- 1International Maize and Wheat Improvement Center (CIMMYT), ICRAF Campus, Nairobi, Kenya
- 2Genetic Innovations Initiative on Accelerated Breeding of the Consultative Group on International Agricultural Research, Nairobi, Kenya
- 3Zambia Agricultural Research Institute (ZARI), Lusaka, Zambia
- 4Department of Research and Specialist Services (DR&SS), Crop Breeding Institute, Harare, Zimbabwe
- 5National Crops Resources Research Institute, National Agricultural Research Organization, Kampala, Uganda
The Genetic Innovation Initiative on Accelerated Breeding (ABI) of The Consultative Group on International Agricultural Research (CGIAR) has been supporting the costing of breeding operations for the CGIAR-National Agricultural Research and Extension Systems-Small to Medium Enterprises (CGIAR-NARES-SME’s) crop breeding networks. The aim is to help these breeding programs to accurately estimate operational costs, develop precise budgets, set appropriate service fees, and choose the best technologies for increased genetic gains. Breeding programs are being guided in using the University of Queensland’s open-source breeding costing tool (UQ-BPCT). This paper outlines the costing strategy and demonstrates the tool’s utility using data from national breeding programs in Uganda (NARO), Zambia (ZARI), and Zimbabwe (DR&SS). Results show that the percentage of budgets allocated to germplasm development ranged from 25% (DR&SS) to 52% (NARO), with conventional methods costing 7 to 47 times more than doubled haploids. Costs for trials varied, with ZARI spending 14% and DR&SS spending 51%. In one breeding cycle, NARO released 5 hybrid varieties, ZARI 2, and DR&SS 1. The programs can be optimized by implementing several strategies: adopting an Enterprise Breeding System, incorporating digital technologies for disease screening and phenotyping, network-based procurement of consumables, using modern breeding techniques like doubled haploids, genomic selection, and speed breeding to shorten cycles, and training personnel for more efficient resource use.
1 Introduction
Maize is the second most cultivated crop after wheat and serves as a primary source of nourishment for human diets and animal feed globally. In sub-Saharan Africa (SSA), maize accounts for 40% of cereal production, and it provides at least 30% of the human calories (Nuss and Tanumihardjo, 2010; Ekpa et al., 2019). To meet the food demands of the increasing human population, which is expected to reach 9.7 billion by 2050, it is estimated that the global maize productivity must be increased by 67% (Ray et al., 2013). Climate change presents a formidable challenge in crop production, manifesting into crop failures due to drought and floods, pest and disease epidemics which greatly undermine efforts to increase food security. Due to the adverse effects of climate change, maize production in SSA is predicted to decline by 10–20%, with a reduction in the land area where the crop is cultivated (Cairns et al., 2013). To mitigate further effects of climate change which may arise from human invasion of forests and wetland degradation to expand the land for maize farming, it is environmentally safer to adopt sustainable farming practices such as production of high yielding varieties (Godfray et al., 2010; Foley et al., 2011). Modernization of pipelines to facilitate cost-effective and rapid development and deployment of resilient, high-yielding crop varieties to withstand effects of climate change is a potential way to offset the production losses under increasing climate variability and ensure food security (Acevedo et al., 2020; Cairns et al., 2021).
Modern maize breeding comprises multiple and resource-intensive processes with the implementation stages (Figure 1), such as pre-breeding, inbred line development and testing, germplasm development and testing, varietal registration and release, and seed systems management (Bishaw and And Gastel, 2009; De Vries et al., 2023). Optimization of the maize breeding entails adopting cost-effective strategies to increase genetic gains per unit cost in breeding programs and, hence, rapid development and release of superior varieties (Atlin et al., 2017; Cobb et al., 2019). A recent analysis of historical maize data has revealed significantly positive but relatively low genetic gains for maize grain yields in national breeding programs compared to similar efforts by International Maize and Wheat Improvement Center (CIMMYT) in Eastern and Southern African (ESA) (Asea et al., 2023; Ligeyo et al., 2024; Mazibuko et al., 2024; Mukaro et al., 2024). Modernizing the national ESA maize breeding programs can enhance cost-effectiveness in resource utilization toward increasing genetic gains in the development of resilient maize varieties for cultivation in emerging agro-ecologies (Cairns et al., 2013; Atlin et al., 2017; Ligeyo et al., 2024).
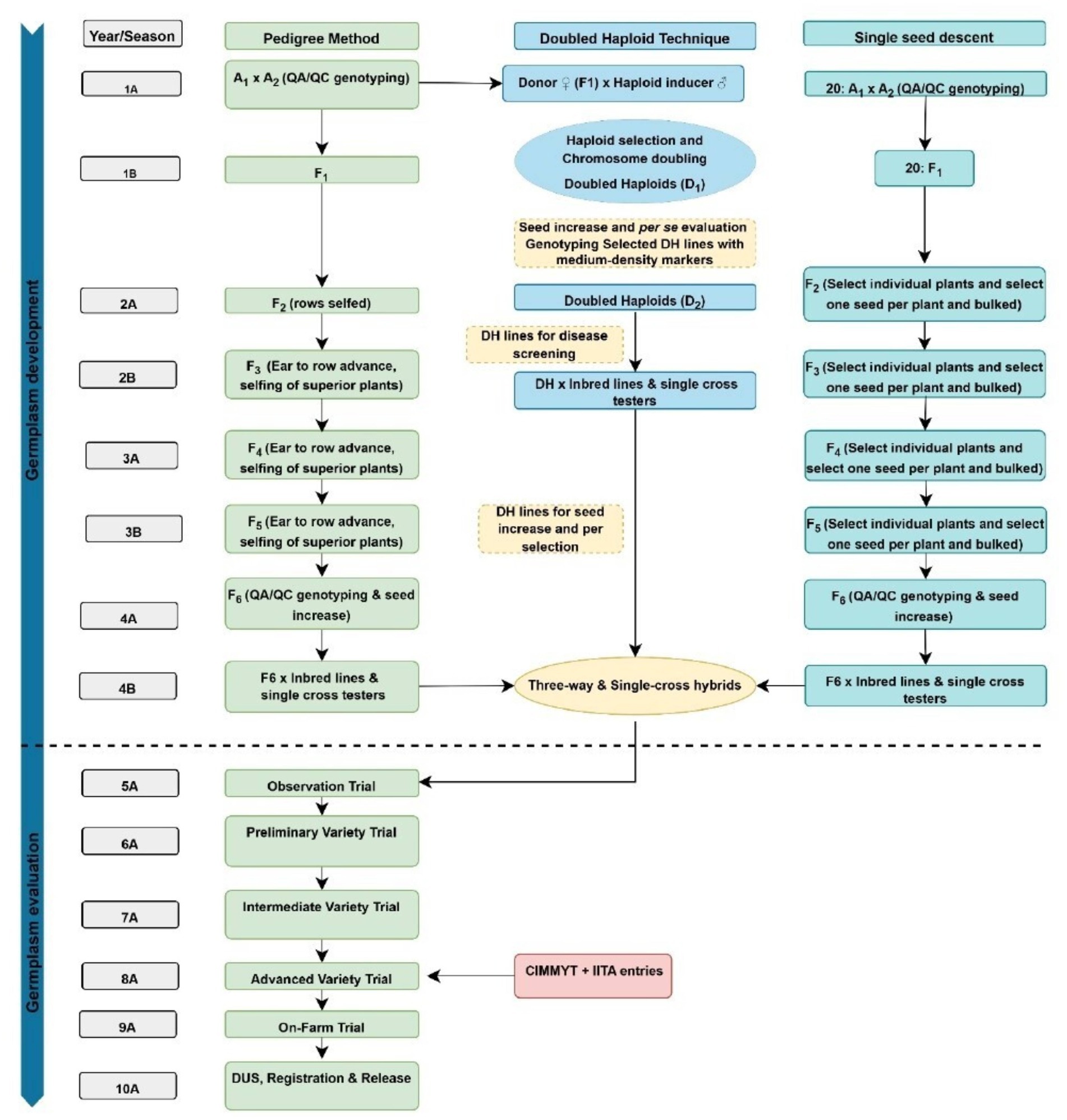
Figure 1. A generic breeding schematic flow showing the key stages in the maize hybrid breeding pipeline. Inbred lines were developed using Pedigree, Single Seed Descent (SSD), and Doubled Haploid (DH). The number of entries, locations, and replications varied among programs.
Plant breeding is the science and art of developing elite crops by creating and reassembling desirable heritable traits for specific products to meet market needs through a resource-intense development process over time (Li, 2020). The breeding costs could be driven by the crop biology factors which determine how the operations are executed, e.g., the available germplasm, the life cycle of the plant, the type of pollination, the complexity of the traits being integrated into a specific genetic background or product profile, and the choice of the breeding method. Furthermore, breeding costs could depend on the available technology, facilities and equipment, skillset, existing regulatory frameworks, funding, collaborative partnerships and their roles, soil and climatic conditions, farmers participation, data management and analysis, and the intellectual property and licensing charges (Ceccarelli, 2009). It is crucial for program managers to plan and budget carefully to ensure the efficient allocation of resources and the achievement of breeding objectives.
The business-oriented strategy for the private breeding programs could serve as a benchmark for the national (public) breeding programs to be more sensitive to the costs of running each stage of a breeding pipeline (Lammerts Van Bueren et al., 2018; Cobb et al., 2019). The private breeding programs are highly structured with specialized and well-resourced product design and germplasm development teams and have established strategic investment mechanisms (Cobb et al., 2019; Brzozowski et al., 2022). The maize breeding programs of national agricultural research and extension systems (NARES) of ESA countries are funded by governments, with additional bilateral and collaborative support from both public and private institutions. Compared to the private maize breeding programs, the national breeding programs have limited funding and personnel, high labor attrition rates and maybe working on multiple target product profiles. The emerging concerns of climate change, a need for self-sufficiency, limited budgets and political accountability have compelled public leaders to set systems and policies from variety development to marketing in responding to national challenges (Barthel et al., 2013). As it has been in the private sector, leaders in public sector are, therefore, demanding accountability for the costs of the resources used in public breeding operations (Barthel et al., 2013; Alford and Greve, 2017). These resource and operation challenges for the NARES are better addressed with clear understanding of operation costs to inform and implement methods, approaches and investments in tools and technologies for increased breeding efficiency, effectiveness and profitability.
Costing is a strategy which enables the public breeding programs to implement the recommended optimization measures, which would ensure that the limited resources are utilized to achieve maximum genetic gains at affordable costs (Mangione et al., 2006; Cobb et al., 2019). Costing could enable the program leaders to set priorities which would increase the chances of being competitive across market segments and to maximize the gains per unit cost of investment (Cottet, 2021). Unfortunately, there has been a lack of information on standardized methods and approaches to determine what it costs to implement the activities of the different stages of a breeding pipeline. The University of Queensland Breeding Program Costing Tool (UQ-BPCT) is a publicly available standalone costing software which was developed recently by researchers at The Queensland Alliance for Agriculture and Food Innovation (QAAFI) of The University of Queensland in Australia.1 This free software has a standardized approach to cost-out breeding operations through financial models of breeding pipelines targeting specific target product profiles using a modular framework incorporating unit costs multiplied by experimental dimensions within defined breeding activities (Mace, 2021). Prior to the development of the UQ-BPCT, breeders did not know of any standardized method for costing breeding stages (B. Das, personal comm.).
A lack of implementation of standardized, harmonized and systematic costing in breeding programs had led to breeding inefficiencies due to failure to identify high-cost areas and effectively allocate resources to cheaper breeding methodologies with higher gains. To address this concern, the Accelerated Breeding Initiative (ABI) of the Consultative Group on International Agricultural Research (CGIAR) has been supporting crop breeding programs within the CGIAR, National Agricultural Research and Extension Systems (NARES) and other Small to Medium Enterprises (SMEs) to cost out their operations using the UQ-BPCT. Besides, this pioneering effort that is being reported here, Excel-based worksheet methods for estimating the operational costs of the different stages of breeding programs have been documented (Wannemuehler et al., 2019; Wannemuehler et al., 2020; Atlin and Econopouly, 2022). Here, we describe the strategy used by the ABI and its crop breeding networks to standardize the costing of breeding programs and demonstrate how the strategy was applied in the costing of three maize program of Eastern and Southern Africa. It also provides recommendations for streamlining operations and attaining cost-effectiveness while enhancing genetic gains for the three costed maize breeding pipelines.
