- 1College of Geosciences, China University of Petroleum, Beijing, China
- 2State Key Laboratory of Petroleum Resources and Prospecting, China University of Petroleum (Beijing), Beijing, China
In recent years, oil and gas exploration has expanded into ultra-deep fields. Thick primary dolomite is developed in the Qigebulak Formation of the Tarim Basin, but there is little research on the petrological characteristics and reservoir genetic mechanisms of the primary dolomite. The Qigebulak Formation consists of the upper, middle, and lower members, and the dolomite can be divided into crystalline dolomite, grain dolomite, and algal framework dolomite. The analysis of the petrological and geochemical characteristics shows that dolomites in the middle member retain the geochemical characteristics of the original deposition. The δ13C of member Ⅱ ranges from 3.2‰ to 4.6‰ (VPDB), and δ18O range from −1.7‰ to 2.1‰ (VPDB). These values are consistent with the distribution range of carbon and oxygen isotopes in seawater during deposition. The REE distribution patterns of the three members of the Qigebulak Formation are relatively flat and right-inclined, with REE < 100 ppm. The LREE/HREE ratio ranges from 6.34 to 23.83, with an average of 13.82, indicating LREE enrichment and HREE relative loss. Overall, the dolomite in the Qigebulak Formation is formed by seawater deposition. Sedimentation is the foundation and premise of reservoir development. Effective reservoirs are mainly developed in the grain banks and microbial mounds, with the reservoir spaces mainly consisting of algal framework pores and intergranular pores. Supergene karstification plays a key role in the formation of the reservoir, and the upper Qigebulak Formation experienced strong surface dissolution. During the burial stage, high temperature and high pressure caused the source rocks of the Yuertusi Formation to dissolve and form organic acidic fluids. The dissolution of these acidic fluids promoted the improvement of the reservoir’s physical properties. Based on a comprehensive analysis of the controlling factors for reservoir development, a reservoir genetic model is established. The primary pores are developed in early sedimentary microbial mounds and grain banks, serving as the foundation for reservoir development. During the Late Sinian period, influenced by the Keping movement, the Tarim Basin experienced tectonic uplift, leading to extensive supergene karst development under the influence of meteoric water. In the burial phase, the degradation of organic matter produced acidic fluids that dissolved dolomite, thereby further enhancing porosity. The dolomite reservoir of the Qigebulak Formation developed under the control of sedimentation, supergene dissolution, and burial dissolution. At present, industrial oil and gas have been discovered in the Sinian system in the Tabei Region, but the overall exploration level is low. The well-developed paleokarst reservoirs suggest that the Sinian system holds promise for oil and gas exploration.
1 Introduction
A large number of explorations and studies have confirmed the significance of dolomite in oil and gas exploration (Asmerom et al., 1991; Bao et al., 1998; Bao, 1999; Arvidson, 1999; Qian et al., 2012; Du et al., 2018). The oil and gas resources in carbonate rocks account for nearly 50% of the world’s reserves, and its output is more than 60% (Shao et al., 2010). Half of the reservoirs are dolomite (Xiong et al., 2021). At present, the global dolomitic oil and gas field reservoirs are mainly Mesozoic Cretaceous and Paleozoic Permian. The oil and gas reserves in the late Ordovician and Neoproterozoic Formations account for 6.72% of the accumulative oil and gas equivalent of dolomite fields in the world (Gu, 2000; Lin, 2002; Feng et al., 2007; Qi and Yun, 2010), which indicates that deep dolomitic oil and gas fields also have exploration potential to some extent (Ma, et al., 2011; Jiao, et al., 2011; Zhang et al., 2013).
The Tarim Basin, with abundant oil and gas resources, is one of three major petroliferous basins in western China. The Tahe Oilfield in the Tarim Basin, the first Paleozoic marine carbonate oilfield discovered in China, is dominated by epigenetic karst (Ma et al., 2009; Zhang et al 2014; Qi, 2014; Guo et al., 2016). Nevertheless, the oil and gas exploration of the Sinian system in the Tarim Basin has not made substantial progress. This is partially because oil and gas exploration of the Sinian system in the Tarim Basin is limited by the burial depth. On the other hand, the lack of understanding of the reservoir of the Sinian Qigebulak Formation is also one of the constraints. In studies of the Qigebulak Formation reservoir in the Tarim Basin, the proposal of the “microbial dolomitization” model in recent years (Chen et al., 2017) has prompted researchers to elaborate on the research progress of the reservoir from the perspective of microbial dolomite (Li et al., 2015; Qian et al., 2017a). Some scholars believe that the paleokarst at the top of the Sinian system is the main contributor to the reservoir of the Qigebulak Formation (He et al., 2010a; Klimchouk et al., 2016; Chen et al., 2020). The relatively limited reservoir studies on the Sinian Qigebulak Formation in the Tarim Basin and the unclear reservoir control factors are among the important constraints of oil and gas exploration.
Based on outcrop profiles and core data, this paper systematically classifies the rock types of dolomite reservoirs in the Qigebulak Formation, Tarim Basin. It also categorizes and describes the reservoir space, elucidates the physical characteristics of different types of dolomite reservoirs, comprehensively summarizes geochemical characteristics, analyzes reservoir genesis, and presents a model for reservoir development through thin-section observation, C–O isotope analysis, and rare earth element (REE) analysis. This study aims to provide valuable insights for ultra-deep oil and gas exploration in the Tarim Basin.
2 Geological setting
The Tarim Basin, located in the south of the Xinjiang Uygur Autonomous Region, is the largest inland basin in China. From the Nanhua period to the Sinian period, it experienced rifting and depression, respectively. During the Nanhua period, the breakup of the Rodinia supercontinent led to the Tarim Basin entering a stage of extensional rift evolution, during which the pre-Sinian crystalline basement began to receive Neoproterozoic deposits (Jin, 2006; Zhang et al., 2013). As the activity of mantle plumes, which drive plate splitting, tends to decline, the tension gradually weakens. The vast area to the north of the ancient uplift is successively a carbonate gentle slope, a carbonate platform edge, an inter-platform depression, and basin facies that developed from south to north (Figure 1b). Three depressions have developed in the northwest, southwest, and northeast margins of the Tarim Basin (Figure 1a), and the basin presents a paleotectonic pattern of being high in the south and low in the north (He et al., 2010a; Zheng et al., 2021). Due to the Kalpin movement at the end of the Late Sinian, the Tarim Plate experienced a regional regression and was uplifted to form an unconformity (Chang, et al., 2011; He et al., 2018). As a result, the Upper Sinian carbonate strata became exposed to the surface and underwent a long-term period of weathering, leaching, and denudation, which provided favorable conditions for the development of epigenetic karst (He et al., 2010a; Turner, 2010; Shi et al., 2016; Ahmad et al., 2022). Subsequently, the Lower Cambrian Yuertus Formation, consisting of cherts, siliceous dolomite, shale, and phosphorite at its bottom, directly covered the Upper Sinian Qigebulak Formation (Yu et al., 2009; He et al., 2018). Outcrop, drilling, and seismic data from the Tarim Basin show the extensive distribution of the unconformity between the Sinian and Cambrian systems (Huang, 2014; Shi et al., 2016). This unconformity is also discovered and reported in other regions worldwide (Saylor et al., 1995; Christie–Blick et al., 1995; Praekelt et al., 2008; Wang et al., 2010a; Peters and Gaines, 2012), indicating that the tectonic event at the end of the Late Sinian is global.
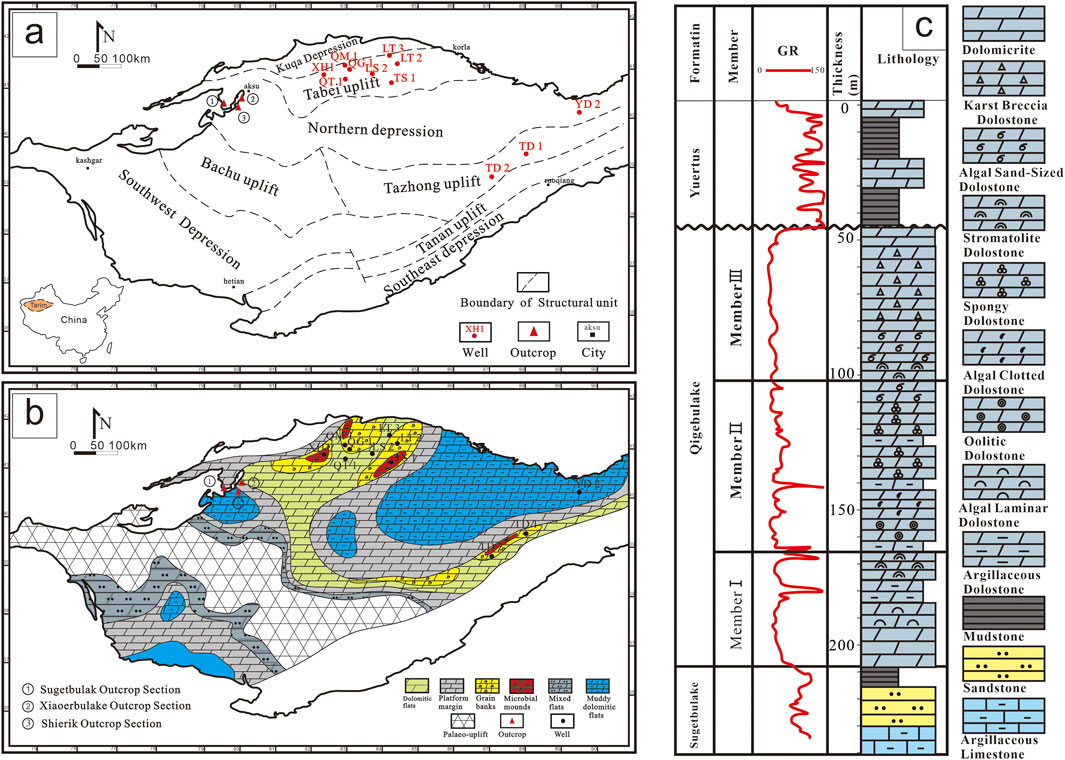
Figure 1. Geological map of the Tarim Basin. (a) Tectonic division map of the Tarim Basin. (b) Lithofacies paleogeography map of upper Sinian in the Tarim Basin. (c) Simplified stratigraphic histogram of the Qigebulak Formation.
