- 1The 7th Geological Brigade of Sichuan, Leshan, China
- 2School of Management, China University of Mining and Technology (Beijing), Beijing, China
- 3Sichuan Vocational College of Finance and Economics, Chengdu, China
- 4China Aerospace Planning and Design Group Co., Ltd., Beijing, China
- 5Institute of Mineral Resources, Chinese Academy of Geological Sciences, Beijing, China
- 6Research Center for Strategy of Global Mineral Resources, Chinese Academy of Geological Science, Beijing, China
Background: The impact of phosphate mining development on surface water pollution and ecological disasters is a highly concerned issue in global mining activities. With the increasing demand for phosphate fertilizers, the environmental impact of phosphate mining is becoming increasingly prominent, especially in the Mabian Jinyang area of Sichuan Province. This study aims to analyze the mechanisms and impacts of water pollution and ecological disasters caused by phosphate mining development, and evaluate the ecological risks caused by heavy metal pollution in water bodies.
Method: We conducted a systematic assessment of the impact of phosphate mining development on water pollution and ecological risks in the Mabian Jinyang area of Sichuan Province using chemical analysis and potential ecological risk index (PERI) method. The total area of the region is approximately 6,400 square kilometers. We conducted systematic water sample collection and analysis at 367 sampling points from January 2020 to December 2021. By collecting water samples from different work areas, measuring the content of heavy metal elements and total phosphorus in the samples, and quantitatively evaluating the potential threat of heavy metal pollution to the aquatic ecosystem.
Result: The research results show that in the mining work area, both heavy metal element indicators and total phosphorus indicators exhibit point pollution, and the total phosphorus concentration in the water body varies greatly with the seasons. One of the main reasons for the excessive total phosphorus is the collapse caused by geological disasters and the non-standard stacking of slag and gangue piles in the mining development area. In addition, in terms of potential ecological hazards, it is positively correlated with the degree of mining development, and the higher the degree of mining development, the greater the potential ecological hazards. Although the potential ecological hazards of various heavy metals in the water bodies of the work area are not high overall, there are still some areas that exhibit mild to moderate ecological hazards, as well as very few areas with strong ecological hazards. These areas are mainly affected by strong ecological risks caused by Cd and As elements.
Meaning: The research findings of this article provide valuable information for mining enterprises and environmental organizations, promoting a balance between phosphate mining and environmental protection, and driving mining activities towards a more sustainable direction. By identifying pollution characteristics and ecological risks, this study provides theoretical support for future environmental management and pollution control measures in mining areas.
1 Introduction
Phosphate rock resources are an important source of fertilizer for global agricultural production, and mining output has increased significantly in the past decades. The global production of phosphate ore has risen to about 240 million tons per year, with countries such as China, Morocco, and the United States leading in mining. In 2019, phosphate mining accounted for approximately 90% of global fertilizer production (Geissler et al., 2019). The mining of phosphate ore in the Jinyang area of Mabian plays a crucial role in the local economy, providing employment opportunities and promoting agricultural production through the supply of phosphate fertilizers. However, the rapid expansion of mining activities has also led to social challenges, including community displacement and water resource conflicts (Othman and Al-Masri, 2007; Ma et al., 2015), especially surface water pollution and ecological disasters, which have become important issues of concern in today’s mining activities (Zhang et al., 2023; Li et al., 2010; Fan et al., 2022; Li et al., 2023). Phosphate mining involves various processes that lead to pollution and contamination. The mining of phosphate ore releases particulate matter, which can be carried by the wind and cause air pollution. In addition, the treatment of mining waste containing toxic elements such as cadmium and mercury often leads to soil pollution. The chemical processes involved in phosphate processing, including the release of phosphoric acid and other harmful by-products, may lead to pollution of surrounding water bodies. These pollutants, especially phosphorus compounds, can be transported through groundwater flow, surface runoff, and sedimentation, leading to widespread ecological damage. Over time, these pollutants will accumulate in water systems, leading to eutrophication, hypoxia, and harmful algal blooms in affected areas (Diahou et al., 2023; Khelifi et al., 2021; Filho et al., 2024). At the same time, facing the challenges of global climate change and ecological degradation, the dual carbon target has become an important component of national and regional environmental policies (Chen et al., 2023; Chen et al., 2024a; Chen et al., 2024b; Chen et al., 2024c; Chen et al., 2024d). In this context, environmental management of phosphate mining is particularly important, and it is urgent to combine the dual carbon policy to promote green mining practices, in order to reduce the negative impact of mining activities on the ecological environment and promote the sustainable use of resources.
The impact of phosphate mining on water environment is mainly reflected in heavy metal and total phosphorus pollution. During the mining process, a large amount of waste and slag are often randomly piled up, resulting in an increase in the concentration of heavy metal elements such as Cu, Pb, Zn, Cd, etc., in the water. In addition, the phosphorus element in phosphate ore enters the water through various pathways, further exacerbating the problem of eutrophication in the water (Luo et al., 2023; Tummala et al., 2024; Wang Y. et al., 2022). These pollutants not only affect water quality, but may also accumulate through the food chain, ultimately endangering human health. Although previous studies have shown that phosphate mining can cause heavy metal pollution to water bodies (Hayder et al., 2023; Al-Hwaiti et al., 2018), current research mostly focuses on the local distribution of pollutants and the analysis of single indicators, lacking a systematic comparison of spatial differences in pollution characteristics and ecological risks between different work areas. In addition, there is still insufficient research on the combined effects of heavy metals and total phosphorus in the development process of mining areas, as well as their comprehensive ecological hazards. Especially limited research has been conducted on the migration patterns and cumulative effects of water pollution under different geographical environmental conditions, resulting in a lack of comprehensive understanding of the mechanisms of pollution diffusion and long-term potential ecological risks. This study aims to explore the potential impact of phosphate mining activities on surface water environment, particularly in terms of heavy metal and total phosphorus pollution. The main objectives of the study include: 1) assessing the levels of heavy metal and total phosphorus pollution in water bodies caused by phosphate mining development in the Mabian Jinyang area of Sichuan Province; 2) Analyze the spatial distribution characteristics of these pollutants and their correlation with mining activities; 3) Quantify the potential ecological risks of aquatic ecosystems using the Potential Ecological Risk Index (PERI) method; 4) Propose environmental protection strategies and recommendations based on research results to promote the sustainable development of mining activities.
This study focuses on the development of phosphate mines in the Mabian Jinyang area of Sichuan Province. Three typical working areas, Laoheba, Liugushui, and Maiziping, were selected. Chemical analysis and potential ecological risk index methods were comprehensively used to systematically analyze the impact of phosphate mine development on the distribution of heavy metals and total phosphorus in water bodies and their potential ecological hazards. The aim of this study is to reveal the characteristics of water pollution and its ecological risks during phosphate mining, and provide scientific basis for the sustainable development of phosphate resources. By filling the gap in existing research on the spatial variability and cumulative risk of water pollution in different work areas, we aim to identify the spatial differences in pollution levels and their causes, in order to provide theoretical support for future environmental management and pollution control measures in mining areas.
2 Methodology
The sampling strategy of this study is to ensure that the sample can represent the water conditions in the study area, as shown in Figure 1. We collected water samples in three typical work areas in the Mabian Jinyang area of Sichuan Province: Laoheba, Liugushui, and Maiziping. The selection of sample collection points is based on factors such as geographical location, land use type, and human activity intensity. The collection of water samples in the work area mainly focuses on the Mabian River basin and its tributaries. In each work area, we collected water samples during the wet and dry seasons to capture the impact of seasonal changes on water quality. Water samples should be collected during periods of stable water level. The instantaneous sampling method should be used for water sample collection, and the water body should be gently disturbed during sampling. Before sampling, the water bottle and stopper should be washed with the water to be collected 3–5 times, and then the sampling bottle should be sunk to a depth of 30 cm underwater for sampling. After water sample collection, it should be promptly sent to the laboratory for analysis and testing. Generally, the period from sampling date to laboratory analysis and testing should not exceed 7 days. Special analysis projects shall be executed in accordance with relevant requirements. Before transporting water samples, a sample delivery form should be filled out, and the sample delivery form and sample label should be checked for accuracy. During the transportation of samples, attention should be paid to shock resistance, antifreeze, sun protection, and pollution prevention. The laboratory should verify and sign for the samples. We used standardized chemical analysis methods to determine heavy metal elements (such as Cu, Pb, Zn, As, Cd, Mn, and Hg) and total phosphorus content in water samples. These analysis results will be used for subsequent pollution assessment and ecological risk analysis. The obtained chemical analysis data was first cleaned to eliminate outliers and missing values. Subsequently, we normalized the data to eliminate the influence of different measurement units on the analysis results. In addition, we also calculated the concentration range, mean, and standard deviation of each element, providing basic data for statistical evaluation. To assess the potential ecological risks of heavy metal pollution in water bodies, we used the Potential Ecological Risk Index (PERI) method (Zhang and Xu, 2023; Wang N. et al., 2022; Cao et al., 2023; Zhao et al., 2024). This method quantifies the potential threat of heavy metal pollution to aquatic ecosystems, helps us identify areas with higher levels of pollution, and evaluates the pollution characteristics and ecological risks between different work areas. We use the average background value of water bodies in Chengdu Economic Zone as the evaluation criterion to ensure the comparability of the evaluation results.
