- 1Department of Biology, Carleton University, Ottawa, ON, Canada
- 2SNSB-Bavarian State Collection of Zoology, Munich, Germany
- 3State Museum of Natural History Stuttgart, Stuttgart, Germany
- 4Agriculture and Agri-Food Canada, Ottawa, ON, Canada
Microgastrinae parasitoid wasps (Hymenoptera: Braconidae) were studied in the St. Lawrence Lowlands ecoregion (~14,100 km2) in Ontario, Canada. This subfamily is one of (if not the) most species-rich clades of Lepidoptera parasitoids and has important applications in the biological control of agricultural pests. The St. Lawrence Lowlands ecoregion is one of the nine southern Canadian ecoregions to be identified as a “crisis ecoregion,” having high biodiversity, high risk of biodiversity loss, and low proportion of land included in protected areas. A total of 3,481 specimens collected from 1905 to 2021 within the region were studied. Two species are recorded for the first time in the Nearctic: Apanteles minornavarroi Fernandez-Triana, 2014 and Protapanteles anchisiades (Nixon, 1973); two species are recorded for the first time in Canada: Promicrogaster virginiana Fernandez-Triana, 2019 and Protapanteles immunis (Haliday, 1834); and two are recorded for the first time in Ontario: Cotesia plathypenae (Muesebeck, 1921) and Alphomelon winniewertzae Deans, 2003. DNA-barcode sequences for 2,173 specimens and 66% of the formally described species were successfully recovered. Using a combination of DNA barcodes and morphological assessment, we document herein a minimum putative species count of 228 and a maximum count of 304. We assess the accuracy of species identification in the ecoregion through DNA barcodes and discuss the use of Barcode Index Numbers (BINs) for species discovery in this taxon. Using BINs, 83% of the formally described species with molecular data can be successfully discriminated. The incredible diversity revealed by DNA-barcoding and the high risk of biodiversity loss in the ecoregion highlight the need for increased taxonomic efforts in this taxon to catalog species before they are potentially lost. Several species are present solely in unique habitats within the study area, such as Sphagnum bogs and wetlands. Other (semi) natural features important for these beneficial insects include hedgerows, riparian zones, ditch banks, and wooded areas. Enrichment of these habitats in proximity to field crops could help maintain microgastrine populations and control Lepidoptera crop pests.
Introduction
Recently, declines in insect populations and biodiversity have become a major concern (Forister et al., 2019; Didham et al., 2020). Anthropogenic activities resulting in habitat loss, habitat fragmentation, and climate change put many insect species at risk of extinction or extirpation (Brühl et al., 2021; Wagner et al., 2021). Insect taxa whose species remain largely undescribed experience an increased risk of losing species that have never been discovered and cataloged. These taxa have been called “dark taxa” (Page, 2016) and contain species that are typically small in size, morphologically cryptic, and hyper-diverse, which often results in them receiving insufficient attention from taxonomists (Hausmann et al., 2020). Hartop and colleagues define them as “groups for which <10% of all species are described and the estimated diversity exceeds 1,000 species” (Hartop et al., 2022, p. 1404). Dark taxa often suffer from a lack of adequate, up-to-date identification literature, high-quality figures or images, lost types, and other historical backlogs. This renders them difficult to work with, even for experts, which can adversely impact biodiversity assessments and species-targeted conservation efforts.
DNA barcoding first gained attention in 2003 with its promise to transform taxonomy by its ability to ascribe a unique identifier, or “barcode” to arthropods (Hebert et al., 2003). Sequences are clustered by different algorithms into molecular operational taxonomic units (MOTUs) (Rosero-Garcia et al., 2017). The Barcode of Life Data System (BOLD; Ratnasingham and Hebert, 2007) uses a Refined Single Linkage Analysis to generate taxonomic units called Barcode Index Numbers (BINs; Ratnasingham and Hebert, 2013). MOTUs (such as BINs) have often been invoked as species proxies: Chimeno et al. (2022) used BINs as species proxies in four dipteran dark taxa to suggest that over a thousand species remain undescribed in Germany; Huemer et al. (2020) used BINs in European Gelechiidae to suggest that both a large number of species remain undescribed and that cryptic species diversity is present; Christian Schmid-Egger and Stefan Schmidt have used BINs when assessing the diversity in many European Hymenoptera taxa (Schmid-Egger et al., 2017; Schmid-Egger and Schmidt, 2021; Schmid-Egger et al., 2024, for some). BINs, however, are not always concordant with species boundaries. Whether or not any MOTU makes an appropriate proxy for species depends on how often they inaccurately represent a taxon’s species concepts (Ryberg, 2015).
Microgastrinae (Hymenoptera: Braconidae) is a subfamily of parasitoid wasps that attacks Lepidoptera larvae. As one of the most speciose groups of Lepidoptera parasitoids, they are economically important as biological control agents of commercial agriculture and forestry pests (Whitfield, 1997). Despite currently including over 3,000 described species (Shaw, 2022), it is estimated that only 5–10% of the subfamily has been described (Rodriguez et al., 2013; Fernandez-Triana et al., 2020), qualifying the subfamily as a dark taxon sensu Hartop et al. (2022). Current traditional species concepts within the taxon have a BIN concordance of approximately 90% (e.g., Fernandez-Triana et al., 2023), rendering Microgastrinae a good candidate for biodiversity assessments using both formally described species and MOTUs.
Canada’s National Capital Region (NCR) has been a historically heavily sampled region for insects, including a wide array of Microgastrinae species—leading to the NCR being called a “biodiversity hotspot” for this group by Fernandez-Triana et al. (2016). Their survey provided a checklist of Microgastrinae in this region, where they found 158 putative species from 21 genera, and calculated an estimated species diversity of 213.35 ± 16.29. A 50 km radius from the Peace Tower (45.42°N, 75.70°W) in downtown Ottawa (followed by Fernandez-Triana et al., 2016) has been the historic delimiter for assessing diversity in the city since 1895 and has been used in many species inventory studies of the area (e.g., Britton, 2015; Brodo et al., 2021). Here, we provide an updated checklist of the dark taxon Microgastrinae from Ontario’s St. Lawrence Lowlands ecoregion. The extension of the survey boundary allows us to assess diversity in an area that is defined based on regional ecological factors (Agriculture and Agri-Food Canada, 1999), rather than an arbitrarily human-defined radius. These ecoregions have been proposed to be used as proxies for differing environmental conditions that may foster distinct adaptative lineages (Forester et al., 2018). We assess species diversity using a combination of described species and MOTUs and discuss whether current DNA barcoding data are sufficient for the identification and delineation of microgastrine species in this area.
Methods
Study area
The St. Lawrence Lowlands ecoregion is a 45,157 km2 area (of which ~14,100 km2 are in Ontario) that makes up part of the larger Mixed Plains Ecozone, defined in 1996 by Agriculture and Agri-Food Canada (Ecological Stratification Working Group, 1996). Its landcover primarily comprises agriculture uses, mixed woods, developed land, grasslands, and wetlands (Natural Resources Canada, 2000; Figure 1). Land use classification data was taken from the 2020 AAFC Land Use dataset.1 Compared to other southern Canadian ecoregions, the St. Lawrence Lowlands ecoregion is among the highest ranked for biodiversity (Kraus and Hebb, 2020) and provides habitat for more than 55 species as risk, designated by COSEWIC (Nature Conservancy of Canada, 2018). It is one of the nine southern Canadian ecoregions to be identified as a “crisis ecoregion”—having high biodiversity, high risk of biodiversity loss, and low proportion of land included in protected areas (Kraus and Hebb, 2020). Within the study area, there are some sampling localities which are just outside (<2 km) the boundary of the ecoregion that are here kept for the sake of data completeness.
