- 1Department of Pharmaceutical Chemistry, College of Pharmacy, King Khalid University, Abha, Asir, Saudi Arabia
- 2School of Materials Science and Engineering, Dongguan University of Technology, Dongguan, China
- 3Faculty of Energy and Environmental Engineering, Environmental Biotechnology Department, ul. Akademicka 2, Silesian University of Technology, Gliwice, Poland
- 4College of Mechatronics and Control Engineering, Shenzhen University, Shenzhen, China
- 5College of Physics and Optoelectronic Engineering, Shenzhen University, Shenzhen, China
- 6Department of Chemistry, Kohat University of Science and Technology, Kohat, Khyber Pakhtunkhwa, Pakistan
- 7Department of Chemistry, Faculty of Science, University of Karachi, Karachi, Pakistan
- 8Department of Pharmacognosy, College of Pharmacy, King Khalid University, Abha, Asir, Saudi Arabia
- 9Institute of Chemical Sciences, Gomal University, Dera Ismail Khan, Pakistan
- 10H.E.J. Research Institute of Chemistry, International Center for Chemical and Biological Sciences, University of Karachi, Karachi, Pakistan
In this study, a copper-containing polyphenol oxidase (PPO) enzyme was extracted from the selected agricultural food products, i.e., Mangifera indica (L.), Solanum tuberosum (L.), and Luffa cylindrica (L.), which has efficiently degraded the azo dyes (Methylene blue, Reactive Black-5, and Toluidine Blue-O) into less toxic compounds. After preparing the dye solution at various concentrations, the PPO enzymes extracted with phosphate buffer were incubated with it for 2 hours. The percentage of decolorization was estimated by using the unprocessed dye solution. The degraded products were characterized by UV–vis and FT-IR. All sets of experiments followed pseudo-first-order kinetics. The highest kinetic degradation rate constant was observed at kt = 0.0213 min─1 (R2 = 0.9258) and kt = 0.0244 min─1 (R2 = 0.7627) in reactive black-5 from Mangifera indica (L.) and Solanum tuberosum (L.), respectively. The enzyme extracted from the peel of Solanum tuberosum (L.) decolorized Reactive Black-5 and Toluidine Blue-O dyes to a greater extent, i.e., 83.29 and 70.84% with reaction times of 120 and 90 min, respectively.
1 Introduction
One of the causes of pollution is that the peels of fruits and vegetables are considered food waste, which is responsible for pollution. Every year, a large amount of food waste is generated during manufacturing and processing in homes and factories when peeling off edible parts like skin, fat, and crusts (Amini Khoozani et al., 2019; Kashif et al., 2020; Logeswaran et al., 2020). According to the FAO (2013) report, an estimated 1.3 billion tons of food meant for human use are wasted annually, harming the environment and natural resources. According to a previous report, urban food waste in Asian nations is projected to increase from 278 to 416 million tons a year between 2005 and 2025 (Melikoglu et al., 2013). However, this food waste contains many microbes that can potentially degrade color pollutants (Ullah et al., 2018). Dyes are a major contributor to environmental pollution; the food processing, leather, textile, pharmaceutical, cosmetic, and paint industries discharge dyes directly into the water, accounting for 10 to 15% of all environmental contamination (Hassaan et al., 2017; Yadav and Chandra, 2019; Gull et al., 2022). These industries generate significant amounts of waste dye water, which harms aquatic flora and fauna. Synthetic azo dyes and natural, non-toxic dyes have been reported to be carcinogenic when left untreated and negatively impact nature (Bafana et al., 2011). The toxicity of azo dyes increases when streamed into water bodies with high pH, temperature, suspended solids, metals, and salts, causing mutation, entering the food chain, increasing biological and chemical oxygen demand, and inhibiting plant germination due to the reduced amount of dissolved oxygen in the water (Khan and Malik, 2014; Hanafi and Sapawe, 2020). In addition, dyes and other pollutants (heavy metals) cause pH changes, increases in biological and chemical oxygen demand (BOD and COD), and other particulate matter (Idrees et al., 2016). Approximately 7 × 107 tons of synthetic dyestuff are produced annually, of which approximately 10,000 tons are used in the textile sector (Chung, 2016). The majority of dyes feature intricate, enduring structures, such as aromatic compounds. Environmentalists are deeply concerned about the harmful nature of dyes. The use of synthetic dyes and the presence of other auxiliary chemicals add to the extremely hazardous nature of textile wastewater.
When these organic compounds react with various disinfectants, particularly chlorine, they produce by-products (DBPs) that are frequently carcinogenic and thus undesirable (Manzoor and Sharma, 2020).
As a result, these dyes are difficult to degrade chemically, physically, and biologically. They have the potential to pollute underground water, making it susceptible to organism growth. Methylene Blue (MB) is a cationic dye and is used to dye cotton, wool, silk, leather, and to coat paper. It is more poisonous, and its long-term effects cause major health issues, including a faster heartbeat, nausea, shock, cyanosis, jaundice, limb paralysis, mental confusion, etc. Reactive Black-5 (RB5) and Toluidine Blue (TB-O) are anionic toxic dyes that cause damage to aquatic ecosystems and human health. Therefore, dye-contaminated water must be treated before being discharged into the environment (Table 1).
Several methods for degrading and decolorizing dyes, such as chemical, physical, and biological degradation, have been reported to treat water pollution (Chung, 2016; Gičević et al., 2020). The study of metal nanoparticle adsorption is highly effective and rapidly removes azo dyes from aqueous solutions (Moussavi and Mahmoudi, 2009; Unal et al., 2019). However, for economic reasons, the enzyme-arbitrated degradation method is environmentally friendly, cost-effective, and low-energy-consuming (Ekins and Zenghelis, 2021). In contrast, other methods have low effectiveness and low potential for secondary contamination. Jadhav et al. (2011) studied that PPO enzymes exhibit a potential to decolorize 90 and 85% of direct Red 5B (160 μg mL−1) and direct Blue GLL (400 μg mL−1) dyes within 48 and 90 h of reaction time, respectively. Haddaji et al. (2015) reported the possible function of enzyme peroxidases identified in three macrophyte species (Arundo donax, Typha angustifolia, and Phragmites australis) for the degradation of dyes. The results showed that the dye decolorization was about 93 and 83% for amaranth and amido black, respectively, after 120 h of reaction time. PPOs are Cu-containing enzymes that are found in fruits, vegetables, cereal grains, etc. Depending on the species and age of the crop, they can be detected in the plant cells. For example, in potatoes, these enzymes are found in the tuber, while in mangoes, they are found in the skin (peel). PPOs are exposed to browning processes when fruits and vegetables are chopped, which break down their cells. Through their oxidation process, PPOs cause fruits and vegetables to turn brown. The ability of PPOs to oxidize phenolic compounds serves as the foundation for their mode of action. The copper-containing enzyme catalyzes two distinct reactions in the presence of molecular oxygen: (a) the monophenolase activity, which results in the hydroxylation of monophenols at the ortho position; and (b) the diphenolase activity, which oxidizes ortho-diphenols to ortho-quinones, producing pigment and melanin on fruits and vegetables.
