- Kunming University of Science and Technology, Geological Survey Center for Nonferrous Metals Resources, Southwest Institute of Geological Survey, Kunming, China
The Maoping superlarge germanium-rich lead–zinc deposit is a typical nonmagmatic hydrothermal deposit that is structurally controlled in the Sichuan–Yunnan–Guizhou lead–zinc polymetallic metallogenic area. The orebodies are distributed in several formations. This paper is based on large-scale alteration mapping combined with porosity and permeability measurements. We delineated the mineralization–alteration zones of different ore-bearing formations, explored the geological significance of porosity and permeability, and proposed prospecting directions. The research results indicate that during the mineralization period, the ore-forming metal fluids migrated from the deep part of the SSW region to the shallow part of the NNE region along the ore-guiding structure (Maoping Fault). Through the ore distribution structure, depressurization boiling occurred in the open space of the NE-trending interlayered sinistral compressive–torsional faults in several ore-bearing formations, resulting in fluid precipitation and the formation of different brecciated hot-melt dolomite lead–zinc mineralization zones. From the orebody to the wallrock, the C2w Formation and D3zg Formation are divided into four different mineralization–alteration zones. Tectonic activity affects the properties, migration, and precipitation of fluids, thereby controlling the alteration characteristics generated during fluid migration and thus changing the porosity and permeability. The porosity and permeability of strata on the NW flank of the anticline are greater than those of strata on the SE flank. On the NW flank, the greater the degree of mineralization–alteration is, the greater the porosity and permeability are, and the porosity of the orebody is lower than that during dolomitization. Finally, we believe that the NW flank of the anticline is an important area for prospecting. The pyrite + striped altered dolomite zone (Zones II–III) in the C2w limestone and the pyrite + strong dolomite zone (Zones II–III) in the D3zg dolomite are important prospecting indicators.
1 Introduction
In the next 2 decades, human consumption of base metals, such as lead, zinc and copper, will exceed the total production of these metals in human history (Ali et al., 2017; Hoggard et al., 2020). To date, nearly 300 germanium-rich lead–zinc (silver) deposits (occurrences) have been discovered in the Sichuan–Yunnan–Guizhou lead–zinc polymetallic metallogenic area in China. These lead–zinc deposits have produced more than 20 million tons of lead and zinc, and the deposits are exceptionally high grade and rich in associated germanium and silver (Han et al., 2022; 2023). This area is the largest lead–zinc industrial base and the world’s primary germanium production base (Han et al., 2022). With the long-term depletion of shallow mineral resources, there is a need for better targeted exploration to help improve research on deeply buried deposits and find deep mineral resources to maintain the supply of base metals for humankind.
Previous studies on mineralization–alteration zones have provided an important basis for the discovery of several deposits and orebodies in the Sichuan–Yunnan–Guizhou lead–zinc polymetallic metallogenic area (Wen et al., 2014; Chen et al., 2016; Zhao et al., 2016). The Maoping superlarge germanium-rich lead–zinc deposit is a typical deposit in the Sichuan–Yunnan–Guizhou lead–zinc polymetallic metallogenic area and is controlled by structure and favorable lithological associations (Han et al., 2019; Han et al., 2022; Wu et al., 2023), and orebodies occur in several ore-bearing formations (Han et al., 2022). Moreover, the mineralization–alteration characteristics and zoning patterns vary among different ore-bearing formations. This seriously restricts the prediction and positioning of deep hidden orebodies in different ore-bearing formations of this deposit and even this type of deposit.
In this study, a large-scale alteration mapping method is used to conduct detailed mapping and sampling on four ore blocks, nine planes, and several profiles of the Maoping lead–zinc deposit. The mineralization–alteration type, strength, mineral combination, structure, and other characteristics are analyzed, and the mineralization–alteration zones are identified. The porosity and permeability characteristics of 106 rocks (minerals) in different mineralization–alteration zones on the two flanks of the Maomaoshan anticline are analyzed. The geological significance of the porosity and permeability and proposed prospecting directions are explored. This study provides a theoretical basis for the deep exploration of other similar hydrothermal deposits.
2 Geological setting
The Maoping superlarge germanium-rich lead–zinc deposit is a typical superlarge CNHT lead–zinc deposit in the Sichuan–Yunnan–Guizhou lead–zinc polymetallic metallogenic area on the southwestern margin of the Yangtze block (Figure 1) (Yan et al., 2003; Qiu et al., 2016; Hu et al., 2017; Wu et al., 2020). The deposit is located at the intersection of the NE-trending Huize–Niujie oblique thrust–strike-slip fault–fold belt, the SN-trending Qujing–Zhaotong concealed fault zone, and the NW-trending Ziyun–Yadu deep fault zone (Figure 2) (Wang et al., 2004; Han et al., 2019). The deposit is mainly controlled by the NE-trending Maoping compressive–torsional fault (the names of these sequential transformation structural planes indicate that their mechanical properties are mainly composed of compressional structures and, secondarily, torsional structures) and the Maomaoshan anticline in its hanging wall (Figure 3). The main structures in the deposit area are NE-trending, NW-trending and N–S-trending structures, with the NE-trending interlayered sinistral compressive–torsional fault dominating (Figure 3). The deposit area is mainly composed of Devonian, Carboniferous, and Permian carbonate–clastic rock series, which are mostly in parallel unconformable or conformable contact. The orebodies mainly occur in the grayish-white–dark gray fine–medium crystalline dolomite of the upper Devonian Zaige Formation (D3zg), the light flesh-red or grayish-white massive fine crystalline dolomitic limestone of the lower Carboniferous Baizuo Formation (C1b), and the light gray–dark gray medium-to thick-layered dolomitic limestone of the upper Carboniferous Weining Formation (C2w) (Figures 3, 4). The magmatic rocks are mainly in the upper Permian Basalt Formation. The deposit is composed of the No. I, II, III and VI orebodies in the ore block to the east of the Luoze River and the Shuilu, Qiancengdong and Hongjianshan ore blocks to the west of the Luoze River (Figure 3). The orebodies are mainly lenticular, vein-like and stratiform-like. The main ore minerals are galena, sphalerite and pyrite, etc., and the gangue minerals are dolomite, calcite, minor quartz and barite, etc. The ore structures are compact, massive, disseminated, vein-like, veinlet-like, massive and stellate, and the ore textures are mainly granular and metasomatic. The main types of wallrock alteration include pyritization, ferritization, dolomitization, calcification and silicification.
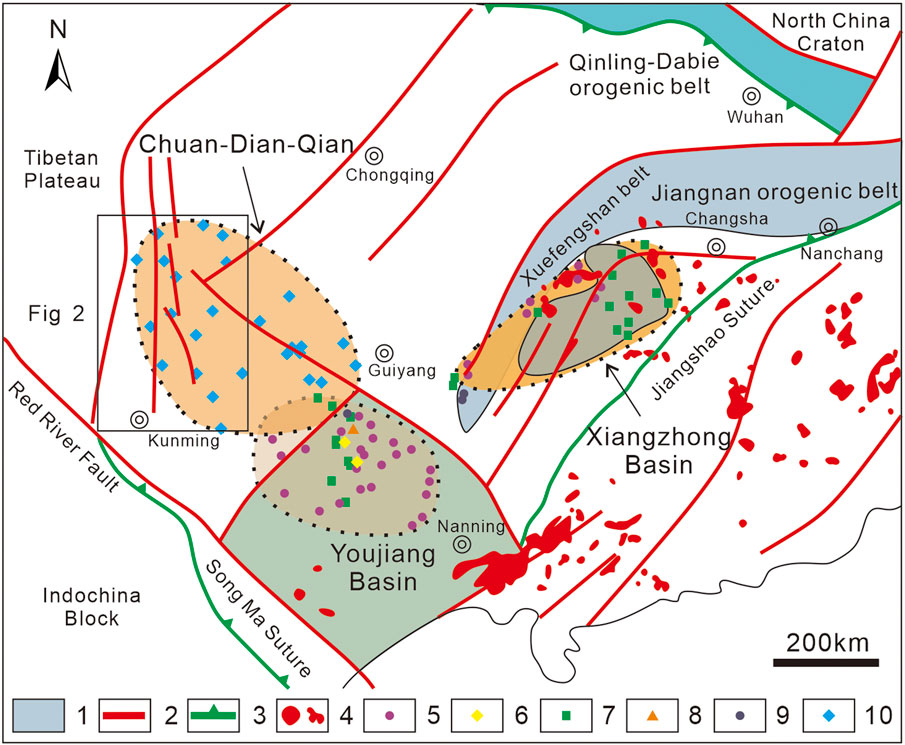
Figure 1. A simplified geological map of the South China Block and adjacent regions showing the structural framework and the distribution of ore deposits in the Yangtze Block (modified from Yan et al., 2003; Qiu et al., 2016; Hu et al., 2017; Wu et al., 2020). 1—Proterozoic basement; 2—Fault/Main boundary fault; 3—Suture zone; 4—Triassic granitoids; 5—Gold deposit; 6—Realgar deposit; 7—Stibnite deposit; 8—Tin deposit; 9—Mercury deposit; 10—Lead–zinc deposit.
