- 1Department of Food Safety, Istituto Zooprofilattico Sperimentale Delle Venezie, Legnaro, Italy
- 2Laboratorio di Chimica Sperimentale, Istituto Zooprofilattico Sperimentale Delle Venezie, Vicenza, Italy
- 3DeFENS—Dipartimento di Scienze per gli Alimenti, la Nutrizione e l'Ambiente, Università Degli Studi di Milano via Celoria 2, Milan, Italy
The sustainability of food systems is of growing concern worldwide, so insects are a growing source of animal proteins for food and feed. Among insect species, the house cricket, Acheta domesticus, is a promising option from a sustainability point of view. This study aimed at evaluating both microbiological and chemical stability of A. domesticus powders during one year of storage at room temperature. Our study was conducted on cricket powders obtained by three different processes: drying at 80°C (P80), drying at 120°C (P120), and lyophilization (PL). Regarding microbiological profile, the pathogens Listeria monocytogenes and Salmonella were not detected, while total viable count and lactic acid bacteria tended to decrease during the storage period. Bacillus cereus was detected at low counts during the entire storage period, but only one replicate of P80 exceeded the limit set for A. domesticus by the European Commission Implementing Regulation 2017/2470. The measured protein contents of the cricket powders immediately after production ranged between 60.6 and 64.3%. The peroxide values were far higher than the limit established by the EU Regulation in all samples. Higher amounts of hexanal and pentanal were detected in P80 and P120 than in PL, indicating that oven drying could enhance lipid oxidation. In conclusion, a one year shelf-life can suitably be ascribed to the analyzed cricket powders from a microbiological point of view, but lacked chemical stability and had a clear early tendency toward rancidity. The samples dried at 120°C and lyophilized never exceeded the limit set by European Commission for B. cereus count in A. domesticus powder. In addition, lyophilized samples showed the lowest values of hexanal and pentanal, the aldehydes that are primarily responsible for the rancid smell. The oxidative status of the final products could be strongly influenced by the powder processing, so for this reason, edible insect species-specific post-rearing protocols should be implemented. These results open an interesting scenario for a long period of storage of insect powder in the absence of a cold chain, making the insect market an increasingly sustainable sector.
1. Introduction
The sustainability of food systems is of growing concern worldwide. The continuous growth of the human population and the subsequent need to increase the supply of food to feed the global population requires the development of efficient production systems. However, differently from the past, the environment can no longer support the burden of a food chain that requires high amounts of water, land, and energy and that causes high impacts in terms of pollution and greenhouse gas emissions. In addition, the current severe climate change makes the production of high quantities of food each year more challenging. Moreover, due to the SARS-CoV-2 pandemic, agriculture has had different impacts, with the disruption of supply chains and their consequences on food systems, seriously endangering the food security of low income countries (Galanakis, 2015; Zurayk, 2020). In order to solve this substantial food insecurity, alternative food sources, must be considered. The alternative sources of food must be sustainable for the planet, healthy and rich in nutrients (Kemsawasd et al., 2022). Note that the widely acknowledged One-health concept, linking human, animal, and environmental health, needs to be considered by policies and businesses. In this scenario, different possibilities exist to develop sustainable food systems, such as edible insect production and processing.
Insects are a promising source of animal proteins for food and feed (Belluco et al., 2022). This is recognized by the long history of humans consuming insects worldwide (van Huis et al., 2013; Jongema, 2015), and insect farming allows the production of sustainable proteins, with low energy input and small environmental footprint compared to conventional farming systems (van Huis, 2020).
Among insects, Acheta domesticus (L.; Orthoptera: Gryllidae) species, is a promising option in terms of protein and lipid contents provided (63–73 and 13–22/100 g dry matter, respectively), similar to those of conventional animal sources such as beef or chicken (Kulma et al., 2019). In addition, regarding production efficiency, A. domesticus shows a 2.1-feed conversion ratio, which means that 2.1 Kg of dried feed is needed to produce 1 Kg of edible product. In comparison, 4.5, 9.1, and 25 Kg of feed are required to produce 1 Kg of edible product from poultry, pigs, and cattle, respectively (van Huis, 2013).
In the case of crickets, the sustainability claim is well-know but the safety of these insects for human health need to be supported by data. In the European Union, for example, food items (including insects) that are not recognized as traditional in its territory are categorized as novel foods (Reg. 2015/2283) and require a pre-market authorization path before entering the market [European Commission (EU), 2015]. It is clear that in the immediate future there will be an increasing need to define trade-offs between safety and sustainability within the One-Health perspective.
The safety aspects of insects’ supply chains, despite have being overlooked for a long period during the first phases of their consideration as food in Western countries, are now well studied. However, considering the need for a species-by-species assessment, some data gaps need to be filled. Among these is the microbiological stability of insect powders. A long shelf-life in the absence of a cold chain could be an important addition to the already demonstrated sustainability advantages of eating insects. The absence of a cold chain allows for relatively low energy requirements along the shelf-life, and for a potential decrease of wasted food, reducing the typical phenomena of perishable, chilled food products (La Scalia et al., 2019).
Recently, two studies have focused on the variation of physicochemical properties of A. domesticus powder for long storage purposes, investigating hydration properties and estimating the powder’s shelf-life (Kamau et al., 2018) and studying the oxidative stability (Singh et al., 2020). However, microbiological parameters and other important indicators of oxidative status have not been considered to date, while the longest storage period was 16 weeks.
The aim of this study was to evaluate the microbial and chemical stability of cricket (A. domesticus) powders during a one year storage period at room temperature. The investigated powders were produced through three different processes: medium temperature drying (80°C), high temperature drying (120°C), and lyophilization. For this reason, the study also aimed to determine the best treatment to apply in order to produce a safe and less perishable A. domesticus powder. Since insects were proposed (already since 1970s) as a solution to the world protein shortage (Meyer-Rochow, 1975; Chavunduka, 1976; van Huis et al., 2013), the results of the present work are for the possible processing, use, distribution and preservation mode of A. domesticus powder all over the world, with particular reference for those countries facing food shortage. To our knowledge, this is the first report investigating both the microbiological and chemical quality profiles of A. domesticus powder during one year of storage.
2. Materials and methods
2.1. Cricket powder production and extended storage
Cricket powder was produced at the University of Milan as described in Jucker et al. (2021). Briefly, A. domesticus were reared inside plastic containers (71 cm × 46 cm × 35 cm) in a climate-controlled room (27 ± 1°C, 60 ± 5%RH, light: dark 12:12 h). Each rearing container was provided with 30 eggs on cardboard (25 cm × 40 cm) in order to prevent cannibalism by guaranteeing a sufficient surface area was available for the crickets. Water was supplied with a chicken water feeder, while feed was provided ad libitum and consisted of laying hen diet (Sani sapori, Petrini, Italy). When adults appeared, oviposition sites consisting of plastic containers (15 cm diameter) filled with moistened peat were added into each rearing container. Containers with eggs were removed every 3 days and placed in a climate-controlled room at 28 ± 1°C and 60 ± 5% RH for egg hatching.
To produce the powder, crickets (6–8 weeks old) were starved for 24 h and then collected and rinsed with fresh water to eliminate feces and wastes. Crickets were killed by blanching (1 min at 100°C in boiling water) and then dehydrated with a desiccator until a constant weight was reached. Two different temperatures for the desiccation were applied: 80 or 120°C. In addition, applying a third process, crickets were lyophilized for 24 h (CoolSafe Basic, LaboGene, Allerød, Denmark). After thermal treatments or lyophilization, a grinder (Hawos, Queen 2, Bad Homburg, Germany) was used to obtain the final powder. In total, three types of cricket powder were obtained: cricket powder dried at 80°C (P80), cricket powder dried at 120°C (P120), and lyophilized cricket powder (PL). For each process and for each time point, two vacuum packages of cricket powders were stored in the dark at room temperature (22.5 ± 4.5°C) before the analyses. Two vacuum packages (correlating to two samples) of each product were analyzed at 0 (T0), 3 (T1), 6 (T2), 9 (T3), and 12 (T4) months after production.
