- 1State Key Laboratory of Oil and Gas Reservoir Geology and Exploitation, Chengdu University of Technology, Chengdu, Sichuan, China
- 2Institute of Multipurpose Utilization of Mineral Resources, Chinese Academy of Geological Sciences, Chengdu, Sichuan, China
Due to the special tectonic background and complex geological evolution characteristics of the South China Sea, reef dolomite reservoirs (sush as Well Xike 1) are widely developed. Based on the drilling core data of Well Xike 1, the structure and geochemical characteristics of dolomite reservoirs, including carbon, oxygen, hydrogen isotopes and REE were systematically studied using geochemical and petrological methods. It is found that the geochemical characteristics of REE show that the main diagenetic environment of dolomites is a low-temperature alkaline semi open oxidation environment; the carbon and oxygen isotopes of the dolomites are generally lack of correlation, the δD value is significantly lower than the hydrogen isotope value of seawater. Meanwhile, the oxygen isotope value of deep dolomites is negatively biased, which may be due to the increase or decrease of pore water temperature caused by deep thermal convection that related to the regional tectonic movements of the South China Sea. The δ18O value is also consistent with the geological reality of increasing saddle dolomite content in deep dolomites. The distribution of the δ13C value indicates that the dolomite inherited the carbon of the original limestone during dolomitization, while the characteristic of the δD value shows that it may be affected by the mixing of atmospheric precipitation and concentrated seawater in the quasi contemporaneous period. Based on the comprehensive analysis of the geochemical characteristics of the Well Xike 1, it is considered that the higher diagenetic temperature could be an important factor leading to the huge differences between the diagenetic model of deep and shallow dolomites. The geochemical characteristics of the shallow dolomites show that it is mainly reflux infiltration dolomitization under the micro evaporation and concentration sea water environments, while the deep dolomite is transformed by the hot water fluids in the epigenetic diagenetic evolution stage.
1 Introduction
Deceptively simple dolomites [CaMg(CO3)2] are common carbonate rocks throughout the geological records, especially in the Precambrian strata, whereas there are few dolomites in the Holocene strata and the modern natural environment rocks. However, due to the incomplete understanding of its genetic mechanism, there is great uncertainty in the prediction of dolomite distribution (Braithwaite et al., 2004). Modern sea water is oversaturated with dolomites, but the main carbonate minerals precipitated are aragonite, in addition, it has been realized that the direct precipitation reaction of dolomite is bound by kinetic factors (Land., 1998; Arvidson et al., 1999). The dolomitization, therefore, was once considered to be the only way to form dolomites. In recent years, the introduction of microbial dolomite (Mazzullo, 2000; Sánchez-Román et al., 2008; Bontognali et al., 2010; You et al., 2011; Petrash et al., 2017) has provided an additional perspective for geologists to understand the formation of dolomites, and several models of dolomitization related to microbial action are proposed, such as sulfate reduction (Vasconcelos et al., 1995, 1997; Li and Liu., 2013), methane oxidation (Aloisi et al., 2000; Kenward et al., 2009), methanogenesis (Roberts et al., 2004) and biological involvement (Sánchez-Román et al., 2008; Roberts et al., 2013; Petrash et al., 2017).
Coral reefs are composed of different types and complex structures of fixed benthic and in situ carbonate formations (Riding Robert, 2002). About 60% of the world’s oil and gas reserves are distributed in Great Reef oil and gas fields (Arthur and Schlanger, 1979). Coral reef dolomitization model is also a new research direction that cannot be ignored. Budd (1997) believes that the island reef dolomite provides a favorable support for understanding the genetic mechanism of dolomite- island reef dolomite was originally a poorly ordered, microcrystalline structure, rich in calcium and oxygen isotopes (18O) deposited quasi contemporaneously. In normal or evaporated (concentrated) seawater environment, and in the process of diagenetic evolution, the buried (epigenetic) dolomite inherited and different from quasi syngenetic dolomite is formed through a series of diagenesis such as dolomite recrystallization. The geochemical characteristics of island-reef dolomite are Ca rich and with low Sr, Fe and Mn contents. The Sr isotopic age results of many island-reef dolomites show that there is a relatively synchronous dolomitization time in the world, global ocean currents, climate and other related factors are related to the island-reef dolomitization.
Although predecessors have carried out different degrees of research on the petrological and geochemical characteristics of dolomites in Well Xike 1, they have hardly involved the change characteristics of hydrogen isotopes and rare earth elements. In order to explore the genesis of shallow, middle and deep dolomites and the diagenetic model of island-reef dolomites, in this study, taking the Well Xike 1 in Xisha area, South China Sea (Figure 1) as an example, the characteristics of isotopes and the change laws of earth elements of the shallow and middle-deep dolomites were systemically studied. Combined with the petrological characteristics, sedimentary tectonic background and global temperature changes of dolomites, the dolomitization model of the Well Xike 1 island reef is proposed.
2 Geological setting
The South China Sea, located in the southeastern Eurasian Plate (Taylor et al., 1983; Briais et al., 1993; Qiu et al., 2001; Yan et al., 2001; Lu et al., 2014), is one of the biggest marginal seas in the western Pacific Ocean. It is close to the junction part of two super convergence zones, the Tethys and the Pacific Rims (Wang P et al., 2010; D’Hondt S et al., 2012). The Xisha Island area is located in the transitional zone between the continental and oceanic crusts. The South China Sea has special geographical location, complex tectonic environment and submarine landform, which also determines the diversity of the reef systems (Xu H et al., 2015). Cenozoic carbonate reefs are well developed in the South China Sea, while Neogene dolomites are widely distributed in the Xisha Islands. Many geologists have proposed several dolomitization models to explain their genesis and dolomitization process. For example, Shi (2016) pointed out that the main patterns of dolomitization of the islands and reefs of Well Xike 1 can be explained by the infiltration and return flow model and buried diagenetic model.
Since the 1970s, several coring wells have been drilled on the small islands in the Xisha area (Figure 1), such as Wells Xiyong-1, 2, Xichen-1, Xishi-1and Xike-1 (Wei et al., 2007; Bi et al., 2018; Wang et al., 2018). Three sets of dolomites in the lower Miocene, middle-upper Miocene and upper Miocene in the interval from 0 m to 800 m deep in the Well Xichen-1 are divided. Zhai et al. (2015) divided 5 sets of dolomites in the middle Miocene, upper Miocene in the 0–700 m depth interval of Xike-1. The development of the Xisha Islands reefs (Riding Rober, 2002) are distributed almost throughout the Neogene.
Well Xike 1 can be divided into the Sanya Formation (Lower Miocene), the Meishan Formation (Middle Miocene), the Huangliu Formation (Upper Miocene), the Yinggehai Formation (Pliocene) and the Ledong Formation (Quaternary) from bottom to top (Shi et al, 2016). There are seven thick dolomites (the thickness of thick dolomite is more than 15 m and the dolomite content is more than 75%) in the cores of 0–1257.52 m in Well Xike 1, including one in the Pliocene, three in the early Miocene, two in the Middle Miocene and one in the late Miocene. The thickness of dolomites varies (the thickest is 163 m and the thinnest is 15 m). In order to explore the spatial variation relationship of dolomites, the dolomite layers of the Well Xike 1 are divided into three categories: shallow, middle and deep dolomite. The formation thickness and content of dolomite are shown in Table 1.The dolomite layer with thickness <600 m is shallow dolomite (layers 1, 2 and 3, with a total thickness of 203 m), the middle dolomite is buried within 600–1000 m (layers 4, 5 and 6, with a total thickness of 79 m), and the deep dolomite is buried >1000 m (layer 7, with a thickness of 155 m). Thick dolomitic limestone and limestone are developed in the middle and deep layers, most of which are crystalline dolomites. Under the microscope, dolomite usually has fog core and bright edge structures, and the crystallinity of dolomite crystal tends to be better with the increase of the buried depth.
3 Methods and materials
3.1 Materials
The cores of the well Xike 1 was observed and the dolomite samples were collected at different depths. More than 200 fresh carbonate samples, including dolomite samples and a small amount of limestone samples, were collected by drilling cores. All samples are immediately stored in plastic bags to reduce pollution and oxidation. At the institute of sedimentary geology, Chengdu university of technology, 160 carbonate samples were observed by a polarizing microscope. Fifty-one dolomite samples of different layers were selected to analyze the contents of carbon, oxygen, hydrogen isotopes and REE.
3.2 Methods
An Leica polarizing microscope (Leica-Dmrx) was used for optical microscopic identifications, and the thin sections of dolomite cores were observed by transmission light. In addition, this paper also selected some samples for cathodoluminescence, which was completed on the basis of identification and statistics of stained cast thin sections. The instrument used was a CL8200MK5 cathodoluminescence instrument (equipped with Leica polarizing microscope) of the exploration and Development Research Institute of Sinopec Southwest Oil and Gas Company. Considering the comparability of samples, all samples adopted the same test conditions, i.e., beam voltage of 15.8 kv and beam current of 304 μA. The exposure duration is 1.8 s.
Carbon and oxygen isotope analysis is mainly completed by a MAT-253 isotope mass spectrometer of the Nanjing Institute of Geology and Paleontology, Chinese Academy of Sciences. Kiel IV Carbonate Device sample preparation system is used. Reference standard: GBW-04405. It is based on DZ/T0184.17-1997 “Phosphoric acid method for determination of carbon and oxygen isotopes in carbonate minerals or rocks.” Analysis accuracy: The standard deviations of the measured values of δ13C (PDB) and δ18O (PDB) are less than 0.040‰ and 0.080‰.