The evaluation standards for heavy metals in water bodies was shown in Table 1. In this work, the water sample was analyzed for heavy metal elements of Cd, Cr, Cu, Pb, Zn, Co using inductively coupled plasma mass spectrometry (ICP-MS). Using an atomic fluorescence spectrometer (instrument model: AFS-930) to measure As and Hg. The detection limits for As, Cd, Cr, Cu, Pb, Zn, Hg, Co, and pH are 0.05, 0.01, 2.0, 2.0, 5.0, 10, 0.005, 0.1, and 0.01, respectively, with units of μ g/L and dimensionless pH values. The detection limit of inductively coupled plasma mass spectrometer ICP-MS is 10 × 10-12 μ g/L, and the process follows GB/T 8538-2008; The detection limit of AFS-930 is 10-11∼10-12 μ g/L. During the testing, water quality standard samples GBW08606, GBW (E) 080272, GS-BZ50020-90, GSB04-1767-2004, and GSBZ50032-94 were inserted separately (controlling different items). For those without standard samples, the quality was monitored using spiked recovery method. A total of 3 internal monitoring samples were inserted, and the pass rate was 100%. The B element in the water sample was determined by inductively coupled plasma mass spectrometer; Fe2+, Fe3+, I, etc. are determined by spectrophotometry; Cl-, SO42-, F, PO43-, NO3-, etc. are analyzed using ion chromatography; Free CO2, total hardness, total dissolved solids, oxygen consumption, etc. are measured by volumetric method; The pH value is determined using the glass electrode method. MAPGIS is used as the basic platform for mapping, and data processing, parameter statistics, histograms, etc. are all completed in the Geochem Studio software program. The obtained analytical data should be subjected to basic geochemical parameter statistics for the entire region and sub regions (such as geological units), including sample size (N), area (S), mean (X), standard deviation (S), coefficient of variation (CV), background mean (C) gradually excluding the mean plus or minus three times the standard deviation, median (M), maximum value (X), minimum value (X), and lower limit of anomalies (T).
3 Results and discussion
3.1 Results of the complete water analysis
3.1.1 Laoheba work area
It can be seen from Table 2 that the pH values of water samples collected in the Mabian Laoheba working area in 2021 were mostly between 7 and 8.5, with an average value of 7.93, which is weakly alkaline.
It can be concluded from the water quality sample diagram of the work area (Figure 2) that there are a total of 70 water quality samples in the work area. The Laoheba area is divided into Shanshuigou and Haroluo River basins. The water quality is all in Class I area, alkaline earth metal ions exceed alkali metal ions, weak acid radicals exceed strong acid radicals, and carbonate hardness exceeds 50%, which is generally a typical Ca-HCO3 type of water quality. In terms of water quality, the total dissolved solids exceeded the standard only in Shanshuigou, which was caused by the slag dump entering the river channel after rainwater scouring. The chemical oxygen demand of each small watershed in Laoheba was relatively low; the total phosphorus index of the water body was relatively low this year, with an average total phosphorus of 0.94 mg/L. There was one place in Tongchanggang (1.01 mg/L) and two places in Baofengping (1.15 mg/L and 2.20 mg/L), which were slightly exceeded. The maximum value in the basin was in Shanshuigou (52.5 mg/L), which was caused by the scouring of the slag dump and the spoil dump in the rainy season upstream. These results indicate that although the water quality in the Laoheba working area is generally good, the risk of local pollution caused by mining activities still exists, especially near the slag yard. Research suggests that mining area management should strengthen the control and disposal of waste disposal, especially during the rainy season, to reduce potential pollution to water bodies. This provides a scientific basis for pollution control measures in the region, especially for strategies to prevent the spread of heavy metal and phosphorus pollution, which has practical significance.
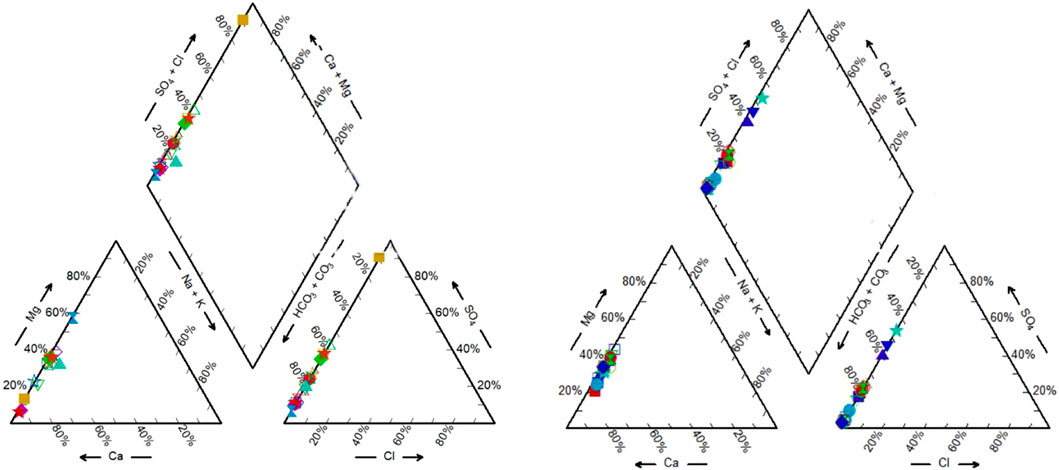
Figure 2. Piper three-line diagram of water quality in the Laoheba area of Mabian (Shanshuigou on the left and Haroluo River on the right).
3.1.2 Liugushui working area
As can be seen from Table 3, the pH values of water samples collected in the Liugu Water Work Area in Mabian in 2021 are mostly between 8.08 and 8.48, with an average value of 8.34, which is alkaline.
From the water quality sample diagram of the work area (Figure 3), it can be concluded that there are a total of 45 water quality samples in the work area. The Liugu Water Work Area is all in the Xuekoushan River Basin. The water quality is all in Class I area, alkaline earth metal ions exceed alkali metal ions, weak acid radicals exceed strong acid radicals, and carbonate hardness exceeds 50%, mainly Ca-HCO3 type water quality. In terms of water quality, the total dissolved solids are less than 1000 mg/L, the total hardness is less than 450 mg/L, SO42- is less than 250 mg/L, and the chemical oxygen demand is not exceeded; the total phosphorus index of the water body is relatively low this year, with an average total phosphorus concentration of 0.039 mg/L. The water quality status of the Liugushui working area reflects that the development of the mining area has a relatively small impact on the water body, which may be related to its geographical location, mining scale, and waste management measures. This provides a basis for maintaining existing environmental management and monitoring measures in the region, while also offering successful experiences for other similar areas, demonstrating how to reduce negative impacts on the environment through reasonable development management.