2.2. Microbiological, water activity, and pH analyses
Microbiological examination of all samples was performed under strictly aseptic conditions. The analyses applied to each sample were: enumeration of lactic acid bacteria (LAB) at 30°C; enumeration of presumptive Bacillus cereus at 30°C; enumeration of coagulase-positive staphylococci (Staphylococcus aureus and other species); enumeration of ß-glucuronidase-positive Escherichia coli at 44°C; enumeration of Enterobacteriaceae; enumeration of molds and yeasts; total viable count (TVC) at 30°C; enumeration of vegetative form of sulfite-reducing clostridia (VRC); enumeration of spores of sulfite-reducing clostridia (SRC); enumeration of vegetative form of sulfite-reducing anaerobic bacteria (VRB); enumeration of spores of sulfite-reducing anaerobic bacteria (SRB); enumeration of coliforms at 30°C; enumeration of Pseudomonas spp.; presence of Salmonella spp. (only at T0); and presence of Listeria monocytogenes (only at T0). The sensitivities (limit of detection—LOD) of the bacteria count analyses and references for the methods employed are reported in Supplementary Table 1. Two replicates for each sample were analyzed at each time point.
Water activity (aw) and pH were measured with the technique based on the dew-point measurement (ISO 18787, 2017; METER Aqualab CX 4-TE) and via the potentiometric method (HACH Sension + MM374 GLP 2-channel), respectively.
2.3. Chemical analyses
2.3.1. Protein content
The amount of nitrogen in each sample at T0 was measured by elemental analysis using a FlashSmart analyzer (Thermo Fisher Scientific, Bremen, Germany). The elemental analyzer operates according to the dynamic ash combustion of the sample. Samples (200 mg) were weighed into tin containers and injected into the combustion reactor with O2. The gases produced were transferred by a He flow into a copper reactor, then swept through CO2 and H2O traps, separated by a chromatographic column, and detected by a thermal conductivity detector (TCD).
Afterward, the percentage of protein content was calculated from the resultant amount of nitrogen using the conversion factor recommended by Boulos et al. (2020) (%N × 5.33) to avoid overestimation of the protein content due to the chitin-derived nitrogen.
2.3.2. Total volatile basic nitrogen
The volatile nitrogenous bases were extracted from the samples using an aqueous solution of 6% perchloric acid (Sigma Aldrich, Steinheim, Germany). Then, the extract underwent steam distillation, and the volatile base components were absorbed by the boric acid in the receiver. The TVB-N concentration was finally measured by titration of the absorbed bases with chloric acid 0.01 N. The procedure is described in the European Regulation 2074/2005 [European Commission (EC), 2005].
2.3.3. Extraction of fat and determination of peroxide value
The fat in the cricket powders was extracted using acetone and hexane, both purchased from Sigma Aldrich (Steinheim, Germany). To this aim, 70 g of each cricket powder were transferred separately into a glass beaker and covered with an acetone:hexane (1:1, v/v) solution. The sample was then shaken and sonicated for 10 min. A volume of 10 mL was then filtered and transferred into a vacuum filtration flask. The extraction procedure was repeated two more times, with the subsequent combination of the three extracts. Finally, the combined extracts were dried using a rotary evaporator.
In order to measure the fat oxidation, 1 g of extracted fat was transferred into a 150 mL flask and diluted with 15 mL of glacial acetic acid (Sigma Aldrich, St. Louis, MO, United States), 10 mL of chloroform (Sigma Aldrich, Steinheim, Germany), and 500 μL of saturated aqueous KI solution (Sigma Aldrich, Steinheim, Germany). After 10 min in the dark, 50 mL of distilled water and 2 mL of 1% starch were added. Finally, the titrand was titrated with a solution of sodium thiosulphate 0.01 N.
2.3.4. Aldehyde content
We evaluated the fat rancidity of three different cricket powders (P80, P120, and PL) by quantifying the secondary oxidation products, i.e., hexanal and pentanal, by headspace gas-chromatography-mass spectrometry (HS-GC–MS).
2.3.4.1. Reagents
Hexanal (purity ≥ 99%), pentanal (purity ≥ 99%), 2-Octanone (purity ≥ 99%), butylhydroxytoluene (BHT, ≥99% purity), and sodium chloride (NaCl, ≥99.0%) were purchased from Sigma Aldrich (Steinheim, Germany). Deionized water was obtained by a MilliQ system (Millipore ®, Merck KGaA, Darmstadt, Germany).
2.3.4.2. Preparation of standard solution for the calibration
In order to obtain two calibration curves, one each for the quantification of the two aldehydes (hexanal and pentanal) by HS-GC–MS, four stock solutions were made up as follows: (i) an extraction solution of saturated NaCl was prepared by solubilizing with deionized water an amount of 25 ± 0.1 g of salt in a 100 ± 0.1 mL volumetric flask; (ii) a solution of 1,000 mg/Kg of both hexanal and pentanal was made up by diluting 10 mg of pure reagents with 10 mL of deionized water; (iii) the latter solution was the diluted 10 times to obtain a solution of 100 mg/Kg; (iv) a 1,000 mg/Kg solution of 2-octanone, used as internal standard, was prepared by diluting 10 mg of pure reagent with deionized water in a 10 ± 0.01 mL flask; (v) a concentrated solution of BHT was prepared by dissolving 5 ± 0.1 g in 10 ± 0.01 mL of deionized water. The diluted standard solutions of hexanal and pentanal at the concentrations of 10, 100, 1,200, 2,500, and 5,000 μg/Kg were then prepared by combining 3 mL of extraction solution, 2 mL of deionized water and corresponding volumes of hexanal and pentanal from stock solutions.
2.3.4.3. Sample preparation
A representative amount of edible cricket powder, 50 ± 0.1 g, was mixed with a spatula. Afterwards, an amount of 1 ± 0.1 g was weighed with an analytical balance (Sartorius®, Göttingen, Germany) directly into a 20 mL headspace vial and diluted with 1 mL of deionized water. Afterward, 3 mL of extraction solution and 10 μL of concentrated BHT solution were added. The vial was immediately closed with a magnetic screw cap fitted with a polytetrafluoroethylene (PTFE) septum. Each sample was prepared in duplicate.
2.3.4.4. Headspace gas chromatography–mass spectrometry
Samples were equilibrated at 90°C with continuous stirring for 30 min. Afterward, a volume of 2 mL of headspace volatiles were transferred with the integrated syringe (heated at 90°C) of the PAL System autosampler (CTC Analytics, Zwingen, Switzerland) in the injector split mode (split ratio 1:20 and injection time delay of 60 s) set at 200°C. The separation of hexanal and pentanal was performed on a gas chromatograph 7890B (Agilent Technologies, Santa Clara, CA, United States) equipped with a DB-WAX capillary column (30 m × 0.25 mm × 0.25 μm, Agilent, J & W Scientific, Folsom, CA, United States). The GC temperature gradient was programmed as described by García-Llatas et al. (2007). Briefly, an initial temperature of 50°C was maintained for 5 min, and increased by 10–100°C/min, followed by heating to 220°C at a rate of 30°C/min and held for 10 min, yielding a total run time of 17 min. The whole process was carried out using a He carrier with a flow rate of 1 mL/min.
The gas-chromatograph was coupled with a 5977B mass spectrometer equipped with a quadrupole analyzer (Agilent Technologies, Santa Clara, CA, United States). Quantification of the target aldehydes was performed in selected ion monitoring (SIM) mode using m/z 44.0–58.0 (pentanal), m/z 41.0–83.0 (hexanal), and m/z 43.0–128.0 (2-octanone) as the quantifying ions (highest intensity). The GC–MS data were collected and analyzed using Mass Hunter Software B.04.00. Calibration curves were constructed from integrated peak areas of the standard aldehydes, plotted as the ratio of their areas and that of the 2-octanone internal standard. As mentioned above, two aliquots of each sample were analyzed and, the resultant data averaged.