The commercial value of food crops can be affected by browning. It may alter their taste, aroma, and nutritional value. As a result, the decay of crops is a factor that produces waste that can affect the biosphere. To date, there has been no report on the decolorization of RB5 by PPOs extracted from Luffa cylindrica (L). In this study, the potential role of three agricultural food-based PPOs [Mangifera indica (L.), Solanum tuberosum (L.), and Luffa cylindrica (L.)] was selected for the decolorization of MB, RB5, and TB-O. Additionally, the most effective ways to optimize various parameters (concentration, time, and pH) influencing the enzyme studies were investigated. The degradation of azo dyes by the PPO enzymes extracted from food waste is an energy-efficient and environmentally friendly approach for wastewater treatment (Mukherjee et al., 2013; Mishra et al., 2019).
2 Materials and methods
2.1 Sample preparation
Ridged gourd (Luffa cylindrica), mango (Mangifera indica), and potato (Solanum tuberosum) were collected from a local fruit and vegetable market in Karachi, Pakistan. The peels of the selected products were sun-dried for 30 days during the summer season, and the dried samples were converted into powder form using a commercial grinder. Finally, the sample was retained in a vial with an appropriate label. Azo dyes such as MB, RB5, and TB-O were supplied by Archroma, located in Karachi, Pakistan. The reagents and chemicals used in this work were potassium dihydrogen phosphate (KH2PO4) and dipotassium hydrogen phosphate (K2HPO4) for the preparation of phosphate buffer, acetic acid (CH3COOH), and sodium acetate (C2H3NaO2) for the preparation of sodium acetate buffer. Acetone (C3H6O) and catechol (C6H6O2), potassium bromide (KBr) for FTIR spectra, and distilled water were used for the preparation of solutions. All chemicals were of analytical grade.
2.2 Extraction of enzyme PPO
Approximately 10 g of each peel powder was dissolved in 0.2 M phosphate buffer maintained at pH 6.8 (120 mL). After homogenization, the sample was filtered through Whatman filter paper and centrifuged at 20,000 rpm for 20 min. PPOs were precipitated from the supernatant by adding up to 100 mL of cold, continuously stirred acetone for 30 min. The precipitate from the mixture was dissolved in 25 mL of phosphate buffer after the mixture was centrifuged at 15,000 rpm for 10 min. The entire process was performed at room temperature.
2.3 Enzyme activity assay
The enzyme activities of PPOs were determined using a UV–visible spectrophotometer (Beckman Coulter), with catechol used as the substrate. The sample vessel contained 2 mL catechol, 1 mL sodium acetate buffer at pH 4.0 (0.2 M), and 1.0 mL enzyme solutions, while the reference vessel contained 2 mL catechol and 1 mL sodium acetate buffer. The absorbance was measured at 420 nm as a one-minute increment (Table 2). One unit of PPO activity is defined as the amount of enzyme that increases the absorbance by 0.001 per minute. The PPO activities were estimated using Equation (1):
where A1S and A2S are the initial and final absorbances of the sample, A1R and A2R are the initial and final absorbances of the control, and t is the reaction time in minutes (3 min). It was observed that the Luffa cylindrica PPOs have a higher value of enzymatic activity, measuring 23.66 U.ml−1, as compared to the PPO enzymes extracted from Solanum tuberosum, which measure 21.33 U.ml−1. On the other hand, the Mangifera indica PPOs show a lower value of enzymatic activity, measuring only 11.0 U.ml−1.
2.4 Activity of PPO on different concentrations of dyes
PPO enzymes derived from different plant resources were studied by monitoring the absorbance at the maximum wavelengths of all the dyes. For instance, the enzymatic activity of MB was monitored at 664 nm, while the activity of Reactive Black—5 and Toluidine Blue—O was examined at wavelengths of 660 and 630 nm, respectively. Enzymatic PPOs from selected resources were mixed with selected sample pollutants (dyes) at increasing concentrations for approximately 2 h. The color removal efficacy was estimated by using unprocessed dyes (control samples) as percent decolorization.
2.5 Effect of time on dye decolorization
The sample contaminated with dyes A, B, and C was incubated with 1 EU/mL of PPO enzyme at pH 6.8 at ambient temperature for 30, 60, 90, and 120-min time intervals. Dye decolorization was monitored at a specified wavelength. The Equation (2) was used to determine the rate of decolorization of azo dyes:
where Ac is the absorbance of the dye before treatment and AT is the absorbance of the dye following treatment.
2.6 Instruments used statistical analysis
An analytical balance, grinder, centrifuge machine, UV–visible spectrophotometer (Beckman Coulter), FTIR (Thermo Nicolet 700), and pH meter were the instruments used in this experiment. Statistical analysis of experimental data, such as correlation coefficient (R2) and pseudo-degradation rate (k), was carried out using SAP Interactive Excel.
3 Results and discussion
3.1 Effect of time on dye decolorization by Mangifera indica (L.) PPOs
The results of the experiment can be seen in Figure 1, which shows the effect of the PPO enzyme extracted from Mangifera indica (L) peel on the decolorization of MB, RB5, and TB-O over time. At pH 6.8 and concentrations of 1.71 × 10−5 M (Conc. 1) and 7.15 × 10−5 M (Conc. 2), only 24 and 9% of MB were decolored, respectively, as shown in Figure 1A. Conversely, at a concentration of 8.57 × 10−5 M (Conc. 3), 19% of MB was decolorized after 90 min. Figures 1B,C show that the rate of decolorization for RB5 and TB-O was high up to 60 min and then stopped at three different concentrations. In a reported study, biosynthesized silver nanoparticles (Ag-NPs) were synthesized using Mangifera indica gum powder to decolorize MB. According to the reported results, Mangifera indica PPOs with Ag-NPs completely decolorized the MB after 75 min of exposure to UV light (Panneerselvi et al., 2022).
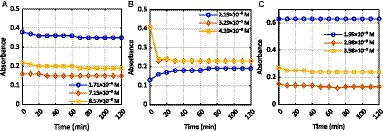
Figure 1. Effect of time on the activity of Mangifera indica (L.) PPOs against the dyes (A) MB, (B) RB5, and (C) TB-O.