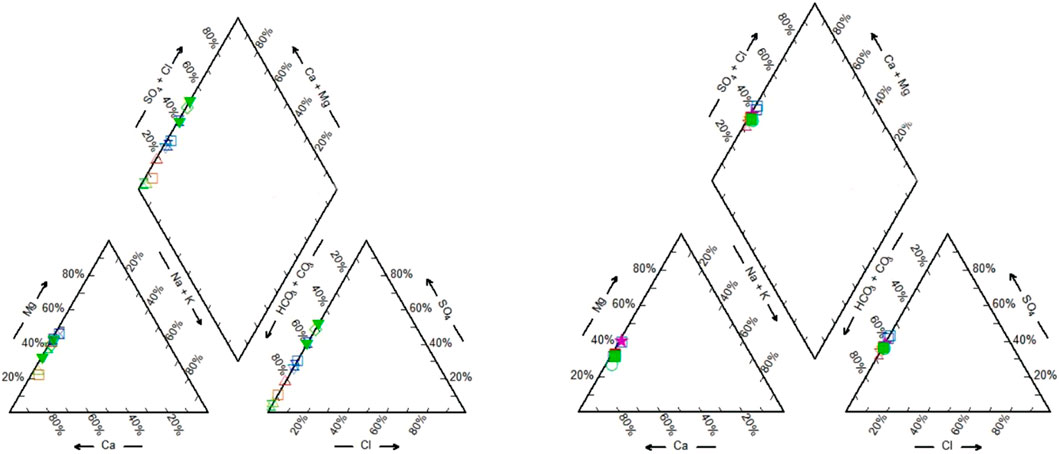
Figure 3. Piper three-line diagram of water quality in the Laoheba area of Mabian. (left is the upper reaches of Xuekoushan River, right is the lower reaches of Xuekoushan River).
3.1.3 Maiziping work area
As can be seen from Table 4, the pH values of water samples collected in the Mabian Laoheba work area in 2021 are mostly between 7.7 and 8.51, with an average value of 8.29, which is weakly alkaline.
From the water quality sample diagram of the work area (Figure 4), it can be concluded that there are a total of 48 water quality samples in the work area. The Maiziping area is divided into the Shanshuigou and Haroluo River basins. The water quality is all in the Class I area. The alkaline earth metal ions exceed the alkali metal ions, the weak acid radicals exceed the strong acid radicals, and the carbonate hardness exceeds 50%, which is a typical Ca-HCO3 type of water quality. In terms of water quality, the total dissolved solids are less than 1000 mg/L, the total hardness is less than 450 mg/L, SO42− is less than 250 mg/L, and the chemical oxygen demand is not exceeded; the total phosphorus index of the water body is relatively low this year, and the average total phosphorus concentration is 0.04 mg/L. The water quality analysis of Maiziping work area shows that the area is also less affected by phosphate mining, especially with total phosphorus and other key water quality indicators remaining at a low level. This result demonstrates the good state of water conservation in the work area, providing valuable experience for other mining areas in water management, and emphasizing the importance of reasonable mining area management and environmental protection measures in maintaining water ecological security.
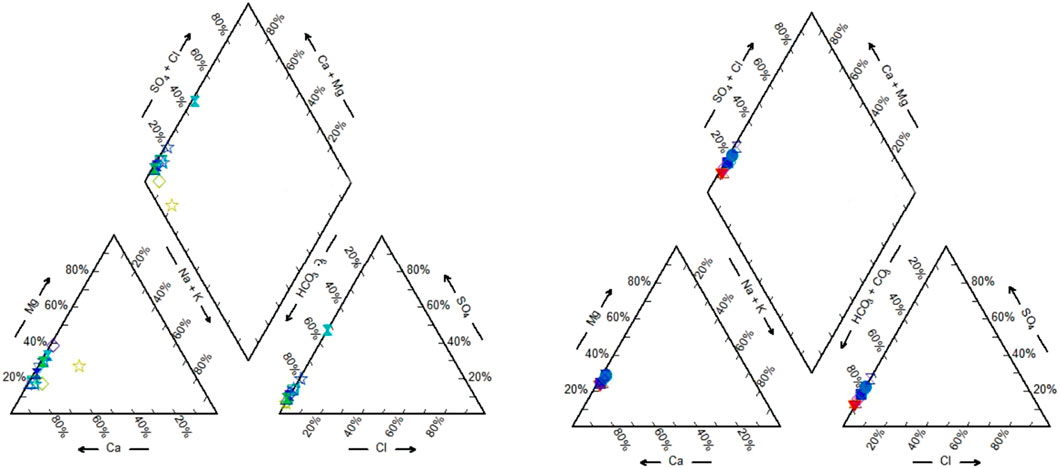
Figure 4. Piper three-line diagram of water quality in Maiziping area, Mabian (left is the Jinhe and Fenyinhe watersheds in Maiziping, right is the Dayuanzi River watershed).
3.2 Heavy metal characteristics of water bodies
3.2.1 Laoheba working area
The water samples collected in the working area are mainly from the Mabian River basin and its tributaries. The indicators of the water samples collected during the flood season are statistically analyzed. The statistical results are shown in Table 5. The coefficients of variation of the test indicators of heavy metals such as Pb, As, Zn, Cd, Cu, and P are as high as 166.72, 78.39, 196.66, 151.5, 169.05, and 664.19, respectively, indicating that the distribution of P and heavy metals such as Cu, Pb, and Zn in the working area is extremely uneven.
The work area is located in the Laoheba area of Mabian, where mining activities are highly developed, mainly concentrated in the Laoheba Shanshuigou, Erba, Baofengping, Tongchanggang and other areas in Yanfeng Township, Mabian County. The main mining species are phosphate and a small amount of lead and zinc. The high values of heavy metals such as Pb, As, Zn, Cd, Cu, and P are all located in the above areas.
The water samples collected this time were contained in pure water bottles. The heavy metals of the water samples were tested at the Testing Center of the Institute of Comprehensive Utilization of Mineral Resources of the Chinese Academy of Geological Sciences, and the analysis results of 8 heavy metal elements were obtained (Table 6). According to the GB3838-2002 water area function and standard classification, according to the surface water environmental function and protection objectives, it is divided into Class I-V according to the function level. 70 surface water samples were collected in the Laoheba work area of Mabian, and the statistical table of the heavy metal analysis results of the water body is as follows.
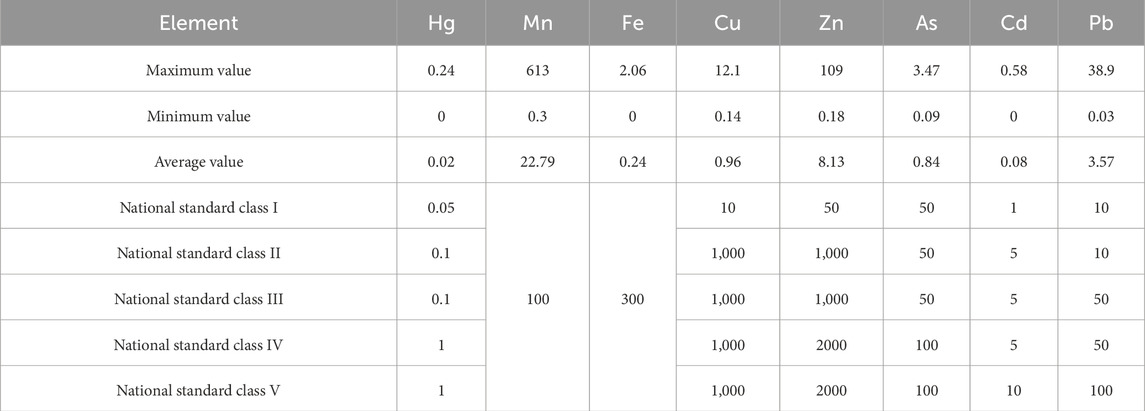
Table 6. Statistical table of water element analysis results in the Mabian Laoheba working area (μg/L).
As can be seen from Table 6, the two elements of Fe and Mn are not classified, which are the standard limits of the supplementary project of surface water sources. The overall content of heavy metals in the mines of the Mabian Laoheba working area is not high. Some elements have a large range of variation in water. The detection rate of each element is 100%, indicating that the content of each element in the water body is relatively low. Hg, Fe, As, and Cd all meet the Class I standard; most of the Mn elements meet the Class I standard, and there are individual exceeding the standard in Shanshuigou (1) and Tongchanggang tailings pond (2); Cu element meets the Class I standard, and only one place in the Erba River slightly exceeds the standard; Zn and Pb elements only slightly exceed the standard in one place in Shanshuigou. From the analysis results of the heavy metal content in the water body, the degree of heavy metal pollution in the Laoheba working area is low. These results reveal that heavy metal pollution in the Laoheba work area is directly related to mining activities, especially in high-risk areas such as slag yards and copper plant embankments. The uneven distribution and local exceedance of heavy metals indicate weak links in environmental management in mining areas. The research results provide a scientific basis for strengthening pollution source monitoring and waste disposal in the region. It is recommended to strengthen anti-seepage measures and waste management in high-risk areas, especially during the rainy season, to prevent pollutants from entering water bodies through surface runoff, thereby reducing the threat of heavy metals to the water environment.