2.3.5. Heavy metal measurements
The heavy metals, lead (Pb) and cadmium (Cd), were measured at T0 by inductively coupled plasma mass spectrometry (ICP-MS) using a iCapQ instrument (Thermo Scientific, Bremen, Germany) following the reference method (UNI EN 15763, 2010).
3. Results
3.1. Microbiological analysis
Figure 1 depicts the counts in the cricket powders of the detected bacteria targets during the one year storage period. TVC and LAB counts in all cricket powders tended to decrease during the storage period (Figures 1A,B). Bacillus cereus was distributed at non-homogeneous levels of contamination in all samples (Figure 1C). However, B. cereus counts were always under 2.11 Log CFU/g reported in one replicate of P80 at T0. Enterobacteriaceae and coliforms were only found in P80 and PL, but not in P120. In P80, Enterobacteriaceae were found at T0 and T1 (3 months) in only one replicate (30 and 10 CFU/g, respectively), while at T3 (9 months), both replicates of P80 contained Enterobacteriaceae and coliforms (Enterobacteriaceae: 60 and 50 CFU/g; coliforms: 10 and 50 CFU/g). In a single replicate of PL at T0, Enterobacteriaceae and coliforms were found, both at the same level (10 CFU/g). Coagulase positive staphylococci were detected at T0 in both replicates of P120 (91 and 150 CFU/g). Counts of molds were 300 and 400 CFU/g only in the two replicates of P80 at T3 (9 months), while counts in all the other replicates and samples were ≤ 100 CFU/g. All the tested replicates and samples were negative for the following bacteria targets: Escherichia coli, VRC, SRC, VRB, SRB, Pseudomonas spp., L. monocytogenes, and Salmonella spp. The pH of the samples was close to neutral, ranging from pH 6.2 to 7.2. For P80 and P120, aw values were ≤ 0.28 and ≤ 0.29, respectively, while PL had a aw value of ≤0.35.
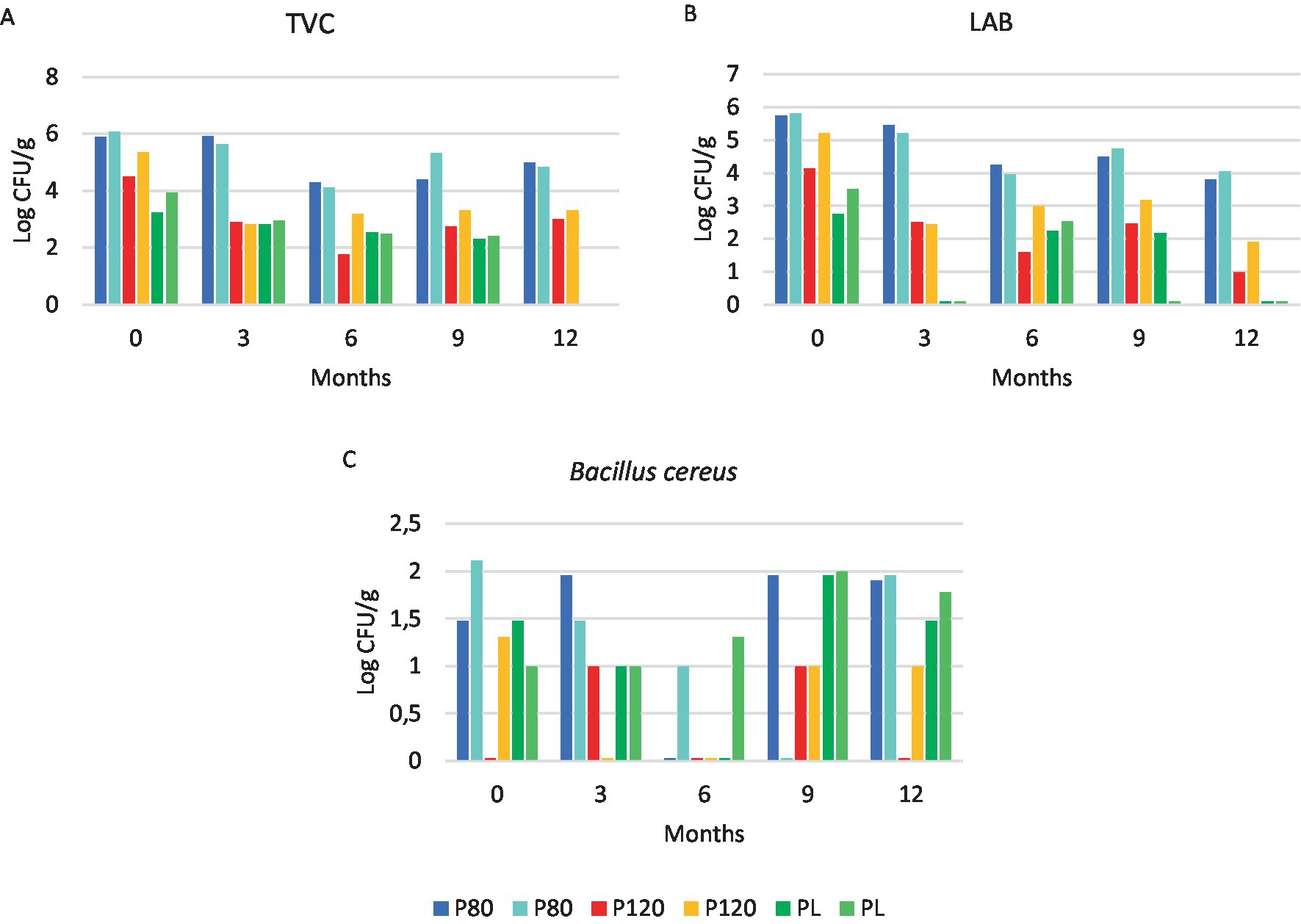
Figure 1. Bacteria counts at five time points in house cricket (Acheta domesticus) powders: freeze-dried (PL), dried at 80°C (P80) and dried at 120°C (P120). Two replicates for each sample were analyzed at each time point. (A) TVC: Total viable count; (B) LAB: lactic acid bacteria; (C) Bacillus cereus.
3.2. Protein content and heavy metals
As crickets are considered alternative protein sources, the protein contents of the three types of cricket powders were measured by elemental analysis at T0. Similar protein contents among the cricket powders were quantified at T0: 60.6% (w/w) for P80, 63.6% (w/w) for P120, and 64.3% (w/w) for PL.
The heavy metal contamination of the three kinds of house cricket powders was assessed by ICP-MS at T0. As shown in Table 1, the accumulation of Cd and Pb in the cricket bodies confirmed the role of A. domesticus as a as a biological indicator of environmental heavy metal contamination.
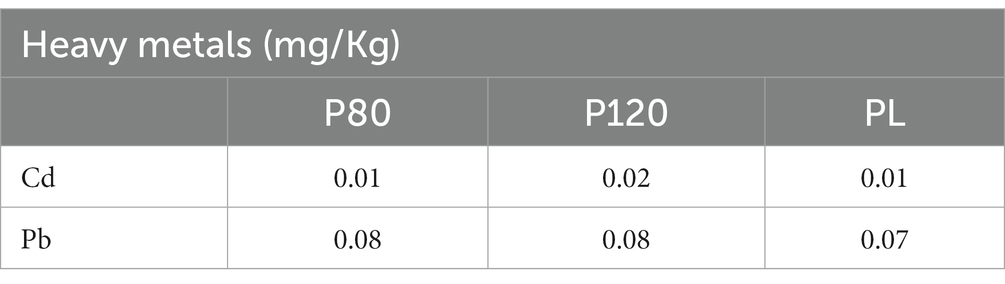
Table 1. Level of the heavy metals cadmium (Cd) and lead (Pb) in house cricket (Acheta domesticus) powders: dried at 80°C (P80), dried at 120°C (P120), and freeze-dried (PL).
3.3. Changes of total volatile nitrogen over time
Total volatile basic nitrogen (TVB-N) is used as a biochemical marker of food spoilage (Holman et al., 2021). The microbiological proliferation and the activities of proteolytic enzyme contribute to the generation of ammonia, biogenic amines as well as other products of amino acid degradation. The three types of powders contained an initial similar amount of TVB-N at T0. From T1 (3 months), while the oven-dried powders (P80 and P120) were characterized by a steady low amount of TVB-N over time, the lyophilized powder (PL) at T2 (6 months) had a higher amount of TVB-N, around 50 mg/Kg, that decreased at T3 (9 months) and T4 (12 months; Figure 2).
