- Louvain Centre for Toxicology and Applied Pharmacology (LTAP), Institut de Recherche Experimentale et Clinique (IREC), Université Catholique de Louvain (UCLouvain), Brussels, Belgium
Since their discovery nearly 40 years ago, B-1 cells have continued to challenge the boundaries between innate and adaptive immunity, as well as myeloid and lymphoid functions. This B-cell subset ensures early immunity in neonates before the development of conventional B (B-2) cells and respond to immune injuries throughout life. B-1 cells are multifaceted and serve as natural- and induced-antibody-producing cells, phagocytic cells, antigen-presenting cells, and anti-/pro-inflammatory cytokine-releasing cells. This review retraces the origin of B-1 cells and their different roles in homeostatic and infectious conditions before focusing on pollutants comprising contact-sensitivity-inducing chemicals, endocrine disruptors, aryl hydrocarbon receptor (AHR) ligands, and reactive particles.
1 Introduction
B lymphocytes are white blood cells produced in the bone marrow and circulate in the blood and lymphatic vessels to defend the body against pathogens by secreting antibodies. B lymphocytes are classified according to their functions and differentiation. B-cell subsets mainly comprise B-2 cells, also called “conventional B cells” or “follicular B cells”. B-2 cells have been implicated in adaptive immunity and generate high-affinity antibodies with precise antigen specificity (LeBien and Tedder, 2008). The activation of B-2 cells results from an immune insult (e.g., an infection) sensed by immune cells, such as T and dendritic cells. Cross-talk between these cells is necessary for optimal adaptive humoral immune responses (den Haan et al., 2014). Plasma cells are fully differentiated B cells dedicated to releasing large amounts of antibodies of one isotype, and memory cells are long-lived B cells that provide long-term immunity (Cancro and Tomayko, 2021).
Less well-described, B-cell subsets also comprise innate-related cells. Indeed, antibody production by marginal zone (MZ) B and B-1 cells is T-independent. MZ B cells are localized in the secondary lymphoid organs and provide antibodies against circulating blood-borne pathogens (Martin et al., 2001). B-1 cells reside primarily in the mesothelial (peritoneal and pleural) cavities, representing the majority (up to 80%) of the B cells (Marcos et al., 1989; Kroese et al., 1992; Ghosn et al., 2010; Hiéronimus et al., 2021). Finally, among innate B cells, regulatory B cells (B reg) are those that reduce the level of inflammation and act as immunosuppressive cells (Dasgupta et al., 2020). Notably, B10 cells are a subset of B reg whose immunoregulatory effects are fully attributable to interleukin-10 production (Tedder, 2015).
This study aimed to highlight the wide range of B-1 cell responses. They remain one of the less-known subsets of B cells because they possess many facets and participate in various immune responses. Many of these functions are possibly undiscovered. In the present paper, we decided against using the term “B-1 lymphocytes” to refer to these cells as it limits them as lymphocytes. However, B-1 cells serve both lymphoid and myeloid functions as they express both sets of surface markers (Popi et al., 2009a). B-1 cells can be seen as pleiotropic multifaceted precursors or as a collection of several subsets of fully differentiated cells (Popi et al., 2012), including adaptive immune cells (Cole et al., 2009; Smith and Baumgarth, 2019). The new understanding that B-1 cells comprise a variety of subsets explains the exponentially growing interest toward these cells in applied immunology and toxicology (Haro et al., 2019; Aziz et al., 2020; Halperin et al., 2022; She et al., 2022).
In this review, we describe the developmental origin(s) of B-1 cells. After reporting their homeostatic functions under healthy conditions, we explain how pathogens unleash B-1 cells from their inhibitory environment and lead to their activation. We then highlight their unexpected roles (beneficial or deleterious) in immune responses to contact sensitivity-inducing chemicals, endocrine disruptors, aryl hydrocarbon receptor (AHR) ligands, and reactive particles.
2 The development of B-1 cells
2.1 The two existing models
The precise ontogeny of B-1 cells is still a matter of debate. Two different models regarding the B-1 cell origin exist. First, the “lineage model” postulates that the development into B-1 or B-2 cells predominantly depends on their respective precursors: fetal neonate for B-1 cells or post-natal bone marrow for B-2 cells. In addition to this concept, the authors also proposed an alternative “selection model,” which suggests a B cell receptor (BCR)-related origin. These two models are presented in Figure 1.
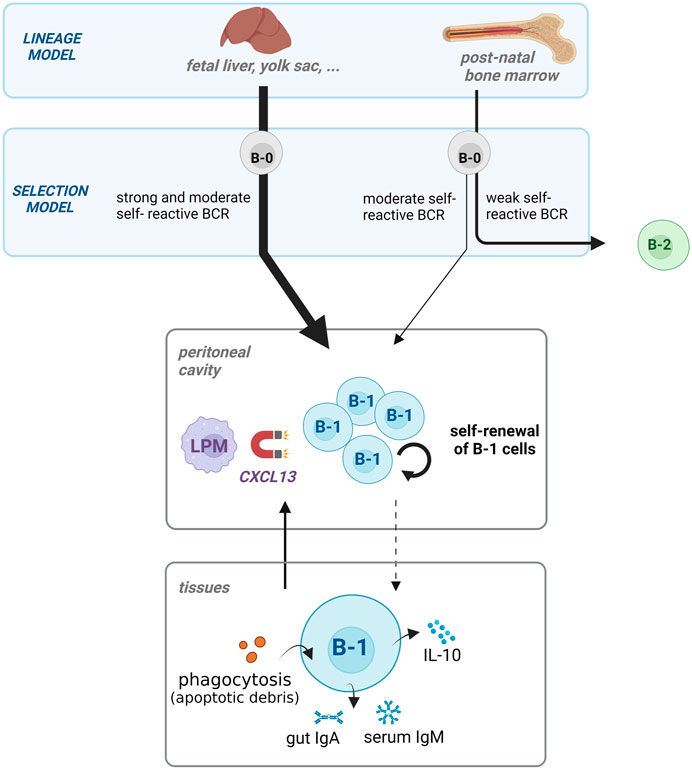
FIGURE 1. The development and function of B-1 cell pool during homeostasis. The development of B-1 cells occurs mostly in the fetus (lineage model). The bone marrow contributes only to a small addition of the B-1 cell pool in adults in contrast to B2 lymphocytes. The precursors (B-0) becoming B-1 cells have BCR with moderate or strong reactivity to self-antigens (selection model). B-1 cells are homed in the peritoneal cavity by CXCL13-releasing large peritoneal macrophages (LPM), where they self-renew. The three main functions of B-1 cells are apoptotic cell phagocytosis, IgM and IgA natural antibody production, and regulatory cytokine (IL-10) release, and contribute to maintaining homeostasis in the tissues.
B-1 and B-2 cells originate from precursors physically and chronologically distant in the lineage model. Indeed, B-1 cells are mainly produced during early life in the yolk sac, placenta, peritoneum and neonatal liver (Baumgarth, 2017; Luo et al., 2022). They are generated in waves from different sequential early precursors, which do not persist after birth (Montecino-Rodriguez and Dorshkind, 2012; Baumgarth, 2017). Self-renewal, not bone marrow, maintains the pool of B-1 cells throughout life (Murakami et al., 1995; Sawai et al., 2016). B-1 cells proliferate autonomously under homeostatic conditions, mainly via IgM-autocrine loops (Krop et al., 1996; Nguyen et al., 2015; Kobayashi et al., 2020). Although new and more precise experiments revealed rare B-1 cell precursors in the post-natal bone marrow (Esplin et al., 2009; Ghosn et al., 2012), they do not significantly contribute to the pool of B-1 cells in adults (Luo et al., 2022). Nevertheless, while this model is mostly right, this discovery brings more nuance to the lineage model.
In the alternative “selection model,” B-1 and B-2 cells share a common B-cell precursor (B-0). B-0 becomes one or the other, according to the BCR on its surface. This model was supported by Graf et al. (2019) who used an inducible transgenic system to swap the BCR of mature B-2 and B-1 cells. Their study demonstrated that expressing a B-1 cell BCR is sufficient to efficiently transform a mature B-2 cell into a B-1 cell. Indeed, B-1 cell-BCRs are very similar (Kantor et al., 1997; Vale et al., 2015) and most B-1 cells target similar self-antigens (Tsuji et al., 2020). Thus, strong and intermediate BCR-signaling (i.e., via self-antigen recognition) on B-0 leads to the development of B-1 cells (Hardy, 2006; Ghosh et al., 2022). While B-1 cells undergo this “positive selection,” B-2 cells follow the opposite trend and are derived from B-0s which are weakly self-reactive.
These two models were initially thought to be mutually exclusive but tend to converge based on new findings. The idea of “layered immunity” indicates that different rules controlling the immunity apply at different ages (Tornberg and Holmberg, 1995; Budeus et al., 2021). This concept comes from the different constraints faced before and after birth. B-2 cells are generated throughout life to produce antibodies that recognize a vast diversity of antigens, notably to fight infectious agents. These features are not shared by B-1 cells, whose antibodies often target the same self-antigens. The new notion of “window of opportunities” explains that the unique origin of B-1 cells, in different waves in the early life, confers them relaxed rules of immune tolerance, including positive selection in response to self-antigens (Hardy and Hayakawa, 2015; Wong et al., 2019). The B-1 cell-reactivities that emerge during this window are still somehow regulated. Indeed, there is a remarkable similarity of the BCR-segments used by B-1 cells (Rowley et al., 2007), amplified by the fetal absence of the enzyme necessary to diversify the BCR (Kantor et al., 1997).
2.2 Mechanisms inhibiting B-1 cell activation
B-1 cells are reactive to self-antigens and can be activated independently of other cells, as opposed to the T cell-dependent activation of B-2 cells (Martin et al., 2001; Alugupalli et al., 2004). Thus, B-1 cells require inhibitory mechanisms to regulate their spontaneous activation. Their localization plays a major role in their response and is regulated by macrophages (Figure 1). The peritoneum acts as a reservoir and an inhibitory environment for B-1 cells (Kawahara et al., 2003; Sindhava et al., 2010; Choi et al., 2012). Large peritoneal macrophages (LPMs) release abundant CXCL13, which is crucial for the localization of B-1 cells (Ansel et al., 2002). The LPMs retain B-1 cells via CXCL-13-mediated homing, as this cavity is closed from the circulation (Okabe and Medzhitov, 2014). Thus, retaining them in the mesothelial cavities decreases the likelihood of B-1 cells encountering activating signals.
Other mechanisms have also been implicated in controlling B-1 cell activation and antibody release. To produce antibodies, B-1 cells require simultaneous toll-like receptor (TLR) and BCR signaling (Sindhava et al., 2010; Sindhava and Bondada, 2012; Alhakeem et al., 2015). Only certain TLR signaling pathways collaborate with precise B-1-cell BCRs (Genestier et al., 2007; Savage et al., 2019). The binding of nucleic acids to endosomal TLR and phospholipids to BCRs demonstrate a pair of TLR and BCR signals that synergize. These mediators are both present under homeostatic conditions during cell death and mediate B-1 cell-produced anti-phospholipid antibodies in the steady state (Kreuk et al., 2019). Thus, B-1 cell-related antibody production is triggered by innate signals and is not strictly dependent on pathogens. In germ-free mice, most natural antibodies are produced by the spleen- and bone marrow-resident B-1 cells (Choi et al., 2012). These microenvironments contain a low number and frequency of B-1 cells, whose activation is notably regulated via CXCR4+ Tregs (Stoermann et al., 2007; Elias et al., 2022). Similar to T cells, B-1 cells express the inhibitory surface markers CD5 (Berland and Wortisoorelbeke, 2002; Smith and Baumgarth, 2019), PD-1 (Blevins et al., 2021; Zhou et al., 2022), and CTLA-4 (Yang et al., 2021) to regulate their activation.
3 B-1 cells in healthy conditions
B-1 cells perform multiple housekeeping functions during the steady state. They are responsible for the efficient clearance and immune tolerance of cellular debris during fetal life and in adults (Parra et al., 2012; Miles et al., 2018). Indeed, B-1 cells release T-independent antibodies targeting apoptotic cells, phagocytose debris, and release anti-inflammatory cytokines (Ogden et al., 2005). The B1 functions during steady state described in the text below are also illustrated at the bottom of the Figure 1.
3.1 Natural antibodies
B-1 cells are already present and are active before the development of adaptive immunity. They produce natural antibodies with low affinity and poly-specificity (recognizing multiple antigens), which serve as a ready-made arsenal to protect the newborn (Baumgarth et al., 2005; Ordoñez et al., 2018). The main isotype of natural antibodies is immunoglobulin M (IgM). The vast majority (>80%) of natural serum IgM is produced by B-1 cells (Choi and Baumgarth, 2008; Choi et al., 2012). B-1 cells are also capable of class switching to produce IgA (up to 50% of physiological serum IgA) and other isotypes (Baumgarth et al., 1999; Baumgarth et al., 2005). B-1 cells in the intestinal lamina propria (Okabe and Medzhitov, 2014; New et al., 2020) represent up to half of the physiological gut IgA-producing cells (Kroese et al., 1989).