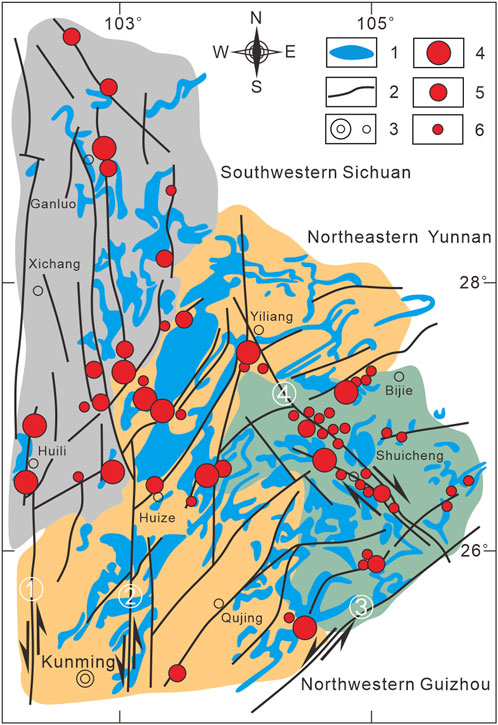
Figure 2. Distribution of the main thrust–fold belts and deposits in the Sichuan–Yunnan–Guizhou zinc–lead poly–metallic mineralization domain (SYGD) (Wang et al. 2004; Han et al. 2019). 1—The upper permian basalt Formation; 2—Main fault; 3—Provincial boundaries; 4—Provincial capital and city/county; 5—Large and medium–sized deposits; 6—Small deposits; ①–Lvzhijiang fault, ②–Xiaojiang fault, ③–Weining–Shuicheng fault, ④–Ziyun–Yadu fault.
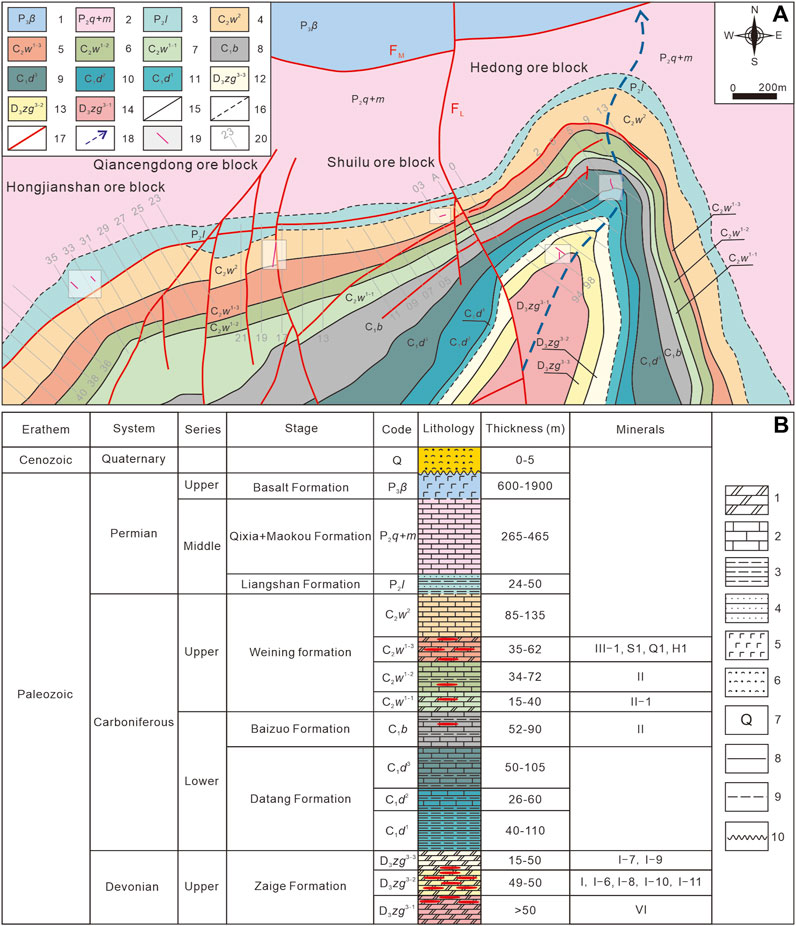
Figure 3. Geological map, actual material plane projection map (A) and Stratigraphic histogram of the Maoping lead-zinc deposit (B) (Wu et al. 2024). Figure 3A: 1—Upper Permian Basalt Formation; 2—Middle Permian Qixia+Maokou Formation; 3—Middle Permian Liangshan Formation; 4—Upper Carboniferous Weining Formation; 5—The third section of the first layer of the Weining Formation in the Upper Carboniferous; 6—The second section of the first layer of the Weining Formation in the Middle Carboniferous; 7—The first section of the first layer of the Weining Formation in the Upper Carboniferous; 8—Lower Carboniferous Baizuo Formation; 9—The third layer of the Lower Carboniferous Datang Formation; 10—The second layer of the Lower Carboniferous Datang Formation; 11—The first layer of the Lower Carboniferous Datang Formation; 12—The third layer of the third member of the Upper Devonian Zaige Formation; 13—The second layer of the third section of the Upper Devonian Zaige Formation; 14—The first layer of the third section of the Upper Devonian Zaige Formation; 15—Integrated contact; 16—Parallel unconformity contact; 17—Fault; 18—Anticline axis; 19—The location of the cross sections; 20—Exploration lines and numbering. Figure 3B: 1—Dolomite; 2—Limestone; 3—Mudstone; 5—Sandstone; 5—Basalt; 6—Residual matter; 7—Stratum code; 8—Integrated contact; 9—False integration contact; 10—Angular unconformity contact.
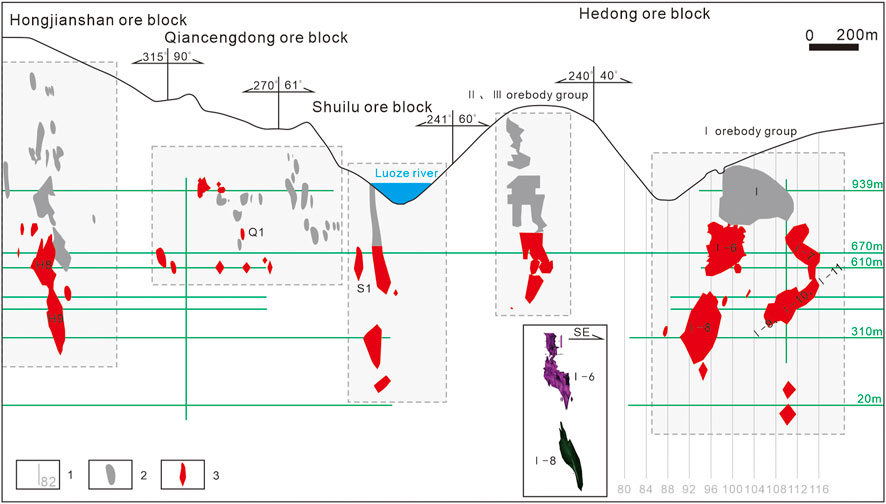
Figure 4. Orebody longitudinal projection map of Maoping lead–zinc deposit. 1—Exploration line and number; 2—Mined out orebodies; 3—Known orebodies.