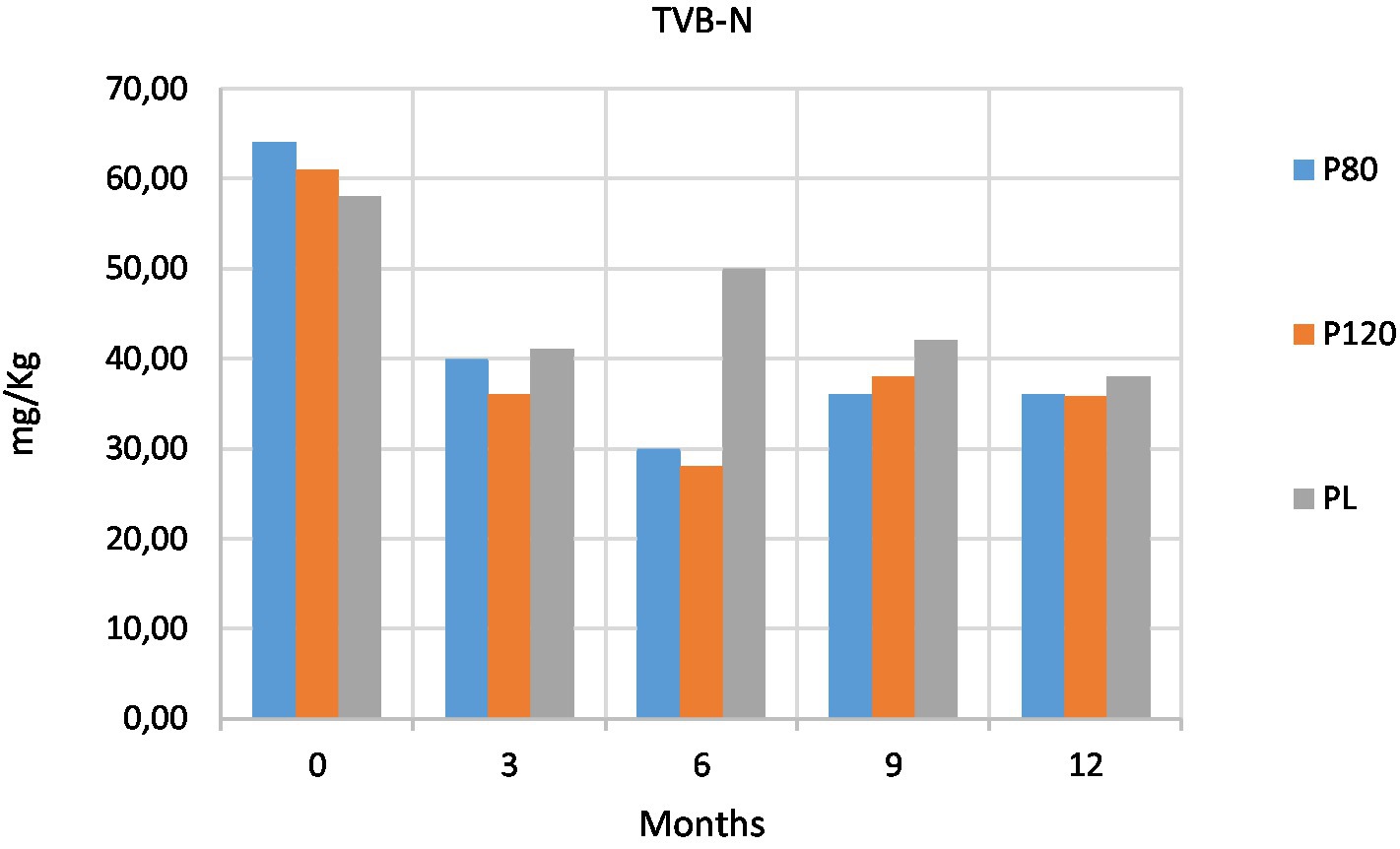
Figure 2. Content of total volatile basic nitrogen (TVB-N, expressed as mg/Kg) at five time points in house cricket (Acheta domesticus) powders: freeze-dried (PL), dried at 80°C (P80), and dried at 120°C (P120).
3.4. Changes of peroxide value and aldehyde content over time
The effect of processing on fat oxidation was evaluated by measuring both peroxide values (PVs) and aldehyde contents. The oxidation is enhanced in samples rich in water, and their primary oxidation can be evaluated measuring the PV. A lower PV was measured in PL at T0 as compared with those powders dried in the oven. The powders dried in the oven at the two different temperatures presented similar PVs at T0. High PVs were observed in oven-dried powder at T4 (Figure 3), whereas lyophilized powder had a high PV at T3.
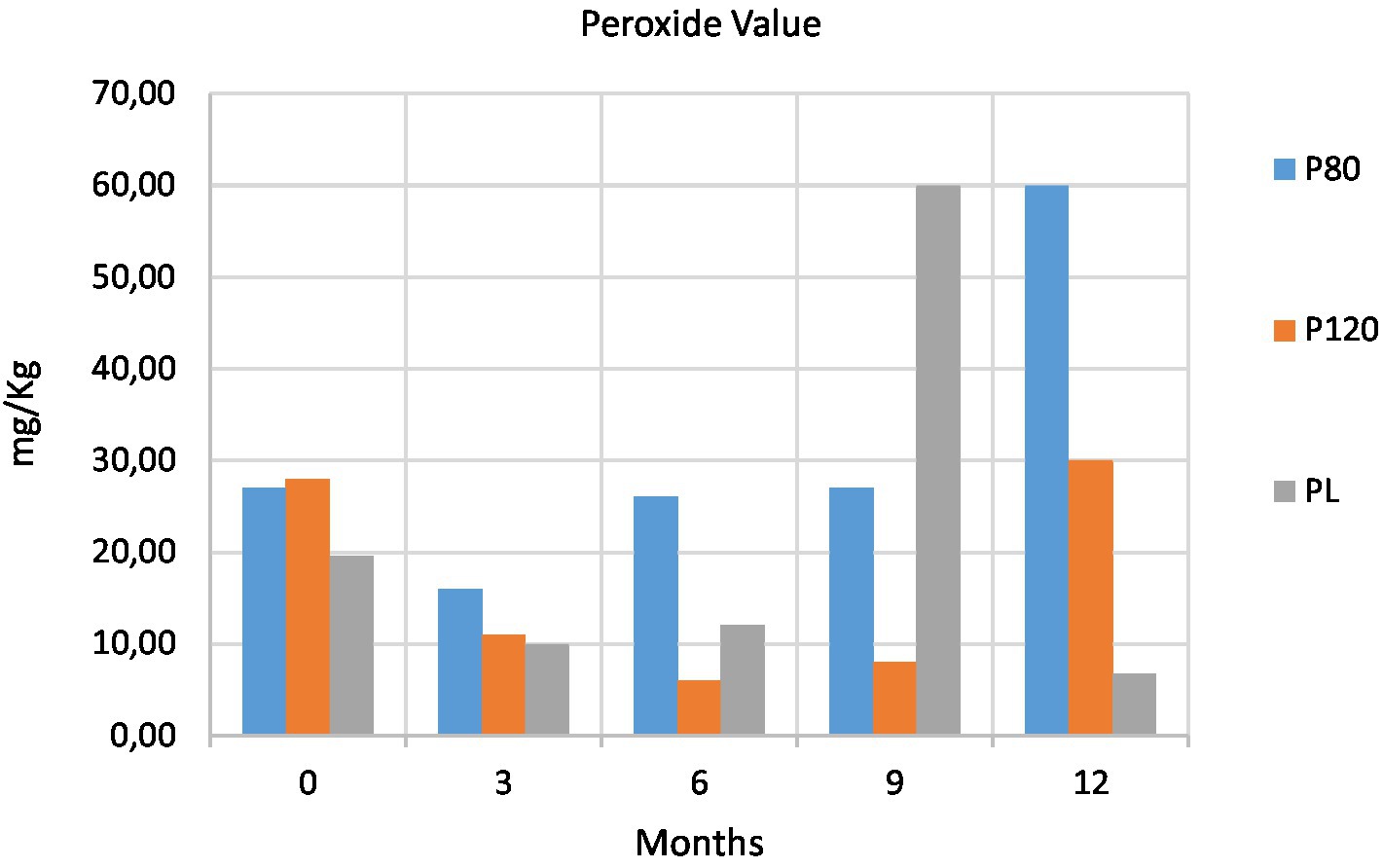
Figure 3. Changes of peroxide value (PV, expressed as mq O2/Kg fat) at five time points in house cricket (Acheta domesticus) powders: freeze-dried (PL), dried at 80°C (P80) and dried at 120°C (P120).
