- 1The Department of Respiratory Diseases and Critic Care Unit, Shenzhen Institute of Respiratory Disease, Shenzhen Key Laboratory of Respiratory Disease, The Second Clinical Medical College of Jinan University (Shenzhen People’s Hospital), Post-Doctoral Scientific Research Station of Basic Medicine, Jinan University, Guangzhou, China
- 2Guangdong Provincial Key Laboratory of Brain Connectome and Behavior, CAS Key Laboratory of Brain Connectom aend Manipulation, The Brain Cogntion and Brain Disease Institute (BCBDI), Shenzhen Institutes of Adavnced Technology, Chinese Academy of Sciences, Shenzhen-Hong Kong Institute of Brain Science-Shnezhen Fundamental Research Institutions, Shenzhen, China
- 3Department of Microbiology and Immunology, Institute of Geriatric Immunology, School of Medicine, Jinan University, Guangzhou, China
Air pollution is one of the major risk factors for lung disease. Microplastics are a ubiquitous environmental pollutant, both indoors and in outdoor air. Microplastics have also been found in human lung tissue and sputum. However, there is a paucity of information on the effects and mechanisms of microplastics on lung disease. In this mini-review, we reviewed the possible mechanisms by which air microplastics’ exposure affects lung disease and, at the same time, pointed out the limitations of current studies.
Introduction
Microplastics are air pollutants that have attracted intense attention in recent years. Microplastics have been detected in the air of large, densely populated cities (e.g., Beijing, Shanghai, London, etc.) (Liu et al., 2019; Li et al., 2020; Wright et al., 2020), and the type and concentration of airborne microplastics are influenced by community lifestyles, anthropogenic activities, and meteorological conditions (Chen et al., 2020; Mbachu et al., 2020). Atmospheric microplastics can settle on the ground or float by wind and air movement. Due to the small size, airborne microplastics can be directly inhaled by humans (Kaya et al., 2018; Chen et al., 2020). Microplastics are present in indoor and outdoor air, although the concentration of microplastics in the indoor environment is greater than that in the outdoor environment. Indoor objects, materials, and furnishings produce plastic debris due to abrasion, and microplastics from these sources have a much greater impact on humans than the polymers contained in food and beverages (Rist et al., 2018). Inhalation of plastic fibers and particles, especially by exposed workers, often leads to respiratory discomfort due to inflammatory responses in the airways and interstitium. Even at very low ambient concentrations, the susceptible individuals are at risk of developing lesions (Atis et al., 2005; Prata, 2018).
The deposition of microplastics in the human respiratory system has been demonstrated. The presence of microplastics has been found in the human lung (Amato-Lourenco et al., 2021; Jenner et al., 2022) and in the sputum of patients with respiratory diseases (Huang et al., 2022). A maximum of 565 particles/10 ml and 21 types of microplastics were identified (polyurethane, polyester, chlorinated polyethylene, and alkyd varnish, accounting for 78.36% of the total microplastics) in sputum. Microplastics are inhaled into the respiratory tract and can be excreted with sputum, and personal smoking habits and invasive tracheal examinations have been associated with microplastic exposure (Huang et al., 2022). However, studies on the effects of these microplastics deposited in the lungs, especially in patients with respiratory diseases, are still in the initial stage. Here, we present an overview of the potential effects and possible mechanisms of microplastics on respiratory health (Figure 1) with the aim of providing an awakening to the detriments of microplastics.
