- 1Sichuan Institute of Land Science and Technology (Sichuan Satellite Application Technology Center), Chengdu, China
- 2The 7th Geological Brigade of Sichuan, Leshan, China
- 3Chengdu Geological Survey Center of the China Geological Survey (Southwest Geological Science and Technology Innovation Center), Chengdu, China
- 4College of Earth and Planetary Sciences, Chengdu University of Technology, Chengdu, China
The Emeishan Large Igneous Province (ELIP) in southwestern China, formed by the Emeishan mantle plume, hosts diverse mineralization systems. This study classifies deposits into two types: those directly and indirectly related to the plume. Directly related deposits (∼260 Ma) include magmatic Fe-Ti-V oxide and Cu-Ni-PGE sulfide deposits, and hydrothermal Nb-Ta-Zr-REE deposits, concentrated in the ELIP’s inner zone. Indirectly related deposits, such as weathering crust-type REE-Sc-Ti ores and Carlin-type gold deposits, formed post-ELIP through weathering, sedimentation, and hydrothermal processes, and are distributed in the middle-outer zones. Spatiotemporal analysis reveals that directly related deposits formed synchronously with ELIP magmatism, while indirectly related deposits exhibit dispersed ages from the Late Permian to Quaternary. The former originated from plume-driven magmatism, whereas the latter were influenced by thermal effects, ancient faults, and later geological processes. The study highlighted the plume’s role in global mineralization, proposing a resource effect law where mantle plumes enhance mineral and hydrocarbon formation. Comparative research with other Large Igneous Provinces is essential to address critical mineral shortages and ensure sustainable resource development.
1 Introduction
Large igneous provinces (LIPs) are widely distributed worldwide (Zhang Q. et al., 2022). One example is the Emeishan Large Igneous Province (ELIP) in southwestern China (Zhang et al., 2024). Since Zhao Ya first named the basalt in the Emeishan area of the Sichuan Province Emeishan basalt in 1929 (Sichuan Bureau of Geology and Mineral Resources, 1991), numerous genetic studies have been conducted. By the early 21st century, mantle plume genesis had been widely accepted (He et al., 2003). The ELIP is a large mafic igneous province covering an area exceeding 2.5 × 105 km2 in southwestern China (as shown in Figure 1), and it has long been a research hotspot for scholars at home and abroad. The eruption time (Mundil et al., 2004) and distribution space (He et al., 2003; Tian Y. et al., 2021), high and low titanium basalt (Jiang et al., 2009), the relationship between layered rock mass and basalt (Zhou et al., 2005), mineralization related to basalt (Hu et al., 2005), mass extinctions (Yin and Song, 2013), and genetic links to mantle plumes have received extensive attention from scholars, and many results have been reported.
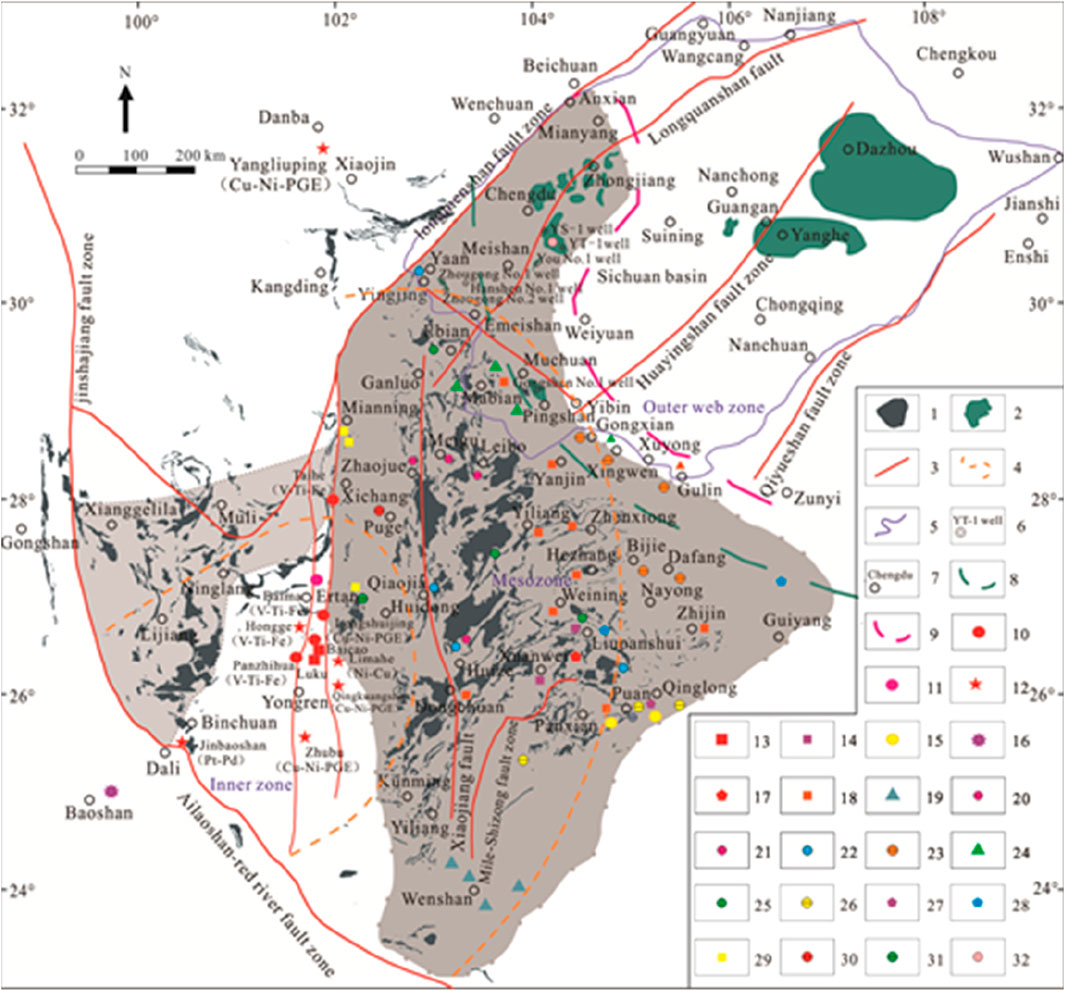
Figure 1. Map of the distribution of Emeishan basalts and deposits related to the Emeishan Mantle Plume (modified after He et al., 2003; Xu and Chung, 2001; He et al., 2008; Ma et al., 2019).
The mantle plume acts as a channel for material and energy exchange among various core, mantle, and crust layers, providing a dynamic mechanism for intraplate tectonic magmatism and mineralization (van der Zwan et al., 2023). The formation of the Emeishan Large Igneous Province (ELIP) has significant implications for the generation of heat and the supply of minerals, creating optimal conditions for mineralization. While extensive research has been conducted globally on mantle plume genesis, eruption timelines, spatial distribution, magmatic evolution, the interplay between layered rock masses and basalt, and the mass extinctions associated with the ELIP, the characterization of various mineralization types within the Emeishan mantle plume’s metallogenic system remains incomplete. The upwelling of the Emeishan mantle plume has led to both endogenous mineralization, including magmatic and hydrothermal processes, and exogenous mineralization resulting from weathering, leaching, and sedimentation in subsequent stages. Additionally, ancient deep faults, rift basins, thermal effects, and reservoir reconstructions associated with the plume exert direct and indirect influences on the concentration of metals, as well as oil and gas resources. Over the past century, considerable detail has been devoted to studying the mineralization processes linked to the Emeishan mantle plume. According to the previous study (Zhang Q. et al., 2022), and the correlation between mineralization and the ELIP, mineralization can be divided into that directly and indirectly related to the Emeishan mantle plume. In many studies, the focus was placed on the former (Zhang Z. et al., 2022; Gao et al., 2004; Luo, 2014), leading to a lack of research on the latter. The reason for this result is that the magmatic deposits and hydrothermal deposits formed during the upwelling of the Emeishan mantle plume are large in scale. These deposits play a key role in the development of the social economy, so scholars have studied them more. For deposits formed later than the ELIP, few scholars believe that they are genetically related to the Emeishan mantle plume. However, with the transformation of economy and society, deposits indirectly related to the Emeishan mantle plume have received more and more attention.
