- 1State Key Laboratory of Oil and Gas Reservoir Geology and Exploitation, Southwest Petroleum University, Chengdu, China
- 2School of Geosciences and Technology, Southwest Petroleum University, Chengdu, China
- 3Chongqing Division, Petro-China Southwest Oil and Gas Field Company, Chongqing, China
The Sichuan Basin is China’s largest natural gas-producing basin and presents significant challenges for deep oil and gas exploration due to its complex geological conditions. Recent studies in the Northeast Sichuan Basin, particularly the Kaijiang-Liangping (KJ-LP) Trough, have identified the presence of strike-slip faults which may influence the deposition of reefs and shoals. Understanding the controlling influence of these fault zones on the distribution and development of hydrocarbon reservoirs is crucial for effective exploration. Traditional fault identification methods are less effective due to the weak seismic response in this area, which results from the complex geological conditions and lack of dissolution cavities. Predicting fault-related fractures remains a key challenge. To address these challenges, this paper proposes a novel fault characterization approach using the Gradient-Driven Signed Pressure Force (G-SPF) Active Contour Model (ACM), an image-processing-based boundary identification technique. The method begins by selecting tensor and symmetry as the primary optimization attributes. Gradient information is then integrated into the ACM to improve the vertical continuity of fault identification. Finally, a two-step iterative process is employed to separately characterize the fault core and damage zone, improving fault zone delineation. The improved methodology significantly enhances strike-slip fault zone characterization. The results also reveal that strike-slip fault zones serve as vertical migration pathways for deep-source hydrocarbons and they facilitate the lateral migration from source rocks within the trough. By integrating structural analysis with sedimentary characteristics, this study highlights the critical role of strike-slip fault zones in hydrocarbon migration and accumulation.
1 Introduction
The Sichuan Basin is China’s largest natural gas-producing region, with significant exploration potential. However, the geological resource exploration rate currently remains at only 18.8% (Yang et al., 2023; Deng et al., 2024). The successful exploration of strike-slip fault-controlled reservoirs in the Tarim Basin (Neng et al., 2022; Ning et al., 2022; Zeng et al., 2024) has increased interest in similar fault systems within the Sichuan Basin. In recent years, a series of strike-slip faults have been identified in the Northeast Sichuan Basin (Wu et al., 2023; Liu et al., 2024), an area where reef-shoal reservoirs are also widely developed (Liu et al., 2017; Long et al., 2020). Investigating the relationship between strike-slip fault zones and reef-shoal reservoirs can guide more efficient and effective exploration efforts in this area.
Strike-slip faults, characterized by horizontal displacement and nearly vertical fault planes, typically have a central fault core sur broader damage zones (Kim et al., 2004; Choi and Kim, 2009; Tang et al., 2022; Silva et al., 2024). The deformation and strain behaviors of these two regions exhibit distinct characteristics (Chester et al., 1993; Torabi et al., 2019; Torabi et al., 2020; Torabi et al., 2021). It is challenging to characterize the strike-slip faults in seismic data due to their limited vertical displacement. Conventional methods for identifying faults typically rely on seismic interpretation and fault attributes such as curvature, coherence, variance, and dip analysis (Zalán, 1987; Pu et al., 2018; Yuan et al., 2021; Brito et al., 2023; Oliveira et al., 2024; Tian et al., 2024). To improve detection accuracy, recent studies have introduced several innovative approaches: Sun et al. (2023) proposed a seismic tensor thickness method to optimally identify strike-slip fault damage zones; Wang et al. (2022) utilized the multi-filtering process and the maximum likelihood method to identify small faults; Cui et al. (2022) applied hybrid attributes and neural networks to enhance imaging of strike-slip faults. However, most studies on strike-slip faults have concentrated on Western China, such as the Tarim Basin, with limited research on the Sichuan Basin. Unlike the fault-karst structures in Tarim, strike-slip faults in Sichuan exhibit weaker seismic responses (Li et al., 2024). As porosity and permeability in fault zones significantly impact reef and shoal gas reservoirs (Garland et al., 2012), accurately characterizing the spatial distribution of strike-slip fault zones in Sichuan is critical for advancing fault-controlled reservoir studies.
Given these challenges in characterizing strike-slip fault zones, advanced imaging techniques like the Active Contour Model (ACM) offer promising tools for more precise boundary identification. The ACM works by evolving curves or contours to detect object boundaries by minimizing an energy function (Kass et al., 1988). It is commonly categorized into two classes: edge-based model (Kichenassamy et al., 1995; Malladi et al., 1995; Caselles et al., 1997; Li et al., 2007) and region-based model (Ronfard, 1994; Chan and Vese, 2001). The edge-based model relies on image gradients to locate boundaries and Shafiq et al. (2015) proposed an edge-based geodesic active contour to segment salt dome. In contrast, region-based models focus on the statistical properties of regions rather than edge gradients. To address cases with weak or complex boundaries, Zhang et al. (2010) proposed the Signed Pressure Force (SPF) ACM, which combines the strengths of both edge-based and region-based approaches.
In this paper, to improve strike-slip fault detection in the Northeast Sichuan Basin, we propose a Gradient-Driven Signed Pressure Force (G-SPF) model. This model takes advantage of the nearly vertical planes characteristic of strike-slip faults and incorporates gradient information within the SPF model to enhance vertical continuity. By integrating seismic attributes with the G-SPF model, we can obtain a clearer spatial distribution of fault zones, which allows us to discuss the relationship between hydrocarbon accumulation and strike-slip fault zones.
2 Geological background
The Sichuan Basin, located in southwestern China, is one of the largest natural gas-rich basins. According to the latest oil and gas resource assessment results released in 2023, the natural gas resources in the Sichuan Basin amount to 38.19 trillion cubic meters (38.19 × 1012 m³) (Deng et al., 2024). This huge resource is the result of the complex tectonic process. The basin is situated on the western margin of the Yangtze Plate and has experienced multi-stage tectonic movements, including collisions with neighboring tectonic blocks such as the Qiangtang and the North China Plate. These tectonic activities have facilitated the generation, migration, and accumulation of oil and gas by modifying geological structures, creating storage spaces, and forming sealing traps.
The Kaijiang-Liangping (KJ-LP) Trough, located in the Northeast Sichuan Basin, is defined by its NE-SW trending geometry (Figure 1; Wei et al., 2005). The stratigraphy of the KJ-LP Trough is primarily composed of deep-water carbonates and clastic rocks. In the Upper Permian Changxing to the Early Triassic formation, the platform margin is mainly developed with dolomite reefs and beaches, while the interior of the KJ-LP Trough is dominated by siliceous mudstone and shale (Liu C. et al., 2016; Xu et al., 2023).
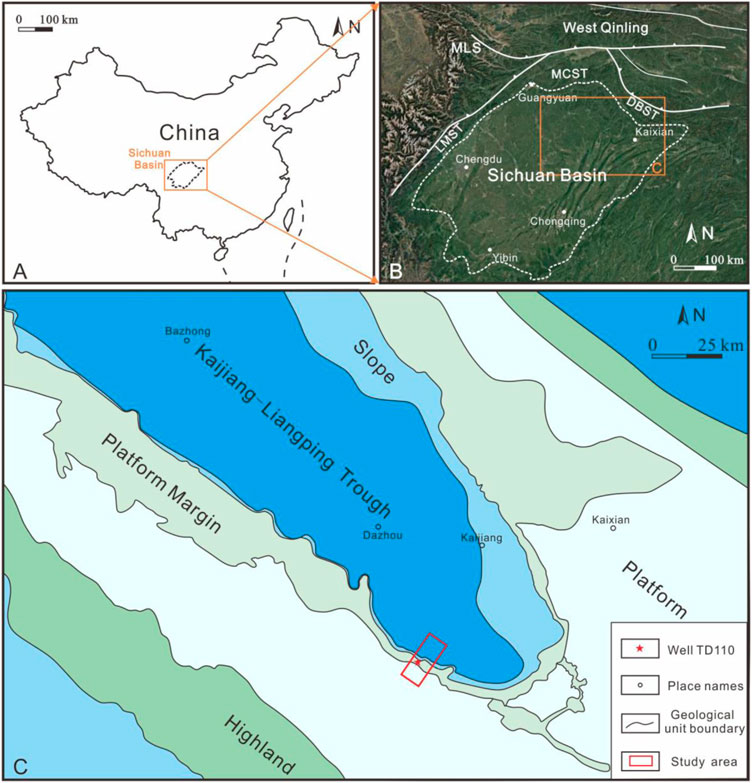
Figure 1. (A) Location of the Sichuan basin in China. (B) Location of the Kaijiang-Liangping Trough in China. MLS-Mianlue Structure belt, LMST-Longmen Shan Thrust belt, MCST-Micang Shan Thrust belt, DBST-Daba Shan Thrust belt. (C) Distribution map of the sedimentary units in the KJ-LP Trough.