3 Materials and methods
In this study, a large-scale alteration mapping method was used to conduct detailed mapping and systematically determine the mineralization–alteration zoning characteristics within different ore-bearing strata of the deposit. On the basis of the systematic study of mineralization–alteration characteristics (Table 1), the porosity and permeability of representative rocks were analyzed. The rock samples for the porosity and permeability study were collected from the NW and SE flanks of the Maomaoshan anticline, and they were collected from the surface of the mining area and the mining tunnels to obtain a total of 106 pieces. Among them, 58 fresh samples were collected from different lithological formations on the surface. A total of 48 samples from the tunnel were collected from the 32–33+first line of 670 m of the H8 orebody in the Hexi Hongjianshan ore block, the 34+first line of 610 m of the H8 orebody in the Hexi Hongjianshan ore block, the 18th line of the 755 m of the Q1 orebody in the Hexi Qiancengdong ore block, the second line of the 670 m of the S1 orebody in the Hexi Shuilu ore block, the 116th line of the 760 m of the I-7 orebody in the Hedong ore block, the 98+first line of the 683 m of the I-6 orebody in the Hedong ore block, the 92nd line of the 670 m in the Hedong ore block, the 96–112nd line of the 610 m of the I-6 orebody in the Hedong ore block, the stope of the 490 m of the I-8 orebody in the Hedong ore block, the stope of the 430 m of the I-8 orebody in the Hedong ore block, the 90–96th lines of the 370 m of the I-8 orebody in the Hedong ore block, and the 98–104th lines of the 310 m of the I-8 orebody in the Hedong ore block. The samples were tested for porosity and permeability by using a QKY-2 gas porosity tester and a STY-2 gas permeability tester at the China University of Petroleum (Beijing). The former had a measurement accuracy of 0.5%, while the latter had a relative error of less than 5%. Nitrogen was used as the working medium in the experiment, and the test was repeated five times. The average value was taken as the result for porosity or permeability (Table 2).
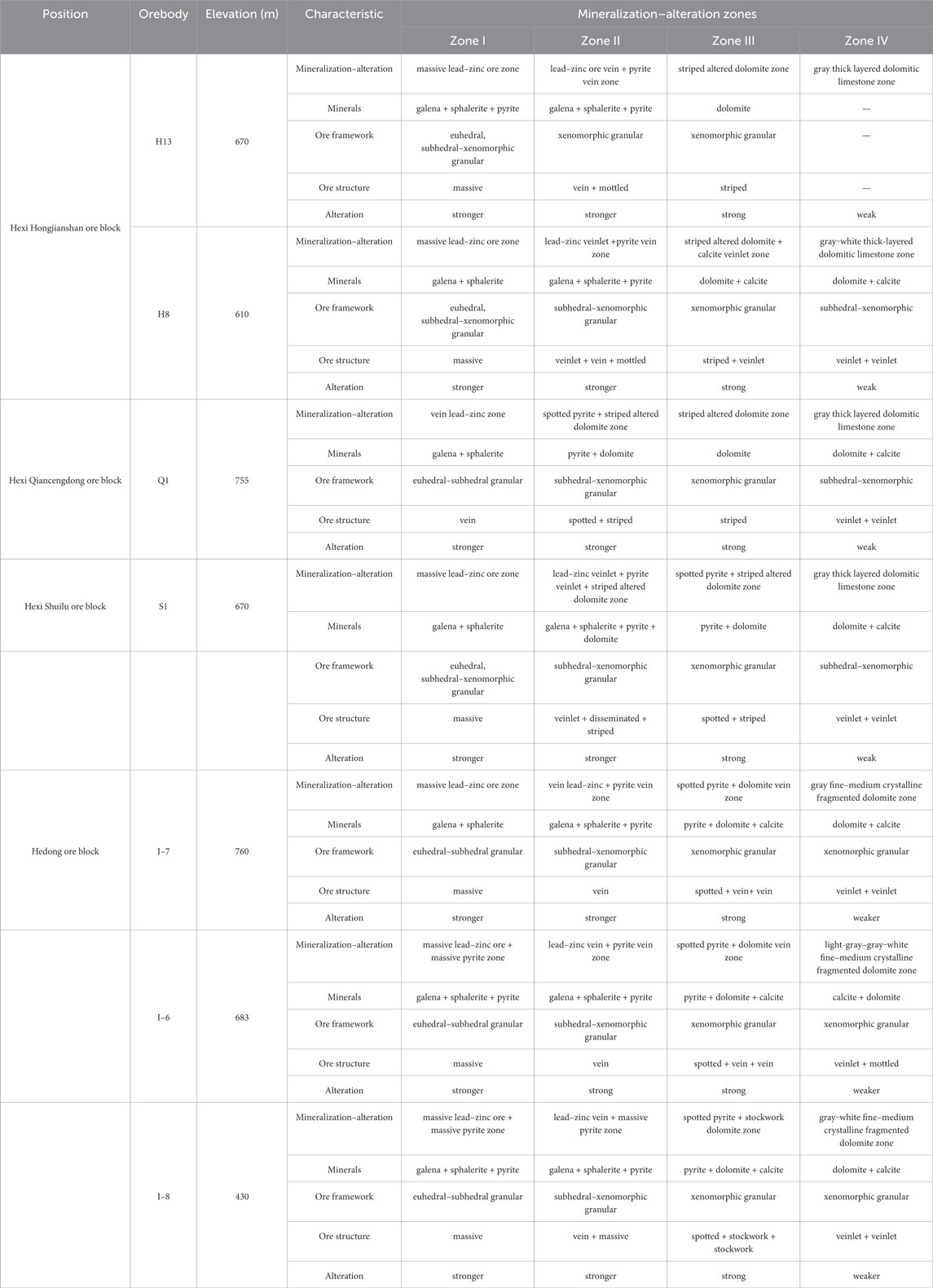
Table 1. Typical characteristics of the mineralization–alteration zones of different orebodies in the NW flank of the anticline.

Table 2. Porosity and permeability results for different positions, different ore-bearing formations, and different alteration zones.
4 Results
4.1 Characteristics and zones of mineralization–alteration
The mineralization of the Maoping lead–zinc deposit is mainly that of galena and sphalerite. The wallrock alteration type is relatively simple, and the intensity changes and zoning are obvious. These mainly include pyritization, (iron) dolomitization and calcification. The main alteration characteristics are as follows (Figures 5–7).