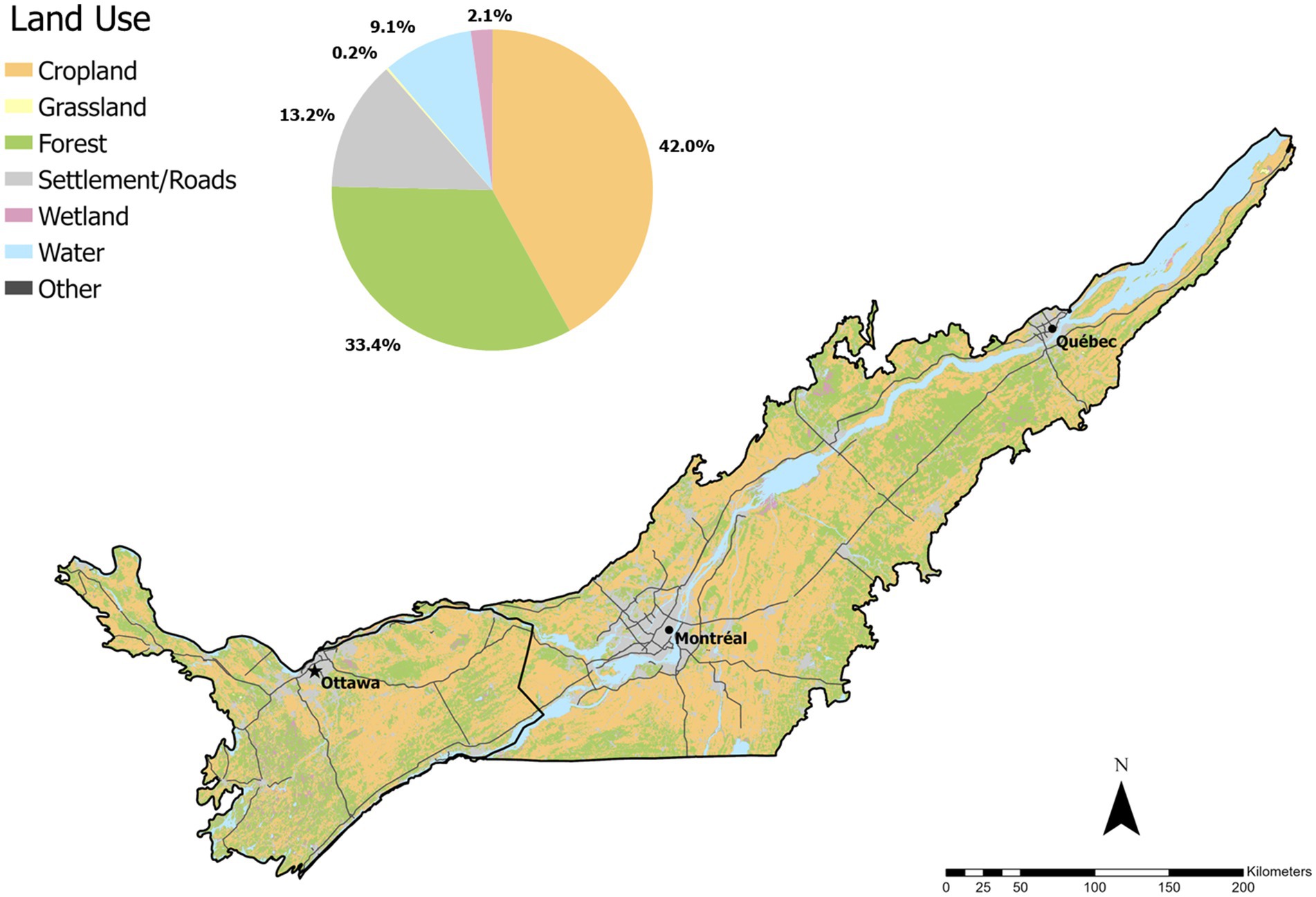
Figure 1. Map depicting land use classifications of the St. Lawrence Lowlands ecoregion (ecoregion 132; 45,157 km2).
Sampling
This study is based on a total of 3,481 specimens (Figure 2). The specimens were either stored in the Canadian National Collect of Insects (CNC) or on loan from the Center for Biodiversity Genomics (CBG), University of Guelph. Specimens were collected from 1905 to 2021 within the study region. With such a wide time series, sampling methodologies were not consistent, with both collectors and collection methods varying—from sweeping and rearing to Malaise trapping. Sampling intensity varied throughout the study region, with locations closer to the city of Ottawa being more heavily sampled (see Figure 2).
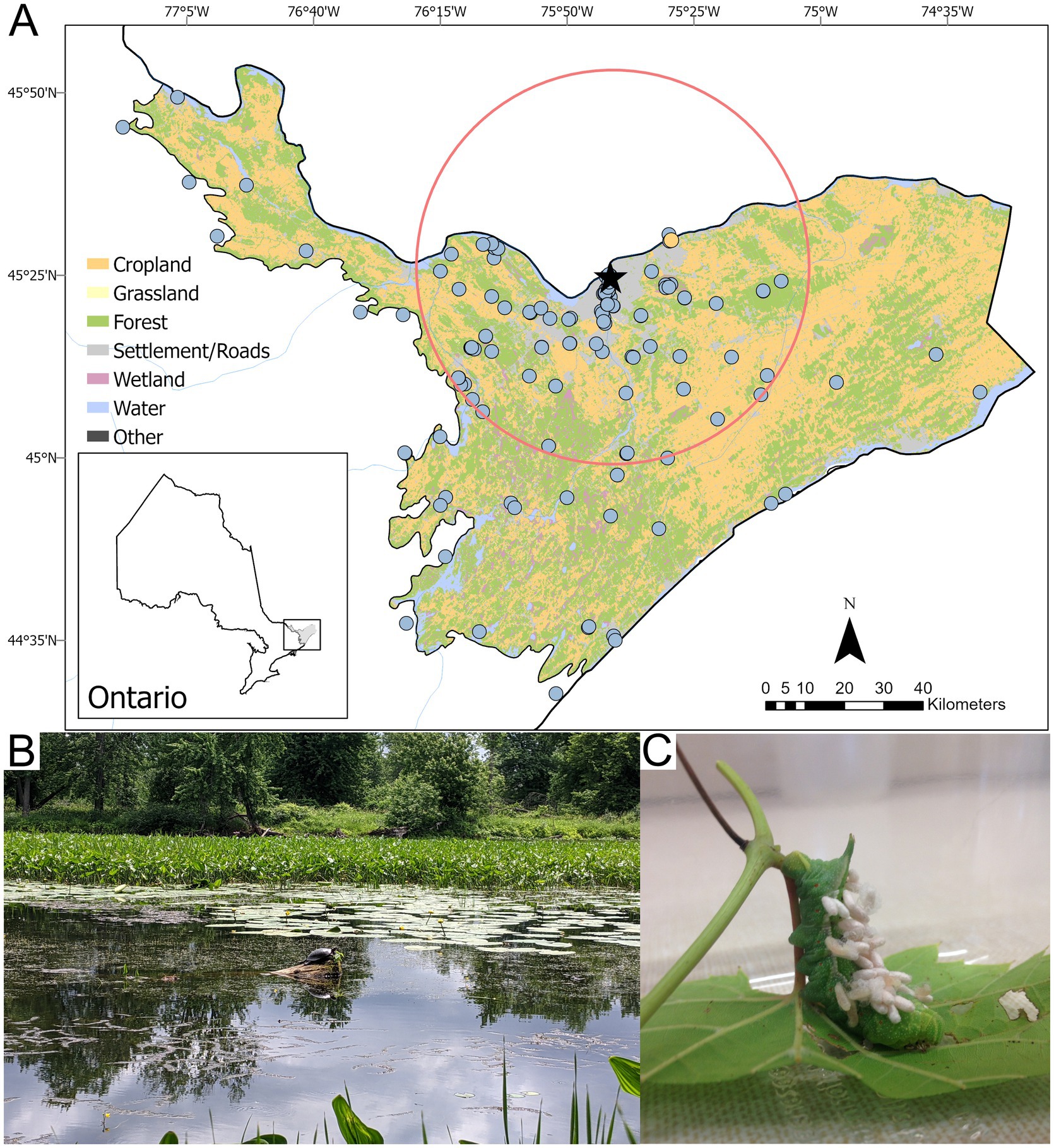
Figure 2. Collecting sites. (A) Collection localities (1905–2021) denoted by blue circles. Ontario’s portion of the St. Lawrence Ecoregion (ecoregion 132; ~14,100 km2) outlined and shaded. Pink circle denotes a 50 km radius from the Ottawa Peace Tower (star), the limit followed by Fernandez-Triana et al. (2016). (B) Petrie Island (yellow circle), a collection site area along the Ottawa river in which many unique MOTUs were collected. (C) Gregarious larvae of Cotesia sp. emerging from a live sphinx moth caterpillar collected in the study area.
Pre-existing material from the St. Lawrence Lowlands ecoregion
The data for the majority of the specimens (2,721) used for this assessment were pulled from either the CNC or BOLD databases. Less than 10% of specimens from the CNC database had associated molecular data, whereas the majority of specimens from the BOLD database (>90%) had associated DNA barcodes. The CNC representatives of duplicate specimens (with data in both databases) were removed from our records to keep any associated molecular data.
Additional sequenced material from the St. Lawrence Lowlands ecoregion
Over 2,000 unidentified Microgastrinae specimens from the study region were morphologically sorted to genus in 2022, following the generic descriptions outlined in Fernandez-Triana et al. (2020). Specimens within each genus were sorted to morphospecies—morphologically similar specimens that may represent a single species. A subsample of representatives from each morphospecies, totalling 760 specimens representing over 100 morphospecies, had one mid-leg removed with tweezers under a microscope and stored in 90+% ethanol. These tissue samples were sent to the Center for Biodiversity Genomics for COI-5P sequencing,2 and results were made available and analyzed in the BOLD database.3
Species identification
Specimens without molecular data (all housed in the CNC) were previously identified to species by taxonomic experts. Many of these identifications were confirmed by Jose Fernandez-Triana and had been used by Fernandez-Triana et al. (2016) for their biodiversity assessment of the Ottawa area. Specimens with associated molecular data fell into two groups: material that was sorted morphologically before sequencing (760 specimens, see above), and sequenced material with public molecular data from the BOLD database (1,485). Sequences and morphology of specimens who fell within a BIN containing a formally described species were verified. The identifications of well-supported specimens were updated to the described species. Many BINs did not have an association with a formally described species. Because this is a hyper-diverse and poorly known group, for some cases it was not possible to establish with certainty whether a BIN corresponds to a new or described species due to time-constraints and lack of adequate identification keys. In these cases, each BIN was given an interim name for (i.e., Glyptapanteles jft14, Choeras mb01) which acts as a placeholder until the MOTU is formally described or associated with an already existing species. Records from the BOLD database for which specimens were not available for morphological assessment had their identifications taken at face value.