The fat rancidity of the three cricket powders was evaluated using HS-GC–MS by quantifying some secondary oxidation products, i.e., the hexanal and pentanal aldehydes. Detailed contents of pentanal and hexanal are reported in Supplementary Table 2.
Firstly, the levels of hexanal and pentanal in PL were lower than in the oven-dried powders (P80 and P120) at T0 (Figure 4; Supplementary Figure 1), indicating a lower oxidation state for the lyophilized product. In P80 and P120, we observed the degradation of the peroxides at 3 months (Figure 3), with the consequent increase of hexanal and pentanal at the same time point (Figure 4). While the aldehyde contents in P80 and P120 decreased at T2 (6 months) and T3 (9 months; Figure 4; Supplementary Figure 1), the increase of PV at T3 (Figure 3) led to subsequent higher amounts of aldehydes at T4 (12 months; Figure 4; Supplementary Figure 1). On the other hand, we detected an increase of hexanal in PL at T1 (3 months) that decreased over the time with steady values of pentanal during the entire storage period.
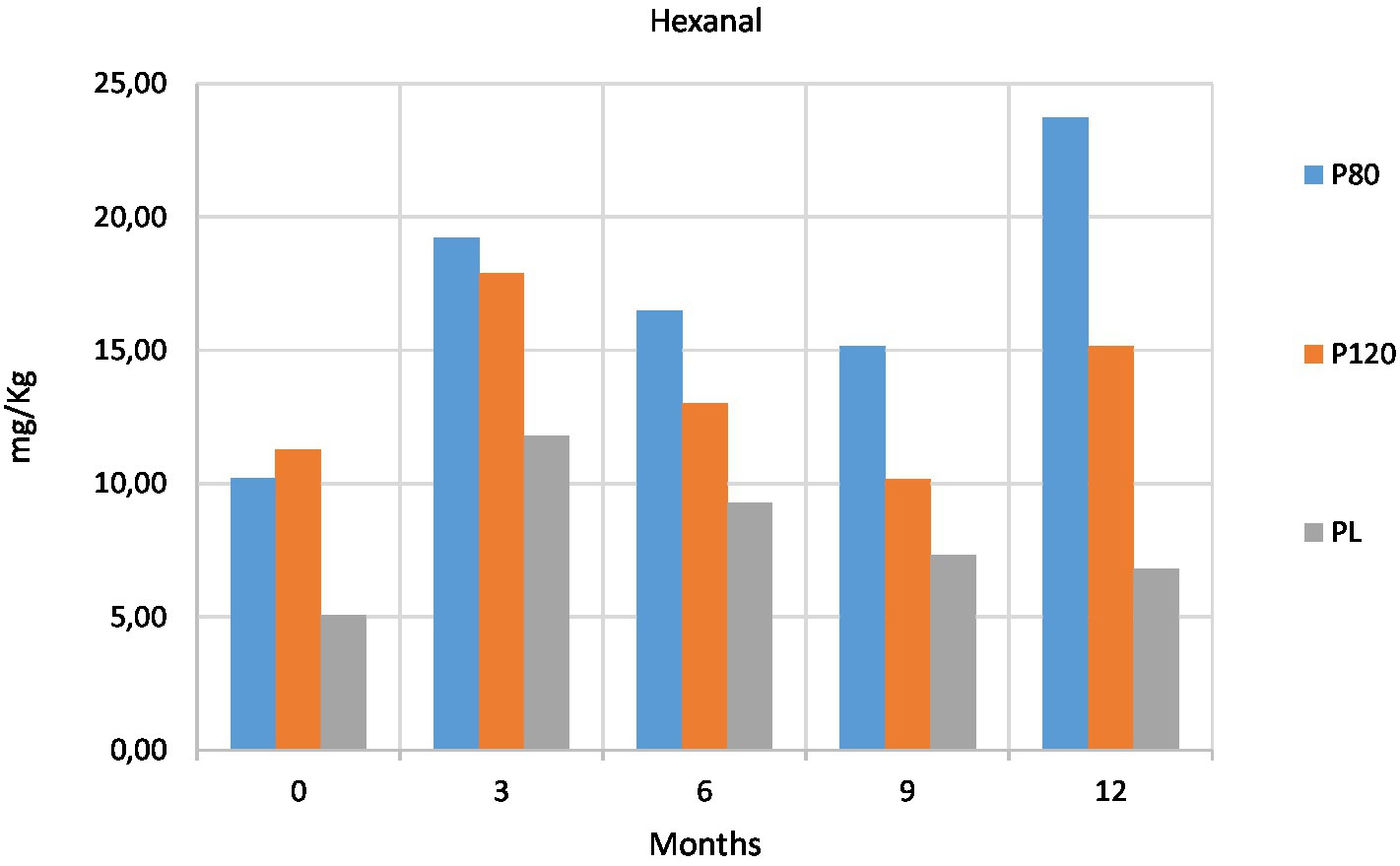
Figure 4. Content (mg/Kg) of hexanal at five time points in house cricket (Acheta domesticus) powders: freeze-dried (PL), dried at 80°C (P80), and dried at 120°C (P120).
4. Discussion
The present study aimed to investigate the microbiological and chemical quality of A. domesticus powder during a one year storage period at room temperature. Microbiological analyses did not reveal any concerns about bacterial counts or pathogen presence. In the absence of specific legislative criteria covering insects or insects based products microbiological and chemical results will be discussed in the context of specifications set by the Commission Implementing Regulation 2017/2470 [European Commission (EU), 2017]. Bacillus cereus was detected at low, stable counts during the entire storage period, although one replicate of P80 exceed the B. cereus limit (≤ 100 CFU/g) set by the European Commission Implementing Regulation 2017/2470 on authorized insects products. Jucker et al. (2021) also reported low counts of B. cereus (10 CFU/g) in cricket powder. TVC and LAB counts tended to decrease during the storage period in all three cricket powders. Jucker et al. (2021) reported lower TVC counts at T0 in powder from crickets fed with control diet. Regulation 2017/2470 set a limit for TVC in authorized A. domesticus powders of 105 CFU/g. All samples of P120 and PL were compliant with this criterion at all tested time points, while P80 was compliant only after the first 6 months of storage (T2). Regarding molds, only two replicate of P80 analyzed at T3 (9 months) had levels of mold higher than the limit set by Regulation 2017/2470 (which demands a level ≤ 100 CFU/g). Only one replicate of P120 exceeded the limit set for coagulase positive-staphylococci (≤ 100 CFU/g), while all the other microbiological criteria were met.
The pH and aw are key factors influencing the shelf-life and stability of food products during storage. The investigated cricket powders had pH values ranging from slightly acidic to basic, so were suitable for a wide range of microorganisms to grow. The reported pH values were in accordance with those reported by previous studies (Vandeweyer et al., 2017; Singh et al., 2020). However, the aw values were very low and able to guarantee the stability of the powders since, in general, at low aw values (< 0.61), predominantly yeasts and molds can proliferate, with filamentous fungi being by far the predominant microorganisms (Tapia et al., 2020).