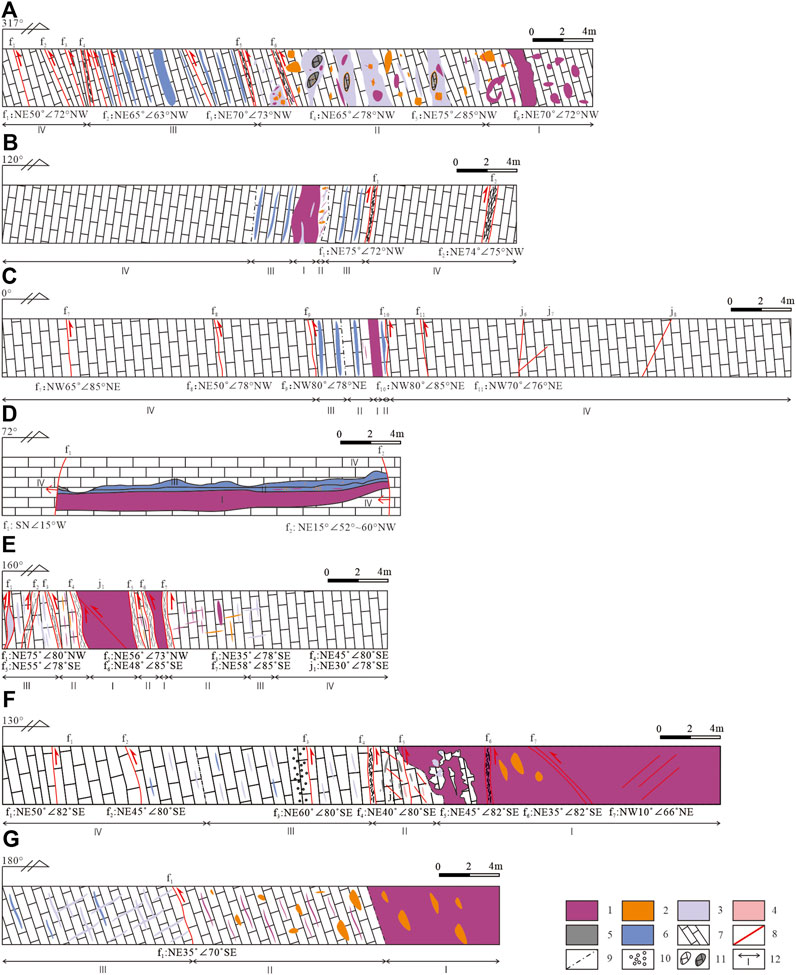
Figure 5. Typical mineralization–alteration zones geological profile of Maoping lead–zinc deposit. The expression of fault occurrence is: trend∠dip–direction. 1—Orebody; 2—Pyrite; 3—Calcite; 4—Sphalerite; 5—Galena; 6—Dolomite; 7—Formation; 8—Fault; 9—Alteration boundary; 10—Corrosion hole; 11—Wall-rock breccia; 12—Alteration zoning. (A)—the 32–33+1st line of 670 m of the H8 orebody in the Hexi Hongjianshan ore block; (B)—the 34+1st line of 610 m of the H8 orebody in the Hexi Hongjianshan ore block; (C)—the 18th line of the 755 m of the Q1 orebody in the Hexi Qiancengdong ore block; (D)—the 2nd line of the 670 m of the S1 orebody in the Hexi Shuilu ore block; (E)—the 116th line of the 760 m of the I-7 orebody in the Hedong ore block; (F)—the 98+1st line of the 683 m of the I-6 orebody in the Hedong ore block; (G)—the stope of the 430 m of the I-8 orebody in the Hedong ore block.
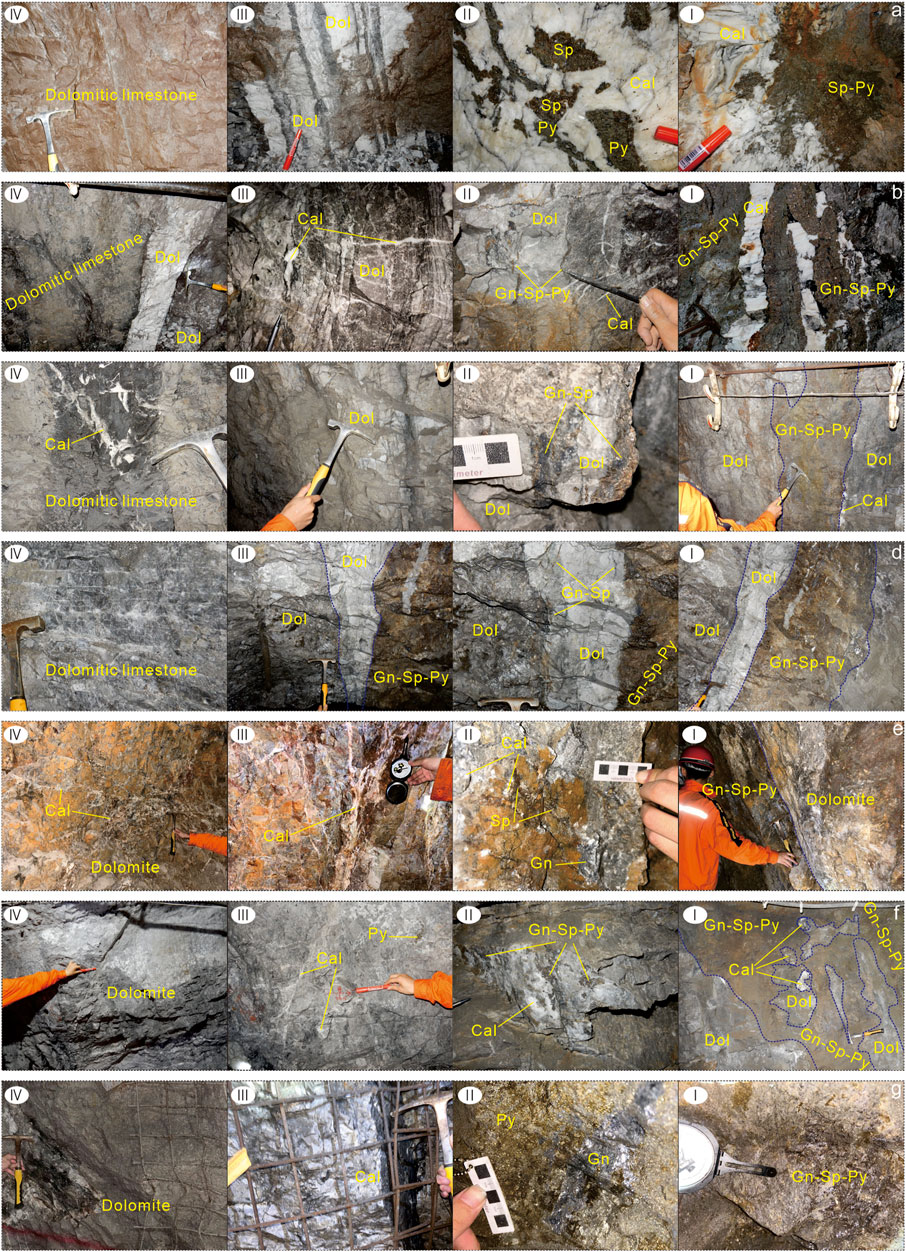
Figure 6. Geological profile of Maoping lead–zinc deposit mineralization–alteration zones field macroscopic characteristics. (A, B) is the same as Figure 4; I-IV represents various mineralization–alteration zones.