Influenced by the tectonic activities of surrounding plates and regional transgression-regression events, the KJ-LP Trough developed multiple unconformities. These unconformities reflect the complex geological history. The formation timing of the KJ-LP Trough is still controversial. The more widely accepted viewpoint is that the Trough was primarily formed during the Late Permian to Early Triassic (Ma et al., 2006; Liu S. et al., 2016). Since the east side of the KJ-LP Trough formed with faults (Wei et al., 2005), we are more likely to call it an intracratonic rift. It was formed under the control of the “Emei Tafrogeny” and the subduction of the Mianlue Ocean in the north (Liu et al., 2015; Mo et al., 2019; Liu et al., 2021). During the late Middle Permian, under the activity of basement fault blocks, the intra-platform depression formed by differential uplift of strata (Wu et al., 2019; Wang et al., 2021).
Northeast and Central Sichuan Basin both belong to the western margin of the Yangtze Plate, and have undergone similar plate tectonic movement. However, the KJ-LP Trough is close to the Qinling Fold Belt and the Mianlue Suture Zone (Figure 1B), which adds to the complexity of strike-slip fault development. The evolution of strike-slip faults in Northeast Sichuan is likely to be more complex than those in Central Sichuan. It undergoes at least four stages. During the late Sinian, depressions such as the Deyang-Anyue Depression developed under the extensional environment (Gu et al., 2021; Ma et al., 2023; Wu et al., 2023). The area was subjected to oblique extensional stress, and high-angle strike-slip faults gradually formed along pre-existing basement weaknesses. In the Early Cambrian to Ordovician, the tectonic setting changed from the extensional to the compressional (Liang et al., 2023; Ma et al., 2023). The scale of the stress was limited, and the activity of the strike-slip faults was weak. However, parts of the faults were reactivated and grew upward (Wu et al., 2023). In the Late Permian to Early Triassic, the Mianlue Ocean subducted (He et al., 2011; Feng et al., 2021), and the KJ-LP Trough developed along the northwest direction. The strike-slip faults gradually reactivated and experienced minor extensional movement. In the Middle and Late Triassic, the Indonesian Movement caused significant uplift and erosion in Western Sichuan (Li et al., 2003), leading to strong strike-slip fault activity. These faults developed along pre-existing fault zones. The deformation intensity was strong and positive flower structures formed under strong oblique compression (Li et al., 2024; Liu et al., 2024; Wu et al., 2024). After the Triassic, strike-slip fault activity mostly ceased, with only minor reactivation observed in localized areas.
3 Seismic response of strike-slip faults
The characterization of strike-slip faults is closely linked to the fault zone width. To explore the seismic responses of fault zones with varying widths, we use a forward model to study their different seismic responses. However, given the structural complexity of strike-slip fault zones, as well as imaging challenges related to resolution, signal strength, and other factors, the forward model may not fully capture real seismic responses. Therefore, we complement the model analysis with actual seismic data, aiming to identify and summarize the diverse seismic responses associated with strike-slip faults.
3.1 Seismic forward model of strike-slip faults
We create a seismic forward model of faults with varying widths using the acoustic wave equation. The velocity values used in the models were referenced from real well logs, and the corresponding velocity values were listed on the right side of the model (Figure 2A). In our seismic forward modeling, the source spacing was set to 40m, and the receiver spacing was 25 m. A Ricker wavelet with a dominant frequency of 25 Hz was used as the source wavelet. The Kirchhoff post-stack depth migration (PSDM) was used to process the data.
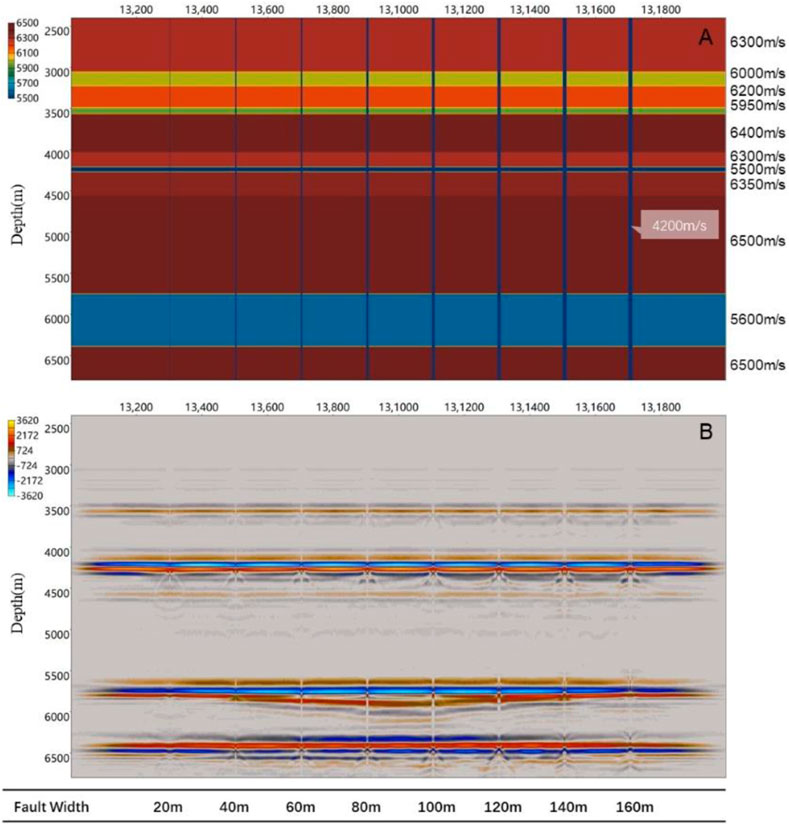
Figure 2. (A) Velocity models for faults with different widths. (B) Forward Modeling Results of Pre-Stack Depth Migration.
The width of a strike-slip fault zone is likely related to its degree of activity, with wider fault zones typically indicating stronger tectonic movements or multi-phase tectonic deformation. Forward modeling reveals several key insights about the behavior of strike-slip fault zones (Figure 2B). Weak reflections, bending, or deformation of seismic events are the primary indicators of fault presence. As the fault zone width increases, the seismic responses become more pronounced, with enhanced weak reflection features at the fault zones. This suggests a direct correlation between the width of anomalous bands and fault zone width, supporting the potential for characterizing strike-slip fault zones through seismic data. Additionally, shallow layers are particularly sensitive to multiples, which can complicate fault detection. In regions with weaker amplitudes, identifying faults becomes challenging unless supported by stronger events from adjacent layers. Finally, overlapping fault zones can create complex geological structures, further complicating the interpretation of seismic waveforms and reflections.
3.2 Seismic response
According to the evolution periods we discussed above, the strike-slip faults in the Sichuan Basin were deep-rooted structures developed since the Sinian. These faults are characterized by their high-angle, roughly near-vertical fault planes.