2 Materials and methods
2.1 Overview of the costing strategy
The costing implementation strategy and the potential transformation impacts on various stakeholders are summarized in Figure 2. Supporting the costing of breeding programs within the CGIAR-NARES-SMEs networks would enhance the following capabilities: (1) accuracy of determining the costs of running a particular activity, (2) ease of identification of the activities and items that make the largest contribution to the cost of breeding activity, (3) conducting “what if” scenarios which allow for exploration of the consequences of changing elements, (4) evaluating the resource costs and its implications on the breeding activity or project (e.g., increasing numbers of trials, implementing partial replication, etc.), (5) estimation of costs of fees for service activities, and (6) building of accurate breeding program budgets. It is anticipated that these capabilities will eventually enhance management planning and accuracy in reporting the cost implications of the breeding programs. The CGIAR-NARES-SMEs crop breeding networks exist within the mandate countries in Eastern and Southern Africa, West and Central Africa, Central and West Asia and North Africa, South Asia, Southeast Asia and the Pacific, and Latin America and the Caribbean (LAC) regions (McIntire and Dobermann, 2023).
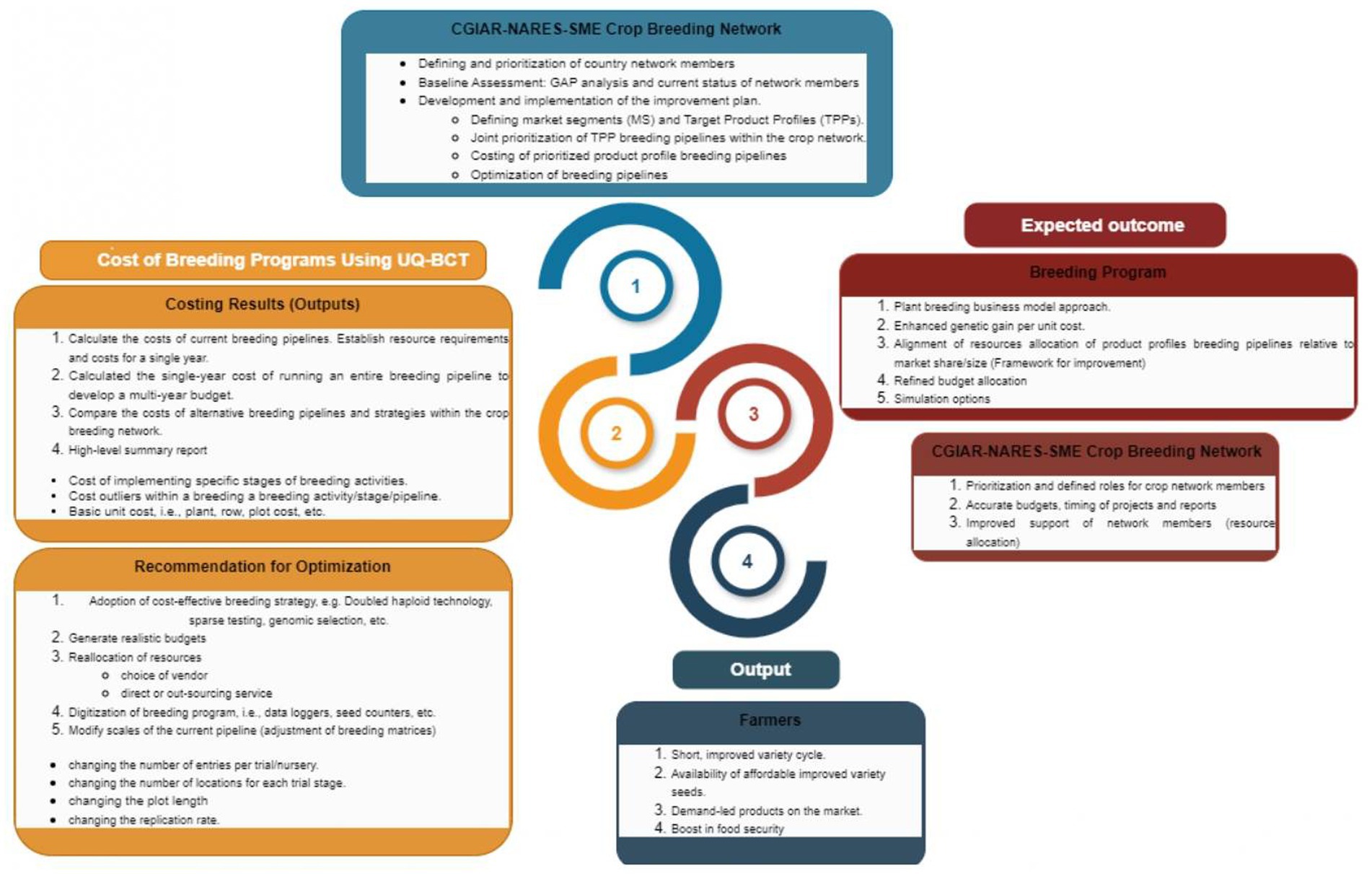
Figure 2. Schematic flow of breeding program assessment and implementation of the improvement plan, i.e. costing of the breeding program using the University of Queensland Breeding Costing Tool (UQ-BPCT) within the crop breeding network.
2.1.1 Engaging the programs for costing of their breeding pipelines
To initiate the costing support, a communication on the need for the costing of the pipelines originates from the crop breeding leads, and this is followed by planning for a joint inception meeting with the ABI team. The program to be costed provides an overview of the breeding metrics for the operational pipelines of the individual crops. The implementing staff of the breeding program is identified, and the timelines for costing are agreed upon. The implementing staff, which generally is a breeder, a research manager or a research associate, will work with the ABI team to capture the items and labor data into a standard Excel template, upload the data into the UQ-BPCT, conduct the costing within the UQ-BPCT, and write the costing report.
The three national maize programs reported here were part of the many others that were supported to cost their breeding pipelines. The target market segments and the priority traits for the product profiles of the three programs are described in Tables 1, 2. For similarity in crop cycle lengths and other similar aspects of the agro-ecologies which could influence the breeding operations, the report focuses on the costing of the intermediate product profiles which span the mid-altitudes target product environment of Eastern (Uganda) and Southern (Zambia and Zimbabwe) African countries (Table 1). The reported maize programs have mainly relied on conventional breeding methods and are now integrating modern methods such as marker assisted and genomic selection breeding, and double haploid technology for germplasm development. These NARES programs work in close collaborations and share resources with CGIAR centers such as CIMMYT and IITA. The programs have also transitioned from trait-based breeding into product profile-based breeding approaches. This strategy allows organizations to develop varieties that are well-aligned with the needs of consumers, farmers and processors within the target market segments. The medium maturity maize accounts for the highest percentage of the market share in Uganda (38%), Zambia (69%) and Zimbabwe (31%) (Table 1).
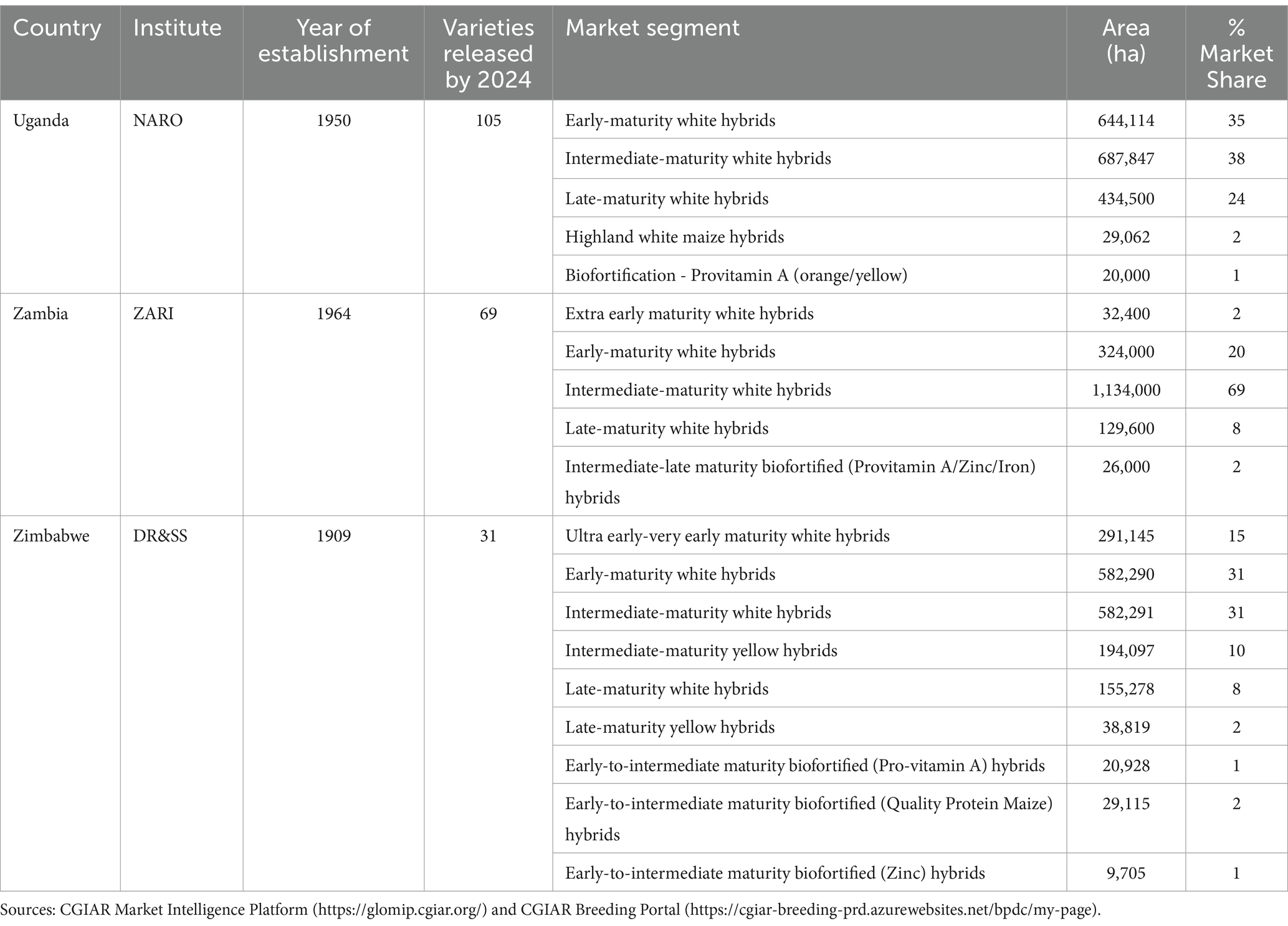
Table 1. The targeted market segments for the maize breeding programs in Uganda, Zambia, and Zimbabwe.
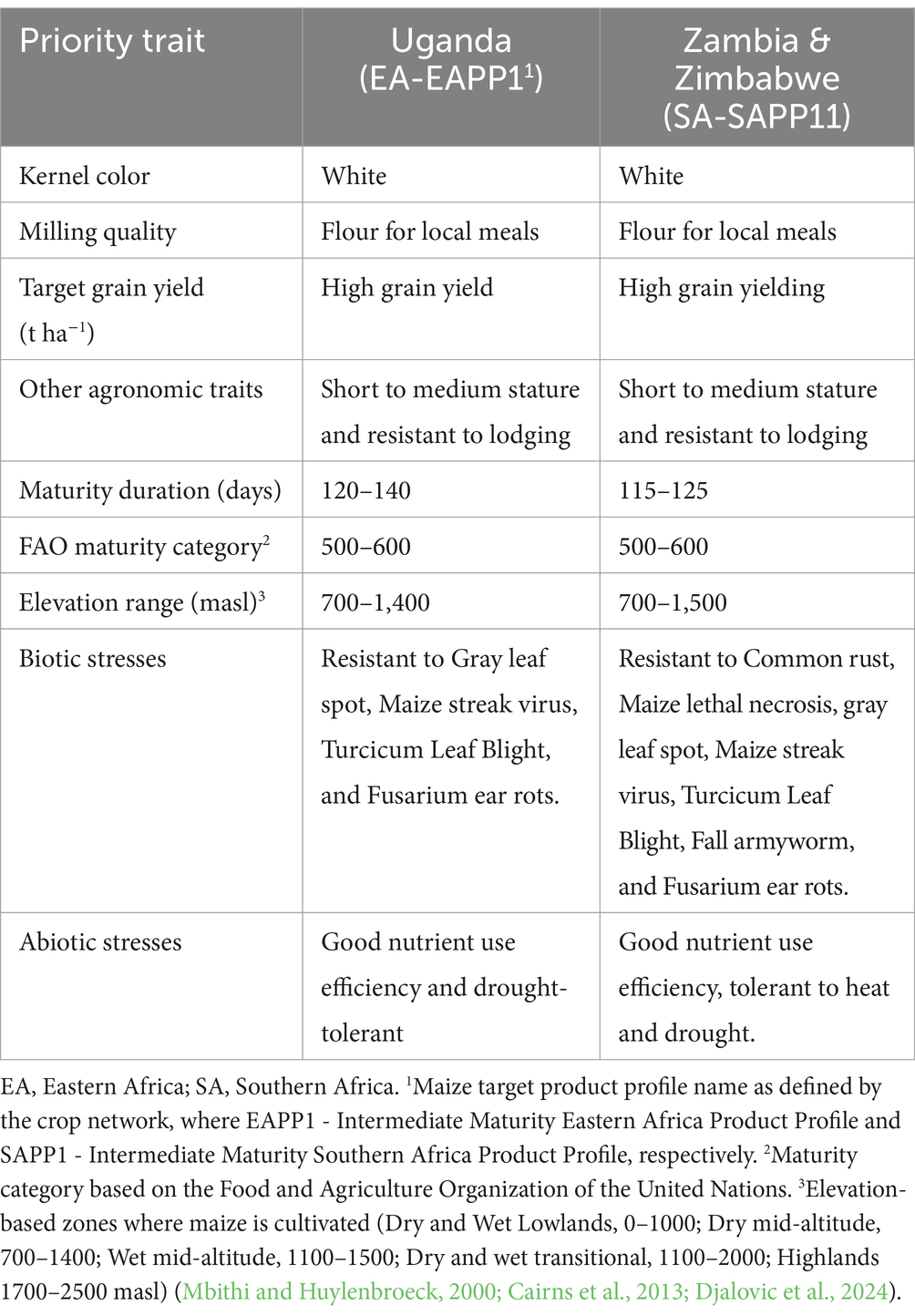
Table 2. Description of the mid-altitude agroecological zones and the priority traits for the maize varieties bred for the zone in Eastern and Southern Africa.