Another characteristic of B-1 cell-produced antibodies is their preferential targeting of non-protein antigens, such as lipids (i.e., cardiolipin, phosphorylcholine (PC), oxidized low-density lipoprotein (oxLDL) (Shaw et al., 2000; Ordoñez et al., 2018) and nucleotides (Enghard et al., 2010). Additionally, these are “altered-” or “neo-” self-antigens. Although intracellular in healthy conditions, they change conformation and become available on “unwanted” cells, including apoptotic cells. Altered self-antigens function as tags to facilitate clearance of immunogenic cells and cellular debris (Navratil et al., 2004; Eggleton et al., 2008). For example, B-1 cells are the main producers of natural anti-PC antibodies (Mercolino et al., 1988; Masmoudi et al., 1990). This altered self-antigen becomes exposed during cell death, notably on the membrane of lysed-red blood cells (RBC) (Cox and Hardy, 1985). Natural IgM specific for altered self-antigen recognize cells during early apoptosis (Mercolino et al., 1988; Kim, 2010) and promote local deposition of “eat-me signals” (C1q and mannose-binding lectin) for their rapid clearance (Ogden et al., 2005; Chen et al., 2009).
3.2 Phagocytosis
B-1 cells share several characteristics with macrophages. Both cell types express myeloid cell markers, such as F4/80, class II major histocompatibility complex molecules (MHC II), and CD80/CD86 (Popi et al., 2009a; Parra et al., 2012; Popi, 2015). The phagocytic ability of B-1 cells is greater than that of any other B cells. While B-2 cells can uptake pathogens via internalization of the antigen-BCR complex, B-1 cells are professional phagocytes (Gao et al., 2012) competent to engulf large bodies (>0.5 µm), including apoptotic debris through actin polymerization (Brito et al., 2010; Parra et al., 2012).
3.3 Interleukin-10 production
B-1 cells are the main source of B-cell-produced interleukin-10 (IL-10) in homeostatic conditions (O'Garra et al., 1992). The spontaneous release of IL-10 by peritoneal B-1 cells is not shared by splenic B-2 cells and has thus been extensively studied. Unlike B-2 cells, separate TLR or BCR signaling promotes IL-10 release (Sindhava et al., 2010; Sindhava and Bondada, 2012; Alhakeem et al., 2015). IL-10 signaling prevents B-1 cell activation, blocks the cell cycle, and prevents IgM release (Alhakeem et al., 2015; O'Garra et al., 1990). Thus, the spontaneous release of IL-10 maintains peritoneal B-1 cells in a state of slow proliferation and less IgM release. In addition to autocrine effects, IL-10 released from B-1 cells stimulates other cells to release IL-10. This cytokine helps maintaining tolerance, notably during physiologically occurring apoptosis (Miles et al., 2018).
4 B-1 cells in pathological conditions
In response to infection or inflammation, B-1 cells from the mesothelial cavities become activated (and often gain surface CD11b expression) (Ghosn et al., 2008) and migrate to the injury site and lymphoid organs. A fraction of the migrated B-1 cells remains undifferentiated and continues their three synergistic functions (natural antibody production, phagocytosis and IL-10 release) (Rauch et al., 2012). The remaining B-1 cells differentiate into different B-1 cell-derived cells (B1CD) (Lopes and Mariano, 2009). Differentiation signals have not yet been clearly determined. However, it has been established that the pool of B-1 cells adapts to the pathogen under inflammatory conditions (Patel and Kearney, 2015; New et al., 2020). Thus, whether studied at a given time or over a lifetime, the B-1 cell subset contains cells with variations in their functions/specificities, as explained below and in Figure 2 (Halperin et al., 2022).
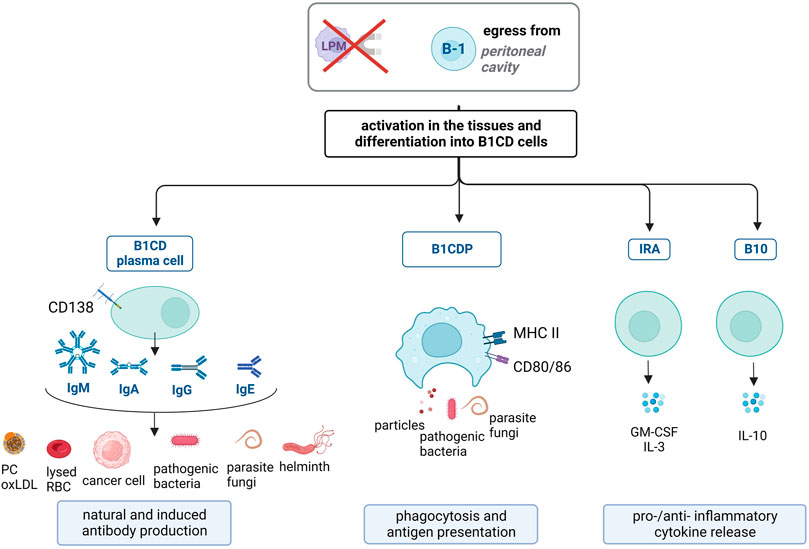
FIGURE 2. B-1 cell responses in pathological conditions. Under pathological conditions, B-1 cells leave mesothelial cavities and get activated. They differentiate into B-1 cell-derived (B1CD) cells, comprising four subpopulations. B1CD plasma cells are CD138+ and release natural antibodies (mostly IgM, with low specificity to (self-)antigens, including phosphorylcholine (PC) and oxLDL present on apoptotic cells, lysed red blood cells (RBC) and cancer cells) and induced antibodies (IgM, IgA, IgG, and IgE, with high specificity to antigens, including pathogens). B1CD phagocytes (B1CDP) are phenotypically similar to macrophages, phagocytose and present antigens to T cells via MHC II and CD80/CD86. Innate response activator (IRA) release pro-inflammatory GM-CSF and IL-3. B10 cells exclusively release immunosuppressive IL-10.
4.1 Natural and induced antibody-producing B1CD cells
Natural antibodies produced by B-1 cells under homeostatic conditions are beneficial for fighting infection. While natural antibodies target altered-self antigens on apoptotic cells, they also help clear pathogens (Ordoñez et al., 2018; Webster et al., 2022) and tumor antigens (Díaz-Zaragoza et al., 2015; Haro et al., 2019). Indeed, natural antibodies are non-specific and possess low affinity for their antigens. Hence, these poly-specific antibodies take advantage of the resemblance of epitopes, such as PC on both damaged cells and pathogens (Patel and Kearney, 2015). B-1 cells ensure first-line defense because their natural antibodies are already present in the blood and quickly bind to pathogens during early infection (Haas et al., 2005; Smith and Baumgarth, 2019). During infection, the levels of natural antibodies remain stable in the serum, as they are produced independently of pathogens (Baumgarth et al., 1999). However, some B-1 cells quickly migrate from the peritoneal cavity to the infected zone and secondary lymphoid organs (Choi and Baumgarth, 2008; Russo and Mariano, 2010). The lack of T-cell dependency allows B-1 cells to rapidly release high levels of natural antibodies locally (Baumgarth et al., 1999; Ordoñez et al., 2018). Some B-1 cells differentiate into CD138+ B-1 cell-derived plasma cells (B1CD plasma cells) to release even higher amounts of (poly-specific) natural IgM (Yang et al., 2007; Choi and Baumgarth, 2008; Holodick et al., 2014; Savage et al., 2017; Ordoñez et al., 2018). Natural anti-PC antibodies, produced by B-1 cells and B1CD plasma cells, have been well characterized and shown to be protective against the development of infections with helminths (Ubeira et al., 1987), bacteria (such as Streptococcus pneumoniae (Briles et al., 1982; Haas et al., 2005) and mycobacteria (Ordoñez et al., 2018).
Moreover, B1CD plasma cells also release “induced antibodies” or “pathogen-specific antibodies” in response to infection. A fraction of migrated B-1 cells undergo affinity maturation, increasing their specificity to the antigen (Chen et al., 2003; Alugupalli et al., 2004) and class-switch toward IgA (Kroese et al., 1989; New et al., 2020), IgG (Hirose et al., 1993; Chen et al., 2003) and IgE (Oliveira et al., 2005; Ghosh et al., 2012). These induced antibodies represent new additions to the B-1 cell repertoire. Antigen-specific B1CD plasma cells act as memory B-1 cells (Choi and Baumgarth, 2008; Smith and Baumgarth, 2019) and provide long-term protection (Cole et al., 2009). After the resolution of the activating signal, B1CD plasma and B-1 cells migrate back into the body cavities via CXCL13-mediated homing (Ansel et al., 2002; Ghosn et al., 2008). Repeated or prolonged activation of B-1 cells and migration out of the peritoneal cavity lead to expansion, affinity maturation or class-switching to IgG (Murakami et al., 1997; Ishikawa and Matsushima, 2007; Enghard et al., 2010). In older individuals, B-1 cells are less likely to spontaneously release low-affinity natural autoIgM because a fraction of them have adapted to pathogens and now release high-affinity IgG antibodies (Holodick et al., 2016; Rodriguez-Zhurbenko et al., 2019; Luo et al., 2022). It seems that B-1 cells also develop adaptive immune responses to cell death and apoptosis. In some cases, B-1 cells release harmful antibodies against lysed red blood cells (RBCs) that no longer clear apoptotic debris but instead destroy healthy RBC, leading to autoimmune hemolytic anemia (Murakami et al., 1992; Murakami et al., 1995; Murakami and Honjo, 1996; Dei Zotti et al., 2021). These pathological autoantibodies can activate the complement pathway, Fc receptor-mediated phagocytosis, or agglutination of healthy RBCs (Stahl et al., 2000).
4.2 B-1 cell-derived phagocytes (B1CDP)
Although B-1 cells phagocytose apoptotic cells and pathogens (Brito et al., 2010; Gao et al., 2012), their phagocytic ability is even stronger after differentiation into B1CDP (Figure 2). The uptake of pathogens by B-1 cells can be antigen-independent, similar to macrophages (Parra et al., 2012), or antigen-dependent. The latter is preferentially used by B-1 cells (Cruz-Leal et al., 2014; Vo et al., 2014) and serves during antigen presentation and differentiation into B1CDP (Popi et al., 2016). B-1 cells then use two different receptors to bind and phagocytose pathogens. Antigen recognition via BCR, when combined with TLR signaling, leads to internalization of the antigen–BCR complex and phagocytosis of the pathogen (Mussalem et al., 2012; Parra et al., 2012). Antigen peptides are then processed in the phagolysosome to be presented via MHC II (see below) (Gao et al., 2012; Vo et al., 2014; Yang et al., 2021).
While B-1 cells using this type of phagocytosis transiently lose IgM-BCR surface expression during internalization (Yang et al., 2021), some B-1 cells proceed further and progressively downregulate B cell-associated genes (Popi et al., 2009a). Besides the low expression of CD19 and IgM, they become morphologically and phenotypically similar to monocyte-derived macrophages (Almeida et al., 2001; Popi et al., 2012). Like in macrophages, B1CDP phagocytosis becomes predominantly antigen-independent (Vo et al., 2014). B1CDP migrate from the peritoneum to the infection/inflammation site, where they ingest apoptotic debris, particles, parasites, and bacteria for their rapid clearance (Almeida et al., 2001; Popi et al., 2009b; Popi et al., 2012; Arcanjo et al., 2015).
In response to persistent foreign bodies, macrophages form compact cellular aggregates and granulomas to isolate foreign bodies from the surrounding tissues (Pagan and Ramakrishnan, 2018). In addition to macrophages, B-1 cells are also crucial for granuloma formation. After mycobacterium infection, B-1 cells migrate to the infected lungs to promote compact granuloma formation, whereas the absence of B-1 cells (Xid mouse model) leads to diffused, rather than localized, lung lesions (Russo and Mariano, 2010). B-1 cells collaborate with macrophages to drive granuloma formation, as illustrated using an in vitro model of Paracoccidioides brasiliensis exposure (Vigna et al., 2006). Further demonstrating their similar phenotype and functions, B-1 cells migrate to the foreign body and can be found inside granulomas, then become B1CDP and fuse with macrophages to form plurinucleated giant cells (Almeida et al., 2001). By sharing their cytoplasm, fused cells create longer pseudopodia and phagocytose larger foreign bodies (Milde et al., 2015).
4.3 Antigen-presenting functions of B1CDP and B-1 cells
T cell activation requires two synergizing signals: one from the ligation of the T cell receptor (TCR) with the antigen-bearing MHC and the second from the ligation of T cell-costimulatory molecules, such as CD28 with either CD80 (B7-1) or CD86 (B7-2). Dendritic cells usually serve as MHC and CD80/CD86+ antigen-presenting cells (den Haan et al., 2014). Compared to B-2 cells, B-1 cells express high levels of all these costimulatory molecules (CD80, CD86, CD24, LFA-1, and ICAM-1) as well as MHC II (Mohan et al., 1998; Vigna et al., 2002; Parra et al., 2012) (Figure 2). This expression is constitutive and increases after exposure to pathogens, notably while B-1 cells differentiate into B1CDP (Mussalem et al., 2012).
B-1 cells in the peritoneum and lymphoid organs have high antigen presentation activity to T cells (Mohan et al., 1998; Parra et al., 2012). It has been reported that B-1 cells mediate the differentiation of most T cells, including Th17, Th1, Treg, Th2 and PD-1+ T cells (Zhong et al., 2007; Margry et al., 2014; Enyindah-Asonye et al., 2019). The signals that determine the direction of T cell-differentiation are not yet known. The egress of MHC II-positive B-1 cells outside the peritoneal inhibitory environment in the T cell-enriched thymus and spleen is suspected to be implicated in the activation of autoreactive T cells, leading to excessive inflammation and autoantibody production (Ishikawa and Matsushima, 2007; Pfau et al., 2014a; Pfau et al., 2014b). In their review on the antigen-presenting ability of B-1 cells, Popi et al. mentioned that B-1 cells prime virgin T cells, while B-2 cells re-activate memory T cells (Popi et al., 2016).