Through the analysis of seismic data, we identify six different types of seismic responses in the Northeast Sichuan Basin (Figure 3). The most significant type is the weak amplitude type (Figure 3A), which is characterized by low seismic amplitudes at fault zones, with reflections almost blank. And this weak amplitude exhibits vertical continuity. Another type is marked by chaotic reflections (Figure 3B), which may indicate a wider and more complex fault zone. These two types provide a more overall response. However, when we focus on the details of reflection events, we can identify more detailed features: some reflection events are broken with vertical displacement (Figure 3C), others show differences in the number of events on either side of the fault (Figure 3D), and in certain areas, flexure of reflection events can be seen (Figure 3E). All the above five types have obvious discontinuity. The most difficult type to identify is the flexure of events without displacement (Figure 3F), which only manifests as a slight bulge in the reflection events.
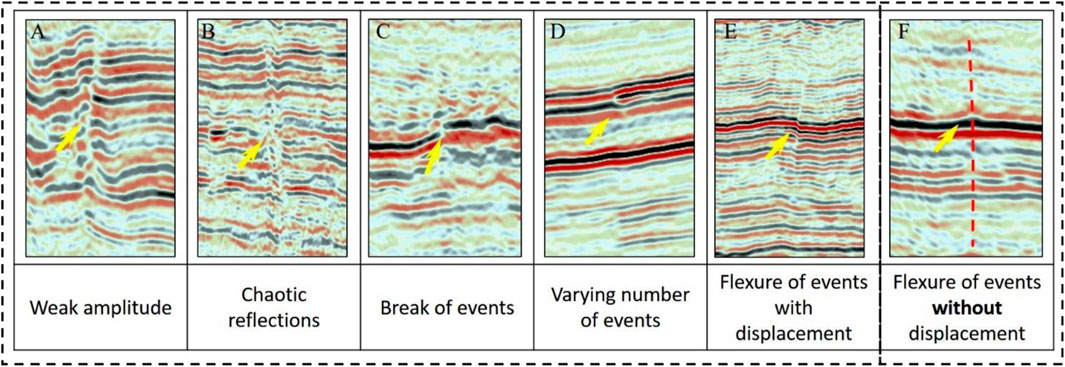
Figure 3. Different seismic responses of strike-slip faults in the Northeast Sichuan Basin: (A) weak amplitude type, (B) chaotic reflections type, (C) break of events type, (D) varying number of events type, (E) flexure of events with displacement type and (F) flexure of events without displacement type.
4 Methods
The general workflow of the proposed method is shown in Figure 4. We first extract tensor and symmetry attributes, preprocess the data, and calculate their gradients. These values are then fed into the G-SPF iterative process, where the contour is updated in each iteration. If the result does not converge, the computation steps are repeated. Once convergence is achieved, the contour for the first G-SPF is output, representing the boundary of the fault damage zones. This contour is then used as the initial boundary for the second iteration, and the attribute values are reset based on the newly obtained contour. After the second G-SPF iteration, we get the boundary of the fault core. The detailed techniques of the method are outlined in the following sections.
4.1 Attributes for strike-slip fault identification
4.1.1 Tensor
The structure tensor represents the gradient information of a point within a defined neighborhood, indicating continuity in the gradient direction. It can be expressed as a matrix. The rank of a matrix reflects the number of indices required to describe it.
For 3D seismic data, the structure tensor is represented as Equation 1.
Here,
The tensor attribute offers local gradient information and orientation sensitivity, making them effective in detecting areas of discontinuity and delineating fault zones. When the seismic response of strike-slip faults displays weak amplitudes or chaotic reflections, the tensor attributes provide a clear response (Li et al., 2019; Zhang et al., 2024).
On the horizon slice of the tensor attribute (Figure 5), we can observe continuous, banded high-value zones, which represent the strike of the faults. These high values gradually decrease outward from the fault core, with a color gradient transitioning from red to blue using a rainbow color scale, indicating a weakening of discontinuities away from the fault core. The tensor attributes not only characterize the fault strike but also reflect the intensity of activity within the fault zone.
On the seismic profile (Figure 6A), the fault zones are characterized by chaotic reflections, discontinuities in the event alignments, and amplitude attenuation, with these features extending vertically. In the tensor attribute profile (Figure 6B), high values showing nearly vertical extensions that correspond to the faults observed in the seismic profile, highlight the fault morphology. The high values (red color) of the tensor attributes indicate areas of concentrated strain and significant discontinuities, suggesting that these red zones likely represent the fault core. The tensor attribute profile enhances the view of the fault zone’s characteristics, offering deeper insights into the fault’s morphology.
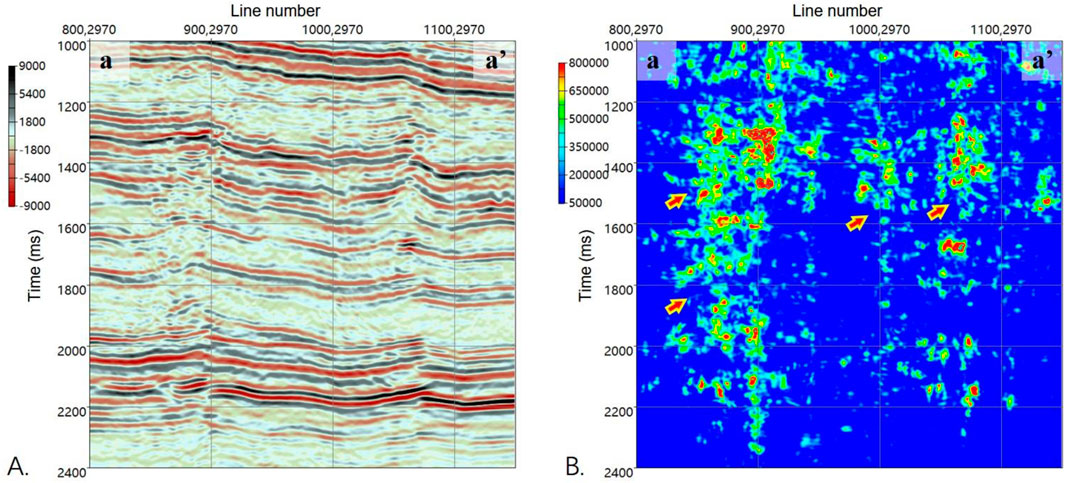
Figure 6. (A) Seismic profile and (B) Tensor attribute of aa’ (the location is shown in Figure 5).
4.1.2 Symmetry
The principle of symmetry in seismic data analysis is based on the concept that a signal remains unchanged under certain transformations, such as rotation or reflection (Manzi et al., 2020; Huang and Wang, 2023). Symmetry is often evaluated over a moving window along seismic traces. The symmetry of the seismic trace s(t) in this case is given by Equation 2.
where
In this formula: S(t) = 1 when the trace is perfectly symmetric, and S(t) = 0 when it is perfectly asymmetric. This attribute focuses on structural continuity, independent of amplitude variations, and helps highlight subtle features in seismic data.
On the symmetry horizon slice (Figure 7), similar to the tensor attributes, anomalous banded zones are observed. However, while the tensor attributes highlight areas of exceptionally high values, the symmetry attribute reveals regions with exceptionally low values. On the seismic profile, the rectangular box in Figure 8A shows a fault with flexure of events but no displacement. In the symmetry profile (Figure 8B), vertical fault bands are visible, whereas the tensor attributes show no response (Figure 8C). This indicates that the symmetry attribute is sensitive to asymmetry in the data, highlighting areas where structural continuity is disrupted, and is effective in revealing subtle features in seismic data. To more accurately characterize strike-slip faults, it is necessary to integrate this approach with the tensor attributes.