2.1.2 Key considerations in costing of breeding operations
2.1.2.1 Availability and policy for sharing breeding operations data
Cost accuracy depends on the availability and quality of the required data from the supportive sections within the institution. Institutions with modern, easy-to-query databases can be more efficient in providing the required information. At the same time, proper records could make it easier to cost a program. Furthermore, different institutions have varying regulations for public data privacy. Some institutions may need help approving personal information such as wages and salaries released into the public domain, which affects the capture of the data on labor rates. However, the extent to which this may be a bottleneck in labor costing is minimal because most institutions may provide data for casuals and other junior staff, contributing to the highest percentage cost in breeding operations. To overcome the challenge of disclosing some sensitive information, the salaries for senior personnel can be taken as fixed costs and hence reducing the interference with the operational costing process. However, the institution should consider this fixed salary cost when building a budget.
2.1.2.2 Personnel for implementation of breeding costing
Collecting the breeding operations data from the different breeding support units requires patience, particularly when seeking approvals and filtering the required information from large institutional datasets. Thus, the program should dedicate breeding staff who would be willing to learn and implement the costing process.
2.1.2.3 Structure of the breeding program
The costing process is based on determining the costs of breeding operations for a specific target product profile whose specific traits would replace those of an obsolete variety (Williamson and Leonelli, 2023). A breeding pipeline refers to the stages for the processes of development, testing and release of a crop variety (Mace, 2021). The stages of each pipeline (e.g., New starts, F1 crosses, F2, … Advanced Varietal Trial) constitute the components of the pipeline (Figure 1). To compare the costs of operations within the activities of each stage of a breeding pipeline, each pipeline should be costed as a separate project. The activities of each stage represent a combination of tasks performed to accomplish a given stage’s goals within a given period, e.g., hybridization (Figure 1).
2.1.2.4 Trial locations and outsourcing of breeding support services
The cost of implementing the breeding operations is affected by factors relating to the operational sites. Conducting trials away from the coordination center is unavoidable because of the multilocational phenotyping, which must be implemented to assess the stability of the traits of interest across multiple locations. For accuracy in costing the travel expenses, there is a need to provide information about the locations where the activities would be implemented, the program personnel to be involved, days of visit, travel frequency, the fuel consumption rate of the vehicle and the accommodation details. In the case of a program which outsources phenotyping and genotyping support, the costs of affected stages are influenced by the fees charged by partners. Outsourced phenotyping of row crops such as maize may attract a cost per row charged by the partners, shipping costs, and permits from the regulatory authorities. Similarly, genotyping services will attract a cost per 96 or 484-well plate of samples for a specific number of genotypes and the associated shipping costs. This information is valuable and must be provided by the program which is to be costed.
2.1.2.5 The biology of the crop vs. the breeding operations calendar
The costing of operations is based on the duration within a year for which the activities of a given stage occur. For the mid-altitude zone of ESA, two maize crop cycles are expected in a year. The cost implementers must understand the crop’s biology and the start/end times for the operations in different components of each pipeline. The financial calendar of the breeding program is important because it determines how the fixed costs are shared across the components. For example, for a biannual crop, the fixed costs for paying the salaries of the permanent staff would be covered in the two seasons of the year. During this period, the permanent workers of the program are paid to work on two subsequent stages, and part of the costs are shared and placed into each stage, e.g., F1 and F2 advancements.
2.1.3 The costing platform
Costing was implemented using the free software called The University of Queensland Breeding Program Costing Tool (UQ-BPCT) (see text footnote 1). The tool has features that calculate the cost of running a crop breeding activity or an entire breeding pipeline, using the prices, costs, and salaries from a single season or year. The CGIAR Genetic Innovations program leaders endorsed the costing tool for use across its crop breeding networks. The data used for the costing had been captured using a customized Microsoft Excel template.
2.1.4 Overview of data capture and calculation of unit costs
The data capture step is critical and involves liaising with the procurement, human resources, and other administrative personnel to gather the basic information for the costing process. The systematic operations of a breeding program are described in a breeding scheme (Figure 1), which is a diagram illustrating the germplasm and key technologies applied in the development and evaluation stages of the breeding process of a given variety. Formalized breeding schemes are a key component of breeding program design and a gateway to conducting plant breeding as a quantitative process (Covarrubias-Pazaran et al., 2022). A breeding scheme is required in costing because it provides the metrics for the stages (components), the number of genotypes, and the activities for the stages of each pipeline (Covarrubias-Pazaran et al., 2022). Additionally, the breeding program provides breeding metrics that align with the design of experiments to implement the activities of the stages within the breeding scheme [e.g., the number of locations, genotypes (entries), replications, and the number of rows or plants per genotype within a replication for each activity] (Figure 1 and Supplementary Tables 1–3).
There are two broad categories of costs in breeding operations, i.e., the operational and fixed costs. Operational costs include items and labor procured for the regular implementation of the tasks of an activity and spent fully in a season (e.g., fertilizer, envelopes, etc.). The items and labor to be applied in any activity depend on the goals of the breeding stage, as defined in the breeding scheme (Figure 1). Fixed costs are equipment or labor costs incurred whether the breeding program has an active project and can be used or recycled for more than one season (e.g., machinery, vehicles, furniture, etc.). Fixed labor includes the costs of paying salaries for permanent and pensionable workers. Fixed costs do not vary by activity of a given stage and are assumed to be shared across the breeding stages.
Upon capturing the data labor, the Excel worksheet calculates unit costs for each item, labor, and fixed costs. Unit costs are the monetary estimates for labor and items (or consumables) labor and items (or consumables) expenses for the smallest experiment units (experiment dimension) within the breeding operations. A demonstration of how to compute the unit costs with examples from maize breeding operations is provided in Supplementary Table 4.
2.1.5 Use of unit costs in the calculation of the costs of activities within the breeding stages
A detailed description of how the UQ-BPCT operates is publicly available online at: https://excellenceinbreeding.org/video/webinar-costing-breeding-programs. Briefly, the activity unit costs of the item and labor were combined and were then assigned the name of an activity, e.g., hybridization. After that, each activity unit cost for items was multiplied by the appropriate consumption quantities. On the other hand, the labor activity unit costs are multiplied by the duration of work or the output parameter relative to the experiment dimension to arrive at the final task costs in a season. The sum of item and labor costs constituted the seasonal operational activity cost for each stage. The fixed costs were assigned to a separate activity and posted once at each breeding stage.
Each activity was analyzed to ensure that the total costs reflected the actual seasonal expenditures. The costing tool allows the user to review the costs by sorting in the activity costs window. This step fixes any issues arising from the unit costs calculation or the quantities assigned to the experiment dimensions. Furthermore, the costs of the components can be compared using preferred methods, such as the bar charts within the UQ-BPCT. The final costing results were exported to an Excel worksheet and were used for downstream analyses. The percentages of the costs for the activities of different stages were computed relative to the stage categories or the overall pipeline cost. For each of the activities, the cost per row was computed while considering the breeding metrics provided earlier by the breeding programs. The relationships between the cost per row, the number of entries, number of rows and the distance traveled (from the coordination center) to the nurseries or trial sites were determined using linear regression models which were implemented in JMP Pro Ver. 18 (SAS Institute Inc., Cary, NC, USA, 1989–2023).
3 Results
3.1 The operational costs for the three national medium maturity maize TPPs in ESA
The three national maize programs are implementing breeding activities for multiple target product profiles. Here, we report the findings for the operational costs of the medium (or intermediate) maturity maize for the mid-altitude zones for Uganda, Zambia and Zimbabwe (Table 2). The fixed costs were highly variable and may not have reflected the immediate program running costs and were not included in the current report. The results of the costing provide insights into the costs of implementing the activities, and the potential optimization strategies in the respective breeding pipelines. Because the product profile names are similar, we use the names of the programs (DR&SS for Zimbawe National Research Institution, NARO for Uganda National Research Institution and ZARI for Zambia National Research Institution) for ease of reporting in the rest of the sections.
All the costed programs had breeding pipelines comprising costs for germplasm development and product testing, nursery and seed production, and national performance and trait confirmation trials and registration stages. Although the three programs were adopting doubled haploid (DH) in inbred line development, the conventional inbred line development methods dominated the process with single seed descent method (SSD) in NARO and the pedigree method in ZARI and DR&SS. Except for ZARI, which had F7, the other two programs had inbred line development stages running from new starts to F6 (Figure 2). DR&SS had the most extensive germplasm testing stages with observational varietal trials and varietal demonstration stages, which were not cost-reported by ZARI and NARO (Figure 3). On the other hand, ZARI lacked IYT.
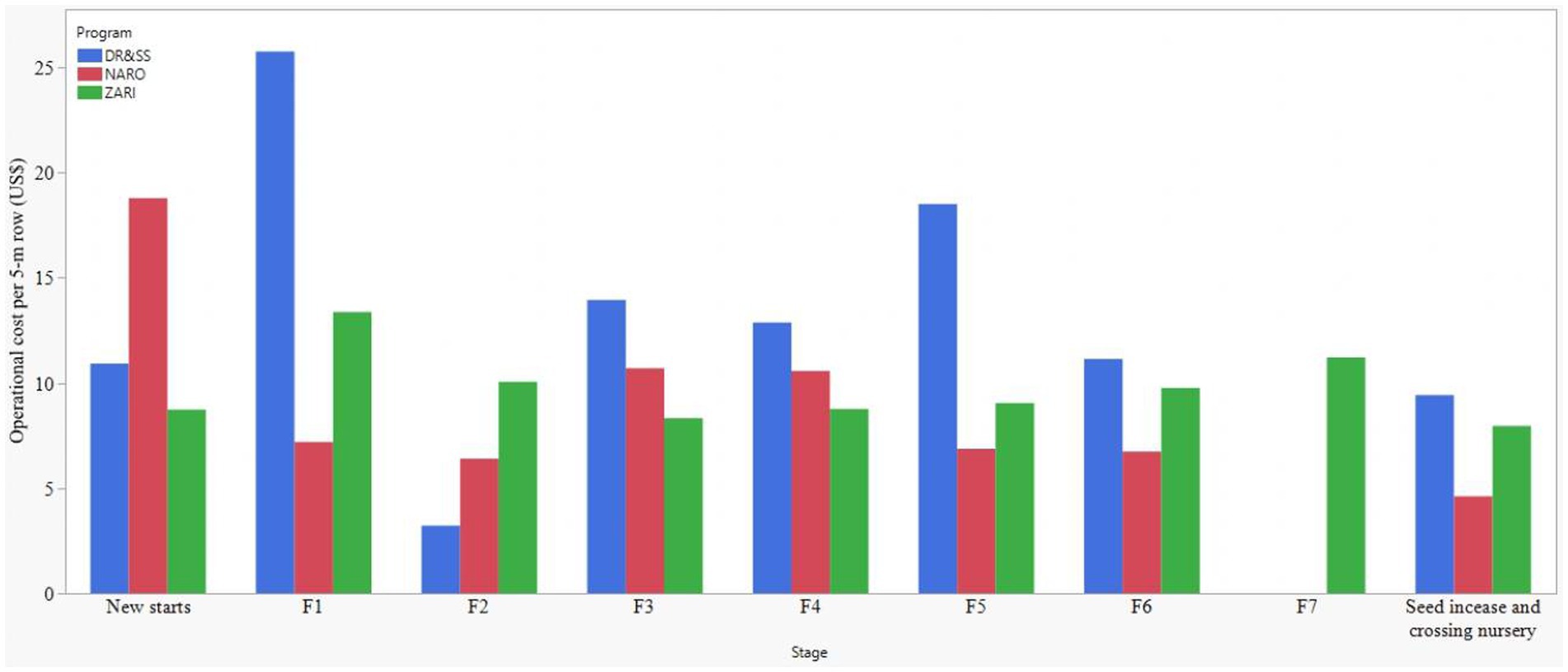
Figure 3. Operational cost of 5-meter rows across the germplasm development breeding stages (components) within three intermediate maturity product profiles of eastern and southern African national maize breeding programs.