Previous literature has proved that the Emeishan mantle plume has a direct genetic relationship with magmatic deposits and hydrothermal deposits formed during the upwelling of the Emeishan mantle plume. This study on the Emeishan mantle plume metallogenic system introduces several innovations. Firstly, it proposes a novel classification of deposits into directly related (formed at ∼260 Ma, synchronous with ELIP, e.g., Fe-Ti-V oxide deposits) and indirectly related (post-ELIP, e.g., weathering crust and oil-gas deposits), addressing a previous gap and emphasizing understudied indirect deposits, revealing their long-term metallogenic effects. Secondly, using zircon U-Pb and other geochronological methods, it systematically analyzes the spatiotemporal distribution, with direct deposits concentrated in the ELIP inner zone and indirect ones dispersed in the middle-outer zones, clarifying their evolution tied to plume activity and subsequent geological processes. Thirdly, it introduces a universal resource effect law applicable to global LIPs, highlighting how mantle plumes enhance mineral and hydrocarbon formation via heat and material release, offering a framework for comparative studies. Lastly, it links regional findings to global critical mineral shortages, advocating enhanced global research for resource security. These innovations deepen the understanding of the Emeishan system and hold broader global significance.
1: Emeishan basalt; 2: concealed basalt; 3: fault zone; 4: boundary of the Emeishan Large Igneous Province; 5: Sichuan Basin boundary; 6: drilling location and name; 7: locations of interest; 8: the old boundary in the northeast of the Emeishan igneous province; 9: new boundary northeast of the Emeishan pyrodiagenetic province; 10: Fe–Ti–V magmatic oxide deposit; 11: volcanic Fe deposit; 12: Cu–Ni–PGE magmatic sulfide deposit; 13: Nb–Ta–Zr–REE deposit related to syenite dikes; 14: modern weathering crust REE–Sc–Ti polymetallic ore; 15: lateritic Au deposit; 16: modern placer type South Red Agate deposit; 17: paleo-weathering crust type Fe deposit; 18: sedimentary Nb–Sc–Ti–Ga–Zr–V–REE polymetallic deposit; 19: sedimentary bauxite deposit; 20: sedimentary conglomerate-type South Red Agate deposit; 21: sedimentary conglomerate-type South Red Agate deposit; 22: sedimentary “Madouzi type” copper deposit; 23: sedimentary pyrite deposit; 24: sand shale-type copper deposit deposited at the bottom of the Feixianguan Formation; 25: basalt copper ore; 26: Carlin-type gold deposit; 27: Dachang antimony and gold deposits, Qinglong, Guizhou; 28: hydrothermal uranium deposit; 29: carbonate syenite complex-type REE deposit in the Panxi area; 30: sedimentary Cu–Re–Mo polymetallic deposit in the Panxi ancient rift; 31: sedimentary coal–copper–rheniumvmolybdenum deposit in the Panxi ancient rift; 32: oil and gas deposits.
2 Classification of deposit types
Scholars have different classifications of deposit types related to the Emeishan mantle plume (Hu et al., 2005; Gao et al., 2004). Zhang Q. et al. (2022) proposed a simple and clear division scheme, which is consistent with the actual situation. Based on the principle of “simultaneity” of direct correlation, this study followed the classification method of Zhang Z. et al. (2022). Based on the temporal correlation between mineralization and mantle plume event, deposits were divided into those directly and indirectly related to the Emeishan mantle plume (Table 1). The foci of this study included providing information regarding the deposit types related to the Emeishan mantle plume that has not been mentioned in the past and analyzing the genesis of these deposits.
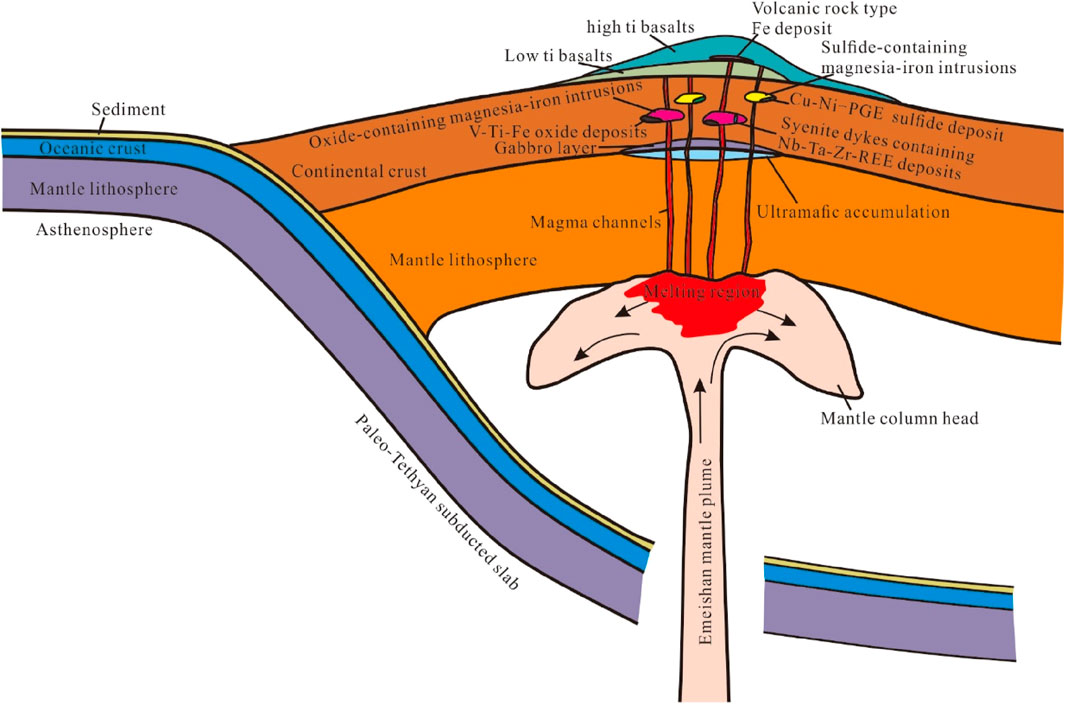
Figure 2. Evolution of an ore deposit directly related to the Emeishan mantle plume (modified after Zhang Z. et al., 2022; Bai et al., 2022).
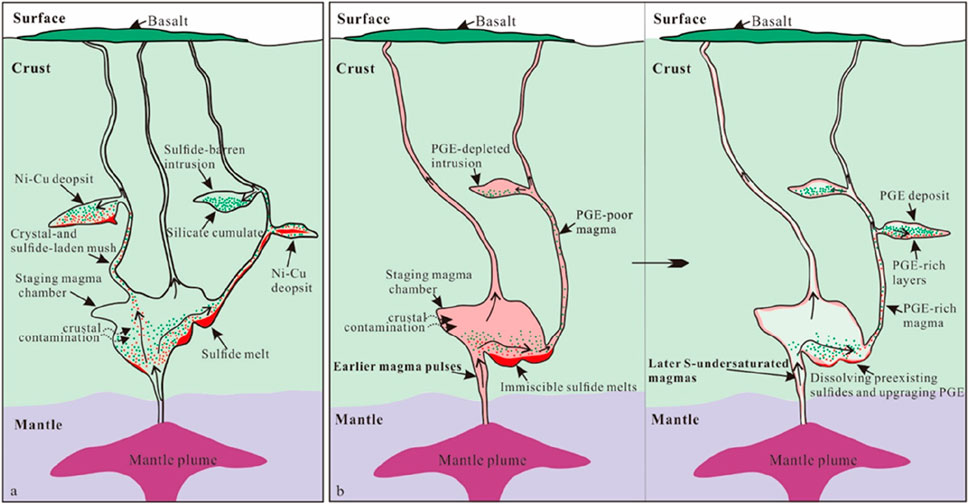
Figure 3. Evolution of Cu–Ni–PGE sulfide deposits in the Emeishan mantle plume metallogenic system (modified after Wang et al., 2018).