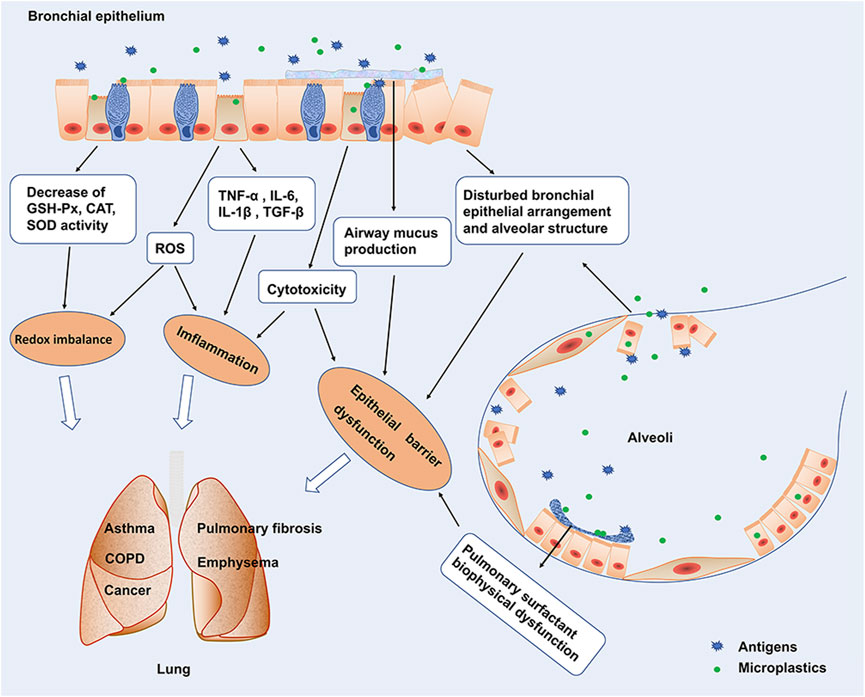
FIGURE 1. Potential effects and possible mechanisms of microplastics on respiratory. Microplastic exposure interferes with the biophysical function of pulmonary surfactants and disrupts alveolar structure and airway barrier function. Exposure of microplastics to lung epithelial cells inhibits epithelial cell proliferation and induces epithelial cell apoptosis. Microplastics stimulates the production of pro-inflammatory cytokines such as TNF-α, IL-6, IL-1β production, and TGF-β production by lung epithelial cells. In addition, exposure to microplastics caused a significant decrease in oxidative stress-related GSH-Px, CAT, and SOD activities, induced ROS production, and Redox imbalance.
Possible mechanisms by which microplastics affect respiratory health
Epithelial barrier dysfunction
On the alveolar surface, there is a complex layer of surface-active substances consisting of proteins and lipids. These pulmonary surfactants form a complex structural fluid-fluid interface and form a stable film in the alveoli, which has the effect of reducing surface tension, preventing alveolar collapse during exhalation, and reducing the energy consumed by respiration. In addition, pulmonary surfactants have important transport properties in the lung. The pulmonary surfactant flows up the terminal airways and reduces the formation of liquid plugs that can obstruct terminal airways at end-expiration (Hermans et al., 2015). Further up the bronchial tree, the mixing of pulmonary surfactant with airway mucus improves the mobility and clearance of mucus by bronchial epithelial cilia. Pulmonary surfactant flowing up the airway tree helps to remove particulates and pathogens inhaled from the alveoli and distal airways (Podgorski and Gradon, 1990). Thus, disruption of surface-active substances reduces lung defenses. Nanoplastics are transferred to the alveolar air-water interface by vesicle fusion and cause biophysical dysfunction of pulmonary surfactant by disrupting its ultrastructure and mobility, leading to collapse of the pulmonary surfactant film (Li L. et al., 2022a). Polystyrene microplastics (PS-NPs) adsorb more phospholipid components of pulmonary surfactant than that of proteins. Polystyrene accelerates the conversion between ascorbic acid and deoxyascorbic acid, generates hydrogen peroxide (HOOH) in the simulated lung fluid, and leads to an increase in hydroxyl radical (-OH) content, which ultimately alters the phase behavior, surface tension, and membrane structure of pulmonary surfactant (Shi et al., 2022). The change in surface tension in turn affects the migration of nanoparticles and the collapse of the surfactant film. Furthermore, in vitro experimental studies revealed that PS-NPs reduce trans-epithelial resistance by depleting tight junction proteins. Expression of cellular matrix metallopeptidase 9 and surface-active protein A were elevated after PS-NPs treatment, suggesting that PS-NPs exposure may reduce lung repair capacity and cause lung injury (Yang et al., 2021). The disruption of epithelial barrier function makes it easier for foreign substances, such as allergens and toxins, to enter the interstitium and bloodstream, which may lead to the development of lung diseases such as asthma and chronic obstructive pulmonary disease (COPD) (Zhao et al., 2020).
Cytotoxicity
Pulmonary epithelial cells are located at the interface between the host and the environment, where they are directly exposed to inhaled air as well as to airborne microplastics. Microplastics are able to internalize into epithelial cells, and the rate of internalization is influenced by physicochemical properties such as particle size and surface charge. Microplastics with small particle size are internalized more rapidly than larger particles. The internalization and intracellular accumulation of microplastics with positive surface charge is greater than that of microplastics with negative surface charge. In vitro experiments showed that internalization of microplastics led to blebbing morphology and activation of apoptotic signals in alveolar epithelial cells under cyclic stretches (Yang et al., 2021). After giving nasal microplastic drops (1–5 μm, 300 µg/20 µl) to mice, microplastics were found in the airways, alveoli, and interstitium, indicating that microplastics can penetrate the alveolar epithelial barrier (Lu et al., 2021). Pathological examination of rats with tracheal injection of microplastics (a maximum concentration of 2 mg/200 μl) showed disruption of alveolar structure and disorganized arrangement of bronchial epithelium (Fan et al., 2022). These studies suggest that microplastic exposure will cause lung injury. Therefore, prolonged exposure to microplastics may lead to the development and progression of lung diseases.