Data analysis
Using the maximum species and generic limits, abundance-frequency counts were calculated using the Chao1 method (Chao and Chiu, 2012), and from this, species richness estimates were generated. Continuous diversity profiles were generated using Hills numbers—a set of diversity indices—that indicate effective species numbers over parameter q, where q0 = species richness, q1 = Shannon’s index, and q2 = Simpson index. This parameter dictates how sensitive the effective species number is to a species’ relative abundance (Chao et al., 2014; Jost, 2019). Diversity profiles depict the evenness of a community: a diversity profile for a community in which all species are represented by the same number of specimens would have the same effective species number over q, i.e., a slope of 0, whereas a community containing some species with many representatives and others with few, will show a steep decline over q. Calculations were performed in R (v. 4.2.2) using the SpadeR package.
BIN assessment
We compared BINs to currently accepted species concepts to gain insight on their congruence. A BIN’s relationship to traditional taxonomic identification was categorized following Ratnasingham and Hebert (2013): a BIN can represent a match, split, merge, or a mixture when compared to species concepts. When traditional taxonomy and BINs align, they are considered to be concordant and a “match.” “Splits” arise when a single species is linked to multiple BINs. “Merges” occur when a single BIN is associated with two or more species. “Mixtures” are a mix of the former two categories: they occur when two BINs are associated with two species, yet sequences from at least one species fall within both BINs. We investigated BIN categorization in respect to their maximum within-BIN distances and the distance to their nearest neighbor (nearest BIN). A ratio of nearest neighbor p-distance to maximum within-BIN p-distances distance (p-distance to nearest neighbor:maximum within-BIN p-distance) has been used as a method for screening molecular clusters and identifying BIN discrepancies (Gibbs, 2018; Höcherl et al., 2024).
Results
Species
A total of 3,481 specimens from the St. Lawrence Lowlands ecoregion were analyzed for this study. The specimens were collected between 1905 and 2021, with the majority of the specimens (64%) collected since 2001.
In our dataset, a total of 106 described species from 23 genera and 267 BINs were found within the study area (Supplementary material). Combining described species with MOTUs that have no associated species name yielded a maximum putative species count of 304 (Figure 3A). This is undoubtedly an artificially inflated number, as it is likely that many species have been represented twice—once as a formally described species with no molecular data and again as a MOTU with an interim name. Accounting for this fact, a minimum putative species number of 228 was obtained by assuming all described species without molecular data would eventually be associated with BINs present in the dataset (Figure 3B). This is, conversely, an artificially deflated number, as it is unlikely that all described species from the area were molecularly characterized in the dataset.
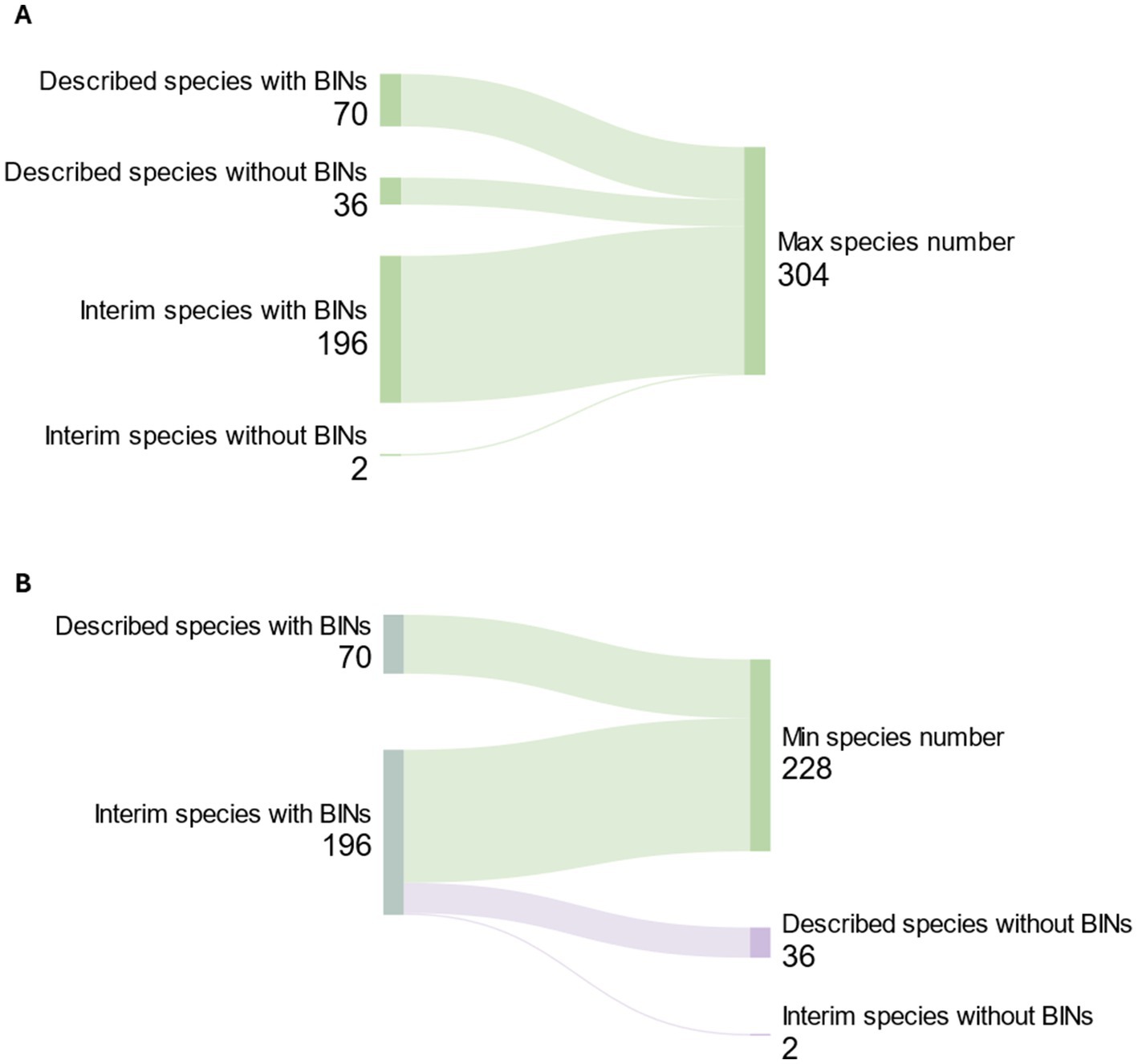
Figure 3. (A) Visualization of the categories contributing to the maximum number of species present from our dataset. (B) Visualization of the categories contributing to the minimum number of species present from our dataset.
Seventy of the 106 described (=nominal) species (66%) have BIN associations. This almost doubles the number of nominal species that were previously referenced with DNA barcodes for the Ottawa region. New species records for the Nearctic are Apanteles minornavarroi Fernandez-Triana, 2014 and Protapanteles anchisiades (Nixon, 1973). New records to Canada are Promicrogaster virginiana Fernandez-Triana, 2019 and Protapanteles immunis (Haliday, 1834). Additionally, new records to the province of Ontario are Cotesia plathypenae (Muesebeck, 1921) and Alphomelon winniewertzae Deans, 2003. Of the described species, 18% are preliminarily designated as non-native. As for the species with interim names, it is impossible to conclude, but most would presumably be native, although some may represent European species still not identified in North America.
The most diverse genera were Cotesia (51), Apanteles (43), Microplitis (39), Dolichogenidea (23), Choeras (26), Glyptapanteles (26), and Microgaster (20 putative species). Choeras and Glyptapanteles showed the biggest increase in diversity compared to the data from Fernandez-Triana et al. (2016), with a 520 and 873% increase, respectively. Lathrapanteles is the only genus for which we report less generic diversity, with one instead of two species in the area. This is due to the erroneous report of Lathrapanteles papaipemae within the 2016 study—the specimen in question in fell outside of their study region. The most abundant species and interim names were Glyptapanteles militaris (363 specimens), Microplitis varicolor (110), Glyptapanteles pallipes (109), Dolichogenidea mb01 (97), Pholetesor ornigis (96), Dolichogenidea ensiger (95), Hypomicrogaster zonaria (80), Apanteles nephoptericis (79), Cotesia atalantae (79), Lathrapanteles heleios (78), and Cotesia jft02 (71).
Estimation of taxon diversity
In our dataset of 2,173 successfully sequenced specimens associated to 267 BINs, 92 BINs are represented only by a single sequence (34%, “singletons”) and 51 by only two sequences (19%, “doubletons”). This marks more than half of the BINs in our dataset as “rare.” Chao1 diversity estimates generate an expected BIN/putative species richness of 391 (95% CI [355, 453]), meaning that currently an estimated 79% of the species are represented in the checklist and characterized taxonomically or via molecular characterization. Continuous diversity profiles were generated for the subfamily as a whole (Figure 4) as well as the six most speciose genera (Figure 5), with Hill numbers representing effective number of species over parameter q—a measure of sensitivity to relative abundance (q0 = species richness, q1 = Shannon’s index, q2 = Simpson’s index). The steep decline and shallow confidence intervals of Glyptapanteles and Dolichogenidea are indicative of their lack of evenness—both genera contain BINs (putative species) with many collected specimens as well as many BINs (putative species) represented by singletons and doubletons.