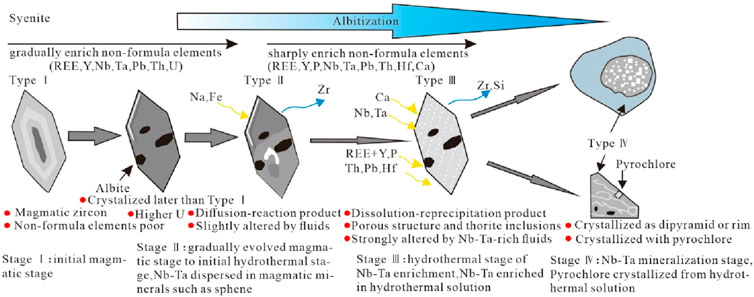
Figure 4. Formation patterns of I, II, III, and IV zircons in the four stages of Nb–Ta mineralization in the Baicao and Luku deposits (modified after Zeng and Liu, 2022).
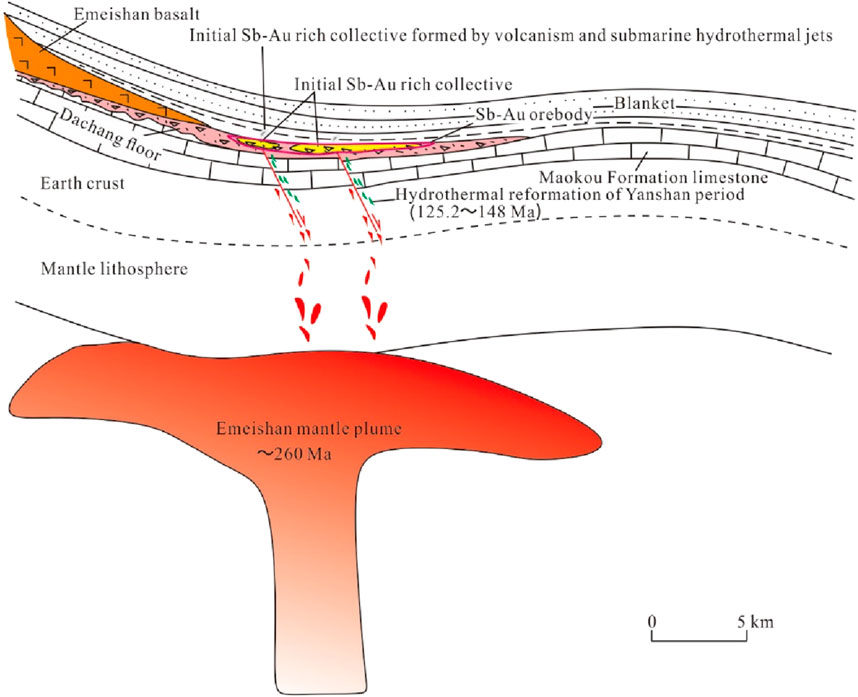
Figure 6. Metallogenic model of the Sb–Au deposit in Dachang, Qinglong, and Guizhou (modified after Du et al., 2020).
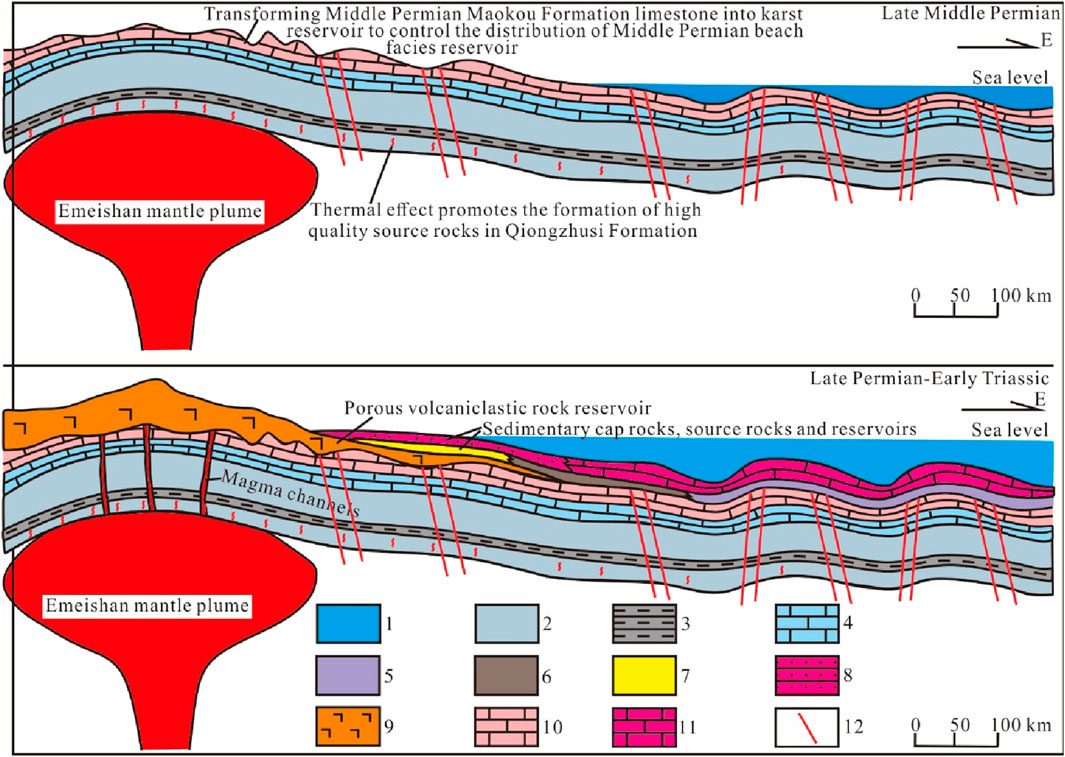
Figure 7. The influence model of the Emeishan mantle plume on oil and gas deposition (modified after Yang et al., 2007).
3 Spatiotemporal distribution of deposits
3.1 Spatiotemporal distribution of deposits directly related to the Emeishan mantle plume
The investigation of the spatiotemporal distribution of deposits is essential for elucidating the metallogenic system associated with the Emeishan mantle plume. Various scholars have employed zircon U–Pb and 40Ar/39Ar geochronological methods to study Ni–Cu–PGE sulfide deposits. As illustrated in Figure 8, the metallogenic ages of Ni–Cu–PGE sulfides, Fe–Ti–V oxides, volcanic-type Fe, and Nb–Ta–Zr–REE deposits linked to syenite veins align with the formation age of the Emeishan Large Igneous Province (ELIP) at approximately 260 Ma. This temporal congruence indicates that the mineralization of these four deposit types is closely associated with mantle plume events, establishing a direct relationship with the mantle plume dynamics.
