- 1CIIMAR, Centro Interdisciplinar de Investigação Marinha e Ambiental, Universidade do Porto, Terminal de Cruzeiros do Porto de Leixões, Av. General Norton de Matos, S/N, Matosinhos, Portugal
- 2ICBAS, Instituto de Ciências Biomédicas de Abel Salazar, Universidade do Porto, Porto, Portugal
- 3ALGAplus, Produção e Comercialização de Algas e seus Derivados Ltd., Ílhavo, Portugal
- 4SPAROS Lda, Área Empresarial de Marim, Lote C, Olhão, Portugal
- 5RIASEARCH Lda, Cais da Ribeira de Pardelhas, Murtosa, Portugal
This study evaluated the replacement of fish oil (sardine oil) by different combinations of alternative marine lipid ingredients as sources of eicosapentaenoic acid (EPA) and docosahexaenoic acid (DHA) in European sea bass (Dicentrarchus labrax) throughout 14 days of ice storage. A practical diet (SARDINE) was used as a control, which included 9% sardine oil and 4.4% soybean oil, providing 2.3% of EPA + DHA. Two other experimental diets were formulated to achieve the same EPA + DHA values but completely devoid of soybean oil. In the ALGABLEND diet, 6.7% of salmon oil (salmon by-product) and 2% of algae biomass (Algaessence Feed™) partially replaced sardine oil. In the ALGAOIL diet, sardine oil was totally replaced with 10.1% salmon by-product oil and 3.3% algae oil (Veramaris®). All diets were equally well-accepted by European sea bass, resulting in similar growth performance, somatic indexes, and whole body composition. At the end of the trial, no significant differences were found in the EPA + DHA levels of fish muscle between dietary treatments, resulting in high values of EPA + DHA (> 0.62 g 100 g−1). Furthermore, replacing fish oil did not significantly affect the organoleptic and chemical properties of the fish samples. Parameters such as pH, water holding capacity, lipid oxidation, antioxidant capacity, color and texture presented similar values to those obtained for fish fed the SARDINE diet during the 14 days of storage in ice. In conclusion, these results show that combining algal oil (Veramaris®), algae blend (Algaessence Feed™) and salmon by-product oil can be a successful strategy for the fortification of European sea bass muscle in EPA and DHA while ensuring fish freshness, nutritional quality, and consumers’ health.
1. Introduction
Fish is an important source of essential macronutrients, vitamins and minerals, being our primary source of omega-3 long-chain polyunsaturated fatty acids (n-3 LC-PUFA), specifically the omega-3 fatty acids EPA (eicosapentaenoic acid; C20:5n-3) and DHA (docosahexaenoic acid; C22:6n-3) (Maulu et al., 2021). Eating fish is associated with the prevention of cardiovascular diseases, improved immune function, brain development in infants, and mental and metabolic health in adults (Larsen et al., 2011; Ruxton, 2011; Li et al., 2020; Maulu et al., 2021). In fact, strong evidence shows that consuming fish regularly, more than one fatty fish meal per week, can lower the risk of primary cardiac arrest by 50% and prevents brain disorders that are dramatically increasing worldwide (Raatz et al., 2013; Kokubun et al., 2020). Therefore, the European Food Safety Authority recommends a daily intake between 0.25 and 0.50 g of EPA + DHA to prevent cardiovascular accident risk in European adults (EFSA, 2012). Aquaculture will play an increasing role in meeting this recommendation since it contributes to nearly half of the global fish consumption (FAO, 2020). In the future, the importance of tailoring fish diets to create value-added products that meet consumer expectations, coupled with a heightened emphasis on food fortification and food safety, will elevate nutrition as a crucial control point in aquaculture (Glencross et al., 2023).
Aquaculture has been growing leading to an increasing global demand for fish meal (FM) and fish oil (FO), key protein and lipid sources in aquafeeds, derived from either forage (reduction) fishery resources or aquaculture by-products (Glencross et al., 2023; Newton et al., 2023). But the use of fish meal and fish oil in aquafeeds has been globally decreasing, and reached a peak of approximately 3.6 million tonnes per annum and 0.9 million tonnes per annum respectively, as reported by Glencross et al. (2023). Although Newton et al. (2023) have recently shown that marine ingredients tend to have a lower environmental footprint compared to terrestrial ingredients, Life Cycle Assessments (LCA) do not account for the stock status of fisheries. Additionally, commonly vegetable lipid sources like soybean oil (SyO) are witnessing a price surge (Nagappan et al., 2021). This rise in ingredient costs, coupled with the overexploitation of small pelagic fish in some regions, has created financial and environmental challenges to the feed industry (Montero et al., 2005; Nagappan et al., 2021). Therefore, identifying sustainable and suitable ingredients for aquafeeds to meet the increasing global aquaculture production, while still delivering healthy and safe products to consumers is a priority.
Several studies have shown that vegetable oils (VO) do not affect the growth performance and feed conversion of freshwater and marine fish feeds, and can hence be good alternative dietary lipid sources (Bell et al., 2001; Rosenlund et al., 2001; Turchini et al., 2009; Dikel et al., 2013; Pereira et al., 2019). However, others studies have reported negative effects of VO on fish health and disease resistance (Montero and Izquierdo, 2010; Nasopoulou and Zabetakis, 2012). In European sea bass (Dicentrarchus labrax), a major species in Mediterranean aquaculture, the use of SyO has been the focus of several studies, with survival, growth performance and feed intake not being compromised when used to partially replace FO (Izquierdo et al., 2003; Montero et al., 2005; Dikel et al., 2013). In fact, several VO can replace up to 60% of the FO in European sea bass diets without compromising their growth and feed utilization, namely if essential fatty acids requirements are met (Izquierdo et al., 2003). However, the use of plant based-substitutes may lead to significant changes in the nutritional quality of fillets, including a significant decrease in the n-3/n-6 ratio and lower levels of n-3 LC-PUFA, due to the absence of EPA and DHA in vegetable sources (Izquierdo et al., 2005a; Shah et al., 2018). The inclusion of certain VO in marine fish diets can compromise not only fish health and nutritional quality, but also sensory properties (Glencross, 2009; Castro et al., 2022), such as appearance, odour, flavour, and texture by altering the muscle fatty acid (FA) profiles (Turchini et al., 2004, 2007; Grigorakis et al., 2009Alexi et al., 2017). For instance, fish fed with diets high in omega-6 polyunsaturated fatty acids (n-6 PUFA) such as plant based-diets had increased level of these fatty acids (FAs) in their flesh, leading to an increase in the relative amount of n-6 PUFA-derived volatile aldehydes, which negatively impacted the general aroma of fish muscle and produced an off-flavour attribute in sensory analysis (Turchini et al., 2004, 2007).
Some of the limitations associated with poor quality of fish muscle after the dietary inclusion of plant based-substitutes of FO can be overcome by including micro - and macroalgae in the feed. As an example, a diet for gilthead seabream (Sparus aurata), was formulated with a combination of Chlorella sp., Tetraselmis sp., and DHA-rich Schizochytrium sp. replacing 33% of FM and resulted in effective muscle accumulation of EPA + DHA (Ferreira et al., 2022). Besides their richness in n-3 LC-PUFA, algae also contain various bioactive compounds that can enhance antioxidant properties and delay lipid peroxidation, improve sensory attributes and prolong the shelf life of fish post-harvest (Wells et al., 2017; Ben Atitallah et al., 2019; Katerina et al., 2020; Annamalai et al., 2021; Chen et al., 2021; Nagappan et al., 2021; Tavakoli et al., 2022). Microalgae are also rich in minerals as selenium that have been shown to greatly minimize gaping (Tavakoli et al., 2022). For instance, the addition of just 5% Schizochytrium sp. to Atlantic salmon (Salmo salar) feed not only improved EPA + DHA retention efficiency, but also reduced muscle gaping and improved fillet quality (Kousoulaki et al., 2016). The use of Schizochytrium oil (Veramaris ®) in FO-free diets improved the taste of Hawaiian yellowtail (Seriola rivoliana) without affecting fillet yield or survival (Meigs et al., 2020). Likewise, Takyar et al. (2019) observed improved fillet quality in rainbow trout (Oncorhynchus mykiss) when the diet included extracts of Spirulina latensis and Chlorella vulgaris, and in addition, a significant delay in lipid oxidation and an extension of shelf life when stored at 4°C was also observed. Furthermore, another study by Ben Atitallah et al. (2019) indicated that even a small inclusion of microalgae (specifically Isochrysis, Picochlorum, and Chlorella) at just 1% can enhance the water holding capacity (WHC), thereby reducing dehydration during storage.
Despite several promising results, the application of microalgal biomass or extracted oils as a source of n-3 LC-PUFA faces challenges due to high production costs (Liu et al., 2022). Still, their use at low inclusion levels in combination with recycling activities, such as FO production from fish waste or agro-industry by-products, can be a cost-effective strategy to provide alternative n-3 LC-PUFA for aquafeeds. The Atlantic salmon is an excellent example, accounting for 35% of the overall estimated consumption of aquaculture products, and a recent study showed that it demonstrated high lipid content in heads (21.5%), frames (17.2%), and trimmings (26.4%) (Malcorps et al., 2021). In fact, Malcorps et al. (2021) observed that the highest EPA + DHA were observed for heads (1.53 g 100 g−1), followed by trimmings (1.74 g 100 g−1), skin (1.21 g 100 g−1) and viscera (1.10 g 100 g−1). This highlights its significant industry potential, namely in the utilization of various by-products in aquafeeds (Stevens et al., 2018; Malcorps et al., 2021). Such by-product can be further processed to manufacture cost-effective oils for aquafeeds.
The present study aimed to assess the feasibility of replacing high quality FO – mainly sardine oil (SdO) – by alternative marine lipid sources, while maintaining European sea bass growth performance and fillet nutritional value for consumers. In addition, organoleptic and chemical quality traits were evaluated during 14 days of cold storage.
2. Materials and methods
2.1. Ethical statement
The experiments with fish were approved by the Ethical Committee of Riasearch Lda (Murtosa, Portugal) in compliance with the National Competence Authority and the guidelines of the European Union Directive 2010/63/EU.
2.2. Ingredients and experimental diets
Commercially available salmon by-product oil (SmO; Sopropêche, France), algae oil (AO; Veramaris®, Blair, NE, United States) and Algaessence Feed™ (AF, a blend of macro- and microalgae species Gracilaria sp., Nanochloropsis sp. and Shizochitrium sp.; ALGAplus Lda., Portugal), were selected based on their n-3 LC-PUFA richness and economic feasibility to replace FO (mainly SdO), still ensuring high EPA and DHA levels (Table 1). Based on the nutritional requirements of European sea bass (NRC, 2011), three isoproteic (54% dry matter, DM), isolipidic (18% DM) and isoenergetic (24 kJ g−1 DM) diets were formulated with moderate levels of protein from marine ingredients (15.0% FM and 3.5% hydrolyzed fish protein), 10.0% of poultry meal and different mixtures of lipid sources (Table 2). A commercially based diet was used as control - SARDINE - with 9.0% of SdO and 4.4% of soybean oil (SyO). The other two experimental diets were completely devoid of SyO, while SdO was replaced as follows: in ALGABLEND, SdO was partially replaced by 6.2% of SmO and 2% of AF; in ALGAOIL diet, SdO was totally replaced by a 10.1% of SmO and 3.3% of AO. All experimental diets were supplemented with mono-calcium phosphate, L-lysine and DL-methionine, and were extruded and produced by SPAROS Lda. (Olhão, Portugal). Proximate composition and FA profile of the experimental diets are presented in Tables 2 3 respectively.