The effect of internalization of microplastics on cell viability is still under discussion. Goodman et al. (2021) reported that treatment with high concentrations (100 μg/ml) of microplastics did not exhibit toxicity to human alveolar A549 cells, with cell survival rates above 93%. However, microplastics inhibit cell proliferation and cause significant changes in cell morphology. However, Yang et al. (2021) evaluated the relationship between polystyrene microplastics and lung injury using two types of human lung epithelial cells (BEAS-2B) and human alveolar epithelial cells (HPAEpiC) and noted that polystyrene microplastics significantly reduced cell viability in a dose-dependent manner (Yang et al., 2021). Pro-apoptotic proteins such as A549DR5, caspase-3, caspase-8, caspase-9, and cytochrome c were significantly upregulated in alveolar epithelial cells after treatment with polystyrene nanomicroplastics, suggesting that PS-NPs can induce apoptosis (Xu et al., 2019). This may be related to the chemical nature of microplastics, surface charge, concentration, exposure time, photoaging of the surface, and whether other toxic substances are adsorbed on the surface. For example, a study by Kecheng et al. opined that fresh Phenol-formaldehyde resin microplastic (PF-MP) treatment had no significant effect on cell viability, but photo-aged PF-MP was able to significantly reduce cell survival (Zhu et al., 2020).
Inflammatory response and redox imbalance
Rather than acting as a simple physical barrier, the airway epithelium detects allergens, microplastics, and other irritants and then helps to organize the subsequent immune response by releasing a large number of secretory signals. Microplastics’ exposure induces epithelial cell inflammation. The pro-inflammatory cytokine TNF-α may have a momentous impact on microplastics-induced airway inflammation. Transtracheal injection of polystyrene microplastics increased the expression of the pro-inflammatory cytokines TNF-α, IL-6, and IL-1β in alveolar lavage fluid in rats (Fan et al., 2022). Lu et al. (2021) observed that microplastic exposure increased the production of TNF-α in BALF of mouse. Expression of inflammatory proteins (TGF-β and TNF-α) in the lung tissue of rats exposed to polystyrene microplastics increased in a concentration-dependent manner (Lim et al., 2021). In in vitro experiments, PS-NPs induced significant increases in pro-inflammatory cytokines such as IL-8, NF-κβ, and TNF-α (Xu et al., 2019). TNF-α is closely associated with dysregulated airway inflammation in asthma. Whereas TNF-α is involved in the interaction between mast cells and smooth muscle cells and might be closely related to the development of airway hyperresponsiveness in asthmatic patients. In addition, TNF-α has the ability to promote neutrophil recruitment, induction of glucocorticoid resistance, myocyte proliferation, and stimulation of fibroblast growth and maturation into myofibroblasts by promoting the expression of TNF-α (Berry et al., 2007). These are all latent mechanisms that are associated with refractory asthma. The above results suggest that microplastics can affect and would be harmful to asthma patients. Moreover, TGF-β is also involved in pulmonary diseases. For instance, TGF-β regulates a variety of cellular processes such as epithelial cell growth inhibition, alveolar epithelial cell differentiation, fibroblast activation, and extracellular matrix organization, which are associated with tissue remodeling in pulmonary fibrosis and emphysema. Upregulation of TGF-β is seen in major lung diseases such as pulmonary fibrosis, emphysema, bronchial asthma, and lung cancer (Saito et al., 2018). Collectively, overexpression of TGF-β induced by microplastics may engage in the course of these respiratory diseases.