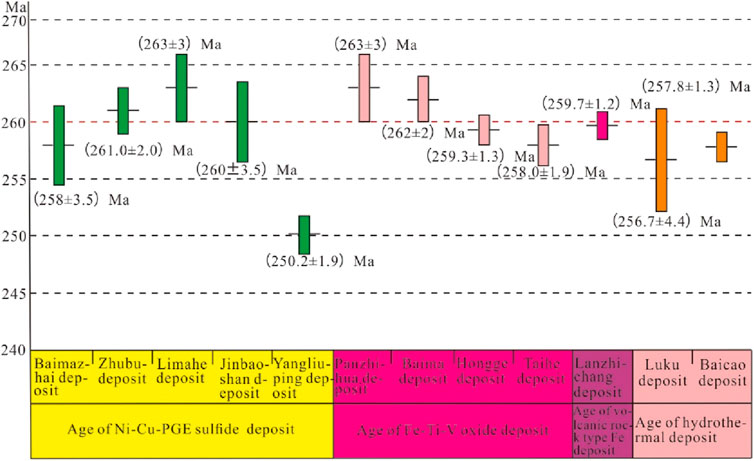
Figure 8. Metallogenic age distribution of ore deposits directly related to the Emeishan mantle plume. Note: The age of the Baimazhai deposit was obtained from Wang et al. (2005), that of Zhubu and Limahe deposits from Zhou et al. (2008), that of Jinbaoshan deposit from Tao et al. (2009), that of the Yangliuping deposit from Wang et al. (2007), that of the Panzhihua, Baima, Hongge, and Taihe deposits from Zhong et al. (2009), that of the Fe deposit in Lanzhichang from Zeng et al. (2016).
The deposits under discussion are primarily located within the inner zone of the ELIP, with a minor distribution in the middle zone along the north-south fault zone. Vertically, these deposits are situated in the middle and lower sections of the magma channel. Previous research indicates that the intrusion depth of the rock mass containing V–Ti–Fe oxide ore is greater than that of the rock mass associated with Ni–Cu–PGE sulfide ore. Notably, Nb–Ta–Zr–REE deposits linked to syenite veins typically intrude into the gabbro hosting Fe–Ti–V oxide deposits (Wang et al., 2018), suggesting similar vertical positions for these ore types. Additionally, volcanic-type Fe deposits in the Emeishan basalts are closely associated with ancient volcanic edifices and are predominantly found at the top of the magma channel, although weathering and erosion have led to their rarity in the Panxi area. Thus, ore deposits directly related to the Emeishan mantle plume arise from the diagenesis and mineralization of basaltic magma and its hydrothermal fluids across various spatial positions within the magma channel. The vertical zonation of these deposits, from the bottom to the top of the magma channel, is as follows: Fe–Ti–V oxides, Nb–Ta–Zr–REE, and Ni–Cu–PGE sulfide volcanic-type Fe deposits, as shown in Figure 9.
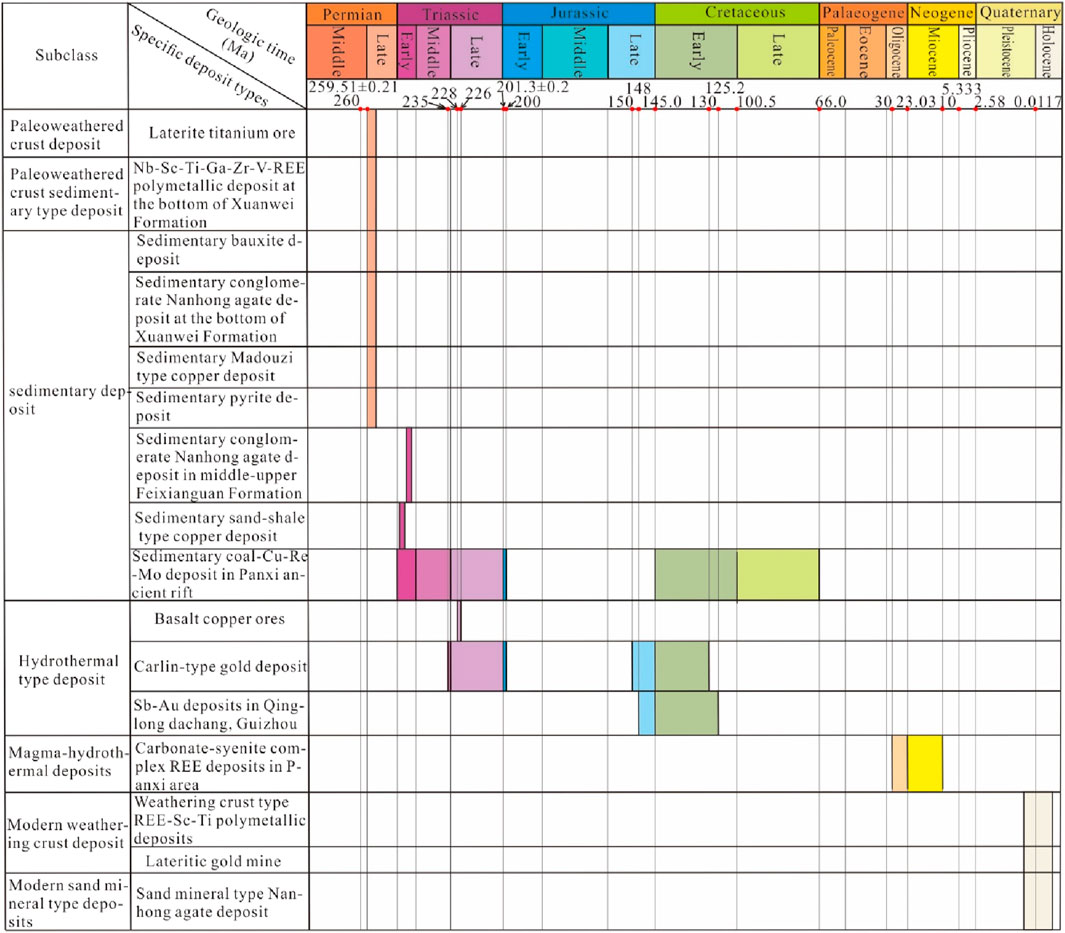
Figure 9. Mineralization age distribution of ore deposits indirectly associated with the Emeishan mantle plume.
3.2 Spatiotemporal distribution of deposits indirectly related to the Emeishan mantle plume
Deposits indirectly related to the Emeishan mantle plume are spatiotemporally dispersed. In terms of time, the ore-forming epochs of the deposits indirectly related to the Emeishan mantle plume occurred in the Late Permian, Triassic, Early Jurassic, Late Jurassic, Cretaceous, Paleogene, Neogene, and Quaternary.
The Panxi ancient rift hosts sedimentary coal, copper, rhenium, and molybdenum deposits predominantly in the rift’s inner zone, with other deposit types primarily in the middle and outer zones of the Emeishan Large Igneous Province. Modern weathering crust-type rare earth, scandium, titanium polymetallic deposits are localized within the Emeishan basalt’s upper weathering crust, while laterite-type gold deposits originate from Quaternary soils overlying Middle Permian Maokou Formation limestones. Nanhong agate sand-type deposits are found in Quaternary sediments, and Dachang, Qinglong, and Guizhou antimony and gold deposits are associated with siliceous alteration zones above Maokou Formation limestones and below Emeishan basalt. Copper deposits are localized at basalt tops, and oil and gas deposits are concentrated in high-porosity basalt interiors, karstified Maokou limestones, and post-basaltic reservoirs. Paleo-weathering crust (laterite)-type iron ores, Nb–Sc–Ti–Ga–Zr–V–REE ores, sedimentary bauxite, conglomerate-type Nanhong agate, “Madouzi”-type copper ores, and pyrite are situated at the base of Upper Permian Xuanwei, Longtan, and Wujiaping formations. Sand shale-type copper deposits are at the base of the Lower Triassic Feixianguan Formation, with some conglomerate-type Nanhong agate in the middle–upper Feixianguan Formation’s purple conglomerate layer. Carlin-type gold deposits span from Upper Permian Longtan to Lower Triassic Jialingjiang formations. Deposits linked to modern weathering and sedimentation are in Quaternary strata, those related to basalt paleo-weathering and sedimentation are in “sink area” strata sourced from Emeishan basalt, and those associated with hydrothermal processes are in Maokou Formation, Emeishan basalt, and subsequent sedimentary sequences.
