NF2 alteration in mesothelioma
- 1Division of Cancer Biology, Aichi Cancer Center Research Institute, Nagoya, Japan
- 2Division of Molecular and Cellular Oncology, Nagoya University Graduate School of Medicine, Nagoya, Japan
The NF2 tumor suppressor gene is a frequent somatically mutated gene in mesothelioma, with 30%–40% mesotheliomas showing NF2 inactivation. NF2 encodes merlin, a member of the ezrin, radixin, and moesin (ERM) family of proteins that regulate cytoskeleton and cell signaling. Recent genome analysis revealed that NF2 alteration may be a late event in mesothelioma development, suggesting that NF2 mutation confers a more aggressive phenotype to mesothelioma cells and may not be directly caused by asbestos exposure. The Hippo tumor-suppressive and mTOR prooncogenic signaling pathways are crucial cell-signaling cascades regulated by merlin. Although the exact role and timing of NF2 inactivation in mesothelioma cells remain to be elucidated, targeting the NF2/merlin-Hippo pathway may be a new therapeutic strategy for patients with mesothelioma.
1 Introduction
Mesothelioma shows frequent somatic alterations in a few tumor suppressor genes, such as BAP1, NF2, CDKN2A/2B, and TP53. Other infrequently but characteristically mutated genes identified in mesothelioma are those involved in histone modifications and RNA processing (Bueno et al., 2016; Hmeljak et al., 2018). While oncogenic driver mutations of tyrosine kinases are rarely detected in mesothelioma, a promoter mutation in TERT that is essential for telomerase activity is frequently observed, especially in non-epithelioid histology (Tallet et al., 2014; Pirker et al., 2020). Chromosomal abnormalities in mesothelioma are characterized by frequent chromosomal loss, with some showing extensive chromosome loss, termed “genomic near-haploidization” (Hmeljak et al., 2018). Chromosomal rearrangements, including chromoplexy and chromothripsis, have also been detected in the mesothelioma genome, possibly resulting in the potential for neoantigen presentation (Mansfield et al., 2020). A recent comprehensive analysis using multiple sampling detected subclonal populations in mesothelioma tissues, suggesting that some genomic changes are early events and others are late (Meiller et al., 2021; Zhang et al., 2021). Among them, NF2 mutation seems to be a late event, indicating that the direct asbestos effect might not be related to the genomic damage of NF2 (Meiller et al., 2021; Zhang et al., 2021). Nonetheless, targeting the Hippo signaling pathway, which is regulated by merlin (NF2 product), is a promising therapeutic modality for developing inhibitors against transcriptional enhanced associated domain (TEAD) transcriptional factors. Thus, while the roles and timing of NF2 inactivation in mesothelioma cells remain to be fully elucidated, the study of NF2 in mesothelioma is a focus of much research to develop new diagnostic and therapeutic tools against this formidable disease.
2 NF2
NF2 was originally discovered to be responsible for a familial cancer syndrome, neurofibromatosis type II, and is located in chromosome 22q12 (Rouleau et al., 1993; Trofatter et al., 1993). The protein encoded by NF2 is moesin-ezrin-radixin-like protein (merlin, also known as neurofibromin 2 or schwannomin), a member of the Band 4.1 family of cytoskeletal linker proteins (Petrilli and Fernandez-Valle, 2016). Merlin is a 70-kDa protein with three distinct domains, consisting of the FERM (4.1, ezrin, radixin, and moesin) domain at the N-terminus, an alpha-helical domain and a C-terminal domain (Figure 1A). In contrast to other ezrin, radixin, and moesin (ERM) family of proteins, merlin does not have an actin-binding site in the C-terminal domain but instead has a unique actin-binding motif in the N-terminal domain.
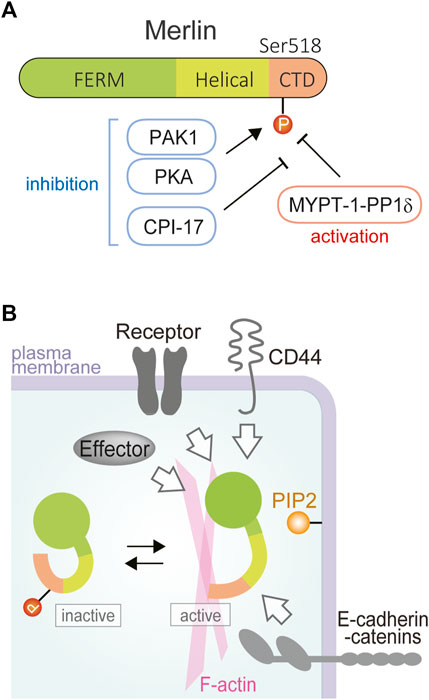
FIGURE 1. Domain structure and activation-inactivation of merlin. (A) Merlin is composed of N-terminal FERM (4.1/ezrin/radixin/moesin) domain (green), a central alpha-helical domain (light green), and a C-terminal domain (CTD; orange). Ser518 is one of the major phosphorylation sites, phosphorylated with PAK or PKA and dephosphorylated with myosin phosphatase holoenzyme MYPT-1-PP1δ that is encoded by PPP1R12A for the major regulatory subunit MYPT-1 and by PPP1CB for the catalytic subunit PP1β/δ. (B) Merlin activation is regulated by many membrane-associated proteins such as receptor tyrosine kinases, CD44, and E-cadherin-alpha catenin. Merlin activation is thought to be induced by dephosphorylation and lipid binding. Merlin interacts with the plasma membrane and the cortical actin skeleton to exert tumor-suppressive activity.
The tumor suppressive activity of merlin is regulated by conformational changes in the merlin molecule, which are due to the head-tail interaction of the merlin molecule, such as by phosphorylation modification (Figures 1A, B). One specific mechanism to inactivate merlin is thought to be the phosphorylation of the S518 residue in the tail domain by protein kinase A (PKA) or p21-activated kinase (PAK), while myosin phosphatase MYPT1-PP1δ activates merlin by dephosphorylating S518. Whether the open or closed form of merlin acts as a functional tumor suppressor is still unclear, but a structural study suggested that lipid binding of unphosphorylated merlin causes the open conformation of merlin and activates its tumor-suppressor function (Chinthalapudi et al., 2018).
Merlin indirectly links F-actin, transmembrane proteins, and intracellular effectors as a scaffold protein to regulate intracellular pathways that control cell survival and proliferation (McClatchey and Giovannini, 2005; Curto and McClatchey, 2008). These molecules include cell adhesion molecules and receptor tyrosine kinases (RTKs) that receive extracellular conditions/signals and downstream molecules associated with intracellular signal transduction cascades such as phosphoinositide 3-kinase (PI3K)/Akt, Hippo, and mammalian target of rapamycin (mTOR) pathways, which are prooncogenic or tumor suppressive pathways that have extensively been studied (Sato and Sekido, 2018).