Redox imbalance may be an additional mechanism by which microplastics induce effects on chronic respiratory diseases. Oxidative stress caused by redox imbalance is one of the mechanisms contributing to the pathogenesis of several lung diseases such as asthma, COPD, and acute respiratory distress syndrome (ARDS) (Hecker, 2018). Exposure to microplastics isolated from the environment showed a depletion of antioxidants in simulated lung fluid (Abbasi et al., 2019), and microplastics’ exposure also result in increased ROS production in mouse lung tissue. Inflammation induced by ROS is the initial stage leading to tissue damage. What’s more, microplastic exposure alters the oxidative status and antioxidant capacity of bronchial epithelial cells. GSH-Px, CAT, and SOD activities associated with oxidative stress are significantly reduced by microplastics in a dose-dependent manner, resulting in excessive oxidative stress (Li Z. et al., 2022b). Both inflammation and redox imbalance are closely related to pathophysiological processes; therefore, the potential threat of inhaled microplastics should be given adequate attention.
Synergistic effects with allergens
As aforementioned, microplastics increase the permeability of allergens by disrupting alveolar barrier function. Our previous study found that co-exposure to microplastics (300 µg in 20 µl saline) and HDM (house dust mite) increased airway mucus secretion and aggravated HDM-induced airway inflammation in asthmatic mice. In addition, co-exposure to microplastics and HDM induced increased expression of MALT1 in lung tissue of mice (Lu et al., 2021). MALT1 is widely expressed in lymphoid, mast, and endothelial cells, acting as a scaffolding protein and as a substrate-specific protease. MALT1 protease has bifacial roles in the allergic disease by mediating IgE-dependent mast cell cytokine production and histamine-induced endothelial permeability (Alfano et al., 2020). This suggests that co-exposure to microplastics and allergens may synergistically exacerbate allergic diseases.
Limitations
In studies investigating the side effects of microplastics, microplastics of different chemical materials, particle sizes, or concentrations have been used. Among them, polystyrene microplastics are the most used. Polystyrene microplastics are indeed one of the major air pollutants; however, they do not exist alone in the real environment. In the air, microplastics are present together with other pollutants and even have organic substances or metal ions adsorbed on their surfaces. Microplastics and these substances cause a synergistic effect in terms of side effects on humans. Therefore, in-depth studies are needed to investigate the effects of mixed exposure to multiple microplastics on respiratory health. Secondly, microplastics can release harmful chemicals in the natural environment or even in the physiological environment; whether it is these chemical hazards or the particles themselves that have greater health side effects has not been reported. Furthermore, except in some occupational settings, the concentration of microplastics in the air is very low, but long-term exposure, and the relationship between such small doses of long-term microplastics exposure and respiratory disease, needs more clinical observational studies and long-period animal model experiments. Finally, it has been found that nano-microplastics section inhaled via airways can be transferred to other organs via blood circulation; what are the effects of these microplastics transferred to other organs on these organs? Whether microplastics deposited in the lungs and other organs can be removed from the body, how they are removed, and how long it takes to do so are all issues that need to be further explored.
In conclusion, although research on the respiratory health effects of microplastics remains scarce, the negative effects that microplastics can have on respiratory health are clearly evident. This review provides an overview of the possible threats and mechanisms of respiratory health effects of airborne microplastics and points out the limitations of current research. Meanwhile, we herein call for more attention to be given to microplastics and the respiratory health threats it poses.
Author contributions
Design of the work: KL, SC, and LW. Literature investigation and selection: KL, DZ, and YF. Drafting the article: KL. Critical revision of the article: LL, GC, SC, and LW. All authors approved the final version of the manuscript.
Funding
This work was financially supported by the Shenzhen Science and Technology Program (JCYJ20210324113006015), Guangdong Basic and Applied Basic Research Foundation (2021A1515110433), and by the China Postdoctoral Science Foundation (2021M701424).
Conflict of interest
The authors declare that the research was conducted in the absence of any commercial or financial relationships that could be construed as a potential conflict of interest.
Publisher’s note
All claims expressed in this article are solely those of the authors and do not necessarily represent those of their affiliated organizations, or those of the publisher, the editors and the reviewers. Any product that may be evaluated in this article, or claim that may be made by its manufacturer, is not guaranteed or endorsed by the publisher.