2.3. Growth trial and fish sampling
The growth trial was performed in Riasearch Lda. (Murtosa, Portugal), with European sea bass juveniles obtained from a commercial fish farm. Fish were individually weighed (g) and 12 homogeneous groups of 20 fish (71.3 ± 13.2 g) were randomly distributed by 350 L fiberglass tanks within a saltwater recirculation system (water temperature of 21°C, salinity of 18‰, flow rate at 700 L/h (200% water renewal/h) and 12 h light/12 h dark photoperiod regime). Levels of total ammonium (NH4+), nitrite (NO2−) and nitrate (NO3−), as well as pH were daily monitored to ensure levels within the recommended ranges for marine species (NH4+ < 0.05 mg L−1; NO2− < 0.5 mg L−1; NO3− < 5 mg L−1; 7.5 < pH ≤ 8). Each diet was randomly assigned to quadruplicate groups of fish, which were hand fed until visual apparent satiation, three times daily, 7 days a week, for 116 days.
Twenty fish from the initial stock, after 48 h fasting period, were sacrificed by an ice bath and weighed (g). Ten of these fish were collected and stored at −20°C for whole body composition analysis; dorsal muscle was collected from another 10 fish, immediately frozen in dry ice, and stored at −80°C for further analysis of dry matter, lipid content and FA profile.
After the feeding trial, all fish were sacrificed by an ice bath and individually weighed (g) for further calculation of growth performance parameters after 48 h fasting period. Four fish from each tank (16 fish per dietary treatment) were collected and stored at −20°C for whole body proximate composition analysis. Other 4 fish from each tank were sampled to collect the viscera and liver to determine the viscerosomatic and hepatosomatic indexes (VSI and HSI). The right dorsal muscle (1 g) was collected and immediately frozen in dry ice and stored at −80°C for analysis of dry matter, lipid content and FA profile. The left muscle from the same fish was used for pH, water holding capacity (WHC), antioxidant capacity, instrumental color, and texture evaluation. Additionally, 12 fish per tank were collected and immediately placed intact in new polystyrene boxes, covered with ice, for shelf-life evaluation over 14 days. Four fish from each tank were sampled on days 3, 7 and 14 for muscle evaluation of pH, WHC, antioxidant capacity, instrumental color and texture (Figure 1).
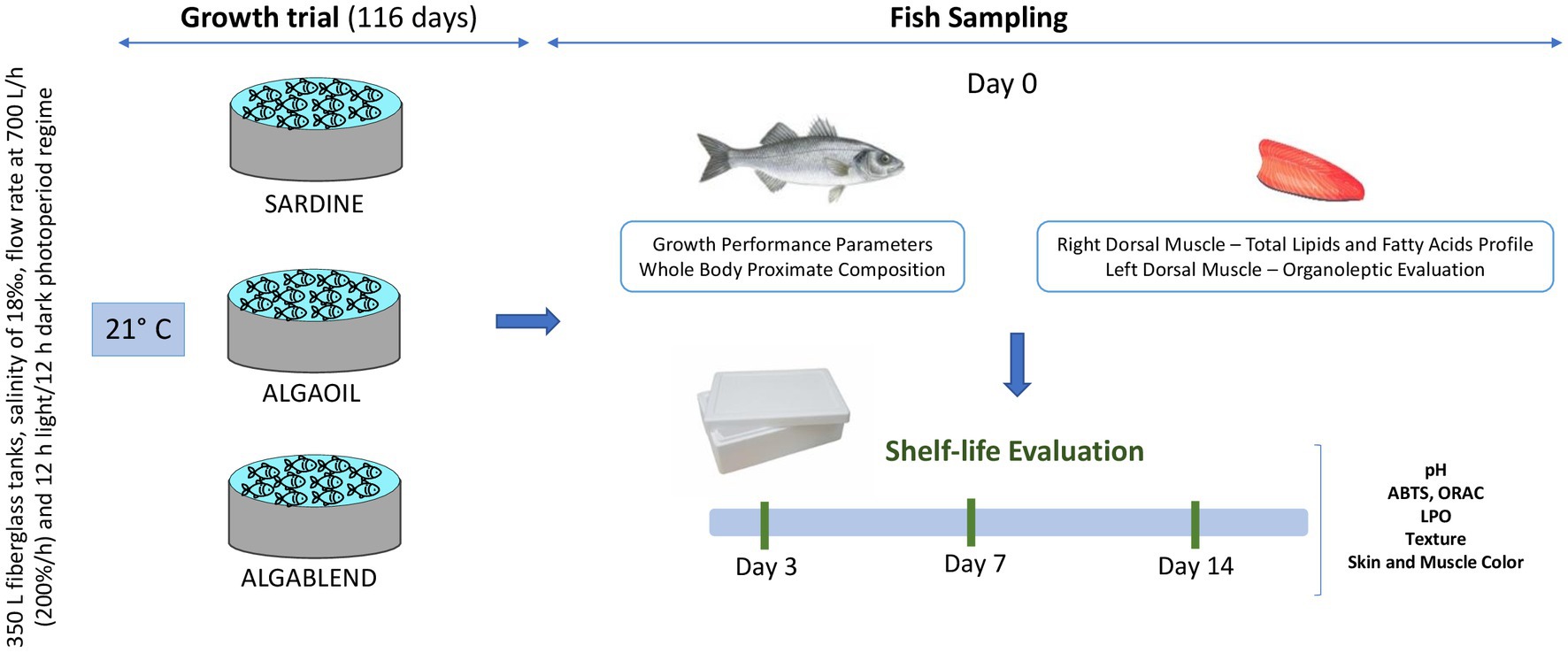
Figure 1. Schematic representation of the experimental design. Fish were fed with three experimental diets (SARDINE; ALGABLEND and ALGAOIL) for 116 days. At the end of the trial, fish were sampled for growth performance parameters and whole body proximate composition analysis. The right dorsal muscle was collected for analysis of dry matter, lipid content and fatty acid profile while the left muscle from the same fish was used for pH, water holding capacity (WHC), antioxidant capacity, instrumental color, and texture evaluation. Additionally, 12 fish per tank were collected and immediately placed intact in new polystyrene boxes, covered with ice, for shelf-life evaluation over 14 days. Four fish from each tank were sampled on days 3, 7 and 14 for muscle evaluation of pH, WHC, antioxidant capacity, instrumental color and texture.
2.4. Proximate composition analysis
The three experimental diets and one pool of 4 fish per tank from each treatment (n = 4) were ground, homogenized and freeze-dried before being analyzed for ash, protein, lipids and energy. Proximate composition analyses followed AOAC (2006) methods: dry matter (in an oven at 105°C for 24 h); ash (incineration in a muffle furnace at 550°C for 6 h; Nabertherm L9/11/B170, Bremen, Germany); crude protein by quantification of nitrogen (N) using a Leco nitrogen analyzer (Model FP-528; Leco Corporation, St. Joseph, United States) and conversion (N × 6.25) to equivalent protein; crude fat (petroleum ether extraction), using a Soxtec extractor (Model ST 2055 Soxtec™; FOSS, Hillerod, Denmark) and gross energy determined in an adiabatic bomb calorimetric system (Model Werke C2000, IKA, Staufen, Germany).
2.5. Lipid content and fatty acid analysis
Total muscle lipids were extracted and quantified gravimetrically using a Soxtec extractor (Model ST 2055 Soxtec™; FOSS, Hillerod, Denmark). The FA from experimental diets and muscles were transmethylated by direct acidic methylation according to Parrish et al. (2015), with some modifications. Briefly, 1 mg mL−1 or 0.5 mg mL−1 of an internal standard solution of tricosanoic acid (C23:0; Capillary GC, purity ≥99%, Sigma – Aldrich, United States) dissolved in toluene was added to ingredients, diets and muscle samples. The samples were then transmethylated by adding 3 mL of methylation solution prepared with methanol:dicloromethane:HCL (10:1:1, v/v/v) and heated at 100°C for 1 h. After cooling at room temperature, 1.8 mL of extraction solution n-Hexane:dicloromethane (4:1 v/v) and 5 mg of BHT were added. Samples were vortexed and centrifuged at 700 g for 5 min at room temperature, and the upper organic layer containing the fatty acid methyl esters (FAME) was carefully transferred to another tube with 1 g of anhydrous sodium sulfate. FAME were placed in a new tube heated at 37°C and dried under a stream of nitrogen gas. Finally, FAME were recovered in 1.5 mL of n-hexane and analyzed in a gas chromatograph, using a Shimadzu Nexis GC-2030 gas chromatograph (Kyoto, Japan) equipped with a flame-ionization detector (FID) and a Shimadzu AOC-20i auto-injector. Separation was carried out on an OmegaWax 250 capillary column (30 m × 0.25 mm I.D., film thickness 0.25 μm). Operating conditions were as follows: split mode, with a split ratio of 1:50 and an injection volume of 1 μL. The injector and detector temperatures were kept at 250 and 280°C, respectively. A flow rate of 25 mL min−1 of helium as a carrier gas, 40 mL min−1 of hydrogen and 400 mL min−1 of air were provided. The column thermal gradient was as follows: initial temperature 50°C for 2 min, which was increased at 50°C min−1 to 174°C, hold for 14 min, then increased 2°C min−1 to 210°C, hold for 50 min. FAME were identified by comparison of retention times with a known standard mixture (Sigma 47,885-U Supelco 37 Component FAME Mix, United States) and quantified using the software GC solution for GC systems (Shimadzu, Kyoto, Japan). FAME contents in ingredients, diets and muscles were expressed as % of total FAME. The amount of FA expressed in g 100 g−1 of edible part, were calculated using an internal standard (C23:0) as a reference, according to Joseph and Ackman (1992).
2.6. Color and instrumental texture evaluation
Skin and muscle color measurements were performed immediately after sampling in fish from day 0, and after storage in ice in fish from days 3, 7 and 14 as described by Marques et al. (2022). All measurements were made at room temperature with a CR-400 chroma meter (Konica Minolta Inc., Osaka, Japan), with an aperture of 8 mm, and standard illuminant D65 using the CIE 1976 (L*, lightness; a*, redness; b*, yellowness). Primarily, the equipment was calibrated with a white plate reference standard. Color parameters were measured at three points above the lateral line by applying the colorimeter to the raw skin and flesh of the 16 fish per dietary treatment. After flashing, L*, a*, and b* values were recorded. To estimate the overall changes in color during storage time, Delta E value (ΔE = [ΔL*^2 + Δa*^2 + Δb*^2] ^1/2) was calculated based on L*, a* and b* values determined at day 0 (Oliveira and Balaban, 2006).
Texture profile analysis (TPA) was measured using a TA.XT plus Texture Analyzer (Stable Micro System, Godalming, UK) equipped with a 5 kg load cell. All measurements were carried out at room temperature, applying a cylindrical 2.0 mm diameter probe. Each sample was compressed twice (probe speed of 1 mm s−1; probe penetration depth of 4 mm; wait time between penetrations of 5 s) on the thickest part, and at three different points of each raw fillet to obtain an average value for each parameter [hardness (N), adhesiveness (J), springiness (−), cohesiveness (−), chewiness (J), and resilience (−)] (Batista et al., 2020). The Exponent software package, version 6.1.10.0 (Stable Micro Systems, Godalming, United Kingdom), was used to calculate all parameters.
2.7. pH and WHC
Muscle pH and WHC, two indicators of fillet quality, were evaluated immediately after fish sampling at day 0, and after storage in ice for 3, 7 and 14 days (16 fish per dietary treatment at each sampling point). All pH measurements were carried out at room temperature through a digital flat tip pH electrode for food surfaces (model HI 14140, Hanna Instruments, Rhode Island, United States). Muscle pH was measured at three different points of each raw fillet to obtain an average value by applying the pH probe to the left raw fillet of the 16 fish per dietary treatment.
For WHC analysis, approximately 1 g of raw fillet was placed in 1.5 mL tubes with filter paper. The tubes were centrifuged at 1381 g for 4 min at 4°C (model 5430R, Eppendorf, New York, United States). The samples were weighed after centrifugation and dried at 70°C for 12 h (Goes et al., 2015). The dry samples were weighed once more, and the equation below was used to calculate WHC:
2.8. Evaluation of total antioxidant capacity
Muscle antioxidant capacity was evaluated as described by Valente et al. (2015) with minor modifications. Approximately 350 mg of fish muscle hydrolysates from days 0, 3, 7 and 14 (16 fish per dietary treatment at each sampling point) were produced by enzymatic hydrolysis with pepsin from pork stomach mucosa (Sigma-Aldrich, Missouri, United States) at an enzyme/substrate ratio of 1:100 (w/w) for 5 h in 0.1 M Glycine–HCl buffer at pH 2.0 and 37°C. After, the mixture was heated in a boiling water bath at 100°C for 5 min to inactivate enzymatic activity. Samples were centrifuged at maximum speed for 5 min at 4°C and the pH of the supernatant was adjusted to 7.0 with NaOH 1 M. All samples were stored at −80°C until further use.