Hydrogen isotopic analyses were conducted on the fluid inclusions hosted in the mineral particles (dolomite). Samples were first degassed of labile volatiles by heating under vacuum to 150°C for 3 h. Water was released by heating the samples to approximately 500°Cby means of an induction furnace. Water was converted to hydrogen by passage over heated zinc powder at 400°C (Friedman, 1953), and the hydrogen was analyzed with a MAT-252 mass spectrometer. The analyses of standard water samples suggest a precision for δD of ±3‰ (1σ). The hydrogen isotopic analyses were performed at the Analytical Laboratory of Beijing Research Institute of Uranium Geology (ALBRIUG).
REE concentrations were determined at the Key Laboratory of Marine Geology and Environment, Institute of Oceanology, Chinese Academy of Sciences. About 40 mg of each sample was first digested with 0.6 ml HNO3 and 2 ml HF in a Teflon beaker. After standing with seal-capping for 2 h, the beaker was placed on a hotplate at about 150°C for 24 h. A 0.25 ml HClO4 was added to the residue, and it again was heated to near dryness in an open-top beaker on a hotplate at about 150°C. The residue was redissolved with 1 ml HNO3+1 ml H2O and sealed again, and it remained on a hotplate at about 120°C for an additional 12 h. The residue was diluted by 1% HNO3 solution to produce a volume of 40 g, and it was subsequently analyzed by ICP-MS to determine the REEs in the sample solution.
4 Results
4.1 Petrological characteristics of dolomite
From the core observations, the crystal diameter of dolomite increases with the increase of depth, and the proportion of crystal dolomite in the rock increases with the increase of depth, in the form of yellowish brown sugar.
4.1.1 Shallow dolomite (0–600 m)
The dolomites in the second member of the Yinggehai Formation are micrite powder dolomites, and the crystal diameters are mostly less than 50 um. Common red algae structures can be seen. The dolomites of the Huangliu Formation are usually microcrystalline silty dolomites. In the second member of the Huangliu Formation below 462 m depth, the diameters of the dolomite crystal formed are large, so the content of the silty dolomite is great. For most of the silty dolomites and micritic (micro) dolomites, there have residual reef textures, grain textures or original matrix silty textures (Figure 2).
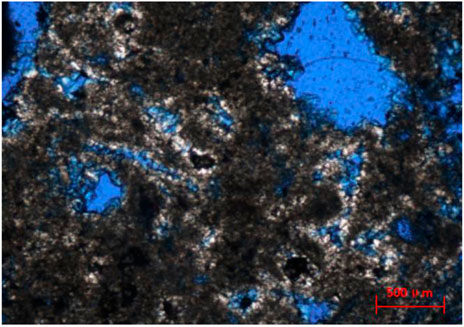
FIGURE 2. Petrological characteristics of shallow dolomite sample. Microcrystalline bioclastic dolomite at the top (288.91 m) of the second member of the Yinggehai Formation. The echinoderm bioclastic in the middle of the photo is completely dolomitized, with a diagonal length of 1.6 mm (1N).
4.1.2 Middle dolomite (600–1000 m)
The middle dolomites are mainly composed of powder crystal - fine crystal dolomites. The dolomite crystals are semi automorphic automorphic crystals. Fog-bright edge dolomites are developed in the dolomites of the layers 4, 5 and 6, and bioclastic (foraminifera, echinoderms and algae clumps) components are developed in varying degrees. Some bioclastic components has residual structures (but the preservation of original components is worse than that of the shallow dolomites). By observing the core samples, it can be found that in the 620–646.5 m interval, the color is mainly light grayish white - light grayish yellow—grayish yellow and white. A large number of white marly filled insect pores, mold pores, cracks, etc., with obvious debris fillings; through scanning electron microscope observations, it is mainly fine and powder crystals, showing euhedral—semi euhedral shapes. There are obvious intergranular pores between the crystals. Corrosion is developed in some depths, and solution pores on the crystal surface can be observed. The fillings are mainly powder crystal fine-grained dolomites. Under the microscope, it is observed as flat crystal plane, euhedral semi euhedral. The crystals are in multi-faceted contacts or dense mosaic contacts, and it is difficult to find point contacts. In addition, argillaceous dolomites with poor crystal shapes and small crystals can be found, and the grains have complex curved surfaces with complementary shapes (Figure 3).
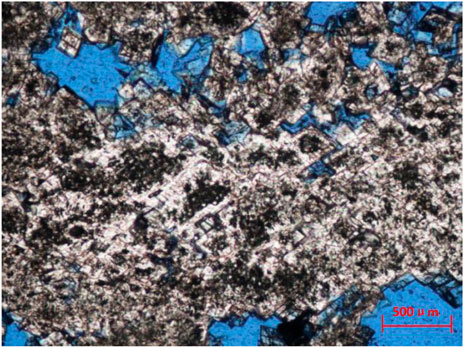
FIGURE 3. Petrological characteristics of middle dolomites. The powdery residual coral dolomites in the first member of the Meishan Formation (628.86 m) have experienced excessive dolomitization, and the content of “fog-bright edge” powdery dolomites is high, with a diagonal length of 1.6 mm (1N).
4.1.3 Deep dolomite (>1000 m)
The underlying stratum of the Meishan Formation is the first member of the Sanya Formation. The main lithology is grayish white dolomites, maroon dolomites and earthy yellow dolomites. Most dolomites have good permeability and have developed a large number of macropores (Figure 4). Deep dolomites have over-developed dolomitization, and dolomites are characterized by fog-bright edges. Compared with the cemented dolomites, it can be found that the pore water medium formed by them has the same geological conditions and periods. Compared with the cemented dolomite, the “bright edge” of the fog-bright edge has the same composition. Under the cathodoluminescence, the optical characteristics of them are similar. In the second member of the Sanya Formation, the saddle dolomites with medium coarse crystal are more common, and the crystallization degree of crystals will gradually increase with the increase of buried depth.
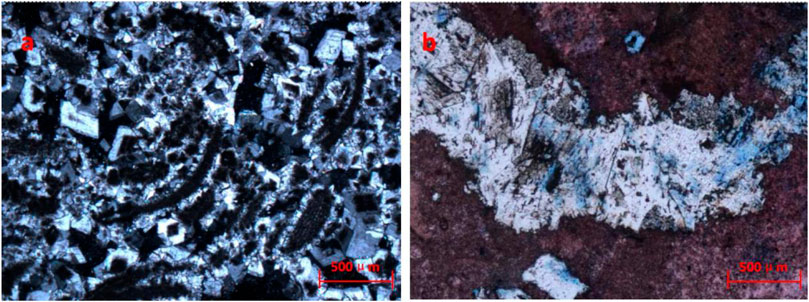
FIGURE 4. Petrological characteristics of deep dolomites. (A) microscopic characteristics of bright edge of dolomite fog center, 1170.42 m, diagonal length of 1.6 mm (XN); (B) medium and coarse-grained saddle dolomite is filled in the limestone fracture. The dolomite can see fog center and bright edge, single polarization, 1239.42 m (1N); From the overall trend, cement dolomites and excessive dolomitized fog-bright edge dolomites are widely distributed in Well Xike 1. The greater the burial depth, the greater the dolomite content, and the number of dolomite crystals increases with the increase of burial depth. In addition, the order degrees of dolomites in the Well Xike 1 range from 0.4355 to 0.6000, and generally increases with the increase of the burial depth (Shi et al., 2016). The change of dolomite content from shallow to deep layers reflects the self adjustment process of dolomite structures from recrystallization to ideal composition in the process of burial-diagenesis.
In addition, the deep dolomites also have experienced excessive dolomitization: in the Sanya Formation, the saddle dolomites in the limestones are commonly replaced by calcites along the cleavages. The dolomite crystal is large, the crystal plane is curved, and has wavy extinction. The fluid inclusions in 82 dolomite samples with a depth of more than 1064 m tested by Shi et al. (2016) have a homogenization temperature of 129.5°C–268.5°C and an average value of 184.6°C. It is speculated that the paleofluid temperature was high when it was formed. The saddle dolomites formed at higher temperature are prone to de dolomitization after the thermal fluids (upwelling due to tectonic action) subsides. In general, The dolomites in the lower part of the Sanya Formation and Mei-2 Member seldom undergo “decloudization”, indicating that the dolomite lattice under the excessive dolomitization is relatively stable, so it is not easy to be replaced by calcite.
4.2 Carbon and oxygen isotopes
The geochemical characteristics of dolomites are an important information carrier for the genesis and formation mechanism of dolomites. The enrichment and loss, migration and preservation of elements are not only the records of sedimentary environments, but also an important calibration of geological processes (Hong H, 2004; Ni S et al., 2009; Hu Zuowei, 2010). For carbon, oxygen and hydrogen isotopes, carbon isotopes, they are mainly used to study paleotemperature, salinity and fluid properties, and hydrogen isotopes are mainly used to trace fluid properties in combination with the covariance law of oxygen isotopes (Craig, 1961; Holser, 1979; Richard, 2013).
The carbon and oxygen isotopes of 51 carbonate rock samples were analyzed in Well Xike 1. Kaufman et al. found that in order to ensure the effectiveness of data, the value of δ18O cannot be less than −10‰, and Mn/Sr <10 can be used as a basis to determine whether the carbon isotope maintains the original composition (Kaufman A J et al., 1995).
4.2.1 Carbon and oxygen isotopes of shallow dolomite
In Well Xike 1, 13 shallow dolomite samples, 10 middle dolomite samples and 28 deep dolomite samples are selected. The carbon and oxygen isotope statistics are shown in Table 2. It can be seen that the δ18O values are greater than −10‰. According to Kaufman’s theory, the data validity can be guaranteed.