The effect of PPOs extracted from mango peel (Mangifera indica) on the degradation of MB was observed by exposing the solution to UV (664 nm) for 120 min. The gradual decolorization of the dye occurred at a concentration of 1.71 × 10−5 M until 90 min had elapsed. At the 100-min mark, the enzyme activity stopped as the absorbance of the solution remained constant, indicating a stable concentration. It was also observed that the enzyme PPO stopped working after 30 min (at a concentration of 7.15 × 10−5 M) and 80 min (at a concentration of 8.57 × 10−5 M), respectively (Supplementary Table S1A). Figure 1B displays the activity of the Mangifera indica (L.) enzyme in breaking down RB5 at three different concentrations. During the experiment, it was observed that the decolorization of RB5 (2.19 × 10−5 M) increased with the concentration of the solution, as evidenced by the increase in absorbance (Supplementary Table S1B). This caused more light to be absorbed by the sample, resulting in a small amount of light being detected by the detector. It is possible that the formation of a complex in the solution also contributed to the increase in absorbance. In the cases of Conc. 2 (3.29 × 10−5 M) and Conc. 3 (4.39 × 10−5 M), RB5 degraded rapidly within the first 10 min of the process. After this initial period, the absorbance of the dye-containing Mangifera indica PPOs became stable, indicating that no further degradation of the dye had occurred. During the course of the experiment (Figure 1C), it was observed that the PPO enzyme began the process of decolorizing the dye after 40 min, which continued up to 70 min. However, the enzyme activity came to a halt between 80 and 120 min. At a concentration of 2 (2.98 × 10−5 M), the enzyme exhibited a slow degradation rate of TB-O up to 70 min, after which the absorbance of the solution increased (Supplementary Table S1C), indicating an upsurge in concentration. Further investigation revealed that complex formation had occurred within the sample solution, leading to the observed increase. Additional research is required to ascertain the precise nature of the complex that was formed. In a previous study, the degradation of dyes by Mangifera indica PPOs coupled with metallic nanoparticles was reported. For example, the synthesis of iron nanoparticles from leaf extract (Mangifera indica) was examined for its degradation potential for the photocatalytic removal of dyes (Congo red and Brilliant Green) from wastewater. The results showed that the maximum percentage of photo-catalytic degradation achieved was 72.6 and 80.87% for Congo Red, and 72.88 and 82% for Brilliant Green, with biomass and NPs, respectively (Zulfikar et al., 2021). However, in the present study, the decolorization of selected textile dyes was studied without the combination of any other technique. In this study, the biodegradation of selected textile dyes was investigated only by extracting PPOs from the peels of Mangifera indica, which is considered food waste.
3.2 Effect of time on dye decolorization by Solanum tuberosum (L.) PPOs
Figure 2 shows the effectiveness of the PPO enzyme, which was extracted from Solanum tuberosum (L.) peels, in discoloring azo dyes for 120 min. During the experiment, the absorbance of the solution continuously decreased and eventually stabilized after 70 min. This indicates that the enzyme was active in breaking down MB at concentrations of 1.71 × 10−5 M and 7.15 × 10−5 M, respectively (Figure 2A). At 8.57 × 10−5 M, the absorbance of the solution quickly decreased until 30 min, but it then gradually decreased until 70 min and remained stable between 80 and 120 min (Supplementary Table S2A). This suggests that the activity of the PPO enzymes, which were extracted from Solanum tuberosum (L.), stopped.
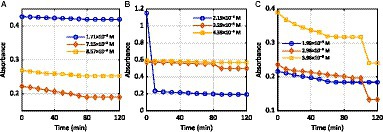
Figure 2. Effect of time on the activity of Solanum tuberosum (L.) PPOs against the dyes (A) MB, (B) RB5, and (C) TB-O.
In our previous study, potato stem powder (PSP) and potato leaf powder (PLP) were used as adsorbents to remove MB and malachite green dyes from an aqueous solution (Gupta et al., 2016). As shown in Figure 2B, the PPO enzymes actively degraded RB5 within the first 10 min of the experiment, as indicated by the sudden drop in absorbance (Supplementary Table S2B). After that, no enzymatic activity was observed in the degradation of RB5 (2.19 × 10−5 M). The enzyme, extracted from Solanum tuberosum (L.), gradually degraded the RB5 (3.29 × 10−5 M and 4.39 × 10−5 M) until 80 min had elapsed. At that point, there was a quick fall in absorbance as the enzymatic activity became faster. However, from 90 min onwards, the enzyme did not show any further activity in the dye degradation process. Samarghandy et al. (2011) investigated the biosorption of RB5 from an aqueous solution using biomass prepared from Solanum tuberosum peel. The removal rates were 81.21 and 95.06% for 0.2 and 1.0 g of biomass, respectively.
The degradation of TB-O by Solanum tuberosum PPOs at different concentrations was studied to determine the effect of time. The results, shown in Figure 2C, indicate that the reaction continued for 70 min. After that, the enzymatic activity ceased, as indicated by the stable absorbance of the solution (as shown in Supplementary Table S2C) at 1.99 × 10−5 M. However, at concentrations of 2.98 × 10−5 and 3.98 × 10−5 M, the rates of dye decolorization were relatively low after 60 and 50 min, respectively. This may be due to the inhibition of the product. These results confirmed previously published research (Saravanan et al., 2017).
3.3 Effect of time on dye decolorization by Luffa cylindrica (L.) PPOs
The decolorization of selected color pollutants by Luffa cylindrica (L.) PPOs were examined by varying the times of incubation at three different concentrations. In the current study, three different azo dyes were selected as sample contaminants for decolorization. Our results revealed that the highest decolorization rate of dye A, i.e., MB, at the concentration of 8.57× 10ˉ5 M (conc. 3), was found to be 64% after 2 h of the experiment, as shown in Figure 3A.
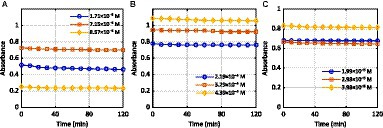
Figure 3. Effect of time on the activity of Luffa cylindrica (L.) PPOs against the dyes (A) MB, (B) RB5, and (C) TB-O.
Figures 3B,C demonstrate the activity of Luffa cylindrica (L.) PPOs against RB5 dye, resulting in 39% degradation at a low concentration of 2.19× 10ˉ5 M and a retention duration of 10 min. In the case of TB-O, the highest degradation was observed at a concentration of 2.98 × 10ˉ5 M after 2 h of the experiment (Supplementary Table S3). This may indicate that under environmental conditions, the highest dose of Luffa cylindrica (L.) PPOs is required for the complete removal of selected dyes. The limited experimental data available are not sufficient to fully support the claim that the degradation of selected dyes from the PPOs extracted from Luffa cylindrica peels, considered food waste, is a suitable method from an environmental point of view. However, the results of the experiments do show promise. Further research is needed to determine the viability of this method on a large scale. The studies indicate that fungal immobilization and biosorption have been evaluated to determine their effects on the native structure of Luffa cylindrica to degrade dyes. For example, cells of Proteus vulgaris NCIM-2027 immobilized on Luffa cylindrica completely decolorized C.I. Reactive Blue 172. The immobilized cells were used to continuously decolorize single and reactive dye mixtures in a fixed-bed bioreactor (Saratale et al., 2011). Another study evaluated the effects of fungal immobilization and biosorption on the structure of Luffa cylindrica by using a scanning electron microscope. The results showed that A. terreus QMS-1 can remove up to 92% of the dye in just 24 h when 1% glucose and 1% ammonium sulfate are present at pH 5.0. Furthermore, the bioreactor was operated continuously for 12 h with 100 ppm of Congo Red dye in simulated textile effluent, resulting in 97% decolorization (Laraib et al., 2020).