3.2.2 Liugushui working area
The water samples collected in the working area are mainly from the Xuekoushan River basin and its tributaries, a tributary of the Mabian River. The indicators of the water samples collected during the flood season were statistically analyzed. The statistical results are shown in Table 7. The coefficients of variation of the test indicators of heavy metals such as Pb, As, Zn, Cd, Cu and P are not high. Only the coefficient of variation of Cd is slightly higher at 254.60, indicating that the distribution of heavy metal elements and P in the working area is relatively uniform.
The working area is located in the Liugushui phosphorus, lead and zinc ore cluster area in Mabian, Sichuan. The mining activities are highly developed, mainly concentrated in Liugushui, Wenshuitang and Niukejiao in Xuekoushan Township, Mabian County. The main mining species are phosphorus and lead-zinc ore. The high values of heavy metals such as Pb, As, Zn, Cd, Cu and P are all located in the above areas.
The water samples collected this time were stored in pure water bottles. The heavy metals in the water samples were tested at the Testing Center of the Institute of Comprehensive Utilization of Mineral Resources, Chinese Academy of Geological Sciences, and the analysis results of 8 heavy metal elements were obtained (Table 8).
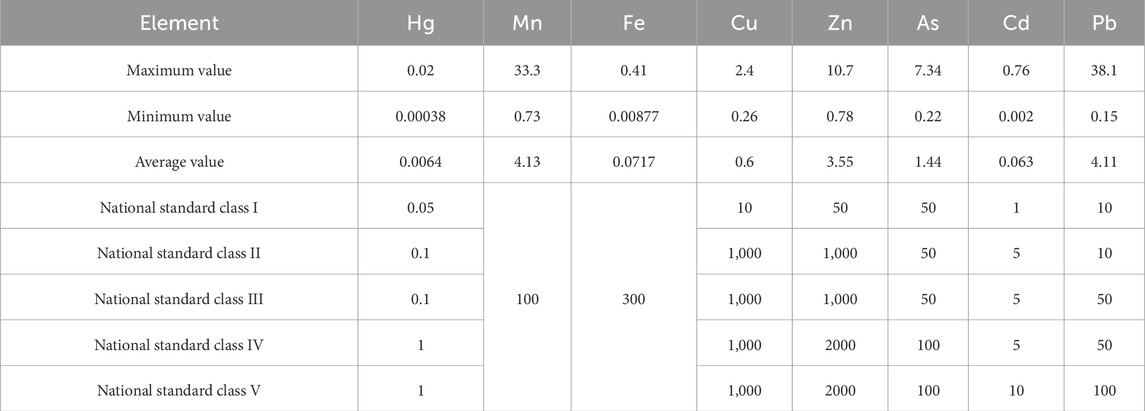
Table 8. Statistical table of element analysis results of the Xuekoushan River Basin water body (μg/L).
According to the GB3838-2002 water body function and standard classification, according to the surface water environmental function and protection objectives, it is divided into Class I-V according to the function level. 45 surface water samples were collected in the Xuekoushan River Basin work area in Mabian, and the statistical table of the heavy metal analysis results of the water body is as follows.
As can be seen from Table 8, the two elements Fe and Mn are not classified, which are the standard limits of the supplementary project for surface water sources. The overall content of heavy metals in the mines in the Mabian Laoheba working area is not high, and the variation range of some elements in the water is large. The detection rate of each element is 100%, indicating that the content of each element in the water body is relatively low. Except for 3 sampling points where Pb exceeds the standard, the water samples of Xuekoushan River meet the Class I standard; Pb element exceeds the standard in individual cases such as water inrush in the Niukejiao tunnel and leaching in the slag yard. From the analysis results of the heavy metal content in the water body, the degree of heavy metal pollution in the Liugushui working area is related to the mining activities in the mining area. The heavy metal analysis results of the Liugushui working area show that although mining activities have had a certain impact on the environment, the overall pollution level is relatively low, especially the heavy metal content of most sampling points does not exceed the standard. This indicates that through appropriate environmental management measures, the spread of pollution during the mining process can be effectively controlled. It is suggested to further strengthen the monitoring of heavy metals such as Cd with significant fluctuations, especially in areas with local exceedances such as Jiankejiao, to ensure effective control of pollution risks.
3.2.3 Maiziping work area
The water samples collected in the work area are mainly from the Jinhe River and Fenyinhe River basins in the Maiziping area of Mabian and the upper reaches of the Dayuanzi River, a tributary of the Mabian River. The indicators of the water samples collected this time were mathematically statistically analyzed. The statistical results are shown in Table 9. The coefficients of variation of the test indicators of heavy metals such as Pb, Zn, and Cd are as high as 380.69, 336.32, and 327.18, respectively, indicating that the distribution of heavy metal elements such as Pb, Zn, and Cd in the work area is extremely uneven.
The work area is located in the Maiziping area of Mabian, Sichuan. The mining activities are highly developed, mainly concentrated in Maiziping Village and Heizu in Yonghong Township, Mabian County. The main mining species are phosphorus, lead and zinc ore, etc. Heavy metals such as Pb, As, Zn, Cd, Cu and high values of P are all located in the above areas.
The water samples collected this time were contained in pure water bottles. The heavy metals of the water samples were tested at the Testing Center of the Institute of Comprehensive Utilization of Mineral Resources of the Chinese Academy of Geological Sciences, and the analysis results of 8 heavy metal elements were obtained (Table 10). According to the GB3838-2002 water area function and standard classification, according to the environmental functions and protection targets of surface water areas, they are divided into Class I-V according to the function level. 48 surface water samples were collected in the Mabian Laoheba work area, and the statistical table of the heavy metal analysis results of the water body is as follows.
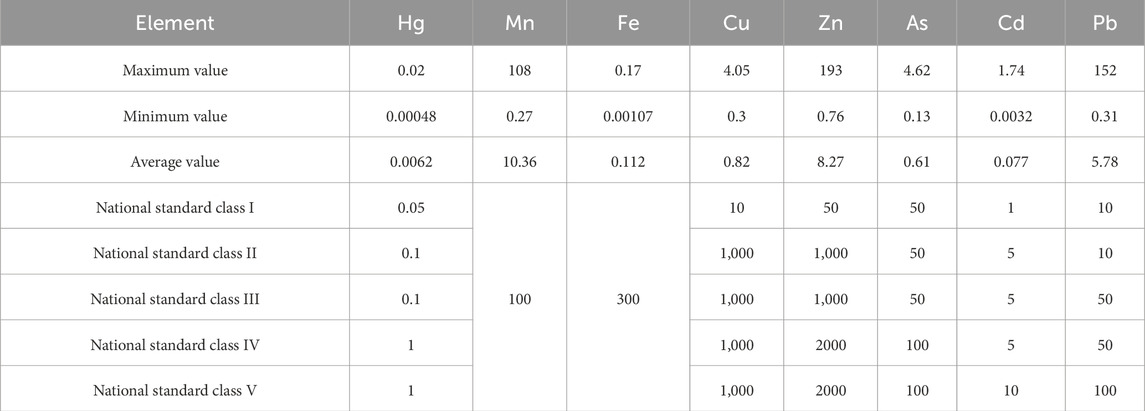
Table 10. Statistical table of water element analysis results in the Mabian Maiziping work area (μg/L).
As can be seen from Table 10, the two elements Fe and Mn are not classified, which are the standard limits of the supplementary project for surface water sources. The overall content of heavy metals in the mines of the Maiziping working area in Mabian is not high. Some elements have a large range of variation in water. The detection rate of each element is 100%, indicating that the content of each element in the water body is relatively low. Hg, Fe, Cu, and As all meet the Class I standard; most of the Mn, Pb, Zn, and Cd elements meet the Class I standard, and only a few slightly exceeded the standard in the sheep shed (1) in the northern section of Maiziping. From the analysis results of the heavy metal content in the water body, the heavy metal pollution level in the Maiziping working area is relatively low. The distribution characteristics of heavy metals in the Maiziping work area reveal the potential impact of mining activities on water quality. Although most heavy metal concentrations are within the national standard range, local exceedances are worth paying attention to. The pollution prevention and control measures in mining areas need to be further strengthened, especially in the management of heavy metal containing waste, to prevent highly variable heavy metals from entering water bodies through surface runoff. This result provides important reference for local governments and mining area managers to formulate environmental management policies, and suggests implementing stricter waste management and environmental monitoring mechanisms to reduce the negative impact of mining activities on the water environment.