The Upper Sinian Qigebulak Formation developed a set of light gray, thick-bedded dolomite (Zhu et al., 2008; Warren, 2000; Bao et al., 2019), mainly micritic in structure, and algal dolomite is well-developed (Figure 1c). The Qigebulak Formation can be divided into member Ⅰ, member Ⅱ, and member Ⅲ. Member Ⅰ is micritic dolomite and microbial dolomite, and the microbial content increases upward. Member Ⅱ is dominated by grain dolomite and algal framework dolomite. Algal dolomite is developed in the lower part of member Ⅲ, and member Ⅲ is affected by surface weathering, and surface dissolution forms a set of slump karst breccia (Figure 1c).
3 Samples and methods
Through observation, description, and sampling of the Qigebulak Formation of Upper Sinian in the Tarim Basin, 185 samples were collected from the outcrop, and 144 thin sections were prepared, of which 20 thin sections were stained with alizarin red to distinguish dolomite and calcite under a microscope. Six coring wells in the Qigebulak Formation were observed in the core library of Sinopec Northwest Bureau, with a total length of 263.5 m, and 75 thin sections were prepared.
Carbon and oxygen isotope analyses were performed on bulk carbonate that was powdered by grinding to <200 mesh to avoid calcite filled in vugs, pores, or cracks and other authigenic minerals and eliminate diagenetic influences on the isotopic composition. A total of 22 outcrop samples were selected for carbon and oxygen isotope analysis. Approximately 1 mg of the sample was reacted with 100% H3PO4 at 75°C for 16 h in vacuum to generate and trap CO2 gas at a temperature of 110°C. The carrier gas used was 99.999% helium. Carbon and oxygen isotopes were then measured on an Elementar IsoFLOW-Precision stable isotope ratio mass spectrometer (No. A2018A01292, made in Germany) at the State Key Laboratory of Petroleum Resource and Prospecting at the China University of Petroleum (Beijing). The analytical precision of the isotopic analysis was at least ±0.5‰. The carbon and oxygen isotope values were standardized to the Vienna Pee Dee Belemnite (VPDB) standard and herein recorded using conventional δ13C and δ18O notations, respectively (Allan and Wiggins, 1993).
A total of 34 dolomite samples were selected for the analysis of REEs. A measure of 50 mg of sample powder was digested with 0.5 mL HNO3 and then dried again; this process was repeated again. Then, 5 mL HNO3 (1.42 g/mL) was added to the dried sample and heated at 130°C for 3 h. Finally, the solution was diluted with ultrapure H2O to a volume of 50 mL for trace element analysis. The obtained solutions were analyzed via “NexION 300D” inductively coupled plasma mass spectrometry (ICP–MS). The relative uncertainties of these measurements are better than 4% using DLS 88a4 and CCH–1–4 as standards. The post-Archean Australian shale (PAAS) data in the normalized calculation of REE were obtained from McLennan (1989). Ce anomaly values were calculated as follows: Ce/Ce* = Cen/(Prn2/Ndn) (Lawrence et al., 2006).
4 Results
4.1 Petrographic characteristics
According to the observation of the Xiaoerbulak Xigou and Sugetbulak outcrop profiles in the northwestern area of the Tarim Basin, the Sugetbulak Formation lies under the Qigebulak Formation, and at the top of the Sugetbulak Formation is a set of red siltstones (Figure 2g). With the continuous rise of the sea level, primary dolomite began to be deposited in the early Qigebulak Formation, but some clastic components were still mixed into it. The outcrop shows that a large set of thick primary dolomite (Figure 2d) has developed from the top to the bottom in the Qigebulak Formation, approximately 200 m thick, and thick massive micritic dolomite (Figure 2e), lamellar dolomite (Figure 2f), algal lamellar dolomite (Figure 2h), and stromatolite dolomite (Figure 2c) can be seen among them. The dolomite is extremely thick and widely distributed as a whole. This large-scale and relatively uniform dolomite is most likely to be the origin of primary precipitation. The top of the Qigebulak Formation is in unconformity contact with the Yuertus Formation. The Yuertus Formation is a set of black mudstone and argillaceous dolomite with a thickness of approximately 10 m, which is a set of high-quality source rocks (Figure 2a). The Late Sinian Kalpin Movement uplifted the Tarim Basin on a large scale; the top of the Qigebulak Formation was denuded to form a weathering crust (Figure 2i), and karst breccias can be seen in the lower part of the unconformity (Figure 2b; Figure 2i).
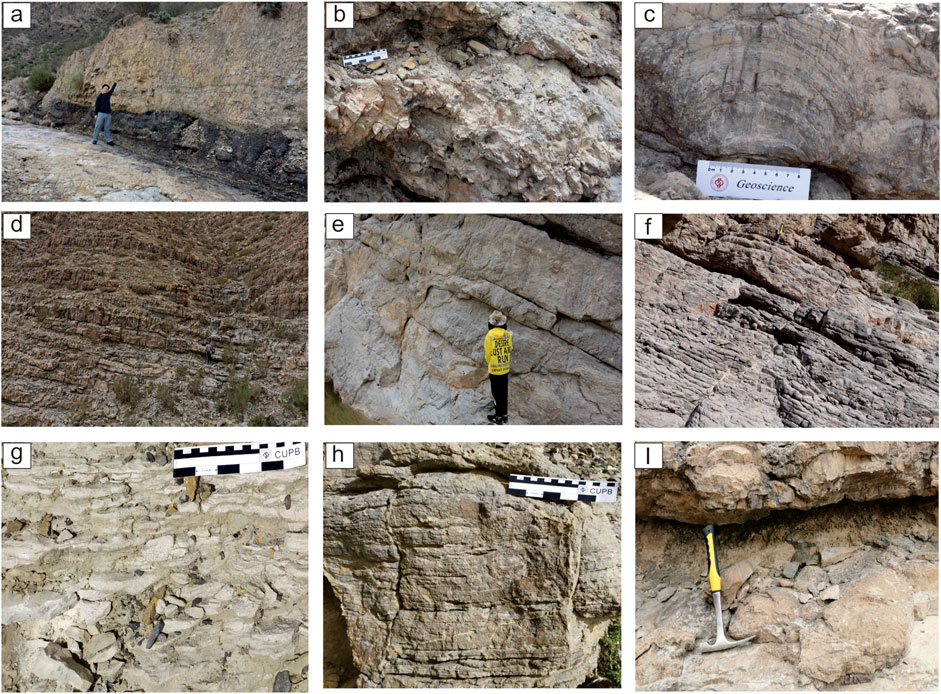
Figure 2. Outcrop characteristics of the Qigebulak Formation in the Tarim Basin. (a). Unconformity between Sinian and Cambrian on the Sugetbulak section, where the lower part is the Sinian Qigebulak Formation and the upper part is the Cambrian Yuertus Formation. (b). Karst breccia dolomite at the top of the Qigebulak Formation, Sugetbulak section. (c). Stromatolite dolomite of the Qigebulak Formation, Xiaoerbulak Xigou section. (d). Macroscopic characteristics of a large set of thick primary dolomite in the Qigebulak Formation, Sugetbulak section. (e). Thick-layered massive micrite dolomite of the Qigebulak Formation, Xiaoerbulak Xigou section. (f). Thick-bedded algal laminar dolomite in the Qigebulak Formation, Xiaoerbulak Xigou section. (g). Red siltstone at the top of the Sugetbulak Formation, Sugetbulak section. (h). Laminated stromatolite dolomite of the Qigebulak Formation, Sugetbulak section. (i). Weathering crust at the top of the Qigebulak Formation showing the remnant yellow mudstone from weathering, Xiaoerbulak Xigou section.
4.1.1 Crystalline dolomite
The Qigebulak Formation mainly develops micrite dolomite (Figure 3a) and silt-sized crystalline dolomite (Figure 3b), with less fine–crystalline dolomite (Figure 3c) and medium–coarse crystalline dolomite (Figure 3d). It is generally light gray to gray–white, with a blocky feature of medium thickness.