The antioxidant activity of muscle hydrolysates was determined by the radical scavenging potential of [2,2′-azino-bis(3-ethylbenzothiazoline-6-sulfonic acid)] ABTS and peroxyl radical generated by 2,2′-azobis-(2-amidinopropane) hydrochloride AAPH. The ABTS assay was performed according to the protocol described by Gonçalves et al. (2009). Briefly, the concentration of ABTS radical was adjusted to an initial absorbance of 0.700 ± 0.02 at 734 nm. 20 μL of sample or Trolox was added to 180 μL of the ABTS solution in a flat-bottom 96-well microplate. The mixture was incubated for 5 min at 30°C, protected from the light, and the absorbance read at 734 nm. Oxygen-radical absorbance-capacity assay (ORAC-FL), which measures the radical scavenging potential of the peroxyl radical generated by AAPH, was performed in flat-bottomed 96-well microplates, following the method described by Dávalos et al. (2004) with minor modifications. 120 μL of fluorescein solution was added to 20 μL of the sample, and preincubated for 10 min at 37°C. AAPH solution (60 μL) was added rapidly using a multichannel pipette and the fluorescence was recorded every minute for 80 min. The excitation and emission wavelengths were set at 485 and 528 nm, respectively. All measurements were performed using a multi-mode microplate reader (BioTek Synergy™ HTX, Vermont, United States). For both analyses, Trolox was used as a standard curve and the final results were expressed as μmoles of Trolox equivalents (TE) per g wet weight of fish muscle. All the analyses were performed in triplicate.
2.9. Thiobarbituric acid-reactive substances
Lipid peroxidation (LPO) values of fillet samples from 16 fish per dietary treatment were determined by measuring the concentration of thiobarbituric acid-reactive substances (TBARS), mainly composed of malondialdehyde (MDA) (Charles et al., 2022). Homogenized muscle samples (300 mg) were mixed with a solution of 12% trichloroacetic acid, 60 mM of Tris–HCl with diethylenetriaminepentaacetic acid (DTPA) 0.1 mM, and 0.73% of thiobarbituric acid. The mixture was heated at 100°C for 60 min, cooled with ice, and then centrifuged at 13000 g at 4°C for 5 min. The absorbance was measured at 535 nm at 25°C with a multi-mode microplate reader (BioTek Synergy™ HTX, Vermont, United States). The results were expressed as mg of MDA formed per Kg of fresh tissue.
2.10. Calculations
Growth, feed utilization and somatic parameters were calculated as follows: Daily growth index (DGI) =100 x [(final body weight) 1/3 - (initial body weight)1/3] / days of the experiment; Final condition factor (K) = 100 × (FBW / final body length3); Voluntary feed intake (VFI) = 100 × dry feed intake/average body weight / days of experiment; Feed conversion ratio (FCR) = dry feed intake / weight gain; Protein efficiency ratio (PER) = (FBW – IBW) / total protein intake (g); Hepatosomatic index (HSI) = 100 × (liver weight / FBW); Viscerosomatic index (VSI) = 100 × (weight of viscera / FBW).
Nutrient utilization parameters were calculated as follows: Protein (P), Lipids (L) or Energy (E) = (FBW × final carcass P, L or E content) – (IBW × initial carcass P, L or E content / ABW / days of experiment; P, L or E retention = 100 × (FBW × final carcass P, L or E content − IBW × initial carcass P, L or E content) / (dry feed intake × P, L or E content in the diet) (Marques et al., 2022).
2.11. Statistical analysis
All statistical analyses were performed using IBM SPSS® Statistics 27.0.1 software (IBM Corporation, New York, United States). Data were tested for normality and homogeneity of variances by Shapiro–Wilk and Levene’s tests, respectively, and transformed whenever required before being submitted to a one-way ANOVA. When this test showed significance, individual means were compared using HSD Tukey Test. A two-way ANOVA, with two factors, dietary treatment and days of storage in ice (0, 3, 7 and 14) was used to compare fish muscle pH, WHC, antioxidant capacity, LPO, color and texture. In all cases, the level of significance was set at 0.05.
3. Results
3.1. Ingredients and experimental diets
Table 1 shows the results of the FA profile of all lipid sources used to formulate the experimental diets. Differences are observed in all classes of FA, that is, in total SFA, MUFA and PUFA: while SdO, AO and AF displayed higher percentages of SFA (>26%), SmO has a higher percentage of MUFA, mainly due to the richness in oleic acid (C18:1n-9; OA) - 38.7%. PUFA also differed between lipid sources, ranging from 32.4 in SmO to 69.3% in AO. Within PUFA, major differences were noticed in the percentages of C18:2n-6 (linoleic acid; LA) and C18:3n-3 (α-linolenic acid; ALA), which were more than 10x higher in SmO and SyO in comparison to the other lipid sources. SdO and AO were very rich sources of EPA (>19% in SdO and > 18% in AO), while AF and AO were highly rich sources of DHA (> 39% in AF and > 42% in AO). It should be noted that due to the low lipid level of AF biomass (4%), the total amount of DHA (8.4% DM) is much lower than that found in AO (33.6% DM). EPA and DHA were both absent in SyO. These differences were reflected in the sum of EPA and DHA (highest in AO, followed by AF and SdO), in the DHA/EPA ratio (highest in AF) and in the n-3/n-6 ratio (highest in AO, followed by AF and SdO). Subsequently, all experimental diets had different FA profiles (Table 3). The dietary SFA fraction was similar in all diets (24.1–24.7% of total FA), with the exception of the ALGAOIL diet showing the lowest SFA percentage (20.7%). Regarding MUFA, SARDINE diet exhibited the lowest value (24.2%), followed by ALGABLEND (33.4%) and ALGAOIL (36.2%). The sum of PUFA was also variable between experimental diets, with SARDINE having the highest PUFA value (46.8%), followed by ALGAOIL (40.3%) and ALGABLEND (38.1%). As expected EPA was more abundant in SARDINE and ALGABLEND, mostly due to the high EPA content of SdO included at 9.0 and 6.7% in these diets. A 3.3% dietary inclusion of AO in ALGAOIL diet and 2% of AF in ALGABLEND resulted in the highest DHA concentrations of these diets. Such differences were reflected in DHA/EPA ratio, being higher in ALGAOIL (1.9) followed by ALGABLEND (0.9) and SARDINE (0.7). The n-3/n-6 ratio was <1 in SARDINE diet (0.9) and above 1 in ALGABLEND (1.7) and ALGAOIL diet (1.4). All experimental diets had similar percentages of EPA + DHA (15.4 to 16.1% total FA), corresponding to approximately 2.4 g of EPA + DHA per 100 g of dry matter (DM).
3.2. Growth performance, whole body composition and nutrient utilization
All diets were equally well accepted by European sea bass, resulting in similar voluntary feed intake (VFI) and feed conversion ratio (FCR) for all dietary treatments. At the end of the trial, all fish increased their initial body weight by more than double, and no significant differences were observed for final body weight (158.2–167.2 g), daily growth index or condition factor (Table 4). Likewise, HSI and VSI were not significantly affected among dietary treatments (HSI 1.2–1.4% and VSI 7.7–8.1%) (Table 4). The final whole body proximal composition did not differ with the experimental diets. In addition, both nutrient and energy gain, and nutrient retention (protein, lipids and energy) remained similar among groups regardless of the dietary lipid sources tested (Table 4).
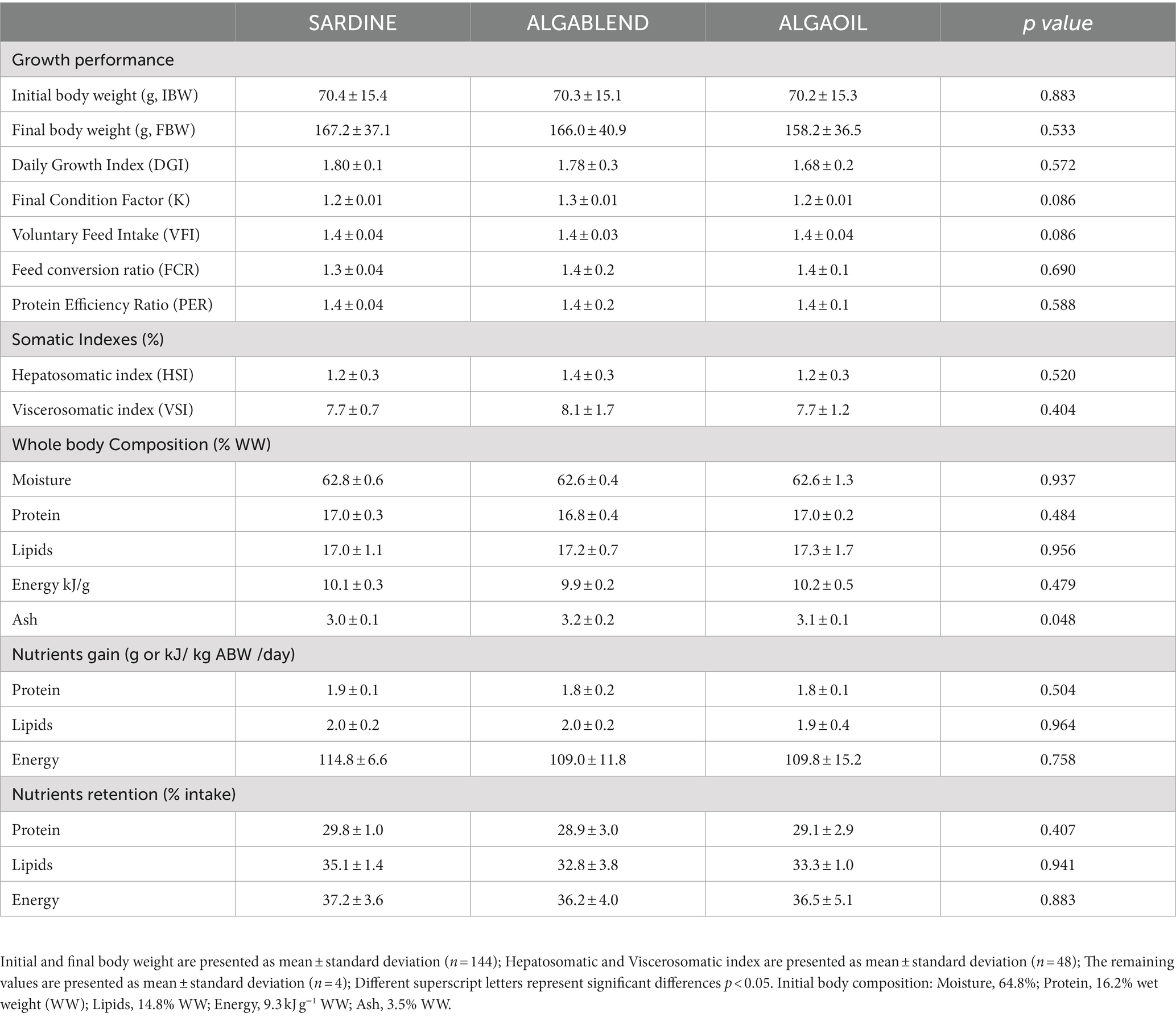
Table 4. Growth performance, somatic indexes, whole-body composition and macro-nutrients gain and retention of European sea bass fed experimental diets.