After a comprehensive analysis of the heavy metal characteristics in the water bodies of the Laoheba working area, Liugushui working area, and Maiziping working area, it can be clearly seen that although the water bodies in the three regions generally show lower levels of heavy metal pollution, there are significant differences in pollution distribution and potential ecological risks. Due to mining activities and poor waste management, the Laoheba work area has experienced scattered heavy metal pollution, especially with prominent levels of Cu and Cd pollution. The distribution of heavy metals in the Liugushui working area is relatively uniform, but the pollution levels of As and Pb are relatively high, indicating long-term environmental risks. The Maiziping work area exhibits uneven distribution of Pb, Zn, and Cd, and the potential ecological hazards of Mn and As are significant. These differences reflect the intensity of mining activities, environmental management measures, and the impact of geological conditions on heavy metal pollution in water bodies in different work areas, emphasizing the importance of targeted environmental policies and pollution control measures. Overall, although the potential ecological hazards of heavy metals in current water bodies are not high, continuous monitoring and effective environmental management are crucial for mitigating the impact of future phosphate mining on the water environment.
3.3 Total phosphorus pollution in surface water
The rapid urbanization and mining development in the region have caused pollution of nutrients such as nitrogen and phosphorus in rivers and potential eutrophication of water bodies. It is of great significance to identify the characteristics of nitrogen and phosphorus pollution in regional watershed water bodies and their evolution trends. The project selected the Shanshuigou Basin in the Laoheba area of Mabian, the Xinihe Basin in Erbawan, and the Haroluo River Basin as research objects. Sample points were set up in the basin according to spatial distribution, land use type, human activity intensity, etc. Water samples were collected in June 2021 to study the spatiotemporal distribution characteristics of total phosphorus pollution in the basin water bodies, analyze its pollution sources, and evaluate its eutrophication level.
3.3.1 Laoheba working area
The results showed that the total phosphorus index of the water bodies in the small watersheds of the Mabian Laoheba work area was relatively low this year, with a total phosphorus concentration of 0.0008–52.5 mg/L, an average of 0.94 mg/L (Figure 5). There was one copper factory embankment (1.01 mg/L) and two storm plains (1.15 mg/L, 2.20 mg/L), slightly exceeding the standard. The maximum value in the watershed was in Shanshui Gou (52.5 mg/L), which is within the scope of Zhongyi Mining and has been suspended. After investigation, it was found that there were slag piles above the mining site that were washed into the river by rainwater, and only three other places slightly exceeded the standard (the average value of these three places was 1.45 mg/L). It is caused by the collection and erosion during the rainy season in the upstream slag yard and waste dump. The maximum allowable discharge concentration of total phosphorus shall refer to GB18918-2002 “Pollutant Discharge Standards for Urban Sewage Treatment Plants.” The standard can be as shown in Table 11. There are 66 samples that meet the first level standard, accounting for 94.29%, 3 samples that meet the second level standard, accounting for 4.29%, and 1 sample that exceeds the third level standard, accounting for 1.43% (Figure 4). From a small watershed perspective, the average total phosphorus in the Harroro River Basin is 0.232 mg/L, the West Ni River Basin in Erba Bay is 0.189 mg/L, and the Shanshuigou Basin is 0.10 mg/L (excluding the maximum value of 52.5 mg/L).

Table 12. Statistics of pollution degree and potential ecological hazard of each soil sampling point in the Laoheba working area.
Whether it is heavy metal elements or total phosphorus indicators, the working area shows point pollution. Heavy metal elements such as Zn and Pb are only slightly exceeded in the Shanshuigou and Tongchanggang basins. The maximum value of total phosphorus pollution overlaps with the excessive heavy metals in Shanshuigou. Combined with the field sampling situation, the reasons for point source total phosphorus pollution are:
1. Comparison shows that the water samples in the flood season are rich in water and have good fluidity. Compared with the total phosphorus of samples collected in the Laoheba area in 2020, the average total phosphorus is 0.053 mg/L. The collection density in 2021 is higher, with an average of 0.94 mg/L, indicating that the total phosphorus pollution of water samples in the flood season is relatively small; the water volume is small and the fluidity is poor in the dry season. The total phosphorus of water samples collected in the Mabian Laoheba working area in 2020 is 8.315 mg/L on average; indicating that the total phosphorus concentration in the water body varies greatly with the seasons.
2. Total phosphorus exceeding the standard is mainly concentrated in mining areas, which are related to industrial site construction, tailings and gangue slag dumps, spoil dumps, tailings ponds, irregular stacking, collapse, domestic and industrial sewage, etc.
3. The collapse caused by geological disasters within the scope of mining development, the irregular stacking of slag and gangue piles, or the collapse of retaining walls without timely repair, leading to river erosion, is one of the reasons for the excessive total phosphorus.
3.3.2 Liugushui working area
According to the analysis and test results, the total phosphorus index of the water bodies in the small watersheds of the Liugushui working area in Mabian was relatively low this year, with a total phosphorus concentration of 0.0116–0.14 mg/L and an average of 0.0388 mg/L, which did not exceed the standard. Water samples from the lower reaches of the Xuekoushan River were collected from the lower reaches of the basin, which flows through Xuekoushan Township and the sand and gravel factory. The results showed that the total phosphorus concentration in the lower reaches of the Xuekoushan River was 0.108–0.94 mg/L, with an average of 0.260 mg/L. The total phosphorus concentration increased relative to the upstream mining area, indicating that the total phosphorus concentration is not only affected by mining activities, but also by domestic sewage and other production activities. The maximum allowable emission mass concentration of total phosphorus refers to GB18918-2002 “Pollutant Discharge Standards for Urban Sewage Treatment Plants,” and the Xuekoushan River Basin has reached the first-level standard.
3.3.3 Maiziping work area
According to the sampling and analysis test results, the total phosphorus index of the water bodies in the small watersheds of Maiziping work area in Mabian is relatively low this year, and there is no total phosphorus exceeding the standard in the region. The total phosphorus concentration is 0.01–0.51 mg/L, with an average of 0.04 mg/L. From the perspective of small watersheds, the average total phosphorus value of Yinhe River is 0.04 mg/L, the average total phosphorus value of Jinhe River is 0.043 mg/L, and the total phosphorus concentration of Dayuanzi River Daheba-Meiziwan section is 0.01–0.20 mg/L, with an average total phosphorus value of 0.067 mg/L. The maximum allowable emission mass concentration of total phosphorus refers to GB18918-2002 “Pollutant Emission Standards for Urban Wastewater Treatment Plants.” The standards are shown in Table 11, and all samples exceed the third-level standard.
3.4 Assessment of potential ecological hazards in water bodies
3.4.1 Laoheba working area
Based on Table 12 and Figure 6, it can be seen that the pollution degree of each heavy metal in the water body of the Mabian Laoheba working area is generally: Cu > Cd > Zn > Mn=Hg > Pb=As.
It can be seen from Figure 7 that the potential ecological hazard of each heavy metal in the water body of the Mabian Laoheba working area is not high, mainly with slight and moderate ecological hazards, and a small amount of strong ecological hazards. In comparison, the hazard levels of Cd and As are slightly stronger, with 2 strong ecological hazards.