3.4 Effect of initial concentration on dye decolorization
Figures 4A–C shows the effect of concentration on the decolorization of azo dye by the PPO enzymes from Mangifera indica (L.), Solanum tuberosum (L.), and Luffa cylindrica (L.). The decolorization rate of RB5 by Mangifera indica PPOs was found to be the highest (54.97%) at concentration 3 (4.39 × 10−5 M), as indicated in Table 3. The concentration was recorded in ascending order, and the results indicate that higher concentrations have a greater effect on the decolorization of the dye.
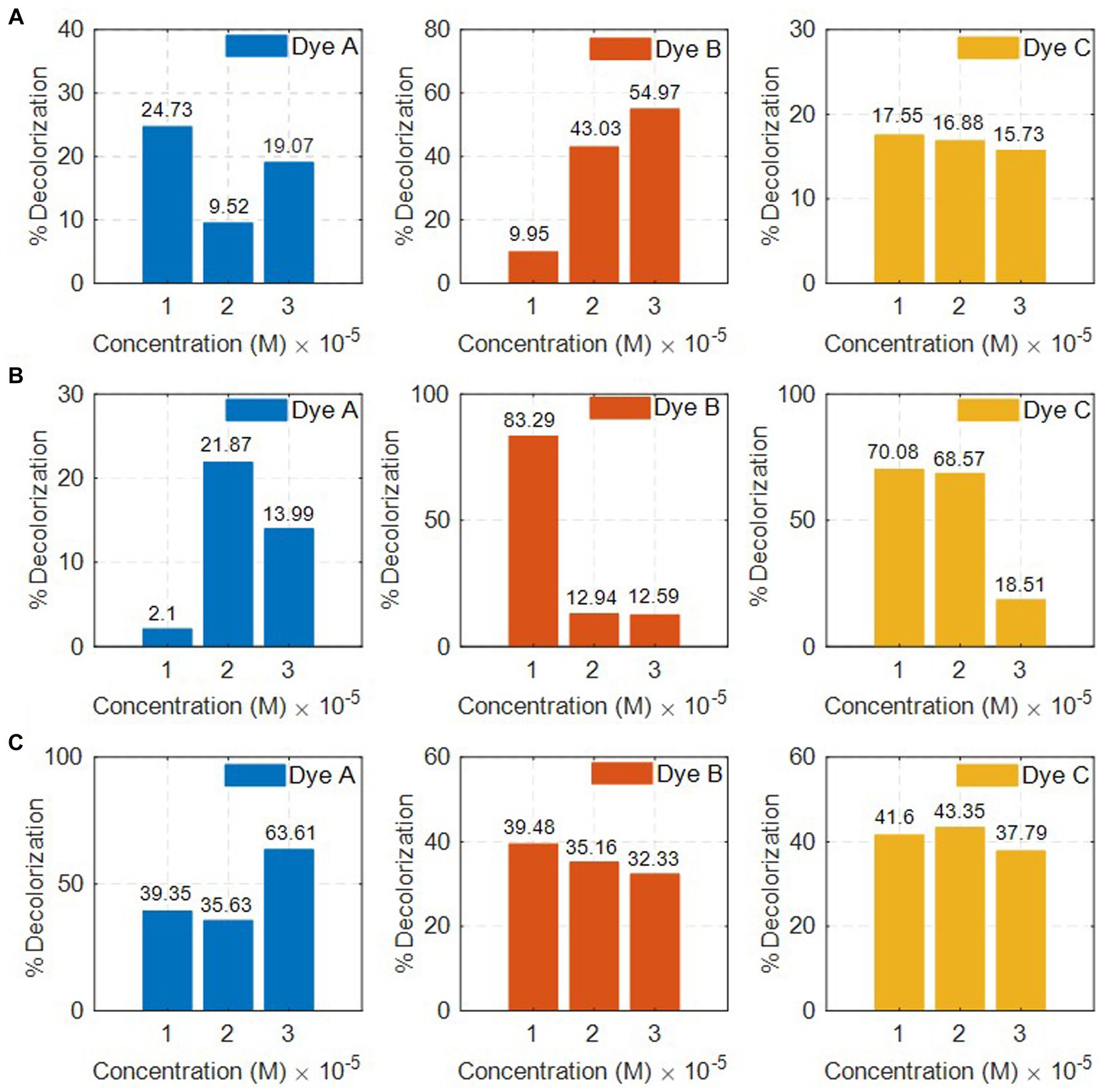
Figure 4. Decolorization of dyes by (A) Mangifera indica (L.), (B) Solanum tuberosum (L.), and (C) Luffa cylindrica (L.) w.r.t. concentration.
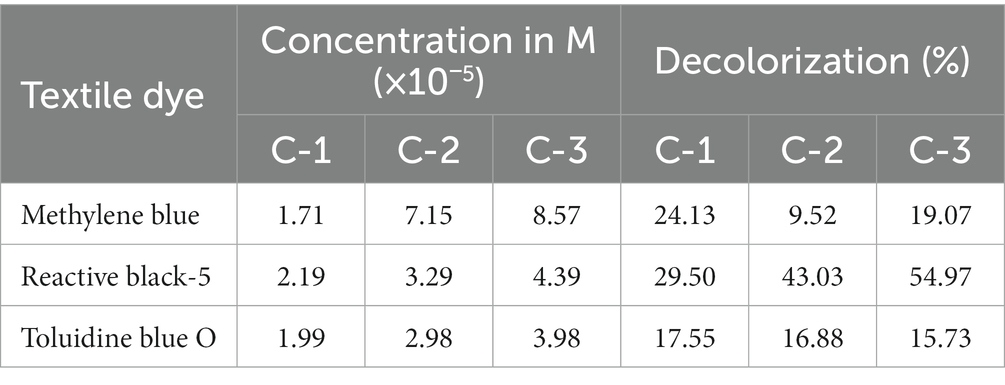
Table 3. Percent decolorization of textile dyes by Mangifera indica (L.) at different concentrations.
A study found that Mangifera indica effectively removes 87% of safranin-T was observed from Mangifera indica as a precursor (Benjelloun et al., 2021). Although there have been studies on the removal of dyes from various sources, none have been conducted on the removal of dyes from the PPO enzyme extracted from the peel of Mangifera indica. The rate of decolorization of dyes by PPOs from Solanum tuberosum and Luffa cylindrica is provided in Tables 4, 5, respectively. The results indicate that the PPO enzymes extracted from Solanum tuberosum caused a significant reduction in the color of RB5 dye by 83.29% at a concentration of 2.19 × 10−5 M. On the other hand, Luffa cylindrica PPOs showed a high removal rate (43.35%) for TB-O dye among all the dyes tested at a concentration of 2.98 × 10−5 M.