3.3. Muscle lipid content and fatty acid profile
All fish increased muscle total lipid content from the beginning to the end of the trial, and the final percentage of lipids in muscle weight was not significantly altered by the replacement of SdO with alternative marine lipid sources, ranging between 4.5 and 5.3% (Table 5). However, muscle FA profile was deeply affected by the lipid sources used, namely SFA, MUFA and PUFA due to the differences in the FA composition in of each source (Table 5). SFAs relative levels decreased in muscle of fish fed ALGAOIL; MUFA increased in fish fed ALGABLEND and ALGAOIL in comparison with SARDINE fed fish, mainly due to decreased concentration of C18:1 n-9 (oleic acid; OA). Contrarily, the PUFA relative levels in SARDINE fed fish increased significantly compared with those fed ALGABLEND diet, mainly due to the higher concentration of C18:2 n-6 (linoleic acid; LA) in SARDINE diet, resulting in the highest muscle n-6 PUFA in those fish. Although n-3 PUFA muscle levels remained similar among dietary treatments (values ranged between 20 to 21.6%), the muscle EPA abundance was highest in the SARDINE fed fish (7.2% total FA), followed by the ALGABLEND diet (6.5% total FA) and ALGAOIL diets (4.6% total FA). On the contrary, the highest percentage of DHA was observed in fish fed the ALGAOIL diet (12% total FA) compared to the remaining diets that did not reach 10%. Nevertheless, the muscle fresh weight content of EPA + DHA did not vary among dietary treatments, and all fillets contained more than 0.62 g of EPA + DHA per 100 g of wet weight.
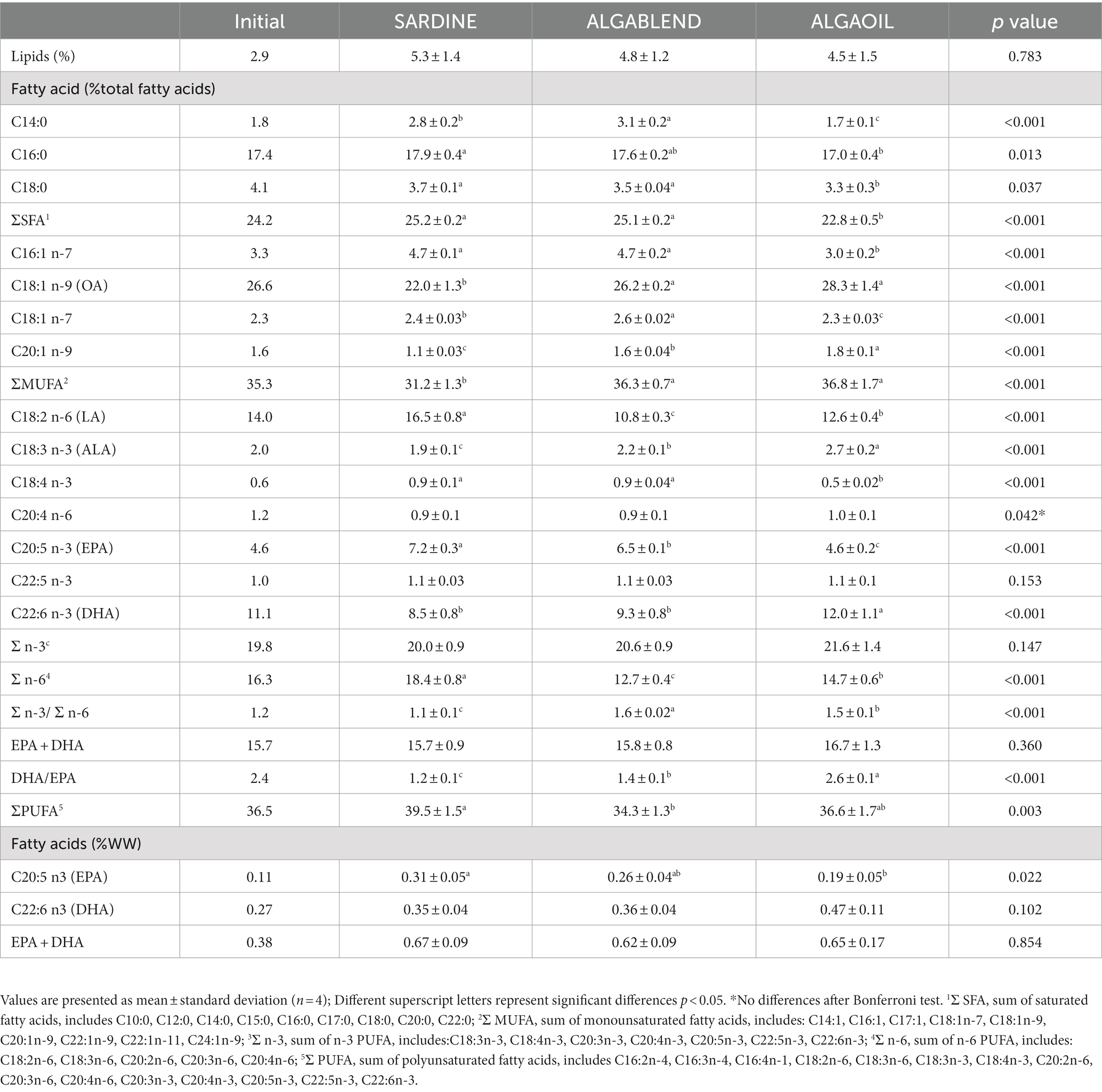
Table 5. Muscle total lipid content and fatty acid composition of the European sea bass fed experimental diets.
3.4. pH and water holding capacity
No statistical differences (p > 0.05) between dietary treatments were found for the pH and WHC parameters in European sea bass muscle (Figure 2). Likewise, no statistical differences were observed for the interaction between the dietary treatment and storage time. However, a gradual increase in pH was observed over time of storage in ice for all dietary treatments, with significant differences between storage days: pHd0 < pHd3 < pHd14. WHC decreased with storage time, with significant differences between days 3 and 14, which exhibit lower values.
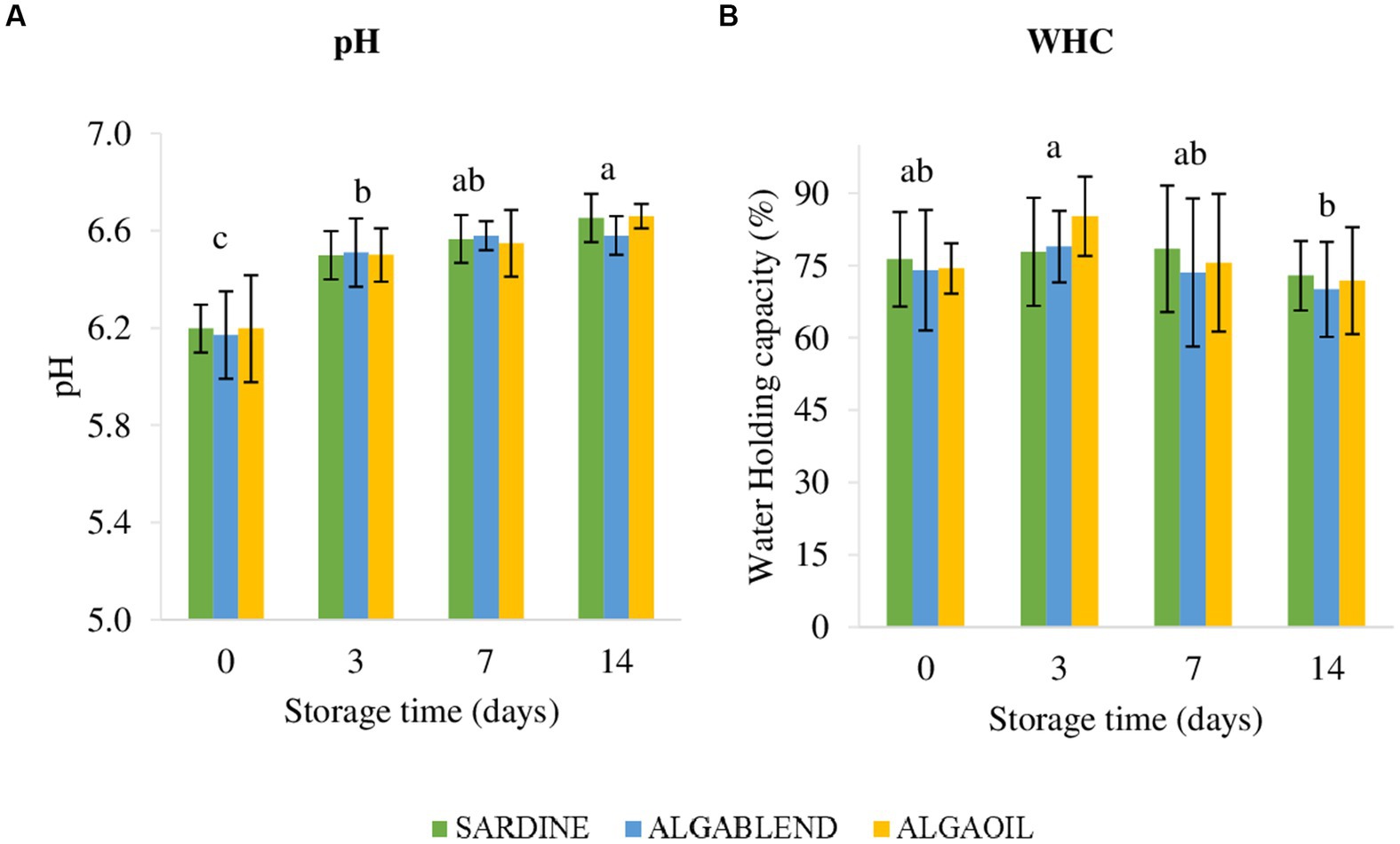
Figure 2. pH and Water Holding Capacity of fillets. pH and Water Holding Capacity (WHC%) in sea bass fillets submitted to different storage times in ice (0, 3, 7 and 14 days). Different lowercase letters indicate significant differences (p < 0.05) between days regardless of the dietary treatment. Values are presented as mean ± standard deviation (n = 16).
3.5. Antioxidant capacity and lipid peroxidation
Regardless of the dietary treatments, ABTS significantly decreased after day 0, presenting differences between day 0 and the remaining days: ABTSd0 > (ABTSd3 = ABTSd7 = ABTSd14) (Figure 3A). No statistical differences were found for ORAC assay in European sea bass muscle antioxidant capacity between diets or time of storage (Figure 3B). Muscle lipid peroxidation (LPO), varied with storage time: LPO values remained constant up to 3 days of storage in ice, but decreased significantly afterwards ([LPOd0 = LPOd3] > [LPOd7 = LPOd14]).