Clinically, carriers with NF2 germline mutations are predisposed to bilateral vestibular schwannomas, those of other cranial and peripheral nerves, meningiomas, and ependymomas (Ferner, 2007). Interestingly, patients and carriers of NF2 familial cancer syndrome do not seem to present a significantly increased risk of mesothelioma (Asthagiri et al., 2009), and only a few rare cases of mesothelioma development with constitutional NF2 mutation have been reported so far (Baser et al., 2005). Patients with NF2 germline mutation have very different prognoses depending on ages of onset, tumor types, and types of genetic mutation; in a 30-year follow-up study of 353 patients, those observed to be symptomatic at age ≤25 had relatively poor prognoses, with about 10% dying 15 years after diagnosis, and the average life expectancy of those who died was in the 30s (Forde et al., 2021). Meanwhile, only about 10% of NF2 patients died within 15 years of diagnosis, and many survived for a long time (Forde et al., 2021). Therefore, although many NF2 patients reach the age of morbidity for mesothelioma onset, the development of mesothelioma has not specifically been reported, suggesting that NF2 germ line mutation is unlikely to be a predisposing mutation for familial mesothelioma development like BAP1.
NF2 somatic mutations have also been observed in sporadic tumors related to NF2 cancer syndrome such as meningioma (Nassiri et al., 2021), vestibular schwannoma (Carlson et al., 2018), and other malignancies, albeit at low frequency, including breast (Lang et al., 2020), liver (Zhang et al., 2017), and kidney (Hacking et al., 2023) cancers.
3 NF2 alteration in mesothelioma
Somatic mutation of NF2 is harbored in 30%–40% of pleural mesotheliomas (Bueno et al., 2016; Hmeljak et al., 2018; Sato and Sekido, 2018; Hiltbrunner et al., 2022). Peritoneal mesothelioma are 10%–20% of all mesothelioma cases, and NF2 mutation is seen in 21%–35% of cases (Hung et al., 2020; Hiltbrunner et al., 2022; Offin et al., 2022). In addition to non-sense/missense mutations or small/large deletions with loss of heterozygosity, resulting in bi-allelic loss of function, other structural abnormalities including gene rearrangement that disrupt the NF2 region can be observed in mesotheliomas (Bueno et al., 2016; Hmeljak et al., 2018). Noticeably, NF2 mutations are found more frequently in sarcomatoid rather than in epithelioid mesothelioma (Quetel et al., 2020).
Recent genome analysis with multi-sampling of the same patient’s mesothelioma tissues revealed clonality of mesothelioma cells, which showed that BAP1/3p21 loss is an early event, while NF2/22q loss is a late event (Zhang et al., 2021). Intra-tumor heterogeneity of NF2 mutation in pleural mesothelioma has also been observed in another study, indicating that NF2 mutation is a late event that may result in more aggressive phenotypes (Meiller et al., 2021).
Besides NF2, mesotheliomas harbor frequent inactivation of genes of the Hippo pathway, which seems to be an important signaling pathway regulated by merlin in mesothelial cells (Figure 2). LATS2 genetic alterations have been observed in 7%–11% of mesothelioma cases (Murakami et al., 2011; Bueno et al., 2016; Tranchant et al., 2017; Hmeljak et al., 2018). A comprehensive genome analysis of mesothelioma tissues detected frequent allelic loss among several Hippo pathway gene regions such as MST1 and LATS1 (Bueno et al., 2016). Epigenetic alterations in the promoter sequences of these component genes have also been detected (Hmeljak et al., 2018).
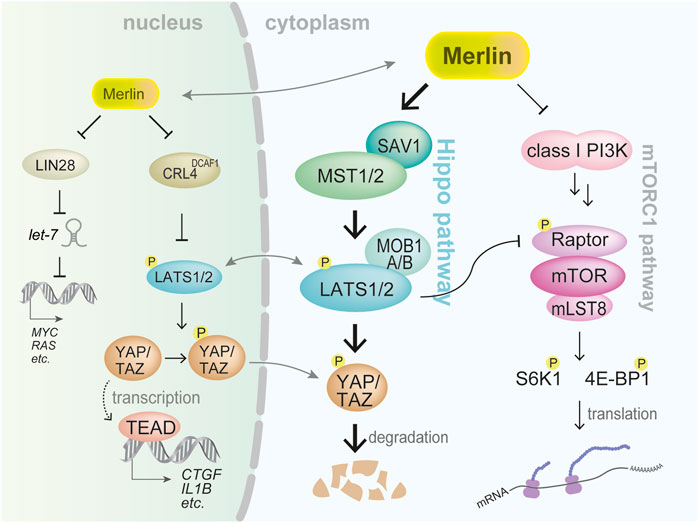
FIGURE 2. Representative signaling pathways regulated by merlin. Two significant downstream signaling pathways mediated through the cytoplasm are the Hippo and mTORC1 pathways. Under merlin inactivation, underphosphorylated (active) YAP/TAZ transcriptional coactivators bind to TEAD family transcription factor resulting in the expression of multiple prooncogenic genes such as CTGF and IL1B. Merlin also regulates mTORC1 activation that enhances translation of proteins. Merlin is also thought to translocate into the nucleus to exert its tumor-suppressive function.
These results suggest that the overall frequency of genetic abnormalities in NF2 and the Hippo pathway component genes may be underestimated and slightly higher than those previously reported in mesothelioma tissues. Regarding NF2, whole-exome sequencing alone cannot detect translocation and large deletion of NF2, which occasionally happens in mesothelioma. Even point mutations of NF2 might have been evaluated as wild-type in the samples analyzed, because in the above studies examining multiple specimens, 22% (Zhang et al., 2021) and 38% (Meiller et al., 2021) of the NF2 mutations detected were subclonal. Therefore, despite the presence of NF2 alterations, it is possible that NF2 gene abnormalities were overlooked depending on the location of the tumor tissue analyzed. A more important implication may be that NF2 mutations are not caused by a direct inflammatory response or DNA damage induced by asbestos. This idea is consistent with the absence of reports of increased risk of mesothelioma in NF2 germline mutation carriers.