The carbon and oxygen isotopes of shallow dolomite samples have the following characteristics:
There are great differences of δ13C and δ18O values between dolomite-rich samples and calcite-rich samples, and 18O and 13C values in dolomites are significantly higher than those in limestones. Of dolomite samples, δ18O value varies from 1.79‰ to 5.589‰, with an average of 3.33‰; δ13C value varies from 1.56‰ to 4.33‰, with an average of 2.61‰ (Figure 5). There is no obvious correlation between carbon and oxygen isotopes (Figure 6). Of shallow dolomite samples from Well Xike 1, the δ13CPBD value is close to the present value and is in the global range of normal Neogene marine carbonates synthesized by Budd (1997) δ13C value, and the Mn/Sr variation range of shallow dolomites is 0.167–0.316. The color of carbon isotope in shallow dolomites indicates that it inherits the carbon in the original limestone.
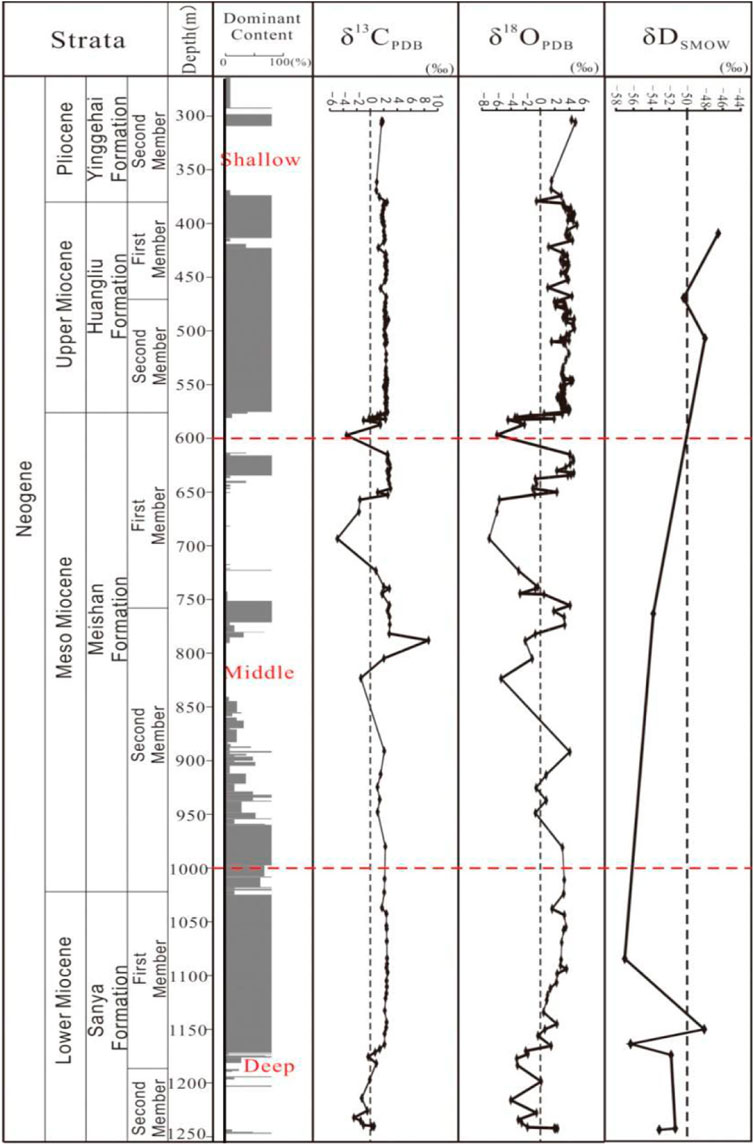
FIGURE 5. Distribution of dolomites, carbon, oxygen and hydrogen isotopes in Well Xike 1 [Data source: partial data of carbon and oxygen isotopes are from Shi et al. (2016)].
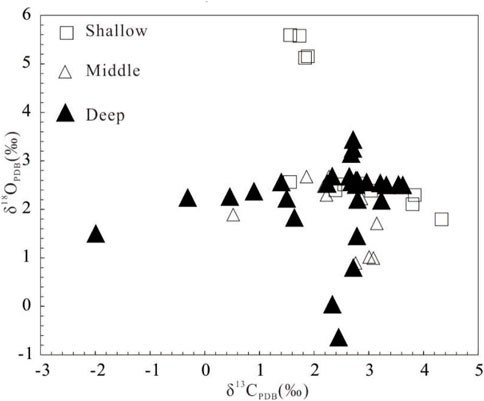
FIGURE 6. Correlation diagram of carbon and oxygen isotopes of shallow, middle and deep dolomites in Well Xike 1.
4.2.2 Carbon and oxygen isotopes of middle dolomite
The correlation between carbon and oxygen isotopes is not good. Through sorting and statistics of 10 groups of dolomite data, The average value of δ13C is 2.39‰, ranging from 0.52‰ to 3.14‰; the average value of δ18O is 1.883‰, ranging from 0.891‰ to 2.675‰. According to the statistical data, its carbon isotope value is close to the value of modern marine carbonate deposition, which is also in line with the global standard of normal Neogene marine carbonates synthesized by Budd (1997) δ13C range, and its Mn/Sr variation range is 0.07–0.31. The marine color of its carbon isotope is also shown, which indicates that it inherited the carbon of the original limestone in the process of dolomitization.
4.2.3 Carbon and oxygen isotopes of deep dolomite
In deep dolomite samples, as shown in Figure 6, the correlation between the carbon and oxygen isotopes is not good. Through sorting and statistics of 28 groups of dolomite data in deep layer, it can be found that, the average value of δ13C is 2.245‰, ranging from −1.99‰ to 3.62‰, while the average value of δ18O is 2.20‰, ranging from −0.639‰ to 3.435‰. According to the statistical data, the carbon isotope value is close to the value of modern marine carbonate deposition, slightly lower than the value of shallow and middle dolomite, which is also in line with the global standard of normal Neogene marine carbonates synthesized by Budd (1997) δ13C range, and its Mn/Sr variation range is 0.11–0.67. The marine color of its carbon isotope is also shown, which indicates that the carbon of the original limestone was inherited in the process of dolomitization.
In terms of the average value of oxygen isotope, the deep layer is 1.449‰ lower than the shallow layer, close to the value of middle dolomite (about 0.002‰), and the sedimentary value of dolomite rich samples is relatively similar to that of limestone. It can be seen from Table 2 that this situation is particularly prominent in the Sanya formation, which also indirectly indicates that there is a great difference between dolomite and shallow layer in terms of Genesis.
While at 1182.5 and 1185.5 m, the δ18O value has negative bias (respectively −1.99‰ and −1.70‰), and it is positively correlated with δ13C value (1.5‰ and 0.82‰ respectively). It is generally believed that rainwater and underground fresh water are rich in light oxygen isotopes and carbon isotopes, which make it δ18O and δ13C presents a large negative value (Wei Xi, 2006 ab). The carbon and oxygen isotope characteristics at this place indicate that there is no obvious rain water or underground fresh water leaching environment in the deep dolomite during diagenetic evolution.
Deep dolomite δ18O has a negative bias trend, δ18O is a function of temperature and salinity (which can reflect the properties of diagenetic fluids) and is more sensitive to temperature to some extent (Huang, 2010), δ18O value will decrease with the increase of pore water temperature, so the rise of formation water temperature caused by deep thermal convection will have a direct impact on the formation of some dolomites, which is consistent with the geological reality of the increase of saddle dolomite content in the deep part of well Xike 1. Huang et al. (2015) believed that saddle-shaped dolomite is an important diagenetic mineral in sedimentary rocks and is widely distributed in hydrothermal and other relatively high temperature diagenetic environments. For this reason, it is also commonly used as a semi-quantitative geological thermometer and indicator mineral for certain special fluids.
4.3 Hydrogen isotope
Hydrogen isotope test is carried out for dolomites in different layers of well Xike 1. The analysis results are shown in Table 3:
It can be seen from the analysis results that among the three samples in the shallow dolomites (579.26 m shallow), The maximum value of δD is −46.7‰ and the minimum value is −50.3‰; in the middle and deep dolomite interval (770.05–1257.4 m well section), the maximum value of δD is −48.1‰ and the minimum value is −57.0‰.
4.4 REE data
The detailed REE element parameters of shallow, middle and deep dolomite samples from the Well Xike 1 are shown in Table 4. The REE distribution model of seawater standardized by PAAS is mainly characterized by negative Ce anomaly and relative enrichment of heavy rare earths (Zhang J et al., 1996). After PAAS standardization, the REE test results of shallow, middle and deep dolomite samples in Well Xike 1 (a total of 20 samples) show that the samples of dolomites in different layers have good similarities with the PAAS distribution curve of modern seawater, which are highlighted by LREE loss, negative Ce anomaly and positive Y anomaly (Figures 7, 8).
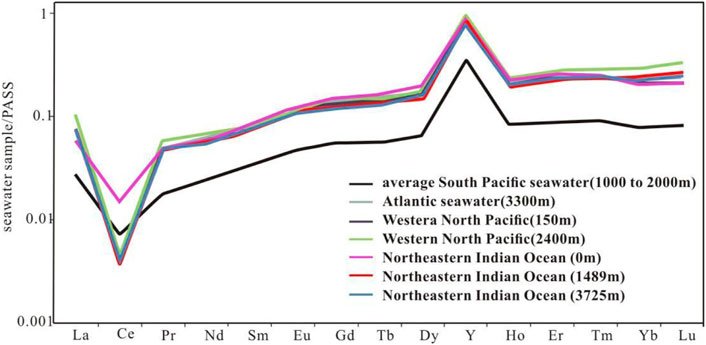
FIGURE 7. Distribution curves of REE in modern seawater (Wang et al., 2017; Wang et al., 2018).