On the implementation of the activities of the project, it was observed that the main nursery operations for germplasm crossing, selfing for advancement or seed increase were conducted at the coordination research stations (Golden Valley for ZARI and Namulonge for NARO), but for DR&SS which had nurseries in Harare, Muzarabani or Chisumbanje. For germplasm testing stages, ZARI had four main locations, NARO had eight, and DR&SS had eight locations with different environments. The three programs conducted conventional on-farm testing of their germplasm before entering them into multilocational national performance trials, trait validation, and release.
The operational cost for the NARO breeding pipeline was US$85,660, which constituted the cost of germplasm development (52%) and product testing (43%). The rest of the costs went to the isolation block, seed increase, and varietal release costs. Within the germplasm development cost, SSD inbred line development method constituted 57% and on average costs $733.20 per elite line. The doubled haploid line development was allocated 43% of the germplasm development cost and on average costed $15.49 per inbred line. The program had five hybrids evaluated under on-farm trials and would eventually release the five per breeding cycle (Table 3 and Supplementary Table 1). The cost for evaluating the Distinctness, Uniformity and Stability (DUS) for traits and varietal registration was $235 per variety (Table 3). The stages with the highest operational costs per row were the new starts and preliminary yield trials (Figures 3, 4). Overall, the highest costs for on-station nurseries included the labor for pollination, harvesting and seed handling, and the purchase of pollination bags (Table 4). The costs of labor for crop guarding and collection of data for plant architecture traits, flowering time and disease scores were high in both on-station and off-station trials. Travel, per-diem and accommodation costs were high for off-station trials (Table 4).
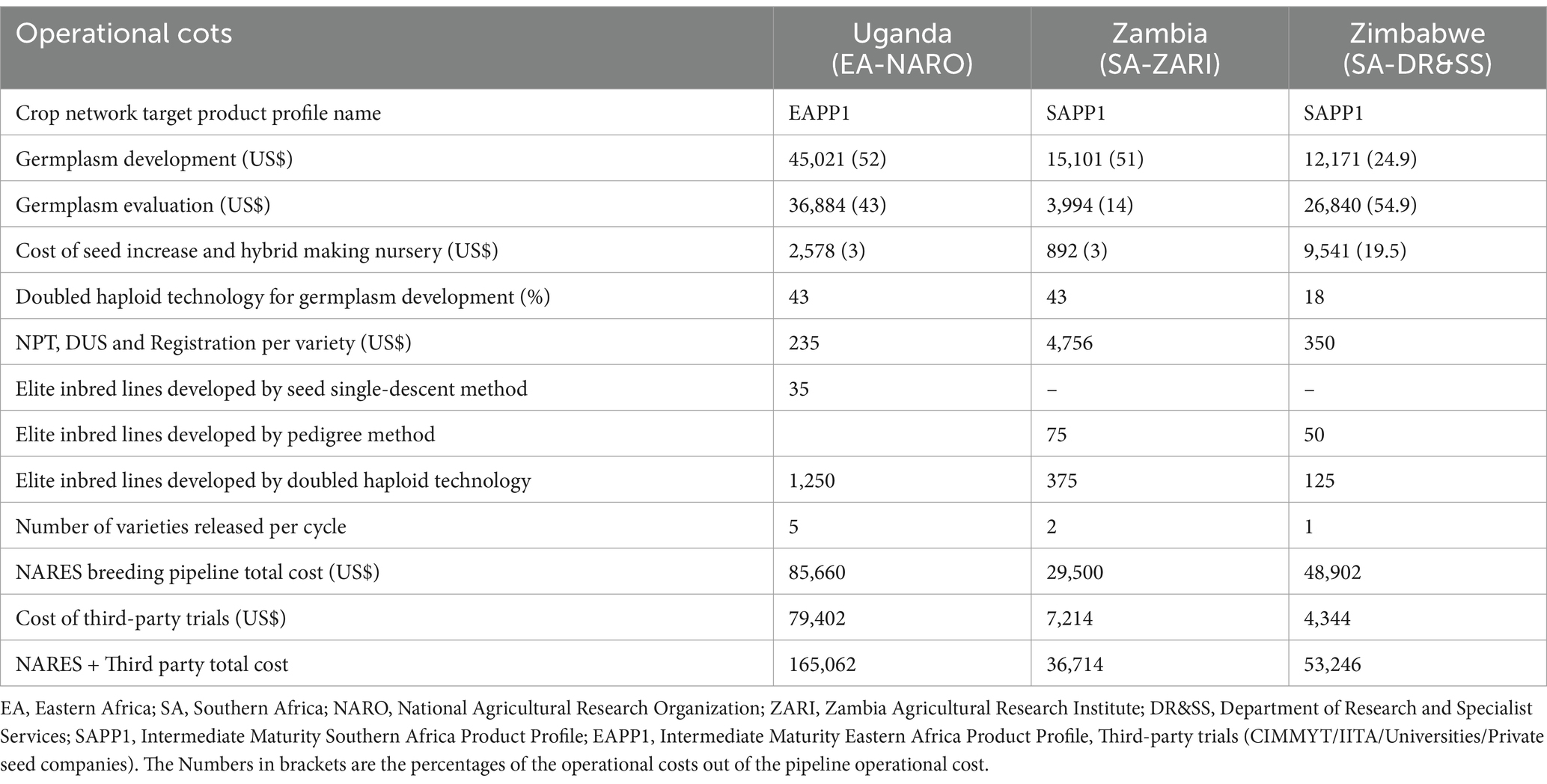
Table 3. Description and operational costs (US$) maize Mid-altitude Eastern and Southern Africa intermediate product profile breeding pipeline.
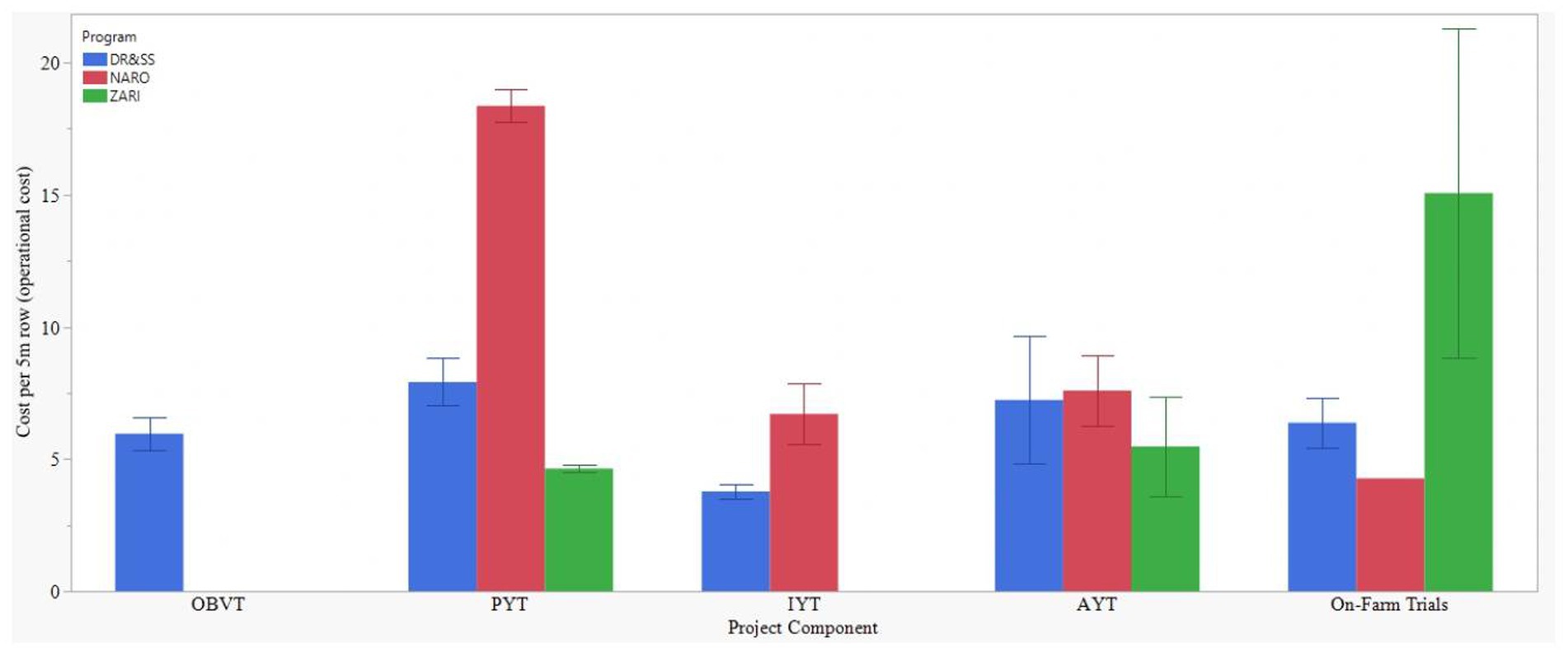
Figure 4. Costs of 5-meter rows across the germplasm evaluation stages (components) within three intermediate maturity product profiles of eastern and southern African national maize breeding programs.
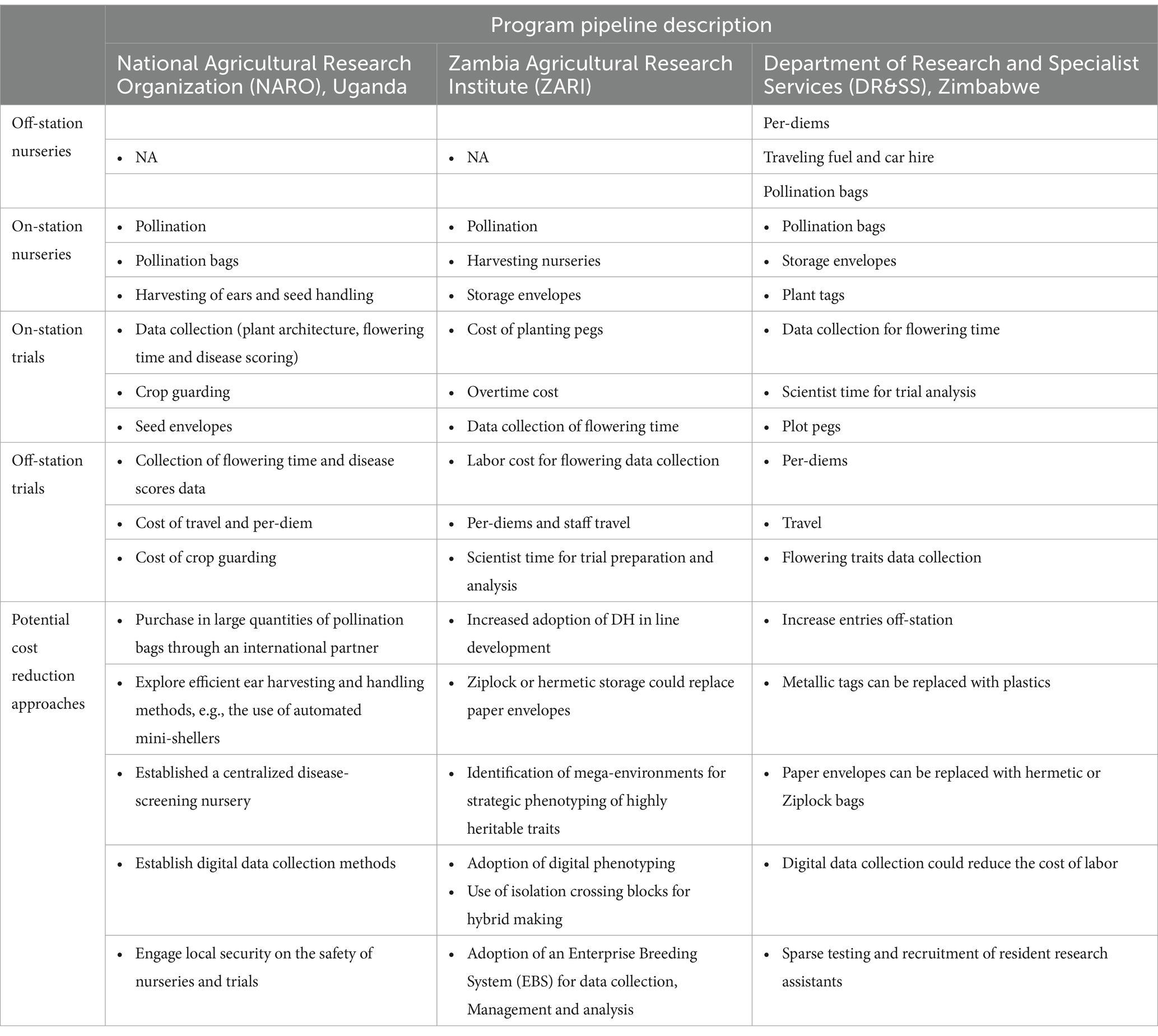
Table 4. A list of the three most expensive items and labor within the breeding programs of Eastern and Southern Africa.