4.4 Innate response activator (IRA) cells and B-1 cell-derived B10 cells
After lipopolysaccharide (LPS) injection, B-1 cells from mesothelial cavities migrate to the injection site and secondary lymphoid organs, and some differentiate into IRA cells. Unlike B-1 cells, IRA cells release high amounts of granulocyte-macrophage colony-stimulating factor (GM-CSF) and IL-3 to activate innate immune cells (Rauch et al., 2012; Chousterman and Swirski, 2015) (Figure 2). They also maintain their own activation, as they are both the producers and targets of GM-CSF. This cytokine increases survival, proliferation, and differentiation of dendritic cells, monocytes, and macrophages, which in turn release pro-inflammatory cytokines and activate T cells and Th1 responses (Hilgendorf et al., 2014). IRA cells are implicated in inflammatory diseases, including multiple sclerosis (MS) (Li et al., 2015), sepsis-induced cytokine storms (Rauch et al., 2012), and atherosclerosis (Hilgendorf et al., 2014). In addition, IRA cells are CD138+ cells and release IgM. In the case of bacterial infection, IRA cells locally release opsonizing IgM, which is sufficient to confer survival to infected hosts (Rauch et al., 2012; Weber et al., 2014). IRA cells are also present in human tonsils and probably act as the first line of defense against upper respiratory tract infection (Chiappini et al., 2015).
B-1 cells exhibit immunosuppressive activity via antigen-presenting function and spontaneous IL-10 release (Margry et al., 2014; Dasgupta et al., 2020). B-1 cells differentiate into B10 cells (Matsushita et al., 2016; Ran et al., 2020) in response to innate signals that combine both TLR and BCR- or CD40-mediated activation (Yanaba et al., 2009; Dasgupta et al., 2020). B10 cells almost exclusively release IL-10 (Yanaba et al., 2009) This secretion impedes the concomitant release of IgM in B10 cells (Maseda et al., 2012; Tedder, 2015). In cases of infection or inflammation, B10 cells expand and migrate to the injury site or secondary lymphoid organs to dampen inflammation and promote Treg accumulation, as observed in experimental autoimmune encephalomyelitis (Matsushita et al., 2010), Listeria monocytogenes infection (Horikawa et al., 2013), allergy (Matsushita et al., 2016) and other pathologies [reviewed in (DiLillo et al., 2010)].
5 B-1 cell responses to reactive chemicals and particles
The implication of B-1 cells in pathogen-mediated diseases has been studied since their discovery. Thus, sufficient observations have allowed for the distinction between B-1 and B1CD cell responses to infections and inflammation. Understanding the implications of B-1 cells in response to chemical compounds and inhaled particles is still new in immunotoxicology and there is limited research regarding the responses of B-1 cells to pollutants. Nevertheless, we have highlighted this under-studied immune population and depicted their probable role in responses to reactive agents in the following text and Figure 3. Also, B-1 cells are often defined more by their function than by their panel of surface markers. Therefore, in the present section, all B-1 and B1CD cells are considered “B-1 cells”.
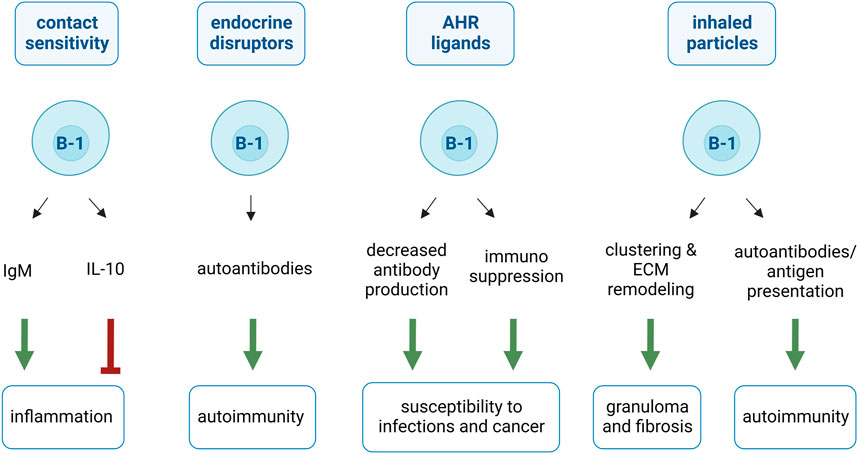
FIGURE 3. Proposed effects of B-1 cells in response to chemicals and particles. B-1 cells sense chemicals and particles and amplify (green arrows) or dampen (red arrow) associated diseases. B-1 cells are activated by contact sensitivity-inducing haptens, produce IgM and promote inflammatory pathology. B-1 cells also limit inflammatory responses by releasing anti-inflammatory cytokines (IL-10). B-1 cells promote autoimmunity by sensing endocrine disruptors and increasing their autoantibody production. AHR ligands reduce B-1 cell ability to secrete antibodies but favor their immunosuppressive activities. These two effects explain susceptibility to infection and cancer associated to AHR ligands. B-1 cells mediate inhaled particle-induced granuloma formation and fibrosis by clustering cells and particles and remodeling extracellular matrix (ECM). B-1 cells are also implicated in inhaled particle-induced autoimmune diseases by secreting autoantibodies and presenting self-antigens.
5.1 B-1 cells and allergic contact sensitivity (CS)
Contact sensitivity, also called skin allergy or allergic dermatitis, is a typical example of delayed-type hypersensitivity. It is mediated by effector T cells that induce an inflammatory reaction against exogenous or endogenous antigens. Other cells, such as monocytes, eosinophils, and neutrophils, are also involved (Vocanson et al., 2009). The team of Askenase found that B-1 cells are crucial in the development of CS in addition to T cells [reviewed in (Askenase, 2001)]. The pathology is attributable to B-1 cells and not to other types of B cells because the transfer of CS-B-1 cells is sufficient to induce CS in untreated mice (Itakura et al., 2005).
CS can be triggered by chemicals, such as trinitrophenyl chloride (TNP-Cl), oxazolone (OX), and fluorescein isothiocyanate (FITC) (Campos et al., 2006). After skin sensitization, some haptens are absorbed by the skin and bind, often covalently, skin proteins. The hapten-self protein conjugates constitute the neoantigens. Some of them are drained to the peritoneum (Askenase, 2001), where they encounter and activate B-1 cells (Itakura et al., 2005) alongside signals produced by activated NK T cells such as IL-5 (Itakura et al., 2013), IL-33 (Komai-Koma et al., 2011) or IL-4 (Askenase et al., 2005). Stimulated B-1 cells migrate to the spleen and lymph nodes via IL-4-related signaling and CXCL13 chemoattraction to produce IgM antibodies specific to the conjugates. This novel IgM circulates in the serum and leads to the recruitment of T cells in lymphoid organs within few hours (Mori et al., 2000; Askenase, 2001). Finally, T cells are activated in the spleen by dendritic cells, which are derived from Langerhans cells that have processed neoantigens in the skin. These T cells become CS-effector T cells and return to circulation 4 days after CS initiation (Askenase, 2001).
During the second encounter with the chemical, the induced IgM targeting anti-hapten/self-protein conjugate is already circulating and is thus rapidly found in the skin. IgM produced by B-1 cells activates the classical complement pathway and promotes recruitment of T cells, including CS-effector T cells, to the skin for induction of inflammation (Askenase et al., 1999; Tsuji et al., 2000; Askenase et al., 2015). Further demonstrating the crucial role of these B-1 cell-produced antibodies in the pathogenesis, injections with recombinant anti-hapten pentameric IgM trigger CS-symptoms in naïve mice (Paliwal et al., 2002) (Figure 3).
Alternatively, during the first exposure to a CS-inducing agent, some B-1 cells migrate to the spleen and differentiate into B10 cells. These cells release IL-10 to dampen T cell-mediated inflammatory responses and promote Treg expansion. The transfer of B-1 cell-derived B10 cells significantly attenuates CS responses (Yanaba et al., 2008; Matsushita et al., 2016). These contradictory findings strongly suggested that B-1 cells differentiate into deleterious antigen-specific IgM-releasing or beneficial B-1 cell-derived B10 cells (Figure 3).
5.2 B-1 cells and environmental estrogens
Estrogens enhance polyclonal B-cell activation and self-reactive B-cell survival (Fish, 2008). This is especially true for the B-1 cells. Estrogen receptors are highly expressed by the B-1 subset in older individuals compared to that in young B-1 and B-2 cells (Yurino et al., 2004; Diehl et al., 2011). Estrogens maintain the population of self-reactive B-1 cells, leading to an increased risk of autoantibody production in females, especially in older individuals (Figure 3) (Ansar Ahmed et al., 1989; Webster et al., 2022). Estrogen enhances the levels of anti-lysed RBC antibodies (Yurino et al., 2004). Interestingly, primary autoimmune hemolytic anemia, caused by pathological anti-RBC antibodies produced by B-1 cells, is most prevalent in aged and female individuals (Dei Zotti et al., 2021).
Endocrine disruptors (ED) or environmental estrogens found in plastics (bisphenol-A and phthalates), detergents, surfactants, pesticides and industrial chemicals (Tapiero et al., 2002) also activate B-1 cells. Schielen et al. (1995b) observed specific activation of B-1 cells in a rat model exposed to hexachlorobenzene (HCB). This ED is known to induce pathological skin lesions in human and rats (Michielsen et al., 1997; Michielsen et al., 1999). HCB treatment elevated the number of splenic IgM- and IgG-producing cells. Among B-cell subsets in the spleen of treated rats, only B-1 cells were expanded. The enhanced antibodies measured in the serum were similar to natural antibodies and characterized by their poly-reactivity to altered self-antigens including DNA and PC (Schielen et al., 1993; Schielen et al., 1995a). The significant correlation between IgM levels and skin lesion severity in HCB-treated animals strongly suggested a role of B-1 cells in the development of inflammatory lesions (Schielen et al., 1995b).
The impact of ED on B-1 cell-mediated autoimmunity has been evaluated in a lupus-prone model, NZB/NZW F1 mice. Progressive accumulation of splenic B-1 cells is observed in old NZB/NZW F1 mice. It leads to pathological anti-DNA IgG deposition, resulting in immune complex formation (Ito et al., 2004; Ishikawa and Matsushima, 2007). These antibodies originate from natural anti-DNA IgM produced by B-1 cells (Enghard et al., 2010). The class-switch from beneficial IgM-to pathological IgG antibodies reactive to DNA can be modulated by sex-hormones and is accelerated by estrogens (Roubinian et al., 1978). Yurino et al. (2004) reported the effects of ED bisphenol-A and diethylstilbestrol on B-1 cells in ovarectomized NZB/NZW F1 mice. ED worsened immune complex deposition in the kidneys and significantly increased the levels of anti-DNA IgG in addition to anti-RBC antibodies. The number of splenic B-1 cells were not further increased by ED in this lupus-prone model. In vitro, environmental estrogens stimulate the release of IgM, including anti-DNA IgM, by B-1 cells, especially in old mice. This is not the case of B-2 cells, which release very little IgM and are not activated by these molecules (Yurino et al., 2004). It remains to be elucidated whether Ig class switching from IgM to IgG occurs in ED-exposed B-1 cells.
5.3 B-1 cells and the AHR
The AHR is a crucial barrier organ sensor (reviewed in (Cella and Colonna, 2015; Gutiérrez-Vázquez and Quintana, 2018). AHR is expressed by both innate and adaptive immune cells (Wang et al., 2020) as well as by tissue-specific cells, such as epithelial cells (Qian et al., 2022) and keratinocytes (Uberoi et al., 2021). This transcription factor modulates immune responses and favors either immunosuppressive responses or Th1/Th17 inflammation (Quintana et al., 2008; Duarte et al., 2013). It is an evolutionarily old intracellular receptor (Hahn et al., 2017) that acts as a transcription factor and integrates signals from various sources. AHR ligands include dietary chemicals [indole-3-carbinol (Kahalehili et al., 2020), polyphenols (Gouédard et al., 2004; Mohammadi-Bardbori et al., 2012), glucosinolates (Koper et al., 2020)], endogenous small chemicals [tryptophan and metabolites (Rannug et al., 1995; Zelante et al., 2013)], and other pollutants [dioxins (Faiad et al., 2016; Miret et al., 2016), polychlorobiphenyls (Farmahin et al., 2016; Liu et al., 2019) benzo [a] pyrene (Nakatsuru et al., 2004), and diesel exhaust particles (Weng et al., 2018)].
Peritoneal CD5+ B-1 cells are among the B cells that express AHR in greater levels (Villa et al., 2017). The impact of AHR signaling in B-1 cells was studied in a CD5+ murine B-cell lymphoma line (CH12.LX), often considered a B-1 cell model. CH12.LX cells express surface CD11b, MHC II, alpha-4-integrin, IgM, and B220 and lack B-2 cell-associated CD23 (Rasmussen and Pfau, 2012). AHR is greatly expressed in CH12.LX (Sulentic et al., 1998). 2,3,7,8-tetrachlorodibenzo-p-dioxin (TCDD) prevents CH12.LX from releasing antibodies in response to LPS (Sulentic et al., 1998). Follow-up studies reviewed by Sher and Monti demonstrated that TCDD-mediated AHR signaling suppresses pathways necessary for plasma cell differentiation (Sherr and Monti, 2013). Thus, B-1 cells may respond to AHR agonists by limiting the antibody release (Figure 3).