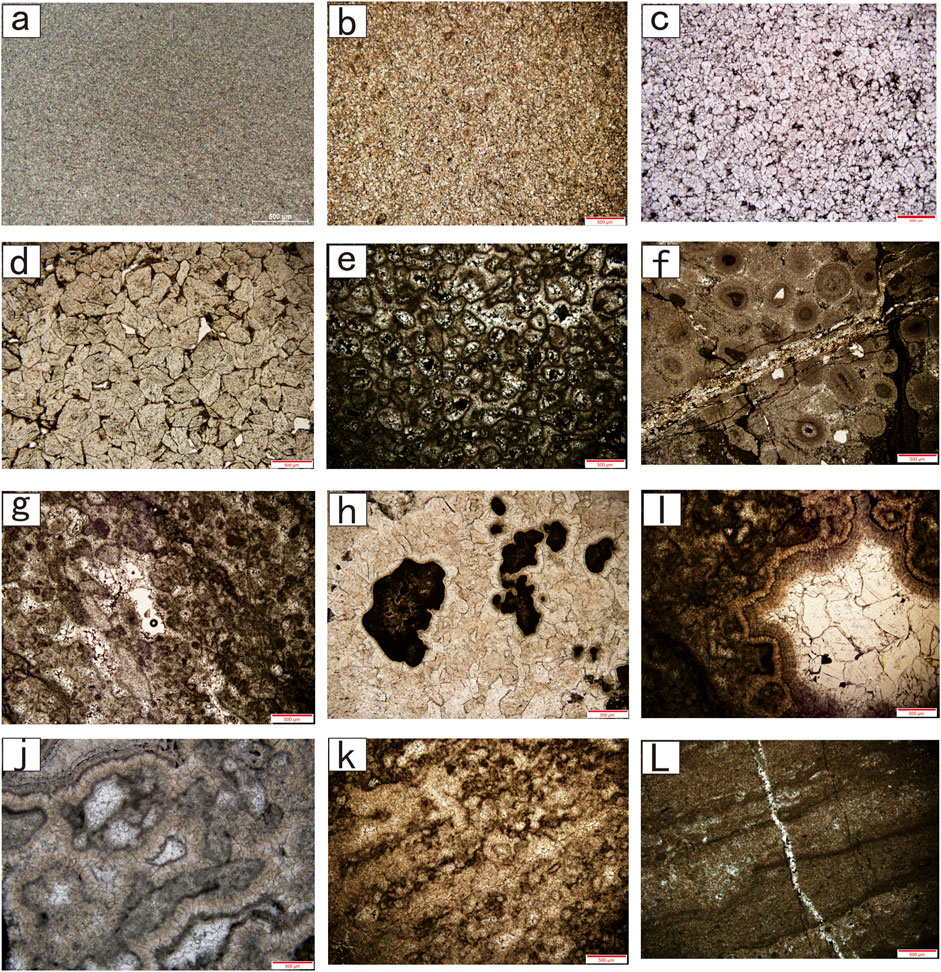
Figure 3. Petrological characteristics of the upper Sinian Qigebulak Formation in the Xiaoerbulak Xigou section. (a). Dense micrite dolomite, single polarization. (b). Silt-sized crystalline dolomite, single polarized light. (c). Fine crystalline dolomite, filled with organic matter in the intercrystalline pores, single polarized light. (d). Medium crystalline dolomite, with developed intercrystalline pores, visible black organic matter filling, single polarized light. (e). Algal pellet dolomite with developed intragranular cavity pores, single polarized light. (f). Oolitic dolomite, where later fractures are filled with calcite, black organic matter can be observed in intergranular pores, single polarized light. (g). Algal sand-sized dolomite, where the sand debris is mainly residual algal spherules and algal clots, showing intergranular dissolved pores, single polarized light. (h). Algal clotted dolomite, single polarized light. (i). Spongy dolomite, where lace cavity holes are first cemented by comb-shaped dolomite and then cemented with bright crystal calcite, single polarized light (j). Spongy dolomite, where lace cavity developed, filled with calcite, single polarized light. (k). Algal laminar dolomite, micrite structure, single polarized light. (l). Stromatolite dolomite, where the bright layer and dark layer are clearly distinguished, single polarized light.
4.1.2 Grain dolomite
The grains mainly consist of algal debris (Figure 3g), oolites (Figure 3f), and algal spherules (Figure 3e). Grain dolomite is mainly developed in members Ⅱ and Ⅲ of the Qigebulak Formation, and cement consists of micrite and micrite-powder crystal structure, indicating that the dolomite is mainly of primary sedimentary origin.
4.1.3 Algal framework dolomite
The algal framework dolomite is developed in the upper, middle, and lower members of the Qigebulak Formation. The algal framework dolomite mainly includes stromatolite dolomite (Figures 2c, 3l), algal laminar dolomite (Figures 2f, 3k), and spongy dolomite (Figures 3i,j). Laminated, wavy, and columnar stromatolites can be observed. There are obvious biogenic structures in the algal framework dolomite, but the dolomite is still dominated by micrite and micrite-powder crystal structures, indicating that it originated from the primary sedimentary environment.
4.2 Geochemical characteristics
4.2.1 Carbon and oxygen isotope
Previous studies (Coplen, et al., 1983; Jacobsen and Kaufman, 1999; Allan and Matthews, 1977; He et al., 2007; Derry, 2010; Ren et al., 2016; Bilal et al., 2022; Rahim et al., 2022) summarized the distribution of carbon and oxygen isotopes in marine carbonate sediments; the δ13C mean value ranges from −5‰ to 5‰ (VPDB), and the δ18O mean value ranges from −5‰ to 2‰ (VPDB). Analysis of the Qigebulak group data shows that the δ13C mean value ranges from 1.0‰ to 8.4‰ (VPDB), the δ18O mean value ranges from −7.5‰ to −2.1‰ (VPDB), except for five data samples from the top and two from the bottom. All other data conform to the distribution pattern of carbon and oxygen isotopes in marine carbonate sediments summarized by Jacobsen, so it can be concluded that the dolomite in the study area is of marine carbonate origin. δ13C of member Ⅲ ranges from 1.0‰ to 3.8‰ (VPDB), δ18O ranges from −7.5‰ to −1.1‰ (VPDB), δ13C of member Ⅱ ranges from 3.2‰ to 4.6‰ (VPDB), δ18O ranges from −1.7‰ to 2.1‰ (VPDB), δ13C of member Ⅰ ranges from 2.2‰ to 8.4‰ (VPDB), and δ18O of member Ⅰ ranges from −7.0‰ to 0.7‰ (VPDB) (Table 1). There are seven data anomalies at the top and bottom of the Qigebulak Formation, which are caused by the influence of atmospheric fresh water and clastic materials (Figure 6). The analysis of unaffected data revealed no obvious distinction between different types of dolomite (Figure 4), indicating that the diagenetic fluid is the same and the origin of dolomite is consistent.
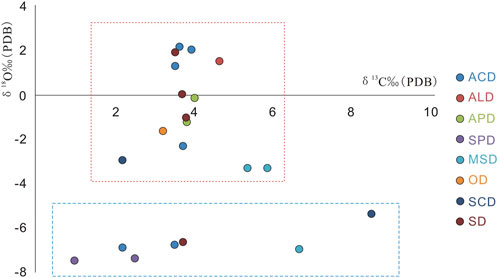
Figure 4. Plot of δ13C versus δ18O for the Qigebulak Formation dolomites. ACD, algal clotted dolomite; ALD, algal laminar dolomite; APD, algal pellet dolomite; SPD, spongy dolomite; MSD, mud-sized crystalline dolomite; OD, oolitic dolomite; SCD, silt-sized crystalline dolomite; SD, stromatolite dolomite.
4.2.2 Rare earth elements
Minerals formed in different diagenetic fluids usually retain REE patterns from their parent fluids (Webb and Kamber, 2000; Bau and Dulski, 1996; Hu et al., 2010). The REE pattern of dolomite is almost unaffected during diagenesis (Banner et al., 1988; Banner, 1995), but it will be affected by atmospheric precipitation and terrigenous debris (Qing and Mountjoy, 1994b; Frimmel, 2009; Wang et al., 2021).
Table 1 provides a summary of REE concentration of Qigebulak Formation dolomite. Figure 5 shows the REE distribution patterns of different members and lithologies. The REE distribution patterns of the three members of the Qigebulak Formation are relatively flat and right-inclined (Figures 5a–c), with REE < 100 ppm. The LREE/HREE ratio ranges from 6.34 to 23.83, with an average of 13.82, indicating LREE enrichment and HREE relative loss (Table 1). Overall, the dolomite of the Qigebulak Formation is formed by seawater deposition (marine carbonates are usually ƩREE < 100 ppm, LREE preferentially enter carbonate) (Qing and Mountjoy, 1994b; Liu et al., 2008; Liu et al., 2017). However, the contents of REE in members Ⅲ and Ⅰ are higher. Member Ⅲ ƩREE is 0.71–43.57 ppm, and member Ⅰ ƩREE is 5.95–116.05 ppm (xq-10–3 is siltstone, ƩREE is 116.05 ppm), which is higher than member Ⅱ ƩREE (0.60–1.99 ppm).
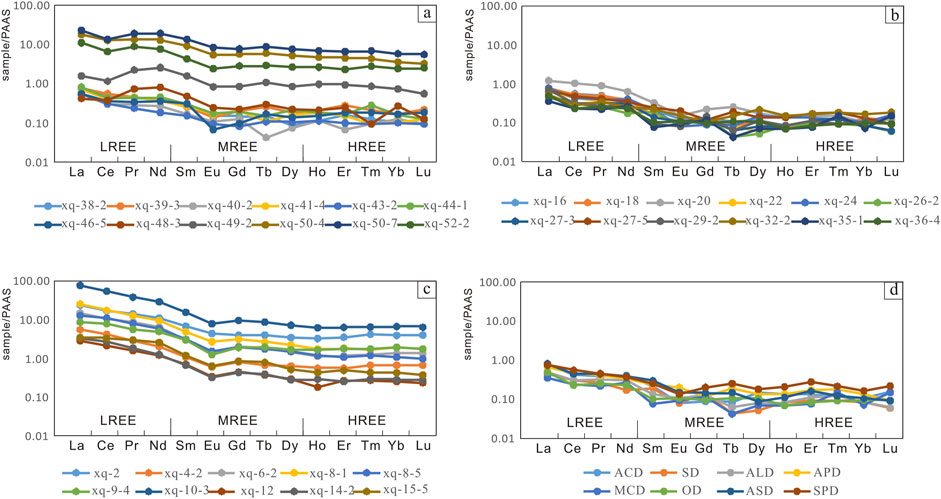
Figure 5. Distribution patterns of rare earth elements in different members and lithologies of the Qigebulak Formation. (a) Distribution patterns of rare earth elements in member Ⅲ. (b) Distribution patterns of rare earth elements in member Ⅱ. (c) Distribution patterns of rare earth elements in member I. (d) Distribution patterns of rare earth elements of different lithologies. ACD, algal clotted dolomite; ALD, algal laminar dolomite; APD, algal pellet dolomite; SPD, spongy dolomite; MSD, mud-sized crystalline dolomite; OD, oolitic dolomite; SCD, silt-sized crystalline dolomite; SD, stromatolite dolomite.
