- 1State Key Laboratory for Managing Biotic and Chemical Threats to the Quality and Safety of Agro-Products, College of Food and Pharmaceutical Sciences, Ningbo University, Ningbo, China
- 2State Key Laboratory for Managing Biotic and Chemical Threats to the Quality and Safety of Agro-products and Institute of Agro-product Safety and Nutrition, Zhejiang Academy of Agricultural Sciences, Hangzhou, Zhejiang, China
- 3College of Veterinary Medicine, Nanjing Agricultural University, Nanjing, Jiangsu Province, China
- 4Institute of Preventive Veterinary Sciences and Department of Veterinary Medicine, Zhejiang University College of Animal Sciences, Hangzhou, China
Introduction: The presence of linezolid-resistant enterococci found in animal-derived food has attracted attention for possible transmission to human-derived enterococci through the food chain. Linezolid-resistant enterococci in farms have been widely reported, but enterococci carrying antimicrobial resistance (AMR) genes poxtA, optrA, or cfr(D) in slaughterhouse environments have not been well addressed.
Methods: Enterococcus was isolated from the samples collected from two slaughterhouses in Hangzhou, and the Enterococcus carrying linezolidin-resistant genes was identified by PCR. The minimum inhibitory concentration (MIC) of the Enterococcus carrying linezolidin-resistant genes was determined by microbroth dilution method. Finally, the whole genome of strains carrying two or more linezoline resistance genes was sequenced using the Oxford Nanopore Technology
Results: Here, 291 enterococci strains were isolated from 309 samples (94.17%). A total of 4 poxtA-positive enterococci and 42 optrA-positive enterococci were identified based on PCR. The antimicrobial susceptibility test showed that the highest rate of florfenicol resistance was 97.82% and the rate of multidrug resistance (MDR) was 95.65%. Two strains carried multiple linezolid resistance genes, among which Enterococcus casseliflavus CQFYY22-063 cocarrying optrA, poxtA, and cfr(D) was isolated from the duck cecum, and Enterococcus faecium CQFYH22-006 cocarrying optrA and poxtA was isolated from slaughterhouse sewage for the first time. Furthermore, Oxford Nanopore Technology revealed that the optrA gene of strain CQFYY22-063 was located on the Inc18-type plasmid pFYY063-optrA-70K, and the poxtA and cfr(D) genes were located on the Inc18-type plasmid pFYY063- poxtA-12K. Meanwhile, the poxtA gene of strain CQFYH22-006 was located on the Rep3-type plasmid pFYH006-poxtA-25K, and the optrA gene was located on the chromosome.
Discussion: Together, linezolid resistance in slaughterhouses deserves extensive attention, indicating the need to strengthen the monitoring of different links in the food production chain within the One Health concept.
1. Introduction
Enterococci are gram-positive bacteria found in the environment and intestines of animals and humans and are a building block of normal microbes (Staley et al., 2014). Some strains of Enterococcus, such as Enterococcus faecium EF1, E. faecium NCIMB 11181, E. faecium NCIMB 10415, E. faecium SF68, E. faecium M-74 and E. faecium NRRL-B 2354 are used as probiotics and feed additives to prevent diarrhea or to improve growth in animals (Franz et al., 2011; Hanchi et al., 2018; Ahmad et al., 2022; Shao et al., 2022). But part of the E. faecium and Enterococcus faecalis are important pathogens that cause human infections, often causing diseases such as urinary and soft tissue infections, septicemia or meningitis (Bender et al., 2018; Park et al., 2020). Notably, E. faecium is on the global list of priority pathogens called ESKAPE (Tacconelli et al., 2018). Antimicrobial resistance (AMR) is recognized as a global public health crisis that urgently needs to be addressed (Li et al., 2017; Guan et al., 2022; Lin et al., 2022; Ma et al., 2022; Tang et al., 2022c). Enterococcus has inherent resistance to cephalosporins, anti-staphylococcal penicillin, amtrannan, aminoglycosides, lincoamides, and streptin (Golob et al., 2019). In addition, antimicrobial resistance genes (ARGs) obtained from other sources are transferred and exchanged under different conditions, leading to the emergence of multiple AMR in Enterococcus, which increases the prevalence of Enterococcus and increases the cost of treatment (Cui et al., 2016; Hao et al., 2019).
Linezolid, the first oxazolidone drug to be approved for sale, has attracted global attention because of its importance as an antibacterial agent of last resort for gram-positive strains (Bender et al., 2018). With the increasing frequency of linezolid use in the clinic, linezolid-resistant Enterococcus gradually appeared (Schwarz et al., 2021; Xu et al., 2022). Linezolid resistance can be associated with a point mutation in the 23S rRNA gene or a mobile cfr, optrA, or poxtA resistance gene on the plasmid (Antonelli et al., 2018; Wang et al., 2020). Transferable linezolid resistance genes, including optrA, optrA, and cfr, have been detected in many different species of Enterococcus and from different animal sources around the world (Sadowy, 2018; Schwarz et al., 2021). Many studies have shown that most Enterococcus (93.3%) exhibit a MDR phenotype (Hu et al., 2019). In addition, more Enterococcus carrying poxtA were found in environmental samples than in clinical samples, and the prevalence of E. faecium was higher than that of Enterococcus faecalis (Hao et al., 2019; Huang et al., 2019). The optrA gene was found to be more common in Enterococcus isolated from livestock than in Enterococcus isolated from humans (Yang et al., 2015), the detection rate of the optrA gene was 2.0%, and the positive rate of the optrA gene in the clinic increased from 0.4% in 2004 to 3.9% in 2014 (Cui et al., 2016; Liu et al., 2020). The presence of plasmids carrying ARGs indicated that Enterococcus isolates could transfer between them and promote the further spread of linezolid resistance genes (Cinthi et al., 2022b). Meanwhile, the presence of other ARGs on the plasmids, such as fexA and erm(A), may contribute to the persistence of linezolid resistance genes (Tang et al., 2020, 2021; Huang et al., 2022). With extensive studies on linezolid resistance genes, various variants have gradually emerged, such as cfr(D), cfr(B), poxtA2, and optrA, suggesting that further monitoring of enterococcal resistance is needed (Saavedra et al., 2020; Cinthi et al., 2022a).
The complete genome sequence can effectively analyze the genome characteristics, ARGs, plasmids, and other elements (Wang et al., 2013; Zhu et al., 2015; Peng et al., 2017), and has been an essential means of antimicrobial resistance research in recent years (Tang et al., 2022a,b). The ARGs predicted by the genome have a corresponding good relationship with the AMR phenotype (Zheng et al., 2022; Zhou et al., 2022; Li et al., 2022b; Tang et al., 2022b).
Linezolid resistance gene-mediated enterococcal resistance is widely found in various food animals, among which pigs and chickens are more studied (Osman et al., 2019; Schwarz et al., 2021; Xu et al., 2022). Few studies have been conducted in slaughterhouses and their environment. Enterococcus isolates from slaughterhouses and the environment are often multidrug-resistant (MDR) and carry essential risk-resistant genes. It has been reported that most of the Enterococcus strains isolated from duck slaughterhouses in Chengdu, China were MDR (90.3%), of which the optrA gene (90.7%) was commonly observed (Li et al., 2022a). According to Na′s report, 35.9% of Enterococcus isolates from duck feces and carcasses from four slaughterhouses in southern Korea were MDR, 2.3% of which harbor the optrA gene (Na et al., 2019). Yu et al. reported that 72.13% of Enterococcus isolates from broilers slaughterhouses in Tai’an, China, were MDR, with 18.03% being linezolid-resistant (Yu et al., 2022a). Notably, a vancomycin-resistant E. faecium was isolated from a transport crate in a chicken slaughterhouse in Germany (Savin et al., 2020). Previous reports indicate that Enterococcus is widespread in slaughterhouses and their environment, posing a potential threat to public health safety. This study focused on monitoring the presence and transmission mechanism of linezolid resistance genes in slaughterhouses and their environment and evaluated the transferability of plasmids carrying optrA and poxtA resistance genes to E. faecalis, providing a solid data reference for theoretical research on AMR.
2. Materials and methods
2.1. Sample acquisition
From May to September 2022, 96 duck cecum samples and 96 environmental samples were collected from a duck slaughterhouse in Fuyang, Zhejiang Province, China, and 33 pig carcass samples and 87 environmental samples were collected from a pig slaughterhouse in Xiaoshan, Zhejiang Province, China. Slaughterhouse sewage was dipped into a 2-ml tube with a sterile cotton swab (HUABAO, Ningbo). Then, all samples were placed at 2–8°C and shipped back to the laboratory for sample pretreatment within 24 h after collection.
2.2. Isolation and identification of enterococci
Samples were mixed with 5 ml of buffered protein water (Landbridge, Beijing) in sterile tubes and incubated at 37°C for 12–18 h. After incubation, each sample (10 μl) was inoculated on Enterococcus chromogenic medium (Landbridge, Beijing) and incubated at 37°C for 24–48 h. Purple single colonies were picked on subscoring Enterococcus chromogenic plates with Brian Heart Infusion (Landbridge, Beijing) plates and finally identified using MALDI-TOF MS (Bruker, Germany).
2.3. Screening of linezolid resistance genes
PCR screening of all isolated enterococci was performed according to the primers of previously reported linezolid resistance genes optrA, poxtA, and cfr (Supplementary Table S1).