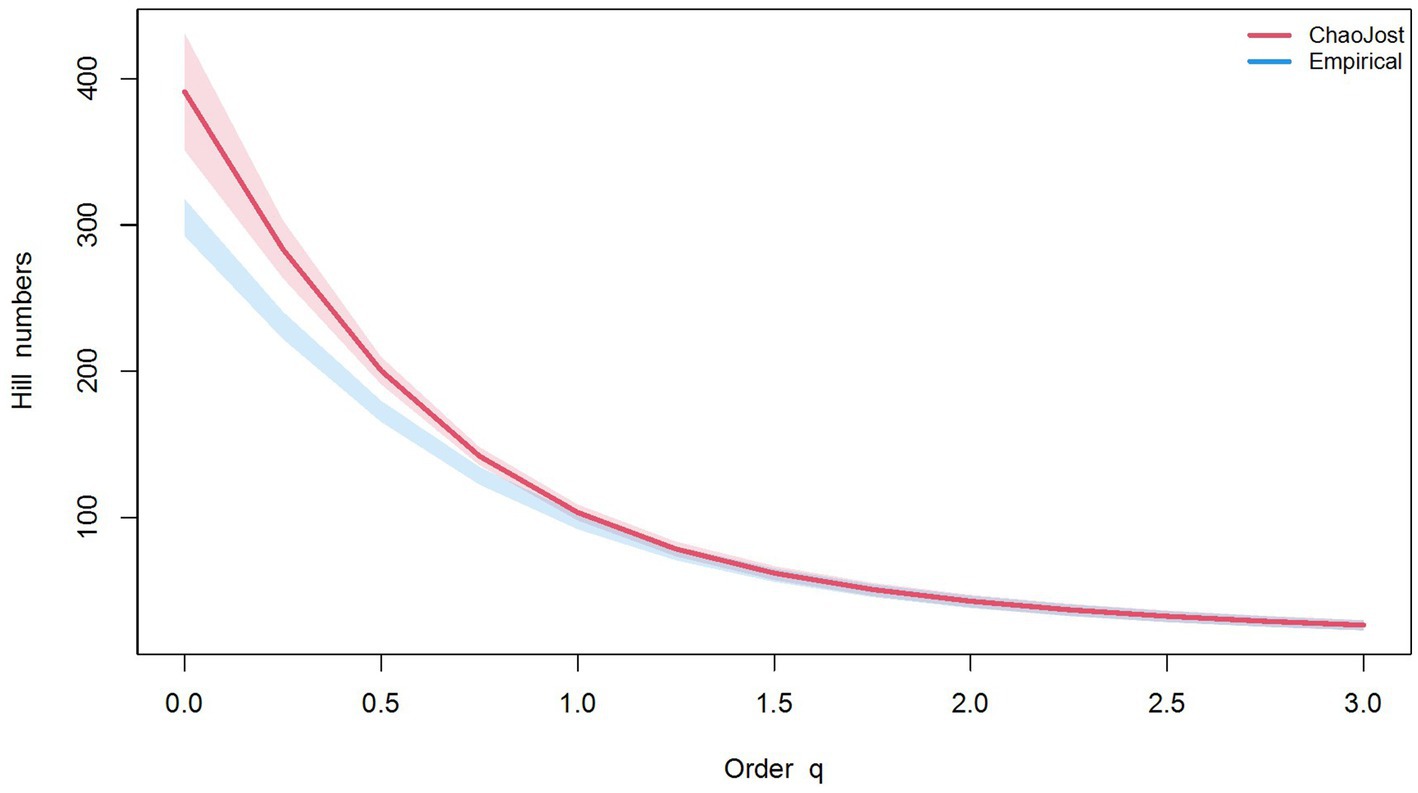
Figure 4. Continuous diversity profiles for all Microgastrinae collected in the St. Lawrence Lowlands ecoregion. The red line represents the ChaoJost estimated diversity profiles while the dotted blue line represents the empirical upper species numbers. Shaded areas represent 95% confidence intervals (number of permutations = 100). Diversity measured through Hill numbers by q0 = species richness, q1 = Shannon’s index, q2 = Simpson’s index.
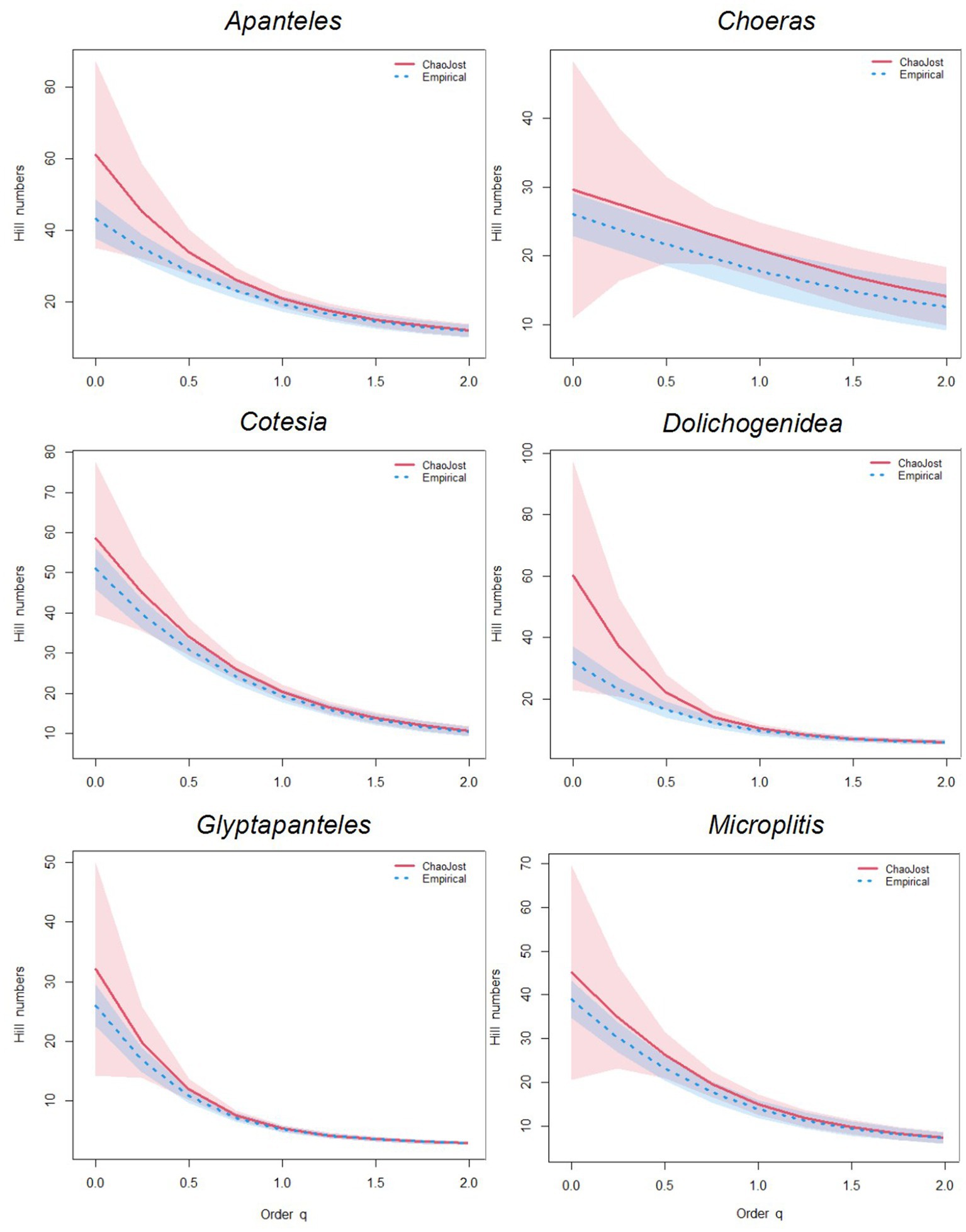
Figure 5. Continuous diversity profiles for the six most diverse genera in the St. Lawrence Lowlands ecoregion. The red line represents the ChaoJost estimated diversity profiles while the dotted blue line represents the empirical upper species numbers. Shaded areas represent 95% confidence intervals (number of permutations = 100). Diversity measured through Hill numbers by q0 = species richness, q1 = Shannon’s index, q2 = Simpson’s index.
Based on these data, we uncovered a pattern of generic diversity that is rather unique in the entire Holarctic region (when compared to studies for North America summarized in Fernandez-Triana et al. (2011) and Fernandez-Triana et al. (2016), and some studies for Europe such as Broad et al., 2016 and Höcherl et al., 2024). The prevalence of Cotesia as the most speciose genus is a common finding in biodiversity inventories for temperate regions, as is the high diversity of Apanteles and Dolichogenidea (usually with the former more diverse in the Nearctic and the latter more diverse in the Palaearctic). The increased diversity of Glyptapanteles, when compared with the results reported in 2016, is significant but was expected to occur with a larger survey area, and due to the species richness and morphological crypsis of this genus. However, the number of putative Microplitis species is above what was expected, and the most unusual case is Choeras. Until now, only six species of Choeras were known from North America (Fernandez-Triana et al., 2020), therefore, the finding of 26 putative species in this genus within the St. Lawrence Lowlands ecoregion is extraordinary and highlights a need for further taxonomic investigation.
Assessing the use of BINs as proxies for species
The 2,173 sequences obtained represent 267 BINs. Of these, 47 are concordant with formally described species (Table 1) and 38 split described species (Table 2). Six of the latter also merge two or more species and are therefore considered mixtures (Table 3). The remaining 182 BINs are designated as MOTUs (putative species) until they can be formally and properly associated with a species name.