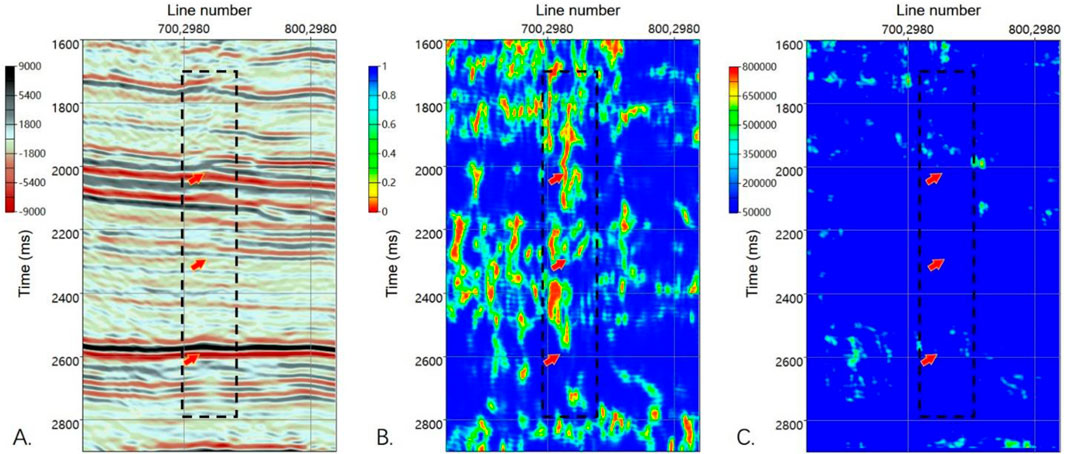
Figure 8. (A) Seismic profile, (B) symmetry attribute, and (C) tensor attribute of BB’ (the location is shown in Figure 7).
4.2 Boundary extraction based on gradient-driven signed pressure force Active Contour Model
Since the strike-slip faults have nearly vertical fault planes, enhancing vertical continuity in their delineation is essential. In this paper, we apply a G-SPF model. The gradient can represent both the direction and intensity changes of attribute values. By integrating the gradient into the energy function, the contour is guided to evolve towards the boundaries of strike-slip faults, enabling more accurate fault zone delineation.
The core of the proposed method involves evolving a contour, represented by a Level Set Function (LSF) denoted as ϕ(x,y), which implicity defines the contour. Specifically, ϕ(x,y) = 0 defines the contour itself, ϕ(x,y) > 0 indicates the inside of the contour, and ϕ(x,y) < 0 marks the outside. The contour evolves iteratively by minimizing a predefined energy function, driving it towards the boundaries of the fault zones.
Let
where
The signed pressure force
where
The proposed G-SPF model consists of two main components: the Global-SPF, which characterizes the global distribution, and the Gradient-SPF, which incorporates gradient-driven forces for more localized analysis. For each attribute, the
The
where
The term
Attributes used to identify faults actually detect discontinuities. The attribute values in fault zones show significant changes compared to non-fault areas, where the values are more stable and have a broader distribution. Therefore, the attribute data often follows a non-normal distribution and exhibits a large deviation from the median. The MAD is a robust statistical measure that helps us obtain a more stable estimate of the median which can be defined as:
The parameters
where
The
where
By calculating the Euler-Lagrange equation of the Equation 3 and following Fang et al. (2019), the final evolution equation can be expressed as:
4.3 Two-step iterative extraction of fault damage zone and fault core
The fault is not merely a plane but a complex fault zone, consisting of a narrow fault core and broader damage zones on either side (Choi and Kim, 2009; Torabi et al., 2020). The deformation and strain in these two regions behave differently (Chester et al., 1993; Torabi et al., 2019; Torabi et al., 2021). The fault damage zone is more widely distributed, with lower strain intensity and weaker seismic signals, while the fault core shows more intense changes in seismic data. The proposed method applies a two-step iterative process that progressively refines the results, allowing us to approximate both the fault damage zone and the more localized fault core.
In the first iteration, we obtain the fault zone boundary. Following this, the attribute values outside the boundary are adjusted to the average value of the inside region, and this boundary is used as the initial contour for the second iteration. This adjustment effectively smooths the attribute distribution, isolating the region of interest for more refined analysis.
The process then moves to a second iteration, where the modified attributes are fed back into the G-SPF for another round of contour evolution. The G-SPF process iterates again until convergence is achieved, resulting in the extraction of the fault core - a more concentrated zone within the fault system characterized by higher strain and deformation. This two-step process enables a more detailed and focused extraction, first capturing the broader fault damage zone and then producing a more refined result within the fault system.
5 Result
In this chapter, we apply the proposed method to seismic data from the Northeast Sichuan Basin and compare results with common attributes to observe the outcomes.
In the variance horizon slice (Figure 9A), high-value banded zones are also observed, but compared to the tensor attribute in Figure 5 and the symmetry attribute in Figure 7, the distribution is more limited, indicating that variance is less effective in identifying fault zones than the other two attributes. The variance attribute captures some of the discontinuities but does not highlight the fault zone as clearly or extensively as the tensor and symmetry attributes do.
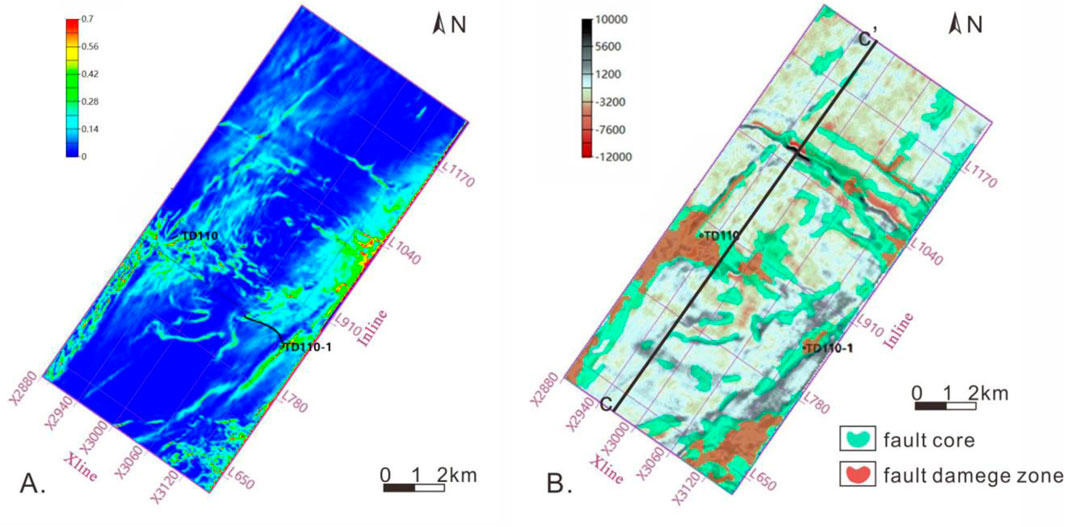
Figure 9. (A) Variance horizon slice of the Base Permian Liangshan Formation (P1l). (B) seismic horizon slice of the Base Permian Liangshan Formation (P1l) with detection results from the proposed method.
Among these three different attributes, the tensor attribute highlights areas of deformation, with high values corresponding to regions of significant strain, while the symmetry attribute is sensitive to asymmetry in the data, highlighting areas where structural continuity is disrupted. In contrast, the proposed method, which combines tensor and symmetry attributes along with a two-step iterative process, refines the fault zone boundaries, producing clearer and more focused results (Figure 9B). This method effectively isolates both the damage zones and the fault core, providing a more accurate delineation of the fault structure.
From the profile results, the tensor struggles to identify flexure events without displacement and cannot delineate fault structures, particularly the detailed inner zones (Figure 10A). Symmetry can help with detecting faults that the tensor may fail to capture. However, while it indicates potential fault locations, symmetry tends to capture many subtle features, making it challenging to effectively isolate the primary fault structures (Figure 10B). Variance, as a commonly used attribute for fault detection, can identify discontinuities in stratigraphy but often includes unnecessary features like stratigraphic layers, fractures, or even noise. Moreover, it has difficulty in accurately representing fault structures (Figure 10C).