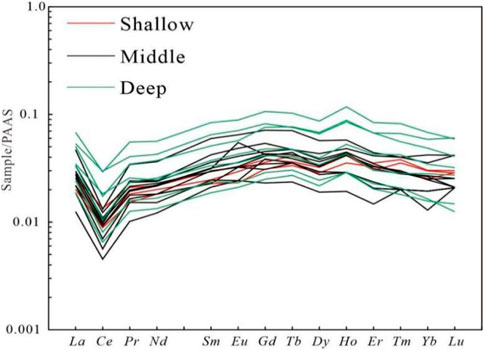
FIGURE 8. Distribution curves of REE in shallow, middle and deep limestones and dolomite samples of Well Xike 1.
5 Discussions
Diagenetic environment and diagenesis affect δ13C and δ18O values (Peng et al., 2006). Moreover, carbon and oxygen isotopes are also controlled by temperature and salinity, which can well reflect the changes of paleotemperature and paleosalinity in the diagenetic environment of dolomites. For hydrogen isotope, there is a certain linear relationship between δD and δ18O values. In addition, the hydrogen isotope can also be used to judge the relationship between surface water and groundwater (Craig, 1961; Holser, 1979; Richard, 2013).
5.1 Analysis of carbon and oxygen isotopes
5.1.1 Analysis of carbon and oxygen isotopic characteristics of shallow dolomites
Analysis of 13 shallow dolomite samples from Well Xike 1 shows that the δ18O value is higher than the limestone of the formation produced by these dolomites by varying degrees. The research data of Fouke (1994) show that the dolomites formed in the Miocene Pliocene sea water has the value of δ18O that significantly higher than that of calcite.
From the experimental results of the determination of δ18O fractionation coefficient of carbonate water, it can be seen that when Ca is replaced by Mg, the δ18O fractionation coefficient will increase. The experimental results of high magnesium calcite also show that when MgCO3 increases by 1 mol, δ18O will increase by 0.06‰ (Tarutani et al., 1969) and 0.17‰ (Jiménez-López et al., 2004). Analyzing the theoretical calculations and experimental results of calcite and dolomite coprecipitation, it can be found that when mg increases by 1%, the δ18O value will increase by 0.05–0.14‰ (Fritz et al., 1970; Schmidt et al., 2005; Vasconcelos et al., 2005; Chacko et al., 2008). It is precisely because there is a certain difference in δ18O values between calcite and synsedimentary dolomites. This phenomenon can reasonably explain the δ18O of dolomite-rich samples in Well Xike 1 is positive relative to contemporaneous sedimentary limestones.
In Well Xike 1, when the Mg content in dolomites increases by 1%, the δ18O value increases by 0.29‰, which shows that the formation process of dolomites not only includes its own fractionation effect in mineralogy, but also other external factors, thus resulting in the increase of δ18O value.
The δ18O value increases with the decrease of the paleo ocean temperature. The Well Xike 1 atoll was formed during the relatively cold period from the end of Miocene to the beginning of Pliocene. According to the sample test results, the average δ18O value is about 3.33‰, which is slightly higher than the average δ18O value range (2.0‰–3.5‰) of tertiary island reef dolomites formed by normal seawater in Pacific Ocean and Caribbean Sea according to Budd (1997). From this point of view, the global low temperature is not the dominant factor among the relevant factors leading to the positive value of δ18O.
The δ18O (PDB) value of the modern marine carbonate deposits is about 0‰, and the seawater is concentrated after evaporation, which makes the increase of the δ18O value (Huang, 2010). Sibley (1990) believes that if the salinity of sedimentary medium exceeds the normal seawater during the formation process, the δ18O value of sediments will exceed 2‰. The fluids formed by dolomites has slightly higher salinity than the sea water, but it can be found by analyzing the carbonate sequence of the Well Xike 1 that there is no gypsum layer with volumetric significance, which also shows that the salinity of fluids is insufficient compared with the required saturation of gypsum. Therefore, the judgment that the dolomite forming fluids is micro evaporation concentrated seawater can be implemented by using the δ18O value.
5.1.2 Analysis of carbon and oxygen isotopic characteristics of middle and deep dolomites
The average value of δ18O of middle buried dolomites is 1.448‰, which is lower than that of the shallow dolomites (Secend Member of the Meishan Formation), and it is only 0.002‰ lower than that of deep dolomites. There may be two reasons:
(1) There is a large change in magnesium oxide content in the 10 groups of dolomite samples, resulting in a high proportion of limestone containing dolomites and limestone dolomites. This phenomenon is particularly prominent in the Secend member of the Meishan Formation. There are great differences in the chemical composition, which will also have a certain impact on the δ18O and carbon isotope values of the middle buried dolomites.
(2) There may be similar genesis between the middle and deep buried dolomites, which leads to the similarity of the δ13C and δ18O values.
In terms of the average value of δ18O, the deep layer is 1.448‰ lower than the shallow layer, and it is close to the value of middle buried dolomites. Moreover, the sedimentary value of dolomiterich samples is relatively similar to that of limestones. It can be seen from Table 2 that this situation is particularly prominent in the Sanya Formation, which also indicates that there is a great difference between dolomites and shallow layer in terms of genesis. The δ18O value will decrease with the increase of pore water temperature (Hu et al., 2010). Therefore, the increase of formation water temperature will have a direct impact on the formation of dolomites, which is consistent with the geological reality of the increase of saddle dolomite content in the deep part of the Well Xike 1 (e.g., buried depth greater than 1100 m).
5.1.3 Diagenetic environment of carbon and oxygen isotope reaction in dolomites
5.1.3.1 Comparison of diagenetic environments of dolomites of different genesis
The δ18O value is a function of temperature and salinity, which can reflect the nature of diagenetic fluid to a certain extent (Huang, 2010). According to the paleosalinity calculation formula proposed by Keith et al. (1964),
If the Z value is less than 120, it means that it is a continental diagenetic environment, and if the Z value is greater than 120, it means that it is a marine diagenetic environment. The Z values of shallow buried to deep dolomites in Well Xike 1 are calculated by the carbon and oxygen isotope values.
The statistical results of δ13C, δ18O isotopic and Z values of dolomites of different origins are shown in Table 5.
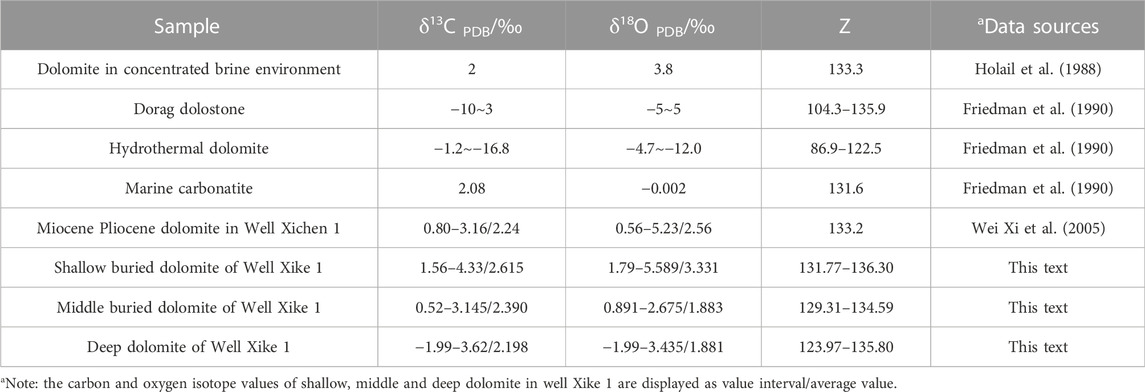
TABLE 5. Dolomites of different genesis and δ13C, δ18O and Z value of shallow, middle and deep dolomites of Well Xike 1.
The Z value of shallow, middle buried and deep dolomites exceeds 120, so it can be judged that they are formed in a marine environment. According to the statistics and comparison between the measured carbon and oxygen isotope values and the calculated Z values of different types of carbonate rocks, it is found that the variation range of carbon and oxygen isotopes and Z value of the shallow dolomites of Wells Xike 1 and Xichen 1 are similar (while the Z value of the middle and deep dolomites is slightly lower), and the quasi syngenetic dolomites related to the concentrated seawater is equivalent. This also indicates that the shallow dolomites of the Wells Xike 1 and Xichen 1 have similar genesis. However, the variation range of the carbon and oxygen isotopes and the decrease of the Z value of deep dolomites are closer to the saddle dolomites that related to hydrothermal activity. It indicates that the genesis of deep dolomites is different from that of the shallow dolomites.
5.1.3.2 Comparison of δ13C and δ18O discrimination diagrams of carbonate in different marine environments
δ13C and δ18O values of the shallow, middle and deep dolomites of Well Xike 1 are put into the δ13C and δ18O discrimination diagram of carbonate rocks in different marine environments (Milliman, 1978), the results are shown as follows (Figure 9):
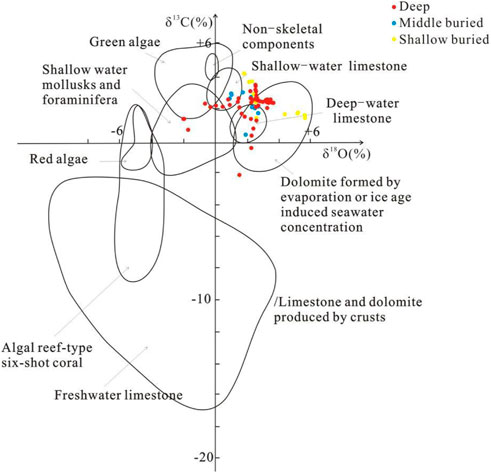
FIGURE 9. Distribution of δ13C and δ18O values in different types of marine carbonate rocks (modified according to Milliman, 1978).