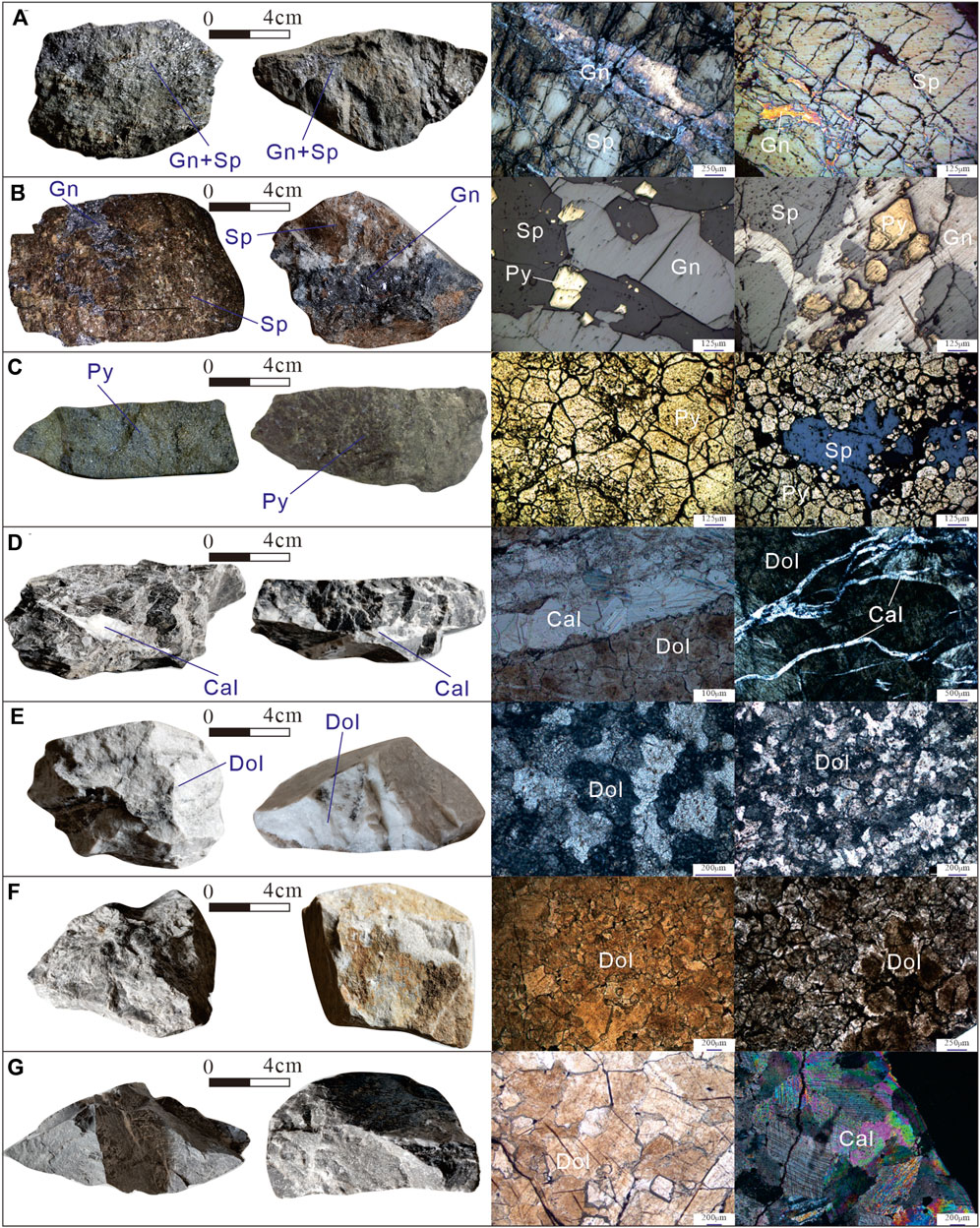
Figure 7. Typical mineralization–alteration samples of the Maoping lead–zinc deposit and their microscopic and Macroscopic characteristics. (A)—Massive lead–zinc ore in dolomite of D3zg formation, (B)—Massive lead–zinc ore in dolomite of C2w formation; (C)—Massive pyrite, (D)—vein calcite, (E)—Striped altered dolomite zone, (F)—Unaltered dolomite, (G)—Unaltered dolomitic limestone.
Pyritization: Pyritization is mainly distributed in the dolomite, calcite, joints and faults near the ore in massive structures, disseminated structures, masses, veins, and veinlets, and pyrite is also common in the orebodies. The intensity of pyritization is related to its distance from the orebody. The pyritization near the orebodies is strong, and the pyritization far from the orebodies gradually weakens. In addition, the pyritization is also related to elevation, and deep pyritization is obviously stronger than shallow pyritization.
Dolomitization: Due to the influence of hydrothermal activity, the original dolomite limestone or dolomite is recrystallized, resulting in a significant increase in porosity, which is beneficial for hydrothermal activity and mineralization, thus leading to the formation of altered dolomite. Dolomite is mainly found in striped structures, veins, masses, veinlets, and irregular structures; is mainly distributed on the sides of orebodies and the sides of interlayer faults; and has a semi-idiomorphic granular framework. The particle sizes are 0.2–1 mm, and the color is diverse—mainly white, gray–white, beige, and flesh red; in addition, corrosion holes and crystal cavities have developed. Dolomitization is more evident in the C2w dolomitic limestone, where it appears as a stripe; the D3zg dolomite mainly appears in a rice-grain-type form, with the presence of long-axis parallel interlayer faults.
Calcification: Calcification is present mainly in the forms of stockwork, veins, masses, and veinlets, and it is present mainly on the sides of interlayer faults and on the sides of orebodies in dolomitic limestone and dolomite.
Based on the composition, structure, framework, and strength of the altered rocks, combined with their spatial relationships with orebodies, the mineralization–alteration zones were delineated. From the orebodies to the wallrocks in the hanging wall, the mineralization–alteration zones of the C2w Formation exhibit the following order: massive lead–zinc ore zone (I) → lead–zinc ore vein + pyrite vein zone + striped altered dolomite (Zone II) → striped altered dolomite zone (Zone III) → gray thick-layered dolomitic limestone zone (Zone IV). The mineralization–alteration zones of the D3zg Formation exhibit the following order: massive lead–zinc ore + massive pyrite zone (Zone I) → lead–zinc ore vein + pyrite vein zone (Zone II) → spotted pyrite + dolomite vein zone (Zone III) → gray–white fine–medium crystalline dolomite zone (Zone IV). The detailed features and descriptions are shown in Figures 5–7; Table 1.
4.2 Porosity and permeability results for the two flanks of the anticline
For all the measured rock samples, the overall porosity and permeability changed greatly; the porosities ranged from 0.91% to 10.08%, and the permeabilities ranged from 0.003005251 – 17.68385095 × 10−3 μm2 (Table 2).
The porosity–permeability scatter plot shows a positive correlation between the two flanks, and the slope (R) is 0.28827 ± 0.06658 (Figure 8).
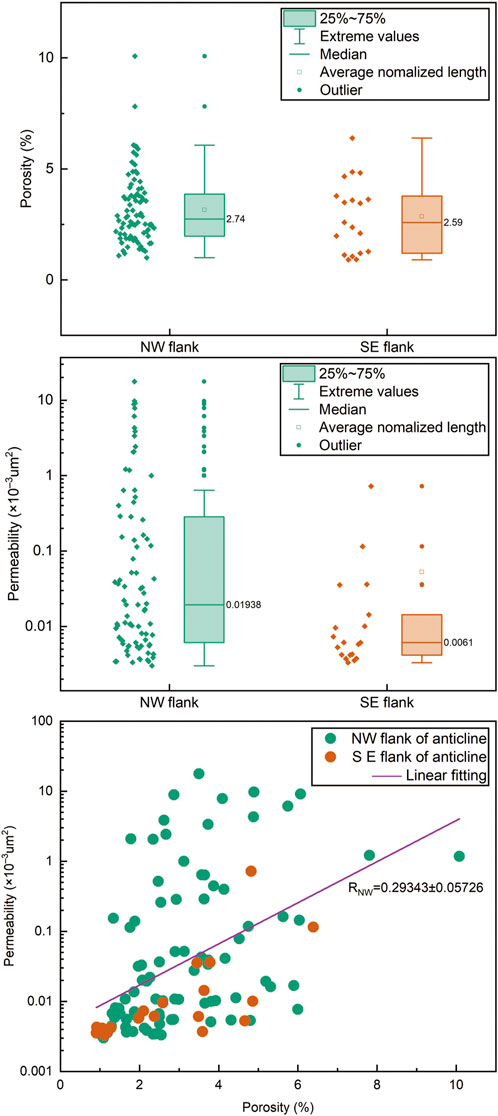
Figure 8. Distribution and correlation maps of porosity and permeability on the two flanks of the anticline.
The porosity of 1%–10.08% in the NW flank was greater than that of 0.91%–6.39% in the SE flank. The median value of the NW flank (2.74%) was greater than that of the SE flank (2.59%) (Figure 8).
The permeability of 0.003005251 – 17.68385095 × 10−3 μm2 in the NW flank was greater than that of 0.003311688 – 0.723114436 × 10−3 μm2 in the SE flank. In addition, the median value of the NW flank (0.01938 × 10−3 μm2) was greater than that of the SE flank (0.0061 × 10−3 μm2) (Figure 8).
4.3 Porosity and permeability results for the NW flank of the anticline
The porosities and permeabilities of rocks with different degrees of mineralization–alteration in the two main ore-bearing formations (C2w and D3zg) on the NW flank of the anticline were analyzed (Figure 9).