3.3 Evolutionary history of the metallogenic system directly related to the Emeishan mantle plume
The evolutionary history of deposits directly related to the Emeishan mantle plume is closely related to the magmatism and hydrothermal action during its upwelling. The upwelling of the Emeishan mantle plume reached the super-thick lithosphere. Due to the upwelling of the mantle plume, a magmatic channel was formed in weak structural parts of the lithosphere. The basic–ultrabasic ore-bearing magma was positioned during the channel operation, forming Cu–Ni–PGE magmatic sulfide and V–Ti–Fe magmatic oxide deposits. In the late stage of the magmatic evolution, partial melting of the mantle plume and crust occurred when the mantle plume bottom invaded the crust, and assimilation and contamination–separation crystallization occurred in the crustal magma chamber, forming an alkaline magma, which was initially enriched in rare earth metal elements. In the late magmatic hydrothermal stage, rare earth metal elements were enriched to the greatest extent, and Nb–Ta–Zr–REE deposits related to syenite veins were formed. With further evolution of the magma, basalt magma overflowed the surface, and the volcanic rock-type Fe deposits formed near the crater.
3.4 Evolution history of the metallogenic system indirectly related to the Emeishan mantle plume
The Emeishan mantle plume’s upwelling has significantly influenced the genesis and evolution of associated mineral deposits through its thermal, channeling, and enrichment effects on ore-forming elements. This process has yielded distinct evolutionary patterns among deposit types that serve as sources, conduits, and spaces for mineralization. Modern weathering crust-type and sand-type deposits primarily originated from the weathering of Emeishan basalts during the Quaternary period. In contrast, paleo-weathering crust-type, paleo-weathering crust-sedimentary, and sedimentary-type deposits are linked to the weathering and erosion of Emeishan basalts post the main eruption phase of the ELIP. These basalts, acting as a “source area,” contributed to the formation of “sink areas” in strata such as the Xuanwei, Longtan, Wujiaping, and Feixianguan formations. The metallogenic system reflects these deposit types following the plume’s upwelling. The Emeishan mantle plume provided the material basis for the initial enrichment of ore-forming elements in hydrothermal deposits; for instance, copper in basaltic copper deposits was enriched during the late stages of Emeishan basalt magma crystallization and further mineralized through subsequent hydrothermal processes. Carlin-type gold deposits contain gold-rich cumulates formed by the Emeishan mantle plume’s magmatic event, with gold enrichment later activated by magmatic hydrothermal activity, migrating along deep faults to ore-bearing rocks. The Dachang antimony and gold deposit in Qinglong, Guizhou, resulted from volcanic activity associated with the Emeishan mantle plume, with initial enrichment of antimony and gold in the “Dachang layer,” followed by Yanshan period hydrothermal fluid superimposition and final enrichment and mineralization. The carbonate-syenite complex rock-type REE deposit in the Panxi area is associated with ancient deep faults formed by the plume’s upwelling, later activated by strike-slip faults from the India-Asia continental collision, with carbonate magma intrusion along these faults forming the deposit. Deposits providing metallogenic space include sedimentary coal-copper-rhenium-molybdenum deposits and oil and gas deposits in the Panxi paleo-rift, the former related to the paleo-rift’s formation by the Emeishan mantle plume’s upwelling, and the latter to oil and gas accumulation due to the plume’s thermal effects, including hydrocarbon generation and the formation of high-porosity volcaniclastic rock reservoirs, beach facies reservoirs, karst reservoirs, and cap rock and reservoir overlying basalt. The evolutionary history of these deposits is intricately linked to the provenance layer or initial enrichment layer of ore-forming elements, ancient deep faults, and the ore storage space provided by the formation of the ELIP.
3.5 Implications for other mantle plume metallogenic systems worldwide
The significant amount of magma generated by the upwelling of the mantle plume often forms a LIP, which is a major geological event. Regardless of a magnesian LIP or a siliceous large igneous rock, the huge amount of heat and material related to it can form rich mineral resources.
Researchers have systematically studied deposits directly related to the Emeishan mantle plume. These deposits are often distributed in the center of the mantle plume; as the magma channel is distributed in the center of the mantle plume, magmatic deposits and magmatic–hydrothermal deposits are also often located there. Moreover, the distribution of such deposits is spatiotemporally concentrated, and their distribution is extremely dispersed. In contrast, deposits indirectly related to the mantle plume are widely distributed over the entire area of the LIP; furthermore, there is a lack of research on deposits indirectly related to the Emeishan mantle plume. Thus, collaborative research should be conducted in the future to achieve more prospecting breakthroughs and new discoveries.
In mafic LIPs, the partial melting of the mantle plume forms picrite magma. When the magma rises to the crust, a magma chamber is formed in the deep part of the crust and geological processes, such as fractional crystallization, crustal contamination, and immiscibility, occur. The primitive magma in the deep mantle can supplement and mix with the magma of the magma chamber. In these processes, oxides (including chromite and vanadium–titanium magnetite deposits) and Cu–Ni–PGE sulfide deposits are formed. The formation of the above-mentioned deposits is related to the magma source area and the nature and evolution of the original magma. The formation of vanadium–titanium magnetite deposits may be related to the source area of iron-rich rocks (eclogite and pyroxenite). The source area is partially melted to form iron–titanium-rich picrite magma and then separated and crystallized to form vanadium–titanium magnetite deposits. Cu–Ni–PGE sulfide deposits occur owing to the high degree of partial melting of the mantle peridotite, which leads to the formation of low-titanium picritic magma. During the ascent of the magma channel, it mixes with the crust, resulting in the liquation of the sulfide melt. Whether it is rich in PGEs mainly depends on the degree of crustal contamination and liquation. If the mantle source area contains carbonate, partial melting forms alkali-rich magma such as kimberlite and carbonatite. Kimberlite magma may capture diamonds in the lithosphere to form diamond deposits during the ascent and carbonatite magma can form REE and Nb deposits during the evolution and later hydrothermal action. In addition, mafic–ultramafic magma partially melts in the crust to form intermediate–acidic magma, which further evolves to form a series of hydrothermal deposits. Simultaneously, huge amounts of heat can also cause the metamorphic dehydration of surrounding rocks, resulting in the formation of metamorphic fluids and associated deposits. The siliceous LIP is dominated by felsic rocks, which can only form hydrothermal deposits.
After the formation of the LIP, the source layer or initial enrichment layer of ore-forming elements, ancient deep faults (ore transport channel), and ore storage space can be further enriched, migrated, and stored to form a series of deposits under the later geological action. Ore deposits providing ore-forming sources in LIPs include modern weathering crust-type, modern sand-type, ancient weathering crust-type, sedimentary-type, and hydrothermal-type deposits. Ancient deep faults generated in the mantle plume upwelling provide ore transport channels for later mineralization. In addition, the mantle plume thermal effect improves the maturity of organic matter and promotes the formation of high-quality source rocks. The rifting effect and porous volcaniclastic rocks caused by the upwelling of the mantle plume provide ore-forming space for the formation of sedimentary and hydrocarbon deposits.