Ce anomaly is a sensitive reaction of REDOX; unlike other REE elements, Ce records REDOX changes in a fluid environment, and it is generally believed that positive Ce anomaly in a carbonate rare earth distribution model indicates seawater deposition characteristics (Nothdurft et al., 2004; Wang et al., 2014; Liang et al., 2020; Xiang et al., 2020), while negative Ce anomaly reflects a peroxidation environment (German and Elderfield, 1990; Bau and Dulski, 1996; Frimmel, 2009). The Qigebulak Formation is positive Ce anomaly as a whole, but five samples at the top show negative Ce anomalies, ranging from 0.574 to 0.987 (Table 1), indicating that the Late Sinian was a marine sedimentary environment and the Late Sinian was an oxidation environment, which is related to the tectonic uplift of the Tarim Basin caused by the Keping Movement, and the meteoric precipitation was oxygen-rich fluid. At the same time, the higher ƩREE in the upper member is due to the pollution caused by the formation of certain clastic minerals in the surface water, while the higher ƩREE in the lower member is a positive Ce anomaly, and the higher ƩREE is due to the high content of clastic components in the sedimentary environment of mixed tidal flat. By comparison, rare earth elements in the upper and lower segments of the Qigebulak Formation are affected, while the middle segment is not. By analyzing the distribution patterns of rare earth elements in the middle segment, it can be concluded that the distribution patterns of different types of dolomite are basically the same, indicating that the dolomite is caused by seawater precipitation (Figure 5d).
4.3 Sedimentary characteristics
Due to the influence of continental breakup, the Tarim Basin began to split during the Nanhua Period, forming a Mangar rift in the basin; by the Early Sinian, the basin transformed from a rift to a depression (Qian et al., 2017a). A suite of clastic and argillaceous carbonate rocks deposited in the Sugetbulak Formation, Qigebulak Formation of Upper Sinian, is a carbonate platform deposit of gentle slope type, and the sedimentary facies mainly include open platform, platform edge, and restricted platform; the sedimentary sub-facies can be further divided into microbial mound, grain bank, dolomitic flat, and mixed dolomitic flat. The microbial mounds mainly develop on the windward side of the platform margin, with strong hydrodynamic force, and the lithology is mainly composed of stromatolite dolomite, algal pellet dolomite, and spongy dolomite. The grain banks can be divided into intra-platform banks and marginal-platform banks, and the lithology is mainly oolitic dolomite, algal clotted dolomite, and algal sand-sized dolomite. The dolomitic flat is mainly developed in restricted and open platforms, and the lithology is mainly mud-sized crystalline dolomite, algal lamina dolomite, and algal clotted dolomite. The mixed dolomitic flat is mainly developed in the intra-platform slope zone, and the lithology is mainly siltstone and mud-sized to silt-sized crystalline dolomite.
Combined with the analysis of the comprehensive histogram of the Qigebulak Formation, mixed dolomite flats are mainly developed in the early stage of sedimentation, and as sea levels rise, algal dolomite increases. In member Ⅱ, grain dolomite and algal framework dolomite are mainly developed. Grain banks and microbial mounds are developed in lower member Ⅲ. With the decrease in the sea level, dolomite flats developed in the upper part of member Ⅲ. Slump karst breccia is formed under the action of surface karst.
4.4 Reservoir space and physical properties
Combined with field outcrop and thin section analysis, the dolomite reservoir space of the Qigebulak Formation can be divided into three categories, namely, karst cave type, porous type, and fractured type (Table 2). The karst cave-type reservoir space mainly develops in the upper Qigebulak Formation. The Keping movement lifted the Tarim Basin in the Late Sinian. Atmospheric precipitation penetrates downward along the formation fractures, and the collapse karst breccias are formed under the action of surface water and tectonic movement. Dissolution features are well-developed, and the diameter of karst caves can reach 5–10 cm (Figure 7c). Meanwhile, the process of interlayer water horizontal migration will also cause the dissolution of dolomite and form bedding karst caves, which are distributed in strips, and the diameter of karst caves can reach 10–20 cm (Figure 7a). The pore-type reservoir space can be divided into intercrystalline pores, intergranular pores, moldic pores, and algal framework cavities. Intercrystalline pores are mainly developed in silt-sized and fine crystalline dolostone (Figure 7b). After the effect of interlayer water and high temperature in a buried environment, the dolomite will be dissolved and recrystallized, and the crystal particles will become larger to form silt-sized to fine crystalline dolomite or even medium to coarse crystalline dolostone so as to form intercrystalline pores. Intergranular pores mainly exist between the particles of oolitic dolomite (Figure 7h), algal chlorite dolomite, and algal arenaceous dolomite (Figure 7f). Intergranular pores are preserved by the original sedimentary structure. Moldic pores are formed after the loss of residual particles, which can be oolitic, algal sand debris, bioclast, and terrigenous detrital (Figure 7d). Algal framework cavities are mainly cavity pores of algal chlorite dolomite, algal agglomerate dolomite, and foam sponge dolomite, and the cavity will still retain some residual pores after multistage cementation (Figure 7g). The fractured-type reservoir space mainly includes structural fractures and stylolite (Figure 7e). Structural fractures are formed with tectonic movement, and stylolite is formed by pressure dissolution in a buried environment.
Based on the analysis of six wells and the 263.5 m core in the Qigebulak Formation of the Tarim Basin, the statistics of different lithologies indicate that spongy dolomite, stromatolite dolomite, algal clotted dolomite, and oolitic dolomite reservoirs have relatively good physical properties, and intergranular pores and microbial framework pores were relatively developed. According to the classification analysis of different microfacies (Figure 8a), the average porosity of microbial mounds and grain banks is the highest; the highest porosity of microbial mounds is 3.8%, and that of grain banks is 3.2% (Figure 8b). Dolomite and mixed flats are characterized by dense lithology, which mainly develops mud-sized crystalline dolomite and algal laminar dolomite, and the compaction in the burial stage further reduces its physical properties.
5 Discussions
5.1 Controlling factors of reservoir development
5.1.1 Control of sedimentation relative to the reservoir
The transgression in the early Late Sinian led to the development of a gentle-slope carbonate platform in the Tarim Basin (Shi et al., 2016; He et al., 2017; Bao, et al., 2019), forming a set of widely distributed thick-layered micrite dolomite (Hood et al., 2011). Member Ⅰ of the Qigebulak Formation is dominated by crystalline dolomite. Member Ⅲ mainly develops slump karst breccia dolomite, grain dolomite, and algae framework dolomite, and member Ⅱ mainly develops algae framework dolomite and grain dolomite (Figure 6). From the bottom to the top, grain dolomite and algal-framework dolomite increased significantly. The analysis results of carbon and oxygen isotopes and rare earth elements show that member Ⅱ of the Qigebulak Formation is not affected by atmospheric precipitation and detrital materials. There is no clear distinction between the carbon and oxygen isotopes of different rock types. The content of rare earth elements is low, ƩREE is 0.763–1.088 ppm, and the proportion of LREE is relatively high, reflecting the characteristics of the seawater deposition, and the distribution patterns of rare earth elements in different rock types are basically the same. Member Ⅲ is affected by the weathering crust, and the oxygen-rich fluid on the surface causes δ18O of the dolomite to be low and the content of rare earth elements to increase. It is concluded that a set of primary dolomites of seawater sedimentary origin developed in the Qigebulak Formation. Sedimentation controls the rock type, rock texture, and primary pore type and is the foundation of reservoir development. Different sedimentary facies form different pore types. Micritic dolomite is mainly developed in dolomite flats, and the pores are basically undeveloped; the primary pores are mainly retained in microbial mounds and grain banks. The main lithology is spongy dolomite, stromatolite dolomite, oolitic dolomite, algal sand-sized dolomite, and algal pellet dolomite, and the pore structure is mainly algal framework and intergranular pores. Microbial mounds and grain banks of the Qigebulak Formation are mainly developed in the Tabei uplift area, which is located on the windward side of the platform edge. In conclusion, sedimentary facies control primary dolomite rock types and primary reservoir space types.