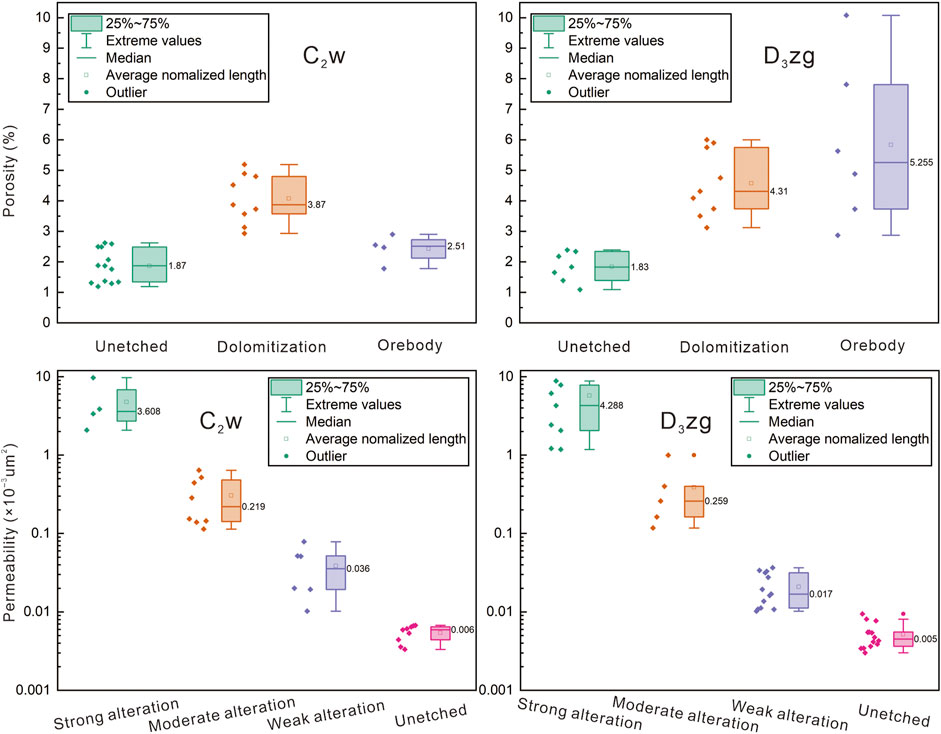
Figure 9. Porosity and permeability characteristics of different types and intensities with mineralization–alteration in NW flank of the anticline.
The porosity results show that in the C2w Formation, the percentage of unaltered rock (1.19%–2.62%; median value: 1.87%) is the lowest, followed by the orebody (1.78%–2.90%; median value: 2.51%), and the highest percentage of dolomitized rock (2.93%–5.19%; median value: 3.87%). The porosity results show that in the D3zg Formation, the percentage of unaltered rock (1.09%–2.39%; median value: 1.83%) is the lowest, followed by dolomitized rock (3.12%–5.90%; median value: 4.31%), and the highest percentage of orebody rock (2.87%–10.08%; median value: 5.26%).
The permeability results show that for both the C2w and D3zg Formations, the permeability also decreases with decreasing erosion. In the C2w Formation, the median values of strong alteration, moderate alteration, weak alteration, and no alteration are 3.608, 0.219, 0.036 and 0.006, respectively; in the D3zg Formation, the median values of strong alteration, moderate alteration, weak alteration, and no alteration are 4.288, 0.259, 0.017 and 0.005, respectively.
5 Discussion
5.1 The geological significance of porosity and permeability
The fault in this study is a complex four-dimensional tectonic unit composed of a fault surface and tectonite, and its tectonic deformation and fluid flow characteristics evolve over time Wibberley and Shipton. (2010). Tectonics are not only the dominant factor controlling the coupling relationship between geological bodies but also the main driving force for fluid migration; various tectonic features, such as faults, fractures, and breccia zones, provide channels for fluid migration in the subsurface (Zhai, 1996). These features can also enhance permeability, hydraulic conductivity, and hydrothermal flow, thereby increasing the mineralization potential of favorable sedimentary locations (Henderson and McCaig, 1996; Cox et al., 2001; Bauer et al., 2022). The degree of rock fragmentation, permeability, local porosity and fluid flux are different in different parts where tectonic deformation occurs (such as bends and branches of fracture zones), which leads to different mineralization types and mineralization intensities (Soden et al., 2014; Zhang, 2017).
Fluids can control the deformation process of rocks and even alter the deformation mechanism of rocks through physical changes or chemical reactions, such as hydrolysis weakening and reducing the friction coefficient between mineral particles Wintsch et al. (1995). The dissolution and precipitation of minerals during water‒rock reactions can cause changes in fluid composition and affect rock properties (Wawrzyniec, 1999). The properties of the fluid are the main factors controlling microprocesses, such as the generation of cementitious materials, pore characteristics, and mineral precipitation in the host rock (Wu et al., 2015). Carbonate minerals not only metasomatically fill pores but also dolomitize and recrystallize in limestone and dolomite (Corbella et al., 2004), forming medium–coarse-grained altered dolomite, allomorphic granular mineral particles at the microscale, and calcite veins, dolomite veins, and pyrite veins, thus increasing the porosity and permeability.
Tectonic action affects the properties, migration, and precipitation of fluids, thereby controlling the alteration characteristics generated during fluid migration and changing the porosity and permeability. Different types and degrees of alteration have different porosities and permeabilities (Figures 8, 9).
Research has shown that, overall, the NW flank of the Maomaoshan anticline has greater porosities and permeabilities than the SE flank (Figure 8). The strata in the NW flank of the Maomaoshan anticline are steeply inclined and inverted (with dip angles of 55°–85°), while those in the SE flank are gently inclined (with dip angles of 20°–35°). The orebody in this deposit is controlled by interlayer faults. Under the action of tectonic forces, when the local principal compressive stress directions on both flanks are consistent, the strata in the NW flank have a large dip angle, and the compressive surface of the NW flank exhibits mainly compressive stress, as well as shear resistance. The strata in the SE flank have a small dip angle, and the compressive surface of the SE flank exhibits mainly shear resistance, followed by tensile resistance. When relative sliding occurs in interlayer faults, they are affected not only by the principal compressive stress but also by the gravity of the rock or the block itself. Both the NW flank and SE flank interlayer faults are known to have experienced relative motion. For the same rock formation, the compressive strength is generally greater than the shear strength, making it more prone to shear deformation. Therefore, the SE flank can achieve only the minimum force required for shear resistance before relative sliding occurs, resulting in compressional–torsional faulting and stress release. The NW flank achieves not only the minimum force required for shear resistance but also the minimum force required for rock compression resistance. Not only does relative sliding occur, but it also causes damage to the rock, forming an open space. This is more conducive to fluid “penetration”.
Therefore, the NW flank of the anticline is more conducive to mineralization.
5.2 Metallogenic patterns and prospecting directions
During the mineralization period, the ore-forming fluid migrated upward along the main ore-guiding structure (NE-trending Maoping sinistral compressive–torsional fault) and generally migrated from deep in the SSW region to shallow in the NNE region. Through the ore distribution structure (SN-trending Luozehe sinistral torsional fault, NE-trending Maomaoshan compound overturned anticline, NNW-trending sinistral torsional–extensional fault, and NE-trending subsequent compressional–torsional fault), the fluid reached the NE-trending interlayered sinistral compressive–torsional faults (Figure 10) (Wu et al., 2023), and hydrothermal “penetration” metasomatism occurred.