In summary, the resource effect caused by the mantle plume upwelling event is significant. Under the background of the shortage and unbalanced distribution of several key mineral resources in the world, research on the mantle plume metallogenic system should be strengthened globally. Furthermore, it is necessary to compare the metallogenic effects with other LIPs worldwide to improve the guaranteed degree of global key minerals and promote the sustainable and healthy development of the global economy.
4 Conclusion
This study systematically reviews the distribution, characteristics, and genetic research progress of deposits related to the Emeishan mantle plume, providing new insights into its metallogenic system. The key findings are as follows.
(1) The ore-forming system of the Emeishan mantle plume includes deposits directly and indirectly associated with plume activity. Directly related deposits primarily consist of magmatic and hydrothermal types, including Fe–Ti–V oxide ores, Cu–Ni–PGE sulfide ores, volcanic iron deposits, and hydrothermal Nb–Ta–Zr–REE deposits linked to syenite dykes. Indirectly related deposits encompass various mineral sources, ore-transporting channels, and metallogenic spaces, such as weathering crusts, sedimentary deposits, carbonatite–syenite complex-type REE deposits, and oil and gas reservoirs in the Panxi region.
(2) The formation ages of deposits directly linked to the Emeishan mantle plume coincide with the emplacement of the Emeishan Large Igneous Province (ELIP) and are concentrated along the north–south fault zone near magmatic conduits. In contrast, indirectly related deposits formed later, with more dispersed ages, primarily occurring in the middle and outer zones of the ELIP.
(3) The evolutionary history of directly related deposits is closely tied to magmatism and hydrothermal processes along magma conduits. Indirectly related deposits, however, are influenced by pre-existing ore-rich layers, deep-seated faults, and ore-hosting structures shaped by the ELIP, each exhibiting distinct evolutionary pathways.
(4) Mantle plume upwelling, a major geological event, generates significant magmatic activity, forming Large Igneous Provinces (LIPs) and releasing substantial heat and material. This process not only enriches mineral resources but also enhances the maturation of organic matter, contributing to the formation of high-quality source rocks and abundant oil and gas reserves. Given the global scarcity and uneven distribution of critical mineral resources, further research on mantle plume-related metallogenic systems is imperative. Comparative studies with other LIPs worldwide will enhance our understanding of their metallogenic effects, strengthening global mineral security and supporting sustainable economic development.
Data availability statement
The original contributions presented in the study are included in the article/supplementary material, further inquiries can be directed to the corresponding authors.
Author contributions
ZL: Conceptualization, Investigation, Writing – original draft. JW: Investigation, Software, Writing – original draft. WZ: Project administration, Software, Writing – original draft. MC: Investigation, Methodology, Writing – original draft. HL: Investigation, Software, Writing – original draft. MZ: Formal Analysis, Methodology, Writing – original draft. YT: Conceptualization, Investigation, Writing – original draft. YG: Resources, Visualization, Writing – original draft.
Funding
The author(s) declare that financial support was received for the research and/or publication of this article. This work was supported by the Sichuan Natural Science Foundation Project (2023NSFSC0270), the National Key Research and Development Program of China (2021YFC2901803, 2021YFC2901903), National Natural Science Foundation of China (92055314, 42272106, 42202105, 91955208), International Geosciences Programme (IGCP741), Science and Technology Project of the Sichuan Provincial Department of Natural Resources (Kj-2022-6), Sichuan provincial government investment geological exploration project (DZ202401, DZ202402).
Conflict of interest
The authors declare that the research was conducted in the absence of any commercial or financial relationships that could be construed as a potential conflict of interest.
Generative AI statement
The author(s) declare that no Generative AI was used in the creation of this manuscript.
Publisher’s note
All claims expressed in this article are solely those of the authors and do not necessarily represent those of their affiliated organizations, or those of the publisher, the editors and the reviewers. Any product that may be evaluated in this article, or claim that may be made by its manufacturer, is not guaranteed or endorsed by the publisher.
References
Bai, Z. J., Zhong, H., Hu, R. Z., Zhu, W. G., and Hu, W. J. (2019). Composition of the chilled marginal rocks of the Panzhihua layered intrusion, Emeishan Large Igneous Province, SW China: implications for parental magma compositions, sulfide saturation history and Fe–Ti oxide mineralization. J. Petrol. 60 (3), 619–648. doi:10.1093/petrology/egz008
Bai, Z. J., Zhong, H., Zhu, W. G., and Hu, W. J. (2022). Mantle plume-subducted oceanic slab interaction contributes to geochemical heterogeneity of the Emeishan large igneous province. Chem. Geol. 611, 121117. doi:10.1016/j.chemgeo.2022.121117
Chen, J., Yang, R. D., Du, L. J., Zheng, L. L., Gao, J. B., Lai, C. K., et al. (2018). Mineralogy, geochemistry and fluid inclusions of the Qinglong Sb-(Au) deposit, Youjiang basin (Guizhou, SW China). Ore Geol. Rev. 92, 1–18. doi:10.1016/j.oregeorev.2017.11.009
Chen, Q., Yu, S., Wen, H., Lan, J., and Luo, C. (2020). A preliminary study on the possible mechanism of enrichment and occurrence state of Nb-Ga-REE in the emeishan basalts from the diandong qianxi region. Bull. Mineral. Petrol. Geochem 39, 1256–1277. doi:10.19658/j.issn.1007-2802.2020.39.069
Dai, S. F., Ren, D. Y., Zhou, Y. P., Zhang, M. Q., and Ward, C. R. (2014). Coal-hosted rare metal deposits: genetic types, modes of occurrence, and utilization evaluation. J. China Coal Soc. 39 (8), 1707–1715. doi:10.13225/j.cnki.jccs.2014.9001
Du, L. J., Chen, J., Yang, R. D., Huang, Z. L., Zheng, L. L., Gao, J. B., et al. (2020). Hydrothermal-volcanic sedimentary and mineralization of the Dachang layer in the middle–late permian, Qinglong, southwestern Guizhou. Dizhi Lunping 66, 440–456. doi:10.16509/j.georeview.2020.02.013
Du, S. J., Wen, H. J., Luo, C. G., Gu, H. N., Yu, W. X., Li, Y., et al. (2019). Mineralogy study of Nb-rich sphene generated from the Emeishan basalts in Eastern Yunnan-Western Guizhou area, China. Acta Min. Sin. 39, 253–263. doi:10.16461/j.cnki.1000-4734.2019.39.040
Gao, Z. M., Zhang, Q., Tao, Y., and Luo, T. Y. (2004). An analysis of the mineralization connected with Emeishan mantle plume. Acta Mineral. Sin. 24 (2), 99–104. doi:10.16461/j.cnki.1000-4734.2004.02.001
Hao, X. F., Peng, Y., Tang, Y., and Fan, J. B. (2021). First discovery of sedimentary sandstone-hosted rhenium deposit in the Fetianshan Formation (K 1 f) in the Puge area, Xichang. Geol. China 48 (6), 1975–1977. doi:10.12029/gc20210623
He, B., Wang, Y. M., and Jiang, X. W. (2004). Paleo-karst landforms on top of limestone of the Maokou Formation in the west of the upper Yangtze platform and its geological significance. Geol. China 31 (1), 46–50. doi:10.12029/gc20040106
He, B., Xu, Y., Xiao, L., Wang, K., and Sha, S. (2003). Generation and spatial distribution of the Emeishan large igneous province: new evidence from stratigraphic records. Acta Geol. Sin. 77 (2), 194–202. doi:10.3321/j.issn:0001-5717.2003.02.007
He, B., Xu, Y. G., Wang, Y. M., Luo, Z. Y., and Wang, K. M. (2005). The magnitude of crustal uplift prior to the eruption of the Emeishan basalt: inferred from sedimentary records. Geotect. Metallogenia 29 (3), 316–320. doi:10.16539/j.ddgzyckx.2005.03.004
He, L., Luo, X., Liu, L. P., Li, P., and Zhou, G. X. (2008). A discussion on depositional environment in Late Permian and distribution of reef-bank in Sichuan Basin. Nat. Gas. Ind. B 28 (1), 28–32. doi:10.3787/j.issn.1000-0976.2008.01.007
Hu, R., Fu, S., Huang, Y., Zhou, M. F., Fu, S., Zhao, C., et al. (2017). The giant South China Mesozoic low-temperature metallogenic domain: reviews and a new geodynamic model. J. Asian Earth Sci. 137, 9–34. doi:10.1016/j.jseaes.2016.10.016
Hu, R., Tao, Y., Zhong, H., and Zhang, Z. W. (2005). Mineralization systems of a mantle plume: a case study from the Emeishan igneous province, southwest China. Earth Sci. Front. (01), 42–54. doi:10.3321/j.issn:1005-2321.2005.01.007
Jiang, H. B., Jiang, C. Y., Qian, Z. Z., Zhu, S. F., Zhang, P. B., and Tang, D. M. (2009). Petrogenesis of high-Ti and low-Ti basalts in emeishan, yunnan, China. Acta petrol. Sin 25, 1117–1134. doi:10.7666/d.y977991
Li, M. (2020). Comprehensive study on the metallogenic model of Dachang antimony deposit in Qinglong county, Guizhou province. Master thesis of Chengdu University of Technology.