The operational cost for the ZARI breeding pipeline was US$29500, which constituted the germplasm development (51%), product testing (14%), the national performance trial, DUS and registration of two varieties (32%) and the rest went to seed increase and testcrossing (Table 4). The pedigree line development method constituted 57% of the germplasm development budget and had a cost output of $114.77 per elite line. The doubled haploid constituted 43% of the germplasm development budget and had a cost output of $17.32 per inbred line. The program took six hybrids for on-farm trials, and they managed to register and release two (Table 3 and Supplementary Table 2). The cost of conducting NPT, DUS and registration of each variety was $4,756 (Table 3). The highest operational costs per 5-m row were observed in OFT and F1 (Figures 3, 4). The highest cost for on-station nurseries was in the labor for pollination and harvesting and the purchase of storage envelopes. Labor for staff overtime and purchasing planting pegs were high costs in on-station trials. Collection of the flowering time data was high for both on-station and off-station trials. Labor for the scientist trial design and analysis and travel, per-diem, and accommodation costs were high in off-station trials (Table 4).
The operational cost for the DR&SS breeding pipeline was US$48,902, with about one-quarter of it going to germplasm development, while product testing took about slightly above half the budget. Seed increase and test-crossing nurseries took about 19.5% of the pipeline cost (Table 3). Conducting NPT, DUS, and varietal registration costs US$350 per variety (Table 3). The pedigree inbred line development method took 82% of the germplasm development budget cost and had a cost output of US$199.60 per elite line, while doubled haploid took the rest (18%) of the cost and had a cost output of US$17.50 per inbred line. The stages with the highest cost per 5-m row were F1 and F5, and these activities were conducted away from the main research coordination center (off-station). The costs of purchasing plant tags were high for on-station nurseries only. The off-station nurseries have high costs, per-diem and accommodation, as with the off-station trials (Table 4). Similarly, the cost of purchasing pollination bags and labor for collection of flowering time data was high for both on-station and off-station nurseries (Table 4). The labor for trial analysis and the costs of purchase of plant pegs were high in on-station trials (Table 4).
A significant negative correlation was observed between the cost per row and the number of rows across breeding stages (ρ = −0.26, p = 0.026; Figure 5). However, the relationship between the number of entries for nurseries or trials was marginal (ρ = 0.23, p = 0.055; Figure 5). Furthermore, analysis of the relationships between the distance from the maize research coordination center to the location where a trial or nursery was conducted and the cost per row revealed a positive correlation in germplasm development and testing stages (Figure 6). Besides their pipeline operations, all programs implemented third-party trials for private companies and CGIAR partners at different locations and scales. Compared to the costs for germplasm testing in their medium maturity projects, NARO and ZARI had about twice as much for third-party trials, while the DR&SS third-party budget was 16% of their program germplasm testing (Table 3). To implement the third-party trials, the programs would agree with the partners to charge a fee based on the number of rows evaluated. Based on the analyses using the data for germplasm development, the minimum cost of implementing the activities was $9.35 per 5-m row, and the minimum for the germplasm evaluation was $5.73 per row (the y-intercept of the regression line). Furthermore, conducting a trial away from the coordination center increased the cost by $1 for every 130 km (Figure 6).
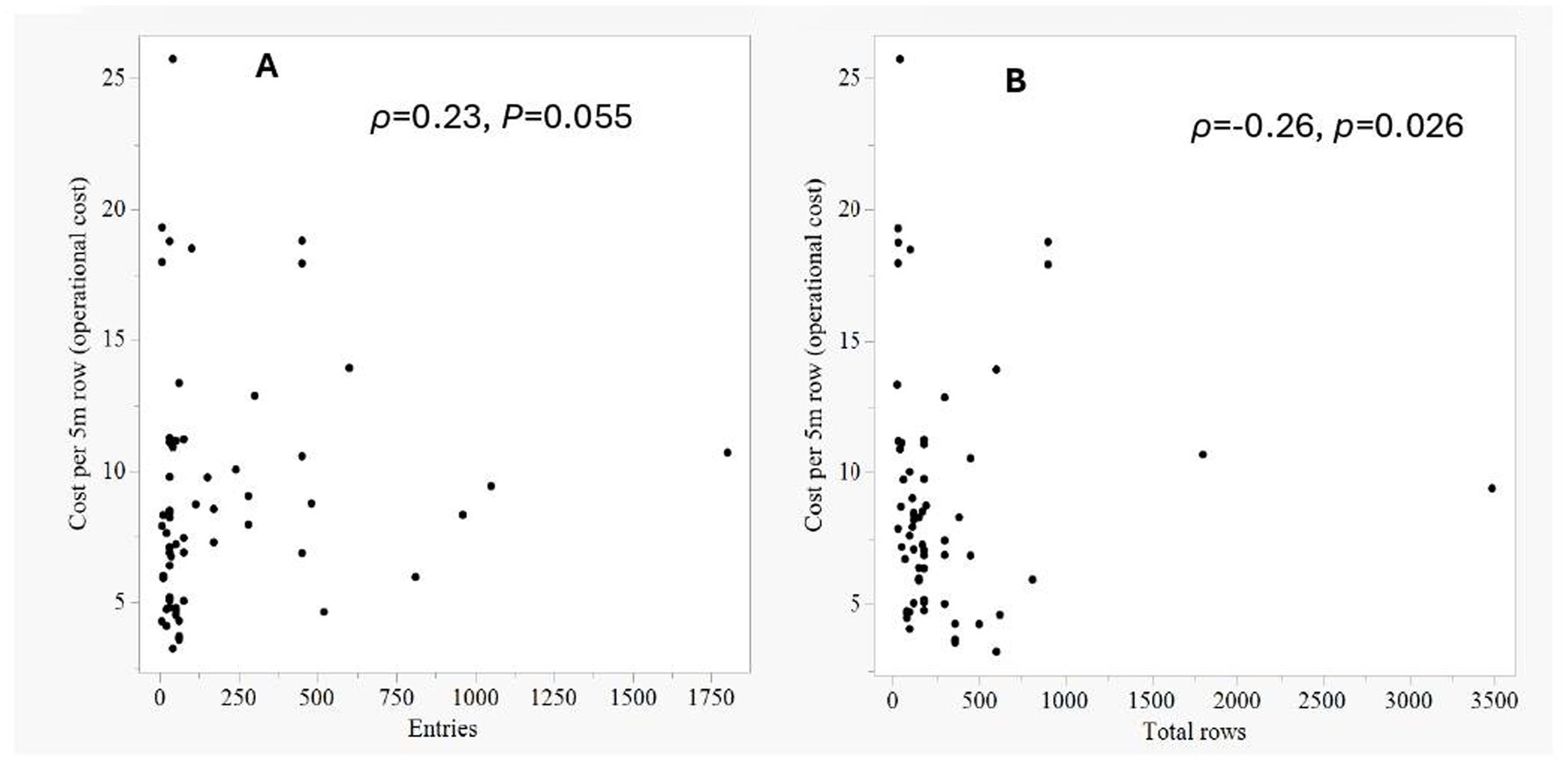
Figure 5. Relationship between the cost of breeding operations per 5-meter row and the number of entries (A) or rows (B) across the stages in a breeding pipeline for eastern and southern African national maize programs.
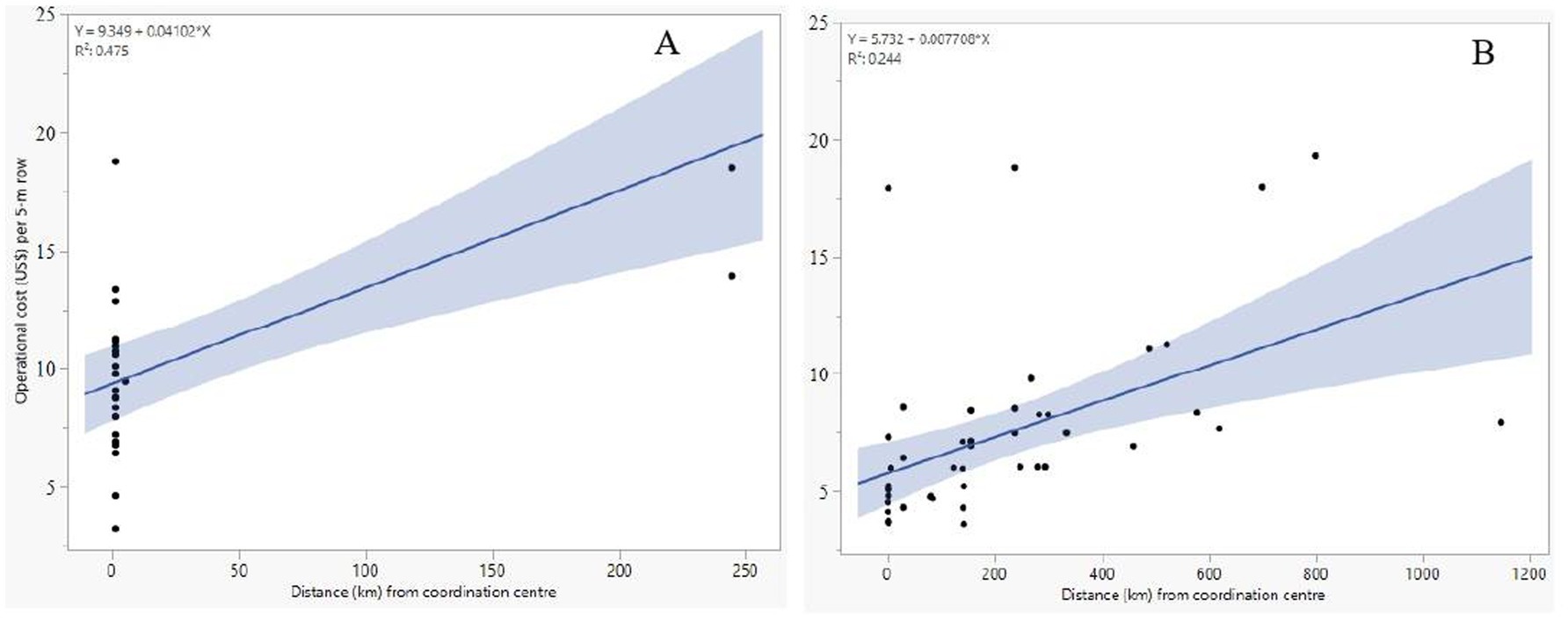
Figure 6. Relationship between the operational cost of a 5-m row and the distance from the coordination center. (A) Germplasm Development and (B) Germplasm testing stages.
4 Discussion
The product profile-based breeding strategy focuses on developing superior varieties to replace older ones that still dominate a particular market (Williamson and Leonelli, 2023). Hybrid maize breeding uses genetically diverse parents with good combining abilities to develop varieties with superior traits relative to their parents (Duvick, 1997b). The genetically diverse parents are developed through SSD, pedigree inbred line development or the DH technology and assigned to heterotic groups based on their combining abilities (Menkir et al., 2004; Fan et al., 2009). Hybrids are derived from a cross between inbred lines of distinct heterotic groups, which show the maximum expression of the trait of interest (e.g., grain yield), and the performance of the resultant hybrid is confirmed by crossing with a tester (Duvick, 1997a). Selections are made on inbred lines for their suitability for male or female parent traits and on hybrid performance from crosses with tester inbred lines (Singh et al., 2021). Thus, maize breeding programs aiming at hybrid development must allocate resources to simultaneously improve populations from different heterotic groups for line and tester development to attain superior hybrid varieties (Goldman, 1997; Lee and Tollenaar, 2007).