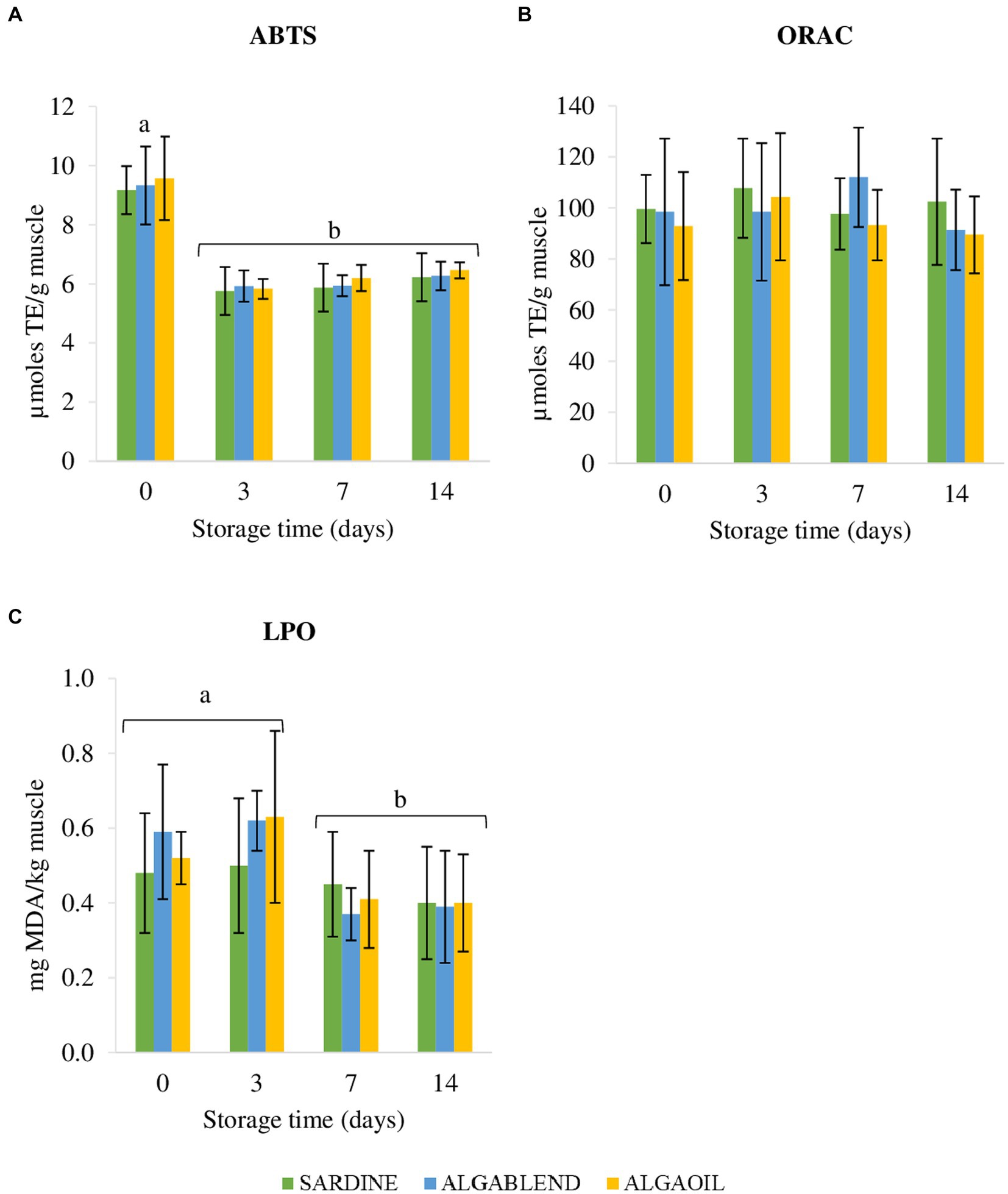
Figure 3. Diet Effects on antioxidant potential and lipid peroxidation of sea bass fillets. ABTS radical scavenging activity (A), ORAC - Oxygen Radical Absorbance Capacity (B) and LPO- lipid peroxidation (C) in sea bass fillets submitted to different storage times in ice (0, 3, 7 and 14 days). Different lowercase letters indicate significant differences (p < 0.05) between days regardless the dietary treatment. Muscle lipid peroxidation is in mg MDA/kg muscle, while ABTS/ORAC assays are in μmoles TE/g muscle. Values are presented as mean ± standard deviation (n = 16).
3.6. Muscle texture and color
No statistical differences were observed between dietary treatments for muscle textural parameters, with the exception of springiness that overall was highest in SARDINE fed fish and lowest for ALGABLEND fed fish. No significant interaction was observed between diet and storage time for any textural parameter (p > 0.05). But all textural parameters, with the exception of resilience and springiness, suffered significant alterations during the 14 days of storage in ice. Hardness slightly increased from slaughter (day 0) up to day 3, which exhibited the highest value, and decreased afterwards (Figure 4 and Supplementary Table S1). Adhesiveness increased from the lowest value on day 0, to the highest value on day 14. The muscle cohesiveness and chewiness decreased significantly and steadily over time.
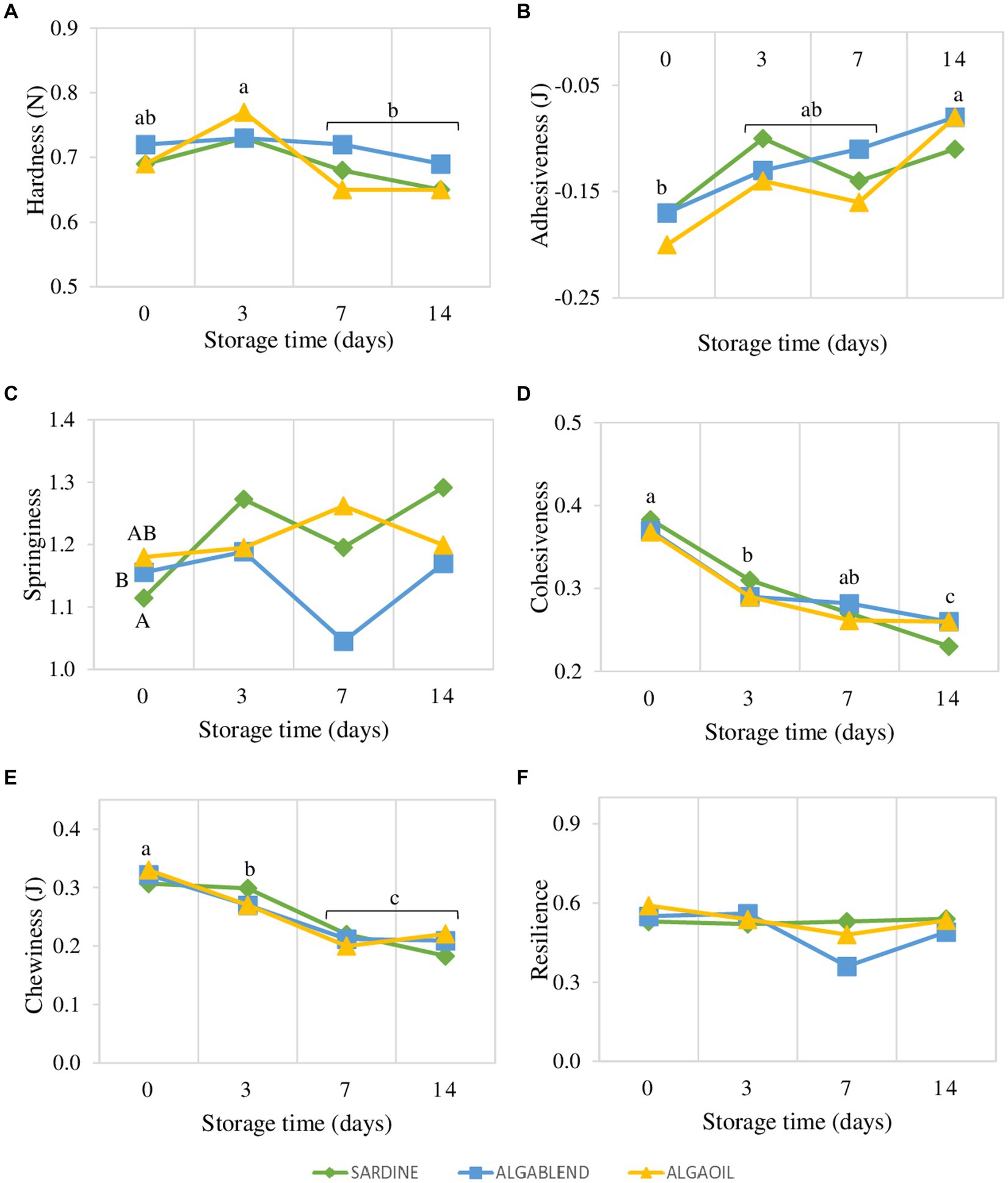
Figure 4. Diet effects on Muscle textural properties (TPA) throughout 14 days of storage in ice of the European sea bass. (A) Hardness (Newton); (B) Adhesiveness (Joule); (C) Springiness; (D) Cohesiveness; (E) Chewiness (Joule); (F) Resilience. Different lowercase letters indicate significant differences (p < 0.05) between days regardless the dietary treatments, while uppercase letters indicate significant differences between diets. No statistical differences were observed for the interaction between dietary treatments and storage time. Values are presented as mean ± standard deviation (n = 16).
No significant differences were observed in European sea bass skin and muscle color among dietary treatments (Table 6). Likewise, no statistical differences were observed for the interaction between the dietary treatment and storage time, but storage time impacted the muscle color significantly. Lightness (L*) increased in skin from day 0 to day 7, and in muscle from day 0 to day 14. In the skin, a* values (toward red color) decreased in European sea bass after 7 days, but significantly increased at day 14; in muscle, a* values significantly decreased throughout storage time. Yellowness (b*) was also significantly affected by storage time, decreasing throughout time in European seabass skin while it decreased in muscle up to 7 days, but significantly increased at day 14. The ΔE values exhibited significant differences between the different days, as indicated in Table 6. Both skin and muscle color demonstrated an increase from day 0 to day 14 (ΔEd0 < [ΔEd3 = ΔEd7] < ΔEd14]).
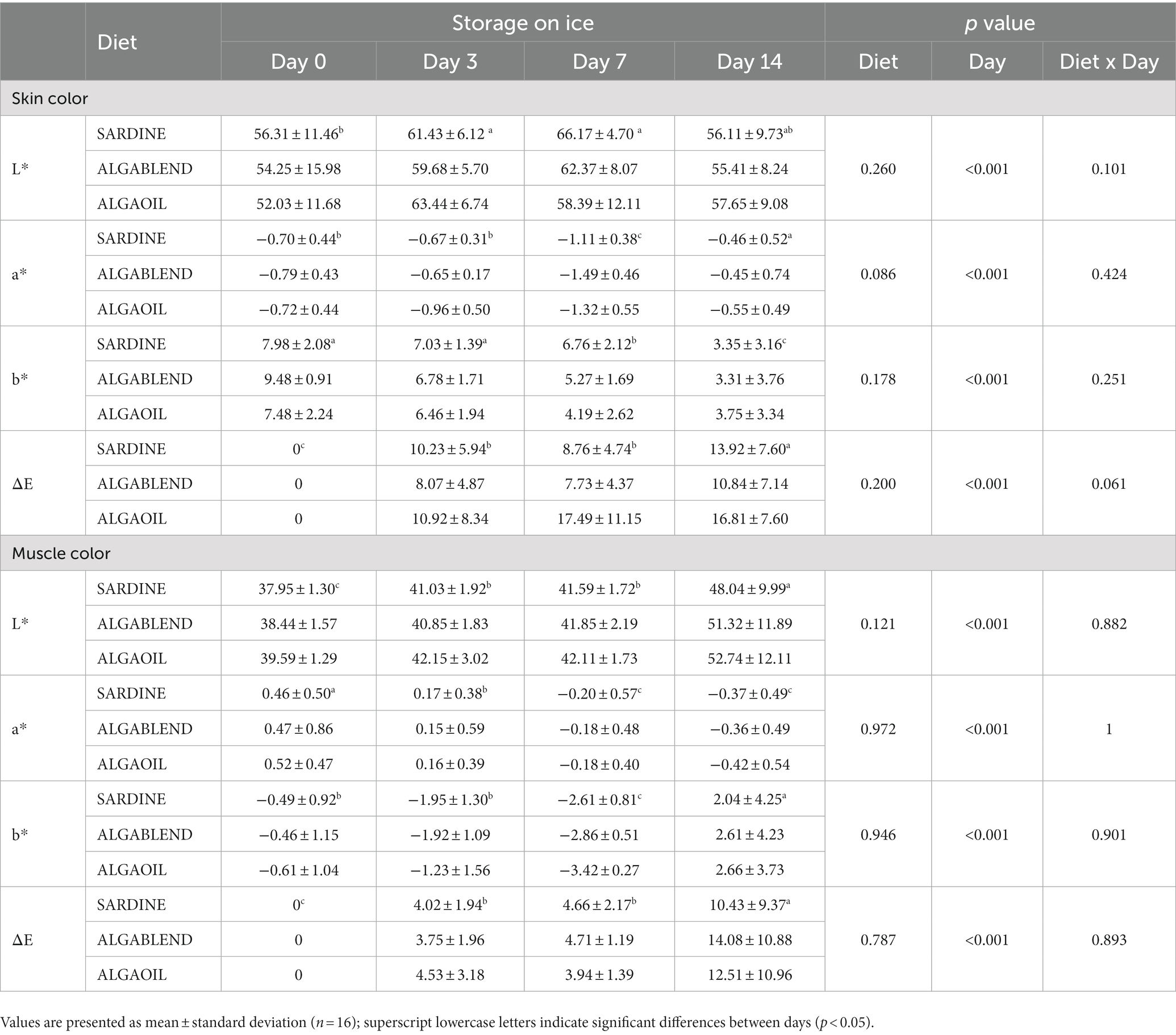
Table 6. Skin and muscle color throughout 14 days of storage in ice of the European sea bass fed experimental diets.