Crickets have not been a part of Mediterranean or Western diets, but due to their sustainable production and nutritive value, these insects are considered excellent alternative sources of proteins. The Regulation 2017/2470 [European Commission (EU), 2017] regulated, in dried and powdered forms of the house cricket, the percentage of protein, which is set at 55–65 (% w/w) calculated as nitrogen × 6.25 (%N × 6.25). In the present study, the percentage of protein in the cricket powders ranged between 60.6 and 64.3% and, thus, these products met the specifications for protein. Note that the percentage of protein was calculated in our study from the amount of nitrogen, but using the conversion factor recommended by Boulos et al. (2020) (%N × 5.33) to avoid overestimation of the protein content due to the chitin-derived nitrogen. Using the conversion factor %N × 6.25, the protein content of our cricket powders would range between 71.2 and 74.7% w/w, above that established by Regulation 2017/2470 [European Commission (EU), 2017]. The subtraction of chitin’s contribution to the calculated protein, using a more appropriate conversion factor, allows us to exclude the insubstantial nutritional value of the nitrogen in chitin.
Insects have strong relationships with ecology and pollution and are used as bioindicators of the industrial intervention and heavy metal contamination of the soil and aquatic environment (Parikh et al., 2020; Marzoli et al., 2023). In this study, we confirmed the possibility of the house cricket to accumulate Cd and Pb and their utility as indicators of the human activity. Note that the European Commission Regulation 2017/2470 established limits for Pb and Cd in dried A. domesticus powder at ≤0.05 and ≤ 0.06 mg/Kg, respectively [European Commission (EU), 2017]. While the Cd was below the limit in the three types of powders in our study, the Pb levels slightly exceeded the European legal limit in all our samples. However, the amount of Pb is in accordance with the contamination level reported in cricket powder fed with control diet by Jucker et al. (2021).
The oven drying process is one of the most widely used and efficient preservation methods because it is effective and cheap. Reducing the aw and the microbial load, oven drying can extend the shelf-life of meat and meat products (Modi et al., 2007). Nevertheless, high temperatures accelerate oxidative phenomena, especially for products rich in lipids, such as cricket powder (Singh et al., 2020).
According to the EU Regulation [European Commission (EU), 2017], wherein the PV must be ≤5 meq O2/Kg fat, the PVs in all our samples were far above the acceptable limit during the entire storage period. Our PVs were in contrast to those reported by Khatun et al. (2021), who found PVs lower than the detection limit for freeze-dried and blanched samples of A. domesticus. On the contrary, our results are in line with those reported by Lenaerts et al. (2018), who found high PVs for freeze-dried and blanched plus freeze-dried Tenebrio molitor.
Perez-Santaescolastica et al. (2022) claimed that the degradation processes responsible for the key flavors of insects are: (i) chemical and enzymatic oxidation of unsaturated fatty acids; (ii) Maillard reaction and Strecker degradation of free amino acids. The linear aldehydes, such as hexanal and pentanal, may result from the oxidative degradation of unsaturated fatty acid, while branched aldehydes mainly originate from Maillard reaction and Streacker degradation. In fact, the types of aldehydes encountered in A. domesticus are those linear (i.e., hexanal, pentanal, octanal, and nonanal) and branched (i.e., 2-methylbutanal, 3-methylbutanal, and benzaldehyde) with hexanal and pentanal being the primarily responsible for the rancid smell (Perez-Santaescolastica et al., 2022).
As reported in the literature, a complementary way to study fat rancidity is to measure the secondary oxidation products, i.e., hexanal and pentanal, by gas chromatographic techniques (Chou and Chin, 1994; Shahidi and Pegg, 1994; Lanciotti et al., 1999). Specifically, peroxide content is no longer considered an informative feature of the oxidative status of food, because peroxides tend to decompose during the storage time. At the beginning of the storage period, high PVs were measured in our three cricket powders, but since peroxides decompose faster than they form, lower PVs were then measured, followed by a consequent increase caused by the formation of new peroxides over time. It is well known that a low PV represents both an early and an advanced stage of oxidation (Shahidi, 1994). For this reason, aldehydes are more reliable markers of lipid oxidation than are peroxides (Grebenteuch et al., 2021). Note that the hexanal is the most important indicator of lipid oxidation of meat products and, as also confirmed by our present measurements in cricket powders, hexanal content increases more than do other aldehydes during storage. Hexanal is a very good indicator of rancidity for food products rich in polyunsaturated fatty acids (Domínguez et al., 2019). Acheta domesticus is characterized by high contents of the polyunsaturated linoleic (30–40%) and palmitic (24–30%) acids (Grapes et al., 1989), and these same lipids were also the most discriminatory biomarkers of the mass spectrometric fingerprint of this insect species (Tata et al., 2022). Note that the formation of hexanal is caused by the degradation of linoleic acid, an unsaturated fatty acid that, after oxidation, undergoes to the homolytic cleavage of the resultant hydroperoxides. Other studies elucidated the mechanisms of formation of a multitude of low abundant aldehydes and found that they arise from the decomposition of minor hydroperoxides formed by autoxidation of unsaturated fatty acids. In this context, the high levels of PV observed in our samples probably include both regular peroxides obtained by the oxidation with O2 and those generated from the autoxidation with the latter leading to the formation of pentanal originating from the 14-hydroperoxide of linoleic acid (Belitz et al., 2009).
Nevertheless, differences in the aldehyde content of cricket powders were observed between different processing methods. Lower amounts of aldehydes were measured in our lyophilized cricket powder than in the oven-dried powders. In accordance with our data, smaller quantities of aldehydes were previously detected in an accelerated shelf-life study of freeze-dried beef (Shahidi and Pegg, 1994). Higher amounts of hexanal were detected in our oven-dried cricket powders, indicating that this drying method could enhance lipid oxidation, whereas freeze-drying may have a protective effect over time. Khatun et al. (2021) reported much lower amounts of aldehydes in oven-dried A. domesticus powders than in freeze-dried powders. On the other hand, much higher contents of hexanal and pentanal were observed in oven-dried locust and silkworms (Mishyna et al., 2020) and in oven-dried yellow mealworm (T. molitor L.) as compared to their freeze-dried counterparts (Kröncke et al., 2019). Moreover, previous studies observed that the majority of the off-flavors, such as aldehydes, esters, ketones, organic acids, alcohols, sulfur-containing compounds are strictly linked to the initial processing method and outlined that both content and profile of the aldehydes change with the different drying methods (Khatun et al., 2021; Perez-Santaescolastica et al., 2022).
The methods employed for killing and drying edible insects can affect the oxidation of the lipid fraction in the final products. Blanching is the most commonly used killing method, both at traditional and industrial levels, since it has sanitary advantages, and it reduces lipid oxidation (Hernández-Álvarez et al., 2021). However, results from different studies are not always consistent. Singh et al. (2020) reported that the highest lipid oxidation occurred in A. domesticus powder subjected to blanching compared to other killing methods. Indeed, thermal treatments with high temperature (blanching and steaming) produced the highest lipid oxidation, which also increased with storage time. Other storage methods, such as freezing, carbon dioxide, vacuum, and plastic bag, resulted in a lower oxidation level of the product (Singh et al., 2020). In general, a long killing time induces stress, which is related to an increase in the metabolism of energy reserves, and promotes the oxidation process (Caligiani et al., 2019).
Regarding drying treatment, methods such as freeze-drying can increase lipid oxidation, in contrast to oven-drying, which can reduce lipid oxidation (Lenaerts et al., 2018; Khatun et al., 2021). This could be explained by the porous structure of the freeze-dried powder, which makes it very susceptible to fat oxidation (Bonazzi and Dumoulin, 2014; Masson, 2014), while brown substances, which can be produced during the Maillard reaction, can reduce lipid oxidation (Zamora and Hidalgo, 2007; Medina-Meza et al., 2014; Lenaerts et al., 2018). The defatting of insect powders can be an effective method to reduce lipid oxidation (Botella-Martínez et al., 2020).
5. Conclusion
The investigated cricket powders showed good microbiological stability during the one year storage period, with LAB and TVC tending to decrease during this period. No microbiological safety concerns were detected. Powders dried at 120°C and lyophilized showed low B. cereus counts.