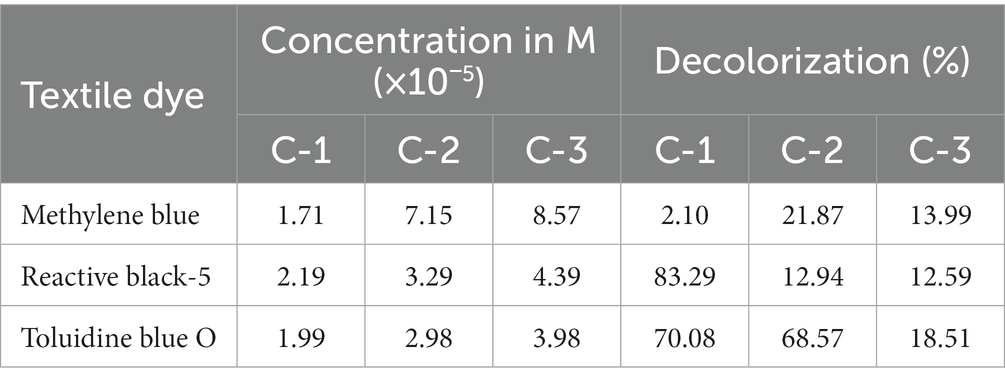
Table 4. Percent decolorization of textile dyes by Solanum tuberosum (L.) at different concentrations.
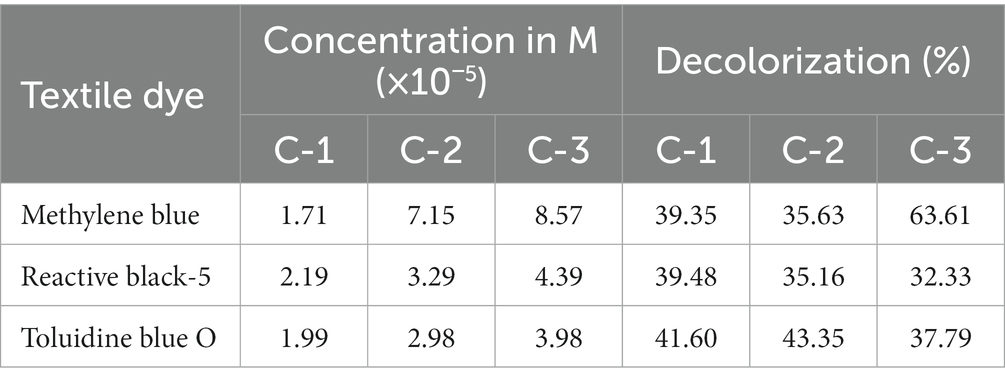
Table 5. Percent decolorization of textile dyes by Luffa cylindrica (L.) at different concentrations.
3.5 Adsorption kinetics
The adsorption phenomenon can be interpreted by applying a kinetic model such as pseudo-first order using Equation (3):
where k1 stands for pseudo-first-order, while qeq and qt represent the biosorbent amount of selected dyes at equilibrium and the biosorbent amount of selected dyes at a specific time (t), respectively.
A linear kinetic model based on the experimental values obtained for removing dyes and a linearized kinetic model fitting was applied to investigate the kinetic mechanism. Tables 6–8 show that the pseudo-first model was best fitted with a high regression coefficient value of R2 = 0.92 on dye A compared with other dyes at constant dye concentration at an equilibrium time of 30 min with Solanum tuberosum (L.) PPOs. The uptake of dyes was so fast in the initial 20 min, and later the adsorption decreased. The adsorption capacity was enhanced with a continuous increase in the concentration of dyes. These results suggested that the adsorption of dyes followed a rate-determining step in which the adsorbent and dye molecules were well-interacted (Benjelloun et al., 2021). Concentration is a sensitive parameter that seriously affects the adsorption capacity of adsorbents, dramatically changing the initial concentration’s adsorption values.
3.6 Analysis of dye structure
Fourier transform-infrared (FTIR) spectral analysis was performed on selected dyes with and without the infusion of the PPO enzymes. The IR spectra of MB at 567.07 cm−1 indicated the alkyl halide (C-C stretching), as shown in Figure 5. Furthermore, aromatic amines that are C-N stretching were detected at 1147.65 cm−1. In addition, the spectra at 1394.53, 1610, and 1643.35 cm−1 indicated C-C ring stretching, N-N curve stretching, and C=C double bond stretching. Before sample treatment, hydrogen atoms bonded by S-H and O-H stretching were observed at 2372.44 and 3444.87 cm−1, respectively. The peaks at 1147.65 and 1394.53 cm−1 disappeared once the contaminant in the sample was treated with the PPO enzymes, and a new peak at 1083.99 cm−1 indicated the carbon–oxygen stretching (Figure 5B).
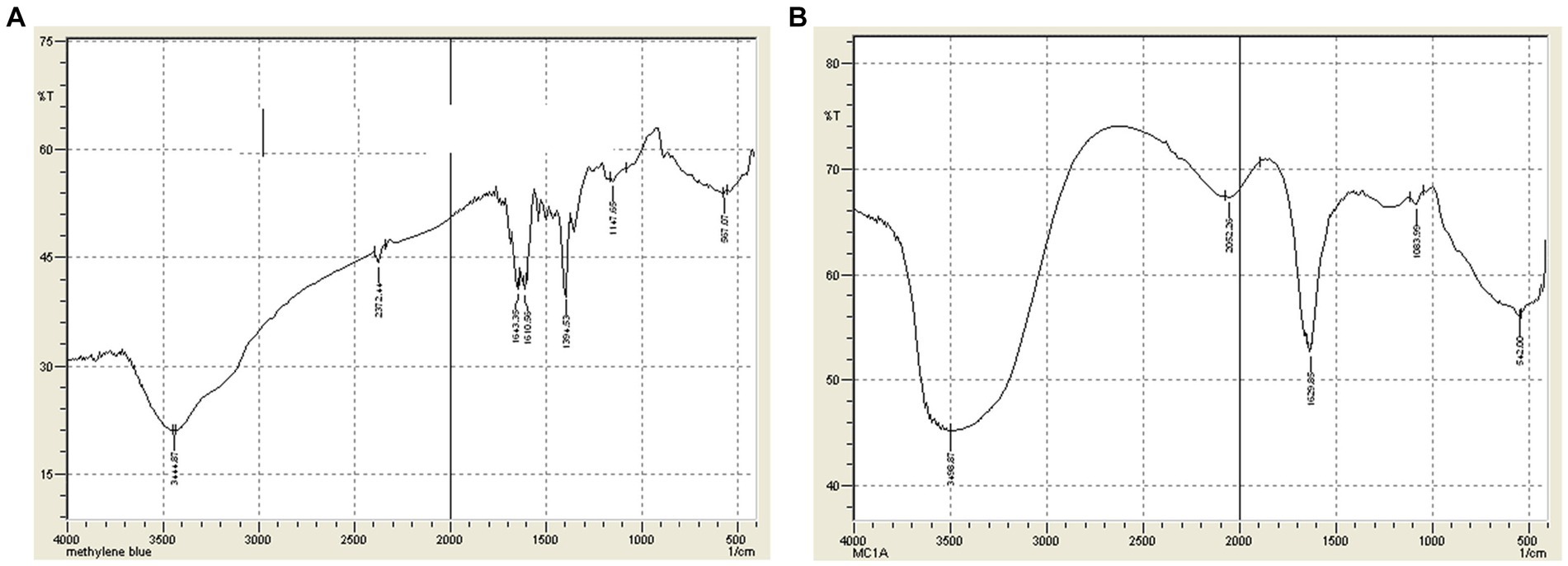
Figure 5. (A) FTIR Spectra of Dye A (MB) before treatment. (B) FTIR Spectra of Dye A (MB) after treatment.