Recent studies on human samples presented similar results on the importance of AHR in B-1-like cells (Blevins et al., 2021; Zhou et al., 2022). Human CD5+ B cells resemble mouse B-1 cells, with innate-like cells releasing the vast majority of the natural IgM in an age-related manner. CD5+ B cells express more basal AHR than CD5− B cells, as well as upstream mediators of programmed death 1 and 2 (PD-1/-2) and its ligands (PDL-1 and PDL-2). Activation of PD-1 initiates signaling cascades that suppress cellular immune responses. It was also found that TCDD-mediated AHR activation on CD5+ B cells significantly increased surface PD-1 and inhibited IgM production. Additionally, lymphocyte-specific protein tyrosine kinase (LCK) is upregulated via AHR activation in these cells and further suppresses IgM release by acting on the same pathway (Blevins et al., 2021; Zhou et al., 2022). Interestingly, the increase in LCK has been implicated as a biomarker for the progression of chronic lymphoid leukemia. This cancer could originate from B-1 cells, as it is characterized by CD5+ B cell/macrophage bi-phenotype tumor cells (Majolini et al., 1998; Borrello and Phipps, 1999). These data suggest that B-1 cells may play a role in TCDD-induced immunosuppressive diseases, such as poor infection control and leukemia (Warren et al., 2000; Vorderstrasse et al., 2003; Gaspard et al., 2022) (Figure 3).
5.4 B-1 cells and particle-induced granuloma
B-1 cells have been implicated in the formation of granulomas triggered by inorganic foreign bodies. Granuloma-inducing particles [silica (Hiéronimus et al., 2021), carbon nanotubes (Hiéronimus et al., 2021) and asbestos (Pfau et al., 2014a; Pfau et al., 2014b)] specifically activate and lead to the migration of B-1 cells. They are recruited to and accumulate in the inflammatory site and lymphoid organ when granuloma formation is in progress. B-1 cells remain in these organs during particle-induced chronic responses (Pfau et al., 2014a; Pfau et al., 2014b; Hiéronimus et al., 2021). Mechanistically, a novel and peculiar property of B-1 cells has been demonstrated as they cluster granuloma-inducing particles. This property seems specific to B-1 cells and reactive particles, as it was not observed in B-2 cells or inert particles (Hiéronimus et al., 2021). Unlike macrophages, B-1 cells are resistant to the cytotoxicity of microparticles. This suggests that B-1 cells take advantage of their unique resistance to the toxicity of particles and cluster them, acting as a shield to protect the surrounding cells and avoid particle distribution in the tissue (Hiéronimus et al., 2021) (Figure 3).
B-1 cells also interact with their environment, cross-talk, and fuse with macrophages to form compact cellular aggregates in response to pathogens (Vigna et al., 2006; Russo and Mariano, 2010). This is also the case for particle-induced granuloma. Indeed, in response to silica particles, B cell-deficient mice (μMT-deficient mice) develop few cellular aggregates and deposit less collagen in their lungs, probably due to the loss of pro-fibrotic signals activating macrophages (Arras et al., 2006). B-1 cells collaborate with macrophages to form granuloma-like structures in vitro. Adding B-1 cells (and not B-2 cells) to macrophages increases the number and size of granuloma-like structures induced by carbon nanotubes (Hiéronimus et al., 2021). In addition, B-1 cells specifically stimulate macrophages to release tissue-inhibitor of metalloproteinase1 in fibrotic-granuloma-inducing conditions (Hiéronimus et al., 2021). Thus, B-1 cells promote both the formation and maturation of granulomas into fibrotic granulomas by acting on the macrophages (Figure 3).
5.5 B-1 cells and particle-induced autoimmunity
Occupational exposure to silica (Pollard, 2016; De Decker et al., 2018) and asbestos (Pfau et al., 2014a; Pfau et al., 2014b) is associated with a high risk of developing autoimmune diseases, such as systemic lupus erythematosus, rheumatoid arthritis, and systemic sclerosis. Considerable experimental data indicate that autoantibodies precede clinical diseases and play a critical role in pathogenesis (Pfau et al., 2011; Ludwig et al., 2017). Amphibole asbestos and silica exposure increases the number of autoantibodies targeting nuclear components (Pfau et al., 2005; Pfau et al., 2008; Foster et al., 2019) and alveolar macrophages during early apoptosis (Pfau et al., 2004; Blake et al., 2008). These autoantibodies are produced under homeostatic conditions by B-1 cells to remove dead and dying cells. Therefore, their elevated production likely results from the specific activation of B-1 cells in response to cytotoxic particles.
Indeed, in the early response to asbestos, peritoneal B-1 cells (and not B-2 cells) transiently lose alpha4 integrin-mediated adhesion, leading to their egress (Pfau et al., 2014a; Pfau et al., 2014b). B-1 cells migrate and accumulate in the injured zone and/or lymphoid organs (Ferro et al., 2014; Hiéronimus et al., 2021). The elevated proportion of B-1 cells in these organs persists for up to 8 months after exposure (Pfau et al., 2014a; Pfau et al., 2014b). Mechanistically, particles do not directly activate antibody production by CH12.LX B-1 cells. However, asbestos-exposed macrophages rapidly enhances spontaneous IgA and IgG production by CH12.LX B-1 cells (Rasmussen and Pfau, 2012). Similarly, peritoneal cells (rich in macrophages and B-1 cells (Ghosn et al., 2010)) exposed to asbestos ex vivo contained more IgA+ B-1 cells than those without exposure (Rasmussen and Pfau, 2012). The exact contribution of B-1 cells to the development or progression of particle-induced autoimmune disease needs to be investigated in more detail.
6 Summary
B-1 cells are mainly generated during fetal life and protect newborns against pathogens. B-1 cells are malleable and perform homeostatic functions, such as phagocytosis, natural antibody production, and IL-10 release. The pool of B-1 cells changes throughout life and even more after infection and exposure to chemicals/inorganic particles. B-1 cells can become autoantibody-producing cells (i.e., B-1 cell-derived plasma cells) or trigger granulomatous fibrosis (i.e., B1CDP), inflammation (i.e., IRA cells), or immunosuppression (i.e., B10). Possible ways to manipulate their functions to prevent the development of chemically induced or exacerbated pathologies remain unanswered. Finally, given the crucial roles of B-1 cells in this plethora of immune responses, they should not be overlooked when developing new in vitro models for predicting the toxicity of chemical compounds.
Author contributions
Conceptualization and writing of the manuscript: LH. Review and editing: FH.
Funding
This work was supported by the « Actions de Recherche Concertées, Fédération Wallonie-Bruxelles (ARC 19/24-098, CYTAID), « Fondation Contre le Cancer » (2019-219) and « European Commission under H2020 project » (874707, Eximious). FH is a Senior Research Associate with the FNRS, Belgium. LH is a PhD student at the UCLouvain with a bursary funded by the European Association of Insulation Manufacturers (EURIMA, Belgium).
Conflict of interest
The authors declare that the research was conducted in the absence of any commercial or financial relationships that could be construed as a potential conflict of interest.
Publisher’s note
All claims expressed in this article are solely those of the authors and do not necessarily represent those of their affiliated organizations, or those of the publisher, the editors and the reviewers. Any product that may be evaluated in this article, or claim that may be made by its manufacturer, is not guaranteed or endorsed by the publisher.
Abbreviations
BCR, B cell receptor; TCR, T cell receptor; TLR, Toll-like receptor; AHR, Aryl hydrocarbon receptor; LPM, Large peritoneal macrophages; PC, Phosphorylcholine; MHC II, Class II major histocompatibility complex molecules; GM-CSF, Granulocyte-macrophage colony-stimulating factor; B1CD cell, B-1 cell-derived cell; B1CDP, B-1 cell-derived phagocytes; IRA, Innate response activator; RBC, Red blood cell; CS, Contact sensitivity; ED, Endocrine disruptors; HCB, Hexachlorobenzene; TCDD, 2,3,7,8-tetrachlorodibenzo-p-dioxin.
References
Alhakeem, S. S., Sindhava, V. J., Mckenna, M. K., Gachuki, B. W., Byrd, J. C., Muthusamy, N., et al. (2015). Role of B cell receptor signaling in IL-10 production by normal and malignant B-1 cells. Ann. N. Y. Acad. Sci. 1362, 239–249. doi:10.1111/nyas.12802
Almeida, S. R., Aroeira, L. S., Frymuller, E., Dias, M. Â. A., Bogsan, C. S. B., Lopes, J. D., et al. (2001). Mouse B-1 cell-derived mononuclear phagocyte, a novel cellular component of acute non-specific inflammatory exudate. Int. Immunol. 13, 1193–1201. doi:10.1093/intimm/13.9.1193
Alugupalli, K. R., Leong, J. M., Woodland, R. T., Muramatsu, M., Honjo, T., and Gerstein, R. M. (2004). B1b lymphocytes confer T cell-independent long-lasting immunity. Immunity 21, 379–390. doi:10.1016/j.immuni.2004.06.019
Ansar Ahmed, S., Dauphinée, M. J., Montoya, A. I., and Talal, N. (1989). Estrogen induces normal murine CD5+ B cells to produce autoantibodies. J. Immunol. 142, 2647–2653. doi:10.4049/jimmunol.142.8.2647
Ansel, K. M., Harris, R. B., and Cyster, J. G. (2002). CXCL13 is required for B1 cell homing, natural antibody production, and body cavity immunity. Immunity 16, 67–76. doi:10.1016/s1074-7613(01)00257-6
Arcanjo, A. F., Larocque-De-Freitas, I. F., Rocha, J. D., Zamith, D., Costa-Da-Silva, A. C., Nunes, M. P., et al. (2015). The PGE2/IL-10 Axis determines susceptibility of B-1 cell-derived phagocytes (B-1CDP) to leishmania major infection. PLoS One 10, e0124888. doi:10.1371/journal.pone.0124888
Arras, M., Louahed, J., Simoen, V., Barbarin, V., Misson, P., van den Brûle, S., et al. (2006). B lymphocytes are critical for lung fibrosis control and prostaglandin E2 regulation in IL-9 transgenic mice. Am. J. Respir. Cell Mol. Biol. 34, 573–580. doi:10.1165/rcmb.2004-0383OC
Askenase, P. W., Bryniarski, K., Paliwal, V., Redegeld, F., Groot Kormelink, T., Kerfoot, S., et al. (2015). A subset of AID-dependent B-1a cells initiates hypersensitivity and pneumococcal pneumonia resistance. Ann. N. Y. Acad. Sci. 1362, 200–214. doi:10.1111/nyas.12975
Askenase, P. W., Itakura, A., Leite-De-Moraes, M. C., Lisbonne, M., Roongapinun, S., Goldstein, D. R., et al. (2005). TLR-dependent IL-4 production by invariant Valpha14+Jalpha18+ NKT cells to initiate contact sensitivity in vivo. J. Immunol. 175, 6390–6401. doi:10.4049/jimmunol.175.10.6390
Askenase, P. W., Kawikova, I., Paliwal, V., Akahira-Azuma, M., Gerard, C., Hugli, T., et al. (1999). A new paradigm of T cell allergy: Requirement for the B-1 cell subset. Int. Arch. Allergy Immunol. 118, 145–149. doi:10.1159/000024052
Askenase, P. W. (2001). Yes T cells, but three different T cells (alphabeta, gammadelta and NK T cells), and also B-1 cells mediate contact sensitivity. Clin. Exp. Immunol. 125, 345–350. doi:10.1046/j.1365-2249.2001.01619.x
Aziz, M., Brenner, M., and Wang, P. (2020). Therapeutic potential of B-1a cells in COVID-19. Shock (Augusta, Ga.) 54, 586–594. doi:10.1097/SHK.0000000000001610
Baumgarth, N. (2017). A hard(y) look at B-1 cell development and function. J. Immunol. 199, 3387–3394. doi:10.4049/jimmunol.1700943
Baumgarth, N., Herman, O. C., Jager, G. C., Brown, L., Herzenberg, L. A., and Herzenberg, L. A. (1999). Innate and acquired humoral immunities to influenza virus are mediated by distinct arms of the immune system. Proc. Natl. Acad. Sci. U. S. A. 96, 2250–2255. doi:10.1073/pnas.96.5.2250
Baumgarth, N., Tung, J. W., and Herzenberg, L. A. (2005). Inherent specificities in natural antibodies: A key to immune defense against pathogen invasion. Springer Seminars Immunopathol. 26, 347–362. doi:10.1007/s00281-004-0182-2
Berland, R., and Wortisoorelbeke, H. H. (2002). Origins and functions of B-1 cells with notes on the role of CD5. Annu. Rev. Immunol. 20, 253–300. doi:10.1146/annurev.immunol.20.100301.064833