The measured protein content was high, supporting the use of A. domesticus powder as a nutrient-rich food source.
Peroxide values in all three types of cricket powders at every time points were present in high amount. However, lyophilized samples showed the lowest values of the aldehydes hexanal and pentanal.
In conclusion, the investigated cricket powders showed microbiological stability during the one year shelf-life, however some concerns were raised about the chemical stability related to lipid oxidation and subsequent aldehydes’ formation. Considering our results and those reported in literature, killing, drying, and powder production processes can play a key role in the oxidative status of the final products.
For this reason, post-rearing protocols should be implemented for each edible insect species, taking into consideration the nutritional profile (especially the lipid profile) and the biological characteristics, in order to avoid loss of quality of the processed insect derivatives.
These results open an interesting scenario for a long period storage of insect powder at room temperature, making the insect supply chain a sustainable option within food systems.
Data availability statement
The raw data supporting the conclusions of this article will be made available by the authors, without undue reservation.
Author contributions
FM and AT contributed to the methodology and data presentation, wrote the original draft, and edited the manuscript. CZ contributed to the methodology and data presentation. SM and CJ reared and processed the insects. RP supervised the work. AR was responsible for funding acquisition. SB supervised the work and was responsible for funding acquisition. All authors contributed to the article and approved the submitted version.
Funding
This research was funded by CARIPLO Foundation within the Project “Modello Allevamento di Insetti Commestibili—MAIC.”
Acknowledgments
The authors thank Andrea Mascaretti from Centro per lo Sviluppo Sostenibile of Milan, Italy.
Conflict of interest
The authors declare that the research was conducted in the absence of any commercial or financial relationships that could be construed as a potential conflict of interest.
Publisher’s note
All claims expressed in this article are solely those of the authors and do not necessarily represent those of their affiliated organizations, or those of the publisher, the editors and the reviewers. Any product that may be evaluated in this article, or claim that may be made by its manufacturer, is not guaranteed or endorsed by the publisher.
Supplementary material
The Supplementary material for this article can be found online at: https://www.frontiersin.org/articles/10.3389/fsufs.2023.1179088/full#supplementary-material
References
Belitz, H. D., Grosch, W., and Schieberle, P. (2009). Food chemistry. Food Chem., 1–1070. doi: 10.1007/978-3-540-69934-7
Belluco, S., Kinyuru, J., and Fisher, B. L. (2022). Editorial: insects: A source of safe and sustainable food? vol. 6 Front. Sustain. Food Syst., 6. doi: 10.3389/fsufs.2022.887289
Bonazzi, C., and Dumoulin, E. (2014). Quality changes in food materials as influenced by drying processes. Mod. Dry. Technol., 1–20. doi: 10.1002/9783527631728.ch14
Botella-Martínez, C., Lucas-González, R., Pérez-Álvarez, J. A., Fernández-López, J., and Viuda-Martos, M. (2020). Assessment of chemical composition and antioxidant properties of defatted flours obtained from several edible insects. Food Sci. Technol. Int. 27, 383–391. doi: 10.1177/1082013220958854
Boulos, S., Tännler, A., and Nyström, L. (2020). Nitrogen-to-protein conversion factors for edible insects on the Swiss market: T. molitor, A. domesticus, and L. migratoria. Front. Nutr. 7:89. doi: 10.3389/fnut.2020.00089
Caligiani, A., Marseglia, A., Sorci, A., Bonzanini, F., Lolli, V., Maistrello, L., et al. (2019). Influence of the killing method of the black soldier fly on its lipid composition. Food Res. Int. 116, 276–282. doi: 10.1016/j.foodres.2018.08.033
Chavunduka, D. M. (1976). Insects as a source of protein to the African. Rhod. Sci. News 9, 217–220.
Chou, S., and Chin, C.-K. (1994). Control of the Production of cis −3-Hexenal, Lipid-Derived Flavor Compound, by Plant Cell Culture, 282–291.
Domínguez, R., Pateiro, M., Gagaoua, M., Barba, F. J., Zhang, W., and Lorenzo, J. M. (2019). A comprehensive review on lipid oxidation in meat and meat products. Antioxidants 8, 428–429. doi: 10.3390/antiox8100429
European Commission (EC) (2005). Commission regulation (EC) no 2074/2005 of 5 December 2005 aying down implementing measures for certain products under regulation (EC) no 853/2004 of the European Parliament and of the council and for the organisation of official controls under regulation. Official Journal of the European Union, 338/27.
European Commission (EU) (2015). Regulation (EU) 2015/2283 of the European Parliament and of the council of 25 November 2015 on novel foods, amending regulation (EU) no 1169/2011 of the European Parliament and of the council and repealing regulation (EC) no 258/97 of the European Parliam. Official Journal of the European Union, 327.
European Commission (EU) (2017). Commission implementing regulation (EU) 2017/2470 of 20 December 2017 establishing the union list of novel foods in accordance with regulation (EU) 2015/2283 of the European Parliament and of the council on novel foods. Official Journal of the European Union, 351.
Galanakis, C. (2015). Food Waste Recovery: Processing Technologies and Industrial Techniques Acadamic Press.
García-Llatas, G., Lagarda, M. J., Romero, F., Abellán, P., and Farré, R. (2007). A headspace solid-phase microextraction method of use in monitoring hexanal and pentane during storage: application to liquid infant foods and powdered infant formulas. Food Chem. 101, 1078–1086. doi: 10.1016/j.foodchem.2006.03.007
Grapes, M., Whiting, P., and Dinan, L. (1989). Fatty acid and lipid analysis of the house cricket, Acheta domesticus. Insect Biochem. 19, 767–774. doi: 10.1016/0020-1790(89)90058-9
Grebenteuch, S., Kroh, L. W., Drusch, S., and Rohn, S. (2021). Formation of secondary and tertiary volatile compounds resulting from the lipid oxidation of rapeseed oil. Foods 10:2417. doi: 10.3390/foods10102417
Hernández-Álvarez, A. J., Mondor, M., Piña-Domínguez, I. A., Sánchez-Velázquez, O. A., and Melgar Lalanne, G. (2021). Drying technologies for edible insects and their derived ingredients. Dry. Technol. 39, 1991–2009. doi: 10.1080/07373937.2021.1915796
Holman, B. W. B., Bekhit, A. E. D. A., Waller, M., Bailes, K. L., Kerr, M. J., and Hopkins, D. L. (2021). The association between total volatile basic nitrogen (TVB-N) concentration and other biomarkers of quality and spoilage for vacuum packaged beef. Meat Sci. 179:108551. doi: 10.1016/j.meatsci.2021.108551
ISO 18787 (2017). Foodstuffs—Determination of water activity [WWW document], 2017. Available at: https://www.iso.org/standard/63379.html
Jongema, Y. (2015). World List of Edible Insects [WWW document]. Available at: https://www.wur.nl/upload_mm/7/4/1/ca8baa25-b035-4bd2-9fdc-a7df1405519a_WORLD LIST EDIBLE INSECTS 2015.pdf
Jucker, C., Belluco, S., Oddon, S. B., Ricci, A., Bonizzi, L., Lupi, D., et al. (2021). Impact of some local organic by-products on Acheta domesticus growth and meal production. J. Insects Food Feed 8, 631–640. doi: 10.3920/JIFF2021.0121
Kamau, E., Mutungi, C., Kinyuru, J., Imathiu, S., Tanga, C., Affognon, H., et al. (2018). Moisture adsorption properties and shelf-life estimation of dried and pulverised edible house cricket Acheta domesticus (L.) and black soldier fly larvae Hermetia illucens (L.). Food Res. Int. 106, 420–427. doi: 10.1016/j.foodres.2018.01.012