References
Abbasi, S., Keshavarzi, B., Moore, F., Turner, A., Kelly, F. J., Dominguez, A. O., et al. (2019). Distribution and potential health impacts of microplastics and microrubbers in air and street dusts from Asaluyeh County, Iran. Environ. Pollut. 244, 153–164. doi:10.1016/j.envpol.2018.10.039
Alfano, D. N., Klei, L. R., Klei, H. B., Trotta, M., Gough, P. J., Foley, K. P., et al. (2020). MALT1 protease plays a dual role in the allergic response by acting in both mast cells and endothelial cells. J. Immunol. 204, 2337–2348. doi:10.4049/jimmunol.1900281
Amato-Lourenco, L. F., Carvalho-Oliveira, R., Junior, G. R., Dos Santos Galvao, L., Ando, R. A., and Mauad, T. (2021). Presence of airborne microplastics in human lung tissue. J. Hazard. Mat. 416, 126124. doi:10.1016/j.jhazmat.2021.126124
Atis, S., Tutluoglu, B., Levent, E., Ozturk, C., Tunaci, A., Sahin, K., et al. (2005). The respiratory effects of occupational polypropylene flock exposure. Eur. Respir. J. 25, 110–117. doi:10.1183/09031936.04.00138403
Berry, M., Brightling, C., Pavord, I., and Wardlaw, A. (2007). TNF-alpha in asthma. Curr. Opin. Pharmacol. 7, 279–282. doi:10.1016/j.coph.2007.03.001
Chen, G. L., Feng, Q. Y., and Wang, J. (2020). Mini-review of microplastics in the atmosphere and their risks to humans. Sci. Total Environ. 703, 135504. doi:10.1016/j.scitotenv.2019.135504
Fan, Z., Xiao, T., Luo, H., Chen, D., Lu, K., Shi, W., et al. (2022). A study on the roles of long non-coding RNA and circular RNA in the pulmonary injuries induced by polystyrene microplastics. Environ. Int. 163, 107223. doi:10.1016/j.envint.2022.107223
Goodman, K. E., Hare, J. T., Khamis, Z. I., Hua, T., and Sang, Q. A. (2021). Exposure of human lung cells to polystyrene microplastics significantly retards cell proliferation and triggers morphological changes. Chem. Res. Toxicol. 34, 1069–1081. doi:10.1021/acs.chemrestox.0c00486
Hecker, L. (2018). Mechanisms and consequences of oxidative stress in lung disease: Therapeutic implications for an aging populace. Am. J. Physiol. Lung Cell. Mol. Physiol. 314, L642–L653. doi:10.1152/ajplung.00275.2017
Hermans, E., Bhamla, M. S., Kao, P., Fuller, G. G., and Vermant, J. (2015). Lung surfactants and different contributions to thin film stability. Soft Matter 11, 8048–8057. doi:10.1039/c5sm01603g
Huang, S., Huang, X., Bi, R., Guo, Q., Yu, X., Zeng, Q., et al. (2022). Detection and analysis of microplastics in human sputum. Environ. Sci. Technol. 56, 2476–2486. doi:10.1021/acs.est.1c03859
Jenner, L. C., Rotchell, J. M., Bennett, R. T., Cowen, M., Tentzeris, V., and Sadofsky, L. R. (2022). Detection of microplastics in human lung tissue using μFTIR spectroscopy. Sci. Total Environ. 831, 154907. doi:10.1016/j.scitotenv.2022.154907
Kaya, A. T., Yurtsever, M., and Bayraktar, S. C. (2018). Ubiquitous exposure to microfiber pollution in the air. Eur. Phys. J. Plus 133, 488. doi:10.1140/epjp/i2018-12372-7
Li, L., Xu, Y., Li, S., Zhang, X., Feng, H., Dai, Y., et al. (2022a). Molecular modeling of nanoplastic transformations in alveolar fluid and impacts on the lung surfactant film. J. Hazard. Mat. 427, 127872. doi:10.1016/j.jhazmat.2021.127872
Li, Y., Shao, L., Wang, W., Zhang, M., Feng, X., Li, W., et al. (2020). Airborne fiber particles: Types, size and concentration observed in Beijing. Sci. Total Environ. 705, 135967. doi:10.1016/j.scitotenv.2019.135967
Li, Z., Chang, X., Hu, M., Fang, J. K., Sokolova, I. M., Huang, W., et al. (2022b). Is microplastic an oxidative stressor? Evidence from a meta-analysis on bivalves. J. Hazard. Mat. 423, 127211. doi:10.1016/j.jhazmat.2021.127211
Lim, D., Jeong, J., Song, K. S., Sung, J. H., Oh, S. M., and Choi, J. (2021). Inhalation toxicity of polystyrene micro(nano)plastics using modified OECD TG 412. Chemosphere 262, 128330. doi:10.1016/j.chemosphere.2020.128330