In the spectra of Dye B (RB5), Carbon-Hydrogen bonds were observed at 603.72 and 653.87 cm−1, while N-H wag (1°, 2° amines) were assigned to the peaks located at 833.25 cm−1 and 871.82 cm−1. The C-N stretching was observed at 1022.27, 1134.14, and 1321.24 cm−1 due to the aliphatic and aromatic amines. The N-H curve was noted at 1604.77 cm−1. Furthermore, the C-C ring stretching was observed at 1446.61 cm−1. The peak at 2085.05 cm−1 2927.94 cm−1 was caused by the presence of N=C=C stretching and H-C=O: C-H stretching, respectively. This may have been caused by the presence of the aldehyde group. The –C ≡ C-H: C-H stretching was observed at 3315.6 cm−1 and the C-H stretching was observed at 3199.9 cm−1. However, it was noted that new peaks were observed at 1000, 1500.1, 1494.05 cm−1, 2085.05 cm−1, and 3500.13 cm−1 due to the stretching of the C-O, C-C single bond, N=C=C, O-H, and asymmetric nitro compound N-O, respectively (Figures 6A,B).
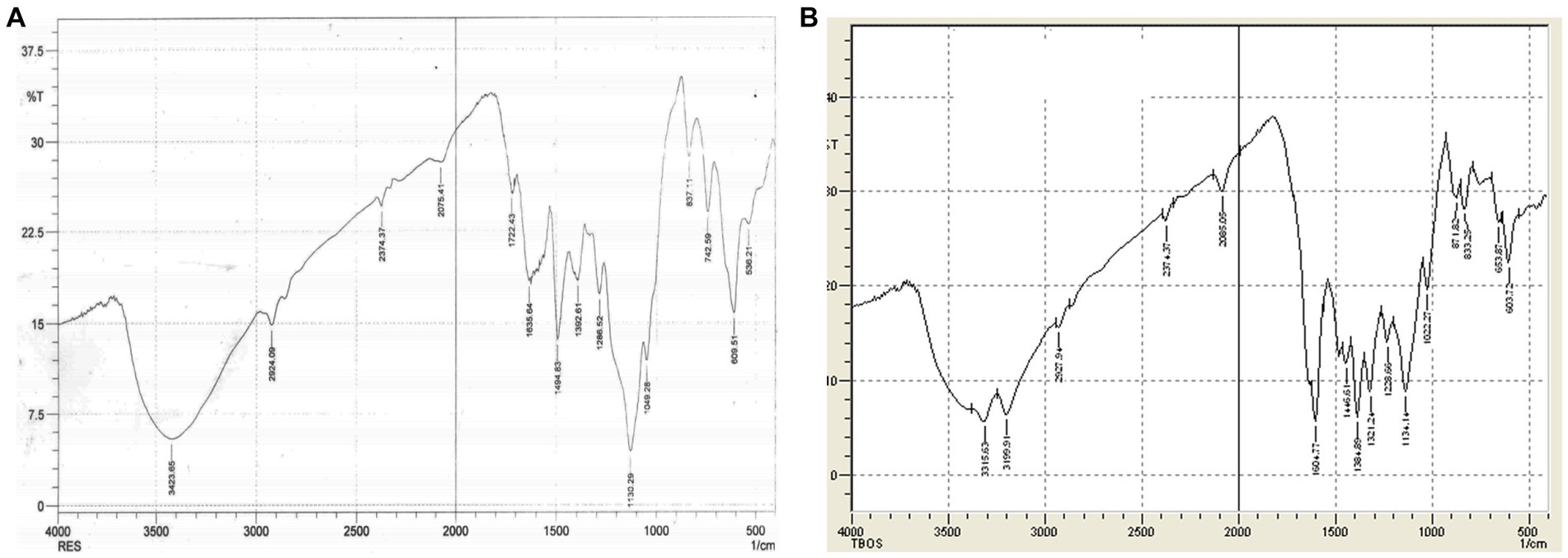
Figure 6. (A) FTIR Spectra of Dye B (RB5) before treatment. (B) FTIR Spectra of Dye B (RB5) after treatment.
The IR spectra of dye C (TB-O) revealed the presence of an alkyl halide group at 540.1 cm−1. Further examination of the spectra showed that the C-O stretching was visible at 1085 cm−1. In addition, the peak at 1637.6 cm−1 showed the presence of N-H bending, while the peak at 3456.4 cm−1 confirmed the presence of O-H stretching. Despite the successful treatment of the TB-O, small peaks were shown due to the presence of alkyl halide (C-Cl) at 875.70 cm−1. Following the peak at 1550.77 cm−1, the asymmetric stretching of the N-O bond was observed. Finally, the appearance of N-H bending and O-H stretching followed by H-bond was observed at 1656.9 cm−1 and 3508.52 cm−1 (Figures 7A,B).