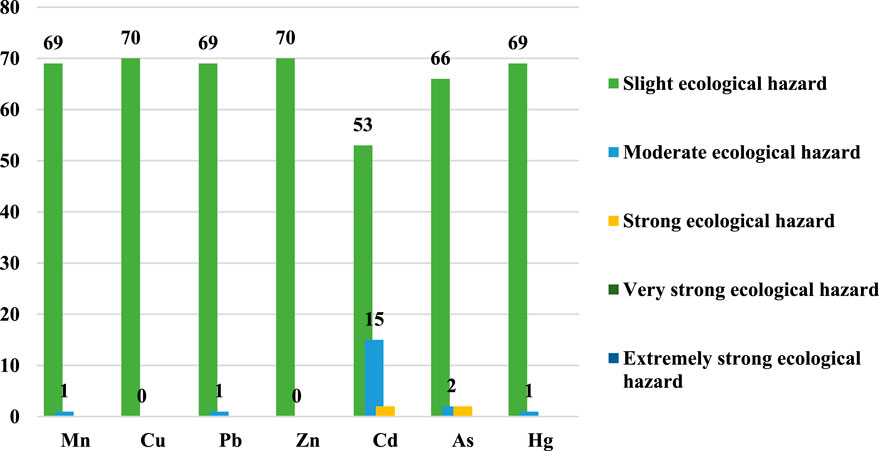
Figure 7. Potential ecological hazards of heavy metals in water bodies in the Mabian Laoheba working area.
Table 14 shows the pollution degree and potential ecological hazard degree of each sampling point in the water body of the Mabian Laoheba working area. It can be seen from the table that the pollution degree of water bodies at the sampling points in the Mabian Laoheba working area is mainly light pollution, accounting for 80%, and the potential ecological hazard is mainly slight ecological hazard, accounting for 97.14%.
3.4.2 Liugushui working area
From Figure 8, it can be seen that the pollution degree of each heavy metal in the water body of Mabian Liugushui working area is generally: As > Pb > Cd > Mn > Zn > Cu=Hg.
It can be seen from Figure 9 that the potential ecological hazard of each heavy metal in the water body of Mabian Liugushui working area is not high, mainly with slight and moderate ecological hazards, and a small amount of strong ecological hazards. In comparison, As has 4 very strong ecological hazards and 3 strong ecological hazards, and the hazard level is slightly higher.
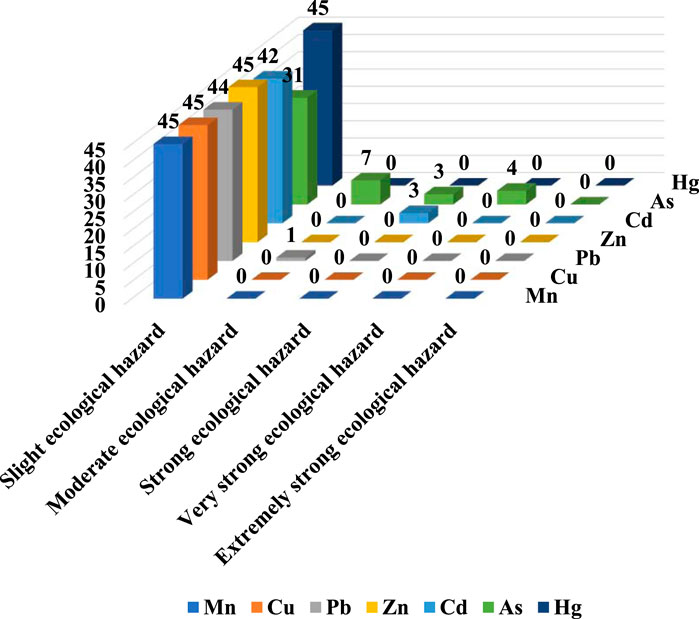
Figure 9. Potential ecological hazards of heavy metals in water bodies in the Liugushui working area in Mabian.
Table 13 shows the pollution degree and potential ecological hazard degree of each sampling point in the water body of the Liugushui working area in Mabian. It can be seen from the table that the pollution degree of water bodies at the sampling points in the Liugushui working area in Mabian is mainly light pollution, accounting for 82.22%, and the potential ecological hazard is mainly slight ecological hazard, accounting for 86.67%.

Table 13. Statistics of pollution degree and potential ecological hazard of each soil sampling point in the Liugushui working area.
3.4.3 Maiziping work area
From Figure 10, it can be seen that the pollution degree of each heavy metal in the water of the Maiziping work area in Mabian is generally: Mn > As > Pb > Zn > Cd > Cu=Hg.
From Figure 11, it can be seen that the potential ecological hazard level of each heavy metal in the water body of the Maiziping work area in Mabian is not high, mainly with slight and moderate ecological hazards, and a small amount of strong ecological hazards. In comparison, Cd has 1 extremely strong ecological hazard.
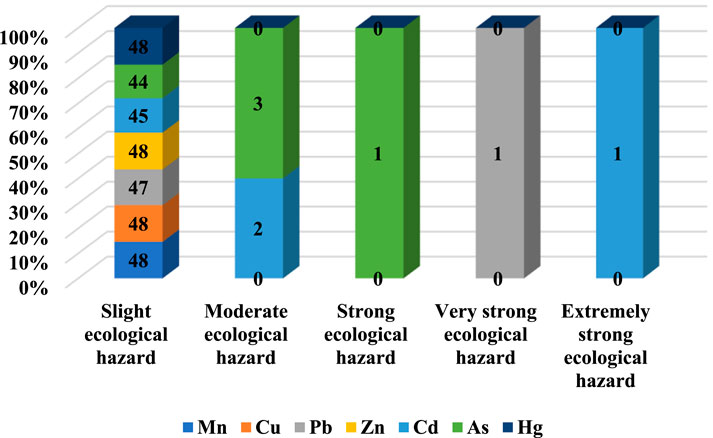
Figure 11. Potential ecological hazards of heavy metals in water bodies in the Maiziping work area in Mabian.
Table 14 shows the pollution degree and potential ecological hazard degree of each sampling point in the water body of the Maiziping work area in Mabian. It can be seen from the table that the pollution degree of water bodies at the sampling points in the Maiziping work area in Mabian is mainly light pollution, accounting for 91.67%, and the potential ecological hazard is mainly slight ecological hazard, accounting for 97.92%.

Table 14. Statistics of pollution degree and potential ecological hazard of soil sampling points in the Maiziping work area.
After a comprehensive analysis of the water conditions in the three working areas of Mabian Laoheba, Liugushui, and Maiziping, covering the results of water analysis, heavy metal characteristics, total phosphorus pollution in surface water, and potential ecological hazard assessment of water bodies, the following patterns can be clearly observed: the role of mining activities in the water environment presents multidimensional characteristics and significant spatial differences in different regions. The Laoheba work area exhibits a high level of heavy metal pollution and total phosphorus levels, which may be related to the intensive mining activities and waste management measures in the area. Although the Liugushui work area has also been affected by mining activities, its water quality is relatively good, which may be attributed to more standardized mining management and environmental monitoring mechanisms. The Maiziping work area exhibits an uneven distribution of heavy metal pollution, which may be closely related to the specific geological conditions and mining activity patterns in the area. The above findings highlight the complexity of the impact of mining activities on water environments and indicate that different regions have varying needs for environmental management strategies. This research not only provides an empirical basis for a deeper understanding of the impact of mining activities on water environments, but also provides a scientific basis for developing environmental management measures that are tailored to regional characteristics. Through comprehensive analysis of water bodies, heavy metal pollution, total phosphorus pollution, and ecological hazard assessment, the impact of mining activities on the water environment can be more comprehensively evaluated, providing theoretical support for achieving a balance between mining development and environmental protection.