Liu, K., Wang, X., Fang, T., Xu, P., Zhu, L., and Li, D. (2019). Source and potential risk assessment of suspended atmospheric microplastics in Shanghai. Sci. Total Environ. 675, 462–471. doi:10.1016/j.scitotenv.2019.04.110
Lu, K., Lai, K. P., Stoeger, T., Ji, S., Lin, Z., Lin, X., et al. (2021). Detrimental effects of microplastic exposure on normal and asthmatic pulmonary physiology. J. Hazard. Mat. 416, 126069. doi:10.1016/j.jhazmat.2021.126069
Mbachu, O., Jenkins, G., Pratt, C., and Kaparaju, P. (2020). A new contaminant superhighway? A review of sources, measurement techniques and fate of atmospheric microplastics. Water Air Soil Pollut. 231, 85. doi:10.1007/s11270-020-4459-4
Podgorski, A., and Gradon, L. (1990). Dynamics of pulmonary surfactant system and its role in alveolar cleansing. Ann. Occup. Hyg. 34, 137–147. doi:10.1093/annhyg/34.2.137
Prata, J. C. (2018). Airborne microplastics: Consequences to human health? Environ. Pollut. 234, 115–126. doi:10.1016/j.envpol.2017.11.043
Rist, S., Almroth, B. C., Hartmann, N. B., and Karlsson, T. M. (2018). A critical perspective on early communications concerning human health aspects of microplastics. Sci. Total Environ. 626, 720–726. doi:10.1016/j.scitotenv.2018.01.092
Saito, A., Horie, M., and Nagase, T. (2018). TGF-Beta signaling in lung health and disease. Int. J. Mol. Sci. 19, E2460. doi:10.3390/ijms19082460
Shi, W., Cao, Y., Chai, X., Zhao, Q., Geng, Y., Liu, D., et al. (2022). Potential health risks of the interaction of microplastics and lung surfactant. J. Hazard. Mat. 429, 128109. doi:10.1016/j.jhazmat.2021.128109
Wright, S. L., Ulke, J., Font, A., Chan, K. L. A., and Kelly, F. J. (2020). Atmospheric microplastic deposition in an urban environment and an evaluation of transport. Environ. Int. 136, 105411. doi:10.1016/j.envint.2019.105411
Xu, M., Halimu, G., Zhang, Q., Song, Y., Fu, X., Li, Y., et al. (2019). Internalization and toxicity: A preliminary study of effects of nanoplastic particles on human lung epithelial cell. Sci. Total Environ. 694, 133794. doi:10.1016/j.scitotenv.2019.133794
Yang, S., Cheng, Y., Chen, Z., Liu, T., Yin, L., Pu, Y., et al. (2021). In vitro evaluation of nanoplastics using human lung epithelial cells, microarray analysis and co-culture model. Ecotoxicol. Environ. Saf. 226, 112837. doi:10.1016/j.ecoenv.2021.112837
Zhao, C., Wang, Y., Su, Z., Pu, W., Niu, M., Song, S., et al. (2020). Respiratory exposure to PM2.5 soluble extract disrupts mucosal barrier function and promotes the development of experimental asthma. Sci. Total Environ. 730, 139145. doi:10.1016/j.scitotenv.2020.139145
Zhu, K., Jia, H., Sun, Y., Dai, Y., Zhang, C., Guo, X., et al. (2020). Enhanced cytotoxicity of photoaged phenol-formaldehyde resins microplastics: Combined effects of environmentally persistent free radicals, reactive oxygen species, and conjugated carbonyls. Environ. Int. 145, 106137. doi:10.1016/j.envint.2020.106137
Keywords: microplastics, lung disease, epithelial barrier dysfunction, inflammatory response, redox imbalance
Citation: Lu K, Zhan D, Fang Y, Li L, Chen G, Chen S and Wang L (2022) Microplastics, potential threat to patients with lung diseases. Front.Toxicol. 4:958414. doi: 10.3389/ftox.2022.958414
Received: 31 May 2022; Accepted: 04 July 2022;
Published: 28 September 2022.
Edited by:
Albert Duschl, University of Salzburg, AustriaCopyright © 2022 Lu, Zhan, Fang, Li, Chen, Chen and Wang. This is an open-access article distributed under the terms of the Creative Commons Attribution License (CC BY). The use, distribution or reproduction in other forums is permitted, provided the original author(s) and the copyright owner(s) are credited and that the original publication in this journal is cited, in accordance with accepted academic practice. No use, distribution or reproduction is permitted which does not comply with these terms.
*Correspondence: Shanze Chen, NDc0MDc2NjEzQHFxLmNvbQ==; Lingwei Wang, bGltZXkxMkB2aXAuc2luYS5jb20=