The three breeding programs adopted the product profile-based breeding method and had varying levels of adoption for the DH technology in line development. The product profile breeding strategy enables the programs to utilize resources to integrate and select the traits preferred by the target market (farmers, consumers or processors) (Matova et al., 2023). The transition from targeting a specific individual trait to the overall desirable traits of a variety or the product profile signals a major step in the modernization of maize breeding. It is also likely to lead to a rapid varietal release for the target market segments in ESA. The rapid development of new varieties to replace the old and obsolete ones is a strategy for enhancing resilience against climate change-related emerging threats to crop production (Chivasa et al., 2022). The transition toward modern breeding methods was also evidenced by allocating 18–43% germplasm development funds in DH line development. The adoption of DH is another milestone most African maize breeding programs have in the past, which relied on conventional breeding methods due to a lack of resources for the modernization of pipeline operations (Bada-Apraku, 2017). With the observed output cost difference where DH was 7–47 times cheaper than the pedigree inbred line development method, the programs can invest in the latter for high throughput inbred line development if the germplasm has a high haploid induction rate (Andorf et al., 2019; Chaikam et al., 2019; Kaur et al., 2023).
The resources allocated for a maize breeding pipeline will translate into elite inbred lines, hybrids, or synthetic varieties. Here, the NARO program had an operational cost almost twice that of DR$SS and nearly three times that of ZARI. NARO released five hybrids, DR&SS released one, and ZARI released two hybrid varieties. If the number of released hybrids were the only output per breeding cycle, then each variety released for the medium maturity product profile of NARO would be valued at $17,132, the DR&SS variety would be valued at $48,902 while that of ZARI would be $14,750. However, it should be noted that the inbred lines developed by each program are major outputs for supporting future breeding efforts. NARO led in developing inbred lines 1,285, followed by ZARI with 450 and DR&SS with 175 inbred lines derived from the combined methods. The programs must prioritize how to apply the resources, depending on the available germplasm diversity and funding, to meet the product profile and the institution’s immediate and long-term goals. A narrow gene pool of the maize germplasm could lead to wastage of resources in germplasm development and evaluation while ending up with very few varieties that meet the market preferences (Govindaraj et al., 2015). There is a need for the programs to conduct pre-breeding analysis (genotyping and phenotypic characterization) to ensure that the characteristics of the target product profiles are reflected within the diverse germplasm collections available for the current and future breeding needs (Govindaraj et al., 2015; Aci et al., 2018).
Adopting robust and cost-effective experimental designs is a key strategy in ensuring that the components of the breeding schemes are properly implemented to enhance genetic gains. The design of experiments is usually led by the breeder and with the support of a biometrician. Additionally, publicly available resources, such as the Enterprise Breeding System (EBS), can help in planning, designing, data management and analysis for better operations of resource-constrained breeding programs (Dipta et al., 2023). Here, programs reported high costs for trial design and analysis by scientists, and this problem could be resolved if the program adopts EBS and other similar available resources to bring down the cost. Adopting EBS would enable the breeding team to establish standard operating procedures to enhance the optimum utilization of resources. While working on the design of experiments, there is a need to develop preconceived breeding metrices to guide the program on the potential costs of implementing the design. The findings of the current analysis showed a significant negative correlation between the number of 5-m rows and the cost per row and a strong association between the distance traveled from the coordination center to the location where a nursery or a trial is located are a useful guide as to where to implement what volume of activity in a nursery or a trial. More rows reduce the cost per row because some costs are spread equally over the rows, e.g., logistical costs. There should be a clear thought about the cost implications of the mating design (or crosses), how many entries, and the number of trial sites, replications, and rows amenable for a given activity at each stage. The design should consider that having many entries in a trial reduces the replication ability. Yet, replication is essential in reducing the effects of the genotype and environment interactions (GxE) and, hence, phenotyping accuracy (Cullis et al., 2020). While the statistical power is enhanced by replication in a site, the number of sites should be a good representation of the targeted evaluation conditions to give reliable phenotypes for the traits of interest (Cullis et al., 2020).
Relatively high costs of travel, per-diem and accommodation when trials and nurseries were conducted off-station. Conducting nurseries away from the main research station brings huge costs due to travel and logistics. This is unless the implementors are in the same location as the nurseries. However, the trials can be located off-station because of the importance of evaluating the candidate varieties in multiple environments where they would eventually be deployed (Chimonyo et al., 2014). Multilocational trials enable the breeding programs to select traits based on performance under diverse environments, and this information is useful in identifying the most stable and putative resilient genotypes across the environments (Kulkarni et al., 2022; Badu-Apraku et al., 2023). While multilocational trials are unavoidable, breeding programs could establish strategies to reduce the costs associated with implementing them. A short-term solution would be to hire and train local research assistants or use resident agricultural research officers to collect data. However, a more strategic approach would be to create and use mega-environments, which are identified using geospatial analysis and envirotyping techniques (Menkir et al., 2000; Yue et al., 2022). To enhance throughput phenotyping while avoiding non-representative environments, programs can use locations with good multi-year genotypic correlations for the traits of interest (Badu-Apraku et al., 2017; Yue et al., 2022). High throughput phenotyping methods, which involve sparse testing to distribute the favorable alleles of the tested genotypes across environments, could enhance cost-effectiveness in germplasm evaluation (Atanda et al., 2022). Where feasible, data collection using digital technologies such as drones and satellite imaging reduces the cost of travel and hence reduces the labor and logistical costs for off-station germplasm evaluation (Dipta et al., 2023; Gano et al., 2024).
The costs of phenotyping for plant architecture-related traits, flowering time, and disease severity/incidence scores were relatively high across the breeding programs. Phenotyping is a resource-intensive task which a breeding program cannot prevent. The plant phenotype can generally be grouped into qualitative or quantitative data, with the former being highly heritable and easy to analyze, while the latter is complex and highly influenced by the GxE interactions (Balduzzi et al., 2017). For example, the flowering time or days to maturity would be similar within agroecology, and it is expected to increase by 4 days for every 100 m increase in altitude (Xue-jun et al., 2013). On the other hand, the incidence and/or severity of maize diseases may be affected by multiple factors such as the existence of favorable environmental conditions, time (growth stage of the crop) of infection, previous disease history, and maize genetics (Munkvold and White, 2016). Breeding programs can strategically plan where each data type must be collected. Due to the complex nature, the quantitative traits data should be collected across representative environments, while the highly heritable traits data can be collected in a few environments. Adopting such a strategy would significantly bring down the labor costs for phenotyping. Additionally, breeding programs can adopt digital data collection methods while reducing the efforts in traditional phenotyping, which involves human visual assessments or measurements, is time-consuming, requires extensive training to enhance accuracy and may limit the ability to capture large datasets for large populations (Xiao et al., 2022). A precaution should be taken that the adoption of a new technology requires some initial capital investment and may require qualified personnel to calibrate the equipment (Hershberger et al., 2022).
The labor for hand pollination was relatively high in the nurseries. This labor is essential when making initial crosses (new starts), generation advance through selfing or backcrossing, generation of pure inbred lines or during test crossing (MacRobert et al., 2014). The conventional methods of implementing any of these activities may involve using special bags or envelopes to pool the pollen from the tassel for targeted pollination and to protect the silks from unwanted pollen. In some cases, detasseling may be done to prevent unwanted pollen formation in female plants. Establishing isolation crossing blocks is another way to overcome the potential of pollen admixture and unwanted gene influx events (MacRobert et al., 2014). Another most recent approach is the utilization of non-pollen-producing inbred lines as female parents—a strategy named Seed Production Technology for Africa and whose implementation in ESA is spearheaded by the CGIAR partners (Voss et al., 2023). Whichever approaches, there is a cost implication, and the breeding program must establish a strategy to reduce the costs of purchasing the bags, envelopes or even the time consumed by the pollination operation. Suppose the pollination task is being implemented by skilled personnel (e.g., skilled casuals or temporary staff, as is common in most programs). In that case, there is a need to ensure that they are well-trained to achieve optimal outputs. Additionally, the breeding program may outsource the operation to an experienced company at an affordable fee, and this may bring down the labor without a need to incur costs on the training of the casual or temporary staff. The high costs of purchasing some consumables for pollination could be reduced by buying in bulk through regional international partners such as CIMMYT or IITA.
The three costed programs implemented third-party trials for the medium maturity product profile at different scales. Conducting trials for the partners is a service that is likely to strengthen the relationships within the regional crop networks and generate income for the service provider. The established network partnerships would be valuable in optimizing the operations for the NARES breeding pipelines through access to germplasm, modern tools and innovations (Krishna et al., 2023). Based on the costing analysis presented here, the cost of running the activities of each stage was determined. Overall, the minimum operational cost for the nurseries was $9.35 per row. In contrast, the cost for running trials was found to be $5.73 per row, and the cost would increase by $1 for every 130 km of travel from the coordination center to a trial location. This information is valuable and can enhance accurate communication with partner third-party programs on the cost of implementing certain breeding stages’ activities. The cost of breeding programs provides reliable figures for use in budgets and tracking of expenditures. These figures are useful for communicating with the administrators overseeing the implementation of programs’ budgets within an institution. With accurate information, the institution can get a good reputation for reliability and accountability, which eases resource mobilization, finance planning, reporting, and management (Edun, 2000). Accuracy and accountability in financial reporting could also boost donors’ or directors’ trust and increase the chances of getting financial support for the projects in the institution (Edun, 2000).
5 Conclusions and recommendations
Plant breeding should be considered an enterprise with clear production pipelines that yield superior varieties to those already in contemporary farming systems. The resources applied in each stage of the variety production pipeline must be accounted for through a standardized costing procedure acceptable to those funding the breeding efforts. Furthermore, the time taken to release a variety could be adjusted by adopting cost-effective breeding methods. Farmers and consumers want the best varieties in the market at any given time. The breeding programs have no excuse for being overwhelmed by the effects of climate change, which can render the old varieties inferior. Still, they must seek ways of enhancing cost-effectiveness in enhancing genetic gains. Hence, costing using the UQ-BPCT enables administrators, donors, and governments to make data-driven decisions, optimize resources efficiently, and drive agricultural development.
Analysis of the costs across the breeding stages of the three African maize breeding pipelines shows significant variations in the operational costs of the stages and even for the entire pipelines. One interesting finding is that all the pipelines have adopted the doubled haploid technology to enhance the rapid development of elite lines. Deployment of DH means that the programs will take fewer years to develop hybrids and synthetic varieties than conventional methods. Any approaches toward modernization of the breeding methods have humongous contributions to the availability of superior varieties for the African populations. Thus, more efforts should involve creating awareness about cost-effective methods for running breeding programs. Through this analysis, some key high costs were identified. While some may have a direct solution by substitution of items or training of personnel, some must need further support from the local authorities (e.g., cost of guarding against thefts or destruction by wildlife).
To enhance genetic gain per dollar invested, programs should adopt strategies that reduce costs while increasing the number of cycles in a year (Pandey and Rajatasereekul, 1999). Some cost reduction strategies were mentioned in the discussion section, and additional program-specific recommendations are in Table 3. Furthermore, the programs could adopt cost-effective operations measures such as genotyping using the appropriate quality check molecular markers (to confirm the parentage or successful introgression of the target genomic region after a cross) in early breeding stages to increase chances of working with the true genotype, and hence reducing the subsequent costs of phenotyping and selection. Incorporation of marker-assisted selection and adoption of DH may be a way to reduce the time required for inbred line development, which significantly reduces the total cost of varietal release (Xu et al., 2017; Chaikam et al., 2019). The costs of genotyping may be less than the subsequent downstream phenotyping costs when working with populations where some crosses were unsuccessful. If feasible, genomic selection could be adopted to fast-track germplasm improvement, which can be very effective if combined with speed breeding (Watson et al., 2018; Kabade et al., 2024). Furthermore, rapid germplasm evaluation techniques such as sparse testing enhance the development of resilient genotypes by maximizing the test environments for the alleles within the tested panel and hence shortening the cycles for the process of identifying and releasing new varieties (Chaikam et al., 2019; Cobb et al., 2019; Atanda et al., 2022; Swami et al., 2023). These technologies are likely to speed up the development and release of varieties while bringing down the costs of conducting activities (Wanga et al., 2021).
Farmers are the ultimate beneficiaries of the breeding programs by acquiring improved varieties. Costing could enhance farmers’ acquisition rate of improved crop varieties by providing a guiding platform for which the breeding programs can fast-track the genetic gains and the associated costs. With rapid breeding, more improved seed varieties become available and affordable to small-scale farmers, and this becomes a motivation for farmers’ engagement in agribusiness (Awotide et al., 2016). The motivated farmers would be more engaged in expanding crop production, and this would translate into increased food security, better incomes and livelihoods for the growers and consumers (Awotide et al., 2016; Ng’Endo et al., 2022; Offornedo et al., 2022).
Data availability statement
The original contributions presented in the study are included in the article/Supplementary material, further inquiries can be directed to the corresponding authors.