Liu, D. (2020). Nb and REE deposits found in the weathering crusts of Emeishan basalt in Xuanwei area, Yunnan Province. Geol. China 47 (2), 540–541. doi:10.12029/gc20200220
Liu, D., Lv, X., Huang, J., and &Cheng, Y. (2018). Geological characteristics and genesis of Nanhong agate from Huize area of the northeast Yunnan province. Contributions Geol. Mineral Resour. Res. 33 (04), 548–553. doi:10.6053/j.issn.1001-1412.2018.04.007
Lu, N. (2018). A comparative study of Baoshan red agate in Yunnan and Sichuan red agate in Liangshan. Master thesis of Chengdu University of Technology.
Luo, W. (2014). Magmatic Cu-Ni-pge sulfide and Fe-Ti-V oxide deposits and their relationship to emeishan basalt in permian emeishan large igneous province, SW China: a review. Earth science—J. Earth Sci. 39 (10), 1443–1454. doi:10.3799/dqkx.2014.126
Ma, X., Yang, Y., Zhang, J., and Xie, J. (2019). A major discovery in Permian volcanic rock gas reservoir exploration in the Sichuan Basin and its implications. Nat. Gas. Ind. B 6 (4), 419–425. doi:10.3787/j.issn.1000-0976.2019.02.001
McLennan, S. M., Hemming, S., McDaniel, D. K., and Hanson, G. N. (1993). Geochemical approaches to sedimentation, provenance, and tectonics, 21–40. doi:10.1130/spe284-p21
Meng, C., Chen, Y., Zhang, Y., Wu, H., Ling, W., Zhang, H., et al. (2015). Genetic relationship between unroofing of the Emeishan large igneous province and the iron-polymetallic deposit in western Guizhou, Southwestern China: constraint from U-Pb geochronology and geochemistry of zircon. Sci. China Earth Sci. 58, 1939–1950. doi:10.1007/s11430-015-5066-x
Mundil, R., Ludwig, K. R., Metcalfe, I., and Renne, P. R. (2004). Age and timing of the Permian mass extinctions: U/Pb dating of closed-system zircons. Science 305 (5691), 1760–1763. doi:10.1126/science.1101012
Sichuan Bureau of Geology and Mineral Resources (1991). Sichuan regional Geology. Beijing: Geology Press.
Song, X. Y., Qi, H. W., Hu, R. Z., Chen, L. M., Yu, S. Y., and Zhang, J. F. (2013). Formation of thick stratiform Fe-Ti oxide layers in layered intrusion and frequent replenishment of fractionated mafic magma: evidence from the Panzhihua intrusion, SW China. Geochem., Geophys., Geosyst. 14 (3), 712–732. doi:10.1002/ggge.20068
Tao, Y., Li, C., Hu, R., Ripley, E. M., Du, A., and Zhong, H. (2007). Petrogenesis of the Pt–Pd mineralized Jinbaoshan ultramafic intrusion in the Permian Emeishan large igneous province, SW China. Contrib. Mineral. Petr. 153, 321–337. doi:10.1007/s00410-006-0149-5
Tao, Y., Ma, Y., Miao, L., and Zhu, F. (2009). SHRIMP U-Pb zircon age of the Jinbaoshan ultramafic intrusion, Yunnan Province, SW China. Chin. Sci. Bull. 54 (1), 168–172. doi:10.1007/s11434-008-0488-x
Tian, E. Y., Gong, D. X., Lai, Y., Qiu, X. L., Xie, H., and Tian, K. Z. (2021b). Genesis and enrichment of sedimentary rare earth in Weining area, Guizhou Province. Earth Sci. 46 (8), 2711–2731. doi:10.3799/dqkx.2020.301
Tian, Y., Li, Y., Meng, F., and Du, Q. (2021a). A study of the petrogenesis and spatial difference of the Emeishan large igneous province: based on geochemical analysis and simulation of the high Ti basalts in the whole region. Acta Petrologica Mineralogica 40 (4), 687–703. doi:10.3969/j.issn.1000-6524.2021.04.002
Van der Zwan, F. M., Augustin, N., Le Saout, M., Seidel, E., Wölfl, A. C., Schade, M., et al. (2023). Tectonically assisted emplacement of oceanic intraplate volcanoes: the Bathymetrists Seamounts, central Atlantic. Geomorphology 441, 108891. doi:10.1016/j.geomorph.2023.108891
Wang, C. Y., Wei, B., Zhou, M. F., Minh, D. H., and Qi, L. (2018). A synthesis of magmatic Ni-Cu-(PGE) sulfide deposits in the∼ 260 Ma Emeishan large igneous province, SW China and northern Vietnam. J. Asian Earth Sci. 154, 162–186. doi:10.1016/j.jseaes.2017.12.024
Wang, C. Y., Zhou, M. F., and Zhao, D. (2005). Mineral chemistry of chromite from the Permian Jinbaoshan Pt–Pd–sulphide-bearing ultramafic intrusion in SW China with petrogenetic implications. Lithos 83 (1-2), 47–66. doi:10.1016/j.lithos.2005.01.003
Wang, D., Li, J., Wang, C., Qu, W., Fu, X., and Fu, D. (2007). New advances in geochronologic study related to Emei mantle plume and their significance. Mineral. Deposits-Beijing 26 (5), 550. doi:10.16111/j.0258-7106.2007.05.001
Wang, F., Zhao, T., and Chen, W. (2012). Advances in study of Nb-Ta ore deposits in Panxi area and tentative discussion on genesis of these ore deposits. Mineral. deposits 31 (2), 293–308. doi:10.16111/j.0258-7106.2012.02.010
Wang, F., Zhu, X., and Wang, Z. (2011). Madouzi-type (nodular) sedimentary copper deposit associated with the Emeishan basalt. Sci. China Earth Sci. 54, 1880–1891. doi:10.1007/s11430-011-4331-x
Wang, W., Zhao, L., Luo, B., Liu, R., Li, Y., Zhao, L., et al. (2021). Relationship between abnormal high pressure evolution of Permian volcanic rocks and natural gas accumulation in the western Sichuan Basin. Acta Petrol. Sin. 42 (11), 1437. doi:10.7623/syxb202111003
Xu, Y., and Chung, S. (2001). The Emeishan large igneous province: evidence for mantle plume activity and melting conditions. Geochimica 30 (1), 1–9. doi:10.19700/j.0379-1726.2001.01.002
Xu, Y., Chung, S.-L., Jahn, B.-m., and Wu, G. (2001). Petrologic and geochemical constraints on the petrogenesis of Permian–Triassic Emeishan flood basalts in southwestern China. Lithos 58 (3-4), 145–168. doi:10.1016/s0024-4937(01)00055-x
Xu, Y., Zhong, Y., Wei, X., Chen, J., Liu, H., Xie, W., et al. (2017). Permian mantle plumes and Earth’s surface system evolution. Bull. Mineral. Petrol. Geochem. 36 (3), 359–373. doi:10.3969/j.issn.1007-2802.2017.03.001