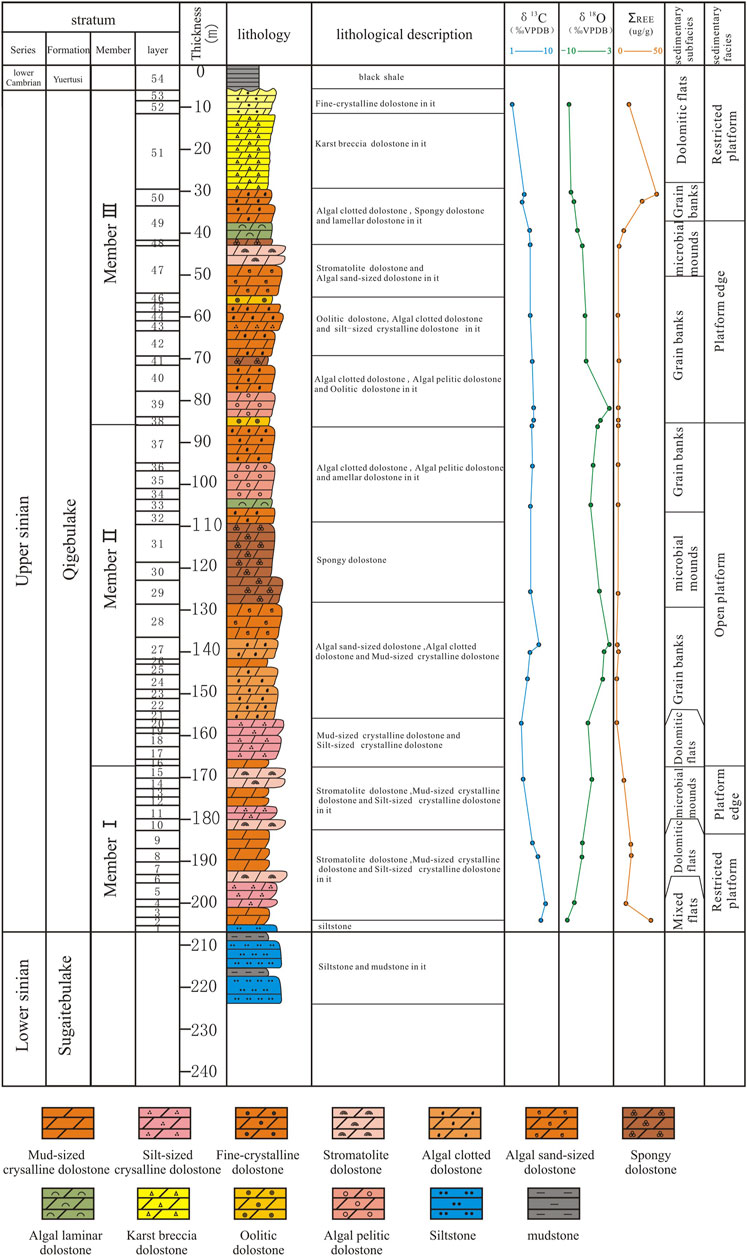
Figure 6. Comprehensive histogram of the Qigebulak Formation of upper Sinian in the Xiaoerbulak section.
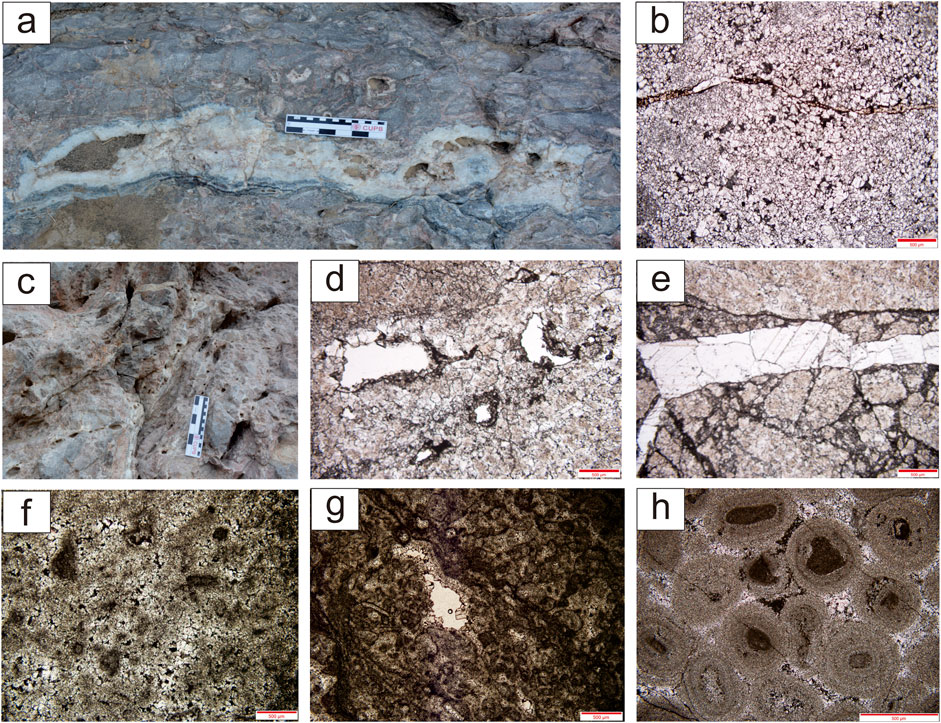
Figure 7. Reservoir space types of the Qigebulak Formation in the Xiaoerbulak section, Keping area, Tarim Basin. (a) Algal clotted dolomite, with layer-parallel cave, 15 cm long, field photograph. (b) Fine crystalline dolomite, with intercrystalline pores, black organic matter filling, plane polarized light. (c) Slump karst breccia, with developed karst breccia cave, cave up to 20 cm, field photograph. (d) Algal sand-sized dolomite, with intergranular dissolution pores, black organic matter filling, plane polarized light. (e) Micritic dolomite, microfracture development, black organic matter filling, plane polarized light. (f) Algae clotted dolomite, with intergranular dissolution pores, organic matter filling, plane polarized light. (g) Spongy dolomite, with lace-like framework pore development, plane polarized light. (h) Oolitic dolomite, with intergranular pores, black organic matter filling, plane polarized light.
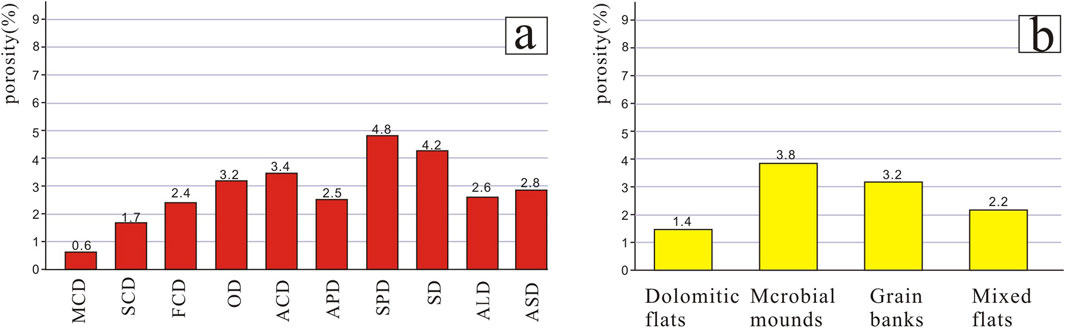
Figure 8. Porosity distribution maps of different dolomites and sedimentary facies. (a) Porosity distribution of different lithologies; (b) Porosity distribution of different microfacies.
5.1.2 Controsurface karst relative to the reservoir
The weathering crust is of great significance to the development of carbonate reservoirs. Since weathering can create a large amount of pore space and greatly improve the quality of the reservoir (Bao et al., 2006; Kerans, 1988; Fritz et al., 1993; Kang, 2008; Peters and Gaines, 2012; Chen et al., 2020), the pore space of most carbonate reservoirs in the world is of karst origin (Praekelt et al., 2008; Hill, 1990). The Keping movement in the Late Sinian caused the uplift of the basin structure, and local areas were exposed to air. Atmospheric precipitation penetrated down along faults, fractures, and high-permeability strata and dissolved dolomite to form pores and caves. With the increase in depth, the saturation of atmospheric precipitation increases, and the solubility of karst water gradually decreases. Epikarst is controlled by unconformities. Epigenetic karstification developed in member Ⅲ of the Qigebulak Formation, weathering crust can be observed in the field outcrops, and a set of slump karst breccia dolomite developed in the lower part of the unconformity surface, which is the direct evidence that the Late Sinian Qigebulak Formation underwent epigenetic karstification. Macroscopically, surface karst can form a large number of dissolution pores with different lengths. The downward penetration of karst water will also lead to intergranular dissolution in the deep strata. The depth of karstification can be calculated by geochemical characteristics. Atmospheric precipitation is oxygen-rich fluid, mainly 16O. The dissolution of atmospheric water into the formation will cause a decrease in δ18O, and the surface minerals carried by the atmospheric water will increase the content of rare earth elements in this formation. The average δ18O value of seawater sedimentary carbonate rocks ranges from −5‰ to 2‰ (VPDB) (Jacobsen and Kaufman, 1999). There are five samples at the top of the upper Qigebulak Formation, whose δ18O is lower than −5‰ (VPDB) and δ18O is −7.5‰ to −6.7‰ (VPDB), which is significantly higher than the REE content in the middle section. At the same time, the negative Ce anomaly indicates that the fluid is an oxidizing environment. Based on the above findings, it is evident that these five samples have experienced obvious epigenetic karstification, and karstification affects the sample xq-48-3, ith the depth reaching approximately 40 m below the unconformity surface.
5.1.3 Transformation of the reservoir by dissolution of organic acid
Organic acid dissolution is another important factor in the formation of dolomite reservoirs. The dissolution in the burial stage will create secondary pore spaces and promote the formation of the reservoirs (Spooner, 1976; Hill, 1990; Mazzullo and Harris, 1992; Seewald, 2003; Davies and Smith, 2006; Zhu et al., 2008). The organic acid fluid contains substances such as H2S, CO2, and SO2, which will cause the dissolution of the rock during the downward flow along the formation fracture, forming secondary pores. There are two ways for the formation of organic acids in the Qigebulak group. First, the Lower Cambrian Yuertus Formation is a set of organic-rich source rocks. In the burial environment, high temperature and high pressure conditions formed organic acid fluids, which entered the Qigebulak Formation along the surface fractures to cause dissolution. Second, the microbial dolomite of the Qigebulak Formation is very developed. The spongy dolomite and the algal pellet dolomite itself have a high content of organic matter. With the increase in burial depth and temperature, organic acid substances will also be produced, which will affect the framework pores. The storage space generated by organic acid dissolution is often filled with asphalt.