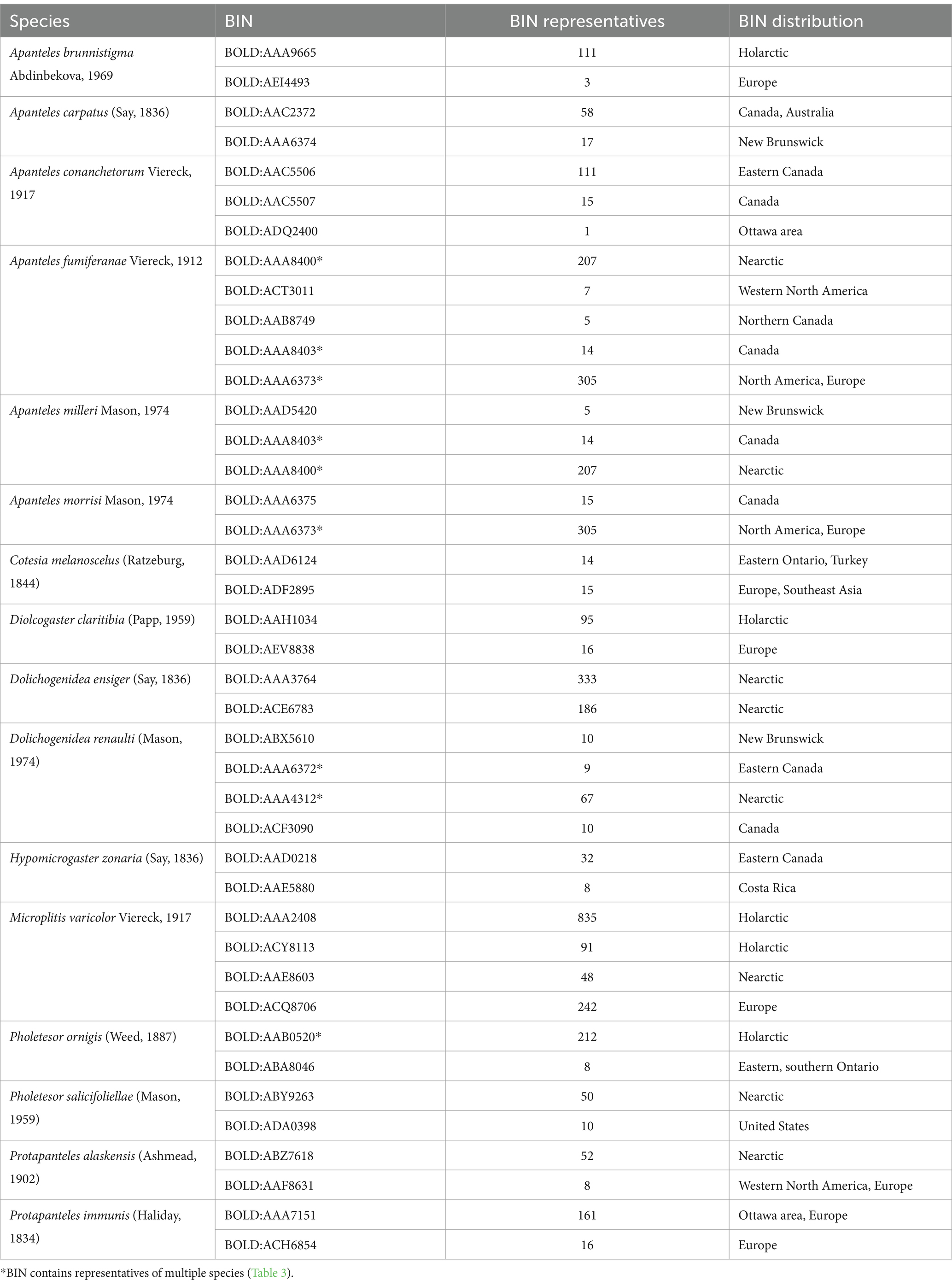
Table 2. Microgastrinae species represented by multiple BINs (“splits”), retrieved on May 24th, 2024.
Of the 106 described species in the study region, 70 have BIN associations. Fifty-four species are associated with a single BIN. Seven of these BINs are also associated with at least one other species (Figure 6; Table 3) and are therefore not successful in species discrimination. The remaining 16 species are associated with multiple BINs. For 11 of these species, their BINs are associated solely with the species in question and no other (Figure 6). This still allows for identification through BINs (rendering them “molecularly identifiable”) and hints at the possibility of morphologically cryptic species complexes [as has often been discussed for several of these species, e.g., Microplitis varicolor (Fernandez-Triana et al., 2011)]. Overall, this results in 58 of the 70 species (83%) being deemed as “molecularly identifiable” through DNA barcoding.
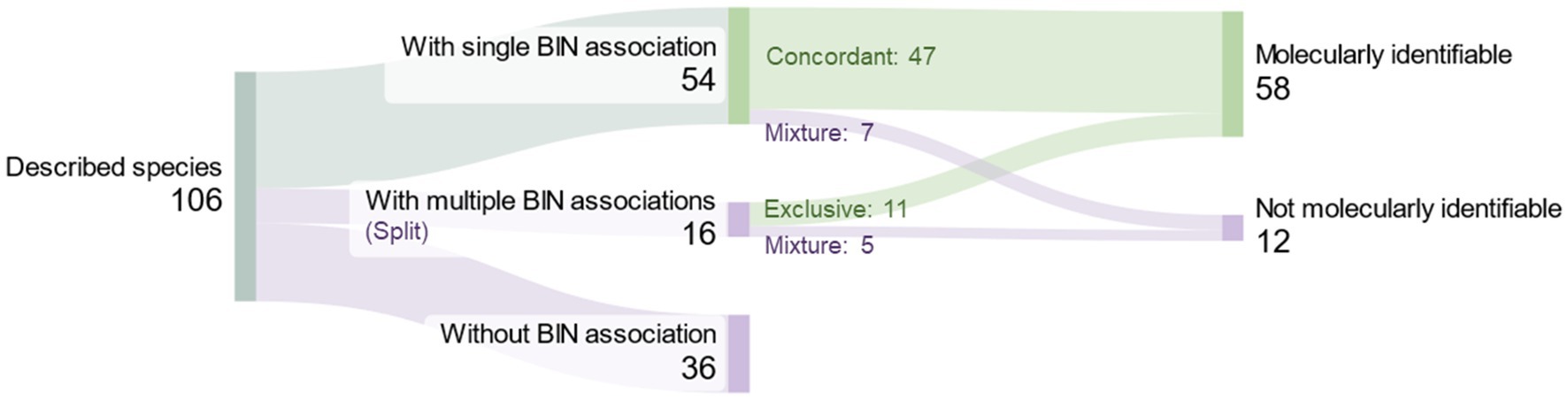
Figure 6. Visualization of the number of described species with molecular data. Molecularly identifiable species are considered species for which a BIN association is sufficient for species identification.
No BIN with a p-distance to its nearest neighbor greater than 2.5% was considered a “mixture” (Figure 7), meaning no BIN with a distance to its neighbor greater than that threshold supports a lumping of two or more species. Investigating the ratio of a BIN’s distance to its nearest neighbor to its maximum within-BIN distance (p-distance to nearest neighbor:maximum within-BIN p-distance) revealed 42 BINs that fell above a ratio of 2 (meaning the distance to the nearest neighbor is more than double the maximum distance within the BIN). Of these, 27 are matches, 14 are splits, and one is a mixture. Twenty-one BINs fell below a ratio of 1. Of these, 10 are matches, eight are splits, and three are mixtures.
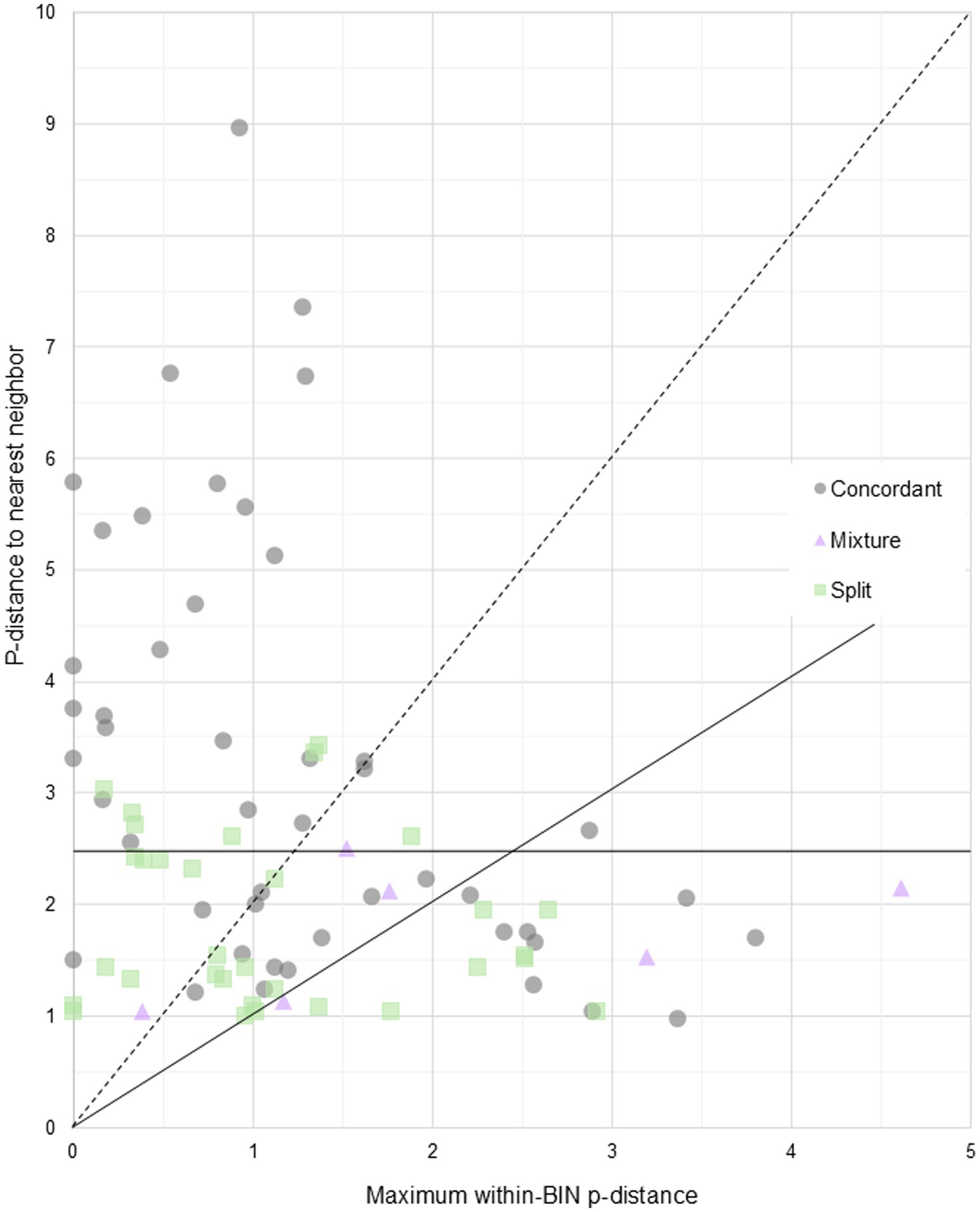
Figure 7. Summary of the maximum within-BIN p-distances and p-distance to the nearest neighbor of the 84 bins representing 70 described species. Horizontal solid line indicates the distance to nearest neighbor threshold (2.5%) in which no BIN merges occur above. Diagonal solid line indicates a inter-BIN distance:intra-BIN distance of 1; diagonal dashed line indicates a ratio of 2. Shape and color represent the various BIN categories in relation to traditional taxonomic identification.