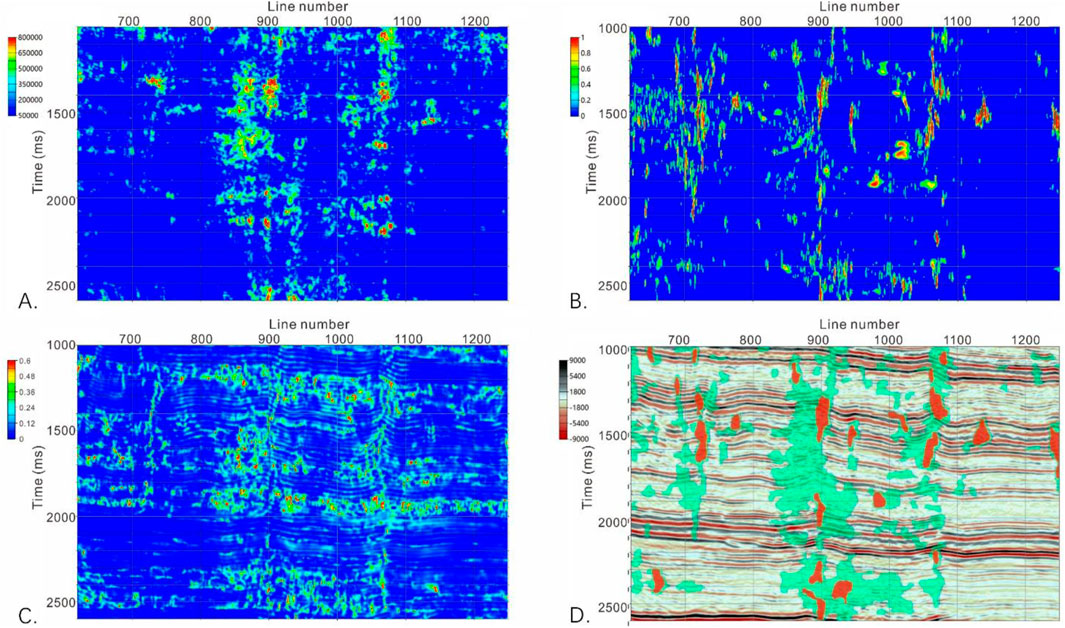
Figure 10. (A) Tensor profile, (B) symmetry profile, (C) variance Profile, and (D) seismic profile with detection results from the proposed method. The position of the profile is shown in Figure 9.
Figure 10D shows the results of applying the proposed method to the original seismic data. The fault zones are more clearly delineated, with distinct boundaries that outline both the broader damage zones and the narrower fault core. This demonstrates the effectiveness of the two-step iterative method, which refines fault zone contours based on both tensor and symmetry attributes, providing a more precise structural interpretation. By integrating tensor and symmetry attributes with G-SPF, the proposed method improves fault zone extraction accuracy, reducing uncertainty and enhancing the delineation of both the damage zones and fault core.
6 Discussion
6.1 Distribution of strike-slip fault zones
From the identification results (Figures 9B, 11), we observe three NW-trending fault zones, labeled a, b, and c, that appear consistently across different layers. This consistent vertical occurrence of fault zones across layers suggests that these strike-slip faults are deep-seated structures with vertical continuity, indicating structural inheritance. Typically, strike-slip faults tend to show increased fracturing and structural complexity in shallower layers due to accumulated tectonic influences over time (Joussineau et al., 2007; Meng et al., 2023; Guo et al., 2024). In the Permian Liangshan Formation (P1l) (Figure 9B), the fault structures are relatively simple, consistent with general expectations for this depth. However, the Feixianguan Formation (the middle one of these three layers) shows a higher degree of structural fragmentation (Figure 11A). This is particularly pronounced around fault zone b, which developed along the platform margin of the trough. Here, faulting combined with sedimentary processes has resulted in increased structural complexity and fragmentation in this layer. The early developed strike-slip faults could influence or control the development of the platform margin along the trough and have implications for hydrocarbon reservoir distribution. Further upward, in the Xujiahe Formation (Figure 11B), fault zone b exhibits branching with two fault cores, suggesting that a new structural movement occurred after the Feixianguan Formation, leading to the branching of the strike-slip fault in the shallower layers.
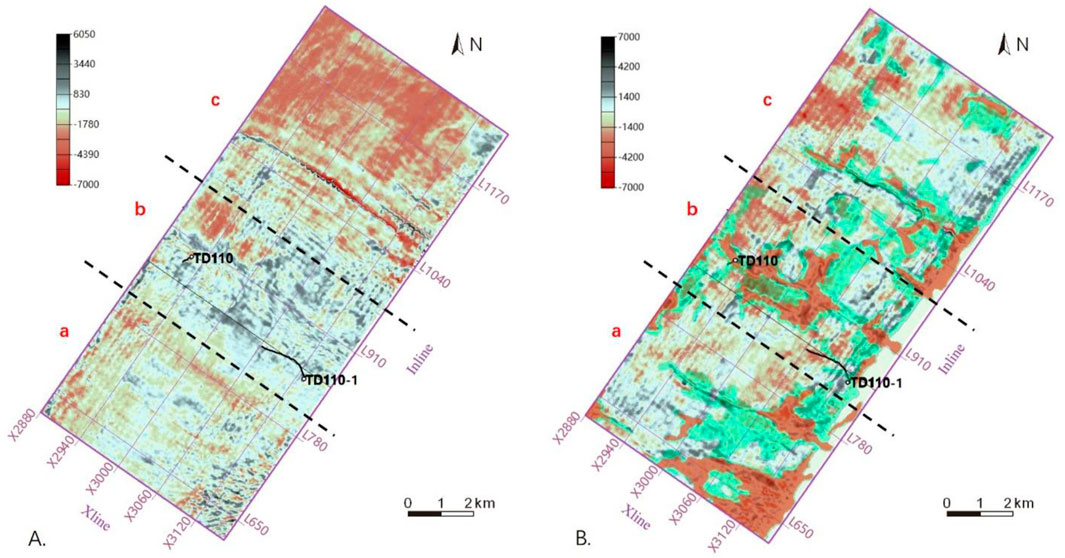
Figure 11. Horizon slice of (A) the base of the Triassic Feixianguan Formation (T1f1) and (B) the base of the first member of the Triassic Xujiahe Formation (T3x1), with detection results from the proposed method respectively.
6.2 Hydrocarbon enrichment in strike-slip fault zones
Strike-slip fault zones, due to their complex structural features and dynamic tectonic history, play a crucial role in the migration and accumulation of hydrocarbons. These fault zones, characterized by horizontal displacement and vertical continuity, can create favorable conditions for hydrocarbon entrapment, particularly when they intersect with porous reservoirs or act as barriers to fluid migration. In the Northeast Sichuan Basin, where large-scale reef and shoal reservoirs are present (Liu et al., 2017; Mo et al., 2019; Long et al., 2020), strike-slip fault zones are especially significant. Their influence on the distribution and development of these reservoirs may enhance the accumulation of hydrocarbons, providing critical pathways for fluid migration and trapping.
For example, the well TD110 is located in the platform margin of the KJ-LP Trough (Figure 1C), where gas production from the Changxing Formation reaches up to 493,800 m³/d. Imaging logging and core analysis reveal that the well contains well-developed fractures in multiple directions, including high-angle fractures (Figure 12A, B). Additionally, dissolution pores have formed along these fractures, some of which are filled with calcite (Figure 12C), indicating multi-period fault activity.
Detection using the G-SPF method identified a strike-slip fault zone intersecting with the wellbore. The fault zone, when analyzed in conjunction with the distribution of sedimentary facies, provides insight into the migration and accumulation of hydrocarbons. Specifically, the Changxing Formation in TD110 is located in a key position for hydrocarbons to migrate from the Permian-Triassic source rocks in the trough. The strike-slip fault zone not only serves as a conduit for the vertical migration of hydrocarbons from deeper source rocks but also facilitates lateral migration from the trough, creating a two-way hydrocarbon supply (Figure 13). This dual role of the fault zone enhances the connectivity between different reservoirs, ensuring more efficient hydrocarbon trapping and accumulation.