2.4. Antimicrobial susceptibility testing
The microbroth dilution method recommended in the American Committee for Clinical and Laboratory Standards Institute (CLSI) document M100-S27 was used to determine the minimum inhibitory concentration (MIC) of Enterococcus to 18 antibiotics (CLSI, 2017; Humphries et al., 2021), including penicillin (PEN), amoxicillin/clavulanate (A/C), erythromycin (ERY), clindamycin (CLI), enrofloxacin (ENR), ofloxacin (OFL), ceftiofur (CEF), cefoxitin (CFX), sulfisoxazole (SF), oxacillin (OXA), vancomycin (VAN), trimethoprim/sulfamethoxazole (SXT), doxycycline (DOX), florfenicol (FFC), tiamulin (TIA), tilmicosin (TIL), gentamicin (GEN), and linezolid (LZD). These antibiotics were purchased from Fosun Diagnostics, Shanghai, China. Enterococcus faecalis ATCC 29212 was used as a quality control strain.
2.5. Whole genome sequencing and analysis
Single colonies were selected on BHI plates and inoculated in 5 ml of BHI broth at 37°C with shaking at 220 rpm for 6–7 h. Then, the bacteria were collected with centrifugation at 9,000 rpm for 2 min, and the genome was extracted using a DNA extraction kit (Generay, Shanghai). Strains carrying one of the linezolid resistant genes were sequenced using Illumina Nova sequencing and assembled using CLC Genomics Workbench 12.0. Strains harboring two or more linezolid-resistant genes were further sequenced using the Oxford Nanopore GridION platform and assembled with Unicycler v0.4.4 (Wick et al., 2017). Easyfig 2.2.3 (Sullivan et al., 2011) was used to compare the gene environments, and BRIG was used to map the circles of the plasmids for comparison (Alikhan et al., 2011). Plasmid types were analyzed using the Center for Genomic Epidemiology (CGE, http://www.genomicepidemiology.org/). Finally, heatmaps were generated by TBtools v1.0 (Chen et al., 2020a).
2.6. Statistical analysis
The Chi-square test was used to analyze whether there were differences in Enterococcus isolation rates among different sources. A probability (P) value<0.05 was considered statistically significant.
3. Results
3.1. Isolation of strains
A total of 291 Enterococcus strains were isolated from 309 samples, with an overall isolation rate of 94.17% (291/309; Table 1). Among them, 104 Enterococcus strains were isolated (88.89%, 104/117), 33 Enterococcus strains were isolated from pig carcass samples, and 71 Enterococcus strains were isolated from environmental samples. A total of 187 strains of Enterococcus were isolated from the Fuyang duck slaughterhouse, with an isolation rate of 97.39% (187/192). Ninety-five Enterococcus strains were isolated from duck cecum samples, and 92 Enterococcus strains were isolated from environmental samples. The isolation rate of Enterococcus in the pig slaughterhouse was significantly lower than that in the duck slaughterhouse, and significant difference was found between them by chi-square test (p < 0.05).
3.2. Screening of linezolid resistance genes
A total of 4 poxtA-positive enterococcal strains were screened out of 291 enterococcal strains, among which 2 strains were from pig slaughterhouses and 2 strains were from duck slaughterhouses, with a positive rate of 1.72% (4/291). There were 42 optrA-positive enterococci strains, of which 18 were from pig slaughterhouses and 24 were from duck slaughterhouses, with a positivity rate of 16.16% (42/291). No cfr-positive enterococci were screened.
The 46 strains of Enterococcus that carried linezolid resistance genes belonged to six species: E. faecalis (39.13%, n = 18), E. faecium (30.43%, n = 14), Enterococcus casseliflavus (13.04%, n = 6), Enterococcus hirae (6.52%, n = 3), Enterococcus mundtii (2.17%, n = 1), and Enterococcus gallinarum (8.70%, n = 4). These included 2 Enterococcus strains, CQFYY22-063 and CQFYH22-006, from a duck slaughterhouse belonging to poxtA and optrA co-occurring enterococci.
3.3. Antimicrobial susceptibility testing of enterococci carrying linezolid resistance genes
Thirty-three strains were resistant to linezolid, including 13 E. faecalis strains, 11 E. faecium, 4 strains of E. casseliflavus, 3 strains of E. gallinarum, and 1 strain each of E. hirae and E. mundtii (Figure 1A). Antimicrobial susceptibility testing of 46 Enterococcus isolates by microbroth dilution is shown in Figure 1B and Supplementary Figure S1.
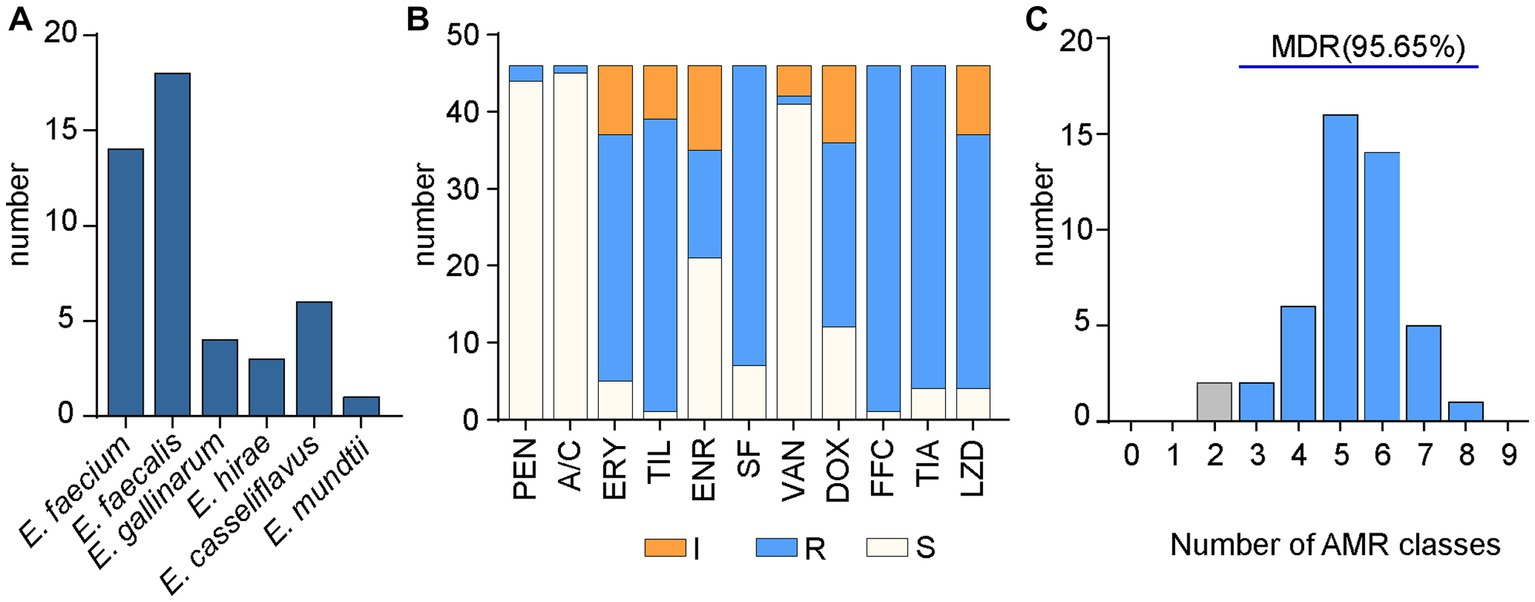
Figure 1. Species and AMR of Enterococcus isolates in this study. (A) Types of Enterococcus isolates. (B) Resistance of Enterococcus to 11 antibiotics. “S” for susceptibility, “I” for mediation, and “R” for resistance. (C) The number of Enterococcus strains resistant to 9 classes of antibiotics and the proportion of MDR strains.
In this study, 33 enterococci strains were found to be resistant to linezolid, with linezolid MIC ranged from 8 ~ 16 μg/ml (Supplementary Figure S1). Enterococci were classified into 24 different AMR subtypes, of which ERY-SF-DOX-TIA-TIL-FFC-LZD accounted for the largest proportion (23.91%) and were resistant to seven antibiotics. This was followed by ERY-SF-DOX-TIA-TIL-FFC (10.87%; Table 2). Of these, 95.65% were MDR (resistant to three or more antibiotics), mainly to chloramphenicol, tetracyclines and macrolides (Figure 1C).
3.4. Molecular characterization of optrA and poxtA-positive isolates
Whole genome sequencing was performed on 42 optrA-positive strains and 4 poxtA-positive strains. There were 35 ARGs for 9 classes of antibiotics detected in all optrA-positive or poxtA-positive strains. Among them, aminoglycosides had the most resistance genes, with 8. Moreover, there were 2 linezolid genes (optrA and poxtA), seven lincosamide genes [lsa(A), lsa(E), lnu(A), lnu(B), lnu(C), lnu(D), and lnu(G)], three amphenicol genes [fexA, fexB, cat, cfr(D), and cat(pC221)], one folate pathway antagonist genes (dfrG), 5 macrolides gene [erm(A), erm(B), mef(A), msr(C) and msr(D)], 2 glycopeptide genes (vanC1XY and vanC2XY), 1 heat gene (clpL) and 2 tetracycline genes [tet(L) and tet(M); Figure 2]. Meanwhile, plasmid replicons include 6 types of 18 different types, namely, Inc18 type (rep1, rep2, repUS1 and repUS11), Rep1 type (rep22), Rep2 type (repUS52), Rep3 type (rep4a, rep27, rep29, rep33), Rep_trans type (rep7a, rep14b, repUS43), and RepA_N type (rep8b, rep9a, rep9b, rep9c, repUS15; Figure 3B). The virulence genes were elrA, srtA, ace, agg, cCF10, cOB1, cad, camE, ebpA, ebpB, ebpC (biofilm production), efaAfs (cell wall adhesion expressed in serum), efaAfm, fsrB, gelE, chylA (hyaluronidase gene), chylB, tpx (antioxidative stress protection), cylA, cylL, cylM, and acm (Figure 3A).