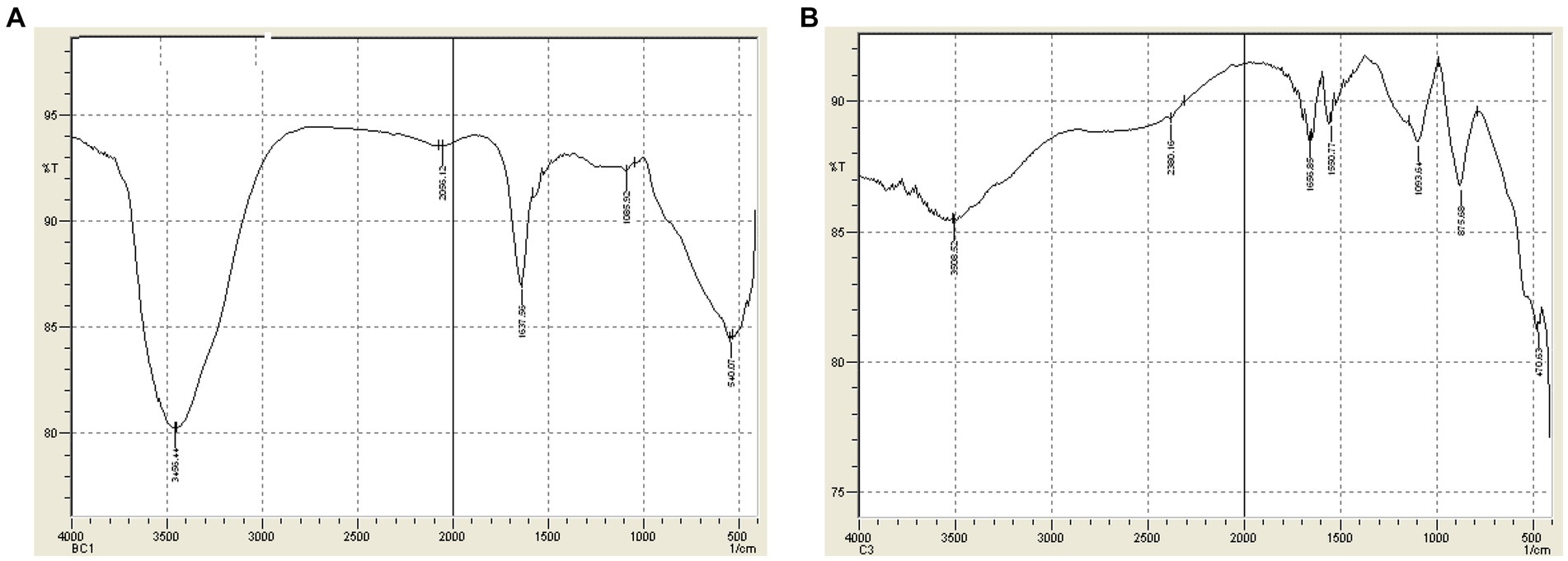
Figure 7. (A) FTIR Spectra of Dye C (TB-O) before treatment. (B) FTIR Spectra of Dye C (TB-O) after treatment.
4 Conclusion
Our research shows that the PPO enzyme was successfully extracted and purified from fruit and vegetable peels. The PPO enzymes derived from Mangifera indica (L.), Solanum tuberosum (L.), and Luffa cylindrica (L.) was applied to three different azo dyes (MB, RB5, and TB-O) for decolorization. The results showed that PPOs derived from Solanum tuberosum (L.) and Mangifera indica (L.) could effectively discolor RB5 dye up to 83.29% (2.19 × 10−5 M) and 54.97% (4.39 × 10−5 M) in comparison to MB and TB-O dyes, while PPOs from Luffa cylindrica (L.) were noticeably more successful than RB5 and TB-O azo dyes, up to 63.61% (8.57 × 10−5 M). In conclusion, the three distinct azo dyes discovered in the effluents generated by the dye industry can be readily discolored by the PPO enzymes, which are derived from a variety of natural sources.
Data availability statement
The original contributions presented in the study are included in the article/Supplementary material, further inquiries can be directed to the corresponding authors.
Author contributions
JU: Conceptualization, Data curation, Funding acquisition, Supervision, Writing – original draft. MI: Conceptualization, Formal analysis, Software, Writing – review & editing. HA: Methodology, Software, Writing – original draft, Formal analysis. SB: Formal analysis, Writing – review & editing, Software. TR: Formal analysis, Writing – review & editing. SM: Formal analysis, Software. AT: Formal analysis, Writing – review & editing. AM: Formal analysis, Writing – review & editing. HU: Formal analysis, Writing – review & editing. SM: Formal analysis, Writing – review & editing.
Funding
The author(s) declare that no financial support was received for the research, authorship, and/or publication of this article.
Acknowledgments
The authors extend their appreciation to the Dean of Scientific Research at King Khalid University for supporting this work through the Large Group Research Program under grant number RGP.2/381/44.
Conflict of interest
The authors declare that the research was conducted in the absence of any commercial or financial relationships that could be construed as a potential conflict of interest.
Publisher’s note
All claims expressed in this article are solely those of the authors and do not necessarily represent those of their affiliated organizations, or those of the publisher, the editors and the reviewers. Any product that may be evaluated in this article, or claim that may be made by its manufacturer, is not guaranteed or endorsed by the publisher.
Supplementary material
The Supplementary material for this article can be found online at: https://www.frontiersin.org/articles/10.3389/fsufs.2023.1320855/full#supplementary-material
References
Amini Khoozani, A., Birch, J., and Bekhit, A. E.-D. A. (2019). Production, application and health effects of banana pulp and peel flour in the food industry. J. Food Sci. Technol. 56, 548–559. doi: 10.1007/s13197-018-03562-z
Bafana, A., Devi, S. S., and Chakrabarti, T. (2011). Azo dyes: past, present and the future. Environ. Rev. 19, 350–371. doi: 10.1139/a11-018
Benjelloun, M., Miyah, Y., Evrendilek, G. A., Zerrouq, F., and Lairini, S. (2021). Recent advances in adsorption kinetic models: their application to dye types. Arab. J. Chem. 14:103031. doi: 10.1016/j.arabjc.2021.103031
Chung, K.-T. (2016). Azo dyes and human health: a review. J. Environ. Sci. Health C 34, 233–261. doi: 10.1080/10590501.2016.1236602
Ekins, P., and Zenghelis, D. (2021). The costs and benefits of environmental sustainability. Sustain. Sci. 16, 949–965. doi: 10.1007/s11625-021-00910-5
Gičević, A., Hindija, L., and Karačić, A., Toxicity of azo dyes in pharmaceutical industry, CMBEBIH 2019: Proceedings of the international conference on medical and biological engineering, 16–18 may 2019, Banja Luka, Bosnia and Herzegovina, Springer, (2020), 581–587.
Gull, S., Batool, S., Li, G., and Idrees, M. (2022). Synthesis of cesium lead halide perovskite/zinc oxide (CsPbX3/ZnO, X= Br, I) as heterostructure photocatalyst with improved activity for heavy metal degradation. Front. Chem. 10:1020484. doi: 10.3389/fchem.2022.1020484
Gupta, N., Kushwaha, A. K., and Chattopadhyaya, M. (2016). Application of potato (Solanum tuberosum) plant wastes for the removal of methylene blue and malachite green dye from aqueous solution. Arab. J. Chem. 9, S707–S716. doi: 10.1016/j.arabjc.2011.07.021
Haddaji, D., Bousselmi, L., Saadani, O., Nouairi, I., and Ghrabi-Gammar, Z. (2015). Enzymatic degradation of azo dyes using three macrophyte species: Arundo donax, Typha angustifolia and Phragmites australis. Desalin. Water Treat. 53, 1129–1138. doi: 10.1080/19443994.2014.884475
Hanafi, M. F., and Sapawe, N. (2020). A review on the water problem associate with organic pollutants derived from phenol, methyl orange, and remazol brilliant blue dyes. Materials Today: Proceedings 31, 141–150. doi: 10.1016/j.matpr.2021.01.258
Hassaan, M. A., El Nemr, A., and Hassaan, A. (2017). Health and environmental impacts of dyes: mini review, American. J. Environ. Sci. Engineer. 1, 64–67. doi: 10.11648/j.ajese.20170103.11
Idrees, M., Batool, S., Hussain, Q., Ullah, H., Al-Wabel, M. I., Ahmad, M., et al. (2016). High-efficiency remediation of cadmium (Cd2+) from aqueous solution using poultry manure–and farmyard manure–derived biochars. Sep. Sci. Technol. 51, 2307–2317. doi: 10.1080/01496395.2016.1205093
Jadhav, U. U., Dawkar, V. V., Jadhav, M. U., and Govindwar, S. P. (2011). Decolorization of the textile dyes using purified banana pulp polyphenol oxidase. Int. J. Phytoremediation 13, 357–372. doi: 10.1080/15226514.2010.495142
Kashif, M., Awan, M., Nawaz, S., Amjad, M., Talib, B., Farooq, M., et al. (2020). Untapped renewable energy potential of crop residues in Pakistan: challenges and future directions. J. Environ. Manag. 256:109924. doi: 10.1016/j.jenvman.2019.109924
Khan, S., and Malik, A. (2014). “Environmental and health effects of textile industry wastewater” in Environmental deterioration and human health: Natural and anthropogenic determinants, Eds. Abdul Malik, Elisabeth Grohmann and Rais Akhtar. Springer. 55–71.