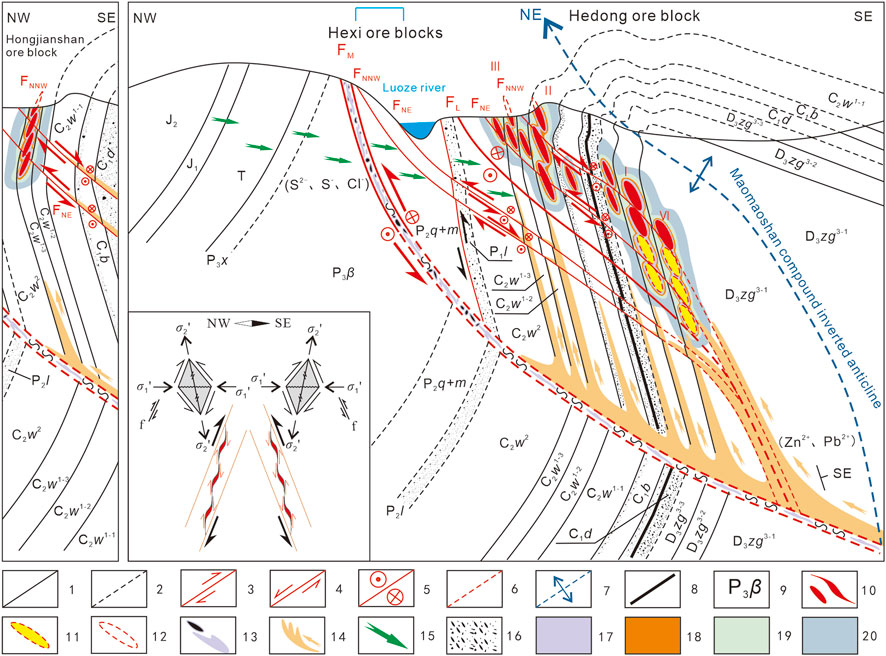
Figure 10. Ore-control model and mineralization–alteration zones pattern of Maoping lead–zinc deposit (According to Wu et al., 2023; Han et al. 2024). 1—Integrated contact; 2—Parallel unconformity contact; 3—Compressive fault; 4—Tensile fault; 5—Torsional fault; 6—Inferred fault; 7—Anticline; 8—Coal-bearing; 9—Formation code; 10—Known orebodies; 11—Predicting orebody; 12—Denuded orebody; 13—Dolomitization and its cemented limestone breccia; 14—Ore-forming metals fluids; 15—Reducing fluids; 16—Argillaceous and sandy clastic rock; 17—zone I; 18—zone II; 19—zone III; 20—zone IV.
Due to the effect of the overlying clastic rock barrier, when deep-source fluids rich in Pb2+, Zn2+, and Ge2+ and other cations and basin fluids rich in reduced sulfur entered the expansion space, boiling decompression occurred in the overlying host carbonate bed under the barrier layer, and the pH increased, resulting in mineral precipitation. The medium–coarse-grained altered dolomite that formed in the early stage of fluid interaction was conducive to further water–rock interactions between the fluid and the surrounding rock, leading to the precipitation of lead and zinc minerals (acid generation) and the mutual promotion of wallrock alteration (acid consumption) (Zhang et al., 2016; Ali et al., 2017; Korges et al., 2018; Zhang et al., 2019), thus forming a zone of brecciated hot-solution dolomite lead–zinc mineralization (Figure 10).
The striped altered dolomite zone (Zones II–III) in the C2w limestone and the pyritization + strong dolomitization zone (Zones II–III) in the D3zg dolomite are important prospecting indicators.
6 Conclusion
From the orebodies to the wallrocks in the hanging wall, the mineralization–alteration zones of the C2w Formation range from the massive lead–zinc ore zone (I) → lead–zinc ore veinlet + pyrite vein zone (Zone II) → spotted pyrite + striped altered dolomite zone (Zone III) → calcite veinlet + gray thick-layered dolomitic limestone zone (Zone IV). The mineralization–alteration zones of the D3zg Formation range from the massive lead–zinc ore + massive pyrite zone (Zone I) → lead–zinc ore vein + pyrite vein zone (Zone II) → spotted pyrite + calcite stockwork/vein zone (Zone III) → calcite veinlet + masses of dolomite + gray–white cataclastic medium–coarse dolomite zone (Zone IV).
The porosity and permeability of the NW flank of the anticline are greater than those of the SE flank. On the NW flank, the greater the degree of mineralization–alteration is, the greater the porosity and permeability are, and the porosity of the orebody is lower than that during dolomitization. Tectonic activity affects the properties, migration, and precipitation of fluids, thereby controlling the alteration characteristics generated during fluid migration and changing the porosity and permeability.
During the mineralization period, the ore-forming metal fluids migrated from the deep part of the SSW region to the shallow part of the NNE region along the ore-guiding structure (Maoping Fault). Through the ore distribution structure, depressurization boiling occurred in the open space of the NE-trending interlayer sinistral compressive–torsional faults in several ore-bearing formations, resulting in fluid precipitation and the formation of different lead–zinc mineralization zones in brecciated hot-melt dolomite.
The NW flank of the Maomaoshan anticline is an important prospecting area. The pyrite + striped altered dolomite zone (Zones II–III) in the C2w limestone and the pyrite + strong dolomite zone (Zones II–III) in the D3zg dolomite are important prospecting indicators.
Data availability statement
The original contributions presented in the study are included in the article/Supplementary Material, further inquiries can be directed to the corresponding author.
Author contributions
JW: Conceptualization, Formal Analysis, Investigation, Methodology, Software, Validation, Visualization, Writing–original draft, Writing–review and editing. RH: Conceptualization, Data curation, Funding acquisition, Investigation, Methodology, Project administration, Resources, Supervision, Validation, Writing–review and editing. YZ: Formal Analysis, Investigation, Project administration, Resources, Writing–review and editing. PW: Formal Analysis, Investigation, Methodology, Resources, Writing–review and editing. HG: Formal Analysis, Investigation, Methodology, Writing–review and editing. LW: Formal Analysis, Investigation, Writing–review and editing. GC: Investigation, Writing–review and editing. XL: Investigation, Writing–review and editing. YY: Investigation, Writing–review and editing. YM: Investigation, Writing–review and editing.
Funding
The author(s) declare that financial support was received for the research, authorship, and/or publication of this article. This work was financed jointly by National Natural Science Foundation of China (42172086, U1133602), Yunnan Major Scientific and Technological Projects (grant no. 202202AG050014), Key Projects of School–Enterprise Cooperation (2020CHYCDZB08), Yunnan Mineral Resources Prediction and Evaluation Engineering Research Center (2011), and Yunnan Provincial Geological Process and Mineral Resources Innovation Team (2012).
Conflict of interest
The authors declare that the research was conducted in the absence of any commercial or financial relationships that could be construed as a potential conflict of interest.
Publisher’s note
All claims expressed in this article are solely those of the authors and do not necessarily represent those of their affiliated organizations, or those of the publisher, the editors and the reviewers. Any product that may be evaluated in this article, or claim that may be made by its manufacturer, is not guaranteed or endorsed by the publisher.
References
Ali, S. H., Giurco, D., Arndt, N., Nickless, E., Brown, G., Demetriades, A., et al. (2017). Mineral supply for sustainable development requires resource governance. Nature 543 (7645), 367–372. doi:10.1038/nature21359
Bauer, T. E., Lynch, E. P., Sarlus, Z., Drejing-Carroll, D., Martinsson, O., Metzger, N., et al. (2022). Structural controls on iron oxide copper–gold mineralization and related alteration in a paleoproterozoic supracrustal belt: insights from the nautanen deformation zone and surroundings, northern Sweden. Econ. Geol. 117, 327–359. doi:10.5382/econgeo.4862
Chen, S. H., Han, R. S., Shentu, L. Y., Wu, P., Qiu, W. L., and Wen, D. X. (2016). Alteration zoning and geochemical element migration in alteration rock of Zhaotong lead-zinc deposit in northeastern Yunnan mineralization concentration Area. J. Jilin Univ. (Earth Sci. Ed.) 46 (3), 711–721. doi:10.13278/j.cnki.jjuese.201603109
Corbella, M., Ayora, C., and Cardellach, E. (2004). Hydrothermal mixing, carbonate dissolution and sulfide precipitation in Mississippi Valley-type deposits. Miner. Deposita 39, 344–357. doi:10.1007/s00126-004-0412-5
Cox, S. F., Knackstedt, M. A., and Braun, J. (2001). Principles of structural control on permeability and fluid flow in hydrothermal systems.