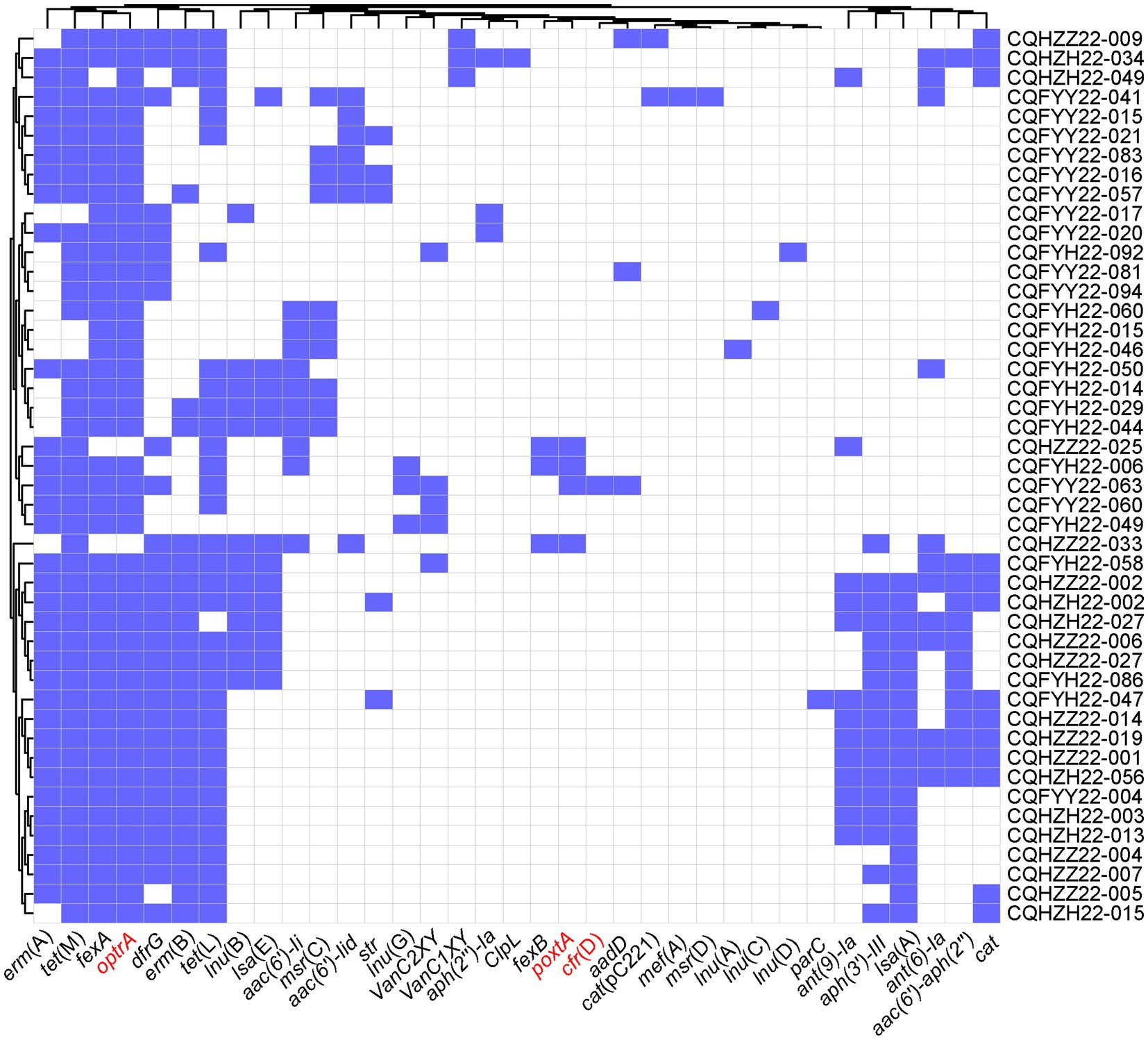
Figure 2. Acquired ARGs in Enterococcus. Lilac indicates the AMR gene, and white represents the absence of the AMR gene.
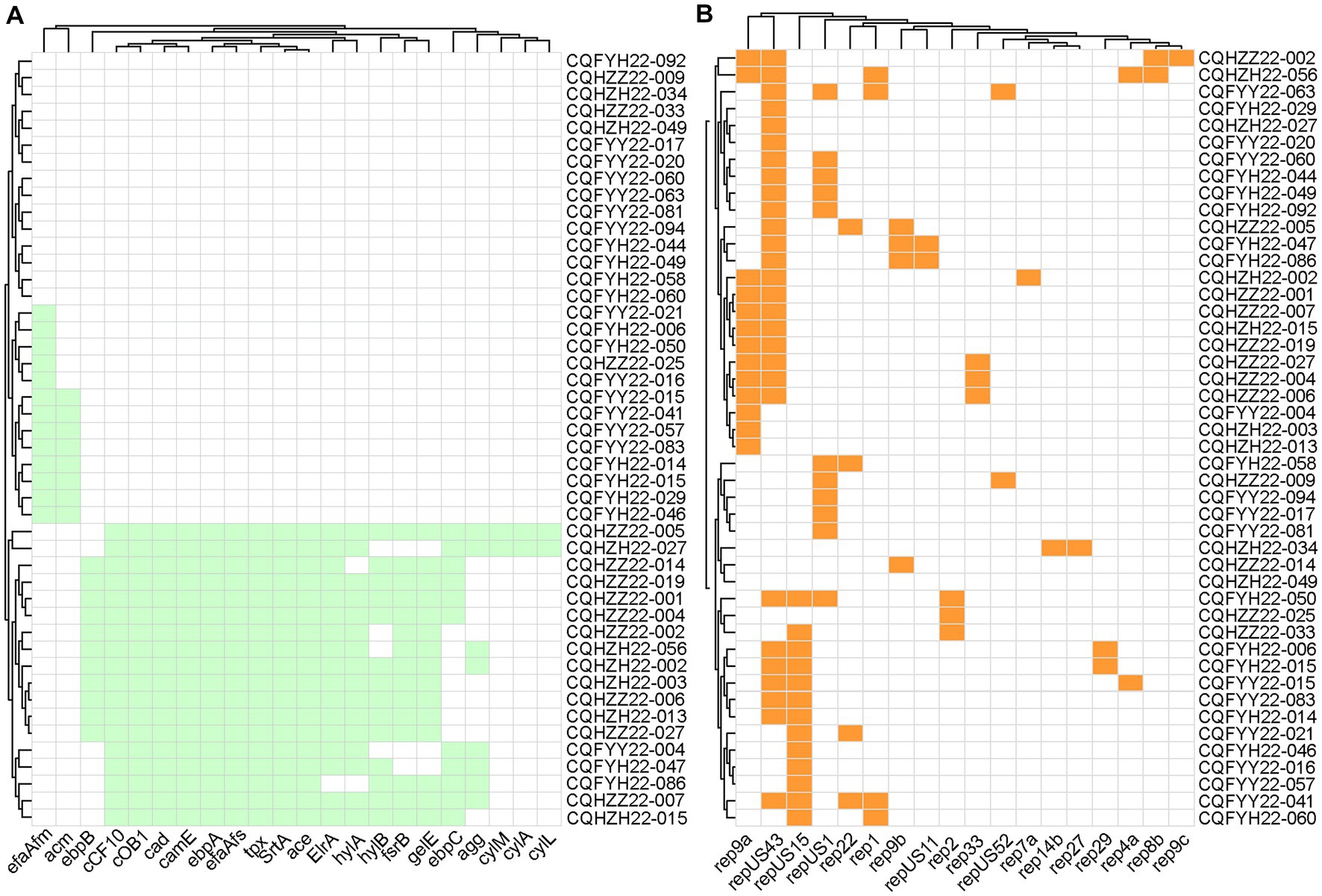
Figure 3. (A) Virulence gene in Enterococcus. Aqua represents the presence of the virulence gene, and white represents the absence of the virulence gene. (B) Plasmid replicon type in Enterococcus. Orange represents the plasmid replicon type, and white represents the absence of the plasmid replicon type.
3.5. Genetic environment of optrA and poxtA copositive isolates
Owing to the presence of both optrA and poxtA genes in strains CQFYH22-006 and CQFYY22-063, they were selected for nanopore sequencing, and their complete genome sequences were obtained. The optrA gene in strain CQFYY22-063 is located on pFYY063-optrA-70K (CP116030). pFYY063-optrA-70K was 70,094 bp in length with 35% GC content (Figure 4A). pFYY063-optrA-70K belonged to the Inc18-type plasmid, and by blastn, pFYY063-optrA-70K was less similar to other plasmids carrying optrA, with pT17-1-optrA-57k (CP109840) having the highest similarity (63% query coverage and 97.90% homology). The overall backbone of pFYY063-optrA-70K was found to be similar to that of pT17-1-optrA-57k by colinear comparison, but there were differences in the 18,084 bp-sized region of pFYY063-optrA-70K, mainly composed of the proteins “parA-padR-ISL3-spaH-spaA- prgN,” which is consistent with the structure of p_unnamed2 (CP060722; Figure 4B).