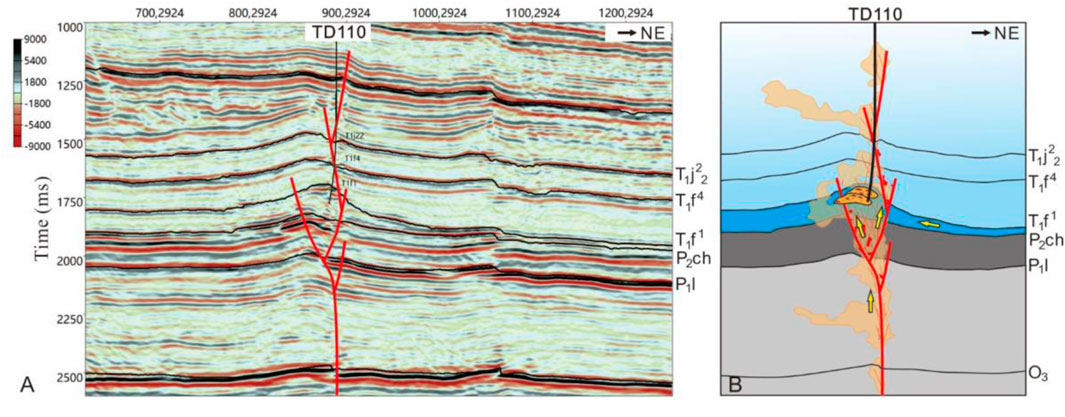
Figure 13. (A) Seismic Profile through Well TD110. (B) Hydrocarbon Accumulation Model of the Trough-Platform Margin Zone. Both the Lower Triassic source rocks in the trough and deeper underlying source rocks supply hydrocarbons to the platform margin zone.
In summary, the strike-slip fault zones in the Northeast Sichuan Basin play a critical role in the distribution and development of the Permian platform margin zones. These deep-seated strike-slip faults provide both structural traps and migration pathways for hydrocarbons, enhancing fluid accumulation and reservoir quality. As demonstrated by the well TD110, the presence of fractures, dissolution pores, and the intersection of fault zones with porous reservoirs make the area highly favorable for hydrocarbon migration and trapping, further solidifying its significance as a hydrocarbon reservoir.
7 Conclusion
(1) Forward modeling of strike-slip faults with varying fault throw widths confirms that fault response strengthens as the throw increases. Using 3D seismic data from the Northeast Sichuan Basin, we identify six distinct types of strike-slip fault seismic responses. Among these, five are associated with amplitude variations or vertical displacement, while the most challenging to detect is the flexure of events without displacement.
(2) Our proposed method demonstrates a significantly enhanced fault identification capability compared to using single attributes or the conventional variance method. It provides clearer fault zone boundaries and enables us to quickly distinguish areas with varying fault intensities.
(3) In the Northeast Sichuan Basin, the strike-slip zones in the platform margin act as migration pathways, connecting the underlying deep-source rocks and the Permian-Triassic source rocks within the trough to the reefs. This connectivity enables hydrocarbons generated from both sources to migrate along the faults toward the reefs, thereby facilitating hydrocarbon accumulation in these areas.
Data availability statement
The data analyzed in this study is subject to the following licenses/restrictions: The original contributions presented in the study are included in the article/supplementary material, further inquiries can be directed to the corresponding author. Requests to access these datasets should be directed to bGlzaXlhbzE5QDE2My5jb20=.
Author contributions
SL: Conceptualization, Formal Analysis, Methodology, Project administration, Software, Validation, Visualization, Writing–original draft, Writing–review and editing. XH: Funding acquisition, Project administration, Resources, Validation, Writing–review and editing. ST: Data curation, Methodology, Supervision, Writing–review and editing. GW: Resources, Writing–review and editing, Conceptualization, Supervision. LF: Writing–review and editing, Data curation, Resources. ML: Investigation, Methodology, Writing–review and editing.
Funding
The author(s) declare that financial support was received for the research, authorship, and/or publication of this article. This study was funded by the National Natural Science Foundation of China (grant no. 42304136).
Acknowledgments
The authors thank the Chongqing Division, and Petro-China Southwest Oil and Gas Field Company for providing the seismic data used in this study.
Conflict of interest
Author LF was employed by Petro-China Southwest Oil and Gas Field Company.
The remaining authors declare that the research was conducted in the absence of any commercial or financial relationships that could be construed as a potential conflict of interest.
Generative AI statement
The author(s) declare that no Generative AI was used in the creation of this manuscript.
Publisher’s note
All claims expressed in this article are solely those of the authors and do not necessarily represent those of their affiliated organizations, or those of the publisher, the editors and the reviewers. Any product that may be evaluated in this article, or claim that may be made by its manufacturer, is not guaranteed or endorsed by the publisher.
References
Brito, L. S. B., Alaei, B., Torabi, A., Leopoldino-Oliveira, K. M., Lino Vasconcelos, D., Bezerra, F. H. R., et al. (2023). Automatic 3D fault detection and characterization — a comparison between seismic attribute methods and deep learning. Interpretation 11, T793–T808. doi:10.1190/INT-2023-0016.1
Caselles, V., Kimmel, R., and Sapiro, G. (1997). Geodesic active contours. Int. J. Comput. Vis. 22, 61–79. doi:10.1023/a:1007979827043
Chan, T. F., and Vese, L. A. (2001). Active contours without edges. IEEE Trans. Image Process. 10, 266–277. doi:10.1109/83.902291
Chester, F. M., Evans, J. P., and Biegel, R. L. (1993). Internal structure and weakening mechanisms of the san andreas fault. J. Geophys. Res. Solid Earth 98, 771–786. doi:10.1029/92JB01866
Choi, J.-H., and Kim, Y.-S. (2009). Fault zone classification and structural characteristics of the southern Yangsan fault in the Sangcheon-ri area, SE Korea. J. Geol. Soc. Korea 45, 9–28.
Cui, L., Wu, K., Liu, Q., Wang, D., Guo, W., Liu, Y., et al. (2022). Enhanced interpretation of strike-slip faults using hybrid attributes: advanced insights into fault geometry and relationship with hydrocarbon accumulation in Jurassic formations of the Junggar Basin. J. Petroleum Sci. Eng. 208, 109630. doi:10.1016/j.petrol.2021.109630
Deng, B., Liu, S., Yao, G., Liao, Y., Zhang, B., Zhang, H., et al. (2024). Distribution pattern and main controlling factors of Paleozoic giant- and medium-sized gas fields of the Sichuan super gas basin in Southwest China. Nat. Gas. Ind. 44, 54–76. doi:10.3787/j.issn.1000-0976.2024.07.005
Fang, J., Liu, H., Zhang, L., Liu, J., and Liu, H. (2019). Active contour driven by weighted hybrid signed pressure force for image segmentation. IEEE Access 7, 97492–97504. doi:10.1109/ACCESS.2019.2929659
Feng, L., Jiang, Y., Liu, F., Gu, Y., Wang, Z., Zhong, K., et al. (2021). Reservoir characteristics and main controlling factors of oolitic shoal reservoir in Feixianguan Formation in the southern part of Kaijiang-Liangping trough, eastern Sichuan Basin. Acta Pet. Sin. 42, 1287–1298. doi:10.7623/syxb202110003
Garland, J., Neilson, J., Laubach, S. E., and Whidden, K. J. (2012). Advances in carbonate exploration and reservoir AnalysisAdvances in carbonate exploration and reservoir analysis. London, United Kingdom: Geological Society of London.