For shallow buried dolomite, the δ18O value varies from 1.79‰ to 5.589‰, with an average value of 3.33‰; the δ13C value varies from 1.56‰ to 4.33‰, and the average value is 2.61‰. Except that some points fall in the freshwater limestone area, most of the δ13C and δ18O values fall in and near the dolomite areas formed by seawater concentrations (dolomites formed by seawater concentration caused by evaporation or ice age), indicating that dolomitization occurs in the period of seawater concentration and salinization.
For the middle buried dolomites, the δ13C value varies from 0.52‰ to 3.14‰, with an average value of 2.39‰, while the δ18O value varies from 0.891‰ to 2.675‰, with an average value of 1.883‰. For the deep dolomites, the δ13C value varies from −1.99‰ to 3.62‰, with an average of 2.198‰, while the δ18O value varies from −1.99‰ to 3.435‰, with an average of 1.881‰. The genesis of the middle and deep buried dolomites is quite different from that of the shallow buried dolomites.
5.2 Diagenetic environment of hydrogen isotope reaction
(1) The hydrogen and oxygen isotopes of the present seawater are relatively stable. The fluctuation range of the δD value is 4‰, and that of the oxygen isotope value is 0.3‰ (Tian et al., 2016). The hydrogen isotopic composition of deep seawater are shown in Table 6.
(2) The molecules containing light isotopes are easy to vaporize, which leads to a wide range of hydrogen and oxygen isotope values on the surface of seawater. Generally, there are hydrogen and oxygen isotopes on the surface of seawater δD = M δ18O. When the ratio of seawater evaporation to precipitation increases, the value of M will decrease. According to the statistical data (Tian et al., 2016), the m values of the North Pacific and the Red Sea are 7.5 and 0.6, respectively.
(3) The evaporation process not only causes changes in δD and δ18O, but also lead to changes in seawater salinity, so δD and δ18O are in direct proportion to salinity. For example, in the Red Sea, the salinity of surface water, intermediate water and deep water changes from 41‰ to 34‰, while the δ18O will also change correspondingly, from 1.9‰ to 0.6‰, δD is reduced from +10‰ to +4‰ (Craig, 1965).
(4) It is found that δD value in atmospheric water is related to altitude, temperature and climate humidity during precipitation (Dansgaard, 1964). There is a certain linear correlation between δD and δ18O values, which can be used to judge the relationship between surface water and groundwater (Craig, 1961; Holser, 1979; Richard, 2013).
The δD and δ18O values of fluids from each source are (Kyser et al., 1984; Zhang et al., 1993; Wang et al., 2011):
Magmatic water: δD −50‰∼−80‰; δ18O+6‰∼+8‰;
Present seawater: δD 0‰;
Atmospheric water (rainwater): δD −350‰∼+50‰; δ18O −44‰∼+10‰;
Among the three samples in the shallow buried dolomites (below 579.26 m), The maximum value of δD is −46.7‰, the minimum value is −50.3‰, and the average value is −48.3‰; while in the middle and deep buried dolomite interval (770.05–1257.4 m well section), the maximum value of δD is −48.1‰, the minimum value is −57.0‰, and the average value is 53.1‰. It can be seen that no matter whether it is shallow, middle or deep dolomites, the δD value is obviously less than the hydrogen isotope value of seawater, and is similar to that of limestone. In Well Xike 1, the average hydrogen isotope value of limestone is −55.67‰ (Yin He, 2020). Richard (2013) believes that the surface fluid δD value is −40‰ ∼ −70‰, it reflects that the diagenetic environment of dolomites is mainly near surface environment. While the hydrogen isotope composition in atmospheric precipitation varies greatly, the δD value ranges from −350‰∼ + 50‰. It is speculated that the dolomite may have been affected by the mixing of atmospheric precipitation and concentrated seawater during the quasi syngenetic period. However, Bi et al. (2018) thinks that the small hydrogen isotope value of the Well Xike 1 may also be related to the decomposition of organic matter.
5.3 Analysis of REE data
The statistical results of REE parameters of the shallow, middle and deep dolomites are shown in Table 7. Compared with the continental crust (Taylor et al., 1985) and carbonate rocks in eastern China (Yan M et al., 1997), the dolomites in layer 7 of Well Xike 1 shows a typical low total rare earth (∑REE) -with an average value of 3.928 μg/g, the ΣREE values of most samples range from 1.74 μg/g to 5.47 μg/g, only a few samples showed high values, but none of them exceeded 100 μg/g. The distribution range of ΣREE indicates marine carbonate rocks (Qing et al., 1994). It can be seen from this feature that no other provenance is mixed into dolomites. In terms of light rare earth content, the average is 3.123 μg/g, and the ∑LREE value ranges from 1.746 μg/g to 7.190 μg/g. In terms of heavy rare earth content, the average value of light rare earth content is 0.805 μg/g, the ∑HREE value ranges from 0.380 μg/g to 1.829 μg/g. On the whole, the REE characteristics of the 7-layer dolomites are basically similar, indicating that there is a relatively similar environment in the diagenetic process.
In the redox environment, the multivalent state characteristics of Ce and Eu will show sensitive changes. Calcium and magnesium ions (Ca2+ and Mg2+) in the lattice structures of dolomites can be replaced by rare earth elements. In the oxidation environment, Ce and Eu in dolomites are relatively less. This is because Ce and Eu ions can be trivalent in the oxidation environment, so they are difficult to replace divalent calcium and magnesium ions in the lattice of dolomites, which are easier to be extracted and exist in the fluids. Under low temperature and alkaline environment, Eu changes from trivalent state to divalent state, which is easier to dissolve and thus is migrated and depleted. According to the analysis of the measured sample data of dolomites in well Xike 1, the dolomite δCe negative anomaly is the lowest, and the δEu values of some dolomites have different negative anomaly characteristics from carbonate rocks in the eastern China, indicating that marine derived fluids are involved in the whole diagenetic process (including dolomitization) or that the diagenesis of dolomites is mainly affected by marine derived fluids. In dolomites the negative anomaly of δCe value reflects its diagenesis in the semi open environment of partial oxidation, while the diagenetic environment corresponding to the negative δEu value anomaly is low temperature and alkaline conditions.
The degree of Eu negative anomaly in shallow dolomite is slightly higher than that in other dolomites, indicating that it may have a lower ambient temperature during its formation-the oxygen isotope characteristics of foraminifera observed in ODP184 voyage sampling core also reflect the seawater cooling event in the South China Sea in the late Pliocene, which may be the reason for the lower diagenetic ambient temperature of shallow dolomite (Li Jianru et al., 2004).
5.4 Sedimentary tectonic setting and paleoclimate in Xisha area
In the South China Sea, in the early Miocene of about 5 Ma, there was obvious magmatic activities in the residual expansion center of the southwest basin (Meng L et al., 2014). From the late Miocene, the Dongsha Movement appeared in the northern South China Sea, and its activity stopped at the end of late Miocene/early morning Miocene (5.5 Ma) (Lin et al., 2009; Zhao et al., 2012). The large-scale dolomites in Wells Xike 1 and Xichen 1 is formed below the Miocene/Pliocene boundary.
The global paleoclimate background of dolomite deposits in Well Xike-1 during the Late Miocene-Pliocene is characterized by gradual cooling. In the middle and late Miocene, the global climate became significantly cooler, marine productivity increased sharply, and organic carbon buried rapidly, which further led to the decrease of bottom water temperature, and eventually led to the expansion and permanent formation of the Antarctic ice sheet at the end of the Miocene (Zachos et al., 2001; Lu and Chen, 2006).
From the end of the Miocene to the early Pliocene, the global climate fluctuated dramatically, experiencing several cooling (about 6.15–5.8 Ma in the Late Miocene), the latest in the Miocene to the Early Pliocene (about 5.7–5.46 Ma), warming (about 5.8–5.7 Ma in the Late Miocene), 5.46–4.9 Ma in the Early Pliocene (Hodell et al., 1986), and 4.6 Ma in the Early Pliocene. Therefore, from the late Miocene to the early Pliocene, the global climate is not always cold, but during the warming period, the ice sheet melts, the sea level rises, and the hydrothermal activity of the seabed is more active than that of the cooling period.
Due to the tectonic movement around 5.5 Ma, the thermal convection generated by deep thermal fluids migration is likely to affect the deposited Huangliu formation sediments. The mineral structures of dolomites in Well Xike 1 generally shows that the mineral structures of dolomites is relatively better in the first member of the Meishan Formation (576.5–758.4 m) with relatively low degree of dolomitization. The reason may be that the temperature of the diagenetic fluids is relatively high (the homogenization temperature of 82 dolomite fluid inclusions below 1064 m well depth tested by Shi et al. (2016) is 129.5°C–268.5°C, with an average of 184.6°C). When the buried depth increases gradually, the proportion of over dolomitized fog-bright edge dolomite and cement dolomite increases significantly.
5.5 Comparison of research results between Wells Xichen 1 and Xike 1
Wells Xichen 1 and Xike 1 have similar dolomite mineral types, including bright crystal cement, various mud crystal fossils, mud crystal debris and mud crystal particles (Wei et al., 2008a; Wei et al., 2008b; Wei et al., 2008c). Dolomite accounts for the majority of dolomites in Well Xichen 1, with a volume fraction of more than 91.1%, up to 100%. From these data, it can be seen that there are relatively loose carbonate rocks in the dolomitization process, which can be fully contacted with water and rock, and can last for a long time to dolomitize.