4. Discussion
The search for alternatives to FO that promote circular economy ensuring good fish growth and high nutritional and sensorial value has been the focus of much research. Particular attention has been centered on the importance of fish and fish products as sources of bioactive n-3 LC-PUFA in human nutrition (Santigosa et al., 2020, 2021). The perception that fish consumption and a healthy lifestyle are intrinsically related, especially in the prevention of heart disease and reduction of inflammatory disorders, has instigated public interest in the nutritional value of n-3 LC-PUFA, particularly in EPA and DHA (Mourente and Bell, 2006; Yagi et al., 2017). In the present study, the feasibility of replacing SdO by different combinations of alternative marine lipid ingredients as sources of EPA and DHA was demonstrated by the trials set for European sea bass.
At the end of the trial, all the experimental diets equally contributed to the growth performance and feed efficiency of European sea bass when compared to the control diet – SARDINE, which contained 9.0% of SdO and 4.4% SyO. Our data suggest that partial (ALGABLEND diet) or total replacement of SdO (ALGAOIL diet) with alternative marine ingredients rich in n-3 LC-PUFA has no adverse effects on growth parameters, as previously reported in other species (Betancor et al., 2016a,b; Santigosa et al., 2020). These results may be attributed to the high and comparable dietary content of EPA + DHA (2.4–2.5% DM) in all experimental diets. Marques et al. (2022) have also demonstrated promising results in terms of feed efficiency and growth performance of European sea bass fed diets containing only 5.5% of FO, but with an EPA + DHA dietary content ranging between 2.0–2.3% DM. Furthermore, in the present study, all experimental diets had a DHA/EPA ratio between 0.7 and 1.9, which falls within the recommended range of values (0.5–2.0) required for normal growth and development of marine fish species (NRC, 2011). These results may apparently be in contrast with some studies reporting fish growth impairment when VO was used instead of FO (Vilhelmsson et al., 2004; Benedito-Palos et al., 2007; Yilmaz et al., 2016), possibly due to the ability of AO and algae biomass to compensate dietary deficiencies, specifically the lack of EPA and DHA observed in VO (Santigosa et al., 2020, 2021).
The present results showed that the somatic indexes (HSI and VSI), whole body composition, or nutrient utilization of European sea bass remained unaffected by the dietary treatments. These findings demonstrate that it is possible to partially or totally replace SdO with alternative sources if n-3 LC-PUFA levels are provided in the diet. Similar results were observed in Atlantic salmon fed with algal oils. Wei et al. (2021) reported no significant impact on somatic indexes when using Schizochytrium oil, while Kousoulaki et al. (2022) showed comparable results when FO was replaced by AO.
The use of vegetable ingredients in aquafeeds is a subject of concern due to their impact on the muscle FA profile, according to numerous studies (Izquierdo et al., 2003, 2005; Bell et al., 2004). For instance, when alternative lipid sources without n-3 LC-PUFA were used in trout feeds, EPA and DHA levels in the fillets were found to decrease by approximately 50% (Santigosa et al., 2020). Montero et al. (2005) observed a reduction of up to 55% of EPA and DHA concentrations in European sea bass muscle when 60% of FO was replaced by VO poor in n-3 LC- PUFA such as EPA and DHA. This poses a major concern for consumers as the lower nutritional quality of the final product can impact the health benefits associated with seafood consumption (Santigosa et al., 2020). In this study, we demonstrated that the incorporation of microalgae biomass or AO is a successful strategy for mitigating the potential reduction of fillet nutritional value caused by decreasing FO in diets for European sea bass. Even when the diet was partially or entirely devoid of FO from forage fish (SdO), fish fed with the alternative diets still met the required EPA + DHA levels that are associated with health benefits. Most health organizations agree that 0.25 g per day of combined EPA and DHA (Intake Recommendations – IRs) is enough for adults to maintain their overall health (FAO, 2010; EFSA, 2012). However, a higher EPA and DHA intake has been recommended for pregnant and lactating women in order to improve brain development of the fetus and breastfed infants (0.2 g DHA plus the daily recommended 0.25 g per day of EPA + DHA) (EFSA, 2012). In our study, all tested diets, even the one totally lacking FO (ALGAOIL diet), were effective in providing fillets with EPA + DHA ranging between 0.62 and 0.65 g per 100 g of fresh weight. Additionally, the utilization of alternative sources such as microalgae can enhance the ratio of n-3 to n-6 PUFA as observed in this study (Σ n-3/ Σ n-6: SARDINE - 1.1; ALGABLEND - 1.6; ALGAOIL - 1.5). This, in turn, reduces inflammation since n-6 PUFA serves as a precursor to pro-inflammatory molecules, whereas eicosanoids derived from n-3 PUFA have opposing effects. These findings underscore the significance of maintaining a balanced n-3/n-6 ratio for disease prevention, including cardiovascular diseases and cancer (Bagga et al., 1997; Remize et al., 2021; Carr et al., 2023). These results also point out the possibility of formulating aquafeeds with alternative sources, greatly reducing the use of FO from forage fish, and without having to rely on VO which directly compete with its utilization for farmed animals and human consumption (from industrially farmed crops) (Sarker et al., 2016).
Supplementation of aquafeeds with micro and macro algae has shown health associated benefits, acting as antioxidants and immunostimulants, improving fish resistance to diseases. Additionally, algal compounds are able to counteract the oxidative damage by free radicals and serve as potential additives for preventing quality deterioration or retaining the quality of fish and fish products during the shelf life period (Ferreira et al., 2021). Although in the present study algae inclusion in diets did not reveal any antioxidant benefits in European sea bass muscle, significant differences were identified during storage time. Namely, the antioxidant potential measured through the scavenging potential of ABTS was higher in fish at day 0 irrespective of the dietary treatments. Likewise, LPO presented a significant decrease between the first three days and the remaining days of storage. Fish products rich in n-3 LC-PUFA may have health-promoting effects for the consumer, but they are prone to decompose during storage time, leading to seafood rancidity and unwanted odors (Jo et al., 2022). Microalgae incorporation in aquafeeds has shown to prevent LPO in seafood (Takyar et al., 2019; Tavakoli et al., 2022), but in the present study, all experimental diets equally contributed to LPO evolution throughout the 14 days of storage in ice. However, oxidative rancidity, generally associated with MDA levels, was inferior to 0.7 mg of MDA/kg in all European sea bass fillets. According to Taşkaya and Yaşar (2018) fish is considered in good condition when TBAR concentration is between 3 and 5 mg of MDA/kg of fillet. This indicates that in our study, European sea bass was in excellent condition for human consumption throughout the 14 days of storage time.
Overall, the present study demonstrated that, FO replacement by marine n-3 LC-PUFA rich sources (SmO, AO and a blend of micro and macroalgae) did not significantly alter the quality traits of European sea bass during storage time. For all experimental diets, pH, one of the important parameters to judge the quality of aquatic products, showed a very high post-mortem value (>6.0) and an upward trend during storage in ice, which is consistent with previous reports (Li et al., 2021). Such increased fillet pH may be due to the accumulation of volatile bases such as ammonia and trimethylamine from the action of microorganisms and endogenous enzymes. On the other hand, WHC showed a decreasing trend with increased time of storage, irrespective of the diets. Örnek et al. (2021) also observed that FO replacement by VO (0, 50 and 100%) in rainbow trout lead to a decrease in WHC over storage time in all experimental diets, indicating muscle protein denaturation (Chan et al., 2022). Besides chemical characteristics, sensory properties such as color and texture are important factors that influence consumer choices. During storage time, skin and muscle color were not impacted by the experimental diets tested in this study. In addition to color, the texture is one of the most important quality attributes, which is dependent on several parameters such as hardness, adhesiveness, cohesiveness, springiness, chewiness and resilience (Hyldig and Nielsen, 2001). In the present study, we observed that FO could be replaced by different combinations of lipid sources without major differences in textural parameters throughout the 14 days of storage in ice, except a significant increase in springiness on fish fed the SARDINE diet. These results on the effect of diets on textural parameters may be expected since no changes on growth or total lipid content in muscles were found, which are known to affect texture properties (Olafsdottir et al., 2004; Liang et al., 2017). However, further studies are recommended, such as sensory analysis of cooked European sea bass fillets, to better understand the true impact of alternative marine lipid sources on sensory properties and consumer preferences.
5. Conclusion
This study demonstrated that European sea bass fed with reduced or no forage fishery resources-derived FO – specifically SdO - and supplemented with alternative marine n-3 LC-PUFA rich sources such as SmO, AO and a blend of micro- and macroalgae attained similar growth performance, somatic indexes and whole body composition as fish fed with a practical diet (SARDINE). After 116 days of feeding with the different experimental diets, no significant differences were observed in EPA + DHA levels in fish muscle between dietary treatments, resulting in levels (> 0.62 g 100 g−1) that are above those recommended by the European Food Safety Authority to prevent the risk of cardiovascular accident in European adults. Moreover, the dietary treatments did not affect the organoleptic and freshness properties of the fish muscle during the 14 days of cold storage in ice, indicating successful FO replacement. Overall, the present study showed that combining high-priced sources of n-3 LC-PUFA (Veramaris® and Algaessence Feed™) with a more affordable oil (SmO) allowed the fortification of European sea bass muscle in EPA and DHA using alternative sources to the traditional FO in aquafeeds while ensuring fish freshness, nutritional quality, and consumers’ health.
Data availability statement
The raw data supporting the conclusions of this article will be made available by the authors, without undue reservation.
Ethics statement
The animal studies were approved by Riasearch Lda. Ethical Committee. The studies were conducted in accordance with the local legislation and institutional requirements. Written informed consent was not obtained from the owners for the participation of their animals in this study because Riasearch owned the fish used in the experiment, so we consider that this consent does not apply.
Author contributions
AF-S and AM: methodology, validation, formal analysis, investigation, validation, and writing – review and editing. MS: formal analysis, funding acquisition, and writing – review. HA and JD: conceptualization and funding acquisition. LV: conceptualization, methodology, resources, writing – review and editing, supervision, project administration, and funding acquisition. All authors contributed to the article and approved the submitted version.
Funding
This work is a result of the project OmegaPeixe (POCI-01-0247-FEDER-069748), supported by Operational Program for Competitiveness and Internationalization (COMPETE 2020), under the PORTUGAL 2020 Partnership Agreement, through the European Regional Development Fund (ERDF) and by FCT – Foundation for Science and Technology to CIIMAR (UIDB/04423/2020 and UIDP/04423/2020).
Conflict of interest
The authors declare that the research was conducted in the absence of any commercial or financial relationships that could be construed as a potential conflict of interest.
Publisher’s note
All claims expressed in this article are solely those of the authors and do not necessarily represent those of their affiliated organizations, or those of the publisher, the editors and the reviewers. Any product that may be evaluated in this article, or claim that may be made by its manufacturer, is not guaranteed or endorsed by the publisher.