Han, R. S., Wu, J. B., Zhang, Y., Chen, Q., and Sun, B. T. (2024). Oblique distribution patterns and the underlying mechanical model of orebody groups controlled by structures at different scales. Sci. Rep. 14 (1), 4591. doi:10.1038/s41598-024-55473-z
Han, R. S., Wu, P., Wang, F., Zhou, G. M., Li, W. Y., and Qiu, W. L. (2019). Four Steps Type’ore-prospecting method for deeply concealed hydrothermal ore deposits—a case study of the Maoping Zn-Pb-(Ag-Ge) deposit in Southwestern China. Geotecton. Metallog. 43, 246–257. doi:10.16539/j.ddgzyckx.2019.02.005
Han, R. S., Wu, P., Zhang, Y., Huang, Z. L., Wang, F., Jin, Z. G., et al. (2022). New research progresses of metallogenic theory for rich Zn-Pb-(Ag-Ge) deposits in the Sichuang-Yunnan-Guizhou Triangle (SYGT) area, southwestern Tethys. Acta Geol. Sin. 96, 554–573.
Han, R. S., Zhang, Y., Ye, T. Z., Chen, Q., Ren, T., Guo, Z. L., et al. (2023). An overview of the metallogeny and geological prospecting model of Mississippi valley type (MVT) lead and zinc deposits. Geotect. Metallogenia 47, 915–932.
Henderson, I. H. C., and McCaig, A. M. (1996). Fluid pressure and salinity variations in shear zone–related veins, central Pyrenees, France: implications for the fault–valve model. Tectonophysics 262, 321–348. doi:10.1016/0040-1951(96)00018-2
Hu, R. Z., Fu, S. L., Huang, Y., Zhou, M. F., Fu, S. H., Zhao, C. H., et al. (2017). The giant South China Mesozoic low-temperature metallogenic domain: reviews and a new geodynamic model. J. Asian Earth Sci. 137, 9–34. doi:10.1016/j.jseaes.2016.10.016
Korges, M., Weis, P., Lüders, V., and Laurent, O. (2018). Depressurization and boiling of a single magmatic fluid as a mechanism for tin-tungsten deposit formation. Geology 46 (1), 75–78. doi:10.1130/g39601.1
Qiu, L., Yan, D. P., Tang, S. L., Wang, Q., Yang, W. X., Tang, X., et al. (2016). Mesozoic geology of southwestern China: indosinian foreland overthrusting and subsequent deformation. J. Asian Earth Sci. 122, 91–105. doi:10.1016/j.jseaes.2016.03.006
Soden, A. M., Shipton, Z. K., Lunn, R. J., Pytharouli, S. I., Kirkpatrick, J. D., Do Nascimento, A. F., et al. (2014). Brittle structures focused on subtle crustal heterogeneities: implications for flow in fractured rocks. J. Geol. Soc. 171, 509–524. doi:10.1144/jgs2013-051
Wang, B. L., Li, L. H., and Zeng, P. S. (2004). Basic geophysic characteristics of Sichuan-Yunnan-Guizhou rhombic block and its relationship with endo-mineralization. J. East China Inst. Technol. 27 (4), 301–308.
Wawrzyniec, T. F. (1999). Dextral transcurrent deformation of the eastern margin of the Colorado Plateau (United States of America) and the mechanics of footwall uplift along the Simplon normal fault (Switzerland/Italy). The University of New Mexico.
Wen, D. X., Han, R. S., Wu, P., and He, J. J. (2014). Altered dolomite features and petro-geochemical prospecting indicators in the Huize lead-zinc deposit. Geol. China 41, 235–245.
Wibberley, C. A. J., and Shipton, Z. K. (2010). Fault zones: a complex issue. J. Struct. Geol. 32, 1554–1556. doi:10.1016/j.jsg.2010.10.006
Wintsch, R. P., Christoffersen, R., and Kronenberg, A. K. (1995). Fluid-rock reaction weakening of fault zones. J. Geophys. Res. Solid Earth, 100(B7): 13021–13032. doi:10.1029/94jb02622
Wu, H. Z., Han, R. S., Qiu, W. L., Hu, Y. Z., and Wu, P. (2015). The pore evolution of ore-bearing sandstone and its restriction to mineralization in liuju copper deposit in chuxiong basin, yunnan. Acta Sedimentol. Sin. 33, 512–523.
Wu, J. B., Han, R. S., Zhang, Y., Sun, B. T., Li, W. Y., Li, D. Q., et al. (2024). The fault–fold structure ore control mechanism of hydrothermal deposits——a case study of the Maoping super-large rich-Ge lead–zinc deposit in Northeastern Yunnan, China. Ore Geol. Rev. 168, 106039. doi:10.1016/j.oregeorev.2024.106039
Wu, J. B., Han, R. S., Zhou, G. M., Shi, Z. L., Zhang, Y., Sun, B. T., et al. (2023). Structural ore-controlling and deep prospecting direction of the Maoping Pb–Zn deposit in Northeastern Yunnan, China. Geotect. Metallogenia 47, 984–1001.
Wu, J. B., Pi, Q. H., Zhu, B., Hu, Y. Y., Li, G., and Wei, C. W. (2020). Late Cretaceous-Cenozoic exhumation of Northwestern Guangxi (China) and tectonic implications: evidence from apatite fission track dating. Geochemistry 80, 125662. doi:10.1016/j.chemer.2020.125662
Yan, D. P., Zhou, M. F., Song, H. L., Wang, X. W., and Malpas, J. (2003). Origin and tectonic significance of a mesozoic multi-layer over-thrust system within the Yangtze block (South China). Tectonophysics 361, 239–254. doi:10.1016/s0040-1951(02)00646-7
Zhai, Y. S. (1996). Several issues on the study of tectonic–fluid–metallogenic processes. Earth Sci. Front. 3, 230–236.
Zhang, R. Z. (2017). Structural control mechanism and deep mineralization prediction of the Zhaoping gold deposit belt. Beijing: China University of Geosciences.
Zhang, Y., Han, R. S., Ding, X., Wang, Y. R., and Wei, P. T. (2019). Experimental study on fluid migration mechanism related to Pb–Zn super-enrichment: implications for mineralisation mechanisms of the Pb–Zn deposits in the Sichuan–Yunnan–Guizhou, SW China. Ore Geol. Rev. 114, 103110. doi:10.1016/j.oregeorev.2019.103110
Zhang, Y., Han, R. S., and Wei, P. T. (2016). Research overview on the migration and precipitation mechanisms of lead and zinc in oreforming fluid system for carbonate-hosted lead—zinc deposits. Geol. Rev. 62 (1), 187–201.
Keywords: porosity and permeability, mineralization-alteration zones, metallogenic patterns, prospecting directions, Maoping superlarge germanium-rich lead-zinc deposit, Sichuan-Yunnan-Guizhou lead-zinc polymetallic metallogenic area
Citation: Wu J, Han R, Zhang Y, Wu P, Gong H, Wang L, Cheng G, Li X, Yang Y and Mi Y (2024) Porosity–permeability characteristics and mineralization–alteration zones of the Maoping germanium-rich lead–zinc deposit in SW China. Front. Earth Sci. 12:1347243. doi: 10.3389/feart.2024.1347243
Received: 30 November 2023; Accepted: 24 June 2024;
Published: 30 July 2024.
Edited by:
Ryan Mathur, Juniata College, United StatesReviewed by:
Liang Qiu, China University of Geosciences, ChinaHua-Wen Cao, Chengdu University of Technology, China
Copyright © 2024 Wu, Han, Zhang, Wu, Gong, Wang, Cheng, Li, Yang and Mi. This is an open-access article distributed under the terms of the Creative Commons Attribution License (CC BY). The use, distribution or reproduction in other forums is permitted, provided the original author(s) and the copyright owner(s) are credited and that the original publication in this journal is cited, in accordance with accepted academic practice. No use, distribution or reproduction is permitted which does not comply with these terms.
*Correspondence: Runsheng Han, NTU0NjcwMDQyQHFxLmNvbQ==