Blake, D. J., Wetzel, S. A., and Pfau, J. C. (2008). Autoantibodies from mice exposed to Libby amphibole asbestos bind SSA/Ro52-enriched apoptotic blebs of murine macrophages. Toxicology 246, 172–179. doi:10.1016/j.tox.2008.01.008
Blevins, L. K., Zhou, J., Crawford, R. B., and Kaminski, N. E. (2021). Identification of a sensitive human immunological target of aryl hydrocarbon receptor activation: CD5(+) innate-like B cells. Front. Immunol. 12, 635748. doi:10.3389/fimmu.2021.635748
Borrello, M. A., and Phipps, R. P. (1999). Fibroblast-secreted macrophage colony-stimulating factor is responsible for generation of biphenotypic B/macrophage cells from a subset of mouse B lymphocytes. J. Immunol. 163, 3605–3611. doi:10.4049/jimmunol.163.7.3605
Briles, D. E., Forman, C., Hudak, S., and Claflin, J. L. (1982). Anti-phosphorylcholine antibodies of the T15 idiotype are optimally protective against Streptococcus pneumoniae. J. Exp. Med. 156, 1177–1185. doi:10.1084/jem.156.4.1177
Brito, R. N. E., Cortez, B., Machado-Santelli, G., Xander, P., De Lorenzo, B., Oliveira, H., et al. (2010). In vitro and in vivo phagocytic ability of mouse B-1 cells. Immunol. Immunogenetics Insights 2, S6156. doi:10.4137/iii.s6156
Budeus, B., Kibler, A., Brauser, M., Homp, E., Bronischewski, K., Ross, J. A., et al. (2021). Human cord blood B cells differ from the adult counterpart by conserved Ig repertoires and accelerated response dynamics. J. Immunol. 206, 2839–2851. doi:10.4049/jimmunol.2100113
Campos, R. A., Szczepanik, M., Lisbonne, M., Itakura, A., Leite-De-Moraes, M., and Askenase, P. W. (2006). Invariant NKT cells rapidly activated via immunization with diverse contact antigens collaborate in vitro with B-1 cells to initiate contact sensitivity. J. Immunol. 177, 3686–3694. doi:10.4049/jimmunol.177.6.3686
Cancro, M. P., and Tomayko, M. M. (2021). Memory B cells and plasma cells: The differentiative continuum of humoral immunity. Immunol. Rev. 303, 72–82. doi:10.1111/imr.13016
Cella, M., and Colonna, M. (2015). Aryl hydrocarbon receptor: Linking environment to immunity. Seminars Immunol. 27, 310–314. doi:10.1016/j.smim.2015.10.002
Chen, M., Aosai, F., Norose, K., Mun, H. S., and Yano, A. (2003). The role of anti-HSP70 autoantibody-forming V(H)1-J(H)1 B-1 cells in Toxoplasma gondii-infected mice. Int. Immunol. 15, 39–47. doi:10.1093/intimm/dxg004
Chen, Y., Khanna, S., Goodyear, C. S., Park, Y. B., Raz, E., Thiel, S., et al. (2009). Regulation of dendritic cells and macrophages by an anti-apoptotic cell natural antibody that suppresses TLR responses and inhibits inflammatory arthritis. J. Immunol. 183, 1346–1359. doi:10.4049/jimmunol.0900948
Chiappini, N., Cantisani, R., Pancotto, L., Ruggiero, P., Rosa, D., Manetti, A., et al. (2015). Innate response activator (IRA) B cells reside in human tonsils and internalize bacteria in vitro. PLoS One 10, e0129879. doi:10.1371/journal.pone.0129879
Choi, Y. S., and Baumgarth, N. (2008). Dual role for B-1a cells in immunity to influenza virus infection. J. Exp. Med. 205, 3053–3064. doi:10.1084/jem.20080979
Choi, Y. S., Dieter, J. A., Rothaeusler, K., Luo, Z., and Baumgarth, N. (2012). B-1 cells in the bone marrow are a significant source of natural IgM. Eur. J. Immunol. 42, 120–129. doi:10.1002/eji.201141890
Chousterman, B. G., and Swirski, F. K. (2015). Innate response activator B cells: Origins and functions. Int. Immunol. 27, 537–541. doi:10.1093/intimm/dxv028
Cole, L. E., Yang, Y., Elkins, K. L., Fernandez, E. T., Qureshi, N., Shlomchik, M. J., et al. (2009). Antigen-specific B-1a antibodies induced by Francisella tularensis LPS provide long-term protection against F. tularensis LVS challenge. Proc. Natl. Acad. Sci. U. S. A. 106, 4343–4348. doi:10.1073/pnas.0813411106
Cox, K. O., and Hardy, S. J. (1985). Autoantibodies against mouse bromelain-modified RBC are specifically inhibited by a common membrane phospholipid, phosphatidylcholine. Immunology 55, 263–269.
Cruz-Leal, Y., Machado, Y., López-Requena, A., Canet, L., Laborde, R., Álvares, A. M., et al. (2014). Role of B-1 cells in the immune response against an antigen encapsulated into phosphatidylcholine-containing liposomes. Int. Immunol. 26, 427–437. doi:10.1093/intimm/dxu042
Dasgupta, S., Dasgupta, S., and Bandyopadhyay, M. (2020). Regulatory B cells in infection, inflammation, and autoimmunity. Cell. Immunol. 352, 104076. doi:10.1016/j.cellimm.2020.104076
De Decker, E., Vanthuyne, M., Blockmans, D., Houssiau, F., Lenaerts, J., Westhovens, R., et al. (2018). High prevalence of occupational exposure to solvents or silica in male systemic sclerosis patients: A Belgian cohort analysis. Clin. Rheumatol. 37, 1977–1982. doi:10.1007/s10067-018-4045-y
Dei Zotti, F., Qiu, A., La Carpia, F., Moriconi, C., and Hudson, K. E. (2021). A new murine model of primary autoimmune hemolytic anemia (AIHA). Front. Immunol. 12, 752330. doi:10.3389/fimmu.2021.752330
Den Haan, J. M. M., Arens, R., and Van Zelm, M. C. (2014). The activation of the adaptive immune system: Cross-talk between antigen-presenting cells, T cells and B cells. Immunol. Lett. 162, 103–112. doi:10.1016/j.imlet.2014.10.011
Díaz-Zaragoza, M., Hernández-Ávila, R., Viedma-Rodríguez, R., Arenas-Aranda, D., and Ostoa-Saloma, P. (2015). Natural and adaptive IgM antibodies in the recognition of tumor-associated antigens of breast cancer (Review). Oncol. Rep. 34, 1106–1114. doi:10.3892/or.2015.4095
Diehl, C. J., Barish, G. D., Downes, M., Chou, M. Y., Heinz, S., Glass, C. K., et al. (2011). Research resource: Comparative nuclear receptor atlas: Basal and activated peritoneal B-1 and B-2 cells. Mol. Endocrinol. 25, 529–545. doi:10.1210/me.2010-0384
Dilillo, D. J., Matsushita, T., and Tedder, T. F. (2010). B10 cells and regulatory B cells balance immune responses during inflammation, autoimmunity, and cancer. Ann. N. Y. Acad. Sci. 1183, 38–57. doi:10.1111/j.1749-6632.2009.05137.x
Duarte, J. H., Di Meglio, P., Hirota, K., Ahlfors, H., and Stockinger, B. (2013). Differential influences of the aryl hydrocarbon receptor on Th17 mediated responses in vitro and in vivo. PLoS One 8, e79819. doi:10.1371/journal.pone.0079819
Eggleton, P., Haigh, R., and Winyard, P. G. (2008). Consequence of neo-antigenicity of the ‘altered self. Rheumatology 47, 567–571. doi:10.1093/rheumatology/ken014
Elias, S., Sharma, R., Schizas, M., Valdez, I., Rampersaud, S., Park, S. M., et al. (2022). CXCR4+ Treg cells control serum IgM levels and natural IgM autoantibody production by B-1 cells in the bone marrow. J. Exp. Med. 219, e20220047. doi:10.1084/jem.20220047
Enghard, P., Humrich, J. Y., Chu, V. T., Grussie, E., Hiepe, F., Burmester, G.-R., et al. (2010). Class switching and consecutive loss of dsDNA-reactive B1a B cells from the peritoneal cavity during murine lupus development. Eur. J. Immunol. 40, 1809–1818. doi:10.1002/eji.200940050
Enyindah-Asonye, G., Nwankwo, A., Hogge, C., Rahman, M. A., Helmold Hait, S., Hunegnaw, R., et al. (2019). A pathogenic role for splenic B1 cells in SIV disease progression in rhesus macaques. Front. Immunol. 10, 511. doi:10.3389/fimmu.2019.00511
Esplin, B. L., Welner, R. S., Zhang, Q., Borghesi, L. A., and Kincade, P. W. (2009). A differentiation pathway for B1 cells in adult bone marrow. Proc. Natl. Acad. Sci. U. S. A. 106, 5773–5778. doi:10.1073/pnas.0811632106
Faiad, W., Hanano, A., Kabakibi, M. M., and Abbady, A. Q. (2016). Immuno-detection of dioxins using a recombinant protein of aryl hydrocarbon receptor (AhR) fused with sfGFP. BMC Biotechnol. 16, 51. doi:10.1186/s12896-016-0282-9
Farmahin, R., Crump, D., O'Brien, J. M., Jones, S. P., and Kennedy, S. W. (2016). Time-dependent transcriptomic and biochemical responses of 6-formylindolo[3,2-b]carbazole (FICZ) and 2,3,7,8-tetrachlorodibenzo-p-dioxin (TCDD) are explained by AHR activation time. Biochem. Pharmacol. 115, 134–143. doi:10.1016/j.bcp.2016.06.005
Ferro, A., Zebedeo, C. N., Davis, C., Ng, K. W., and Pfau, J. C. (2014). Amphibole, but not chrysotile, asbestos induces anti-nuclear autoantibodies and IL-17 in C57BL/6 mice. J. Immunotoxicol. 11, 283–290. doi:10.3109/1547691X.2013.847510
Fish, E. N. (2008). The X-files in immunity: Sex-based differences predispose immune responses. Nat. Rev. Immunol. 8, 737–744. doi:10.1038/nri2394
Foster, M. H., Ord, J. R., Zhao, E. J., Birukova, A., Fee, L., Korte, F. M., et al. (2019). Silica exposure differentially modulates autoimmunity in lupus strains and autoantibody transgenic mice. Front. Immunol. 10, 2336. doi:10.3389/fimmu.2019.02336
Gao, J., Ma, X., Gu, W., Fu, M., An, J., Xing, Y., et al. (2012). Novel functions of murine B1 cells: Active phagocytic and microbicidal abilities. Eur. J. Immunol. 42, 982–992. doi:10.1002/eji.201141519
Gaspard, E., Frenoy, P., Praud, D., Coudon, T., Grassot, L., Mancini, F., et al. (2022). Association between cumulative airborne dioxin exposure and chronic lymphocytic leukemia/non-hodgkin's lymphoma risk in a nested case-control study within the French E3N cohort. Blood 140, 7036–7037. doi:10.1182/blood-2022-166873
Genestier, L., Taillardet, M., Mondiere, P., Gheit, H., Bella, C., and Defrance, T. (2007). TLR agonists selectively promote terminal plasma cell differentiation of B cell subsets specialized in thymus-independent responses. J. Immunol. 178, 7779–7786. doi:10.4049/jimmunol.178.12.7779
Ghosh, D., Pham, T. D., Nanaware, P. P., Sengupta, D., Adler, L. N., Li, C. G., et al. (2022). Regulation of the BCR signalosome by the class II peptide editor, H2-M, affects the development and repertoire of innate-like B cells. Cell Rep. 38, 110200. doi:10.1016/j.celrep.2021.110200
Ghosh, S., Hoselton, S. A., and Schuh, J. M. (2012). μ-chain-deficient mice possess B-1 cells and produce IgG and IgE, but not IgA, following systemic sensitization and inhalational challenge in a fungal asthma model. J. Immunol. 189, 1322–1329. doi:10.4049/jimmunol.1200138
Ghosn, E. E. B., Cassado, A. A., Govoni, G. R., Fukuhara, T., Yang, Y., Monack, D. M., et al. (2010). Two physically, functionally, and developmentally distinct peritoneal macrophage subsets. Proc. Natl. Acad. Sci. U. S. A. 107, 2568–2573. doi:10.1073/pnas.0915000107
Ghosn, E. E. B., Yamamoto, R., Hamanaka, S., Yang, Y., Herzenberg, L. A., Nakauchi, H., et al. (2012). Distinct B-cell lineage commitment distinguishes adult bone marrow hematopoietic stem cells. Proc. Natl. Acad. Sci. 109, 5394–5398. doi:10.1073/pnas.1121632109
Ghosn, E., Yang, Y., Tung, J., Herzenberg, L., and Herzenberg, L. (2008). CD11b expression distinguishes sequential stages of peritoneal B-1 development. Proc. Natl. Acad. Sci. U. S. A. 105, 5195–5200. doi:10.1073/pnas.0712350105
Gouédard, C., Barouki, R., and Morel, Y. (2004). Dietary polyphenols increase paraoxonase 1 gene expression by an aryl hydrocarbon receptor-dependent mechanism. Mol. Cell Biol. 24, 5209–5222. doi:10.1128/MCB.24.12.5209-5222.2004
Graf, R., Seagal, J., Otipoby, K. L., Lam, K.-P., Ayoub, S., Zhang, B., et al. (2019). BCR-dependent lineage plasticity in mature B cells. Science 363, 748–753. doi:10.1126/science.aau8475
Gutiérrez-Vázquez, C., and Quintana, F. J. (2018). Regulation of the immune response by the aryl hydrocarbon receptor. Immunity 48, 19–33. doi:10.1016/j.immuni.2017.12.012
Haas, K. M., Poe, J. C., Steeber, D. A., and Tedder, T. F. (2005). B-1a and B-1b cells exhibit distinct developmental requirements and have unique functional roles in innate and adaptive immunity to S. pneumoniae. Immunity 23, 7–18. doi:10.1016/j.immuni.2005.04.011
Hahn, M. E., Karchner, S. I., and Merson, R. R. (2017). Diversity as opportunity: Insights from 600 million years of AHR evolution. Curr. Opin. Toxicol. 2, 58–71. doi:10.1016/j.cotox.2017.02.003
Halperin, S. T., T Hart, B. A., Luchicchi, A., and Schenk, G. J. (2022). The forgotten brother: The innate-like B1 cell in multiple sclerosis. Biomedicines 10, 606. doi:10.3390/biomedicines10030606
Hardy, R. R. (2006). B-1 B cell development. J. Immunol. 177, 2749–2754. doi:10.4049/jimmunol.177.5.2749
Hardy, R. R., and Hayakawa, K. (2015). Selection of natural autoreactive B cells. Clin. Exp. Rheumatol. 33, S80–S86.