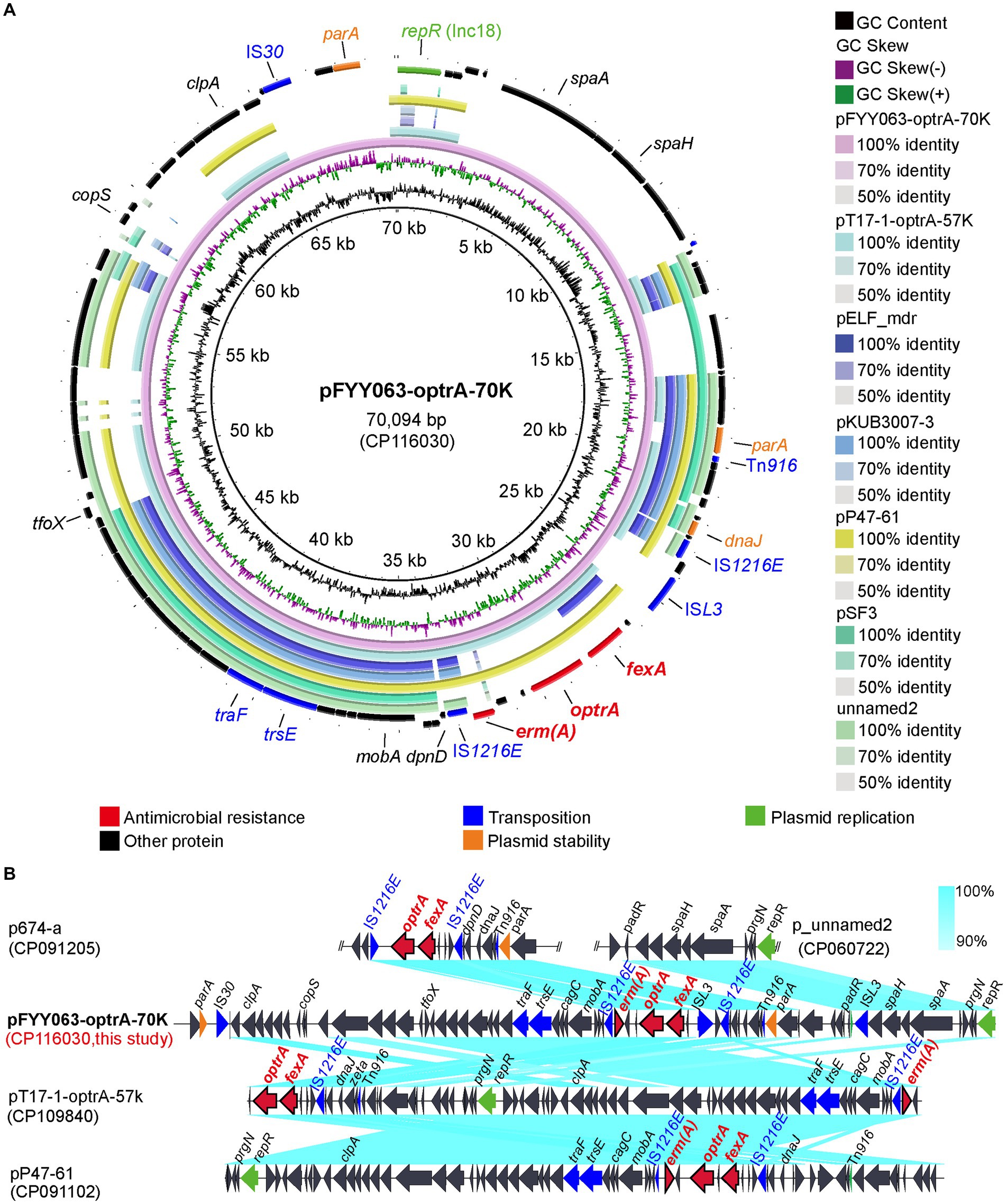
Figure 4. Sequence alignment of plasmid pFYY063-optrA-70K and the gene–environment of optrA gene. (A) Comparison of the circular plasmid sequence between plasmids pFYY063-optrA-70K, pT17-1-optrA-57K, pELF_mdr, pKUB3007-3, pP47-61, pSF3 and unnamed2 of Enterococcus strains. (B) Linear comparison of the draft genome sequences of Enterococcus pFYY063-optrA-70 and Enterococcus pT17-1-optrA-57K, pP47-61, p674-a and p_ unnamed2. Open arrows indicate coding sequences (red arrows, ARGs; green arrows, plasmid replication; blue arrows, transfer and transfer-related sequences; gray arrows, hypothetical and unclassified) and indicate the direction of transcription.
The poxtA gene of strain CQFYY22-063 is located on pFYY063-poxtA-12 K (CP116031). pFYY063-poxtA-12K is 12,478 bp in length and has a GC content of 36% (Figure 5A). pFYY063-poxtA-12K belongs to the Inc18-type plasmid, while the florfenicol resistance gene fexA and the linezolid resistance gene cfr(D) are also present in pFYY063-poxtA-12K. The blastn results demonstrated that pFYY063-poxtA-12K was highly similar to other plasmids carrying poxtA, with pQZ076-4 (CP098029) having high similarity (99% query coverage and 99.98% homology). A colinear comparison suggested the presence of a transfer element IS1216E on the right side of poxtA and cfr(D) and two transfer elements IS1216E in the same direction on both sides of fexA (Figure 5B).
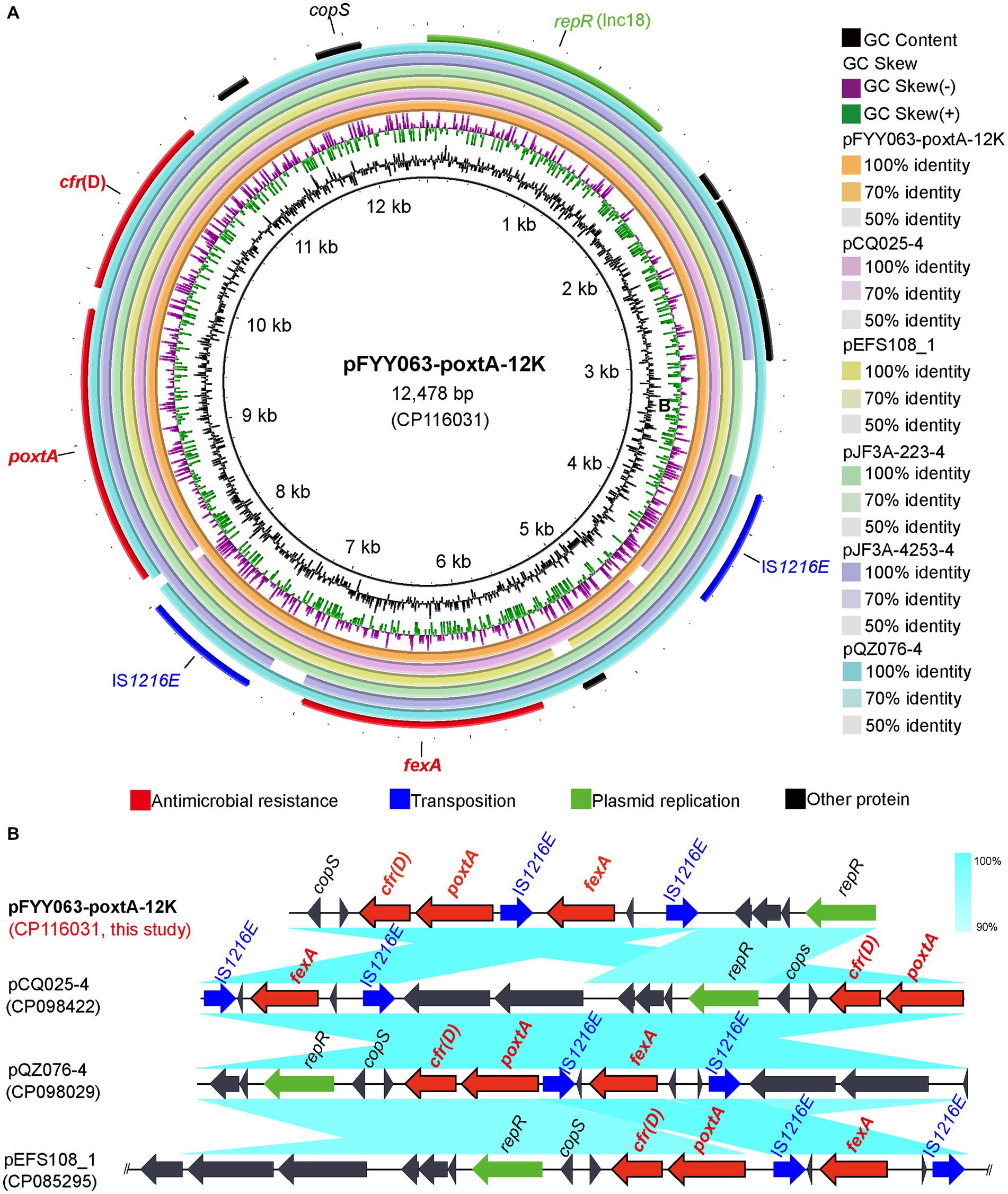
Figure 5. Sequence alignment of plasmid pFYY063-poxtA-12K and the gene–environment of poxtA. (A) Comparison of the circular plasmid sequence between plasmids pFYY063-poxtA-12K, pCQ025-4, pEFS108-1, pJF3A-223-4, pJF3A-2,453-4, and pQZ076-4 of Enterococcus strains. (B) Linear comparison of the draft genome sequences of Enterococcus pFYY063-poxtA-12K and Enterococcus pCQ025-4, pQZ076-4 and pEFS108-1. Open arrows indicate coding sequences (red arrows, ARGs; green arrows, plasmid replication; blue arrows, transfer and transfer-related sequences; gray arrows, hypothetical and unclassified) and the direction of transcription.
The poxtA gene of strain CQFYH22-006 is located on pFYH006-poxtA-25 K (CP116025). pFYH006-poxtA-25K is 25,261 bp in length and has a GC content of 35% (Figure 6A). pFYH006-poxtA-25K is a Rep3-type plasmid. Blastn analysis showed that pFYH006-poxtA-25K is highly similar to other plasmids carrying poxtA, and colinear comparison suggests the presence of an identically oriented transfer element IS1216E on each side of poxtA (Figure 6B).