Discussion
Is the Ottawa area a biodiversity hotspot for Microgastrinae wasps?
Based on current data and in comparison to other regions in North America, Microgastrinae are extremely diverse in the St. Lawrence Lowlands ecoregion. The current putative species counts of 228–304 greatly increase the previous count of 158 species and surpass the estimated diversity of a thorough study published in 2016, only nine years ago. But is the region really a biodiversity hotspot for Microgastrinae wasps? Firstly, the ecoregion provides a variety of habitats—from mixed woods to wetlands to alvars (an environment with limestone bedrock and thin soil)—which may contribute to the astonishing diversity demonstrated here and provide habitats for many species which reach their northernmost limit (Fernandez-Triana, 2014). Many of the localities sampled within the ecoregion harbor unique ecosystems, such as Sphagnum bogs and wetlands that provide a habitat for species not yet found elsewhere in the study region or globally. Although it is expected that certain species will be restricted to specific habitats or ecosystems where their hosts (and in turn, their hosts’ food plant) can be found, the impressive diversity found in the ecoregion is no doubt due in part to a disproportionate amount of sampling occurring in these localities (as demonstrated by the large number of species solely found in a city garden, a rather undistinguished habitat). The CNC, located in Ottawa, is one of the five largest insect collections of its kind in the world,4 which has led to a sampling bias for the area. Moreover, CNC entomologists over the decades (e.g., William Mason and Jose Fernandez-Triana) have focused their studies on this group, collecting and identifying microgastrines specifically.
Estimations of species richness in Microgastrinae have also been conducted by comparing the number of Lepidoptera and Microgastrinae species in a particular region. Fernandez-Triana (2010), Rodriguez et al. (2013), and Fernandez-Triana et al. (2020) estimated Microgastrinae diversity by considering the ratio of lepidopteran to microgastrine species in an area, with an average ratio of approximately 10 lepidopteran species for every microgastrine species. Given this ratio, it is not expected that the St. Lawrence Lowlands ecoregion would be the most diverse area for Microgastrinae in North America, as many areas in the continent harbor higher species diversity for Lepidoptera. For example, the highest diversity of butterflies has been mostly found in western and southern areas of North America (e.g., D’Ercole et al., 2024), and the highest numbers of Noctuoidea moths are found between 30 and 40° N (Zahiri et al., 2017). Therefore, it is expected that some of those areas are more likely to include hotspots for Microgastrinae diversity in North America.
The St. Lawrence Lowlands ecoregion may currently seem a hotspot for microgastrine wasps according to the literature and data available, but this is likely owing to the fact that other areas in North America are massively understudied for this dark taxon. Rather than a biodiversity hotspot, the ecoregion seems to represent a research hotspot for Microgastrinae wasps.
Comparisons to data from previous work (2016)
There are limitations in the comparisons made between the results of this study and those of Fernandez-Triana et al. (2016). Issues with direct comparisons arise due to differences in study areas (~14,100 km2 vs. ~7,800 km2). Despite the substantial increase in area in this study, only 353 out of the 3,481 (~10%) specimens with coordinate data fell outside of the 50 km radius from Ottawa’s Peace Tower. Furthermore, specimens that were collected in Quebec (in particular, the well-sampled Gatineau Park) were included in the 2016 study and are omitted here. Discrepancies between the studies are due to the increase of collection material availability and the increased accessibility of DNA barcoding over the decades. Fernandez-Triana et al. (2016) included specimens collected until 2010 (a total of 1,928 specimens), whereas we studied specimens collected until 2021 (3,481 specimens). Moreover, this study also has the advantage of having access to 2,245 specimens with COI sequence data, whereas Fernandez-Triana et al. (2016) had fewer sequence data available. Although direct comparisons between the studies are difficult, it is apparent that DNA barcoding has revealed an astonishing diversity in a historically well-researched, well-sampled area.
Using BINs as species proxies
Some major shortcomings are associated with the implementation of MOTUs as species proxies. Although BINs have been shown to have a high concordance with described Microgastrinae species, there is a risk of presenting an artificially inflated species number. This would occur if the algorithm used by BOLD is too sensitive and separates a genetically variable species into more than one BIN. Artificially inflated species numbers could also be presented in this study if a species is represented twice—once as a formally described species that has no molecular data associated with it and again as a MOTU. Thus, it is important to note that although many of the species listed here are given interim names, they do not necessarily represent undescribed species. They may simply be MOTUs that have not had their molecular data associated with a described species. This is a major challenge when working with dark taxa and it requires work from individuals with extensive knowledge of the taxon to make those associations. Luckily, due to extensive research in the region, most formally described species in the St. Lawrence Lowlands ecoregion either have associated molecular data or are morphologically distinct. Even in the case that each MOTU is already represented by a formally described species, the lower limit of 228 species is already a substantial (44%) increase from 2016. Conversely, another concern with this approach is an underrepresentation of species, which can occur if the BIN algorithm is too conservative, if separate species have experienced incomplete lineage sorting (Weber et al., 2019), or if species are lost in the minimum count because they have been subtracted to account for the number of species not yet linked to DNA barcodes.
Given that species discrimination based on BINs was successful in 58 out of 70 (83%) of species with molecular data, using BINs as a proxy for microgastrine species in this area can provide valuable information on expected diversity, but has its limitations. Other methods for assessing species identification using COI barcodes, such as general mixed Yule-coalescent models (Monaghan et al., 2005) or Poisson-tree-process models (Zhang et al., 2013), have been preferred, especially for taxa which often exhibit a greater intra-over inter-genetic divergence, such as bumblebees (see Williams et al., 2020, for example). The species diversity estimates presented here should be taken as a rough estimate that highlight the need for increased taxonomic effort in the area. Indeed, the expected diversity calculated for the ecoregion using BINs as species proxies is much higher than previously estimated.
Investigations in BIN categorization with respect to their inter-BIN distances compared to their intra-BIN distances (p-distance to nearest neighbor:maximum within-BIN p-distance) revealed that BIN congruence largely follows this ratio. The larger the quotient, the more likely a BIN is to be congruent with current species concept. Not only has DNA barcoding allowed for a clearer picture of taxonomic diversity, but also for the re-assessment of current taxonomic concepts. Many BIN “matches” fell below an inter-BIN distance to intra-BIN distance value of 1. These species may represent examples of genetic introgression or incomplete lineage sorting (Rheindt et al., 2009). Conversely, many BIN “splits” fell above an inter-BIN distance to intra-BIN distance value of 2. These BINs may represent examples of cryptic diversity and highlight taxa that require further investigation in their taxonomic placement.
Biodiversity
Microgastrinae are highly diverse in the St. Lawrence Lowlands ecoregion. By land cover, the ecoregion contains 42% annual and perennial crop land (Natural Resources Canada, 2000; Nature Conservancy of Canada, 2018). From 2000 to 2010, land conversion led to the expansion of agricultural areas at the loss of forests and wetlands (Nature Conservancy of Canada, 2018). Suitable microhabitats within agricultural landscapes have been shown to be necessary for the maintenance of specialist insect species (Habel et al., 2018). Additionally, habitat transition zones adjacent to cropland, such as hedgerows, riparian zones, ditch banks, and wooded areas, are crucial to maintaining butterfly populations (Habel et al., 2022). In conjunction with land use alterations, pesticide use has also resulted in a decline in insect biomass (Brühl et al., 2021), yet many field edge habitats, despite a community shift compared to more natural habitats, can also be productive from a biomass and insect diversity perspective (Rideout et al., 2024). With Microgastrinae parasitizing exclusively Lepidoptera larvae, they are not only economically important as natural enemies of commercial agriculture and forestry pests (Whitfield, 1997), but are especially susceptible to stochastic changes in population dynamics given their high trophic level (Shaw and Hochberg, 2001).