Yang, R., Bao, M., Liao, L., Wang, W., Wei, H., and Wang, Q. (2007). Ancient weathering crust and its mineralization near the Middle-Upper Permian boundary in western Guizhou Province, China. Acta Mineral. Sin. 27 (1), 41–48. doi:10.16461/j.cnki.1000-4734.2007.01.007
Yin, H., and Song, H. (2013). Mass extinction and Pangea integration during the Paleozoic-Mesozoic transition. Sci. China Earth Sci. 56, 1791–1803. doi:10.1007/s11430-013-4624-3
Zeng, L., Zhang, J., and Sun, T. (2016). Geological characteristics, genesis, and its prospecting exploration enlightenment of Lanzhichang iron deposits in the emeishan large igneous province. J. Jilin Univ. Earth Sci. Ed. 39 (1), 9–0016. doi:10.13278/j.cnki.jjuese.201602110
Zeng, Z., and Liu, Y. (2022). Magmatic–hydrothermal zircons in syenite: a record of NbTa mineralization processes in the Emeishan large igneous province, SW China. Chem. Geol. 589, 120675. doi:10.1016/j.chemgeo.2021.120675
Zhang, A., Guo, Z., Afonso, J. C., Shellnutt, J. G., and Yang, Y. (2024). Mantle plume-lithosphere interactions beneath the emeishan large igneous province. Geophys. Res. Lett. 51 (2), e2023GL106973. doi:10.1029/2023gl106973
Zhang, H., Li, R., Ba, J., Li, X., and Ma, J. (2019). Geochemical characteristics of the lower Triassic Feixianguan Formation in Meigu area, southwestern Sichuan and its significance for the provenance and tectonic setting. J. Min. Pet. 39, 52–59. doi:10.19719/j.cnki.1001-6872.2019.03.07
Zhang, H., Wen, J., and Zhu, H. (2021). Geochemical characteristics and genesis of REE enrichment beds at the bottom of the Upper Permian Xuanwei Formation in Muchuan area, Sichuan province. J. Mineralogy Petrology 41 (4), 172–183. doi:10.19719/j.cnki.1001-6872.2021.02.03
Zhang, Q., Chen, Y., and Liu, X. (2022). Characteristics and geological significance of volcanic ash in bauxite in western Guangxi. Geol. Rev. 68 (2), 531–550. doi:10.16509/j.georeview.2022.02.055
Zhang, T., Chen, X., Liu, Z., Wei, G., Yang, W., Ming, H., et al. (2011). Effect of emeishan mantle plume over the sedimentary pattern of mid-permian Xixia period in Sichuan Basin. Acta Geol. Sin. 85 (8), 1251–1264.
Zhang, Y., Chen, S., Zhang, X., Zhang, X., Xie, C., Chen, C., et al. (2020). Restoration of paleokarst geomorphology of lower permian Maokou Formation and its petroleum exploration implication in Sichuan Basin. Lithol. Reserv. 32 (3), 44–55. doi:10.12108/yxyqc.20200305
Zhang, Z., Hou, T., and Cheng, Z. (2022b). Mineralization related to large igneous provinces. Acta Geol. Sin. 96, 131–154. doi:10.19762/j.cnki.dizhixuebao.2022262
Zhang, Z., Mao, J., Wang, F., and Pirajno, F. (2006). Native gold and native copper grains enclosed by olivine phenocrysts in a picrite lava of the Emeishan large igneous province, SW China. Am. Min. 91 (7), 1178–1183. doi:10.2138/am.2006.1888
Zhong, H., Zhu, W.-G., Hu, R.-Z., Xie, L.-W., He, D.-F., Liu, F., et al. (2009). Zircon U–Pb age and Sr–Nd–Hf isotope geochemistry of the Panzhihua A-type syenitic intrusion in the Emeishan large igneous province, southwest China and implications for growth of juvenile crust. Lithos 110 (1-4), 109–128. doi:10.1016/j.lithos.2008.12.006
Zhou, M.-F., Arndt, N. T., Malpas, J., Wang, C. Y., and Kennedy, A. K. (2008). Two magma series and associated ore deposit types in the Permian Emeishan large igneous province, SW China. Lithos 103 (3-4), 352–368. doi:10.1016/j.lithos.2007.10.006
Zhou, M.-f., Robinson, P. T., Lesher, C. M., Keays, R. R., Zhang, C.-J., and Malpas, J. (2005). Geochemistry, petrogenesis and metallogenesis of the Panzhihua gabbroic layered intrusion and associated Fe–Ti–V oxide deposits, Sichuan Province, SW China. J. Petrol 46 (11), 2253–2280. doi:10.1093/petrology/egi054
Zhou, Y., Lu, L., and Zheng, B. (1986). Paleomagnetic polarity of the permian emeishan basalt in sichuan. Geol. Rev. 32, 465–469. doi:10.16509/j.georeview.1986.05.007
Zhu, B. (2005). 40Ar/39Ar Ar and U-Th-Pb dating for native copper mineralization of two stages from the Emeishan flood basalts in Northeastern Yunnan Province, China. Geochimica 34, 235–147. doi:10.19700/j.0379-1726.2005.03.004
Zhu, C., Xu, M., Yuan, Y., Zhao, Y., Shan, J., He, Z., et al. (2010). Palaeogeothermal response and record of the effusing of Emeishan basalts in the Sichuan basin. Chin. Sci. Bull. 55, 949–956. doi:10.1007/s11434-009-0490-y
Keywords: Emeishan mantle plume, Emei mountain large igneous province, mineralization system, mineralization process, geological science and technology
Citation: Liu Z, Wen J, Zhao W, Chen M, Liu H, Zheng M, Tian Y and Guo Y (2025) Research progress and prospect of Emeishan mantle plume metallogenic system. Front. Earth Sci. 13:1511877. doi: 10.3389/feart.2025.1511877
Received: 15 October 2024; Accepted: 08 April 2025;
Published: 07 May 2025.
Edited by:
Hongjian Zhu, Yanshan University, ChinaReviewed by:
Jiawei Hu, Technical University of Berlin, GermanyKun Zhou, Sichuan Agricultural University, China
Copyright © 2025 Liu, Wen, Zhao, Chen, Liu, Zheng, Tian and Guo. This is an open-access article distributed under the terms of the Creative Commons Attribution License (CC BY). The use, distribution or reproduction in other forums is permitted, provided the original author(s) and the copyright owner(s) are credited and that the original publication in this journal is cited, in accordance with accepted academic practice. No use, distribution or reproduction is permitted which does not comply with these terms.
*Correspondence: Jun Wen, d2p1bjk5MzZAY3VnLmVkdS5jbg==; Wei Zhao, MTE0MjQ2NjE2NkBxcS5jb20=