Supplementary material
The Supplementary material for this article can be found online at: https://www.frontiersin.org/articles/10.3389/fsufs.2023.1224370/full#supplementary-material
References
Alexi, N., Fountoulaki, E., and Grigorakis, K. (2017). Quality of reared gilthead sea bream (Sparus aurata) during ice storage, as affected by dietary fish oil substitution; an instrumental and sensory designation approach. Aquac. Res., 48, 3817–3828. doi: 10.1111/are.13208
Annamalai, S. N., Das, P., Thaher, M. I. A., Abdul Quadir, M., Khan, S., Mahata, C., et al. (2021). Nutrients and energy digestibility of microalgal biomass for fish feed applications. Sustainability 13:13211. doi: 10.3390/su132313211
Bagga, D., Capone, S., Wang, H. J., Heber, D., Lill, M., Chap, L., et al. (1997). Dietary modulation of omega-3/omega-6 polyunsaturated fatty acid ratios in patients with breast cancer. J. Natl. Cancer Inst. 89, 1123–1131. doi: 10.1093/jnci/89.15.1123
Batista, S., Pereira, R., Oliveira, B., Baião, L. F., Jessen, F., Tulli, F., et al. (2020). Exploring the potential of seaweed Gracilaria gracilis and microalga Nannochloropsis oceanica, single or blended, as natural dietary ingredients for European seabass Dicentrarchus labrax. J. Appl. Phycol. 32, 2041–2059. doi: 10.1007/s10811-020-02118-z
Bell, J. G., Henderson, R. J., Tocher, D. R., and Sargent, J. R. (2004). Replacement of dietary fish oil with increasing levels of linseed oil: modification of flesh fatty acid compositions in Atlantic salmon (Salmo salar) using a fish oil finishing diet. Lipids 39, 223–232. doi: 10.1007/s11745-004-1223-5
Bell, J. G., McEvoy, J., Tocher, D. R., McGhee, F., Campbell, P. J., and Sargent, J. R. (2001). Replacement of fish oil with rapeseed oil in diets of Atlantic salmon (Salmo salar) affects tissue lipid compositions and hepatocyte fatty acid metabolism. J. Nutr. 131, 1535–1543. doi: 10.1093/jn/131.5.1535
Ben Atitallah, A., Hentati, F., Dammak, M., Hadrich, B., Fendri, I., Ayadi, M. A., et al. (2019). Effect of microalgae incorporation on quality characteristics and functional and antioxidant capacities of ready-to-eat fish burgers made from common carp (Cyprinus carpio). Appl. Sci. 9:1830. doi: 10.3390/app9091830
Benedito-Palos, L., Saera-Vila, A., Calduch-Giner, J. A., Kaushik, S., and Pérez-Sánchez, J. (2007). Combined replacement of fish meal and oil in practical diets for fast growing juveniles of gilthead sea bream (Sparus aurata L.): networking of systemic and local components of GH/IGF axis. Aquaculture 267, 199–212. doi: 10.1016/j.aquaculture.2007.01.011
Betancor, M. B., Sprague, M., Montero, D., Usher, S., Sayanova, O., Campbell, P. J., et al. (2016a). Replacement of marine fish oil with de novo omega-3 oils from transgenic Camelina sativa in feeds for gilthead sea bream (Sparus aurata L.). Lipids 51, 1171–1191. doi: 10.1007/s11745-016-4191-4
Betancor, M. B., Sprague, M., Sayanova, O., Usher, S., Metochis, C., Campbell, P. J., et al. (2016b). Nutritional evaluation of an epa-dha oil from transgenic Camelina sativa in feeds for post-smolt Atlantic salmon (Salmo salar L.). PLoS One 11:e0159934. doi: 10.1371/journal.pone.0159934
Carr, I., Glencross, B., and Santigosa, E. (2023). The importance of essential fatty acids and their ratios in aquafeeds to enhance salmonid production, welfare, and human health. Frontiers in Animal Science 4:1147081. doi: 10.3389/fanim.2023.1147081
Castro, P. L., Torrecillas, S., Montero, D., Izquierdo, M. S., and Ginés, R. (2022). Effect of combined fishmeal and fish oil replacement on growth performance, flesh quality and shelf life of European sea bass (Dicentrarchus labrax). Aquaculture 560:738452. doi: 10.1016/j.aquaculture.2022.738452
Chan, S. S., Roth, B., Jessen, F., Jakobsen, A. N., and Lerfall, J. (2022). Water holding properties of Atlantic salmon. Compr. Rev. Food Sci. Food Saf. 21, 477–498. doi: 10.1111/1541-4337.12871
Charles, S. B., Hardy, R. W., and Hamidoghli, A. (2022). “Diet analysis and evaluation” in Fish nutrition. eds. R. Hardy and K. Sadasivam. Fourth ed (United States: Academic Press), 709–743.
Chen, W., Luo, L., Han, D., Long, F., Chi, Q., and Hu, Q. (2021). Effect of dietary supplementation with filamentous microalga Tribonema ultriculosum on growth performance, fillet quality and immunity of rainbow trout Oncorhynchus mykiss. Aquac. Nutr. 27, 1232–1243. doi: 10.1111/anu.13264
Dávalos, A., Gómez-Cordovés, C., and Bartolomé, B. (2004). Extending applicability of the oxygen radical absorbance capacity (ORAC-fluorescein) assay. J. Agric. Food Chem. 52, 48–54. doi: 10.1021/jf0305231
Dikel, S., Engin, K., and Arslan, M. (2013). Partial replacement of fish oil with vegetable oils in diets for European seabass (Dicentrarchus labrax): effects on growth performance and fatty acids profile. Turk. J. Fish. Aquat. Sci. 13, 819–825. doi: 10.4194/1303-2712-v13_3_05
EFSA (2012). Scientific opinion on the tolerable upper intake level of eicosapentaenoic acid (EPA), docosahexaenoic acid (DHA) and docosapentaenoic acid (DPA). EFSA J. 10:28158. doi: 10.2903/j.efsa.2012.2815
FAO (2020). The state of world fisheries and aquaculture 2020. Sustainability in action. Rome. FAO. 63, 17–18. doi: 10.4060/ca9229en
Ferreira, M., Ribeiro, P. C., Ribeiro, L., Barata, M., Domingues, V. F., Sousa, S., et al. (2022). Biofortified diets containing algae and selenised yeast: effects on growth performance, nutrient utilization, and tissue composition of gilthead seabream (Sparus aurata). Front. Physiol. 12:2475. doi: 10.3389/fphys.2021.812884
Ferreira, M., Teixeira, C., Abreu, H., Silva, J., Costas, B., Kiron, V., et al. (2021). Nutritional value, antimicrobial and antioxidant activities of micro- and macroalgae, single or blended, unravel their potential use for aquafeeds. J. Appl. Phycol. 33, 3507–3518. doi: 10.1007/S10811-021-02549-2/FIGURES/2
Glencross, B. D. (2009). Exploring the nutritional demand for essential fatty acids by aquaculture species. Rev. Aquac. 1, 71–124. doi: 10.1111/j.1753-5131.2009.01006.x
Glencross, B., Fracalossi, D. M., Hua, K., Izquierdo, M., Mai, K., Øverland, M., et al. (2023). Harvesting the benefits of nutritional research to address global challenges in the 21st century. J. World Aquacult. Soc. 54, 343–363. doi: 10.1111/jwas.12948
Goes, E. S. R., Lara, J. A. F., Gasparino, E., del Vesco, A. P., Goes, M. D., Alexandre Filho, L., et al. (2015). Pre-slaughter stress affects ryanodine receptor protein gene expression and the water-holding capacity in fillets of the Nile tilapia. PLoS One 10:e0129145. doi: 10.1371/journal.pone.0129145
Gonçalves, B., Falco, V., Moutinho-Pereira, J., Bacelar, E., Peixoto, F., and Correia, C. (2009). Effects of elevated CO2 on grapevine (Vitis vinifera L.): volatile composition, phenolic content, and in vitro antioxidant activity of red wine. J. Agric. Food Chem. 57, 265–273. doi: 10.1021/jf8020199
Grigorakis, K., Fountoulaki, E., Giogios, I., and Alexis, M. N. (2009). Volatile compounds and organoleptic qualities of gilthead sea bream (Sparus aurata) fed commercial diets containing different lipid sources. Aquaculture 290, 116–121. doi: 10.1016/j.aquaculture.2009.02.006
Hyldig, G., and Nielsen, D. (2001). A review of sensory and instrumental methods used to evaluate the texture of fish muscle. J. Texture Stud. 32, 219–242. doi: 10.1111/j.1745-4603.2001.tb01045.x
Izquierdo, M. S., Montero, D., Robaina, L., Caballero, M. J., Rosenlund, G., and Ginés, R. (2005). Alterations in fillet fatty acid profile and flesh quality in gilthead seabream (Sparus aurata) fed vegetable oils for a long term period. Recovery of fatty acid profiles by fish oil feeding. Aquaculture 250, 431–444. doi: 10.1016/J.AQUACULTURE.2004.12.001
Izquierdo, M. S., Obach, A., Arantzamendi, L., Montero, D., Robaina, L., and Rosenlund, G. (2003). Dietary lipid sources for seabream and seabass: growth performance, tissue composition and flesh quality. Aquac. Nutr. 9, 397–407. doi: 10.1046/j.1365-2095.2003.00270.x
Jo, H.-G., Chilakala, R., Kim, M.-J., Sin, Y.-S., Lee, K.-S., and Cheong, S.-H. (2022). Assessment of the effects of salt and Salicornia herbacea L. on physiochemical, nutritional, and quality parameters for extending the shelf-life of semi-dried mullets (Chelon haematocheilus). Foods 11:597. doi: 10.3390/foods11040597
Joseph, J. D., and Ackman, R. G. (1992). Capillary column gas chromatographic method for analysis of encapsulated fish oils and fish oil ethyl esters: collaborative study. J. AOAC Int. 75, 488–506. doi: 10.1093/jaoac/75.3.488
Katerina, K., Berge, G. M., Turid, M., Aleksei, K., Grete, B., Trine, Y., et al. (2020). Microalgal Schizochytrium limacinum biomass improves growth and filet quality when used long-term as a replacement for fish oil, in modern salmon diets. Front. Mar. Sci. 7:57. doi: 10.3389/fmars.2020.00057
Kokubun, K., Nemoto, K., and Yamakawa, Y. (2020). Fish intake may affect brain structure and improve cognitive ability in healthy people. Front. Aging Neurosci. 12:76. doi: 10.3389/fnagi.2020.00076
Kousoulaki, K., Mørkøre, T., Nengas, I., Berge, R. K., and Sweetman, J. (2016). Microalgae and organic minerals enhance lipid retention efficiency and fillet quality in Atlantic salmon (Salmo salar L.). Aquaculture 451, 47–57. doi: 10.1016/j.aquaculture.2015.08.027
Kousoulaki, K., Sveen, L., Norén, F., and Espmark, A. (2022). Atlantic salmon (Salmo salar) performance fed low trophic ingredients in a fish meal and fish oil free diet. Front. Physiol. 13:1054. doi: 10.3389/fphys.2022.884740
Larsen, R., Eilertsen, K. E., and Elvevoll, E. O. (2011). Health benefits of marine foods and ingredients. Biotechnol. Adv. 29, 508–518. doi: 10.1016/J.BIOTECHADV.2011.05.017
Li, N., Wu, X., Zhuang, W., Xia, L., Chen, Y., Wu, C., et al. (2020). Fish consumption and multiple health outcomes: umbrella review. Trends Food Sci. Technol. 99, 273–283. doi: 10.1016/j.tifs.2020.02.033
Li, Q., Zhang, J., Zhu, J., Lin, H., Sun, T., and Cheng, L. (2021). Effects of gallic acid combined with epsilon-polylysine hydrochloride incorporated in a pullulan-CMC edible coating on the storage quality of sea bass. RSC Adv. 11, 29675–29683. doi: 10.1039/d1ra02320a
Liang, X. F., Hu, L., Dong, Y. C., Wu, X. F., Qin, Y. C., Zheng, Y. H., et al. (2017). Substitution of fish meal by fermented soybean meal affects the growth performance and flesh quality of Japanese seabass (Lateolabrax japonicus). Anim. Feed Sci. Technol. 229, 1–12. doi: 10.1016/j.anifeedsci.2017.03.006
Liu, Y., Ren, X., Fan, C., Wu, W., Zhang, W., and Wang, Y. (2022). Health benefits, food applications, and sustainability of mi-croalgae-derived n-3 Pufa. Foods 11:1883. doi: 10.3390/foods11131883
Malcorps, W., Newton, R. W., Sprague, M., Glencross, B. D., and Little, D. C. (2021). Nutritional characterisation of European aquaculture processing by-products to facilitate strategic utilisation. Front. Sustainable Food Sys. 5:720595. doi: 10.3389/fsufs.2021.720595
Marques, A., Matos, E., Aires, T., Melo, D., Oliveira, M. B. P. P., and Valente, L. M. P. (2022). Understanding the interaction between terrestrial animal fat sources and dietary emulsifier supplementation on muscle fatty acid profile and textural properties of European sea bass. Aquaculture 560:738547. doi: 10.1016/j.aquaculture.2022.738547
Maulu, S., Nawanzi, K., Abdel-Tawwab, M., and Khalil, H. S. (2021). Fish nutritional value as an approach to children’s nutrition In frontiers in nutrition. Frontiers Media. 8:780844. doi: 10.3389/fnut.2021.780844
Meigs, H., Barrows, F., Sims, A., and Alfrey, K. (2020). Testing diets without fishmeal and fish oil for kampachi - responsible seafood advocate. Global Aquaculture Advocate. Available at: https://www.globalseafood.org/advocate/testing-diets-without-fishmeal-and-fish-oil-for-kampachi/
Montero, D., and Izquierdo, M. S. (2010). “Welfare and health of fish fed vegetable oils as alternative lipid sources to fish oil” in Fish oil replacement and alternative lipid sources in aquaculture feeds (Florida: CRC Press), 439–485.