Within the current Anthropocene context, it is imperative that the biodiversity of such an important taxon continues to be cataloged. This is crucial to enable monitoring and obtain a thorough understanding of how anthropogenic activities may be affecting these parasitoid wasps, and by extension, the ecosystem services they provide. The St. Lawrence Lowlands ecoregion has high biodiversity compared to other southern ecoregions in Canada (Kraus and Hebb, 2020) and is a research hotspot for Microgastrinae wasps. This, combined with the ongoing changes in land use in this region, makes the area a potential model region (“environmental observatory”) for using molecular techniques and integrative taxonomy to gain a better insight into the potential anthropogenically driven biodiversity loss of these parasitoid wasps. Such information will help identify salient conservation practices and management techniques to reduce habitat loss. The threat of losing undescribed species—of which there are many, even in supposedly well-studied areas—is of great concern, and this study highlights the urgency to act.
Data availability statement
The datasets presented in this study can be found in online repositories. The names of the repository/repositories and accession number(s) can be found in the article/Supplementary material.
Ethics statement
The manuscript presents research on animals that do not require ethical approval for their study.
Author contributions
MB: Conceptualization, Data curation, Formal analysis, Investigation, Methodology, Visualization, Writing – original draft, Writing – review & editing, Project administration, Software. AH: Conceptualization, Data curation, Formal analysis, Methodology, Supervision, Validation, Visualization, Writing – original draft, Writing – review & editing. CB: Data curation, Methodology, Writing – original draft, Writing – review & editing. CC: Visualization, Writing – original draft, Writing – review & editing. DL: Funding acquisition, Writing – original draft, Writing – review & editing. JF-T: Conceptualization, Data curation, Formal analysis, Funding acquisition, Investigation, Methodology, Project administration, Resources, Supervision, Visualization, Writing – original draft, Writing – review & editing.
Funding
The author(s) declare that financial support was received for the research and/or publication of this article. Research support for this work was provided, in part, by AAFC Projects J-002305 (Environmental Change One Health Observatory ECO2) and AAFC Project J-002276 (Systematics of beneficial arthropods in support of resilient agroecosystems).
Acknowledgments
We are grateful to the thorough comments given by three reviewers—they have considerably improved the final version of this paper.
Conflict of interest
The authors declare that the research was conducted in the absence of any commercial or financial relationships that could be construed as a potential conflict of interest.
Publisher’s note
All claims expressed in this article are solely those of the authors and do not necessarily represent those of their affiliated organizations, or those of the publisher, the editors and the reviewers. Any product that may be evaluated in this article, or claim that may be made by its manufacturer, is not guaranteed or endorsed by the publisher.
Supplementary material
The Supplementary material for this article can be found online at: https://www.frontiersin.org/articles/10.3389/fsufs.2025.1457055/full#supplementary-material
Footnotes
1. ^https://open.canada.ca/data/en/dataset/7a098ea9-cc31-4d79-b326-89f6cd1fbb7d
3. ^http://www.boldsystems.org
4. ^https://agriculture.canada.ca/en/science/collections/canadian-national-collection-insects-arachnids-and-nematodes
References
Agriculture and Agri-Food Canada (1999). A National Ecological Framework for Canada: Ecoregions. Available online at: http://sis.agr.gc.ca/cansis/nsdb/ecostrat/index.html (Accessed April 4, 2023).
Britton, D. (2015). Annotated checklist of birds of the Ottawa Gatineau District, Ottawa field-Naturalists' Club. Available online at: https://ofnc.ca/wp-content/uploads/2022/02/Ottawa-Annotated-Checklist-of-all-Birds-in-the-Ottawa-Gatineau-District-2021.pdf (Accessed April 5, 2023).
Broad, G. R., Shaw, M. R., and Godfray, H. C. J. (2016). Checklist of British and Irish Hymenoptera-Braconidae. Biodiversity Data J. 4:e8151. doi: 10.3897/BDJ.4.e8151
Brodo, I., Lee, R., Freebury, C., Wong, P. Y., Lewis, C., and McMullin, T. (2021). Additions to the lichens, allied fungi, and lichenicolous fungi of the Ottawa region in Ontario and Quebec, with reflections on a changing biota. Can. Field-Naturalist 135, 1–27. doi: 10.22621/cfn.v135i1.2557
Brühl, C. A., Bakanov, N., Köthe, S., Eichler, L., Sorg, M., Hörren, T., et al. (2021). Direct pesticide exposure of insects in nature conservation areas in Germany. Sci. Rep. 11:24144. doi: 10.1038/s41598-021-03366-w
Chao, A., and Chiu, C. H. (2012). “Estimation of species richness and shared species richness” in Methods and Applications of Statistics in the Atmospheric and Earth Sciences, 76–111.
Chao, A., Chiu, C. H., and Jost, L. (2014). Unifying species diversity, phylogenetic diversity, functional diversity, and related similarity and differentiation measures through hill numbers. Annu. Rev. Ecol. Evol. Syst. 45, 297–324. doi: 10.1146/annurev-ecolsys-120213-091540
Chimeno, C., Hausmann, A., Schmidt, S., Raupach, M. J., Doczkal, D., Baranov, V., et al. (2022). Peering into the darkness: DNA barcoding reveals surprisingly high diversity of unknown species of Diptera (Insecta) in Germany. Insects 13:82. doi: 10.3390/insects13010082
D’Ercole, J., Dapporto, L., Opler, P., Schmidt, C. B., Ho, C., Menchetti, M., et al. (2024). A genetic atlas for the butterflies of continental Canada and United States. PLoS One 19:e0300811. doi: 10.1371/journal.pone.0300811
Didham, R. K., Basset, Y., Collins, C. M., Leather, S. R., Littlewood, N. A., Menz, M. H., et al. (2020). Interpreting insect declines: seven challenges and a way forward. Insect Conserv. Divers. 13, 103–114. doi: 10.1111/icad.12408
Ecological Stratification Working Group (1996). A National Ecological Framework for Canada. Ottawa, Gatineau: Agriculture and Agri-Food Canada, Research Branch, Centre for Land and Biological Resources Research and Environment Canada, State of the Environment Directorate, Ecozone Analysis Branch.
Fernandez-Triana, J. L. (2010). Eight new species and an annotated checklist of Microgastrinae (Hymenoptera, Braconidae) from Canada and Alaska. Zoo Keys 63, 1–53. doi: 10.3897/zookeys.63.565
Fernandez-Triana, J., Smith, M. A., Boudreault, C., Goulet, H., Hebert, P. D., Smith, A. C., et al. (2011). A poorly known high-latitude parasitoid wasp community: unexpected diversity and dramatic changes through time. PLoS One, 6: e23719. doi: 10.1371/journal.pone.0023719
Fernandez-Triana, J. (2014). Towards the conservation of parasitoid wasp species in Canada: preliminary assessment of Microgastrinae (Hymenoptera: Braconidae). Biodivers. Data J. 2:e1067. doi: 10.3897/BDJ.2.e1067
Fernandez-Triana, J., Boudreault, C., Buffam, J., and Mclean, R. (2016). A biodiversity hotspot for Microgastrinae (Hymenoptera, Braconidae) in North America: annotated species checklist for Ottawa, Canada. Zoo Keys 633, 1–93. doi: 10.3897/zookeys.633.10480
Fernandez-Triana, J., Shaw, M. R., Boudreault, C., Beaudin, M., and Broad, G. R. (2020). Annotated and illustrated world checklist of Microgastrinae parasitoid wasps (Hymenoptera, Braconidae). Zoo Keys 920, 1–1089. doi: 10.3897/zookeys.920.39128
Fernandez-Triana, J. L., Shimbori, E. M., Whitfield, J. B., Penteado-Dias, A. M., Shaw, S. R., Boudreault, C., et al. (2023). a revision of the parasitoid wasp genus Alphomelon Mason with the description of 30 new species (Hymenoptera, Braconidae). Zoo Keys 1175, 5–162. doi: 10.3897/zookeys.1175.105068
Forester, B. R., Lasky, J. R., Wagner, H. H., and Urban, D. L. (2018). Comparing methods for detecting multilocus adaptation with multivariate genotype–environment associations. Mol. Ecol. 27, 2215–2233. doi: 10.1111/mec.14584
Forister, M. L., Pelton, E. M., and Black, S. H. (2019). Declines in insect abundance and diversity: we know enough to act now. Conservat. Sci. Pract. 1:80. doi: 10.1111/csp2.80
Gibbs, J. (2018). DNA barcoding a nightmare taxon: assessing barcode index numbers and barcode gaps for sweat bees. Genome 61, 21–31. doi: 10.1139/gen-2017-0096
Habel, J. C., Angerer, V., Gros, P., Teucher, M., and Eberle, J. (2022). The relevance of transition habitats for butterfly conservation. Biodivers. Conserv. 31, 1577–1590. doi: 10.1007/s10531-022-02411-y
Habel, J. C., Teucher, M., and Rödder, D. (2018). Mark-release-recapture meets species distribution models: identifying micro-habitats of grassland butterflies in agricultural landscapes. PLoS One 13:e0207052. doi: 10.1371/journal.pone.0207052
Hartop, E., Srivathsan, A., Ronquist, F., and Meier, R. (2022). Towards large-scale integrative taxonomy (LIT): resolving the data conundrum for dark taxa. Syst. Biol. 71, 1404–1422. doi: 10.1093/sysbio/syac033
Hausmann, A., Krogmann, L., Peters, R. S., Rduch, V., and Schmidt, S. (2020). GBOL III: dark taxa: researchers launch new BIOSCAN project that aims to illuminate thousands of new insect species on Germany's doorstep. iBOL Barcode Bull. 10, 1–4. doi: 10.21083/ibol.v10i1.6242
Hebert, P. D., Bock, D. G., and Prosser, S. W. (2023). Interrogating 1000 insect genomes for NUMTs: a risk assessment for estimates of species richness. PLoS One 18:e0286620. doi: 10.1371/journal.pone.0286620
Hebert, P. D., Cywinska, A., Ball, S. L., and DeWaard, J. R. (2003). Biological identifications through DNA barcodes. Proc. R. Soc. Lond. Ser. B Biol. Sci. 270, 313–321. doi: 10.1098/rspb.2002.2218
Höcherl, A., Shaw, M. R., Boudreault, C., Rabl, D., Haszprunar, G., Raupach, M. J., et al. (2024). scratching the tip of the iceberg: integrative taxonomy reveals 30 new species records of Microgastrinae (Braconidae) parasitoid wasps for Germany, including new Holarctic distributions. Zoo Keys 1188, 305–386. doi: 10.3897/zookeys.1188.112516
Huemer, P., Karsholt, O., Aarvik, L., Berggren, K., Bidzilya, O., Junnilainen, J., et al. (2020). DNA barcode library for European Gelechiidae (Lepidoptera) suggests greatly underestimated species diversity. Zoo Keys 921, 141–157. doi: 10.3897/zookeys.921.49199
Jost, L. (2019). What do we mean by diversity?: the path towards quantification. Mètode Sci. Stud. J. 9, 55–61. doi: 10.7203/metode.9.11472
Kraus, D., and Hebb, A. (2020). Southern Canada’s crisis ecoregions: identifying the most significant and threatened places for biodiversity conservation. Biodivers. Conserv. 29, 3573–3590. doi: 10.1007/s10531-020-02038-x
Monaghan, M. T., Balke, M., Gregory, T. R., and Vogler, A. P. (2005). DNA-based species delineation in tropical beetles using mitochondrial and nuclear markers. Philos. Trans. R. Soc. B Biol. Sci. 360, 1925–1933. doi: 10.1098/rstb.2005.1724
Natural Resources Canada (2000). Canadian land cover, circa 2000 (vector) - geobase series, 1996–2005, open government portal. Available online at: https://open.canada.ca/data/en/dataset/97126362-5a85-4fe0-9dc2-915464cfdbb7(Accessed April 4, 2023).