4 Conclusion
This study takes the development of phosphate mines in the Mabian Jinyang area of Sichuan Province as a case study, systematically exploring the pollution of heavy metals and total phosphorus in water bodies in mining areas and their potential ecological hazards. The research results indicate that the overall water quality of the water bodies in the three working areas of Mabian Laoheba, Liugushui, and Maiziping is Class I water, and the water type is mainly Ca-HCO3 type, which is consistent with the research results of (Wu et al., 2014). Our research results provide additional evidence for understanding the universality of the impact of phosphate mining on the water environment. However, there are significant differences in the distribution and pollution levels of heavy metals and total phosphorus in the three work areas, reflecting the complex impact of phosphate mining development on the surrounding environment. In the Mabian Laoheba work area, the distribution of P element and heavy metal elements such as Cu, Pb, Zn is extremely uneven, manifested as obvious point pollution. Our results provide a scientific basis for developing region specific environmental protection strategies. Related studies have shown that mining activities in similar areas often lead to the accumulation of heavy metals in water bodies, especially near mining areas (Jiao et al., 2023; Liu et al., 2021; Song et al., 2021). Especially Cu and Cd, their pollution levels are the most prominent, but overall, the potential ecological hazards of heavy metals in water bodies are mainly mild and moderate, with only a few areas showing strong ecological hazards. The formation of point pollution may be related to non-standard waste disposal and slag stacking in mining areas, which also provides strong evidence for excessive total phosphorus. However, the ecological risk of heavy metals in the water body of the Laoheba working area is relatively controllable, indicating that with appropriate environmental management measures, the environmental risk in the area can be effectively alleviated. In contrast, the distribution of heavy metal elements and P elements in the Liugushui working area is relatively uniform, and the total phosphorus index of the water in this area is low, with no exceedance observed, indicating that the water environment quality in this working area is currently good. However, it should be noted that although the overall risk of heavy metal pollution is not high, elements such as As and Pb may still have certain long-term impacts on ecosystems, and continuous monitoring and corresponding preventive measures need to be taken. Our research results are consistent with previous studies in terms of heavy metal distribution patterns, which not only validates their findings on the impact of mining methods and mineral types on heavy metal distribution in water bodies, but also further reveals how these factors specifically affect aquatic ecosystems in different mining environments (Pei, 2022; Ma et al., 2023). In the Maiziping work area, the distribution of heavy metal elements such as Pb, Zn, Cd also shows extremely uneven characteristics, and the pollution levels of Mn, As, and Pb are more prominent. According to relevant literature, high concentrations of Mn may lead to degradation of aquatic ecosystems and affect the survival of aquatic organisms (Xie et al., 2022). Similar to other work areas, the total phosphorus index in the water of Maiziping is relatively low and has not exceeded the standard, but the potential ecological hazards of heavy metal pollution are mainly mild and moderate. However, there are still strong ecological hazards in some areas, especially caused by Mn and As, which reminds us to pay special attention to the management and control of these heavy metals in the development of phosphate mines in this region. In addition, in this study, we conducted a preliminary analysis of the potential correlation between pH value and heavy metal concentration. Although no uniform correlation pattern was observed between different work areas, our analysis shows that in certain regions, there is a certain correlation between changes in pH values and specific heavy metal concentrations. This may be due to the significant impact of pH on the solubility and form of heavy metals, which in turn affects their migration and bioavailability in water bodies. However, this correlation is influenced by various factors such as geological conditions, mining development activities, and waste disposal methods. Therefore, we suggest that future research can delve deeper into the interactions between these environmental factors and how they collectively affect the dynamics of heavy metal pollution in water bodies.
This study reveals the spatial distribution characteristics and potential ecological risks of heavy metal and total phosphorus pollution in water bodies during phosphate mining development through detailed analysis of three working areas. We found that the point pollution of heavy metals is closely related to the intensity of mining activities and poor waste management, while the differences in pollution levels between different work areas are related to the mining methods and scale within the region. Although the potential ecological hazards of heavy metals in the water bodies of the current work area are not high, in the long run, continuous mining development may exacerbate these risks. This study not only provides scientific basis for environmental management in phosphate mining development, but also provides important guidance for managers in ecological protection and heavy metal pollution control in mining areas. Firstly, research has shown that poor mining activities and waste management are the main causes of heavy metal pollution in water bodies. This requires mining area managers to develop and implement stricter waste treatment and management policies in future mining development, especially for controlling high-risk heavy metal elements such as Cu, Pb, Zn, As, and Cd. Secondly, considering the point like pollution characteristics of some water bodies in the work area, it is recommended to strengthen environmental monitoring in these areas and establish a long-term and dynamic monitoring mechanism to timely detect and control potential pollution sources. In addition, in the future, efforts should be made to strengthen the protection of communities and ecosystems around mining areas, in order to avoid ecological imbalances and environmental degradation caused by mining development.
The limitations of this study mainly lie in the selection of data collection and analysis methods. Firstly, the collection of water samples is limited to three working areas and may not fully represent the overall water pollution situation in the Mabian Jinyang area. Therefore, there may be regional biases in the research results, and it is recommended to expand the sampling range and cover more work areas in future studies to improve the universality and representativeness of the research. Secondly, although this study employed standardized chemical analysis methods and the Potential Ecological Risk Index (PERI) method, which are effective in assessing the potential threat of heavy metal pollution, some other environmental factors such as water mobility, seasonal variations, and the interaction of other pollution sources may still be overlooked. Future research can enhance the comprehensiveness of assessments by integrating ecotoxicological experiments and multi-media environmental models. Ecotoxicology experiments will provide direct evidence about the response of organisms to heavy metal exposure, while multi-media environmental models can help simulate the migration and transformation of heavy metals in different environmental media, providing a more comprehensive perspective for risk assessment. Finally, due to time constraints in data collection, this study was unable to consider the changing trends of long-term monitoring data. Subsequent research will incorporate long-term data analysis to gain a deeper understanding of the sustained impact of phosphate mining development on the water environment.
Data availability statement
The raw data supporting the conclusions of this article will be made available by the authors, without undue reservation.
Author contributions
YT: Writing–original draft. ZC: Writing–orginal draft. QZ: Writing–review and editing. JL: Writing–review and editing. RW: Writing–review and editing. AQ: Writing–review and editing. QL: Writing–original draft.
Funding
The author(s) declare that financial support was received for the research, authorship, and/or publication of this article. This study was funded by the National Natural Science Foundation of China (72074199 and 71991483), China Geological Survey (DD20221795) and the geological exploration project funded by Sichuan government General Survey of Dayuanzi Phosphorate in Mabian Yi Autonomous County, Leshan City, Sichuan Province (DZ202401) and integration of research on Kunyang-type Phosphorate technology in Sichuan section of the western margin of the Yangtze Platform (207 Geological Team, Sichuan Bureau of Exploration and Development of Geology and Mineral Resources, the number 2023-1).
Conflict of interest
Author AQ was employed by China Aerospace Planning and Design Group Co., Ltd.
The remaining authors declare that the research was conducted in the absence of any commercial or financial relationships that could be construed as a potential conflict of interest.
Publisher’s note
All claims expressed in this article are solely those of the authors and do not necessarily represent those of their affiliated organizations, or those of the publisher, the editors and the reviewers. Any product that may be evaluated in this article, or claim that may be made by its manufacturer, is not guaranteed or endorsed by the publisher.