In addition to the genetic or epigenetic inactivation of NF2 itself, other mechanisms are also known to be possibly involved in merlin inactivation. While MYPT-1-PP1δ (encoded by PPP1R12A and PPP1CB) dephosphorylates (activates) merlin, protein kinase C-potentiated phosphatase inhibitor of 17 kDa (CPI-17) (encoded by PPP1R14A) inhibits MYPT-1-PP1δ (Figure 1A) (Jin et al., 2006). Accordingly, merlin tends to be phosphorylated (inactivated) by CPI-17 overexpression, which has been demonstrated in mesothelioma tissues (Thurneysen et al., 2009). NF2 mRNA was reported to have splicing variants, and the same study indicated that the carboxyl-terminal variant (isoform 2), which may not have tumor suppressive activity, is more significantly expressed in mesothelioma (Thurneysen et al., 2009). NF2 also seemed to be suppressed by overexpression of specific NF2-targeting microRNAs including has-miR-885-3p (Guled et al., 2009).
4 Tumor-suppressive activity of NF2 gene in mesothelioma cells
In vitro transduction of NF2 can suppress proliferation and other malignant phenotypes of NF2-deficient mesothelioma cells (Xiao et al., 2005). In mesothelioma cells, merlin is thought to function as a tumor suppressor through multiple mechanisms. An early study indicated that merlin inhibits focal adhesion kinase (FAK), resulting in the inhibition of the interaction between FAK and its binding partners Src and p85, the regulatory subunit of PI3K (Poulikakos et al., 2006). Thus, one of the prooncogenic effects of NF2 inactivation may be accompanied with the upregulation of FAK activity.
The significance of Nf2 inactivation in mesothelioma pathogenesis was confirmed in Nf2-knockout mouse models in vivo. For instance, Nf2 (+/−) knockout mice showed accelerated mesothelioma tumor formation following asbestos exposure compared to the wild-type littermates with asbestos exposure only (Altomare et al., 2005). Conditional knockout mice targeting Nf2, Bap1, and/or Cdkn2a in cells of the mesothelium exhibited an increased incidence of mesothelioma, and noticeably, the triple-knockout mice showed highly invasive tumors with the shortest survival times (Kukuyan et al., 2019). Similar triple-knockout mouse models also confirmed that, although Bap1 deletion alone did not induce mesothelioma, Bap1 deletion dramatically accelerated mesothelioma development with the combined disruption of Nf2 and Cdkn2ab (Badhai et al., 2020).
Notably, in addition to functioning under the cytoplasmic membrane, merlin is thought to translocate into the cell nucleus (Figure 2). Merlin was shown to bind to CRL4DCAF1 and E3 ubiquitin ligase, thereby inhibiting the function of CRL4DCAF1 in the ubiquitination of its target protein (Li et al., 2010). Thus, merlin acted as a tumor suppressor by inhibiting CRL4DCAF1 in mesothelioma cells (Li et al., 2010). Moreover, CRL4DCAF1 was shown to directly bind to LATS1/2 and induce their ubiquitination and degradation (Li et al., 2014); thus, upregulated CRL4DCAF1 in NF2-inactivated mesothelioma cells accelerates degradation of LATS1/2 and hence activates YAP.
Independent of the Hippo pathway, merlin can exhibit cell-density-dependent, tumor-suppressive activity by inhibiting Lin28B function and promoting let-7 microRNA biogenesis (Hikasa et al., 2016). Since let-7 miRNAs silence RAS and MYC, the downregulation of let-7 by Lin28B induces mesothelioma cell proliferation.
5 Hippo pathway inactivation and targeting drugs
Hippo pathway is a significant signaling pathway regulated by merlin (Figure 2). The Hippo pathway participates in various physiological processes, including cell proliferation, cell differentiation, organ growth, embryogenesis, tissue regeneration, and wound healing (Fu et al., 2022). Dysregulation of this pathway is associated with various diseases including cardiac disease, pulmonary disease, hepatic disease, renal disease, and cancer (Fu et al., 2022). Hippo pathway composed of many molecules and classical core components are MST1 and MST2 kinases, SAV1 (also termed WW45), MOB1A/B, and LATS1 and LATS2 kinases, and a variety of signals and extracellular conditions including cell density, cell polarity, cell attachment, mechanical cues, and soluble factors, regulate the Hippo pathway (Fu et al., 2022).
Under the Hippo pathway activation, MST1 and MST2 kinases phosphorylate (activate) LATS1 and LATS2. MAP4Ks can also phosphorylate LATS1/2. Major targets of LATS1/2 kinases are YAP and TAZ transcriptional coactivators. When YAP/TAZ are phosphorylated, they are either retained within the cytoplasm or degraded. Conversely, when the Hippo pathway is inactivated, under-phosphorylated YAP/TAZ enters the cell nucleus and acts as a transcriptional coactivator. YAP/TAZ binds to distinct types of transcription factors including the TEAD family (Currey et al., 2021). Exogenously transduced constitutively activated mutant YAP S127A or TAZ S89A was shown to induce immortalized human mesothelial cells to form mesothelioma-like tumors in xenograft models (Kakiuchi et al., 2016; Matsushita et al., 2019).
In mesothelioma cells, activated YAP/TAZ enhances the transcription of a several important genes to stimulate cancer-promotion and progression, such as those encoding connective tissue growth factor (CTGF) (Fujii et al., 2012), phospholipase-C beta 4 (PLCB4) (Kakiuchi et al., 2016), and interleukin 1β (IL1B) (Matsushita et al., 2019). CTGF is an extracellular matrix-associated matricellular protein, and its overexpression appears to induce abundant extracellular matrix formation in mesothelioma (Fujii et al., 2012). Besides Hippo inactivation, TGF-β stimulation (Fujii et al., 2012) and β-catenin-TCF-LEF signaling (Jiang et al., 2014) further enhance the expression of CTGF. CTGF expression is higher in the sarcomatoid than in epithelioid mesothelioma, and it mediates the epithelial-mesenchymal transition (EMT) in mesothelioma (Jiang et al., 2014).
Since YAP/TAZ have been recognized as unlikely druggable targets, molecules that block the interaction of YAP/TAZ with TEADs have been vigorously investigated. Verteporfin (Visudyne) was the first small molecule that inhibited YAP-TEAD binding (Liu-Chittenden et al., 2012). Verteporfin suppresses YAP activity along with malignant phenotypes of mesothelioma cells in vitro, but the exact mechanisms by which verteporfin targets YAP are not fully understood (Tranchant et al., 2017). Recently, several groups have developed small molecules to inhibit the auto-palmitoylation of TEAD (Kaneda et al., 2020; Tang et al., 2021). These molecules share a common mechanism of binding to a conserved Cys residue (e.g., Cys359 of TEAD1), a palmitoylation site critical for YAP/TAZ and TEAD interaction, thus inhibiting YAP/TAZ binding to TEAD. These TEAD inhibitors have been shown to suppress tumors in vivo models (Kaneda et al., 2020; Tang et al., 2021). Although some molecules are under clinical study (Zagiel et al., 2022), TEAD palmitoylation inhibitors have also been implicated to have some therapeutic limitations in cancer cells (Sun et al., 2022).