Montero, D., Robaina, L., Caballero, M. J., Ginés, R., and Izquierdo, M. S. (2005). Growth, feed utilization and flesh quality of European sea bass (Dicentrarchus labrax) fed diets containing vegetable oils: a time-course study on the effect of a re-feeding period with a 100% fish oil diet. Aquaculture 248, 121–134. doi: 10.1016/j.aquaculture.2005.03.003
Mourente, G., and Bell, J. G. (2006). Partial replacement of dietary fish oil with blends of vegetable oils (rapeseed, linseed and palm oils) in diets for European sea bass (Dicentrarchus labrax L.) over a long term growth study: effects on muscle and liver fatty acid composition and effectiveness of a fish oil finishing diet. Comp Biochem Physiol B Biochem Mol Biol 145, 389–399. doi: 10.1016/j.cbpb.2006.08.012
Nagappan, S., Das, P., AbdulQuadir, M., Thaher, M., Khan, S., Mahata, C., et al. (2021). Potential of microalgae as a sustainable feed ingredient for aquaculture. J. Biotechnol. 341, 1–20. doi: 10.1016/j.jbiotec.2021.09.003
Nasopoulou, C., and Zabetakis, I. (2012). Benefits of fish oil replacement by plant originated oils in compounded fish feeds. A review. In LWT - Food Sci. Technol. 47, 217–224. doi: 10.1016/j.lwt.2012.01.018
Newton, R. W., Maiolo, S., Malcorps, W., and Little, D. C. (2023). Life cycle inventories of marine ingredients. Aquaculture 565:739096. doi: 10.1016/j.aquaculture.2022.739096
NRC (2011). “Nutrient requirements of fish and shrimp. The National Academy” in Nutrient requirements of fish and shrimp. 1sd ed (Washinton, DC, USA: National Academy Press) doi: 10.17226/13039
Olafsdottir, G., Nesvadba, P., di Natale, C., Careche, M., Oehlenschläger, J., Tryggvadóttir, S. V., et al. (2004). Multisensor for fish quality determination. Trends Food Sci. Technol. 15, 86–93. doi: 10.1016/j.tifs.2003.08.006
Oliveira, A. C. M., and Balaban, M. O. (2006). Comparison of a colorimeter with a machine vision system in measuring color of gulf of mexico sturgeon fillets. Appl. Eng. Agric. 22, 583–587. doi: 10.13031/2013.21211
Örnek, E., Acar, Ü., and Öğütcü, M. (2021). Effects of dietary fish oil replacement by poppy seed oil on growth performance and fillet quality of rainbow trout (Oncorhynchus mykiss). Aquac. Res. 52, 3026–3037. doi: 10.1111/are.15147
Parrish, C. C., Nichols, P. D., Pethybridge, H., and Young, J. W. (2015). Direct determination of fatty acids in fish tissues: quantifying top predator trophic connections. Oecologia 177, 85–95. doi: 10.1007/s00442-014-3131-3
Pereira, R., Basto, A., Conde-Sieira, M., Linares, F., Rodríguez Villanueva, J. L., Sieira, G. P., et al. (2019). Growth performance and nutrient utilisation of Senegalese sole fed vegetable oils in plant protein-rich diets from juvenile to market size. Aquaculture 511:734229. doi: 10.1016/j.aquaculture.2019.734229
Raatz, S. K., Silverstein, J. T., Jahns, L., and Picklo, M. J. (2013). Issues of fish consumption for cardiovascular disease risk reduction. Nutrients 5, 1081–1097. doi: 10.3390/nu5041081
Remize, M., Brunel, Y., Silva, J. L., Berthon, J. Y., and Filaire, E. (2021). Microalgae n-3 PUFAs production and use in food and feed industries. Mar. Drugs 19:113. doi: 10.3390/md19020113
Rosenlund, G., Obach, A., Sandberg, M. G., Standal, H., and Tveit, K. (2001). Effect of alternative lipid sources on long-term growth performance and quality of Atlantic salmon (Salmo salar L.). Aquac. Res. 32, 323–328. doi: 10.1046/j.1355-557x.2001.00025.x
Ruxton, C. H. S. (2011). The benefits of fish consumption. Nutr. Bull. 36, 6–19. doi: 10.1111/J.1467-3010.2010.01869.X
Santigosa, E., Brambilla, F., and Milanese, L. (2021). Microalgae oil as an effective alternative source of EPA and DHA for gilthead seabream (Sparus aurata) aquaculture. Animals 11:971. doi: 10.3390/ani11040971
Santigosa, E., Constant, D., Prudence, D., Wahli, T., and Verlhac-Trichet, V. (2020). A novel marine algal oil containing both EPA and DHA is an effective source of omega-3 fatty acids for rainbow trout (Oncorhynchus mykiss). J. World Aquacult. Soc. 51, 649–665. doi: 10.1111/jwas.12699
Sarker, P. K., Kapuscinski, A. R., Lanois, A. J., Livesey, E. D., Bernhard, K. P., and Coley, M. L. (2016). Towards sustainable aquafeeds: complete substitution of fish oil with marine microalga Schizochytrium sp. improves growth and fatty acid deposition in juvenile Nile tilapia (Oreochromis niloticus). PLoS One 11:e0156684. doi: 10.1371/journal.pone.0156684
Shah, M. R., Lutzu, G. A., Alam, A., Sarker, P., Kabir Chowdhury, M. A., Parsaeimehr, A., et al. (2018). Microalgae in aquafeeds for a sustainable aquaculture industry. J. Appl. Phycol. 30, 197–213. doi: 10.1007/s10811-017-1234-z
Stevens, J. R., Newton, R. W., Tlusty, M., and Little, D. C. (2018). The rise of aquaculture by-products: increasing food production, value, and sustainability through strategic utilisation. Mar. Policy 90, 115–124. doi: 10.1016/j.marpol.2017.12.027
Takyar, M. B. T., Haghighat Khajavi, S., and Safari, R. (2019). Evaluation of antioxidant properties of Chlorella vulgaris and Spirulina platensis and their application in order to extend the shelf life of rainbow trout (Oncorhynchus mykiss) fillets during refrigerated storage. LWT 100, 244–249. doi: 10.1016/j.lwt.2018.10.079
Taşkaya, L., and Yaşar, E. (2018). Determination of some quality properties of “hamsi kaygana” prepared with different additives. Food Sci. Nut. 6, 483–491. doi: 10.1002/fsn3.578
Tavakoli, S., Regenstein, J. M., Daneshvar, E., Bhatnagar, A., Luo, Y., and Hong, H. (2022). Recent advances in the application of microalgae and its derivatives for preservation, quality improvement, and shelf-life extension of seafood. Crit Rev Food Sci Nutr 62, 6055–6068. doi: 10.1080/10408398.2021.1895065
Turchini, G. M., Mentasti, T., Caprino, F., Panseri, S., Moretti, V. M., and Valfre, F. (2004). Effects of dietary lipid sources on flavour volatile compounds of brown trout (Salmo trutta L.) fillet. J. Appl. Ichthyol. 20, 71–75. doi: 10.1046/j.0175-8659.2003.00522.x
Turchini, G. M., Moretti, V. M., Mentasti, T., Orban, E., and Valfrè, F. (2007). Effects of dietary lipid source on fillet chemical composition, flavour volatile compounds and sensory characteristics in the freshwater fish tench (Tinca tinca L.). Food Chem. 102, 1144–1155. doi: 10.1016/j.foodchem.2006.07.003
Turchini, G. M., Torstensen, B. E., and Ng, W.-K. (2009). Fish oil replacement in finfish nutrition. Rev. Aquac. 1, 10–57. doi: 10.1111/j.1753-5131.2008.01001.x
Valente, L. M. P., Rema, P., Ferraro, V., Pintado, M., Sousa-Pinto, I., Cunha, L. M., et al. (2015). Iodine enrichment of rainbow trout flesh by dietary supplementation with the red seaweed Gracilaria vermiculophylla. Aquaculture 446, 132–139. doi: 10.1016/J.AQUACULTURE.2015.05.004
Vilhelmsson, O. T., Martin, S. A. M., Médale, F., Kaushik, S. J., and Houlihan, D. F. (2004). Dietary plant-protein substitution affects hepatic metabolism in rainbow trout (Oncorhynchus mykiss). Br. J. Nutr. 92, 71–80. doi: 10.1079/BJN20041176
Wei, M., Parrish, C. C., Guerra, N. I., Armenta, R. E., and Colombo, S. M. (2021). Extracted microbial oil from a novel Schizochytrium sp. (T18) as a sustainable high DHA source for Atlantic salmon feed: impacts on growth and tissue lipids. Aquaculture 534:736249. doi: 10.1016/j.aquaculture.2020.736249
Wells, M. L., Potin, P., Craigie, J. S., Raven, J. A., Merchant, S. S., Helliwell, K. E., et al. (2017). Algae as nutritional and functional food sources: revisiting our understanding. J. Appl. Phycol. 29, 949–982. doi: 10.1007/s10811-016-0974-5
Yagi, S., Fukuda, D., Aihara, K., Akaike, M., Shimabukuro, M., and Sata, M. (2017). N-3 polyunsaturated fatty acids: promising nutrients for preventing cardiovascular disease. J. Atheroscler. Thromb. 24, 999–1010. doi: 10.5551/jat.RV17013
Keywords: European sea bass, EPA and DHA, fish oil, muscle quality, omega-3 fatty acids, sustainable aquaculture, fish nutrition
Citation: Filipa-Silva A, Marques A, Salgado MA, Abreu H, Dias J and Valente LMP (2023) Exploring alternative marine lipid sources as substitutes for fish oil in Farmed Sea bass (Dicentrarchus labrax) and their influence on organoleptic, chemical, and nutritional properties during cold storage. Front. Sustain. Food Syst. 7:1224370. doi: 10.3389/fsufs.2023.1224370
Edited by:
Ana Sanches Silva, Instituto Nacional Investigaciao Agraria e Veterinaria (INIAV), PortugalReviewed by:
Richard Newton, University of Stirling, United KingdomJayashree Arcot, University of New South Wales, Australia
Fernanda Vilarinho, Instituto Nacional de Saúde Doutor Ricardo Jorge (INSA), Portugal
Copyright © 2023 Filipa-Silva, Marques, Salgado, Abreu, Dias and Valente. This is an open-access article distributed under the terms of the Creative Commons Attribution License (CC BY). The use, distribution or reproduction in other forums is permitted, provided the original author(s) and the copyright owner(s) are credited and that the original publication in this journal is cited, in accordance with accepted academic practice. No use, distribution or reproduction is permitted which does not comply with these terms.
*Correspondence: Luisa M. P. Valente, lvalente@icbas.up.pt