References
Al-Hwaiti, M. S., Brumsack, H. J., and Schnetger, B. (2018). Heavy metal contamination and health risk assessment in waste mine water dewatering using phosphate beneficiation processes in Jordan. Environ. Earth Sci. 77, 661. doi:10.1007/s12665-018-7845-0
Cao, Y., Wang, R., Liu, Y., Li, Y., Jia, L., Yang, Q., et al. (2023). Improved calculations of heavy metal toxicity coefficients for evaluating potential ecological risk in sediments based on seven major Chinese water systems. Toxics 11, 650. doi:10.3390/toxics11080650
Chen, L., Li, R., Zhang, J., Cai, W., Zhang, K., and Sun, Y. (2024a). Equilibrating provincial carbon increments for residential buildings in China under carbon peaking constraints. Environ. Impact Assess. Rev. 105, 107385. doi:10.1016/j.eiar.2023.107385
Chen, L., Liu, S., Cai, W., Chen, R., Zhang, J., and Yu, Y. (2024c). Carbon inequality in residential buildings: evidence from 321 Chinese cities. Environ. Impact Assess. Rev. 105, 107402. doi:10.1016/j.eiar.2023.107402
Chen, L., Liu, S., Cai, W., Li, Y., Lv, G., and Peng, S. (2023). Retrospective carbon reduction potential of residential buildings in China based on equity and efficiency coupling. Build. Environ. 242, 110588. doi:10.1016/j.buildenv.2023.110588
Chen, L., Tan, Y., Lv, G., Cai, W., Gao, X., and Li, R. (2024b). Uncovering the coupling effect with energy-related carbon emissions and human development variety in Chinese provinces. J. Environ. Sci. 139, 527–542. doi:10.1016/j.jes.2023.05.046
Chen, L., You, K., Lv, G., Cai, W., Zhang, J., and Zhang, Y. (2024d). The influence of demographic structure on residential buildings’ carbon emissions in China. J. Build. Eng. 87, 108951. doi:10.1016/j.jobe.2024.108951
Diahou, R. R. C. M., Dallou, G. B., Sondzo, J. S., Bounouira, H., Maazouzi, Y., El Moursli, R. C., et al. (2023). Environmental and human health risk assessments for potentially toxic elements in the soils of a prospective phosphate mining area of hinda district, republic of the Congo. J. Radioanal. Nucl. Chem. 332, 3587–3595. doi:10.1007/s10967-023-09045-6
Fan, C., Liu, Y., Liu, C., Zhao, W., Hao, N., Guo, W., et al. (2022). Water quality characteristics, sources, and assessment of surface water in an industrial mining city, southwest of China. Environ. Monit. Assess. 194, 259. doi:10.1007/s10661-022-09908-7
Filho, P. P., da, C. A., Pereira, W.V. da S., Dias, Y. N., de Moraes, A. L. F., Rodrigues, F. H. S., et al. (2024). Artisanal mining of monazite and cassiterite in the amazon: potential risks of rare earth elements for the environment and human health. Environ. Manage. 73, 1201–1214. doi:10.1007/s00267-024-01964-8
Geissler, B., Mew, M. C., and Steiner, G. (2019). Phosphate supply security for importing countries: developments and the current situation. Sci. Total Environ. 677, 511–523. doi:10.1016/j.scitotenv.2019.04.356
Hayder, R., Hafeez, M., Ahmad, P., Memon, N., Khandaker, M. U., Elqahtani, Z. M., et al. (2023). Heavy metal estimation and quality assurance parameters for water resources in the northern region of Pakistan. Water 15, 77. doi:10.3390/w15010077
Jiao, Y., Liu, Y., Wang, W., Li, Y., Chang, W., Zhou, A., et al. (2023). Heavy metal distribution characteristics, water quality evaluation, and health risk evaluation of surface water in abandoned multi-year pyrite mine area. Water 15, 3138. doi:10.3390/w15173138
Khelifi, F., Caporale, A. G., Hamed, Y., and Adamo, P. (2021). Bioaccessibility of potentially toxic metals in soil, sediments and tailings from a north africa phosphate-mining area: insight into human health risk assessment. J. Environ. Manage. 279, 111634. doi:10.1016/j.jenvman.2020.111634
Li, S., Yuan, Z., Bi, J., and Wu, H. (2010). Anthropogenic phosphorus flow analysis of hefei city, China. Sci. Total Environ. 408, 5715–5722. doi:10.1016/j.scitotenv.2010.08.052
Li, Z., Qiu, Y., Zhao, D., Li, J., Li, G., Jia, H., et al. (2023). Application of apatite particles for remediation of contaminated soil and groundwater: a review and perspectives. Sci. Total Environ. 904, 166918. doi:10.1016/j.scitotenv.2023.166918
Liu, Y., Wang, P., Gojenko, B., Yu, J., Wei, L., Luo, D., et al. (2021). A review of water pollution arising from agriculture and mining activities in central asia: facts, causes and effects. Environ. Pollut. 291, 118209. doi:10.1016/j.envpol.2021.118209
Luo, D., Wang, L., Nan, H., Cao, Y., Wang, H., Kumar, T. V., et al. (2023). Phosphorus adsorption by functionalized biochar: a review. Environ. Chem. Lett. 21, 497–524. doi:10.1007/s10311-022-01519-5
Ma, H., Zhang, Y., Liu, Z., Chen, Y., and Lv, G. (2023). Pollution characteristics of heavy metals in surface sediments of the shuimo river in urumqi, China. Metals 13, 1578. doi:10.3390/met13091578
Ma, S., Hu, S., Chen, D., and Feng, Y. (2015). “A study of the sustainable development of China’s phosphorus resources industry based on system dynamics,” in Proceedings of the 12TH international symposium on process systems engineering and 25TH EUROPEAN symposium on computer aided process engineering. Editors K. V. Gernaey, J. K. Huusom, and R. Gani (Amsterdam: Elsevier Science Bv), 37, 2051–2056. doi:10.1016/b978-0-444-63576-1.50036-4
Othman, I., and Al-Masri, M. S. (2007). Impact of phosphate industry on the environment: a case study. Appl. Radiat. Isot. 65, 131–141. doi:10.1016/j.apradiso.2006.06.014
Pei, L. (2022). Features of metallic ion distribution in non-traditional water agricultural applications in sandy loam in an arid area. Sustainability 14, 11080. doi:10.3390/su141711080
Song, Z., Song, G., Tang, W., Yan, D., Zhao, Y., Zhu, Y., et al. (2021). Molybdenum contamination dispersion from mining site to a reservoir. Ecotox. Environ. Safe. 208, 111631. doi:10.1016/j.ecoenv.2020.111631
Tummala, C. M., Dardona, M., Praneeth, S., Mohanty, S. K., and Dittrich, T. M. (2024). Iron-coated nutshell waste bioadsorbents: synthesis, phosphate remediation, and subsequent fertilizer application. Environ. Res. 240, 117468. doi:10.1016/j.envres.2023.117468
Wang, N., Luo, Y., Liu, Z., and Sun, Y. (2022b). Spatial distribution characteristics and evaluation of soil pollution in coal mine areas in loess plateau of northern shaanxi. Sci. Rep. 12, 16440. doi:10.1038/s41598-022-20865-6
Wang, Y., Kuntke, P., Saakes, M., van der Weijden, R. D., Buisman, C. J. N., and Lei, Y. (2022a). Electrochemically mediated precipitation of phosphate minerals for phosphorus removal and recovery: progress and perspective. Water Res. 209, 117891. doi:10.1016/j.watres.2021.117891
Wu, J., Li, P., Qian, H., Duan, Z., and Zhang, X. (2014). Using correlation and multivariate statistical analysis to identify hydrogeochemical processes affecting the major ion chemistry of waters: a case study in Laoheba phosphorite mine in sichuan, China. Arab. J. Geosci. 7, 3973–3982. doi:10.1007/s12517-013-1057-4
Xie, S., Yu, C., Peng, B., Xiao, H., Zhang, W., Zhou, Z., et al. (2022). A Re-assessment of metal pollution in the dexing mining area in jiangxi Province, China: current status, hydro-geochemical controls, and effectiveness of remediation practices. Int. J. Environ. Sci. Technol. 19, 10707–10722. doi:10.1007/s13762-021-03887-x
Zhang, F., Liu, H., Ma, Y., Li, Y., Tie, C., and Zhao, Q. (2023). Effects of dissolved organic matter on the release of soluble phosphorus and fluoride ion from phosphate ore. Separations 10, 425. doi:10.3390/separations10080425
Zhang, J., and Xu, X. (2023). Spatial distribution characteristics and potential risk assessment of heavy metals in sludge of Shanghai sewage treatment plant: a case study. Sustainability 15, 3465. doi:10.3390/su15043465
Keywords: chemical analysis methods, ecological risk assessment, distribution of heavy metals, sustainable mining, environmental management strategy, identification of pollution sources, environmental impact of phosphate ore
Citation: Tian Y, Chen Z, Zhang Q, Lu J, Wang R, Qin A and Liu Q (2025) Surface water pollution caused by phosphate mining development in Mabian Jinyang area, Sichuan Province in 2021: chemical analysis and potential ecological risk index assessment. Front. Earth Sci. 13:1487950. doi: 10.3389/feart.2025.1487950
Received: 29 August 2024; Accepted: 28 January 2025;
Published: 30 April 2025.
Edited by:
Amita Somya, Amity University Bengaluru, IndiaReviewed by:
Diego De Souza Sardinha, Federal University of Alfenas, BrazilMorufu Olalekan Raimi, Federal University, Otuoke, Nigeria
Ebenezer Aquisman Asare, Ghana Atomic Energy Commission, Ghana
Zhongwen Xu, Sichuan University, China
Liu Chen, Peking University, China
Copyright © 2025 Tian, Chen, Zhang, Lu, Wang, Qin and Liu. This is an open-access article distributed under the terms of the Creative Commons Attribution License (CC BY). The use, distribution or reproduction in other forums is permitted, provided the original author(s) and the copyright owner(s) are credited and that the original publication in this journal is cited, in accordance with accepted academic practice. No use, distribution or reproduction is permitted which does not comply with these terms.
*Correspondence: Qunyi Liu, bHF5Y3N1dEAxNjMuY29t; Zhen Chen, Y2hlbnpoZW4yMDIzMTZAMTYzLmNvbQ==