6 Other therapeutic strategies for NF2-deficient mesothelioma
The merlin-Hippo pathway influences the activation of the PI3K-AKT-mTOR pathway. For example, merlin binds to PI3K enhancer-L and inhibits PI3K (Rong et al., 2004) (Figure 2). Furthermore, LATS1/2 inhibits mTORC1 activation by phosphorylating Raptor (Gan et al., 2020) (Figure 2). In addition, YAP induces AKT activation by inducing miR-29 expression and suppressing PTEN translation (Tumaneng et al., 2012). Therefore, molecules in the merlin-Hippo pathway influence mTORC1 activation at various levels.
To date, mTOR inhibitors have been investigated, and an allosteric mTOR inhibitor, rapamycin, has been demonstrated to have selective growth inhibitory effects in NF2-deficient mesothelioma cells (Lopez-Lago et al., 2009). However, a phase-II study of the mTOR inhibitor everolimus showed its limited clinical activity in advanced pleural mesothelioma (Ou et al., 2015). Meanwhile, a phase-I study using the class I PI3K and mTOR kinase dual inhibitor GDC-0980 (apitolisib) demonstrated partial response in pleural and peritoneal mesothelioma patients (Dolly et al., 2016). When considering what type of mTOR inhibitor to develop in future, it might be necessary to consider the influence of activation/inactivation of molecules of the merlin-Hippo pathway.
Loss of NF2 leads to PAK activation (Kissil et al., 2003). Thus, PAK inhibitors have been considered as for the treatment of NF2-deficient tumors. A recent study indicated that Nf2; Cdkn2a-deficient mouse mesothelioma cells showed less malignant phenotypes with PAK2 loss, suggesting that anti-PAK drugs may be promising for mesothelioma treatment (Sementino et al., 2022).
Another promising therapeutic strategy is the induction of synthetic lethality in mesothelioma cells. For instance, focal adhesion kinase (FAK) inhibition shows synthetic lethality with NF2 loss. VS-4718, a FAK inhibitor, suppressed proliferation and induced apoptosis in merlin-negative mesothelioma cells (Shapiro et al., 2014). However, a phase-II study using another FAK inhibitor, VS-6063 (defactinib), did not confirm clinical benefits as a maintenance therapy for pleural mesothelioma (Fennell et al., 2019). In this regard, E-cadherin expression was shown to be associated with the resistance of NF2-deficient mesothelioma cells to VS-4718, presenting a possible reason for why the clinical study did not show benefits (Kato et al., 2017). Another study showed that defactinib sensitivity using mesothelioma cell lines was not related to inactivating NF2 mutations or protein expression of merlin, but was strongly correlated with FAK activity, especially in epithelioid mesothelioma (Tranchant et al., 2018). Therefore, the hypothesis that FAK inhibitors exhibit synthetic lethality with NF2 mutations is not fully confirmed; however, its biomarker needs to be identified since FAK inhibitors are effective in a subset of mesothelioma cells with high FAK activity, and the study has identified LUM, a regulator of the integrin pathway, as a candidate (Tranchant et al., 2018).
Meanwhile, for NF2-mutated meningiomas, a phase-II study of GSK2256098, another FAK inhibitor, has been shown to be well tolerated, resulting in an improved progression-free survival rate (Brastianos et al., 2022). In addition to FAK, the discovery of new molecules or drugs that can induce synthetic lethal phonotype with NF2 deficiency may provide a new therapeutical strategy for mesothelioma.
7 Ferroptosis induction by YAP
From their discovery, YAP/TAZ have been thought to act as both oncoproteins and tumor-suppressive proteins, depending on the cellular context. The tumor suppressive activities of YAP/TAZ have been reported in the colon, estrogen receptor-positive breast, hematological, and other human solid cancers (Pearson et al., 2021). Ferroptosis refers to regulated cell death that is characterized by the iron-dependent accumulation of lipid hydorperoxides to lethal levels (Stockwell et al., 2017). A central regulator of ferroptosis is glutathione peroxidase 4 (GPX4), which protects cells by eliminating lipid peroxides, and cancer cells with mesenchymal or metastatic property are highly susceptible to ferroptosis (Hangauer et al., 2017; Viswanathan et al., 2017). Notably, ferroptosis was shown to be regulated by cadherin-mediated intercellular interactions and the merlin (NF2)-Hippo pathway (Wu et al., 2019). NF2 inactivation sensitized cancer cells to ferroptosis because YAP induced the expression of the crucial mediators of ferroptosis such as transferrin receptor 1 (TFRC) and acyl-CoA synthetase long chain family member 4 (ACSL4), suggesting that YAP can act as a tumor suppressor dictating ferroptotic death including mesothelioma (Wu et al., 2019). Thus, the inactivation of merlin (NF2)-Hippo pathway status has been proposed as a predictor of the sensitiveness of cancer cells to ferroptosis-induction therapies (Wu et al., 2019).
The results are contrary to previous findings that YAP activation in mesothelioma cells indicates oncogenic function. One explanation for the result might be that YAP activation is affected by various factors, including the extracellular environment and intracellular signaling/metabolism; therefore, changes in such conditions can alter the gene transcription pattern regulated by YAP, resulting in the predominant expression of a group of cancer-promoting genes or, if the conditions are met, those of tumor suppressive genes, including ferroptosis-related genes. Furthermore, loss of function of p53 (Jiang et al., 2015) and BAP1 (Zhang et al., 2018) has been reported to enhance resistance to ferroptosis, and it is possible that YAP functions more in a cancer-promoting manner in mesothelioma cells with those mutations, although further detailed studies are required.
8 NF2 mutation for mesothelioma diagnosis
NF2 deficiency has been shown to have utility as a diagnostic, prognostic, and/or predictive biomarker for mesothelioma. Genetic testing for CDKN2A/2B loss and immunohistochemistry (IHC) for methylthioadenosine phosphorylase (MTAP) loss and BAP1 loss are currently thought to be the most reliable molecular tools for differentiating mesothelioma from other malignancies and, often most critically, from reactive mesothelial hyperplasia, which is a benign change. A recent study indicated that the addition of genetic NF2 screening improved the sensitivity or specificity of mesothelioma diagnosis (Nabeshima et al., 2022). Another study reported that low expression of merlin and high labeling index of survivin were correlated with poor prognosis in pleural mesothelioma patients (Meerang et al., 2016). Similarly, a combination of CDKN2A homozygous deletion and NF2 hemizygous loss is a negative prognostic factor for patients with peritoneal mesothelioma (Singhi et al., 2016). Regarding the IHC study of merlin, which has been recognized as challenging with currently available antibodies for merlin, a recent study indicated that the expression of merlin was absent in 52% of all pleural mesotheliomas and 70% of non-epithelioid tumors (Chapel et al., 2022).