Haro, M. A., Dyevoich, A. M., Phipps, J. P., and Haas, K. M. (2019). Activation of B-1 cells promotes tumor cell killing in the peritoneal cavity. Cancer Res. 79, 159–170. doi:10.1158/0008-5472.CAN-18-0981
Hiéronimus, L., Demazy, R., Christiaens, L., Uwambayinema, F., Geuens, J. F., Yacoub, Y., et al. (2021). Mouse innate-like B-1 lymphocytes promote inhaled particle-induced in vitro granuloma formation and inflammation in conjunction with macrophages. Arch. Toxicol. 96, 585–599. doi:10.1007/s00204-021-03200-2
Hilgendorf, I., Theurl, I., Gerhardt, L. M., Robbins, C. S., Weber, G. F., Gonen, A., et al. (2014). Innate response activator B cells aggravate atherosclerosis by stimulating T helper-1 adaptive immunity. Circulation 129, 1677–1687. doi:10.1161/CIRCULATIONAHA.113.006381
Hirose, S., Wakiya, M., Kawano-Nishi, Y., Yi, J., Sanokawa, R., Taki, S., et al. (1993). Somatic diversification and affinity maturation of IgM and IgG anti-DNA antibodies in murine lupus. Eur. J. Immunol. 23, 2813–2820. doi:10.1002/eji.1830231114
Holodick, N. E., Vizconde, T., Hopkins, T. J., and Rothstein, T. L. (2016). Age-related decline in natural IgM function: Diversification and selection of the B-1a cell pool with age. J. Immunol. 196, 4348–4357. doi:10.4049/jimmunol.1600073
Holodick, N. E., Vizconde, T., and Rothstein, T. L. (2014). Splenic B-1a cells expressing CD138 spontaneously secrete large amounts of immunoglobulin in naïve mice. Front. Immunol. 5, 129. doi:10.3389/fimmu.2014.00129
Horikawa, M., Weimer, E. T., Dilillo, D. J., Venturi, G. M., Spolski, R., Leonard, W. J., et al. (2013). Regulatory B cell (B10 Cell) expansion during Listeria infection governs innate and cellular immune responses in mice. J. Immunol. 190, 1158–1168. doi:10.4049/jimmunol.1201427
Ishikawa, S., and Matsushima, K. (2007). Aberrant B1 cell trafficking in a murine model for lupus. Front. Biosci. 12, 1790–1803. doi:10.2741/2188
Itakura, A., Ikutani, M., Takatsu, K., and Kikuchi, Y. (2013). Interleukin-5 plays a key role in mouse strain-dependent susceptibility to contact hypersensitivity through its effects on initiator B cells. Int. Arch. Allergy Immunol. 161 (2), 98–106. doi:10.1159/000350367
Itakura, A., Szczepanik, M., Campos, R. A., Paliwal, V., Majewska, M., Matsuda, H., et al. (2005). An hour after immunization peritoneal B-1 cells are activated to migrate to lymphoid organs where within 1 Day they produce IgM antibodies that initiate elicitation of contact sensitivity. J. Immunol. 175, 7170–7178. doi:10.4049/jimmunol.175.11.7170
Ito, T., Ishikawa, S., Sato, T., Akadegawa, K., Yurino, H., Kitabatake, M., et al. (2004). Defective B1 cell homing to the peritoneal cavity and preferential recruitment of B1 cells in the target organs in a murine model for systemic lupus erythematosus. J. Immunol. 172, 3628–3634. doi:10.4049/jimmunol.172.6.3628
Kahalehili, H. M., Newman, N. K., Pennington, J. M., Kolluri, S. K., Kerkvliet, N. I., Shulzhenko, N., et al. (2020). Dietary indole-3-carbinol activates AhR in the gut, alters Th17-microbe interactions, and exacerbates insulitis in NOD mice. Front. Immunol. 11, 606441. doi:10.3389/fimmu.2020.606441
Kantor, A. B., Merrill, C. E., Herzenberg, L. A., and Hillson, J. L. (1997). An unbiased analysis of V(H)-D-J(H) sequences from B-1a, B-1b, and conventional B cells. J. Immunol. 158, 1175–1186. doi:10.4049/jimmunol.158.3.1175
Kawahara, T., Ohdan, H., Zhao, G., Yang, Y.-G., and Sykes, M. (2003). Peritoneal cavity B cells are precursors of splenic IgM natural antibody-producing cells. J. Immunol. 171, 5406–5414. doi:10.4049/jimmunol.171.10.5406
Kim, J. (2010). Identification of a human monoclonal natural IgM antibody that recognizes early apoptotic cells and promotes phagocytosis. Hybridoma 29, 275–281. doi:10.1089/hyb.2010.0012
Kobayashi, M., Lin, Y., Mishra, A., Shelly, C., Gao, R., Reeh, C. W., et al. (2020). Bmi1 maintains the self-renewal property of innate-like B lymphocytes. J. Immunol. 204, 3262–3272. doi:10.4049/jimmunol.2000030
Komai-Koma, M., Gilchrist, D. S., Mckenzie, A. N., Goodyear, C. S., Xu, D., and Liew, F. Y. (2011). IL-33 activates B1 cells and exacerbates contact sensitivity. J. Immunol. 186, 2584–2591. doi:10.4049/jimmunol.1002103
Koper, J. E. B., Kortekaas, M., Loonen, L. M. P., Huang, Z., Wells, J. M., Gill, C. I. R., et al. (2020). Aryl hydrocarbon Receptor activation during in vitro and in vivo digestion of raw and cooked broccoli (brassica oleracea var. Italica). Food Funct. 11, 4026–4037. doi:10.1039/d0fo00472c
Kreuk, L. S., Koch, M. A., Slayden, L. C., Lind, N. A., Chu, S., Savage, H. P., et al. (2019). B cell receptor and Toll-like receptor signaling coordinate to control distinct B-1 responses to both self and the microbiota. Elife 8, e47015. doi:10.7554/eLife.47015
Kroese, F. G. M., Ammerlaan, W. A. M., and Deenen, G. J. (1992). Location and function of B-cell lineagesa. Ann. N. Y. Acad. Sci. 651, 44–58. doi:10.1111/j.1749-6632.1992.tb24592.x
Kroese, F. G. M., Butcher, E. C., Stall, A. M., Lalor, P. A., Adams, S., and Herzenberg, L. A. (1989). Many of the IgA producing plasma cells in murine gut are derived from self-replenishing precursors in the peritoneal cavity. Int. Immunol. 1, 75–84. doi:10.1093/intimm/1.1.75
Krop, I., De Fougerolles, A. R., Hardy, R. R., Allison, M., Schlissel, M. S., and Fearon, D. T. (1996). Self-renewal of B-1 lymphocytes is dependent on CD19. Eur. J. Immunol. 26, 238–242. doi:10.1002/eji.1830260137
Lebien, T. W., and Tedder, T. F. (2008). B lymphocytes: How they develop and function. Blood 112, 1570–1580. doi:10.1182/blood-2008-02-078071
Li, R., Rezk, A., Miyazaki, Y., Hilgenberg, E., Touil, H., Shen, P., et al. (2015). Proinflammatory GM-CSF-producing B cells in multiple sclerosis and B cell depletion therapy. Sci. Transl. Med. 7, 310ra166. doi:10.1126/scitranslmed.aab4176
Liu, H., Shi, L., Giesy, J. P., and Yu, H. (2019). Polychlorinated diphenyl sulfides can induce ROS and genotoxicity via the AhR-CYP1A1 pathway. Chemosphere 223, 165–170. doi:10.1016/j.chemosphere.2019.01.169
Lopes, J. D., and Mariano, M. (2009). B-1 cell: The precursor of a novel mononuclear phagocyte with immuno-regulatory properties. Acad Bras Cienc 81, 489–496. doi:10.1590/s0001-37652009000300013
Ludwig, R. J., Vanhoorelbeke, K., Leypoldt, F., Kaya, Z., Bieber, K., McLachlan, S. M., et al. (2017). Mechanisms of autoantibody-induced pathology. Front. Immunol. 8, 603. doi:10.3389/fimmu.2017.00603
Luo, Y., Wang, J., Li, K., Li, M., Xu, S., Liu, X., et al. (2022). Single-cell genomics identifies distinct B1 cell developmental pathways and reveals aging-related changes in the B-cell receptor repertoire. Cell and Biosci. 12, 57. doi:10.1186/s13578-022-00795-6
Majolini, M. B., D'Elios, M. M., Galieni, P., Boncristiano, M., Lauria, F., Del Prete, G., et al. (1998). Expression of the T-cell-specific tyrosine kinase Lck in normal B-1 cells and in chronic lymphocytic leukemia B cells. Blood 91, 3390–3396. doi:10.1182/blood.v91.9.3390
Marcos, M. A. R., Huetz, F., Pereira, P., Andreu, J.-L., Martinez, -A., C., and Coutinho, A. (1989). Further evidence for coelomic-associated b lymphocytes. Eur. J. Immunol. 19, 2031–2035. doi:10.1002/eji.1830191110
Margry, B., Kersemakers, S. C. W., Hoek, A., Arkesteijn, G. J. A., Wieland, W. H., Van Eden, W., et al. (2014). Activated peritoneal cavity B-1a cells possess regulatory B cell properties. PloS one 9, e88869. doi:10.1371/journal.pone.0088869
Martin, F., Oliver, A. M., and Kearney, J. F. (2001). Marginal zone and B1 B cells unite in the early response against T-independent blood-borne particulate antigens. Immunity 14, 617–629. doi:10.1016/s1074-7613(01)00129-7
Maseda, D., Smith, S. H., Dilillo, D. J., Bryant, J. M., Candando, K. M., Weaver, C. T., et al. (2012). Regulatory B10 cells differentiate into antibody-secreting cells after transient IL-10 production in vivo. J. Immunol. 188, 1036–1048. doi:10.4049/jimmunol.1102500
Masmoudi, H., Mota-Santos, T., Huetz, F., Coutinho, A., and Cazenave, P. A. (1990). All T15 Id-positive antibodies (but not the majority of VHT15+ antibodies) are produced by peritoneal CD5+ B lymphocytes. Int. Immunol. 2, 515–520. doi:10.1093/intimm/2.6.515
Matsushita, T., Horikawa, M., Iwata, Y., and Tedder, T. F. (2010). Regulatory B cells (B10 cells) and regulatory T cells have independent roles in controlling experimental autoimmune encephalomyelitis initiation and late-phase immunopathogenesis. J. Immunol. 185, 2240–2252. doi:10.4049/jimmunol.1001307
Matsushita, T., Le Huu, D., Kobayashi, T., Hamaguchi, Y., Hasegawa, M., Naka, K., et al. (2016). A novel splenic B1 regulatory cell subset suppresses allergic disease through phosphatidylinositol 3-kinase-Akt pathway activation. J. Allergy Clin. Immunol. 138, 1170–1182.e9. doi:10.1016/j.jaci.2015.12.1319
Mercolino, T. J., Arnold, L. W., Hawkins, L. A., and Haughton, G. (1988). Normal mouse peritoneum contains a large population of Ly-1+ (CD5) B cells that recognize phosphatidyl choline. Relationship to cells that secrete hemolytic antibody specific for autologous erythrocytes. J. Exp. Med. 168, 687–698. doi:10.1084/jem.168.2.687
Michielsen, C. C., Van Loveren, H., and Vos, J. G. (1999). The role of the immune system in hexachlorobenzene-induced toxicity. Environ. Health Perspect. 107 (5), 783–792. doi:10.1289/ehp.99107s5783
Michielsen, C. P., Bloksma, N., Ultee, A., Van Mil, F., and Vos, J. G. (1997). Hexachlorobenzene-induced immunomodulation and skin and lung lesions: A comparison between Brown Norway, lewis, and wistar rats. Toxicol. Appl. Pharmacol. 144, 12–26. doi:10.1006/taap.1997.8104
Milde, R., Ritter, J., Tennent, G. A., Loesch, A., Martinez, F. O., Gordon, S., et al. (2015). Multinucleated giant cells are specialized for complement-mediated phagocytosis and large target destruction. Cell Rep. 13, 1937–1948. doi:10.1016/j.celrep.2015.10.065
Miles, K., Simpson, J., Brown, S., Cowan, G., Gray, D., and Gray, M. (2018). Immune tolerance to apoptotic self is mediated primarily by regulatory B1a cells. Front. Immunol. 8, 1952. doi:10.3389/fimmu.2017.01952
Miret, N., Pontillo, C., Ventura, C., Carozzo, A., Chiappini, F., Kleiman De Pisarev, D., et al. (2016). Hexachlorobenzene modulates the crosstalk between the aryl hydrocarbon receptor and transforming growth factor-β1 signaling, enhancing human breast cancer cell migration and invasion. Toxicology 366-367, 20–31. doi:10.1016/j.tox.2016.08.007
Mohammadi-Bardbori, A., Bengtsson, J., Rannug, U., Rannug, A., and Wincent, E. (2012). Quercetin, resveratrol, and curcumin are indirect activators of the aryl hydrocarbon receptor (AHR). Chem. Res. Toxicol. 25, 1878–1884. doi:10.1021/tx300169e
Mohan, C., Morel, L., Yang, P., and Wakeland, E. K. (1998). Accumulation of splenic B1a cells with potent antigen-presenting capability in NZM2410 lupus-prone mice. Arthritis Rheum. 41, 1652–1662. doi:10.1002/1529-0131(199809)41:9<1652:AID-ART17>3.0.CO;2-W
