia struthiopteris (L.) Todaro (fiddlehead fern): an updated review. Bull. Natl. Res. Cent 46, 1–8. doi: 10.1186/s42269-022-00822-z
Smith, G. S., Anjum, E., Francis, C., Deanes, L., and Acey, C. (2022). Climate change, environmental disasters, and health inequities: the underlying role of structural inequalities. Curr. Environ. Health Rep. 9, 80–89. doi: 10.1007/s40572-022-00336-w
Stanek, E. C., Lovell, S. T., and Reisner, A. (2019). Designing multifunctional woody polycultures according to landowner preferences in Central Illinois. Agroforestry Syst. 93, 2293–2311. doi: 10.1007/s10457-019-00350-2
Tamariz, G. (2022). Geoforum Agrobiodiversity conservation with illegal-drug crops: AN approach from the prisons in Oaxaca, Mexico. Geoforum 128, 300–311. doi: 10.1016/j.geoforum.2020.10.012
Tharmabalan, R. T. (2021). Lessons from Japan: promoting underutilized food crops through tourism. Int. J. Food Stu. 10, 311–321. doi: 10.7455/ijfs/10.2.2021.a3
Toon, O. B., Bardeen, C. G., Robock, A., Xia, L., Kristensen, H., McKinzie, M., et al. (2019). Rapidly expanding nuclear arsenals in Pakistan and India portend regional and global catastrophe. Sci. Adv. 5, 1–14. doi: 10.1126/sciadv.aay5478
Toon, O. B., Robock, A., Turco, R. P., Bardeen, C., Oman, L., Stenchikov, G. L., et al. (1979). (2007). Consequences of regional-scale nuclear conflicts. Science 315, 1224–1225. doi: 10.1126/science.1137747
U.S. Department of Agriculture. (2019). FoodData Central. Available online at: fdc.nal.usda.gov
Ugochukwu, I. C. I. (2019). Bracken fern toxicity and its associated clinicopathological effects in humans and animals: a review. Comp. Clin. Path 28, 593–597. doi: 10.1007/s00580-018-2636-2
van Tassel, D. L., Tesdell, O., Schlautman, B., Rubin, M. J., DeHaan, L. R., Crews, T. E., et al. (2020). New food crop domestication in the age of gene editing: genetic, agronomic and cultural change remain co-evolutionarily entangled. Front. Plant Sci. 11, 789. doi: 10.3389/fpls.2020.00789
Veteto, J. R. (2014). Seeds of persistence: agrobiodiversity in the American mountain south. Cult. Agric. Food Environ. 36, 17–27. doi: 10.1111/cuag.12026
Wale, E., Chishakwe, N., and Lewis-Lettington, R. (2009). Cultivating participatory policy processes for genetic resources policy: lessons from the Genetic Resources Policy Initiative (GRPI) project. Biodivers Conserv. 18, 1–18. doi: 10.1007/s10531-008-9444-y
Winstead, D. J., and Jacobson, M. G. (2022). Food resilience in a dark catastrophe: a new way of looking at tropical wild edible plants. Ambio 51, 1949–1962. doi: 10.1007/s13280-022-01715-1
Xia, L., Robock, A., Mills, M., Stenke, A., and Helfand, I. (2015). Decadal reduction of Chinese agriculture after a regional nuclear war. Earths Future 3, 37–48. doi: 10.1002/2014EF000283
Keywords: agrobiodiversity, domestication, global catastrophic risk, nutritional value, United States agriculture, wild edible plants (WEP)
Citation: Winstead DJ, Jacobson MG and Di Gioia F (2023) Valorizing staple Native American food plants as a food resilience resource. Front. Sustain. Food Syst. 7:1117805. doi: 10.3389/fsufs.2023.1117805
Received: 13 December 2022; Accepted: 11 May 2023;
Published: 25 May 2023.
Edited by:
Claudia Fuentealba, Pontificia Universidad Católica de Valparaíso, ChileReviewed by:
Bruno Condori, United States Department of Agriculture (USDA), United StatesEduardo Fernández, University of Bonn, Germany
Copyright © 2023 Winstead, Jacobson and Di Gioia. This is an open-access article distributed under the terms of the Creative Commons Attribution License (CC BY). The use, distribution or reproduction in other forums is permitted, provided the original author(s) and the copyright owner(s) are credited and that the original publication in this journal is cited, in accordance with accepted academic practice. No use, distribution or reproduction is permitted which does not comply with these terms.
*Correspondence: Daniel J. Winstead, djw5623@psu.edu