Among the three sections of dolomites in Well Xichen 1, both the δ13C and δ18O values are positive numbers. Among them, the average value of δ13C is 2.24‰, and the average value of δ18O is 2.56‰ (Wei et al., 2008a; Wei et al., 2008b; Wei et al., 2008c), and Wei et al. (2006a, 2006b) think that there is no obvious rainwater or underground fresh water leaching environment during the formation and evolution of dolomites in Well Xichen 1. However, if the dolomites in Well Xichen 1 undergoes alteration of lithogenic and hydrothermal fluids (Wei et al., 2006ab; Wei et al., 2007) during the evolution of metagenetic rocks, the carbon and oxygen isotopes (especially oxygen isotopes) of rocks may change, and the existing test values can not fully represent the characteristics of the original sedimentary environment. Wei et al. (2008a), Wei et al. (2008b), and Wei et al. (2008c) measured the fluid inclusions of authigenic bright dolomite minerals in rock pores or fractures. The results show that the fluid homogenization temperature during inclusion capture is 102.5–296°C, with an average of 156.3°C. The salinity ranged from 0.55 to 6.25%, with an average of 3.75%. The salinity slightly higher than that of the modern seawater reflects the fluid characteristics of dolomitization of brackish water or of medium salinity. The temperature measurement results of the inclusions are similar to the test results of Wells Sike 1 by Shi et al. (2016), which reflects that the similar diagenetic environments.
From the regional tectonic background, during the tectonic movement in the Xisha area in the late Miocene, the thermal convection caused by the upwelling of thermal fluids may have an impact on the deposited sediments of the Huangliu Formation, and the impact on the carbonate rocks of the Sanya Formation is more significant and extensive. High temperature is a necessary thermodynamic condition for the formation of dolomites (Machel, 2004; Huang, 2010). Therefore, the thermal fluids in this period undoubtedly promoted the occurrence of percolation and dolomitization. According to the research on dolomite structure, mineral composition, isotopic characteristics, inclusion type and characteristics of Well Xichen 1, it is shown that the hot water fluids played a role in the evolution process of carbonate rocks in the Xisha Islands. Under magmatism, the hydrothermal fluids can get enough heat, while the formation water and hydrothermal fluids have similar compositions, and dolomites are transformed by diagenetic fluids and hydrothermal fluids of imestone reservoirs.
Other scholars also systematically analyzed the δ13C and δ18O values of Well Xichen 1, and discussed the diagenetic model of dolomites of well Xichen 1 (Xisha Islands):
Previous studies suggested that that the δ18O value of dolomitse in Well xichen-1 is 2‰–5‰ higher than that of non-dolomitic rocks, and the δ13C value is 3‰ higher than that of the non-dolomitic rocks. It is believed that this feature of enrichment of oxygen carbon heavy isotopes is a strong evidence of the increase of seawater salinity beyond the normal seawater. Some studies believe that the values of δ18O of dolomite in Well Xichen 1 are normal, which cannot explain that the diagenetic medium has salinity anomaly higher than sea water, but the δ13C value of the Xisha dolomites is too high and the δ13C value of the symbiotic calcites is too light. The dolomite in Well Xichen can be explained by the mechanism of mixed water dolomitization (He and Zhang, 1990). Combined with the test results of inclusions, some studies thought that dolomites in Well xichen-1 show the characteristics of high temperature and salinity, and it is likely to be affected by hydrothermal activities when it is formed (Zhao, 2010).
6 Conclusion
(1) The geochemical characteristics of REE in reef dolomite reservoirs in Well Xike 1 show that the main diagenetic environment of dolomite is low-temperature alkaline semi open oxidation environment; the carbon and oxygen isotopes of the dolomite are generally lack of correlation, δD is significantly lower than the hydrogen isotope value of seawater. Meanwhile, the oxygen isotope value of deep dolomites is negatively biased, which may be due to the increase or decrease of pore water temperature caused by deep thermal convection that related to the regional tectonic movements of the South China Sea.
(2) The δ18O value is also consistent with the geological reality of increasing saddle dolomite content in deep dolomites. The distribution of δ13C value indicates that the dolomite inherited the carbon of the original limestone during dolomitization, while the characteristic of δD value shows that it may be affected by the mixing of atmospheric precipitation and concentrated seawater in the quasi contemporaneous period.
(3) Based on the comprehensive analysis of the geochemical characteristics of the Well Xike 1, it is considered that the higher diagenetic temperature could be an important factor leading to the huge differences between the diagenetic model of deep and shallow dolomites.
(4) The geochemical characteristics of the shallow dolomites show that it is mainly reflux infiltration dolomitization under the micro evaporation and concentration sea water environments, while the deep dolomite is transformed by the hot water fluids in the epigenetic diagenetic evolution stage (Vasconcelos and McKenzie, 1997; Wei et al., 2006a; Wei, 2006b; Wei et al., 2008a; Wei et al., 2008b; Huang et al., 2009; Wang R. et al., 2018; Wang Z. et al., 2018).
Data availability statement
The original contributions presented in the study are included in the article/supplementary material, further inquiries can be directed to the corresponding author.
Author contributions
XL and ZS are responsible for the idea of this paper and LH and XH are responsible for the experiments.
Conflict of interest
The authors declare that the research was conducted in the absence of any commercial or financial relationships that could be construed as a potential conflict of interest.
Publisher’s note
All claims expressed in this article are solely those of the authors and do not necessarily represent those of their affiliated organizations, or those of the publisher, the editors and the reviewers. Any product that may be evaluated in this article, or claim that may be made by its manufacturer, is not guaranteed or endorsed by the publisher.
References
Aloisi, G., Pierre, C., Rouchy, J., Foucher, J. P., and Woodside, J. (2000). Methane-related authigenic carbonates of eastern mediterranean sea mud volcanoes and their possible relation to gas hydrate destabilisation. Earth Planet. Sci. Lett. 184 (1), 321–338. doi:10.1016/S0012-821X(00)00322-8
Arthur, M. A., and Schlanger, S. O. (1979). Cretaceous “oceanic anoxic events” as causal factors in development of reef-reservoired giant oil fields. Am. Assoc. Pet. Geol. Bull. 63 (6), 870–885. doi:10.1306/2F91848C-16CE-11D7-8645000102C1865D
Arvidson, R. S., and Mackenzie, F. T. (1999). The dolomite prob-lem: Control of precipitation kinetics by temperature and sat-uration state. Am. J. Sci. 299 (4), 257–288. doi:10.2475/ajs.299.4.257
Bi, D. J., Zhai, S. K., Zhang, D. J., Liu, X. F., Liu, X. Y., Jiang, L. J., et al. (2018). Constraints of fluid inclusions and C, O isotopic compositions on the origin of the dolomites in the Xisha Islands, South China Sea. Chem. Geol. 493, 504–517. doi:10.1016/j.chemgeo.2018.07.005
Bontognali, T. R., Vasconcelos, C., Warthmann, R. J., Bernasconi, S. M., Dupraz, C., Strohmenger, C. J., et al. (2010). Dolomite formation within microbial mats in the coastal sabkha of Abu Dhabi (United Arab E-mirates). Sedimentology 57 (3), 824–844. doi:10.1111/j.1365-3091.2009.01121.x
Braithwaite, C. J. R., Rizzi, G., and Darke, G. (2004). The geometry and petrogenesis of dolomite hydrocarbon reservoirs: Introduction. Geol. Soc. Lond. Spec. Publ. 235 (1), 1–6. doi:10.1144/GSL.SP.2004.235.01.01
Briais, A., Patriat, P., and Tapponnier, P. (1993). Updated interpretation of magnetic anomalies and seafloor spreading stages in the South China Sea: Implications for the Tertiary tectonics of Southeast Asia. J. Geophys. Res. 98 (B4), 6299–6328. doi:10.1029/92JB02280
Budd, D. A. (1997). Cenozoic dolomites of carbonate islands: Their attributes and origin. Earth-Science Rev. 42, 1–47. doi:10.1016/S0012-8252(96)00051-7
Chacko, T., and Deines, P. (2008). Theoretical calculation of oxygen isotope fractionation factors in carbonate systems. Geochimica Cosmochimica Acta 72 (15), 3642–3660. doi:10.1016/j.gca.2008.06.001
Craig, H., and Gordon, L. I. (1965). “Deuterium and oxygen-18 variations in the ocean and marine atmosphere,” in Proceedings of the conference on stable isotopes in oceanographic studies and paleotemperatures. Editor E. Tongiorgi (Lower Hutt, New Zealand: Laboratory of Geology and Nuclear Science), 9–130.
Craig, H. (1961). Isotopic variations in meteoric waters. Science 133 (3465), 1702–1703. doi:10.1126/science.133.3465.1702
D'Hondt, S., Inagaki, F., and Alvarez, Z. (2012). IODP expedition 329: Life and habitability beneath the seafloor of the South pacific gyre dolomitization of Neogene seroe domi formation coral reef limestones on curaçao, Netherlands antilles. Hague, Netherlands: Publications Foundation for Scientific Research in the Caribbean Region, 197–198. doi:10.2204/iodp.sd.15.01.2013
Dansgaard, W. (1964). Stable isotopes in precipitation. Tellus 16 (4), 436–468. doi:10.3402/tellusa.v16i4.8993
Fouke, B. W. (1994). Deposition, diagenesis and dolomitization of Neogene seroe domi formation coral reef limestones on curacao. Netherlands Antilles: Publications Foundation for scientific research in the Caribbean region.ISBN: 9074624030
Friedman, G. M., Ghosh, S. K., and Urschel, S. (1990). “Petrophysical characteristics related to depositional environments and diagenetic overprint: A case study of the san andres formation, mabee field, west Texas,” in Geologic and engineering approaches in evaluation of San Andres/Grayburg hydrocarbon reservoirs—Permian Basin. Editor D. G. Bebout, and P. M. Harris (Austin, Texas: Bureau of Economic Geology, The University of Texas at Austin), 125–144.