Page, R. D. (2016). DNA barcoding and taxonomy: dark taxa and dark texts. Philos. Trans. R. Soc. B Biol. Sci. 371:20150334. doi: 10.1098/rstb.2015.0334
Ratnasingham, S., and Hebert, P. D. (2007). BOLD: the barcode of life data system (http://www.barcodinglife.org). Mol. Ecol. Notes 7:355. doi: 10.1111/j.1471-8286.2007.01678.x
Ratnasingham, S., and Hebert, P. D. (2013). A DNA-based registry for all animal species: the barcode index number (BIN) system. PLoS One 8:e66213. doi: 10.1371/journal.pone.0066213
Rheindt, F. E., Christidis, L., and Norman, J. A. (2009). Genetic introgression, incomplete lineage sorting and faulty taxonomy create multiple cases of polyphyly in a montane clade of tyrant-flycatchers (Elaenia, Tyrannidae). Zool. Scr. 38, 143–153. doi: 10.1111/j.1463-6409.2008.00369.x
Rideout, N. K., Alavi, N., Lapen, D., Hajibabaei, M., Mitchell, G. W., Monk, W. A., et al. (2024). Quality versus quantity: response of riparian bird communities to aquatic insect emergence in agro-ecosystems. SSRN, 4835395. doi: 10.2139/ssrn.4835395
Rodriguez, J. J., Fernández-Triana, J. L., Smith, M. A., Janzen, D. H., Hallwachs, W., Erwin, T. L., et al. (2013). Extrapolations from field studies and known faunas converge on dramatically increased estimates of global microgastrine parasitoid wasp species richness (Hymenoptera: Braconidae). Insect Conservat. Divers. 6, 530–536. doi: 10.1111/icad.12003
Rosero-Garcia, D., Bickersmith, S. A., Suaza-Vasco, J. D., Porter, C., Correa, M. M., Conn, J. E., et al. (2017). Molecular operational taxonomic units of mosquitoes (Diptera: Culicidae) collected in high Andean mountain ecosystems of Antioquia, Colombia. Zootaxa 4277, 369–385. doi: 10.11646/zootaxa.4277.3.3
Ryberg, M. (2015). Molecular operational taxonomic units as approximations of species in the light of evolutionary models and empirical data from Fungi. Mol. Ecol. 24, 5770–5777. doi: 10.1111/mec.13444
Schmid-Egger, C., and Schmidt, S. (2021). Unexpected diversity in central European Vespoidea (Hymenoptera, Mutillidae, Myrmosidae, Sapygidae, Scoliidae, Tiphiidae, Thynnidae, Vespidae), with description of two species of Smicromyrme Thomson, 1870. Zoo Keys 1062, 49–72. doi: 10.3897/zookeys.1062.70763
Schmid-Egger, C., Schmidt, S., and Bogusch, P. (2024). DNA barcoding of central European Gasteruptiidae and the rarely-collected families Evaniidae, Stephanidae, Trigonalidae, and Aulacidae (Hymenoptera, Apocrita). Zoo Keys 1189, 275–286. doi: 10.3897/zookeys.1189.114478
Schmid-Egger, C., van Achterberg, K., Neumeyer, R., Morinière, J., and Schmidt, S. (2017). Revision of the west Palaearctic Polistes Latreille, with the descriptions of two species–an integrative approach using morphology and DNA barcodes (Hymenoptera, Vespidae). Zoo Keys 713, 53–112. doi: 10.3897/zookeys.713.11335
Shaw, M. R. (2022). Rearings of four European Microgastrinae (Hymenoptera: Braconidae), three new to Britain including a new species of Cotesia Cameron, 1891. Entomologist's Gazette 73, 165–175. doi: 10.31184/G00138894.733.1853
Shaw, M. R., and Hochberg, M. (2001). The neglect of parasitic Hymenoptera in insect conservation strategies: the British fauna as a prime example. J. Insect Conserv. 5, 253–263. doi: 10.1023/A:1013393229923
Wagner, D. L., Grames, E. M., Forister, M. L., Berenbaum, M. R., and Stopak, D. (2021). Insect decline in the Anthropocene: death by a thousand cuts. Proc. Natl. Acad. Sci. 118:e2023989118. doi: 10.1073/pnas.2023989118
Weber, A. A. T., Stöhr, S., and Chenuil, A. (2019). Species delimitation in the presence of strong incomplete lineage sorting and hybridization: lessons from Ophioderma (Ophiuroidea: Echinodermata). Mol. Phylogenet. Evol. 131, 138–148. doi: 10.1016/j.ympev.2018.11.014
Whitfield, J. B. (1997). “Subfamily Microgastrinae” in Manual of the New World genera of the family Braconidae (Hymenoptera). Special publication. eds. R. A. Wharton, P. M. Marsh, and M. J. Sharkey (Washington: The International Society of Hymenopterists), 333–364.
Williams, P. H., Altanchimeg, D., Byvaltsev, A., De Jonghe, R., Jaffar, S., Japoshvili, G., et al. (2020). Widespread polytypic species or complexes of local species? Revising bumblebees of the subgenus Melanobombus world-wide (Hymenoptera, Apidae, Bombus). Eur. J. Taxonomy 719, 1–120. doi: 10.5852/ejt.2020.719.1107
Zahiri, R., Lafontaine, J. D., Schmidt, B. C., Dewaard, J. R., Zakharov, E. V., and Hebert, P. D. (2017). Probing planetary biodiversity with DNA barcodes: the Noctuoidea of North America. PLoS One 12:e0178548. doi: 10.1371/journal.pone.0178548
Keywords: agriculture, biological control, checklist, dark taxa, DNA-barcoding, Microgastrinae
Citation: Beaudin M, Höcherl A, Boudreault C, Cullingham CI, Lapen D and Fernandez-Triana J (2025) A research hotspot for Microgastrinae parasitoid wasps (Hymenoptera, Braconidae) in North America: DNA barcoding reveals the need for increased taxonomic efforts in dark taxa. Front. Sustain. Food Syst. 9:1457055. doi: 10.3389/fsufs.2025.1457055
Edited by:
Lóránt Dénes Dr. Dávid, John von Neumann University, HungaryReviewed by:
Gavin Broad, Natural History Museum, United KingdomMichael Caterino, Clemson University, United States
Michael Sharkey, University of Kentucky, United States
Copyright © 2025 Beaudin, Höcherl, Boudreault, Cullingham, Lapen and Fernandez-Triana. This is an open-access article distributed under the terms of the Creative Commons Attribution License (CC BY). The use, distribution or reproduction in other forums is permitted, provided the original author(s) and the copyright owner(s) are credited and that the original publication in this journal is cited, in accordance with accepted academic practice. No use, distribution or reproduction is permitted which does not comply with these terms.
*Correspondence: Melanie Beaudin, bWVsYW5pZWJlYXVkaW5AY21haWwuY2FybGV0b24uY2E=