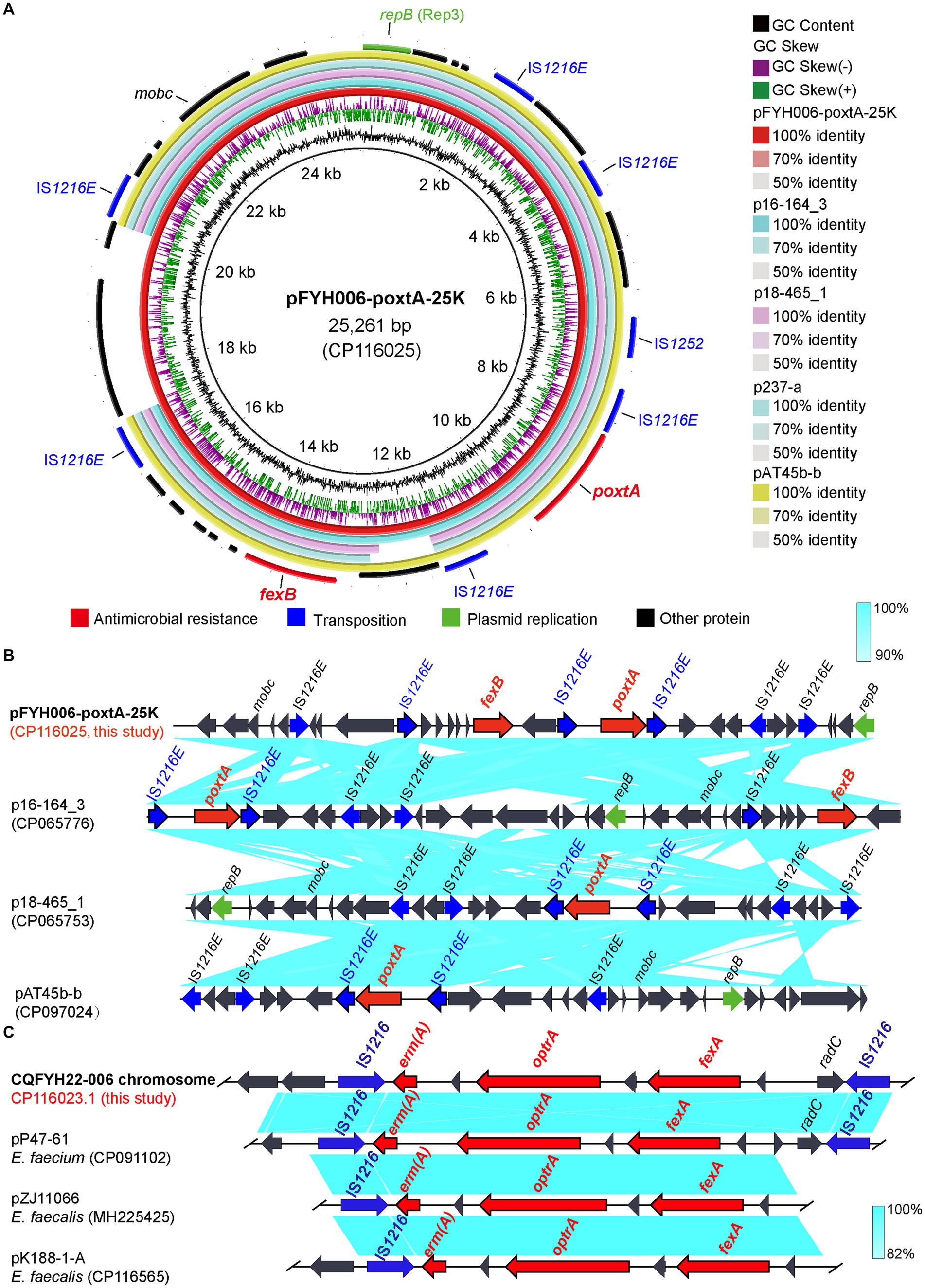
Figure 6. Sequence alignment of plasmid pFYH006-poxtA-25K and the gene–environment of poxtA. (A) Comparison of the circular plasmid sequence between plasmids pFYH006-poxtA-25K, pCQ025-4, pEFS108-1, pJF3A-223-4, pJF3A-2,453-4, and pQZ076-4 of Enterococcus strains. (B) Linear comparison of the draft genome sequences of Enterococcus pFYH006-poxtA-25K and Enterococcus pCQ025-4, pQZ076-4 and pEFS108-1. Open arrows indicate coding sequences (red arrows, ARGs; green arrows, plasmid replication; blue arrows, transfer and transfer-related sequences; gray arrows, hypothetical and unclassified) and the direction of transcription. (C) The genetic environment of optrA on chromosomes. Linear comparison of optrA on Enterococcus chromosomes with draft genome sequences of Enterococcus pP47-61, pZJ11066 and pK188-1-A. Open arrows indicate coding sequences (red arrows, ARGs; green arrows, plasmid replication; blue arrows, transfer and transfer-related sequences; gray arrows, hypothetical and unclassified) and the direction of transcription.
The optrA gene of strain CQFYH22-006 was located on the chromosome, and it also carried the ARGs emr(A) and fexA. The total length of the chromosome was 2,506,224 bp. The selection of regions, including ARGs optrA, emr(A), and fexA for Blastn, was found to be highly similar to that of the E. faecium plasmid pP47-61 (CP091102.1). Colinear comparison showed that optrA, emr(A), and fexA had two opposite transfer elements IS1216 on both sides, and this genetic environment was consistent with pP47-61 (Figure 6C). Therefore, this region in the chromosome might be propagated from the plasmid.
4. Discussion
Enterococci are the main pathogens causing infections in humans and animals (Pillay et al., 2018). Linezolid is considered one of the last lines of defense against methicillin-resistant Staphylococcus aureus and VAN-resistant enterococci. However, in recent years, an increasing number of farm animals, humans and the environment around the world have been found to carry multiple linezolid AMR (Chen et al., 2020c; Biggel et al., 2021; Coccitto et al., 2022). Enterococci carrying linezolid resistance genes may pose a potentially serious threat to the health care system and breeding industry (Chen et al., 2020b). Although linezolid is not used in food animals, isolates with linezolid resistance have been reported in China (Lei et al., 2021), Korea (Yoon et al., 2020), the United States (Tyson et al., 2018b), Spain (Ruiz-Ripa et al., 2020) and Tunisia (Elghaieb et al., 2019) from edible meat, animal manure and farming environments. Meanwhile, enterococci carrying poxtA–optrA or optrA-cfr genes from swine, human, water and environmental samples have been reported in many countries (Moure et al., 2020; Ruiz-Ripa et al., 2020; Biggel et al., 2021; Nüesch-Inderbinen et al., 2022a). The production chain for livestock and poultry includes the slaughtering process, which is crucial in lowering the prevalence of enterococci. In order to ensure the safety of livestock and poultry products, it is necessary to understand the distribution characteristics of enterococci and ARGs in livestock and poultry slaughterhouses.
In this study, 291 enterococci strains were isolated from two slaughterhouses in Zhejiang Province, with a total isolation rate of 94.17%. The detection rate of optrA was 14.43% (42/291), which was lower than the 29.69% (19/64) detection rate reported from Henan Province (Wang et al., 2015). This was much higher than the detection rate of optrA reported from 25 large pig farms in Sichuan Province (6/158; 3.80%; Kang et al., 2019). The detection rate of poxtA was 1.37% (4/291) lower than the 57.89% (66/114) isolated from two pig farms in Henan Province, China, in 2018 (Hao et al., 2019) and lower than the positive rate in Italian pig farms 4.14% (6/145; Fioriti et al., 2020). To date, there are few reports on the isolation of E. casseliflavus from slaughterhouses carrying both optrA and poxtA. In this study, for the first time, E. casseliflavus CQFYY22-063 carrying optrA, poxtA and cfr(D) was isolated from duck cecum (in slaughterhouse), and E. faecium CQFYH22-006 carrying optrA and poxtA was isolated from slaughterhouse sewage.
The phenotype and genotype of lincomycin resistance in enterococci are often not consistent. Rodríguez-Lucas et al. isolated an enterococcus carrying the optrA gene from community patients, but it was not resistant to linezolid (Rodríguez-Lucas et al., 2022). Moreover, Nüesch-Inderbinen et al. also reported a pig-derived isolate of E. faecalis carrying the poxtA2 and cfr(D) genes but susceptive to linezolid (Nüesch-Inderbinen et al., 2022b). Similar results were also found in this study, 13 Enterococcus strains carrying optrA or poxtA genes were not resistant to linezolid, and the MIC of linezolid was 2–4 μg/ml.
95.65% (44/46) of strains belonged to MDR strains, similar to the MDR rate of enterococci isolated from Polish marketed animal foods (Zarzecka et al., 2022). Among them, the resistance rates of tilmicosin, sulfisoxazole, tiamulin, florfenicol and linezolid were all above 70%, which was much higher than the resistance rate of optrA- or poxtA-positive enterococci isolated from retail meat and food-producing animals in Tunisian (Elghaieb et al., 2019). High levels of resistance to tetracyclines, macrolides, and florfenicol, as described above, have also been found in pigs and chickens from Korea (Kwon et al., 2012), the United States (Tyson et al., 2018a), and European countries (de Jong et al., 2018). The widespread prevalence of MDR Enterococcus strains cannot be ignored, so further research on the internal factors mediating the widespread prevalence of Enterococcus is necessary.