Kemsawasd, V., Inthachat, W., Suttisansanee, U., and Temviriyanukul, P. (2022). Road to the red carpet of edible crickets through integration into the human food chain with biofunctions and sustainability: a review. Int. J. Mol. Sci. 23, 1801–1823. doi: 10.3390/ijms23031801
Khatun, H., Claes, J., Smets, R., De Winne, A., Akhtaruzzaman, M., and Van Der Borght, M. (2021). Characterization of freeze-dried, oven-dried and blanched house crickets (Acheta domesticus) and Jamaican field crickets (Gryllus assimilis) by means of their physicochemical properties and volatile compounds. Eur. Food Res. Technol. 247, 1291–1305. doi: 10.1007/s00217-021-03709-x
Kröncke, N., Grebenteuch, S., Keil, C., Demtröder, S., Kroh, L., Thünemann, A. F., et al. (2019). Effect of different drying methods on nutrient quality of the yellow mealworm (Tenebrio molitor L.). Insects 10:84. doi: 10.3390/insects10040084
Kulma, M., Kouřimská, L., Plachý, V., Božik, M., Adámková, A., and Vrabec, V. (2019). Effect of sex on the nutritional value of house cricket, Acheta domestica L. Food Chem. 272, 267–272. doi: 10.1016/j.foodchem.2018.08.049
La Scalia, G., Micale, R., Miglietta, P. P., and Toma, P. (2019). Reducing waste and ecological impacts through a sustainable and efficient management of perishable food based on the Monte Carlo simulation. Ecol. Indic. 97, 363–371. doi: 10.1016/j.ecolind.2018.10.041
Lanciotti, R., Corbo, M. R., Gardini, F., Sinigaglia, M., and Guerzoni, M. E. (1999). Effect of hexanal on the shelf life of fresh apple slices. J. Agric. Food Chem. 47, 4769–4776. doi: 10.1021/jf990611e
Lenaerts, S., Van Der Borght, M., Callens, A., and Van Campenhout, L. (2018). Suitability of microwave drying for mealworms (Tenebrio molitor) as alternative to freeze drying: impact on nutritional quality and colour. Food Chem. 254, 129–136. doi: 10.1016/j.foodchem.2018.02.006
Marzoli, F., Tata, A., Massaro, A., Bragolusi, M., Passabì, E., Cappellozza, S., et al. (2023). Microbiological and chemical safety of Bombyx mori farmed in North-Eastern Italy as a novel food source. J. Insect. Food Feed, 1–16. doi: 10.3920/JIFF2022.0141
Masson, M. L. (2014). Studies on freeze-drying of foods. SpringerBriefs Appl. Sci. Technol., 19–28. doi: 10.1007/978-3-319-08207-3_3
Medina-Meza, I. G., Barnaba, C., and Barbosa-Cánovas, G. V. (2014). Effects of high pressure processing on lipid oxidation: a review. Innov. Food Sci. Emerg. Technol. 22, 1–10. doi: 10.1016/j.ifset.2013.10.012
Meyer-Rochow, V. (1975). Can insects help to ease the problem of world food shortage? Search 6, 261–262.
Mishyna, M., Haber, M., Benjamin, O., Martinez, J. J. I., and Chen, J. (2020). Drying methods differentially alter volatile profiles of edible locusts and silkworms. J. Insects Food Feed 6, 405–415. doi: 10.3920/JIFF2019.0046
Modi, V. K., Sachindra, N. M., Nagegowda, P., Mahendrakar, N. S., and Narasimha Rao, D. (2007). Quality changes during the storage of dehydrated chicken kebab mix. Int. J. Food Sci. Technol. 42, 827–835. doi: 10.1111/j.1365-2621.2007.01291.x
Parikh, G., Rawtani, D., and Khatri, N. (2020). Insects as an indicator for environmental pollution. Environ. Claims J. 33, 161–181. doi: 10.1080/10406026.2020.1780698
Perez-Santaescolastica, C., De Winne, A., Devaere, J., and Fraeye, I. (2022). The flavour of edible insects: a comprehensive review on volatile compounds and their analytical assessment. Trends Food Sci. Technol. 127, 352–367. doi: 10.1016/j.tifs.2022.07.011
Shahidi, F. (1994). Assessment of lipid oxidation and off-flavour development in meat and meat products. Flavor Meat Meat Prod., 247–266. doi: 10.1007/978-1-4615-2177-8_14
Shahidi, F., and Pegg, R.B. (1994). Hexanal as an indicator of the flavor deterioration of meat and meat products, 256–279.
Singh, Y., Cullere, M., Kovitvadhi, A., Chundang, P., and Dalle Zotte, A. (2020). Effect of different killing methods on physicochemical traits, nutritional characteristics, in vitro human digestibility and oxidative stability during storage of the house cricket (Acheta domesticus L.). Innov. Food Sci. Emerg. Technol. 65:102444. doi: 10.1016/j.ifset.2020.102444
Tapia, M. S., Alzamora, S. M., and Chirife, J. (2020). “Effects of water activity (aw) on microbial stability as a hurdle in food preservation” in Water Activity in Foods: Fundamentals and Applications. eds. G. V. Barbosa-Cánovas, A. J. Fontana Jr., S. J. Schmidt, and T. P. Labuza (United States: John Wiley & Sons)
Tata, A., Massaro, A., Marzoli, F., Miano, B., Bragolusi, M., Piro, R., et al. (2022). Authentication of edible insects’ powders by the combination of DART-HRMS signatures: the first application of ambient mass spectrometry to screening of novel food. Foods 11:2264. doi: 10.3390/foods11152264
UNI EN 15763 (2010). Foodstuffs—determination of trace elements—determination of arsenic, cadmium, mercury and lead in foodstuffs by inductively coupled plasma mass spectrometry (ICP-MS) after pressure digestion [WWW Document], 2010. Available at: https://infostore.saiglobal.com/en-us/standards/uni-en-15763-2010-1093145_saig_uni_uni_2544252/
van Huis, A. (2013). Potential of insects as food and feed in assuring food security. Annu. Rev. Entomol. 58, 563–583. doi: 10.1146/annurev-ento-120811-153704
van Huis, A. (2020). Insects as food and feed, a new emerging agricultural sector: a review. J. Insect. Food Feed 6, 27–44. doi: 10.3920/JIFF2019.0017
van Huis, A., van Itterbeeck, J., Klunder, H., Mertens, E., Halloran, A., Muir, G., et al. (2013). Edible insects—Future prospects for food and feed security, FAO forestry paper. FAO
Vandeweyer, D., Crauwels, S., Lievens, B., and Van Campenhout, L. (2017). Microbial counts of mealworm larvae (Tenebrio molitor) and crickets (Acheta domesticus and Gryllodes sigillatus) from different rearing companies and different production batches. Int. J. Food Microbiol. 242, 13–18. doi: 10.1016/j.ijfoodmicro.2016.11.007
Zamora, R., and Hidalgo, F. J. (2007). Coordinate contribution of lipid oxidation and maillard reaction to the nonenzymatic food browning. Crit. Rev. Food Sci. Nutr. 45, 49–59. doi: 10.1080/10408690590900117
Keywords: edible insect, house cricket, volatile compound, fat oxidation, protein content, microbiological safety
Citation: Marzoli F, Tata A, Zacometti C, Malabusini S, Jucker C, Piro R, Ricci A and Belluco S (2023) Microbial and chemical stability of Acheta domesticus powder during one year storage period at room temperature. Front. Sustain. Food Syst. 7:1179088. doi: 10.3389/fsufs.2023.1179088
Edited by:
Li Min, Guangdong Academy of Agricultural Sciences (GDAAS), ChinaReviewed by:
Ma Lukai, Zhongkai University of Agriculture and Engineering, ChinaMilan Zivko Baltić, University of Belgrade, Serbia
Copyright © 2023 Marzoli, Tata, Zacometti, Malabusini, Jucker, Piro, Ricci and Belluco. This is an open-access article distributed under the terms of the Creative Commons Attribution License (CC BY). The use, distribution or reproduction in other forums is permitted, provided the original author(s) and the copyright owner(s) are credited and that the original publication in this journal is cited, in accordance with accepted academic practice. No use, distribution or reproduction is permitted which does not comply with these terms.
*Correspondence: Carmela Zacometti, Y3phY29tZXR0aUBpenN2ZW5lemllLml0
†These authors have contributed equally to this work and share first authorship