Friedman, I. (1953). Deuterium content of natural waters and other substances. Geochimica cosmochimica acta 4 (1-2), 89–103. doi:10.1016/0016-7037(53)90066-0
Fritz, P., and Smith, D. G. W. (1970). The isotopic composition of secondary dolomites. Geochimica Cosmochimica Acta 34 (11), 1161–1173. doi:10.1016/0016-7037(70)90056-6
Hodell, D. A., and Kennett, J. P. (1986). Late miocene–early Pliocene stratigraphy and paleoceanography of the South atlantic and southwest pacific oceans: A synthesis. Paleoceanography 1 (3), 285–311. doi:10.1029/PA001i003p00285
Holail, H., Lohmann, K. C., and Sanderson, I. (1988). Dolomitization and dedolomitization of upper cretaceous carbonates: Bahariya oasis, Egypt. Spec. Publ. 43, 191–207. doi:10.2110/pec.88.43.0191
Holser, W. T., Kaplan, I. R., Sakai, H., and Zak, I. (1979). Isotope geochemistry of oxygen in the sedimentary sulfate cycle. Chem. Geol. 25 (1-2), 1–17. doi:10.1016/0009-2541(79)90079-2
Hong, H., Su-ping, P., and Long-yi, S. (2004). Trace elements and sedimentary settings of Cambrian-Ordovician carbonates in Bachu area, Tarim Basin. Xinjiang Pet. Geol. 25 (6), 631. doi:10.3969/j.issn.1001-3873.2004.06.015
Hu, Z. W., Huang, S. J., and Huang, K. K. (2010a). Preservative evaluation of coeval seawater information for the Triassic marine carbonate rocks in the Huaying Mountain, eastern Sichuan. Geol. China 37 (5), 1374–1382. doi:10.3724/SP.J.1011.2010.01081
Hu, Z. W., Huang, S. J., Li, M. Z., Zang, X. H., Xu, E. S., and Liu, T. (2010b). Preliminary application of the dolomite-calcite oxygen isotope thermometer in studying the origin of dolomite in Feixianguan Formation, Northeast Sichuan, China. J. ChengDu Univ. Technol. Sci. Techonlogy Ed. 39 (1), 1–9. doi:10.3969/j.issn.1671-9727.2012.01.001
Huang, Sijing (2010). Carbonate diagenesis. Beijing, China: Geological Publishing House.ISBN.978-7-116-06938-1
Huang, S. J., Hu, Z. W., Zhong, Y. J., Huang, K. K., and Li, X. N. (2015). Saddledolomitein Permian—triassiccarbonaterocksand sandstones of Sichuan Basin: petrology, formation temperatureand palaeofluids. Journal of Chengdu University of Technology (Science and Technology Edition) 42 doi:10.3969/j.issn.1671-9727.2015.02.01
Huang, S. J., Huang, K. K., and Zhang, X. H. (2009). Chemical thermodynamics foundation of retrograde solubility for carbonate: Solution media related to CO2. J. Chengdu Univ. Technol. Sci. Technol. Ed. 36 (5), 457–464. doi:10.3969/j.issn.1671-9727.2009.05.001
Jiménez-López, C., Romanek, C. S., Huertas, F. J., Ohmoto, H., and Caballero, E. (2004). Oxygen isotope fractionation in synthetic magnesian calcite. Geochimica Cosmochimica Acta 68 (16), 3367–3377. doi:10.1016/j.gca.2003.11.033
Kaufman, A. J., and Knoll, A. H. (1995). Neoproterozoic variations in the C-isotopic composition of seawater: Stratigraphic and biogeochemical implications. Precambrian Res. 73 (1-4), 27–49. doi:10.1016/0301-9268(94)00070-8
Kawabe, I., Toriumi, T., Ohta, A., and Miura, N. (1998). Monoisotopic REE abundances in seawater and the origin of seawater tetrad effect. Geochem. J. 32 (4), 213–229. doi:10.2343/geochemj.32.213
Keith, M. L., and Weber, J. N. (1964). Carbon and oxygen isotopic composition of selected limestones and fossils. Geochim. Cosmochim. Acta 28 (10-11), 1787–1816. doi:10.1016/0016-7037(64)90022-5
Kenward, P., Goldstein, R., Gonzalez, L., and Roberts, J. (2009). Precipitation of low-temperature dolomite from an anaerobic microbial consortium:The role of methanogenic Archaea. Geobiology 7 (5), 556–565. doi:10.1111/j.1472-4669.2009.00210.x
Kyser, T. K., and O'Neil, J. R. (1984). Hydrogen isotope systematics of submarine basalts. Geochimica Cosmochimica Acta 48 (10), 2123–2133. doi:10.1016/0016-7037(84)90392-2
Land, L. S. (1998). Failure to precipitate dolomite at 25°C from di-lute solution despite 1000-fold oversaturation after 32 years. Aquat. Geochem. 4 (3), 361–368. doi:10.1023/A:1009688315854
Li, H., and Liu, Y. (2013). Dolomite problem” and research of ancient lacustrine dolostones. Acta Sedimentol. Sin. 3 (2), 302–315. doi:10.14027/j.cnki.cjxb.2013.02.017
Li, J., and Wang, G. P. (2004). Late Pliocene changs of upper ocean structure in the southern south China sea. Mar. Geol. Quat. Geol. doi:10.16562/j.cnki.0256-1492.2004.04.010
Lin, C., and Chu, F. (2009). On tectonic movement in the South China sea during the cenozoic. Acta Oceanol. Sin. 28 (1), 25–36. doi:10.1061/41042(349)25
Lu, C. L., Zhang, G. C., Yao, Y. J., and Wu, S. G. (2014). Tectonic evolution of the wanan basin, southwestern south China sea. Acta Geol. Sin. - Engl. Ed. 88 (4), 1120–1130. doi:10.1111/1755-6724.12277
Lu, J., and Chen, M. H. (2006). Global climate events since Cenozoic. J. Trop. Oceanogr. 25 (6), 72–79. doi:10.3969/j.issn.1009-5470.2006.06.013
Machel, H. G. (2004). Concepts and models of dolomitization: A critical reappraisal. Geol. Soc. Lond. Spec. Publ. 235 (1), 7–63. doi:10.1144/GSL.SP.2004.235.01.02
Mazzullo, S. J. (2000). Organogenic dolomitization in peritidal to deep-sea sediments. J. Sediment. Res. 70 (1), 10–23. doi:10.1306/2DC408F9-0E47-11D7-8643000102C1865D
Meng, L., and Zhang, J. (2014). The magmatic activity mechanism of the fossil spreading center in the Southwest sub-basin, South China Sea. Sci. China Earth Sci. 57 (7), 1653–1663. doi:10.1007/s11430-013-4788-x
Milliman, J. D. (1978). “Modern sediment carbonate,” in Marine carbonate, translated by institute of GeoloChinese Academy of sciences (Beijing, China: Geological Publishing House), 1. (in Chinese).
Ni, S., Ju, Y., Hou, Q., Wang, S., Liu, Q., Wu, Y., et al. (2009). Enrichment of heavy metal elements and their adsorption on iron oxides during carbonate rock weathering process. Prog. Nat. Sci. 19 (9), 1133–1139. doi:10.1016/j.pnsc.2009.01.008
Peng, R. M., Zhai, Y. S., Wang, Z. G., Han, X. F., Qin, J. W., Wang, J. P., et al. (2006). Characteristics and exploration of submarine sedex deposits in the Langshan-Zhaertai ore concentration area, Inner Mongolia. Mineral. Deposits 25 (S1), 221–224. doi:10.16111/j.0258-7106.2006.s1.058
Petrash, D. A., Bialik, O. M., Bontognali, T. R. R., Vasconcelos, C., Roberts, J. A., McKenzie, J. A., et al. (2017). Mi-crobially catalyzed dolomite formation: From near-surface to burial. Earth-Science Rev. 171, 558–582. doi:10.1016/j.earscirev.2017.06.015
Qing, H., and Mountjoy, E. W. (1994). formation of coarsely crystalline, hydrothermal dolomite reservoirs in the presquile barrier, western Canada sedimentary basin. Am. Assoc. Pet. Geol. Bull. 78 (1), 55–77. doi:10.1306/BDFF9014-1718-11D7-8645000102C1865D
Qiu, X., Ye, S., Wu, S., Shi, X., Zhou, D., Xia, K., et al. (2001). Crustal structure across the Xisha trough, northwestern south China sea. Tectonophysics 341, 179–193. doi:10.1016/S0040-1951(01)00222-0
Richard, A., Boulvais, P., Mercadier, J., Boiron, M. C., Cathelineau, M., Cuney, M., et al. (2013). From evaporated seawater to uranium-mineralizing brines: Isotopic and trace element study of quartz–dolomite veins in the Athabasca system. Geochimica Cosmochimica Acta 113, 38–59. doi:10.1016/j.gca.2013.03.009
Riding, R. (2002). Structure and composition of organic reefs and carbonate mud mounds: Concepts and categories. Earth-Science Rev. 58 (1-2), 163–231. doi:10.1016/S0012-8252(01)00089-7
Roberts, J. A., Kenward, P. A., Fowle, D. A., Goldstein, R. H., Gonzalez, L. A., and Moore, D. S. (2013). Surface chemistry allows for abiotic precipitation of dolomite at low temperature. Proc. Natl. Acad. Sci. U. S. A. 110 (36), 14540–14545. doi:10.1073/pnas.1305403110
Roberts, J., Bennett, P., González, L., Macpherson, G., and Milliken, K. L. (2004). Micro-bial precipitation of dolomite in methanogenic groundwater. Geol. 32 (4), 277. doi:10.1130/G20246.2
Sánchez-Román, M., Vasconcelos, C., Schmid, T., Dittrich, M., Mc, K., Zenobi, R., et al. (2008). Aerobic microbial dolomite at the nanometer scale: Implications for the geologic record. Geol. 36 (11), 879–882. doi:10.1130/G25013A.1
Schmidt, M., Xeflide, S., Botz, R., and Mann, S. (2005). Oxygen isotope fractionation during synthesis of CaMg-carbonate and implications for sedimentary dolomite formation. Geochimica Cosmochimica Acta 69, 4665–4674. doi:10.1016/j.gca.2005.06.025
Shi, Z. Q., Xie, Y. H., and Liu, L. (2016). South China Sea-Reservoir Characteristics and Diagenetic Evolution (in Chinese), 147. Wuhan, China: China University of Geosciences Press–167. 978-7-5625-3975-9.