Mobile genetic elements play an important role in the transmission of ARGs. Plasmids, IS1216E and Tn554 (Murphy et al., 1981), can promote the horizontal transmission of optrA (Yu et al., 2022b). Both clonal transmission and horizontal transfer mediated by the Inc18 plasmid and IS1216E contributed to the spread of poxtA in Enterococcus isolates (Lei et al., 2021). It has been reported that IS1216E-mediated translocation and translocation processes promote the spread and persistence of poxtA in Enterococcus (Shan et al., 2020) and that two parallel oriented IS1216E elements on either side have previously been shown to be responsible for horizontal gene transfer of poxtA (Egan et al., 2020). The presence of other ARGs (fexA, fexB) may lead to coselection of optrA and poxtA. Càmara et al. (2019) reported a high frequency of optrA and fexA in the same isolate. Furthermore, cotransfer of the fexA gene with optrA in E. faecalis has been demonstrated in many countries, such as China (Wang et al., 2015), Spain (Càmara et al., 2019) and the United States(Wardenburg et al., 2019). In this study, strains also carried the fexA gene adjacent to optrA or the fexA gene adjacent to poxtA. In addition, plasmids carrying poxtA genes also cocarry other ARGs, such as cfr(D). These results suggest that the poxtA and cfr(D) genes may be transmitted between strains via IS1216E.
5. Conclusion
In this study, 291 strains of Enterococcus were isolated from two slaughterhouses, and 4 poxtA-positive Enterococcus strains and 42 optrA-positive Enterococcus strains were identified. E. faecium cocarrying poxtA and optrA genes was isolated from duck slaughterhouse sewage. In addition, E. casseliflavus carrying optrA, poxtA and cfr(D) was isolated from a duck cecum for the first time. This study also proves that optrA or poxtA genes are widespread in food animals and the slaughter environment. Animal slaughterhouses may act as reservoirs of transferable oxazolidone resistance genes, which can be transmitted through the food chain to humans and would significantly limit treatment of MDR bacteria. Therefore, the prevalence and spread of poxtA and optrA in Enterococcus in animal slaughterhouses should be continuously monitored to reduce potential public health threats.
Data availability statement
The complete genome sequences of strains CQFYH22-006 and CQFYY22-063 were deposited at GenBank under accession numbers CP116023-CP116025 and CP116026-CP116031, respectively.
Author contributions
BT and DP: conceptualization and supervision. BT: funding acquisition. JN, XL, MW, WW, JM, YS, MY, and HY: investigation. JN, XL, WW, and BT: methodology. JN, XL, and MW: visualization. JN, BT, XL, and MW: writing—original draft. All authors contributed to the article and approved the submitted version.
Funding
This work was supported by the Zhejiang Provincial Natural Science Foundation of China (LY23C180001), the “Leading Goose” R&D Program of Zhejiang Province (2023C03045), and the Collaborative Extension Plan of Major Agricultural Technologies in Zhejiang Province (2021XTTGXM03), and the China Agriculture Research System of MOF and MARA (CARS-42-27).
Conflict of interest
The authors declare that the research was conducted in the absence of any commercial or financial relationships that could be construed as a potential conflict of interest.
Publisher’s note
All claims expressed in this article are solely those of the authors and do not necessarily represent those of their affiliated organizations, or those of the publisher, the editors and the reviewers. Any product that may be evaluated in this article, or claim that may be made by its manufacturer, is not guaranteed or endorsed by the publisher.
Supplementary material
The Supplementary material for this article can be found online at: https://www.frontiersin.org/articles/10.3389/fsufs.2023.1179078/full#supplementary-material
References
Ahmad, N. H., Hildebrandt, I. M., Pickens, S. R., Vasquez, S., Jin, Y., Liu, S., et al. (2022). Interlaboratory evaluation of Enterococcus faecium NRRL B-2354 as a Salmonella surrogate for validating thermal treatment of multiple low-moisture foods. J. Food Prot. 85, 1538–1552. doi: 10.4315/jfp-22-054
Alikhan, N. F., Petty, N. K., Ben Zakour, N. L., and Beatson, S. A. (2011). BLAST ring image generator (BRIG): simple prokaryote genome comparisons. BMC Genomics 12:402. doi: 10.1186/1471-2164-12-402
Antonelli, A., D'Andrea, M. M., Brenciani, A., Galeotti, C. L., Morroni, G., Pollini, S., et al. (2018). Characterization of poxtA, a novel phenicol-oxazolidinone-tetracycline resistance gene from an MRSA of clinical origin. J. Antimicrob. Chemother. 73, 1763–1769. doi: 10.1093/jac/dky088
Bender, J. K., Cattoir, V., Hegstad, K., Sadowy, E., Coque, T. M., Westh, H., et al. (2018). Update on prevalence and mechanisms of resistance to linezolid, tigecycline and daptomycin in enterococci in Europe: towards a common nomenclature. Drug Resist. Updat. 40, 25–39. doi: 10.1016/j.drup.2018.10.002
Biggel, M., Nüesch-Inderbinen, M., Jans, C., Stevens, M. J. A., and Stephan, R. (2021). Genetic context of optrA and poxtA in Florfenicol-resistant enterococci isolated from flowing surface water in Switzerland. Antimicrob. Agents Chemother. 65:e0108321. doi: 10.1128/aac.01083-21
Càmara, J., Camoez, M., Tubau, F., Pujol, M., Ayats, J., Ardanuy, C., et al. (2019). Detection of the novel optrA gene among linezolid-resistant enterococci in Barcelona, Spain. Microb. Drug Resist. 25, 87–93. doi: 10.1089/mdr.2018.0028
Chen, C., Chen, H., Zhang, Y., Thomas, H. R., Frank, M. H., He, Y., et al. (2020a). TBtools: an integrative toolkit developed for interactive analyses of big biological data. Mol. Plant 13, 1194–1202. doi: 10.1016/j.molp.2020.06.009
Chen, L., Han, D., Tang, Z., Hao, J., Xiong, W., and Zeng, Z. (2020b). Co-existence of the oxazolidinone resistance genes cfr and optrA on two transferable multi-resistance plasmids in one Enterococcus faecalis isolate from swine. Int. J. Antimicrob. Agents 56:105993. doi: 10.1016/j.ijantimicag.2020.105993
Chen, Q., Yin, D., Li, P., Guo, Y., Ming, D., Lin, Y., et al. (2020c). First report cfr and optrA co-harboring linezolid-resistant Enterococcus faecalis in China. Infect. Drug Resist. 13, 3919–3922. doi: 10.2147/idr.S270701
Cinthi, M., Coccitto, S. N., D'Achille, G., Morroni, G., Simoni, S., Fioriti, S., et al. (2022a). Characterization of a novel cfr(D)/poxtA-carrying plasmid in an oxazolidinone-resistant Enterococcus casseliflavus isolate from swine manure, Italy. J. Glob. Antimicrob. Resist. 30, 308–310. doi: 10.1016/j.jgar.2022.07.007
Cinthi, M., Coccitto, S. N., Fioriti, S., Morroni, G., Simoni, S., Vignaroli, C., et al. (2022b). Occurrence of a plasmid co-carrying cfr(D) and poxtA2 linezolid resistance genes in Enterococcus faecalis and Enterococcus casseliflavus from porcine manure, Italy. J. Antimicrob. Chemother. 77, 598–603. doi: 10.1093/jac/dkab456
CLSI (2017). “Clinical and laboratory standards institute” in Performance standards for antimicrobial susceptibility testing; twenty-seventh informational supplement M100-S27 (Wayne, PA, USA: CLSI).