9 Conclusion
NF2 alteration is a key genetic change in mesothelioma development, which may occur at a late stage as a subclonal event, suggesting that NF2 alteration may not be directly induced by asbestos exposure. NF2 mutation is thus thought to confer a more aggressive phenotype to mesothelioma cells, possibly involved in the induction of EMT. The Hippo pathway is a crucial signaling cascade that is regulated by merlin (NF2), which is currently considered a promising target for patients with mesothelioma. Future studies are needed to more precisely identify the roles of merlin (NF2) alteration in mesothelioma development and progression, which would lead to the development of new molecular-targeted drugs.
Author contributions
YS and TS conceived, wrote, created figures, and critically proofread the article.
Funding
This work was supported in part by JSPS KAKENHI (22K19472), JRF (Japanese Respiratory Foundation) Grant, and a Research Grant from the Princess Takamatsu Cancer Research Fund (22-25417) for YS and JSPS KAKENHI (22K07162) for TS.
Acknowledgments
YS and TS acknowledge Satomi Mukai for helpful comments on this article. We would like to thank Editage (www.editage.com) for English language editing.
Conflict of interest
The authors declare that the research was conducted in the absence of any commercial or financial relationships that could be construed as a potential conflict of interest.
Publisher’s note
All claims expressed in this article are solely those of the authors and do not necessarily represent those of their affiliated organizations, or those of the publisher, the editors and the reviewers. Any product that may be evaluated in this article, or claim that may be made by its manufacturer, is not guaranteed or endorsed by the publisher.
References
Altomare, D. A., Vaslet, C. A., Skele, K. L., De Rienzo, A., Devarajan, K., Jhanwar, S. C., et al. (2005). A mouse model recapitulating molecular features of human mesothelioma. Cancer Res. 65 (18), 8090–8095. doi:10.1158/0008-5472.CAN-05-2312
Asthagiri, A. R., Parry, D. M., Butman, J. A., Kim, H. J., Tsilou, E. T., Zhuang, Z., et al. (2009). Neurofibromatosis type 2. Lancet 373 (9679), 1974–1986. doi:10.1016/s0140-6736(09)60259-2
Badhai, J., Pandey, G. K., Song, J. Y., Krijgsman, O., Bhaskaran, R., Chandrasekaran, G., et al. (2020). Combined deletion of Bap1, Nf2, and Cdkn2ab causes rapid onset of malignant mesothelioma in mice. J. Exp. Med. 217 (6), e20191257. doi:10.1084/jem.20191257
Baser, M. E., Rai, H., Wallace, A. J., and Evans, D. G. (2005). Neurofibromatosis 2 (NF2) and malignant mesothelioma in a man with a constitutional NF2 missense mutation. Fam. Cancer 4 (4), 321–322. doi:10.1007/s10689-005-0659-8
Brastianos, P. K., Twohy, E. L., Gerstner, E. R., Kaufmann, T. J., Iafrate, A. J., Lennerz, J., et al. (2022). Alliance A071401: Phase II trial of focal adhesion kinase inhibition in meningiomas with somatic NF2 mutations. J. Clin. Oncol. 41, 618–628. doi:10.1200/jco.21.02371
Bueno, R., Stawiski, E. W., Goldstein, L. D., Durinck, S., De Rienzo, A., Modrusan, Z., et al. (2016). Comprehensive genomic analysis of malignant pleural mesothelioma identifies recurrent mutations, gene fusions and splicing alterations. Nat. Genet. 48 (4), 407–416. doi:10.1038/ng.3520
Carlson, M. L., Smadbeck, J. B., Link, M. J., Klee, E. W., Vasmatzis, G., and Schimmenti, L. A. (2018). Next generation sequencing of sporadic vestibular schwannoma: Necessity of biallelic NF2 inactivation and implications of accessory non-NF2 variants. Otol. Neurotol. 39 (9), e860–e871. doi:10.1097/mao.0000000000001932
Chapel, D. B., Hornick, J. L., Barlow, J., Bueno, R., and Sholl, L. M. (2022). Clinical and molecular validation of BAP1, MTAP, P53, and Merlin immunohistochemistry in diagnosis of pleural mesothelioma. Mod. Pathol. 35 (10), 1383–1397. doi:10.1038/s41379-022-01081-z
Chinthalapudi, K., Mandati, V., Zheng, J., Sharff, A. J., Bricogne, G., Griffin, P. R., et al. (2018). Lipid binding promotes the open conformation and tumor-suppressive activity of neurofibromin 2. Nat. Commun. 9 (1), 1338. doi:10.1038/s41467-018-03648-4
Currey, L., Thor, S., and Piper, M. (2021). TEAD family transcription factors in development and disease. Development 148 (12), dev196675. doi:10.1242/dev.196675
Curto, M., and McClatchey, A. I. (2008). Nf2/Merlin: A coordinator of receptor signalling and intercellular contact. Br. J. Cancer 98 (2), 256–262. doi:10.1038/sj.bjc.6604002
Dolly, S. O., Wagner, A. J., Bendell, J. C., Kindler, H. L., Krug, L. M., Seiwert, T. Y., et al. (2016). Phase I study of apitolisib (GDC-0980), dual phosphatidylinositol-3-kinase and mammalian target of rapamycin kinase inhibitor, in patients with advanced solid tumors. Clin. Cancer Res. 22 (12), 2874–2884. doi:10.1158/1078-0432.CCR-15-2225
Fennell, D. A., Baas, P., Taylor, P., Nowak, A. K., Gilligan, D., Nakano, T., et al. (2019). Maintenance defactinib versus placebo after first-line chemotherapy in patients with merlin-stratified pleural mesothelioma: COMMAND-A double-blind, randomized, phase II study. J. Clin. Oncol. 37 (10), 790–798. doi:10.1200/jco.2018.79.0543
Ferner, R. E. (2007). Neurofibromatosis 1 and neurofibromatosis 2: A twenty first century perspective. Lancet Neurol. 6 (4), 340–351. doi:10.1016/S1474-4422(07)70075-3