Author contributions
BD: Conceptualization, Data curation, Formal analysis, Funding acquisition, Investigation, Methodology, Project administration, Resources, Supervision, Validation, Visualization, Writing – original draft, Writing – review & editing. SKM: Conceptualization, Data curation, Formal analysis, Methodology, Visualization, Writing – original draft, Writing – review & editing. OO: Conceptualization, Data curation, Formal analysis, Investigation, Methodology, Visualization, Writing – original draft, Writing – review & editing. SM: Data curation, Formal analysis, Visualization, Writing – original draft, Writing – review & editing. DM: Writing – original draft, Writing – review & editing. LS: Conceptualization, Data curation, Formal analysis, Funding acquisition, Investigation, Methodology, Project administration, Resources, Supervision, Validation, Visualization, Writing – original draft, Writing – review & editing. KM: Conceptualization, Data curation, Funding acquisition, Investigation, Methodology, Project administration, Resources, Supervision, Validation, Visualization, Writing – original draft, Writing – review & editing. RM: Formal analysis, Funding acquisition, Investigation, Methodology, Project administration, Resources, Supervision, Validation, Visualization, Writing – original draft, Writing – review & editing, Conceptualization, Data curation. DC: Conceptualization, Data curation, Formal analysis, Funding acquisition, Investigation, Methodology, Project administration, Resources, Supervision, Validation, Writing – original draft, Writing – review & editing. GA: Conceptualization, Data curation, Formal analysis, Funding acquisition, Investigation, Methodology, Project administration, Resources, Supervision, Validation, Visualization, Writing – original draft, Writing – review & editing. DK: Conceptualization, Data curation, Formal analysis, Funding acquisition, Investigation, Methodology, Project administration, Resources, Supervision, Validation, Visualization, Writing – original draft, Writing – review & editing. LM: Conceptualization, Data curation, Formal analysis, Funding acquisition, Investigation, Methodology, Project administration, Resources, Supervision, Validation, Visualization, Writing – original draft, Writing – review & editing.
Funding
The author(s) declare that financial support was received for the research and/or publication of this article. The breeding activities for the NARO program were mainly funded by the government of Uganda. Similarly, the breeding activities for ZARI and DR&SS were funded by the governments of Zambia and Zimbabwe, respectively. The three programs got supplemental funding from bilateral collaborations. The support for costing of breeding operations and the development of the article was provided by Bill and Melinda Gates Foundation grant number INV-003439.
Acknowledgments
The support provided by the human resource, procurement, and finance departments of ZARI, NARO, and DR&SS is highly appreciated. The breeding activities across the three programs are mainly funded by the Ministry of Agriculture, with some additional funding from other donors.
Conflict of interest
The authors declare that the research was conducted in the absence of any commercial or financial relationships that could be construed as a potential conflict of interest.
Generative AI statement
The authors declare that no Gen AI was used in the creation of this manuscript.
Publisher’s note
All claims expressed in this article are solely those of the authors and do not necessarily represent those of their affiliated organizations, or those of the publisher, the editors and the reviewers. Any product that may be evaluated in this article, or claim that may be made by its manufacturer, is not guaranteed or endorsed by the publisher.
Supplementary material
The Supplementary material for this article can be found online at: https://www.frontiersin.org/articles/10.3389/fsufs.2025.1545600/full#supplementary-material
Footnotes
References
Acevedo, M., Pixley, K., Zinyengere, N., Meng, S., Tufan, H., Cichy, K., et al. (2020). A scoping review of adoption of climate-resilient crops by small-scale producers in low- and middle-income countries. Nat. Plants 6, 1231–1241. doi: 10.1038/S41477-020-00783-Z
Aci, M. M., Lupini, A., Mauceri, A., Morsli, A., Khelifi, L., and Sunseri, F. (2018). Genetic variation and structure of maize populations from Saoura and Gourara oasis in Algerian Sahara. BMC Genet. 19:51. doi: 10.1186/S12863-018-0655-2
Alford, J., and Greve, C. (2017). Strategy in the public and private sectors: similarities, differences and changes. Adm. Sci. 7:35. doi: 10.3390/Admsci7040035
Andorf, C., Beavis, W. D., Hufford, M., Smith, S., Suza, W. P., Wang, K., et al. (2019). Technological advances in maize breeding: past, present and future. Theor. Appl. Genet. 132, 817–849. doi: 10.1007/S00122-019-03306-3
Asea, G., Kwemoi, D. B., Sneller, C., Kasozi, C. L., Das, B., Musundire, L., et al. (2023). Genetic trends for yield and key agronomic traits in pre-commercial and commercial maize varieties between 2008 and 2020 in Ug anda. Front. Plant Sci. 14:1020667. doi: 10.3389/Fpls.2023.1020667
Atanda, S. A., Govindan, V., Singh, R., Robbins, K. R., Crossa, J., and Bentley, A. R. (2022). Sparse testing using genomic prediction improves selection for breeding targets in elite spring wheat. Theor. Appl. Genet. 135, 1939–1950. doi: 10.1007/S00122-022-04085-0
Atlin, G. N., Cairns, J. E., and Das, B. (2017). Rapid breeding and varietal replacement are critical to adaptation of cropping systems in the developing world to climate change. Glob. Food Sec. 12, 31–37. doi: 10.1016/J.Gfs.2017.01.008
Atlin, G. N., and Econopouly, B. F. (2022). Simple deterministic modeling can guide the design of breeding pipelines for self-pollinated crops. Crop Sci. 62, 661–678. doi: 10.1002/Csc2.20684
Awotide, B. A., Karimov, A. A., and Diagne, A. (2016). Agricultural technology adoption, commercialization and smallholder Rice farmers’ welfare in rural Nigeria. Agric. Food Econ. 4:47. doi: 10.1186/S40100-016-0047-8
Bada-Apraku, B. (2017). Key challenges in maize breeding in sub-Saharan Africa. Cambridge Kingdom: Burleigh Dodds Science Publishing.
Badu-Apraku, B., Fakorede, M. A. B., Nelimor, C., Osuman, A. S., Bonkoungou, T. O., Muhyideen, O., et al. (2023). Recent advances in breeding maize for drought, heat and combined heat and drought stress tolerance in sub-Saharan Africa. Cabi Rev. 2023:11. doi: 10.1079/Cabireviews.2023.0011
Badu-Apraku, B., Yallou, C. G., Obeng-Antwi, K., Alidu, H., Talabi, A. O., Annor, B., et al. (2017). Yield gains in extra-early maize cultivars of three breeding eras under multiple environments. Agron. J. 109, 418–431. doi: 10.2134/Agronj2016.10.0566
Balduzzi, M., Binder, B. M., Bucksch, A., Chang, C., Hong, L., Iyer-Pascuzzi, A. S., et al. (2017). Reshaping plant biology: qualitative and quantitative descriptors for plant morphology. Front. Plant Sci. 8:117. doi: 10.3389/Fpls.2017.00117
Barthel, S., Crumley, C., and Svedin, U. (2013). Bio-cultural Refugia—safeguarding diversity of practices for food security and biodiversity. Glob. Environ. Chang. 23, 1142–1152. doi: 10.1016/J.Gloenvcha.2013.05.001
Bishaw, Z., and and Gastel, A. J. G. V. (2009). “Variety release and policy options” in Plant breeding and farmer participation. eds. S. Ceccarelli, E. P. Guimarães, and E. Weltzien (Rome: FAO), 565–587.
Brzozowski, L., Hanson, S., Jannink, J. L., Meints, B., Moore, V., Tufan, H., et al. (2022). Towards equitable public sector plant breeding in The United States. Crop Sci. 62, 2076–2090. doi: 10.1002/Csc2.20800
Cairns, J. E., Chamberlin, J., Rutsaert, P., Voss, R. C., Ndhlela, T., and Magorokosho, C. (2021). Challenges for sustainable maize production of smallholder farmers in sub-Saharan Africa. J. Cereal Sci. 101:103274. doi: 10.1016/J.Jcs.2021.103274
Cairns, J. E., Hellin, J., Sonder, K., Araus, J. L., Macrobert, J. F., Thierfelder, C., et al. (2013). Adapting maize production to climate change in sub-Saharan Africa. Food Secur. 5, 345–360. doi: 10.1007/S12571-013-0256-X
Ceccarelli, S. (2009). “Selection methods part 1: organizational aspects of a plant breeding Programme” in Plant breeding and farmer participation. ed. S. Ceccarelli, E.P. Guimarães & E. Weltzien (Rome: FAO), 195–222.
Chaikam, V., Molenaar, W., Melchinger, A. E., and Boddupalli, P. M. (2019). Doubled haploid technology for line development in maize: technical advances and prospects. Theor. Appl. Genet. 132, 3227–3243. doi: 10.1007/S00122-019-03433-X
Chimonyo, V. G., Mutengwa, C. S., and Chiduza, C. (2014). Genotype × environment interactions and yield stability of stress-tolerant open-pollinated maize varieties in The eastern Cape Province, South Africa. S. Afr. J. Plant Soil 31, 61–68. doi: 10.1080/02571862.2014.868048
Chivasa, W., Worku, M., Teklewold, A., Setimela, P., Gethi, J., Magorokosho, C., et al. (2022). Maize varietal replacement in eastern and southern Africa: bottlenecks, drivers and strategies for improvement. Glob. Food Secur. 32:100589. doi: 10.1016/J.Gfs.2021.100589
Cobb, J. N., Juma, R. U., Biswas, P. S., Arbelaez, J. D., Rutkoski, J., Atlin, G., et al. (2019). Enhancing the rate of genetic gain in public-sector plant breeding programs: lessons from the Breeder’s equation. Theor. Appl. Genet. 132, 627–645. doi: 10.1007/S00122-019-03317-0
Cottet, O. (2021). "Costing of breeding programs: a perspective from industry", in Webinar: Costing for breeding programs. (Available Online: An Online Meeting Organized by Excellence in Breeding of Cgiar Program on 12th May 2021).
Covarrubias-Pazaran, G., Gebeyehu, Z., Gemenet, D., Werner, C., Labroo, M., Sirak, S., et al. (2022). Breeding schemes: what are they, how to formalize them, and how to improve them? Front. Plant Sci. 12:791859. doi: 10.3389/Fpls.2021.791859
Cullis, B. R., Smith, A. B., Cocks, N. A., and Butler, D. G. (2020). The design of early-stage plant breeding trials using genetic relatedness. J. Agric. Biol. Environ. Stat. 25, 553–578. doi: 10.1007/S13253-020-00403-5
De Vries, M. E., Adams, J. R., Eggers, E.-J., Ying, S., Stockem, J. E., Kacheyo, O. C., et al. (2023). Converting hybrid potato breeding science into practice. Plan. Theory 12:230. doi: 10.3390/Plants12020230
Dipta, B., Sood, S., Devi, R., Bhardwaj, V., Mangal, V., Thakur, A. K., et al. (2023). Digitalization of potato breeding program: improving data collection and management. Heliyon 9:E12974. doi: 10.1016/J.Heliyon.2023.E12974
Djalovic, I. L. G., Stojanovic, Z., Djurovic, A., Zivancev, D., Jakšić, S., Jaćimović, S., et al. (2024). Nutritional and chemical quality of maize hybrids from different FAO maturity groups developed and grown in Serbia. Plan. Theory 13:143. doi: 10.3390/Plants13010143
Duvick, D. N. (1997a). “Heterosis: feeding people and protecting natural resources” in The genetics and exploitation of Heterosis in crops. Proceedings of an international symposium. Mexico City. eds. J. G. Coors and P. Shivaji (Mexico City: CIMMYT), 19–29.
Duvick, D. N. (1997b). “Commercial strategies for exploitation of Heterosis” in The genetics and exploitation of Heterosis in crops. Proceedings of an international symposium, Cimmyt, Mexico City, Mexico. eds. J. G. Coors and P. Shivaji (Mexico City: CIMMYT), 295–304.