Sibley, D. F. (1990). Unstable to stable transformations during dolomitization. J. Geol. 98 (5), 739–748. doi:10.1086/629437
Tarutani, T., Clayton, R. N., and Mayeda, T. K. (1969). The effect of polymorphism and magnesium substitution on oxygen isotope fractionation between calcium carbonate and water. Geochimica Cosmochimica Acta 33 (8), 987–996. doi:10.1016/0016-7037(69)90108-2
Taylor, B., and Hayes, D. E. (1983). Origin and history of the South China Sea basin, 27. Washington DC American Geophysical Union Geophysical Monograph Series, 23–56. doi:10.1029/GM027p0023
Taylor, S. R., and McLennan, S. M. (1985). The continental crust: Its composition and evolution. Oxford, England: Blackwell Scientific Publications, 312. doi:10.1017/S0016756800032167
Tian, J. C., and Zhang, X. (2016). Sedimentary geochemistry, 114-115. BeiJing: Geological Publishing House. 978-7-116-09646-2.
Tian, Q., Prange, M., and Merkel, U. (2016). Precipitation and temperature changes in the major Chinese river basins during 1957–2013 and links to sea surface temperature. J. Hydrology 536, 208–221. doi:10.1016/j.jhydrol.2016.02.048
Vasconcelos, C., McKenzie, J. A., Bernasconi, S., Grujic, D., and Tiens, A. J. (1995). Microbial mediation as a possible mechanism for natural dolomite formation at low temperatures. Nature 377 (6546), 220–222. doi:10.1038/377220a0
Vasconcelos, C., and McKenzie, J. A. (1997). Microbial mediation of modern dolomite precipitation and diagenesis under anoxic conditions (Lagoa Vermelha, Rio de Janeiro, Brazil). J. Sediment. Res. 67 (3), 378–390. doi:10.1306/D4268577-2B26-11D7-8648000102C1865D
Vasconcelos, C., Mckenzie, J. A., Warthmann, R., and Bernasconi, S. M. (2005). Calibration of the δ18O paleothermometer for dolomite precipitated in microbial cultures and natural environments. Geol. 33, 317–320. doi:10.1130/G20992.1
Wang, L., Lei, S., and Jia, L. (2011). The application of fluid inclusion in the study of mineral deposit. Gold Sci. Technol. 19 (4), 25–30. doi:10.3969/j.issn.1005-2518.2011.04.005
Wang, P., Tian, J., and Lourens, L. J. (2010). Obscuring of long eccentricity cyclicity in Pleistocene oceanic carbon isotope records. Earth Planet. Sci. Lett. 290 (3-4), 319–330. doi:10.1016/j.epsl.2009.12.028
Wang, R., Yu, K., Jones, B., Wang, Y., Zhao, J., Feng, Y., et al. (2018a). Evolution and development of Miocene “island dolostones” on Xisha islands, south China sea. Mar. Geol. 406, 142–158. doi:10.1016/j.margeo.2018.09.006
Wang, Z., Huang, K., Zhang, D., Liu, X., Luo, W., and You, L. (2017). Rare Earth element and yttrium (REY) geochemistry of cenozoic carbonates on the xuande atoll of the Xisha archipelago, the South China sea: Implications of the impact of dolomitization on REY. Geochem. J. 51 (6), 507–523. doi:10.2343/geochemj.2.0487
Wang, Z., Huang, K., Zhang, D., You, L., Liu, X., and Luo, W. (2018b). Maturation of neogene dolomite from xuande atoll of Xisha archipelago, the South China Sea. Mar. Petroleum Geol. 92, 51–64. doi:10.1016/j.marpetgeo.2018.02.016
Wei, X., Deng, J., and Xie, W. (2005). Constraints on biogenetic reef for mation during evolution of the South China Sea and exploration potential analysis. Earth Sci. Front. 12 (3), 245–252. doi:10.3321/j.issn:1005-2321.2005.03.026
Wei, X. (2006b). “Forming condition of Late-Cenozoic reef facies Carbonate rocks in Xisha sea area and their oil and gas exploration potential,”. PhD Thesis (Beijing, China: China University of Geosciences).
Wei, X., Jia, C. Z., and Meng, W. G. (2008b). Discussion on biogenetic reef distribution and hydrocarbon exploration direction in Xisha sea area since neogene. Oil Geophys. Prospect. 43 (3), 308–315. (in Chi-nese with English Abstract). doi:10.3321/j.issn:1000-7210.2008.03.013
Wei, X., Jia, C. Z., and Meng, W. G. (2008c). Dolomitization characteristics of carbonate rock in Xisha islands and its formation: A case study of well xichen-1. J. Jilin Univ. (Earth Sci. Ed. 38 (2), 217–224. doi:10.13278/j.cnki.jjuese.2008.02.006
Wei, X., Jia, C. Z., Meng, W. G., and Xiong, X. H. (2008a). Biogenetic reefs and its petrological characteristics of well Xichen 1, Xisha sea area, China. Geol. Bull. China 27 (11), 1933–1938. doi:10.3969/j.issn.1671-2552.2008.11.024
Wei, X., Jia, C. Z., Meng, W. G., and Zhang, F. L. (2007). Mineral content and geochemistry characteristics of carbonate rock in well No. Xichen-1 and geological significance. Acta Petrol. Sin. 23 (11), 3015–3025. (in Chinese with English abstract). doi:10.3969/j.issn.1000-0569.2007.11.031
Wei, X., Zhu, Y. J., and Yin, J. H. (2006a). Constrains and growing trend of biological reef in South China Sea Basin. Special Oil Gas Reservoirs 13 (1), 10–15. doi:10.3969/j.issn.1006-6535.2006.01.003
Xu, H., Zhao, X., Eberli, G. P., Liu, X., Zhu, Y., Cai, Y., et al. (2015). Biogenic carbonate formation and sedimentation in the Xisha islands: Evidences from living halimeda. Acta Oceanol. Sin. 34 (4), 62–73. doi:10.1007/s13131-015-0584-0
Yan, M., Chi, Q., Gu, T., and Wang, C. (1997). Chemical composition of upper crust in eastern China. Sci. China Ser. D-Earth. Sci. 40 (5), 530–539. doi:10.1007/BF02877620
Yan, P., Zhou, D., and Liu, Z. (2001). A crustal structure profile across the northern continental margin of the South China Sea. Tectonophysics 338, 1–21. doi:10.1016/S0040-1951(01)00062-2
Yin, H. (2020). MA thesis. Chengdu: Chengdu University of technology.Dolomite Genesis of Miocene Meishan Formation in the well xike-1, Xisha area, south China sea
You, X., Sun, S., and Zhu, J. (2011). Progress in the study of microbial dolomite model. Earth Sci. Front. 18 (4), 52–64. doi:10.11743/ogg20200119
Zachos, J., Pagani, M., Sloan, L., Thomas, E., and Billups, K. (2001). Trends, rhythms, and aber-rations in global climate 65 ma to present. Science 292, 686–693. doi:10.1126/science.1059412
Zhai, S. (2015). A preliminary study on the lithic resource utilization mode of the Mount Dagudui Site in Xiangfen County, Shanxi. Chin. Archaeol. 15 (1), 87–92. doi:10.1515/char-2015-0009
Zhang, J., and Nozaki, Y. (1996). Rare Earth elements and yttrium in seawater: ICP-MS determinations in the east caroline, coral sea, and south Fiji basins of the Western south Pacific ocean. Geochimica Cosmochimica Acta 60 (23), 4631–4644. doi:10.1016/S0016-7037(96)00276-1
Zhang, W., and Chen, Z. (1993). The geology of fluid inclusion. Wuhan: China University of Geosciences Press, 156.(in Chinese). ISBN: 7-5625-0885-2
Zhao, Q. (2010). PhD thesis. Qingdao, China: Chinese Academy of Sciences, 158. (in Chinese with English abstract).The sedimentary research about reef carbonate in Xisha Islands Waters
Keywords: isotope, REE, cenozoic carbonate, dolomite, island dolomitization, South China sea
Citation: Li X, Shi Z, Han L and Hu X (2023) Geochemical characteristics of hydrogen, oxygen, carbon isotopes and REE of cenozoic dolomites in Well Xike 1, Xisha Islands, South China sea and the significance for dolomitization in island-reef areas. Front. Earth Sci. 10:1064808. doi: 10.3389/feart.2022.1064808
Received: 08 October 2022; Accepted: 29 November 2022;
Published: 23 January 2023.
Edited by:
Shuai Yin, Xi’an Shiyou University, ChinaReviewed by:
Qin Zhang, China University of Petroleum, Beijing, ChinaJishun Pan, North China University of Water Conservancy and Electric Power, China
Copyright © 2023 Li, Shi, Han and Hu. This is an open-access article distributed under the terms of the Creative Commons Attribution License (CC BY). The use, distribution or reproduction in other forums is permitted, provided the original author(s) and the copyright owner(s) are credited and that the original publication in this journal is cited, in accordance with accepted academic practice. No use, distribution or reproduction is permitted which does not comply with these terms.
*Correspondence: Zhiqiang Shi, c3pxY2R1dDEyQDEyNi5jb20=