Forde, C., King, A. T., Rutherford, S. A., Hammerbeck-Ward, C., Lloyd, S. K., Freeman, S. R., et al. (2021). Disease course of neurofibromatosis type 2: A 30-year follow-up study of 353 patients seen at a single institution. Neuro Oncol. 23 (7), 1113–1124. doi:10.1093/neuonc/noaa284
Fu, M., Hu, Y., Lan, T., Guan, K. L., Luo, T., and Luo, M. (2022). The Hippo signalling pathway and its implications in human health and diseases. Signal Transduct. Target Ther. 7 (1), 376. doi:10.1038/s41392-022-01191-9
Fujii, M., Toyoda, T., Nakanishi, H., Yatabe, Y., Sato, A., Matsudaira, Y., et al. (2012). TGF-beta synergizes with defects in the Hippo pathway to stimulate human malignant mesothelioma growth. J. Exp. Med. 209, 479–494. doi:10.1084/jem.20111653
Gan, W., Dai, X., Dai, X., Xie, J., Yin, S., Zhu, J., et al. (2020). LATS suppresses mTORC1 activity to directly coordinate Hippo and mTORC1 pathways in growth control. Nat. Cell. Biol. 22 (2), 246–256. doi:10.1038/s41556-020-0463-6
Guled, M., Lahti, L., Lindholm, P. M., Salmenkivi, K., Bagwan, I., Nicholson, A. G., et al. (2009). CDKN2A, NF2, and JUN are dysregulated among other genes by miRNAs in malignant mesothelioma-A miRNA microarray analysis. Genes. Chromosom. Cancer 48, 615–623. doi:10.1002/gcc.20669
Hacking, S. M., Pavlick, D., Wang, Y., Carneiro, B. A., Mullally, M., Lu, S., et al. (2023). Comprehensive genomic profiling of NF2-mutated kidney tumors reveals potential targets for therapy. Oncologist 2023, oyad040. doi:10.1093/oncolo/oyad040
Hangauer, M. J., Viswanathan, V. S., Ryan, M. J., Bole, D., Eaton, J. K., Matov, A., et al. (2017). Drug-tolerant persister cancer cells are vulnerable to GPX4 inhibition. Nature 551 (7679), 247–250. doi:10.1038/nature24297
Hikasa, H., Sekido, Y., and Suzuki, A. (2016). Merlin/NF2-Lin28B-let-7 is a tumor-suppressive pathway that is cell-density dependent and hippo independent. Cell. Rep. 14 (12), 2950–2961. doi:10.1016/j.celrep.2016.02.075
Hiltbrunner, S., Fleischmann, Z., Sokol, E. S., Zoche, M., Felley-Bosco, E., and Curioni-Fontecedro, A. (2022). Genomic landscape of pleural and peritoneal mesothelioma tumours. Br. J. Cancer 127 (11), 1997–2005. doi:10.1038/s41416-022-01979-0
Hmeljak, J., Sanchez-Vega, F., Hoadley, K. A., Shih, J., Stewart, C., Heiman, D., et al. (2018). Integrative molecular characterization of malignant pleural mesothelioma. Cancer Discov. 8 (12), 1548–1565. doi:10.1158/2159-8290.cd-18-0804
Hung, Y. P., Dong, F., Torre, M., Crum, C. P., Bueno, R., and Chirieac, L. R. (2020). Molecular characterization of diffuse malignant peritoneal mesothelioma. Mod. Pathol. 33 (11), 2269–2279. doi:10.1038/s41379-020-0588-y
Jiang, L., Kon, N., Li, T., Wang, S. J., Su, T., Hibshoosh, H., et al. (2015). Ferroptosis as a p53-mediated activity during tumour suppression. Nature 520 (7545), 57–62. doi:10.1038/nature14344
Jiang, L., Yamashita, Y., Chew, S. H., Akatsuka, S., Ukai, S., Wang, S., et al. (2014). Connective tissue growth factor and beta-catenin constitute an autocrine loop for activation in rat sarcomatoid mesothelioma. J. Pathol. 233 (4), 402–414. doi:10.1002/path.4377
Jin, H., Sperka, T., Herrlich, P., and Morrison, H. (2006). Tumorigenic transformation by CPI-17 through inhibition of a merlin phosphatase. Nature 442 (7102), 576–579. doi:10.1038/nature04856
Kakiuchi, T., Takahara, T., Kasugai, Y., Arita, K., Yoshida, N., Karube, K., et al. (2016). Modeling mesothelioma utilizing human mesothelial cells reveals involvement of phospholipase-C beta 4 in YAP-active mesothelioma cell proliferation. Carcinogenesis 37 (11), 1098–1109. doi:10.1093/carcin/bgw084
Kaneda, A., Seike, T., Danjo, T., Nakajima, T., Otsubo, N., Yamaguchi, D., et al. (2020). The novel potent TEAD inhibitor, K-975, inhibits YAP1/TAZ-TEAD protein-protein interactions and exerts an anti-tumor effect on malignant pleural mesothelioma. Am. J. Cancer Res. 10 (12), 4399–4415.
Kato, T., Sato, T., Yokoi, K., and Sekido, Y. (2017). E-cadherin expression is correlated with focal adhesion kinase inhibitor resistance in Merlin-negative malignant mesothelioma cells. Oncogene 36 (39), 5522–5531. doi:10.1038/onc.2017.147
Kissil, J. L., Wilker, E. W., Johnson, K. C., Eckman, M. S., Yaffe, M. B., and Jacks, T. (2003). Merlin, the product of the Nf2 tumor suppressor gene, is an inhibitor of the p21-activated kinase, Pak1. Mol. Cell. 12 (4), 841–849. doi:10.1016/s1097-2765(03)00382-4
Kukuyan, A. M., Sementino, E., Kadariya, Y., Menges, C. W., Cheung, M., Tan, Y., et al. (2019). Inactivation of Bap1 cooperates with losses of Nf2 and Cdkn2a to drive the development of pleural malignant mesothelioma in conditional mouse models. Cancer Res. 79 (16), 4113–4123. doi:10.1158/0008-5472.can-18-4093
Lang, G. T., Jiang, Y. Z., Shi, J. X., Yang, F., Li, X. G., Pei, Y. C., et al. (2020). Characterization of the genomic landscape and actionable mutations in Chinese breast cancers by clinical sequencing. Nat. Commun. 11 (1), 5679. doi:10.1038/s41467-020-19342-3
Li, W., Cooper, J., Zhou, L., Yang, C., Erdjument-Bromage, H., Zagzag, D., et al. (2014). Merlin/NF2 loss-driven tumorigenesis linked to CRL4(DCAF1)-mediated inhibition of the hippo pathway kinases Lats1 and 2 in the nucleus. Cancer Cell. 26 (1), 48–60. doi:10.1016/j.ccr.2014.05.001