5.2 Reservoir development model
The development of dolomite reservoirs in the Upper Sinian Qigebulak Formation in the Tarim Basin is mainly controlled by the dominant reservoir facies, epigenetic karstification, and organic acid dissolution. The Qigebulak Formation is mainly composed of primary dolomite deposits (Zhao et al., 1977; Bao et al., 2019), with microbial mounds and grain banks as the dominant reservoir facies belt, and the primary pores developed in the early depositional period (Figure 9a). Affected by the Keping movement, the structure was uplifted in the Late Sinian, and the Tabei and Tazhong uplift areas were denuded to form a weathering crust (Figure 9b). After the leaching of surface water and atmospheric water, slump karst breccia formed in the lower part of the unconformity, dissolved pores developed, and the physical properties of primary dolomite were improved. During the burial stage, after the compaction of the Qigebulak Formation, the primary pores almost disappeared, and only the framework pores and intergranular pores of the biomound and grain shoal facies remained. The organic acid dissolution under the high-temperature and high-pressure environment further improved the physical properties of the reservoir (Figure 9c).
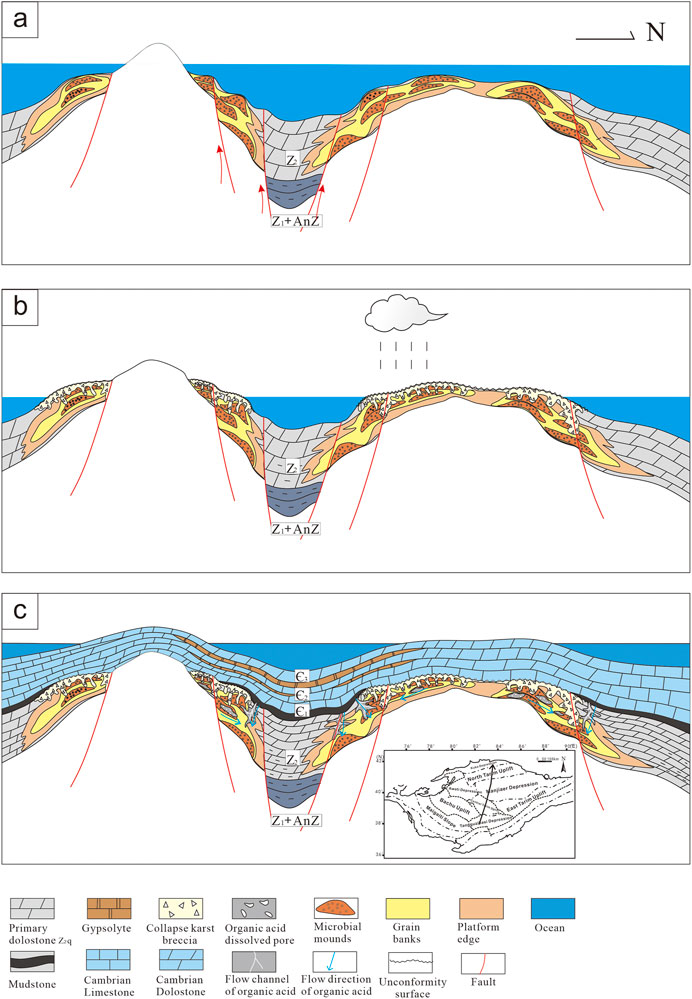
Figure 9. Reservoir development model of the Qigebulak Formation in the Tarim Basin. (a) Early Sinian tectonic-sedimentary model; (b) Late Sinian tectonic-sedimentary model; (c) Cambrian tectonic-sedimentary model.
6 Conclusion
The Qigebulak Formation in the Tarim Basin developed large sets of thick primary dolomites, which can be divided into three categories, namely, crystalline dolomite, grain dolomite, and algal framework dolomite. The grain size of different types of dolomite exhibits a micritic structure, and the analysis of geochemical characteristics shows that the genesis of different dolomites is consistent.
The sedimentary sub-facies of the Qigebulak Formation are biohillock, grain beach, cloud flat, and mixed cumulus flat. Biodomes and grain beach facies are the dominant reservoir facies. The dominant reservoir rocks are foam dolomite, stromatolite dolomite, oolitic dolomite, algal sand-sized dolomite, and algal pellet dolomite. The pore structures are algal framework pores and intergranular pores.
The development of the dolomite reservoir in the Qigebulak Formation is mainly controlled by dominant reservoir facies, surface karst, and organic acid dissolution. Sedimentary facies control the primary dolomite types and primary reservoir space types. In the Late Sinian, tectonic uplift caused supergene karstification to take place at the top of the Qigebulak Formation, and the karstification influence could reach 40 m below the unconformity. The dissolution of organic acids in the burial stage further improves the physical properties of the dolomite reservoir.
Data availability statement
The original contributions presented in the study are included in the article/Supplementary Material; further inquiries can be directed to the corresponding author.
Author contributions
ZL: writing – original draft. ZB: funding acquisition, project administration, supervision, and writing – review and editing. YS: investigation and writing – original draft. SB: investigation and writing – original draft. YL: methodology and writing – original draft. TW: investigation and writing – original draft. YT: investigation and writing – original draft. MW: methodology and writing – original draft. KL: investigation and writing – original draft.
Funding
The author(s) declare that financial support was received for the research and/or publication of this article. This research was funded by the National Key Research and Development Program of China, grant number 2017YFC0603104. This work was financially supported by the National Key R&D Program “Formation Mechanism, Characterization Technology, and Distribution Prediction of Ultra-deep Effective Reservoirs” (grant number 2017YFC0603104).
Acknowledgments
The authors specially thank editors and reviewers for their constructive comments and suggestions to improve the manuscript.
Conflict of interest
The authors declare that the research was conducted in the absence of any commercial or financial relationships that could be construed as a potential conflict of interest.
Publisher’s note
All claims expressed in this article are solely those of the authors and do not necessarily represent those of their affiliated organizations, or those of the publisher, the editors and the reviewers. Any product that may be evaluated in this article, or claim that may be made by its manufacturer, is not guaranteed or endorsed by the publisher.
References
Ahmad, I., Shah, M. M., Janjuhah, H. T., Trave, A., Antonarakou, A., and Kontakiotis, G. (2022). Multiphase diagenetic processes and their impact on reservoir character of the late triassic (rhaetian) kingriali formation, upper indus basin, Pakistan. Minerals 12, 1049. doi:10.3390/min12081049
Allan, J. R., and Matthews, R. K. (1977). Carbon and oxygen isotopes as diagenetic and stratigraphic tools: surface and subsur-face data, Barbados, West Indies. Geology 5 (1), 16–20. doi:10.1130/0091-7613(1977)5<16:caoiad>2.0.co;2
Allan, J. R., and Wiggins, W. D. (1993). Dolomite reservoirs: geochemical techniques for evaluating origin and distribution. Tulsa, Okla: American Association of Petroleum Geologists.
Arvidson, R. S. (1999). The dolomite problem; control of precipitation kinetics by temperature and saturation state. Am. J. Sci. 299 (4), 257–288. doi:10.2475/ajs.299.4.257
Asmerom, Y., Jacobsen, S., Knoll, A. H., Butterfield, N. J., and Swett, K. (1991). Strontium isotopic variations of Neoproterozoic seawater: implications for crustal evolution. Geochem. Cosmochim. Acta 55, 2883–2894. doi:10.1016/0016-7037(91)90453-c
Banner, J. L. (1995). Application of the trace element and isotope geochemistry of strontium to studies of carbonate dia-genesis. Sedimentology 42 (5), 805–824. doi:10.1111/j.1365-3091.1995.tb00410.x
Banner, J. L., Hanson, G. N., and Meyers, W. J. (1988). Rare earth element and Nd isotopic variations in regionally extensive dolomites from the Burlington–Keokuk Formation (Mississippian): implications for REE mobility during carbonate diagenesis. J. Sediment. Res. 58 (3), 415–432.
Bao, Z. D. (1999). Episodic carbonate deposits on the triassic continental slope in southern China. Acta Geol. Sin. 73 (1), 93–103. (in Chinese with English abstract). doi:10.1111/j.1755-6724.1999.tb00815.x
Bao, Z. D., Ji, H. C., Liang, T., Wei, M. Y., Shi, Y. Q., Li, Z. F., et al. (2019). Massive primary dolostones in the Meso–Neoproterozoic: cases study on typical platforms in China. J. Palaeogeogr. 21 (06), 869–884. (in Chinese with English abstract).
Bao, Z. D., Jin, Z. J., Sun, L. D., and Wang, Z. M. (2006). Sea–Level fluctuation of the Tarim area in the early paleozoic: respond-ence from geochemistry and karst. Acta Geol. Sin. 80 (3), 366–373. (in Chinese with English abstract).
Bao, Z. D., Zhu, J. Q., Jiang, M. S., and Xia, Y. (1998). Strontium isotope evolution: responding with sea–level fluctuation: an example of Ordovician in Middle Tarim Area. Sci. Geol. Sin. 7 (3), 329–333. (in Chinese with English abstract).
Bau, M., and Dulski, P. (1996). Distribution of yttrium and rare–earth elements in the Penge and Kuruman iron–formations, Transvaal supergroup, South Africa. Precambrian Res. 79 (1–2), 37–55. doi:10.1016/0301-9268(95)00087-9
Bilal, A., Mughal, M. S., Janjuhah, H. T., Ali, J., Niaz, A., Kontakiotis, G., et al. (2022). Petrography and provenance of the sub-himalayan kuldana formation: implications for tectonic setting and palaeoclimatic conditions. Minerals 12, 794. doi:10.3390/min12070794
Chang, J., Qiu, N. S., Zuo, Y. H., and Li, C. C. (2011). The new evidence on tectonic uplift in Kepingtage area, Tarim, China: constraints from (U–Th)/He ages. Chin. J. Geophys. 54, 163–172. ([in Chinese with English abstract]).