Montecino-Rodriguez, E., and Dorshkind, K. (2012). B-1 B cell development in the fetus and adult. Immunity 36, 13–21. doi:10.1016/j.immuni.2011.11.017
Mori, M., Morris, S. C., Orekhova, T., Marinaro, M., Giannini, E. H., and Finkelman, F. D. (2000). IL-4 promotes the migration of circulating B cells to the spleen and increases splenic B cell survival. J. Immunol. 164, 5704–5712. doi:10.4049/jimmunol.164.11.5704
Murakami, M., and Honjo, T. (1996). Anti-red blood cell autoantibody transgenic mice: Murine model of autoimmune hemolytic anemia. Semin. Immunol. 8, 3–9. doi:10.1006/smim.1996.0002
Murakami, M., Nakajima, K., Yamazaki, K., Muraguchi, T., Serikawa, T., and Honjo, T. (1997). Effects of breeding environments on generation and activation of autoreactive B-1 cells in anti-red blood cell autoantibody transgenic mice. J. Exp. Med. 185, 791–794. doi:10.1084/jem.185.4.791
Murakami, M., Tsubata, T., Okamoto, M., Shimizu, A., Kumagai, S., Imura, H., et al. (1992). Antigen-induced apoptotic death of Ly-1 B cells responsible for autoimmune disease in transgenic mice. Nature 357, 77–80. doi:10.1038/357077a0
Murakami, M., Yoshioka, H., Shirai, T., Tsubata, T., and Honjo, T. (1995). Prevention of autoimmune symptoms in autoimmune-prone mice by elimination of B-1 cells. Int. Immunol. 7, 877–882. doi:10.1093/intimm/7.5.877
Mussalem, J. S., Squaiella-Baptistão, C. C., Teixeira, D., Yendo, T. M., Thies, F. G., Popi, A. F., et al. (2012). Adjuvant effect of killed Propionibacterium acnes on mouse peritoneal B-1 lymphocytes and their early phagocyte differentiation. PloS one 7, e33955. doi:10.1371/journal.pone.0033955
Nakatsuru, Y., Wakabayashi, K., Fujii-Kuriyama, Y., Ishikawa, T., Kusama, K., and Ide, F. (2004). Dibenzo[A,L]pyrene-induced genotoxic and carcinogenic responses are dramatically suppressed in aryl hydrocarbon receptor-deficient mice. Int. J. Cancer 112, 179–183. doi:10.1002/ijc.20365
Navratil, J. S., Sabatine, J. M., and Ahearn, J. M. (2004). Apoptosis and immune responses to self. Rheum. Dis. Clin. North Am. 30, 193–212. doi:10.1016/S0889-857X(03)00110-8
New, J. S., Dizon, B. L. P., Fucile, C. F., Rosenberg, A. F., Kearney, J. F., and King, R. G. (2020). Neonatal exposure to commensal-bacteria-derived antigens directs polysaccharide-specific B-1 B cell repertoire development. Immunity 53, 172–186.e6. doi:10.1016/j.immuni.2020.06.006
Nguyen, T. T. T., Elsner, R. A., and Baumgarth, N. (2015). Natural IgM prevents autoimmunity by enforcing B cell central tolerance induction. J. Immunol. 194, 1489–1502. doi:10.4049/jimmunol.1401880
O'Garra, A., Chang, R., Go, N., Hastings, R., Haughton, G., and Howard, M. (1992). Ly-1 B (B-1) cells are the main source of B cell-derived interleukin 10. Eur. J. Immunol. 22, 711–717. doi:10.1002/eji.1830220314
O'Garra, A., Stapleton, G., Dhar, V., Pearce, M., Schumacher, J., Rugo, H., et al. (1990). Production of cytokines by mouse B cells: B lymphomas and normal B cells produce interleukin 10. Int. Immunol. 2, 821–832. doi:10.1093/intimm/2.9.821
Ogden, C. A., Kowalewski, R., Peng, Y., Montenegro, V., and Elkon, K. B. (2005). IGM is required for efficient complement mediated phagocytosis of apoptotic cells in vivo. Autoimmunity 38, 259–264. doi:10.1080/08916930500124452
Okabe, Y., and Medzhitov, R. (2014). Tissue-specific signals control reversible program of localization and functional polarization of macrophages. Cell 157, 832–844. doi:10.1016/j.cell.2014.04.016
Oliveira, F. L., Aguiar, A. M., Borojevic, R., and El-Cheikh, M. C. (2005). IgE expression on the surface of B1 and B2 lymphocytes in experimental murine schistosomiasis. Braz J. Med. Biol. Res. 38, 1033–1042. doi:10.1590/s0100-879x2005000700006
Ordoñez, C., Savage, H. P., Tarajia, M., Rivera, R., Weeks-Galindo, C., Sambrano, D., et al. (2018). Both B-1a and B-1b cells exposed to Mycobacterium tuberculosis lipids differentiate into IgM antibody-secreting cells. Immunology 154, 613–623. doi:10.1111/imm.12909
Pagan, A. J., and Ramakrishnan, L. (2018). The Formation and function of granulomas. Annu. Rev. Immunol. 36, 639–665. doi:10.1146/annurev-immunol-032712-100022
Paliwal, V., Tsuji, R. F., Szczepanik, M., Kawikova, I., Campos, R. A., Kneilling, M., et al. (2002). Subunits of IgM reconstitute defective contact sensitivity in B-1 cell-deficient xid mice: Kappa light chains recruit T cells independent of complement. J. Immunol. 169, 4113–4123. doi:10.4049/jimmunol.169.8.4113
Parra, D., Rieger, A. M., Li, J., Zhang, Y.-A., Randall, L. M., Hunter, C. A., et al. (2012). Pivotal Advance: Peritoneal cavity B-1 B cells have phagocytic and microbicidal capacities and present phagocytosed antigen to CD4+ T cells. J. Leukoc. Biol. 91, 525–536. doi:10.1189/jlb.0711372
Patel, P. S., and Kearney, J. F. (2015). Neonatal exposure to pneumococcal phosphorylcholine modulates the development of house dust mite allergy during adult life. J. Immunol. 194, 5838–5850. doi:10.4049/jimmunol.1500251
Pfau, J. C., Brown, J. M., and Holian, A. (2004). Silica-exposed mice generate autoantibodies to apoptotic cells. Toxicology 195, 167–176. doi:10.1016/j.tox.2003.09.011
Pfau, J. C., Hurley, K., Peterson, C., Coker, L., Fowers, C., and Marcum, R. (2014a). Activation and trafficking of peritoneal B1a B-cells in response to amphibole asbestos. J. Immunotoxicol. 11, 90–98. doi:10.3109/1547691X.2013.796024
Pfau, J. C., Li, S., Holland, S., and Sentissi, J. J. (2011). Alteration of fibroblast phenotype by asbestos-induced autoantibodies. J. Immunotoxicol. 8, 159–169. doi:10.3109/1547691X.2011.562257
Pfau, J. C., Sentissi, J. J., Li, S. A., Calderon-Garcidueñas, L., Brown, J. M., and Blake, D. J. (2008). Asbestos-induced autoimmunity in C57Bl/6 mice. J. Immunotoxicol. 5, 129–137. doi:10.1080/15476910802085756
Pfau, J. C., Sentissi, J. J., Weller, G., and Putnam, E. A. (2005). Assessment of autoimmune responses associated with asbestos exposure in Libby, Montana, USA. Environ. Health Perspect. 113, 25–30. doi:10.1289/ehp.7431
Pfau, J. C., Serve, K. M., and Noonan, C. W. (2014b). Autoimmunity and asbestos exposure. Autoimmune Dis. 2014, 782045. doi:10.1155/2014/782045
Pollard, K. M. (2016). Silica, silicosis, and autoimmunity. Front. Immunol. 7, 97. doi:10.3389/fimmu.2016.00097
Popi, A. F. (2015). B-1 phagocytes: The myeloid face of B-1 cells. Ann. N. Y. Acad. Sci. 1362, 86–97. doi:10.1111/nyas.12814
Popi, A. F., Longo-Maugéri, I. M., and Mariano, M. (2016). An overview of B-1 cells as antigen-presenting cells. Front. Immunol. 7, 138. doi:10.3389/fimmu.2016.00138
Popi, A. F., Motta, F. L. T., Mortara, R. A., Schenkman, S., Lopes, J. D., and Mariano, M. (2009a). Co-ordinated expression of lymphoid and myeloid specific transcription factors during B-1b cell differentiation into mononuclear phagocytes in vitro. Immunology 126, 114–122. doi:10.1111/j.1365-2567.2008.02883.x
Popi, A. F., Osugui, L., Perez, K. R., Longo-Maugéri, I. M., and Mariano, M. (2012). Could a B-1 cell derived phagocyte "be one" of the peritoneal macrophages during LPS-driven inflammation? PloS one 7, e34570. doi:10.1371/journal.pone.0034570
Popi, A. F., Zamboni, D. S., Mortara, R. A., and Mariano, M. (2009b). Microbicidal property of B1 cell derived mononuclear phagocyte. Immunobiology 214, 664–673. doi:10.1016/j.imbio.2008.12.007
Qian, M., Liu, J., Zhao, D., Cai, P., Pan, C., Jia, W., et al. (2022). Aryl hydrocarbon receptor deficiency in intestinal epithelial cells aggravates alcohol-related liver disease. Cell Mol. Gastroenterol. Hepatol. 13, 233–256. doi:10.1016/j.jcmgh.2021.08.014
Quintana, F. J., Basso, A. S., Iglesias, A. H., Korn, T., Farez, M. F., Bettelli, E., et al. (2008). Control of Treg and TH17 cell differentiation by the aryl hydrocarbon receptor. Nature 453, 65–71. doi:10.1038/nature06880
Ran, Z., Yue-Bei, L., Qiu-Ming, Z., and Huan, Y. (2020). Regulatory B cells and its role in central nervous system inflammatory demyelinating diseases. Front. Immunol. 11, 1884. doi:10.3389/fimmu.2020.01884
Rannug, U., Rannug, A., Sjöberg, U., Li, H., Westerholm, R., and Bergman, J. (1995). Structure elucidation of two tryptophan-derived, high affinity Ah receptor ligands. Chem. Biol. 2, 841–845. doi:10.1016/1074-5521(95)90090-x
Rasmussen, D. L., and Pfau, J. C. (2012). Asbestos activates CH12.LX B-lymphocytes via macrophage signaling. J. Immunotoxicol. 9, 129–140. doi:10.3109/1547691X.2011.631953
Rauch, P. J., Chudnovskiy, A., Robbins, C. S., Weber, G. F., Etzrodt, M., Hilgendorf, I., et al. (2012). Innate response activator B cells protect against microbial sepsis. Science 335, 597–601. doi:10.1126/science.1215173
Rodriguez-Zhurbenko, N., Quach, T. D., Hopkins, T. J., Rothstein, T. L., and Hernandez, A. M. (2019). Human B-1 cells and B-1 cell antibodies change with advancing age. Front. Immunol. 10, 483. doi:10.3389/fimmu.2019.00483
Roubinian, J. R., Talal, N., Greenspan, J. S., Goodman, J. R., and Siiteri, P. K. (1978). Effect of castration and sex hormone treatment on survival, anti-nucleic acid antibodies, and glomerulonephritis in NZB/NZW F1 mice. J. Exp. Med. 147, 1568–1583. doi:10.1084/jem.147.6.1568
Rowley, B., Tang, L., Shinton, S., Hayakawa, K., and Hardy, R. R. (2007). Autoreactive B-1 B cells: Constraints on natural autoantibody B cell antigen receptors. J. Autoimmun. 29, 236–245. doi:10.1016/j.jaut.2007.07.020
Russo, R. T., and Mariano, M. (2010). B-1 cell protective role in murine primary Mycobacterium bovis bacillus Calmette-Guerin infection. Immunobiology 215, 1005–1014. doi:10.1016/j.imbio.2010.01.003
Savage, H. P., Kläsener, K., Smith, F. L., Luo, Z., Reth, M., and Baumgarth, N. (2019). TLR induces reorganization of the IgM-BCR complex regulating murine B-1 cell responses to infections. Elife 8, e46997. doi:10.7554/eLife.46997
Savage, H. P., Yenson, V. M., Sawhney, S. S., Mousseau, B. J., Lund, F. E., and Baumgarth, N. (2017). Blimp-1–dependent and –independent natural antibody production by B-1 and B-1–derived plasma cells. J. Exp. Med. 214, 2777–2794. doi:10.1084/jem.20161122
Sawai, C. M., Babovic, S., Upadhaya, S., Knapp, D. J. H. F., Lavin, Y., Lau, C. M., et al. (2016). Hematopoietic stem cells are the major source of multilineage hematopoiesis in adult animals. Immunity 45, 597–609. doi:10.1016/j.immuni.2016.08.007
Schielen, P., Denbesten, C., Vos, J. G., Vanbladeren, P. J., Seinen, W., and Bloksma, N. (1995a). Immune effects of hexachlorobenzene in the rat: Role of metabolism in a 13-week feeding study. Toxicol. Appl. Pharmacol. 131, 37–43. doi:10.1006/taap.1995.1044
Schielen, P., Schoo, W., Tekstra, J., Oostermeijer, H. H. A., Seinen, W., and Bloksma, N. (1993). Autoimmune effects of hexachlorobenzene in the rat. Toxicol. Appl. Pharmacol. 122, 233–243. doi:10.1006/taap.1993.1192
Schielen, P., Van Rodijnen, W., Pieters, R. H., and Seinen, W. (1995b). Hexachlorobenzene treatment increases the number of splenic B-1-like cells and serum autoantibody levels in the rat. Immunology 86, 568–574.