Li, W., You, L., Cooper, J., Schiavon, G., Pepe-Caprio, A., Zhou, L., et al. (2010). Merlin/NF2 suppresses tumorigenesis by inhibiting the E3 ubiquitin ligase CRL4(DCAF1) in the nucleus. Cell. 140 (4), 477–490. doi:10.1016/j.cell.2010.01.029
Liu-Chittenden, Y., Huang, B., Shim, J. S., Chen, Q., Lee, S. J., Anders, R. A., et al. (2012). Genetic and pharmacological disruption of the TEAD-YAP complex suppresses the oncogenic activity of YAP. Genes. Dev. 26 (12), 1300–1305. doi:10.1101/gad.192856.112
Lopez-Lago, M. A., Okada, T., Murillo, M. M., Socci, N., and Giancotti, F. G. (2009). Loss of the tumor suppressor gene NF2, encoding merlin, constitutively activates integrin-dependent mTORC1 signaling. Mol. Cell. Biol. 29 (15), 4235–4249. doi:10.1128/MCB.01578-08
Mansfield, A. S., Peikert, T., and Vasmatzis, G. (2020). Chromosomal rearrangements and their neoantigenic potential in mesothelioma. Transl. Lung Cancer Res. 9 (1), S92–S99. doi:10.21037/tlcr.2019.11.12
Matsushita, A., Sato, T., Mukai, S., Fujishita, T., Mishiro-Sato, E., Okuda, M., et al. (2019). TAZ activation by Hippo pathway dysregulation induces cytokine gene expression and promotes mesothelial cell transformation. Oncogene 38 (11), 1966–1978. doi:10.1038/s41388-018-0417-7
McClatchey, A. I., and Giovannini, M. (2005). Membrane organization and tumorigenesis--the NF2 tumor suppressor, Merlin. Genes. Dev. 19 (19), 2265–2277. doi:10.1101/gad.1335605
Meerang, M., Berard, K., Friess, M., Bitanihirwe, B. K., Soltermann, A., Vrugt, B., et al. (2016). Low Merlin expression and high Survivin labeling index are indicators for poor prognosis in patients with malignant pleural mesothelioma. Mol. Oncol. 10 (8), 1255–1265. doi:10.1016/j.molonc.2016.06.005
Meiller, C., Montagne, F., Hirsch, T. Z., Caruso, S., de Wolf, J., Bayard, Q., et al. (2021). Multi-site tumor sampling highlights molecular intra-tumor heterogeneity in malignant pleural mesothelioma. Genome Med. 13 (1), 113. doi:10.1186/s13073-021-00931-w
Murakami, H., Mizuno, T., Taniguchi, T., Fujii, M., Ishiguro, F., Fukui, T., et al. (2011). LATS2 is a tumor suppressor gene of malignant mesothelioma. Cancer Res. 71 (3), 873–883. doi:10.1158/0008-5472.CAN-10-2164
Nabeshima, K., Hamasaki, M., Kinoshita, Y., Matsumoto, S., and Sa-Ngiamwibool, P. (2022). Update of pathological diagnosis of pleural mesothelioma using genomic-based morphological techniques, for both histological and cytological investigations. Pathol. Int. 72 (8), 389–401. doi:10.1111/pin.13235
Nassiri, F., Liu, J., Patil, V., Mamatjan, Y., Wang, J. Z., Hugh-White, R., et al. (2021). A clinically applicable integrative molecular classification of meningiomas. Nature 597 (7874), 119–125. doi:10.1038/s41586-021-03850-3
Offin, M., Yang, S. R., Egger, J., Jayakumaran, G., Spencer, R. S., Lopardo, J., et al. (2022). Molecular characterization of peritoneal mesotheliomas. J. Thorac. Oncol. 17 (3), 455–460. doi:10.1016/j.jtho.2021.09.012
Ou, S. H., Moon, J., Garland, L. L., Mack, P. C., Testa, J. R., Tsao, A. S., et al. (2015). Swog S0722: Phase II study of mTOR inhibitor everolimus (RAD001) in advanced malignant pleural mesothelioma (MPM). J. Thorac. Oncol. 10 (2), 387–391. doi:10.1097/JTO.0000000000000360
Pearson, J. D., Huang, K., Pacal, M., McCurdy, S. R., Lu, S., Aubry, A., et al. (2021). Binary pan-cancer classes with distinct vulnerabilities defined by pro- or anti-cancer YAP/TEAD activity. Cancer Cell. 39 (8), 1115–1134. doi:10.1016/j.ccell.2021.06.016
Petrilli, A. M., and Fernandez-Valle, C. (2016). Role of Merlin/NF2 inactivation in tumor biology. Oncogene 35 (5), 537–548. doi:10.1038/onc.2015.125
Pirker, C., Bilecz, A., Grusch, M., Mohr, T., Heidenreich, B., Laszlo, V., et al. (2020). Telomerase reverse transcriptase promoter mutations identify a genomically defined and highly aggressive human pleural mesothelioma subgroup. Clin. Cancer Res. 26 (14), 3819–3830. doi:10.1158/1078-0432.ccr-19-3573
Poulikakos, P. I., Xiao, G. H., Gallagher, R., Jablonski, S., Jhanwar, S. C., and Testa, J. R. (2006). Re-expression of the tumor suppressor NF2/merlin inhibits invasiveness in mesothelioma cells and negatively regulates FAK. Oncogene 25 (44), 5960–5968. doi:10.1038/sj.onc.1209587
Quetel, L., Meiller, C., Assié, J. B., Blum, Y., Imbeaud, S., Montagne, F., et al. (2020). Genetic alterations of malignant pleural mesothelioma: Association with tumor heterogeneity and overall survival. Mol. Oncol. 14 (6), 1207–1223. doi:10.1002/1878-0261.12651
Rong, R., Tang, X., Gutmann, D. H., and Ye, K. (2004). Neurofibromatosis 2 (NF2) tumor suppressor merlin inhibits phosphatidylinositol 3-kinase through binding to PIKE-L. Proc. Natl. Acad. Sci. U. S. A. 101 (52), 18200–18205. doi:10.1073/pnas.0405971102
Rouleau, G. A., Merel, P., Lutchman, M., Sanson, M., Zucman, J., Marineau, C., et al. (1993). Alteration in a new gene encoding a putative membrane-organizing protein causes neuro-fibromatosis type 2. Nature 363 (6429), 515–521. doi:10.1038/363515a0
Sato, T., and Sekido, Y. (2018). NF2/Merlin inactivation and potential therapeutic targets in mesothelioma. Int. J. Mol. Sci. 19 (4), E988. doi:10.3390/ijms19040988
Sementino, E., Kadariya, Y., Cheung, M., Menges, C. W., Tan, Y., Kukuyan, A. M., et al. (2022). Inactivation of p21-activated kinase 2 (Pak2) inhibits the development of nf2-deficient tumors by restricting downstream hedgehog and wnt signaling. Mol. Cancer Res. 20 (5), 699–711. doi:10.1158/1541-7786.MCR-21-0837