Chen, L. P., Zhang, H., Cai, Z. X., Cong, F., Huang, S., and Tang, P. (2020). Characteristics and formation mechanisms of the unconformity–related paleokarst reservoirs in the Upper Sinian, Northwestern Tarim Basin, China. Mar. Petroleum Geol. 120 (2020), 104559. doi:10.1016/j.marpetgeo.2020.104559
Chen, Y. N., Shen, A. J., Pan, L. Y., Zhang, J., and Wang, X. F. (2017). Features, origin and distribution of microbial dolomite reservoirs: a case study of 4th Member of Sinian Dengying Formation in Sichuan Basin, SW China. Petroleum Explor. Dev. 44, 745–757. (in Chinese with English abstract). doi:10.1016/s1876-3804(17)30085-x
Christie–Blick, N., Dyson, I. A., and Borch, C. C. V. D. (1995). Sequence stratigraphy and the interpretation of Neoproterozoic earth history. Precambrian Res. 73, 3–26. doi:10.1016/0301-9268(94)00096-a
Coplen, T. B., Kendall, C., and Hopple, J. (1983). Comparison of stable isotope reference samples. Nature 302, 236–238. doi:10.1038/302236a0
Davies, G. R., and Smith, L. B. (2006). Structurally controlled hydrothermal dolomite reservoir facies: an overview. AAPG Bull. 90, 1641–1690. doi:10.1306/05220605164
Derry, L. A. (2010). A burial diagenesis origin for the Ediacaran Shuram–Wonoka carbon isotope anomaly. Earth Planet. Sci. Lett. 294 (1), 152–162. doi:10.1016/j.epsl.2010.03.022
Du, Y., Fan, T. L., Hans, G. M., and Gao, Z. Q. (2018). Genesis of upper cambrian–lower ordovician dolomites in the tahe oil-field, Tarim Basin, NW China: several limitations from petrology, geochemistry, and fluid inclusions. Mar. Petroleum Geol. 91, 43–70. doi:10.1016/j.marpetgeo.2017.12.023
Feng, Z. Z., Bao, Z. D., Wu, M. B., Jin, Z. K., Shi, X. Z., and Luo, A. R. (2007). Lithofacies palaeogeography of the ordovician in Tarim area. J. Palaeogeogr. 9, 447–460. (in Chinese with English abstract).
Frimmel, H. E. (2009). Trace element distribution in Neoproterozoic carbonates as palaeo environmental indicator. Chem. Geol. 258 (3–4), 338–353. doi:10.1016/j.chemgeo.2008.10.033
Fritz, R. D., Wilson, J. L., and Yurewicz, D. A. (1993). Paleokarst related hydrocarbon reservoirs, 18. Tulsa, Oklahoma: Society for Sedimentary Geology Core Workshop.
German, C. R., and Elderfield, H. (1990). Application of the Ce anomaly as a paleoredox in dicator: the ground rules. Paleoceanography 5 (5), 823–833. doi:10.1029/pa005i005p00823
Gu, J. Y. (2000). Characteristics and origin analysis of dolomite in lower ordovician of Tarim Basin. Xinjing pet. Geol. 21, 120–122. (in Chinese with English abstract).
Guo, C., Chen, D., Qing, H., Dong, S., Li, G., Wang, D., et al. (2016). Multiple dolomitization and later hydro-thermal alteration on the upper Cambrian–lower Ordovician carbonates in the northern Tarim basin, China. Mar. Petroleum Geol. 72, 295–316. doi:10.1016/j.marpetgeo.2016.01.023
He, J. Y., Qing, H. R., and Xu, B. (2018). The unconformity–related palaeokarst in the uppermost Ediacaran carbonate rocks in the northwestern Tarim Block, NW China: implication for sedimentary evolution during the Ediacaran–Cambrian transition. Int. Geol. Rev. 61, 839–852. doi:10.1080/00206814.2018.1474498
He, J. Y., Wu, G. H., Li, Q. M., Ma, F., and Li, H. H. (2010a). Petroleum geologic characteristics and hydrocarbon exploration objectives of Sinian in Tarim Basin. Xinjiang Pet. Geol. 31, 482–484. (in Chinese with English abstract).
He, X. B., Xu, B., and Yuan, Z. Y. (2007). C–isotope composition and correlation of the upper neoproterozoic in keping area, Xinjiang. Chin. Sci. Bull. 52, 504–511. (in Chinese with English abstract). doi:10.1007/s11434-007-0050-2
He, Z. L., Zhang, J. T., and Ding, Q. (2017). Factors controlling the formation of high–quality deep to ultra–deep carbonate res-ervoirs. Oil Gas Geol. 38 (4), 633–644. (in Chinese with English abstract).
Hill, C. A. (1990). Sulfuric acid speleogenesis of carlsbad cavern and its relationship to hydrocarbons, Delaware basin, New Mexico and Texas (1). AAPG Bull. 74, 1685–1694. doi:10.1306/0c9b2565-1710-11d7-8645000102c1865d
Hood, A. V. S., Wallace, M. W., and Drysdale, R. N. (2011). Neoproterozoic aragonite–dolomite seas? Widespread marine do-lomite precipitation in Cryogenian reef complexes. Geology 39, 871–874. doi:10.1130/g32119.1
Hu, W. X., Chen, Q., Wang, X. J., and Cao, J. (2010). REE models for the dis–crimination offluids in the formation and evolution of dolomite reservoirs. Oil Gas Geol. 31 (6), 810–818.
Huang, T. Z. (2014). Structural interpretation and petroleum exploration targets in northern slop of middle Tarim Basin. Petroleum Geol. Exp. 36, 257–267. (in Chinese with English abstract).
Jacobsen, S. B., and Kaufman, A. J. (1999). The Sr, C and O isotopic evolution of Neoproterozoic seawater. Chem. Geol. 161, 37–57. doi:10.1016/s0009-2541(99)00080-7
Jiao, C. L., Zheng, X. J., He, B. Z., Chen, D. Z., Li, C. C., and Liu, Z. B. (2011). Characteristics and genetic types of lower Paleozoic dolomite reservoirs in Tarim Basin. Geol. China 38 (4), 1008–1015. (in Chinese with English abstract).
Jin, Z. J. (2006). New progresses in research of China’s typical superimposed basins and reservoiring of hydrocarbons (Part II): taking Tarim basin as an example. Oil Gas Geol. 27, 281–294. (in Chinese with English abstrac).
Kang, Y. Z. (2008). Reservoir features and petroleum distribution of Paleozoic carbonate paleokarst in China. Nat. Gas. Ind. 28, 1–12. (in Chinese with English abstract).
Kerans, C. (1988). Karst–controlled reservoir heterogeneity in Ellenburger Group carbonates of west Texas. AAPG Bull. 72, 86–97. doi:10.1306/703c996f-1707-11d7-8645000102c1865d
Klimchouk, A. B., Auler, A. S., Bezerra, F. H. R., Cazarin, C. L., Balsamo, F., and Dublyansky, Y. (2016). Hypogenic origin, geologic controls and functional organiza-tion of a giant cave system in Precambrian carbonates, Brazil. Geomorphology 253, 385–405. doi:10.1016/j.geomorph.2015.11.002
Lawrence, M. G., Greig, A., Collerson, K. D., and Kamber, B. S. (2006). Rare earth element and yttrium variability in South East Queensland waterways. Aquat. Geochem. 12, 39–72. doi:10.1007/s10498-005-4471-8
Li, P. W., Luo, P., Chen, M., Song, J. M., Jin, T. F., and Wang, G. Q. (2015). Characteristics of upper sinian dolostone reservoirs in northwestern margin of Tarim Basin. Mar. Orig. Pet. Geol. 20, 1–12. (in Chinese with English abstract).
Liang, T., Bao, Z. D., Zhu, X. E., Guo, Y., Li, J., and Jones, B. (2020). Rare earth elements in dolostones and limestones from the Mesoproterozoic Gaoyuzhuang Formation, North China: implications for penecontemporaneous dolomitization. J. Asian Earth Sci. 196, 104374–104414. doi:10.1016/j.jseaes.2020.104374
Lin, Z. M. (2002). Carbonate rock reservoir features and oil–gas accumulating conditions in the ordovician of tahe oil-field in northern Tarim Basin. Acta Petrol. Sin. 23, 23–26. (in Chinese with English abstract).
Liu, C., Xie, Q. B., Tang, Y., Wang, G. W., Song, Y. F., Tang, Y., et al. (2017). Rare earth element characteristics of the carboniferous Huanglong Formation dolomites in eastern Sichuan Basin, southwest China: Implications for origins of dolomitizing and diagenetic fluids. Mar. Petroleum Geol. 81, 33–49. doi:10.1016/j.marpetgeo.2016.12.030
Liu, Y. S., Hu, Z. C., Gao, S., Günther, D., Xu, J., Gao, C. H., et al. (2008). In situ analysis of major and trace elements of anhydrous minerals by la–icp–ms without applying an internal standard. Chem. Geol. 257, 34–43. doi:10.1016/j.chemgeo.2008.08.004
Ma, F., Xu, H. X., Gu, J. Y., Shao, L.Y., Wu, J. H., Liu, Y. F., et al. (2009). Cambrian dolomite origin and reservoir evolution in east Tarim Basin. Petroleum Exploration Dev. 36 (2), 144–155. (in Chinese with English abstract).