Shaw, P. X., Hörkkö, S., Chang, M. K., Curtiss, L. K., Palinski, W., Silverman, G. J., et al. (2000). Natural antibodies with the T15 idiotype may act in atherosclerosis, apoptotic clearance, and protective immunity. J. Clin. Invest. 105, 1731–1740. doi:10.1172/JCI8472
She, Z., Li, C., Wu, F., Mao, J., Xie, M., Hun, M., et al. (2022). The role of B1 cells in systemic lupus erythematosus. Front. Immunol. 13, 814857. doi:10.3389/fimmu.2022.814857
Sherr, D. H., and Monti, S. (2013). The role of the aryl hydrocarbon receptor in normal and malignant B cell development. Semin. Immunopathol. 35, 705–716. doi:10.1007/s00281-013-0390-8
Sindhava, V. J., and Bondada, S. (2012). Multiple regulatory mechanisms control B-1 B cell activation. Front. Immunol. 3, 372. doi:10.3389/fimmu.2012.00372
Sindhava, V., Woodman, M. E., Stevenson, B., and Bondada, S. (2010). Interleukin-10 mediated autoregulation of murine B-1 B-cells and its role in Borrelia hermsii infection. PLoS One 5, e11445. doi:10.1371/journal.pone.0011445
Smith, F. L., and Baumgarth, N. (2019). B-1 cell responses to infections. Curr. Opin. Immunol. 57, 23–31. doi:10.1016/j.coi.2018.12.001
Stahl, D., Lacroix-Desmazes, S., Heudes, D., Mouthon, L., Kaveri, S. V., and Kazatchkine, M. D. (2000). Altered control of self-reactive IgG by autologous IgM in patients with warm autoimmune hemolytic anemia. Blood 95, 328–335. doi:10.1182/blood.v95.1.328.001k10_328_335
Stoermann, B., Kretschmer, K., Düber, S., and Weiss, S. (2007). B-1a cells are imprinted by the microenvironment in spleen and peritoneum. Eur. J. Immunol. 37, 1613–1620. doi:10.1002/eji.200636640
Sulentic, C. E. W., Holsapple, M. P., and Kaminski, N. E. (1998). Aryl hydrocarbon receptor-dependent suppression by 2,3,7,8-Tetrachlorodibenzo-p-dioxin of IgM secretion in activated B cells. Mol. Pharmacol. 53, 623–629. doi:10.1124/mol.53.4.623
Tapiero, H., Ba, G. N., and Tew, K. D. (2002). Estrogens and environmental estrogens. Biomed. Pharmacother. 56, 36–44. doi:10.1016/s0753-3322(01)00155-x
Tedder, T. F. (2015). B10 cells: A functionally defined regulatory B cell subset. J. Immunol. 194, 1395–1401. doi:10.4049/jimmunol.1401329
Tornberg, U. C., and Holmberg, D. (1995). B-1a, B-1b and B-2 B cells display unique VHDJH repertoires formed at different stages of ontogeny and under different selection pressures. Embo J. 14, 1680–1689. doi:10.1002/j.1460-2075.1995.tb07157.x
Tsuji, N., Rothstein, T. L., and Holodick, N. E. (2020). Antigen receptor specificity and cell location influence the diversification and selection of the B-1a cell pool with age. J. Immunol. 205, 741–759. doi:10.4049/jimmunol.1901302
Tsuji, R. F., Kawikova, I., Ramabhadran, R., Akahira-Azuma, M., Taub, D., Hugli, T. E., et al. (2000). Early local generation of C5a initiates the elicitation of contact sensitivity by leading to early T cell recruitment. J. Immunol. 165, 1588–1598. doi:10.4049/jimmunol.165.3.1588
Ubeira, F. M., Leiro, J., Seoane, R., and Regueiro, B. J. (1987). The antiphosphorylcholine plaque-forming cell responses induced by the nematode Trichinella in BWF1 mice. Med. Microbiol. Immunol. 176, 143–150. doi:10.1007/BF00193895
Uberoi, A., Bartow-Mckenney, C., Zheng, Q., Flowers, L., Campbell, A., Knight, S. A. B., et al. (2021). Commensal microbiota regulates skin barrier function and repair via signaling through the aryl hydrocarbon receptor. Cell Host Microbe 29, 1235–1248.e8. doi:10.1016/j.chom.2021.05.011
Vale, A. M., Nobrega, A., and Schroeder, J. R. H. W. (2015). The role of evolutionarily conserved germ-line DH sequence in B-1 cell development and natural antibody production. Ann. N. Y. Acad. Sci. 1362, 48–56. doi:10.1111/nyas.12808
Vigna, A. F., Almeida, S. R., Xander, P., Freymüller, E., Mariano, M., and Lopes, J. D. (2006). Granuloma formation in vitro requires B-1 cells and is modulated by Paracoccidioides brasiliensis gp43 antigen. Microbes Infect. 8, 589–597. doi:10.1016/j.micinf.2005.06.033
Vigna, A. F. G., Godoy, L. C., Rogerio De Almeida, S., Mariano, M., and Lopes, J. D. (2002). Characterization of B-1b cells as antigen presenting cells in the immune response to gp43 from Paracoccidioides brasiliensis in vitro. Immunol. Lett. 83, 61–66. doi:10.1016/s0165-2478(02)00070-6
Villa, M., Gialitakis, M., Tolaini, M., Ahlfors, H., Henderson, C. J., Wolf, C. R., et al. (2017). Aryl hydrocarbon receptor is required for optimal B-cell proliferation. EMBO J. 36, 116–128. doi:10.15252/embj.201695027
Vo, H., Chiu, J., Allaimo, D., Mao, C., Wang, Y., Gong, Y., et al. (2014). High fat diet deviates PtC-specific B1 B cell phagocytosis in obese mice. Immun. Inflamm. Dis. 2, 254–261. doi:10.1002/iid3.41
Vocanson, M., Hennino, A., Rozières, A., Poyet, G., and Nicolas, J.-F. (2009). Effector and regulatory mechanisms in allergic contact dermatitis. Allergy 64, 1699–1714. doi:10.1111/j.1398-9995.2009.02082.x
Vorderstrasse, B. A., Bohn, A. A., and Lawrence, B. P. (2003). Examining the relationship between impaired host resistance and altered immune function in mice treated with TCDD. Toxicology 188, 15–28. doi:10.1016/s0300-483x(02)00749-7
Wang, X.-S., Cao, F., Zhang, Y., and Pan, H.-F. (2020). Therapeutic potential of aryl hydrocarbon receptor in autoimmunity. Inflammopharmacology 28, 63–81. doi:10.1007/s10787-019-00651-z
Warren, T. K., Mitchell, K. A., and Lawrence, B. P. (2000). Exposure to 2,3,7,8-tetrachlorodibenzo-p-dioxin (TCDD) suppresses the humoral and cell-mediated immune responses to influenza A virus without affecting cytolytic activity in the lung. Toxicol. Sci. 56, 114–123. doi:10.1093/toxsci/56.1.114
Weber, G. F., Chousterman, B. G., Hilgendorf, I., Robbins, C. S., Theurl, I., Gerhardt, L. M., et al. (2014). Pleural innate response activator B cells protect against pneumonia via a GM-CSF-IgM axis. J. Exp. Med. 211, 1243–1256. doi:10.1084/jem.20131471
Webster, S. E., Ryali, B., Clemente, M. J., Tsuji, L., and Holodick, N. E. (2022). Sex influences age-related changes in natural antibodies and CD5+ B-1 cells. J. Immunol. 208, 1755–1771. doi:10.4049/jimmunol.2101150
Weng, C.-M., Wang, C.-H., Lee, M.-J., He, J.-R., Huang, H.-Y., Chao, M.-W., et al. (2018). Aryl hydrocarbon receptor activation by diesel exhaust particles mediates epithelium-derived cytokines expression in severe allergic asthma. Allergy 73, 2192–2204. doi:10.1111/all.13462
Wong, J. B., Hewitt, S. L., Heltemes-Harris, L. M., Mandal, M., Johnson, K., Rajewsky, K., et al. (2019). B-1a cells acquire their unique characteristics by bypassing the pre-BCR selection stage. Nat. Commun. 10, 4768. doi:10.1038/s41467-019-12824-z
Yanaba, K., Bouaziz, J.-D., Matsushita, T., Tsubata, T., and Tedder, T. F. (2009). The development and function of regulatory B cells expressing IL-10 (B10 cells) requires antigen receptor diversity and TLR signals. J. Immunol. 182, 7459–7472. doi:10.4049/jimmunol.0900270
Yanaba, K., Bouaziz, J. D., Haas, K. M., Poe, J. C., Fujimoto, M., and Tedder, T. F. (2008). A regulatory B cell subset with a unique CD1dhiCD5+ phenotype controls T cell-dependent inflammatory responses. Immunity 28, 639–650. doi:10.1016/j.immuni.2008.03.017
Yang, Y., Li, X., Ma, Z., Wang, C., Yang, Q., Byrne-Steele, M., et al. (2021). CTLA-4 expression by B-1a B cells is essential for immune tolerance. Nat. Commun. 12, 525. doi:10.1038/s41467-020-20874-x
Yang, Y., Tung, J., Ghosn, E., Herzenberg, L., and Herzenberg, L. (2007). Division and differentiation of natural antibody-producing cells in mouse spleen. Proc. Natl. Acad. Sci. U. S. A. 104, 4542–4546. doi:10.1073/pnas.0700001104
Yurino, H., Ishikawa, S., Sato, T., Akadegawa, K., Ito, T., Ueha, S., et al. (2004). Endocrine disruptors (environmental estrogens) enhance autoantibody production by B1 cells. Toxicol. Sci. 81, 139–147. doi:10.1093/toxsci/kfh179
Zelante, T., Iannitti, R. G., Cunha, C., De Luca, A., Giovannini, G., Pieraccini, G., et al. (2013). Tryptophan catabolites from microbiota engage aryl hydrocarbon receptor and balance mucosal reactivity via interleukin-22. Immunity 39, 372–385. doi:10.1016/j.immuni.2013.08.003
Zhong, X., Gao, W., Degauque, N., Bai, C., Lu, Y., Kenny, J., et al. (2007). Reciprocal generation of Th1/Th17 and Treg cells by B1 and B2 B cells. Eur. J. Immunol. 37, 2400–2404. doi:10.1002/eji.200737296
Zhou, J., Blevins, L. K., Crawford, R. B., and Kaminski, N. E. (2022). Role of programmed cell death protein-1 and lymphocyte specific protein tyrosine kinase in the aryl hydrocarbon receptor- mediated impairment of the IgM response in human CD5(+) innate-like B cells. Front. Immunol. 13, 884203. doi:10.3389/fimmu.2022.884203
Keywords: autoimmunity, autoantibody, granuloma, inflammation, B-1 lymphocytes, B1 cells
Citation: Hiéronimus L and Huaux F (2023) B-1 cells in immunotoxicology: Mechanisms underlying their response to chemicals and particles. Front. Toxicol. 5:960861. doi: 10.3389/ftox.2023.960861
Received: 03 June 2022; Accepted: 31 March 2023;
Published: 18 April 2023.
Edited by:
Stefan F. Martin, University of Freiburg Medical Center, GermanyReviewed by:
Courtney E. W. Sulentic, Wright State University, United StatesRaimo Kalevi Pohjanvirta, University of Helsinki, Finland
Copyright © 2023 Hiéronimus and Huaux. This is an open-access article distributed under the terms of the Creative Commons Attribution License (CC BY). The use, distribution or reproduction in other forums is permitted, provided the original author(s) and the copyright owner(s) are credited and that the original publication in this journal is cited, in accordance with accepted academic practice. No use, distribution or reproduction is permitted which does not comply with these terms.
*Correspondence: Léa Hiéronimus, bGVhLmhpZXJvbmltdXNAdWNsb3V2YWluLmJl
†ORCID: Hiéronimus Léa, orcid.org/0000-0002-7004-7256; Huaux François, orcid.org/0000-0002-4048-7605