Shapiro, I. M., Kolev, V. N., Vidal, C. M., Kadariya, Y., Ring, J. E., Wright, Q., et al. (2014). Merlin deficiency predicts FAK inhibitor sensitivity: A synthetic lethal relationship. Sci. Transl. Med. 6 (237), 237ra68. doi:10.1126/scitranslmed.3008639
Singhi, A. D., Krasinskas, A. M., Choudry, H. A., Bartlett, D. L., Pingpank, J. F., Zeh, H. J., et al. (2016). The prognostic significance of BAP1, NF2, and CDKN2A in malignant peritoneal mesothelioma. Mod. Pathol. 29 (1), 14–24. doi:10.1038/modpathol.2015.121
Stockwell, B. R., Friedmann Angeli, J. P., Bayir, H., Bush, A. I., Conrad, M., Dixon, S. J., et al. (2017). Ferroptosis: A regulated cell death nexus linking metabolism, redox biology, and disease. Cell. 171 (2), 273–285. doi:10.1016/j.cell.2017.09.021
Sun, Y., Hu, L., Tao, Z., Jarugumilli, G. K., Erb, H., Singh, A., et al. (2022). Pharmacological blockade of TEAD-YAP reveals its therapeutic limitation in cancer cells. Nat. Commun. 13 (1), 6744. doi:10.1038/s41467-022-34559-0
Tallet, A., Nault, J. C., Renier, A., Hysi, I., Galateau-Salle, F., Cazes, A., et al. (2014). Overexpression and promoter mutation of the TERT gene in malignant pleural mesothelioma. Oncogene 33 (28), 3748–3752. doi:10.1038/onc.2013.351
Tang, T. T., Konradi, A. W., Feng, Y., Peng, X., Ma, M., Li, J., et al. (2021). Small molecule inhibitors of TEAD auto-palmitoylation selectively inhibit proliferation and tumor growth of NF2-deficient mesothelioma. Mol. Cancer Ther. 20 (6), 986–998. doi:10.1158/1535-7163.mct-20-0717
Thurneysen, C., Opitz, I., Kurtz, S., Weder, W., Stahel, R. A., and Felley-Bosco, E. (2009). Functional inactivation of NF2/merlin in human mesothelioma. Lung Cancer 64 (2), 140–147. doi:10.1016/j.lungcan.2008.08.014
Tranchant, R., Quetel, L., Montagne, F., De Wolf, J., Meiller, C., De Koning, L., et al. (2018). Assessment of signaling pathway inhibitors and identification of predictive biomarkers in malignant pleural mesothelioma. Lung Cancer 126, 15–24. doi:10.1016/j.lungcan.2018.10.015
Tranchant, R., Quetel, L., Tallet, A., Meiller, C., Renier, A., de Koning, L., et al. (2017). Co-Occurring mutations of tumor suppressor genes, LATS2 and NF2, in malignant pleural mesothelioma. Clin. Cancer Res. 23 (12), 3191–3202. doi:10.1158/1078-0432.CCR-16-1971
Trofatter, J. A., MacCollin, M. M., Rutter, J. L., Murrell, J. R., Duyao, M. P., Parry, D. M., et al. (1993). A novel moesin-ezrin-radixin-like gene is a candidate for the neurofibromatosis 2 tumor suppressor. Cell. 72 (5), 791–800. doi:10.1016/0092-8674(93)90406-g
Tumaneng, K., Schlegelmilch, K., Russell, R. C., Yimlamai, D., Basnet, H., Mahadevan, N., et al. (2012). YAP mediates crosstalk between the Hippo and PI(3)K-TOR pathways by suppressing PTEN via miR-29. Nat. Cell. Biol. 14 (12), 1322–1329. doi:10.1038/ncb2615
Viswanathan, V. S., Ryan, M. J., Dhruv, H. D., Gill, S., Eichhoff, O. M., Seashore-Ludlow, B., et al. (2017). Dependency of a therapy-resistant state of cancer cells on a lipid peroxidase pathway. Nature 547 (7664), 453–457. doi:10.1038/nature23007
Wu, J., Minikes, A. M., Gao, M., Bian, H., Li, Y., Stockwell, B. R., et al. (2019). Intercellular interaction dictates cancer cell ferroptosis via NF2-YAP signalling. Nature 572 (7769), 402–406. doi:10.1038/s41586-019-1426-6
Xiao, G. H., Gallagher, R., Shetler, J., Skele, K., Altomare, D. A., Pestell, R. G., et al. (2005). The NF2 tumor suppressor gene product, merlin, inhibits cell proliferation and cell cycle progression by repressing cyclin D1 expression. Mol. Cell. Biol. 25 (6), 2384–2394. doi:10.1128/MCB.25.6.2384-2394.2005
Zagiel, B., Melnyk, P., and Cotelle, P. (2022). Progress with YAP/TAZ-TEAD inhibitors: A patent review (2018-present). Expert Opin. Ther. Pat. 32 (8), 899–912. doi:10.1080/13543776.2022.2096436
Zhang, M., Luo, J. L., Sun, Q., Harber, J., Dawson, A. G., Nakas, A., et al. (2021). Clonal architecture in mesothelioma is prognostic and shapes the tumour microenvironment. Nat. Commun. 12 (1), 1751. doi:10.1038/s41467-021-21798-w
Zhang, N., Zhao, Z., Long, J., Li, H., Zhang, B., Chen, G., et al. (2017). Molecular alterations of the NF2 gene in hepatocellular carcinoma and intrahepatic cholangiocarcinoma. Oncol. Rep. 38 (6), 3650–3658. doi:10.3892/or.2017.6055
Keywords: mesothelioma, genome, tumor suppressor gene, NF2, Hippo pathway
Citation: Sekido Y and Sato T (2023) NF2 alteration in mesothelioma. Front. Toxicol. 5:1161995. doi: 10.3389/ftox.2023.1161995
Received: 01 March 2023; Accepted: 06 April 2023;
Published: 25 April 2023.
Edited by:
Marie-Claude Jaurand, Institut National de la Santé et de la Recherche Médicale (INSERM), FranceReviewed by:
Didier Jean, Institut National de la Santé et de la Recherche Médicale (INSERM), FranceCopyright © 2023 Sekido and Sato. This is an open-access article distributed under the terms of the Creative Commons Attribution License (CC BY). The use, distribution or reproduction in other forums is permitted, provided the original author(s) and the copyright owner(s) are credited and that the original publication in this journal is cited, in accordance with accepted academic practice. No use, distribution or reproduction is permitted which does not comply with these terms.
*Correspondence: Yoshitaka Sekido, ysekido@aichi-cc.jp