Coccitto, S. N., Cinthi, M., Fioriti, S., Morroni, G., Simoni, S., Vignaroli, C., et al. (2022). Linezolid-resistant Enterococcus gallinarum isolate of swine origin carrying cfr, optrA and poxtA genes. J. Antimicrob. Chemother. 77, 331–337. doi: 10.1093/jac/dkab408
Cui, L., Wang, Y., Lv, Y., Wang, S., Song, Y., Li, Y., et al. (2016). Nationwide surveillance of novel oxazolidinone resistance gene optrA in Enterococcus isolates in China from 2004 to 2014. Antimicrob. Agents Chemother. 60, 7490–7493. doi: 10.1128/aac.01256-16
de Jong, A., Simjee, S., Garch, F. E., Moyaert, H., Rose, M., Youala, M., et al. (2018). Antimicrobial susceptibility of enterococci recovered from healthy cattle, pigs and chickens in nine EU countries (EASSA study) to critically important antibiotics. Vet. Microbiol. 216, 168–175. doi: 10.1016/j.vetmic.2018.02.010
Egan, S. A., Shore, A. C., O'Connell, B., Brennan, G. I., and Coleman, D. C. (2020). Linezolid resistance in Enterococcus faecium and Enterococcus faecalis from hospitalized patients in Ireland: high prevalence of the MDR genes optrA and poxtA in isolates with diverse genetic backgrounds. J. Antimicrob. Chemother. 75, 1704–1711. doi: 10.1093/jac/dkaa075
Elghaieb, H., Freitas, A. R., Abbassi, M. S., Novais, C., Zouari, M., Hassen, A., et al. (2019). Dispersal of linezolid-resistant enterococci carrying poxtA or optrA in retail meat and food-producing animals from Tunisia. J. Antimicrob. Chemother. 74, 2865–2869. doi: 10.1093/jac/dkz263
Fioriti, S., Morroni, G., Coccitto, S. N., Brenciani, A., Antonelli, A., Di Pilato, V., et al. (2020). Detection of oxazolidinone resistance genes and characterization of genetic environments in enterococci of swine origin, Italy. Microorganisms 8:2021. doi: 10.3390/microorganisms8122021
Franz, C. M., Huch, M., Abriouel, H., Holzapfel, W., and Gálvez, A. (2011). Enterococci as probiotics and their implications in food safety. Int. J. Food Microbiol. 151, 125–140. doi: 10.1016/j.ijfoodmicro.2011.08.014
Golob, M., Pate, M., Kušar, D., Dermota, U., Avberšek, J., Papić, B., et al. (2019). Antimicrobial resistance and virulence genes in Enterococcus faecium and Enterococcus faecalis from humans and retail red meat. Biomed. Res. Int. 2019:2815279. doi: 10.1155/2019/2815279
Guan, C., Tang, B., Yang, H., Ma, J., Huang, Y., and Liu, C. (2022). Emergence of plasmid-mediated tigecycline resistance gene, tet(X4), in Escherichia fergusonii from pigs. J. Glob. Antimicrob. Resist. 30, 249–251. doi: 10.1016/j.jgar.2022.06.029
Hanchi, H., Mottawea, W., Sebei, K., and Hammami, R. (2018). The genus Enterococcus: between probiotic potential and safety concerns-an update. Front. Microbiol. 9:1791. doi: 10.3389/fmicb.2018.01791
Hao, W., Shan, X., Li, D., Schwarz, S., Zhang, S. M., Li, X. S., et al. (2019). Analysis of a poxtA- and optrA-co-carrying conjugative multiresistance plasmid from Enterococcus faecalis. J. Antimicrob. Chemother. 74, 1771–1775. doi: 10.1093/jac/dkz109
Hu, F., Guo, Y., Yang, Y., Zheng, Y., and Wang, F. J. E. J. O. C. M. (2019). Resistance reported from China antimicrobial surveillance network (CHINET) in 2018. Eur. J. Clin. Microbiol. Infect. Dis. 38, 2275–2281. doi: 10.1007/s10096-019-03673-1
Huang, Z., Bai, Y., Wang, Q., Yang, X., Zhang, T., Chen, X., et al. (2022). Persistence of transferable oxazolidinone resistance genes in enterococcal isolates from a swine farm in China. Front. Microbiol. 13:1010513. doi: 10.3389/fmicb.2022.1010513
Huang, J., Wang, M., Gao, Y., Chen, L., and Wang, L. (2019). Emergence of plasmid-mediated oxazolidinone resistance gene poxtA from CC17 Enterococcus faecium of pig origin. J. Antimicrob. Chemother. 74, 2524–2530. doi: 10.1093/jac/dkz250
Humphries, R., Bobenchik, A. M., Hindler, J. A., and Schuetz, A. N. (2021). Overview of changes to the clinical and laboratory standards institute performance standards for antimicrobial susceptibility testing, M100, 31st edition. J. Clin. Microbiol. 59:e0021321. doi: 10.1128/jcm.00213-21
Kang, Z. Z., Lei, C. W., Kong, L. H., Wang, Y. L., Ye, X. L., Ma, B. H., et al. (2019). Detection of transferable oxazolidinone resistance determinants in Enterococcus faecalis and Enterococcus faecium of swine origin in Sichuan Province, China. J. Glob. Antimicrob. Resist. 19, 333–337. doi: 10.1016/j.jgar.2019.05.021
Kwon, K. H., Hwang, S. Y., Moon, B. Y., Park, Y. K., Shin, S., Hwang, C. Y., et al. (2012). Occurrence of antimicrobial resistance and virulence genes, and distribution of enterococcal clonal complex 17 from animals and human beings in Korea. J. Vet. Diagn. Investig. 24, 924–931. doi: 10.1177/1040638712455634
Lei, C. W., Chen, X., Liu, S. Y., Li, T. Y., Chen, Y., and Wang, H. N. (2021). Clonal spread and horizontal transfer mediate dissemination of phenicol-oxazolidinone-tetracycline resistance gene poxtA in enterococci isolates from a swine farm in China. Vet. Microbiol. 262:109219. doi: 10.1016/j.vetmic.2021.109219
Li, Y., Ed-Dra, A., Tang, B., Kang, X., Müller, A., Kehrenberg, C., et al. (2022b). Higher tolerance of predominant Salmonella serovars circulating in the antibiotic-free feed farms to environmental stresses. J. Hazard. Mater. 438:129476. doi: 10.1016/j.jhazmat.2022.129476
Li, R., Xie, M., Zhang, J., Yang, Z., Liu, L., Liu, X., et al. (2017). Genetic characterization of mcr-1-bearing plasmids to depict molecular mechanisms underlying dissemination of the colistin resistance determinant. J. Antimicrob. Chemother. 72, 393–401. doi: 10.1093/jac/dkw411
Li, J., Yang, L., Huang, X., Wen, Y., Zhao, Q., Huang, X., et al. (2022a). Molecular characterization of antimicrobial resistance and virulence factors of Enterococcus faecalis from ducks at slaughterhouses. Poult. Sci. 101:101646. doi: 10.1016/j.psj.2021.101646
Lin, J., Tang, B., Zheng, X., Chang, J., Ma, J., He, Y., et al. (2022). Emergence of Incl2 plasmid-mediated colistin resistance in avian Escherichia fergusonii. FEMS Microbiol. Lett. 369:fnac016. doi: 10.1093/femsle/fnac016
Liu, B. G., Yuan, X. L., He, D. D., Hu, G. Z., Miao, M. S., and Xu, E. P. (2020). Research progress on the oxazolidinone drug linezolid resistance. Eur. Rev. Med. Pharmacol. Sci. 24, 9274–9281. doi: 10.26355/eurrev_202009_23009
Ma, J., Zhou, W., Wu, J., Liu, X., Lin, J., Ji, X., et al. (2022). Large-scale studies on antimicrobial resistance and molecular characterization of Escherichia coli from food animals in developed areas of eastern China. Microbiol. Spectr. 10:e0201522. doi: 10.1128/spectrum.02015-22
Moure, Z., Lara, N., Marín, M., Sola-Campoy, P. J., Bautista, V., Gómez-Bertomeu, F., et al. (2020). Interregional spread in Spain of linezolid-resistant Enterococcus spp. isolates carrying the optrA and poxtA genes. Int. J. Antimicrob. Agents 55:105977. doi: 10.1016/j.ijantimicag.2020.105977
Murphy, E., Phillips, S., Edelman, I., and Novick, R. P. (1981). Tn554: isolation and characterization of plasmid insertions. Plasmid 5, 292–305. doi: 10.1016/0147-619x(81)90006-8
Na, S. H., Moon, D. C., Choi, M. J., Oh, S. J., Jung, D. Y., Kang, H. Y., et al. (2019). Detection of oxazolidinone and phenicol resistant enterococcal isolates from duck feces and carcasses. Int. J. Food Microbiol. 293, 53–59. doi: 10.1016/j.ijfoodmicro.2019.01.002
Nüesch-Inderbinen, M., Biggel, M., Zurfluh, K., Treier, A., and Stephan, R. (2022a). Faecal carriage of enterococci harbouring oxazolidinone resistance genes among healthy humans in the community in Switzerland. J. Antimicrob. Chemother. 77, 2779–2783. doi: 10.1093/jac/dkac260
Nüesch-Inderbinen, M., Haussmann, A., Treier, A., Zurfluh, K., Biggel, M., and Stephan, R. (2022b). Fattening pigs are a reservoir of florfenicol-resistant enterococci harboring oxazolidinone resistance genes. J. Food Prot. 85, 740–746. doi: 10.4315/jfp-21-431
Osman, K. M., Badr, J., Orabi, A., Elbehiry, A., Saad, A., Ibrahim, M. D. S., et al. (2019). Poultry as a vector for emerging multidrug resistant Enterococcus spp.: first report of vancomycin (van) and the chloramphenicol-florfenicol (cat-fex-cfr) resistance genes from pigeon and duck faeces. Microb. Pathog. 128, 195–205. doi: 10.1016/j.micpath.2019.01.006
Park, K., Jeong, Y. S., Chang, J., Sung, H., and Kim, M. N. (2020). Emergence of optrA-mediated linezolid-nonsusceptible Enterococcus faecalis in a tertiary care hospital. Ann. Lab. Med. 40, 321–325. doi: 10.3343/alm.2020.40.4.321
Peng, Z., Liu, S., Meng, X., Liang, W., Xu, Z., Tang, B., et al. (2017). Genome characterization of a novel binary toxin-positive strain of Clostridium difficile and comparison with the epidemic 027 and 078 strains. Gut Pathog. 9:42. doi: 10.1186/s13099-017-0191-z
Pillay, S., Zishiri, O. T., and Adeleke, M. A. (2018). Prevalence of virulence genes in Enterococcus species isolated from companion animals and livestock. Onderstepoort. J. Vet. Res. 85, e1–e8. doi: 10.4102/ojvr.v85i1.1583
Rodríguez-Lucas, C., Fernández, J., Vázquez, X., de Toro, M., Ladero, V., Fuster, C., et al. (2022). Detection of the optrA gene among polyclonal linezolid-susceptible isolates of Enterococcus faecalis recovered from community patients. Microb. Drug Resist. 28, 773–779. doi: 10.1089/mdr.2021.0402