Gu, Z., Lonergan, L., Zhai, X., Zhang, B., and Lu, W. (2021). The formation of the Sichuan Basin, south China, during the late ediacaran to early cambrian. Basin Res. 33, 2328–2357. doi:10.1111/bre.12559
Guo, J., Yang, R., Geng, F., Wang, L., Zhang, S., Wang, L., et al. (2024). Segmentation characteristics of strike-slip fault zone and its reservoir control mechanisms in the southwestern Tarim Basin. Front. Earth Sci. 12. doi:10.3389/feart.2024.1464924
He, D., Li, D., Zhang, G., Zhao, L., Fan, C., Lu, R., et al. (2011). Formation and evolution of multi-cycle superposed Sichuan Basin, China. Chin. J. Geol., 589–606. doi:10.3969/j.issn.0563-5020.2011.03.001
Huang, Y., and Wang, R. (2023). Characterization of natural fractures in tight sandstone reservoirs in Yuanba area, northern Sichuan Basin. Unconv. Resour. 3, 155–163. doi:10.1016/j.uncres.2023.03.001
Joussineau, G. d., Mutlu, O., Aydin, A., and Pollard, D. D. (2007). Characterization of strike-slip fault–splay relationships in sandstone. J. Struct. Geol. 29, 1831–1842. doi:10.1016/j.jsg.2007.08.006
Kass, M., Witkin, A., and Terzopoulos, D. (1988). Snakes: active contour models. Int. J. Comput. Vis. 1, 321–331. doi:10.1007/BF00133570
Kichenassamy, S., Kumar, A., Olver, P., Tannenbaum, A., and Yezzi, A. (1995). Proceedings of IEEE international conference on computer vision, 810–815.
Kim, Y.-S., Peacock, D. C. P., and Sanderson, D. J. (2004). Fault damage zones. J. Struct. Geol. 26, 503–517. doi:10.1016/j.jsg.2003.08.002
Li, C., Kao, C.-y., Gore, J. C., and Ding, Z. (2007). 2007 IEEE conference on computer vision and pattern recognition, 1–7.
Li, H., Liu, J., Zheng, M., Li, S., Long, H., Li, C., et al. (2024). The seismic identification of small strike-slip faults in the deep Sichuan Basin (SW China). PROCESSES 12, 1508. doi:10.3390/pr12071508
Li, Y., Sun, J., Wei, H., and Song, S. (2019). Architectural features of fault-controlled karst reservoirs in the Tahe oilfield. J. Petroleum Sci. Eng. 181, 106208. doi:10.1016/j.petrol.2019.106208
Liang, S., Li, Z., Zhang, W., and Gao, Y. (2023). The characteristics of strike-slip faults and their control on hydrocarbon distribution in deep carbonate reservoirs of the central Sichuan Basin. Front. Earth Sci. 11. doi:10.3389/feart.2023.1064835
Liu, C., Xie, Q., Wang, G., Song, Y., and Qi, K. (2016a). Dolomite origin and its implication for porosity development of the carbonate gas reservoirs in the Upper Permian Changxing Formation of the eastern Sichuan Basin, Southwest China. J. Nat. Gas Sci. Eng. 35, 775–797. doi:10.1016/j.jngse.2016.09.027
Liu, J., Wu, G., Tang, Q., Wu, Y., Zhang, W., and Zhao, Z. (2024). Effects of intracratonic Strike-slip Fault on the differentiation of carbonate microfacies: a case study of a permian platform margin in the Sichuan Basin (SW China). Acta Geol. Sin. - Engl. Ed. 98, 936–954. doi:10.1111/1755-6724.15203
Liu, M., Yang, W., Jin, H., Mo, W., Wu, S., and Su, N. (2017). Distribution of platform edge reefs and beach as well as their major controlling factors over the Changxing-Feixianguan formations in northeast Sichuan Basin, China. J. Nat. Gas Geoscience 2, 109–118. doi:10.1016/j.jnggs.2017.06.002
Liu, S., Sun, W., Song, J., Deng, B., Zhong, Y., Luo, C., et al. (2015). Tectonics-controlled distribution of marine petroleum accumulations in the Sichuan Basin, China. Earth Sci. Front. 22, 146–160. doi:10.13745/j.esf.2015.03.013
Liu, S., Wang, Y., Sun, W., Zhong, Y., Hong, H., Deng, B., et al. (2016b). Control of intracratonic sags on the hydrocarbon accumulations in the marine strata across the Sichuan Basin, China. J. Chengdu Univ. Technol. (Sci. Technol. Ed.) 43, 1–23. doi:10.3969/j.issn.1671-9727.2016.01.01
Liu, S., Yang, Y., Deng, B., Zhong, Y., Wen, L., Sun, W., et al. (2021). Tectonic evolution of the Sichuan Basin, southwest China. Earth-Science Rev. 213, 103470. doi:10.1016/j.earscirev.2020.103470
Li, Y., Allen, P. A., Densmore, A. L., and Qiang, X. (2003). Evolution of the longmen Shan foreland basin (western sichuan, China) during the late triassic indosinian orogeny. Basin Res. 15, 117–138. doi:10.1046/j.1365-2117.2003.00197.x
Long, S., You, Y., Jiang, S., Liu, G., Feng, Q., Gao, Y., et al. (2020). Integrated characterization of ultradeep reef-shoal reservoir architecture: a case study of the Upper Permian Changxing Formation in the giant Yuanba gas field, Sichuan Basin, China. J. Petroleum Sci. Eng. 195, 107842. doi:10.1016/j.petrol.2020.107842
Ma, B., Liang, H., Wu, G., Tang, Q., Tian, W., Zhang, C., et al. (2023). Formation and evolution of the strike-slip faults in the central Sichuan Basin, SW China. Petroleum Explor. Dev. 50, 373–387. doi:10.1016/S1876-3804(23)60394-5
Ma, Y., Mou, C., Tan, Q., and Yu, Q. (2006). A discussion on Kaijiang-Liangping ocean trough. Oil and Gas Geol. 27, 326–331. doi:10.11743/ogg20060306
Malladi, R., Sethian, J. A., and Vemuri, B. C. (1995). Shape modeling with front propagation: a level set approach. IEEE Trans. Pattern Analysis Mach. Intell. 17, 158–175. doi:10.1109/34.368173
Manzi, M. S. D., Cooper, G. R. J., Malehmir, A., and Durrheim, R. J. (2020). Improved structural interpretation of legacy 3D seismic data from Karee platinum mine (South Africa) through the application of novel seismic attributes. Geophys. Prospect. 68, 145–163. doi:10.1111/1365-2478.12900
Meng, Y., Chen, H., Luo, Y., Zhao, Y., Tang, D., and He, F. (2023). Architecture of intraplate strike-slip fault zones in the Yanchang Formation, Southern Ordos Basin, China: characterization and implications for their control on hydrocarbon enrichment. J. Struct. Geol. 170, 104851. doi:10.1016/j.jsg.2023.104851
Mo, W., Su, N., Wei, G., Yang, W., Liu, M., and Xing, F. (2019). Structural control, seismic expression, and types of reefs of rifting margins in the late Permian Changxing Age, Sichuan Basin, China. Arabian J. Geosciences 12, 788. doi:10.1007/s12517-019-4720-6
Neng, Y., Li, Y., Qi, J., Ma, X., Zuo, L., and Chen, P. (2022). Deformation styles and multi-stage evolution history of a large intraplate Strike-Slip Fault system in a paleozoic superimposed basin: a case study from the Tarim Basin, NW China. Front. Earth Sci. 10. doi:10.3389/feart.2022.837354
Ning, F., Lin, H., Zhou, C., Yun, J., Li, P., and Song, H. (2022). Characteristics of strike-slip fault-related reservoirs and the significance for hydrocarbon accumulation in the central Tarim Basin: insights from the modern karst model. Front. Earth Sci. 10. doi:10.3389/feart.2022.987020
Oliveira, L. S. B., Nogueira, F. C. C., Vasconcelos, D. L., Torabi, A., Alaei, B., Balsamo, F., et al. (2024). Seismic and outcrop-based 3D characterization of fault damage zones in sandstones, Rio do Peixe Basin, Brazil. J. Struct. Geol. 189, 105276. doi:10.1016/j.jsg.2024.105276
Pu, R., Li, K., Dong, M., Cao, Z., and Xu, P. (2018). The 3D seismic characteristics and significance of the strike-slip faults in the Tazhong area (Tarim Basin, China). Interpretation 7, T1–T19. doi:10.1190/INT-2016-0135.1
Ronfard, R. (1994). Region-based strategies for active contour models. Int. J. Comput. Vis. 13, 229–251. doi:10.1007/BF01427153
Shafiq, M. A., Wang, Z., and AlRegib, G. (2015). 2015 IEEE global conference on signal and information processing (GlobalSIP), 596–600.