Edun, A. (2000). The role of evaluation in accountability in donor-funded projects. IDS Bull. 31, 48–52. doi: 10.1111/J.1759-5436.2000.Mp31001007.X
Ekpa, O., Palacios-Rojas, N., Kruseman, G., Fogliano, V., and Linnemann, A. R. (2019). Sub-Saharan African maize-based foods - processing practices, challenges and opportunities. Food Rev. Int. 35, 609–639. doi: 10.1080/87559129.2019.1588290
Fan, X. M., Zhang, Y. M., Yao, W. H., Chen, H. M., Tan, J., Xu, C. X., et al. (2009). Classifying maize inbred lines into heterotic groups using a factorial mating design. Agron. J. 101, 106–112. doi: 10.2134/Agronj2008.0217
Foley, J. A., Ramankutty, N., Brauman, K. A., Cassidy, E. S., Gerber, J. S., Johnston, M., et al. (2011). Solutions for a cultivated planet. Nature 478, 337–342. doi: 10.1038/Nature10452
Gano, B., Bhadra, S., Vilbig, J. M., Ahmed, N., Sagan, V., and Shakoor, N. (2024). Drone-based imaging sensors, techniques, and applications in plant phenotyping for crop breeding: a comprehensive review. Plant Phenome J. 7:20100. doi: 10.1002/Ppj2.20100
Godfray, H. C. J., Beddington, J. R., Crute, I. R., Haddad, L., Lawrence, D., Muir, J. F., et al. (2010). Food security: The challenge of feeding 9 billion people. Science 327, 812–818. doi: 10.1126/Science.1185383
Goldman, I. L. (1997). “Inbreeding and outbreeding in The development of a modern Heterosis concept” in The genetics and exploitation of Heterosis in crops. Proceedings of an international symposium, Mexico (Mexico City: CIMMYT), 7–18.
Govindaraj, M., Vetriventhan, M., and Srinivasan, M. (2015). Importance of genetic diversity assessment in crop plants and its recent advances: an overview of its analytical perspectives. Genet. Res. Int. 2015, 1–14. doi: 10.1155/2015/431487
Hershberger, J., Mbanjo, E. G. N., Peteti, P., Ikpan, A., Ogunpaimo, K., Nafiu, K., et al. (2022). Low-cost, h andheld near-infrared spectroscopy for root dry matter content prediction in cassava. Plant Phenome J. 5:20040. doi: 10.1002/Ppj2.20040
Kabade, P. G., Dixit, S., Singh, U. M., Alam, S., Bhosale, S., Kumar, S., et al. (2024). Speedflower: a comprehensive speed breeding protocol for Indica and japonica rice. Plant Biotechnol. J. 22, 1051–1066. doi: 10.1111/Pbi.14245
Kaur, H., Kyum, M., S andhu, S., Singh, G., and Sharma, P. (2023). Protocol optimization and assessment of genotypic response for inbred line development through doubled haploid production in maize. BMC Plant Biol. 23:4228. doi: 10.1186/S12870-023-04228-5
Krishna, V. V., Lantican, M. A., Prasanna, B. M., Pixley, K., Abdoulaye, T., Menkir, A., et al. (2023). Impact of Cgiar maize germplasm in sub-Saharan Africa. Field Crop Res. 290:108756. doi: 10.1016/J.Fcr.2022.108756
Kulkarni, A. P., Kuchanur, P. H., Satihal, D. G., Zaidi, P. H., and Rahut, D. B. (2022). Stress-resilient maize hybrid adoption factors and impact: evidence from rain-fed Agroecologies of Karnataka state, India. Front. Sustain. Food Syst. 6:909588. doi: 10.3389/Fsufs.2022.909588
Lammerts Van Bueren, E. T., Struik, P. C., Van Eekeren, N., and Nuijten, E. (2018). Towards resilience through systems-based plant breeding. A review. Agron. Sustain. Dev. 38:42. doi: 10.1007/S13593-018-0522-6
Lee, E. A., and Tollenaar, M. (2007). Physiological basis of successful breeding strategies for maize grain yield. Crop Sci. 47, S-202–S-215. doi: 10.2135/Cropsci2007.04.0010ipbs
Li, C. (2020). Breeding crops by design for future agriculture. J. Zhejiang Univ. Sci. 21, 423–425. doi: 10.1631/Jzus.B2010001
Ligeyo, D. O., Saina, E., Awalla, B. J., Sneller, C., Chivasa, W., Musundire, L., et al. (2024). Genetic trends in The Kenya Highl and maize breeding program between 1999 and 2020. Front. Plant Sci. 15:1416538. doi: 10.3389/Fpls.2024.1416538
Mace, E. (2021). "Costing breeding pipelines using the UQ breeding program costing tool,” in Webinar: Costing for Breeding Programs. (Available Online: An Online Meeting Organized by Excellence in Breeding - a Cgiar Program on 12th May 2021).
Macrobert, J. F., Setimela, P. S., Gethi, J., and Worku, M. (2014). Maize hybrid seed production manual. Mexico: Cimmyt.
Mangione, D., Senni, S., Puccioni, M., Gr ando, S., and Ceccarelli, S. (2006). The cost of participatory barley breeding. Euphytica 150, 289–306. doi: 10.1007/S10681-006-0226-X
Matova, P. M., Kamut ando, C. N., Warburton, M. L., Williams, W. P., Magorokosho, C., Shimelis, H., et al. (2023). New techniques for breeding maize (Zea Mays) varieties with fall armyworm resistance and market-preferred traits for sub-Saharan Africa. Plant Breed. 142, 1–11. doi: 10.1111/Pbr.13063
Mazibuko, P., Mutengwa, C., Magorokosho, C., Kutywayo, D., and Kamut ando, C. N. (2024). Genetic gains of grain yield among the maize cultivars released over a century from The National Breeding Program of Zimbabwe. Agronomy 14:246. doi: 10.3390/Agronomy14020246
Mbithi, L. M., and Huylenbroeck, V. (2000). A comparison of maize production in Kenya before and during liberalization period. Tropicultura 18, 181–186.
McIntire, J., and Dobermann, A. (2023). The CGIAR needs a revolution. Global Food Security 38:100712. doi: 10.1016/j.gfs.2023.100712
Menkir, A., Kling, J., Jagtap, S. S., and Aliu, B. A. (2000). Gis based classification of maize testing locations in West and Central Africa. Maydica 45, 143–150.
Menkir, A., Melake-Berhan, A., The, C., Ingelbrecht, I., and Adepoju, A. (2004). Grouping of tropical mid-altitude maize inbred lines on The basis of yield data and molecular markers. Theor. Appl. Genet. 108, 1582–1590. doi: 10.1007/S00122-004-1585-0
Mukaro, R., Chaingeni, D., Sneller, C., Cairns, J. E., Musundire, L., Prasanna, B. M., et al. (2024). Genetic trends in The Zimbabwe’s National Maize Breeding Program over two Decades. Front. Plant Sci. 15:1391926. doi: 10.3389/Fpls.2024.1391926
Munkvold, G. P., and White, D. G. (2016). Compendium of corn diseases. St. Paul, MN: Minnesota Amer Phytopathological Society.
Ng’Endo, M., Kinyua, M., Chebet, L., Mutiga, S., Ndungu, J., Nyongesa, O., et al. (2022). The importance of market signals in crop varietal development: lessons from Komboka Rice variety. CABI Agric. Biosci. 3:122. doi: 10.1186/S43170-022-00122-6
Nuss, E. T., and Tanumihardjo, S. A. (2010). Maize: a paramount staple crop in The context of global nutrition. Compr. Rev. Food Sci. Food Saf. 9, 417–436. doi: 10.1111/J.1541-4337.2010.00117.X
Offornedo, Q., Menkir, A., Babalola, D., and Gedil, M. (2022). Developing and deploying an efficient genotyping workflow for accelerating maize improvement in developing countries. Gates Open Res. 6:3. doi: 10.12688/Gatesopenres.13338.3
Pandey, S., and Rajatasereekul, S. (1999). Economics of plant breeding: The value of shorter breeding cycles for Rice in Northeast Thail and. Field Crop Res. 64, 187–197. doi: 10.1016/S0378-4290(99)00059-3
Ray, D. K., Mueller, N. D., West, P. C., and Foley, J. A. (2013). Yield trends are insufficient to double global crop production by 2050. PLoS One 8:E66428. doi: 10.1371/Journal.Pone.0066428
Singh, D. P., Singh, A. K., and Singh, A. (2021). “Hybrid Varieties” in Plant breeding and cultivar development. eds. D. P. Singh, A. K. Singh, and A. Singh (New York, NY: Academic Press, Elsevier), 357–377.
Swami, P., Deswal, K., Rana, V., Yadav, D., and Munjal, R. (2023). “Speed breeding—a powerful tool to breed more crops in less time accelerating crop research” in Abiotic stresses in wheat: Unfolding the Challenges. eds. A. P andey, M. Hamurcu, O. P. Gupta, and S. Gezgin (New York, NY: Academic Press), 33–49.
Voss, R. C., Cairns, J. E., Olsen, M., Muteti, F. N., Magambo Kanyenji, G., Hamadziripi, E., et al. (2023). Innovative approaches to integrating gender into conventional maize breeding: lessons from the seed production technology for Africa project. Front. Sociol. 8:1254595. doi: 10.3389/Fsoc.2023.1254595
Wanga, M. A., Shimelis, H., Mashilo, J., and Laing, M. D. (2021). Opportunities and challenges of speed breeding: a review. Plant Breed. 140, 185–194. doi: 10.1111/Pbr.12909
Wannemuehler, S. D., Luby, J. J., Yue, C., Bedford, D. S., Gallardo, R. K., and Mccracken, V. A. (2019). A cost–benefit analysis of Dna informed apple breeding. HortScience 54, 1998–2004. doi: 10.21273/Hortsci14173-19
Wannemuehler, S. D., Yue, C., Shane, W. W., Gallardo, R. K., and Mccracken, V. (2020). Estimated implementation costs of DNA-informed breeding in a peach breeding program. HortTechnology 30, 356–364. doi: 10.21273/Horttech04499-19
Watson, A., Ghosh, S., Williams, M. J., Cuddy, W. S., Simmonds, J., Rey, M.-D., et al. (2018). Speed breeding is a powerful tool to accelerate crop research and breeding. Nat. Plants 4, 23–29. doi: 10.1038/S41477-017-0083-8
Williamson, H. F., and Leonelli, S. (2023). “Cultivating responsible plant breeding strategies: conceptual and normative commitments in data-intensive agriculture” in Towards responsible plant data linkage: Data challenges for agricultural research and development. eds. H. F. Williamson and S. Leonelli (Cham: Springer Cham), 301–317.
Xiao, Q., Bai, X., Zhang, C., and He, Y. (2022). Advanced high-throughput plant phenotyping techniques for genome-wide association studies: a review. J. Adv. Res. 35, 215–230. doi: 10.1016/J.Jare.2021.05.002
Xu, Y., Li, P., Zou, C., Lu, Y., Xie, C., Zhang, X., et al. (2017). Enhancing genetic gain in The era of molecular breeding. J. Exp. Bot. 68, 2641–2666. doi: 10.1093/Jxb/Erx135
Xue-Jun, C., Guang-Cai, C., Qun, S., Dong-Bin, W., Jing, C., Ya-Xiong, Y. U., et al. (2013). Altitude effects on maize growth period and quality traits. Acta Ecol. Sin. 33, 233–236. doi: 10.1016/J.Chnaes.2013.05.002
Keywords: costing of breeding operations, breeding program costing tool, resource allocation, optimization of breeding operations, maize breeding
Citation: Das B, Mutiga SK, Odiyo O, Madahana S, Milic D, Sinyinda L, Mwansa K, Mukaro R, Chaingeni D, Asea G, Kwemoi DB and Musundire L (2025) Costing of the breeding operations for the national maize programs in Eastern and Southern Africa. Front. Sustain. Food Syst. 9:1545600. doi: 10.3389/fsufs.2025.1545600
Edited by:
Miroslav Zoric, LoginEKO Research and Development Center, SerbiaReviewed by:
Tatenda Musimwa, Iowa State University, United StatesBojan Mitrovic, Maize Research Institute Zemun Polje, Serbia
Copyright © 2025 Das, Mutiga, Odiyo, Madahana, Milic, Sinyinda, Mwansa, Mukaro, Chaingeni, Asea, Kwemoi and Musundire. This is an open-access article distributed under the terms of the Creative Commons Attribution License (CC BY). The use, distribution or reproduction in other forums is permitted, provided the original author(s) and the copyright owner(s) are credited and that the original publication in this journal is cited, in accordance with accepted academic practice. No use, distribution or reproduction is permitted which does not comply with these terms.
*Correspondence: Samuel K. Mutiga, cy5tdXRpZ2FAY2dpYXIub3Jn; Lennin Musundire, bC5tdXN1bmRpcmVAY2dpYXIub3Jn
†ORCID: Biswanath Das, https://orcid.org/0000-0003-2928-5030
Samuel K. Mutiga, https://orcid.org/0000-0001-9919-6231
Olivia Odiyo, https://orcid.org/0009-0003-7721-164X
Sammy Madahana, https://orcid.org/0000-0001-8754-9976
Dragan Milic, https://orcid.org/0000-0002-7449-4539
Ronica Mukaro, http://orcid.org/0009-0002-0840-0773
Davison Chaingeni, https://orcid.org/0009-0008-5851-430X
Godfrey Asea, http://orcid.org/0000-0003-1441-2199
Lennin Musundire, https://orcid.org/0000-0002-6721-3735