Laraib, Q., Shafique, M., Jabeen, N., Naz, S. A., Nawaz, H. R., Solangi, B., et al. (2020). Luffa cylindrica immobilized with aspergillus terreus QMS-1: an efficient and cost-effective strategy for the removal of Congo red using stirred tank reactor. Pol. J. Microbiol. 69, 193–203. doi: 10.33073/pjm-2020-022
Logeswaran, J., Shamsuddin, A. H., Silitonga, A. S., and Mahlia, T. M. I. (2020). Prospect of using rice straw for power generation: a review. Environ. Sci. Pollut. Res. 27, 25956–25969. doi: 10.1007/s11356-020-09102-7
Manzoor, J., and Sharma, M. (2020). Impact of textile dyes on human health and environment, impact of textile dyes on public health and the environment IGI Global, 162–169.
Melikoglu, M., Lin, C. S. K., and Webb, C. (2013). Analysing global food waste problem: pinpointing the facts and estimating the energy content, central European. J. Engineer. 3, 157–164. doi: 10.2478/s13531-012-0058-5
Mishra, S., Chowdhary, P., and Bharagava, R. N. (2019). Conventional methods for the removal of industrial pollutants, their merits and demerits. Emerg. Eco-friendly Approaches for Waste Manag., 1–31. doi: 10.1007/978-981-10-8669-4_1
Moussavi, G., and Mahmoudi, M. (2009). Degradation and biodegradability improvement of the reactive red 198 azo dye using catalytic ozonation with MgO nanocrystals. Chem. Eng. J. 152, 1–7. doi: 10.1016/j.cej.2009.03.014
Mukherjee, S., Basak, B., Bhunia, B., Dey, A., and Mondal, B. (2013). Potential use of polyphenol oxidases (PPO) in the bioremediation of phenolic contaminants containing industrial wastewater. Rev. Environ. Sci. Biotechnol. 12, 61–73. doi: 10.1007/s11157-012-9302-y
Panneerselvi, V., Shankar, K., Muthukrishnan, P., and Prabhu, A. (2022). Mangifera indica resin assisted synthesis of nano silver: assessing their photocatalytic degradation of methylene blue, anticorrosive and antioxidant activity. J. Clust. Sci. 33, 123–133. doi: 10.1007/s10876-020-01965-7
Samarghandy, M. R., Hoseinzadeh, E., Taghavi, M., and Rahmani, A. (2011). Biosorption of reactive black 5 from aqueous solution using acid-treated biomass of potato peel waste. Bioresources 6, 4840–4855. doi: 10.15376/biores.6.4.4840-4855
Saratale, G. D., Saratale, R. G., Chang, J. S., and Govindwar, S. P. (2011). Fixed-bed decolorization of reactive blue 172 by Proteus vulgaris NCIM-2027 immobilized on Luffa cylindrica sponge. Int. Biodeterior. Biodegradation 65, 494–503. doi: 10.1016/j.ibiod.2011.01.012
Saravanan, C., Rajesh, R., Kaviarasan, T., Muthukumar, K., Kavitake, D., and Shetty, P. H. (2017). Synthesis of silver nanoparticles using bacterial exopolysaccharide and its application for degradation of azo-dyes. Biotechnology Reports 15, 33–40. doi: 10.1016/j.btre.2017.02.006
Ullah, A., Khan, D., Khan, I., and Zheng, S. (2018). Does agricultural ecosystem cause environmental pollution in Pakistan? Promise and menace. Environ. Sci. Pollut. Res. 25, 13938–13955. doi: 10.1007/s11356-018-1530-4
Unal, B. O., Bilici, Z., Ugur, N., Isik, Z., Harputlu, E., Dizge, N., et al. (2019). Adsorption and Fenton oxidation of azo dyes by magnetite nanoparticles deposited on a glass substrate. J. Water Process Engineer. 32:100897. doi: 10.1016/j.jwpe.2019.100897
Yadav, S., and Chandra, R. (2019). “Environmental health hazards of post-methanated distillery effluent and its biodegradation and decolorization” in Environmental biotechnology: For sustainable future, Eds. Ranbir Chander Sobti, Naveen Kumar Arora and Richa Kothari. Springer. 73–101.
Keywords: food waste, polyphenol oxidase, textile dyes, biodegradation, wastewater treatment
Citation: Uddin J, Idrees M, Ahmed H, Batool S, Rahman TU, Mehmood S, Tanoli AK, Muhsinah AB, Ullah H and Musharraf SG (2024) Biodegradation and decolorization of methylene blue, reactive Black-5, and toluidine blue-O from an aqueous solution using the polyphenol oxidase enzyme. Front. Sustain. Food Syst. 7:1320855. doi: 10.3389/fsufs.2023.1320855
Edited by:
Muzaffar Hasan, Central Institute of Agricultural Engineering (ICAR), IndiaReviewed by:
Gunjan Mukherjee, Chandigarh University, IndiaChristine Jeyaseelan, Amity University, India
Copyright © 2024 Uddin, Idrees, Ahmed, Batool, Rahman, Mehmood, Tanoli, Muhsinah, Ullah and Musharraf. This is an open-access article distributed under the terms of the Creative Commons Attribution License (CC BY). The use, distribution or reproduction in other forums is permitted, provided the original author(s) and the copyright owner(s) are credited and that the original publication in this journal is cited, in accordance with accepted academic practice. No use, distribution or reproduction is permitted which does not comply with these terms.
*Correspondence: Muhammad Idrees, bS5pZHJlZXM4MjIzQGdtYWlsLmNvbQ==; Jalal Uddin, amFsYWx1ZGRpbmFtaW5AZ21haWwuY29t