Silva, T. A., Gomes, I. F., Miranda, T. S., Correia Filho, O. J., Medeiros, C. E. B., Carvalho, B. R. B. M., et al. (2024). Quantifying damage zones width in strike-slip faults: insights from a two-dimensional finite-element modeling approach. J. Struct. Geol. 186, 105201. doi:10.1016/j.jsg.2024.105201
Sun, C., Shen, F., Wen, Z., Zhang, Y., Scarselli, N., Li, S., et al. (2023). Seismic analysis of fault damage zones in the northern Tarim Basin (NW China): implications for growth of ultra-deep fractured reservoirs. J. Asian Earth Sci. 255, 105778. doi:10.1016/j.jseaes.2023.105778
Tang, Q., Tang, S., Luo, B., Luo, X., Feng, L., Li, S., et al. (2022). Seismic description of deep Strike-Slip Fault damage zone by steerable pyramid method in the Sichuan Basin, China. Energies 15, 8131. doi:10.3390/en15218131
Tian, F., Zheng, W., Zhao, A., Liu, J., Liu, Y., Zhou, H., et al. (2024). Seismic identification and characterization of deep strike-slip faults in the Tarim craton basin. Appl. Sci. 14, 8235. doi:10.3390/app14188235
Torabi, A., Balsamo, F., Nogueira, F. C. C., Vasconcelos, D. L., Silva, A. C. E., Bezerra, F. H. R., et al. (2021). Variation of thickness, internal structure and petrophysical properties in a deformation band fault zone in siliciclastic rocks. Mar. Petroleum Geol. 133, 105297. doi:10.1016/j.marpetgeo.2021.105297
Torabi, A., Ellingsen, T. S. S., Johannessen, M. U., Alaei, B., Rotevatn, A., and Chiarella, D. (2020). “Fault zone architecture and its scaling laws: where does the damage zone start and stop?,” in Integrated Fault seal analysis (London, United Kingdom: Geological Society of London), 496, 0.
Torabi, A., Johannessen, M. U., and Ellingsen, T. S. S. (2019). Fault core thickness: insights from siliciclastic and carbonate rocks. Geofluids 2019, 1–24. doi:10.1155/2019/2918673
Wang, Q., Zhang, Y., Xie, Z., Zhao, Y., Zhang, C., Sun, C., et al. (2022). The advancement and challenges of seismic techniques for ultra-deep carbonate reservoir exploitation in the Tarim Basin of northwestern China. Energies 15, 7653. doi:10.3390/en15207653
Wang, X., Li, B., Yang, X., Wen, L., Xu, L., Xie, S., et al. (2021). Characteristics of “Guangyuan-Wangcang” trough during late middle permian and its petroleum geological significance in northern Sichuan Basin, SW China. Petroleum Explor. Dev. 48, 655–669. doi:10.1016/S1876-3804(21)60052-6
Wei, G., Liu, D., Zhang, L., Yang, W., Jin, H., Wu, S., et al. (2005). The exploration region and natural gas accmulation in sichuan basin. Nat. Gas. Geosci. 16, 437–442. doi:10.11764/j.issn.1672-1926.2005.04.437
Wu, S., Wei, G., Yang, W., Duan, S., Jin, H., Xie, W., et al. (2019). Development characteristics of reefs on the platform margin of Changxing Formation in eastern Kaijiang-Liangping ocean trough and its significance for petroleum geological exploration. China Pet. Explor. 24, 457–465. doi:10.3969/j.issn.1672-7703.2019.04.006
Wu, Y., Liu, J., Feng, L., Pang, Y., Tang, Q., Lliu, X., et al. (2023). Characteristics of the strike-slip faults and their effects on the gas accumulation in the southeastern Kaijiang-Liangping trough,Sichuan Basin. Mar. Orig. Pet. Geol. 28. doi:10.3969/j.issn.1672-9854.2023.03.007
Wu, Y., Tao, X., Zhao, Z., Tang, Q., Huang, T., Liu, J., et al. (2024). Microspectrometer-enabled real-time concentration monitoring in the microfluidic protein enrichment chip. Geol. Bull. China 15, 1–11. doi:10.3390/bios15010001
Xu, S., Xia, M., Zhu, Y., Li, T., and Lin, Y. (2023). Evolution stage and tectonic sedimentary characteristics of the Kaijiang-Liangping Trough. Fault-Block Oil and Gas Field 30. doi:10.6056/dkyqt202306011
Yang, Y., Wen, L., Zhou, G., Zhan, W., Li, H., Song, Z., et al. (2023). New fields, new types and resource potentials of hydrocarbon exploration in Sichuan Basin. Shiyou Xuebao/Acta Pet. Sin. 44, 2045–2069. doi:10.7623/syxb202312004
Yuan, H., Chen, S., Neng, Y., Zhao, H., Xu, S., Wang, X., et al. (2021). Composite strike-slip deformation belts and their control on oil and gas reservoirs: a case study of the northern part of the shunbei 5 strike-slip deformation belt in Tarim Basin, northwestern China. Front. Earth Sci. 9. doi:10.3389/feart.2021.755050
Zalán, P. (1987). Identification of strike-slip faults in seismic sections. Proc. Am. Assoc. Pet. Geol. Annu. Meet. 1988, 116–118. doi:10.1190/1.1892142
Zeng, L., Shi, J., Ma, Q., Lyu, W., Dong, S., Cao, D., et al. (2024). Strike-slip fault control on karst in ultra-deep carbonates, Tarim Basin, China. AAPG Bull. 108, 235–260. doi:10.1306/09212321161
Zhang, K., Zhang, L., Song, H., and Zhou, W. (2010). Active contours with selective local or global segmentation: a new formulation and level set method. Image Vis. Comput. 28, 668–676. doi:10.1016/j.imavis.2009.10.009
Keywords: strike-slip fault zones, seismic response, gradient-driven signed pressure force model, two-step iterative extraction, the Northeast Sichuan Basin
Citation: Li S, Huang X, Tang S, Wu G, Feng L and Li M (2025) Characterization of strike-slip fault zones by gradient-driven signed pressure force model in the Northeast Sichuan Basin. Front. Earth Sci. 13:1540415. doi: 10.3389/feart.2025.1540415
Received: 05 December 2024; Accepted: 31 January 2025;
Published: 26 February 2025.
Edited by:
Ahmed Henaish, Zagazig University, EgyptReviewed by:
David Vasconcelos, Federal University of Campina Grande, BrazilQing Chen, Chongqing University of Science and Technology, China
Copyright © 2025 Li, Huang, Tang, Wu, Feng and Li. This is an open-access article distributed under the terms of the Creative Commons Attribution License (CC BY). The use, distribution or reproduction in other forums is permitted, provided the original author(s) and the copyright owner(s) are credited and that the original publication in this journal is cited, in accordance with accepted academic practice. No use, distribution or reproduction is permitted which does not comply with these terms.
*Correspondence: Siyao Li, bGlzaXlhbzE5QDE2My5jb20=