Ma, F., Yang, L. M., Gu, J. Y., Chen, X., Zhao, Z., Jin, Y. N., et al. (2011). The summary on exploration of the dolomite oilfields in the world. Acta Sedimentologica Sinica. 29 (5), 1010–1021. (in Chinese with English abstract).
Mazzullo, S. J., and Harris, P. M. (1992). Mesogenetic dissolution: its role in porosity development in carbonate reservoirs (1). AAPG Bull. 76, 607–620. doi:10.1306/bdff8880-1718-11d7-8645000102c1865d
McLennan, S. M. (1989). Rare earth elements in sedimentary rocks; influence of provenance and sedimentary processes. Rev. Mineral. Geochem. 21, 169–200.
Nothdurft, L. D., Webb, G. E., and Kamber, B. S. (2004). Rare earth element geochemistry of Late Devonian Reefal carbonates, Canning Basin, Western Australia: confirmation of a seawater REE proxy in Ancient limestones. Geochem. Cosmochim. Acta 68 (2), 263–283. doi:10.1016/s0016-7037(03)00422-8
Peters, S. E., and Gaines, R. R. (2012). Formation of the‘great unconformity’ as a trigger for the Cambrian explosion. Nature 484, 363–366. doi:10.1038/nature10969
Praekelt, H. E., Germs, G. J. B., and Kennedy, J. H. (2008). A distinct unconformity in the cango caves group of the neoprotero-zoic to early paleozoic saldania belt in South Africa: its regional significance. S. Afr. J. Geol. 111, 357–368. doi:10.2113/gssajg.111.4.357
Qi, L. X. (2014). Exploration practice and prospects of giant carbonate field in the Lower Paleozoic of Tarim Basin. Oil Gas Geol. 35, 771–779. (in Chinese with English abstract).
Qi, L. X., and Yun, L. (2010). Development characteristics and main controlling factors of the Ordovician carbonate karst in Tahe Oilfield. Oil Gas Geol. 31, 1–12. (in Chinese with English abstract).
Qian, Y. X., He, Z. L., Li, H. L., Chen, Y., Jin, T., Sha, X. G., et al. (2017a). Discovery and discussion on origin of botryoidal dolostone in the upper sinian in north Tarim Basin. J. Palaeogeogr. 19, 197–210. (in Chinese with English abstract).
Qian, Y. X., You, D. H., Cheng, D. Z., and Qing, H. R. (2012). Petrology, geochemical characteristics and genesis of the upper Middle Cambrian dolomite in Kuroktage,northeast Tarim: comparison with Whirlpool point profile in western Canada basin. Acta Petrol. Sin. 28 (8), 2525–2541. (in Chinese with English abstract).
Qing, H., and Mountjoy, E. W. (1994b). Rare earth element geochemistry of dolomites in the Middle Devonian Presqu'ile barrier, Western Canada Sedimentary Basin: implicationsforfluid rock ratios during dolomitization. Sedimentology 41 (4), 787–804. doi:10.1111/j.1365-3091.1994.tb01424.x
Rahim, H., Qamar, S., Shah, M. M., Corbella, M., Martín-Martín, J. D., Janjuhah, H. T., et al. (2022). Processes associated with multiphase dolomitization and other related diagenetic events in the jurassic samana suk formation, himalayan foreland basin, NW Pakistan. Minerals 12, 1320. doi:10.3390/min12101320
Ren, Y., Zhong, D. K., and Gao, C. L. (2016). Carbon and oxygen isotope compositions and its paleoenvironment implication of Lower Cambrian Longwangmiao Formation in the east part of Sichuan Basin. Mar. Orig. Pet. Geol. 21 (4), 11–20.
Saylor, B. Z., Grotzinger, J. P., and Germs, G. J. B. (1995). Sequence stratigraphy and sedimentology of the neoproterozoic kui-bis and schwarzrand subgroups (nama group), southwestern Namibia. Precambrian Res. 73, 153–171. doi:10.1016/0301-9268(94)00076-4
Seewald, J. S. (2003). Organic–inorganic interactions in petroleum–producing sedimentary basins. Nature 426, 327–333. doi:10.1038/nature02132
Shao, L. Y., Han, J., and Ma, F. (2010). Cambrian dolomite reservoir and facies control characteristics in Eastern Tarim Basin. Acta Sedimentol. Sin. 28 (5), 953–960.
Shi, K. B., Liu, B., Tian, J. C., and Pan, W. Q. (2016). Sedimentary characteristics and lithofacies paleogeography of sinian in Ta-rim Basin. Acta Pet. Sin. 37, 1344–1360. (in Chinese with English abstract).
Spooner, E. (1976). The strontium isotopic composition of seawater and seawater oceanic crust interaction. Earth Planet. Sci. Lett. 31, 167–174. doi:10.1016/0012-821x(76)90108-4
Turner, S. A. (2010). Sedimentary record of late neoproterozoic rifting in the NW Tarim Basin, China. Precambrian Res. 181, 85–96. doi:10.1016/j.precamres.2010.05.015
Wang, G. Z., Liu, S. G., Ma, Y. S., Xu, G. S., and Cai, X. Y. (2010a). Characteristics of subaerial karstification and late reconstruc-tion in the Dengying Formation, Sichuan Basin, southwestern China. J. Earth Sci. 21, 290–302. doi:10.1007/s12583-010-0093-1
Wang, L., Hu, W., Wang, X., Cao, J., and Chen, Q. (2014). Seawater normalized REE patterns of dolomites in Geshan and Panlongdong sections, China: implications for tracing dolomitization and diageneticfluids. Mar. Petroleum Geol. 56, 63–73. doi:10.1016/j.marpetgeo.2014.02.018
Wang, Y., Shi, Z. J., Qing, H. R., Tian, Y., and Gong, X. (2021). Petrological characteristics, geochemical characteristics, and dolomite model of the lower cambrian Longwangmiao Formation in the periphery of the Sichuan Basin, China. J. Petroleum Sci. Eng. 202 (2021), 108432. doi:10.1016/j.petrol.2021.108432
Warren, J. K. (2000). Dolomite: occurrence, evolution and economically important associations. Earth Sci. Rev. 52 (1–3), 1–81. doi:10.1016/s0012-8252(00)00022-2
Webb, G. E., and Kamber, B. S. (2000). Rare earth elements in Holocene reefal microbialites: a new shallow seawater proxy. Geochimica Cosmochimica Acta 64 (9), 1557–1565. doi:10.1016/s0016-7037(99)00400-7
Xiang, P. F., Ji, H. C., Shi, Y. Q., Huang, Y., Sun, Y., Xu, X., et al. (2020). Petrographic, rare earth elements and isotope constraints on the dolomite origin of Ordovician Majiagou Formation (Jizhong Depression, North China). Mar. Petroleum Geol. 120, 104374. doi:10.1016/j.marpetgeo.2020.104374
Xiong, S. Y., Zhou, G., and Hao, Y. (2021). Reservorir space type, genesis and evolution of dolomite reservoir in the guanwushan Formation of midlle Devonian,Western sichuan. Acta Geol. Sin. 95 (12), 3899–3914.
Yu, B. S., Dong, H. L., Widom, E., Chen, J. Q., and Lin, C. S. (2009). Geochemistry of basal Cambrian black shales and cherts from the Northern Tarim Basin, Northwest China: implications for depositional setting and tectonic history. J. Earth Sci. 34, 418–436. doi:10.1016/j.jseaes.2008.07.003
Zhang, D. M., Bao, Z. D., and Pan, W. Q. (2014). The Middle Cambrian evaporate platform dolomite reservoirs characteristics and their genetic mechanism in Xiaoerbulak, Tarim Basin. Nat. Gas. Geosci. 25 (4), 498–507.
Zhang, p, Hou, G. T., Pan, W. Q., et al. (2013). Research on the effectiveness of fractures in sinian–cambrian dolomite reservoir in Tarim Basin. Acta Sci. Nat. Univ. Pekin. 49 (6), 993–1001.
Zhao, C. L., Xu, Y. K., Bai, G. Y., and Lai, X. K. (1977). Sedimentary characteristics and facies analysis of the gaoyuzhuang for-mation – wumishan Formation in the north–central taihang mountains. J. East China Petroleum Inst. 3, 118–138.
Zheng, J. F., Liu, Y., and Zhu, Y. J. (2021). Geochemical features and its geological significances of the upper sinian qigeblak Formation in wushi area,Tarim Basin. J. Palaeogeogr. Chin. Ed. 23 (5), 983–988.
Keywords: primary dolomite, reservoir controlling factors, genetic model of dolomite reservoir, Upper Sinian, Tarim Basin
Citation: Li Z, Bao Z, Su Y, Ban S, Li Y, Wang T, Tao Y, Wei M and Lu K (2025) Characteristics and genetic model of dolomite reservoirs in the Qigebulak Formation, Upper Sinian, Tarim Basin, China. Front. Earth Sci. 13:1456597. doi: 10.3389/feart.2025.1456597
Received: 28 June 2024; Accepted: 31 March 2025;
Published: 06 May 2025.
Edited by:
George Kontakiotis, National and Kapodistrian University of Athens, GreeceReviewed by:
Vincenzo Tripodi, National Research Council (CNR), ItalyIhtisham Islam, Shaheed Benazir Bhutto University, Pakistan
Copyright © 2025 Li, Bao, Su, Ban, Li, Wang, Tao, Wei and Lu. This is an open-access article distributed under the terms of the Creative Commons Attribution License (CC BY). The use, distribution or reproduction in other forums is permitted, provided the original author(s) and the copyright owner(s) are credited and that the original publication in this journal is cited, in accordance with accepted academic practice. No use, distribution or reproduction is permitted which does not comply with these terms.
*Correspondence: Zhidong Bao, YmFvemhkQGN1cC5lZHUuY24=