Ruiz-Ripa, L., Feßler, A. T., Hanke, D., Sanz, S., Olarte, C., Eichhorn, I., et al. (2020). Detection of poxtA- and optrA-carrying E. faecium isolates in air samples of a Spanish swine farm. J. Glob. Antimicrob. Resist. 22, 28–31. doi: 10.1016/j.jgar.2019.12.012
Saavedra, S. Y., Bernal, J. F., Montilla-Escudero, E., Torres, G., Rodríguez, M. K., Hidalgo, A. M., et al. (2020). National surveillance of clinical isolates of Enterococcus faecalis resistant to linezolid carrying the optrA gene in Colombia, 2014-2019. Rev. Panam. Salud Publica 44:e104. doi: 10.26633/rpsp.2020.104
Sadowy, E. (2018). Linezolid resistance genes and genetic elements enhancing their dissemination in enterococci and streptococci. Plasmid 99, 89–98. doi: 10.1016/j.plasmid.2018.09.011
Savin, M., Bierbaum, G., Hammerl, J. A., Heinemann, C., Parcina, M., Sib, E., et al. (2020). ESKAPE Bacteria and extended-Spectrum-β-lactamase-producing Escherichia coli isolated from wastewater and process water from German poultry slaughterhouses. Appl. Environ. Microbiol. 86:e02748–19. doi: 10.1128/aem.02748-19
Schwarz, S., Zhang, W., Du, X. D., Krüger, H., Feßler, A. T., Ma, S., et al. (2021). Mobile oxazolidinone resistance genes in gram-positive and gram-negative bacteria. Clin. Microbiol. Rev. 34:e0018820. doi: 10.1128/cmr.00188-20
Shan, X., Li, X. S., Wang, N., Schwarz, S., Zhang, S. M., Li, D., et al. (2020). Studies on the role of IS1216E in the formation and dissemination of poxtA-carrying plasmids in an Enterococcus faecium clade A1 isolate. J. Antimicrob. Chemother. 75, 3126–3130. doi: 10.1093/jac/dkaa325
Shao, Y., Zhen, W., Guo, F., Hu, Z., Zhang, K., Kong, L., et al. (2022). Pretreatment with probiotics Enterococcus faecium NCIMB 11181 attenuated Salmonella Typhimurium-induced gut injury through modulating intestinal microbiome and immune responses with barrier function in broiler chickens. J. Anim. Sci. Biotechnol. 13:130. doi: 10.1186/s40104-022-00765-5
Staley, C., Dunny, G. M., and Sadowsky, M. J. (2014). Environmental and animal-associated enterococci. Adv. Appl. Microbiol. 87, 147–186. doi: 10.1016/b978-0-12-800261-2.00004-9
Sullivan, M. J., Petty, N. K., and Beatson, S. A. (2011). Easyfig: a genome comparison visualizer. Bioinformatics 27, 1009–1010. doi: 10.1093/bioinformatics/btr039
Tacconelli, E., Carrara, E., Savoldi, A., Harbarth, S., Mendelson, M., Monnet, D. L., et al. (2018). Discovery, research, and development of new antibiotics: the WHO priority list of antibiotic-resistant bacteria and tuberculosis. Lancet Infect. Dis. 18, 318–327. doi: 10.1016/s1473-3099(17)30753-3
Tang, B., Chang, J., Chen, Y., Lin, J., Xiao, X., Xia, X., et al. (2022a). Escherichia fergusonii, an underrated repository for antimicrobial resistance in food animals. Microbiol. Spectr. 10:e0161721. doi: 10.1128/spectrum.01617-21
Tang, B., Elbediwi, M., Nambiar, R. B., Yang, H., Lin, J., and Yue, M. (2022b). Genomic characterization of antimicrobial-resistant Salmonella enterica in duck, chicken, and pig farms and retail markets in Eastern China. Microbiol. Spectr. 10:e0125722. doi: 10.1128/spectrum.01257-22
Tang, B., Tang, Y., Zhang, L., Liu, X., Chang, J., Xia, X., et al. (2020). Emergence of fexA in mediating resistance to florfenicols in Campylobacter. Antimicrob. Agents Chemother. 64, e00260–e00220. doi: 10.1128/aac.00260-20
Tang, B., Wang, Y., Luo, Y., Zheng, X., Qin, X., Yang, H., et al. (2021). Coexistence of optrA and fexA in Campylobacter. mSphere 6, e00125–e00121. doi: 10.1128/mSphere.00125-21
Tang, B., Zheng, X., Lin, J., Wu, J., Lin, R., Jiang, H., et al. (2022c). Prevalence of the phenicol resistance gene fexA in Campylobacter isolated from the poultry supply chain. Int. J. Food Microbiol. 381:109912. doi: 10.1016/j.ijfoodmicro.2022.109912
Tyson, G. H., Nyirabahizi, E., Crarey, E., Kabera, C., Lam, C., Rice-Trujillo, C., et al. (2018a). Prevalence and antimicrobial resistance of enterococci isolated from retail meats in the United States, 2002 to 2014. Appl. Environ. Microbiol. 84, e01902–e01917. doi: 10.1128/aem.01902-17
Tyson, G. H., Sabo, J. L., Hoffmann, M., Hsu, C. H., Mukherjee, S., Hernandez, J., et al. (2018b). Novel linezolid resistance plasmids in Enterococcus from food animals in the USA. J. Antimicrob. Chemother. 73, 3254–3258. doi: 10.1093/jac/dky369
Wang, Y., Li, X., Fu, Y., Chen, Y., Wang, Y., Ye, D., et al. (2020). Association of florfenicol residues with the abundance of oxazolidinone resistance genes in livestock manures. J. Hazard. Mater. 399:123059. doi: 10.1016/j.jhazmat.2020.123059
Wang, Y., Lv, Y., Cai, J., Schwarz, S., Cui, L., Hu, Z., et al. (2015). A novel gene, optrA, that confers transferable resistance to oxazolidinones and phenicols and its presence in Enterococcus faecalis and Enterococcus faecium of human and animal origin. J. Antimicrob. Chemother. 70, 2182–2190. doi: 10.1093/jac/dkv116
Wang, Q., Tang, B., Song, L., Ren, B., Liang, Q., Xie, F., et al. (2013). 3DScapeCS: application of three dimensional, parallel, dynamic network visualization in Cytoscape. BMC Bioinform. 14:322. doi: 10.1186/1471-2105-14-322
Wardenburg, K. E., Potter, R. F., D'Souza, A. W., Hussain, T., Wallace, M. A., Andleeb, S., et al. (2019). Phenotypic and genotypic characterization of linezolid-resistant Enterococcus faecium from the USA and Pakistan. J. Antimicrob. Chemother. 74, 3445–3452. doi: 10.1093/jac/dkz367
Wick, R. R., Judd, L. M., Gorrie, C. L., and Holt, K. E. (2017). Unicycler: resolving bacterial genome assemblies from short and long sequencing reads. PLoS Comput. Biol. 13:e1005595. doi: 10.1371/journal.pcbi.1005595
Xu, C., Wang, N., Li, D., Schwarz, S., and Du, X. D. (2022). Recombination events that occur in a poxtA-carrying Enterococcus faecium during the conjugation process. J. Antimicrob. Chemother. 77, 1228–1236. doi: 10.1093/jac/dkac027
Yang, W., Yuan, L., Jiachang, C., Stefan, S., Lanqing, C., Zhidong, H., et al. (2015). A novel gene, optrA, that confers transferable resistance to oxazolidinones and phenicols and its presence in Enterococcus faecalis and Enterococcus faecium of human and animal origin. (8), 2182.
Yoon, S., Son, S. H., Kim, Y. B., Seo, K. W., and Lee, Y. J. (2020). Molecular characteristics of optrA-carrying Enterococcus faecalis from chicken meat in South Korea. Poult. Sci. 99, 6990–6996. doi: 10.1016/j.psj.2020.08.062
Yu, L., Liu, Y., Liu, M., Li, Z., Li, L., and Wang, F. (2022a). Research note: molecular characterization of antimicrobial resistance and virulence gene analysis of Enterococcus faecalis in poultry in Tai'an, China. Poult. Sci. 101:101763. doi: 10.1016/j.psj.2022.101763
Yu, Y., Ye, X. Q., Liang, H. Q., Zhong, Z. X., Cheng, K., Sun, J., et al. (2022b). Lilium spp., as unnoticed environmental vector, spreading optrA-carrying Enterococcus spp. Sci. Total Environ. 816:151540. doi: 10.1016/j.scitotenv.2021.151540
Zarzecka, U., Zakrzewski, A. J., Chajęcka-Wierzchowska, W., and Zadernowska, A. (2022). Linezolid-resistant Enterococcus spp. isolates from foods of animal origin-the genetic basis of acquired resistance. Foods 11:975. doi: 10.3390/foods11070975
Zheng, X., Ma, J., Lu, Y., Sun, D., Yang, H., Xia, F., et al. (2022). Detection of tet(X6) variant-producing Proteus terrae subsp. cibarius from animal cecum in Zhejiang, China. J. Glob. Antimicrob. Resist. 29, 124–130. doi: 10.1016/j.jgar.2022.02.011
Zhou, W., Lin, R., Zhou, Z., Ma, J., Lin, H., Zheng, X., et al. (2022). Antimicrobial resistance and genomic characterization of Escherichia coli from pigs and chickens in Zhejiang, China. Front. Microbiol. 13:1018682. doi: 10.3389/fmicb.2022.1018682
Keywords: Enterococcus, linezolid, poxtA, optrA, Cfr(D)
Citation: Ni J, Long X, Wang M, Ma J, Sun Y, Wang W, Yue M, Yang H, Pan D and Tang B (2023) Transmission of linezolid-resistant Enterococcus isolates carrying optrA and poxtA genes in slaughterhouses. Front. Sustain. Food Syst. 7:1179078. doi: 10.3389/fsufs.2023.1179078
Edited by:
Shoukui He, Shanghai Jiao Tong University, ChinaReviewed by:
Xiang Wang, University of Shanghai for Science and Technology, ChinaZhong Peng, Huazhong Agricultural University, China
Nitin Dhowlaghar, The University of Tennessee, Knoxville, United States
Copyright © 2023 Ni, Long, Wang, Ma, Sun, Wang, Yue, Yang, Pan and Tang. This is an open-access article distributed under the terms of the Creative Commons Attribution License (CC BY). The use, distribution or reproduction in other forums is permitted, provided the original author(s) and the copyright owner(s) are credited and that the original publication in this journal is cited, in accordance with accepted academic practice. No use, distribution or reproduction is permitted which does not comply with these terms.
*Correspondence: Biao Tang, dGJfNDExQDE2My5jb20=; Daodong Pan, ZGFvZG9uZ3BhbkAxNjMuY29t
†These authors have contributed equally to this work