- 1Guangxi Key Laboratory of Forest Ecology and Conservation, College of Forestry, Guangxi University, Nanning, China
- 2Key Laboratory of Environment Change and Resources Use in Beibu Gulf, Ministry of Education, Nanning Normal University, Nanning, China
- 3Guangxi Key Laboratory of Earth Surface Processes and Intelligent Simulation, Nanning Normal University, Nanning, China
- 4Laibin Jinxiu Dayaoshan Forest Ecosystem Observation and Research Station of Guangxi, Laibin, China
Introduction: Altitude-induced variations in hydrothermal conditions and vegetation affect the carbon (C), nitrogen (N), and phosphorus (P) contents in both soil and microbial biomass. However, the specific patterns of changes in these nutrients and their stoichiometry in response to altitudinal changes remain unclear.
Methods: In this study, we analyzed the altitudinal patterns of soil and microbial biomass C:N:P stoichiometry across three soil layers (0–20, 20–40, and 40–60 cm) in the subtropical mountain ecosystems on Daming Mountain, South China.
Results: Our results revealed that soil C content and C:N ratio in the 0–20 cm layer initially increased and then decreased with increasing altitude. Meanwhile, soil P content in the 40–60 cm layer decreased. Soil microbial biomass P content initially decreased and then increased with altitude across all soil layers, whereas soil microbial biomass C:P and N:P ratios exhibited opposite trends. For soil and microbial biomass C:P and N:P ratios along the altitudinal gradient, soil microbial growth was mainly restricted by P, with this limitation being more pronounced at mid-to-low altitudes. Soil microbial biomass C, N, and P contents were positively correlated with soil C, N, and P contents and their ratios, available N (AN), available P (AP), and soil water content (SWC), but negatively correlated with soil bulk density, soil pH and soil temperature. In contrast, microbial biomass C, N, and P stoichiometric ratios exhibited the opposite or non-significant relationships with these factors. Notably, AN, AP, and SWC were the dominant factors affecting soil microbial biomass C:N:P stoichiometry.
Discussion: These findings contribute to the understanding of soil nutrient stoichiometry and their responses to environmental changes in subtropical mountain ecosystems.
1 Introduction
Ecological stoichiometry focuses on the dynamic balance of energy and multiple chemical elements within ecosystems (Elser et al., 2000a; Elser et al., 2000b). It reveals how organisms respond to external resources and the mechanisms underlying nutrient supply and balance in ecological processes. This is primarily achieved by scaling up carbon (C), nitrogen (N), and phosphorus (P) (Koerselman and Meuleman, 1996; Wang et al., 2018), which are the most essential elements in living organisms. The ecological stoichiometry of these elements can reflect nutrient cycling and coupling relationships across different components (Hobbie et al., 2002; Schindler, 2003). Soil and microorganisms play crucial roles in biogeochemical cycles (Li et al., 2021). Soil C, N, and P contents are the nutrient bases for plant growth. Their stoichiometric ratios are key indicators affecting soil organic matter mineralization and the effectiveness of soil microbial biomass (Paul, 2007; Qiao et al., 2020; Wang et al., 2021). Moreover, soil microorganisms act as decomposers of plant and soil nutrients. Their biomass C, N, and P contents typically refer to the total C, N, and P contents in microorganisms smaller than 5,000 μm3 (Brookes, 2001). Soil microbial biomass can reflect soil quality changes, influencing the cycles of soil materials and the energy flows driven by microorganisms (Falkowski et al., 2008; Gao et al., 2022). Therefore, the stoichiometric relationships between soil nutrients and microbial biomass can effectively reflect the structure and function of biogeochemical cycles in soil ecosystems (Li et al., 2012).
Mountain ecosystems have integral ecological processes due to their highly heterogeneous environments determined by climate and topography (Christmann and Menor, 2021; Zhang et al., 2021; Nandy et al., 2022; Zhao et al., 2025). In particular, altitude is a key topographic factor that has received considerable attention in research on responses to environmental changes (Beniston, 2003; Jiang et al., 2019; Hou et al., 2021). Environmental shifts, including temperature, precipitation, and light at different altitudes, exert direct or indirect impacts on the distribution of soil nutrients and microbial biomass by regulating soil physicochemical properties (Körner, 2007; Rahbek et al., 2019; He et al., 2020). Soil and microbial biomass ecological stoichiometry have been widely studied across large-scale latitude and longitude patterns, revealing general biogeographic patterns (Pan et al., 2021; Gao et al., 2022; Wan et al., 2023; Feng et al., 2024). However, variations in soil and microbial biomass stoichiometry along altitudinal gradients remain unclear, likely due to differences in geographical location, altitude range, and vegetation types (Chang et al., 2016; He et al., 2016; Liu et al., 2021; Xiong et al., 2022; Jia et al., 2023; Tong et al., 2023; Zhang et al., 2023; Zhu et al., 2024). Some studies have demonstrated that soil and microbial biomass C, N, and P contents increase with rising altitude (Chang et al., 2016; Liu et al., 2021; Xiong et al., 2022; Jia et al., 2023). However, in other studies, the opposite trend was observed in soil C, N, and P stoichiometric ratios (Tong et al., 2023), as well as in microbial biomass C and P contents (Zhang et al., 2023). In addition, non-linear relationships have been detected in the altitudinal patterns of soil and microbial biomass stoichiometry (He et al., 2016; Zhu et al., 2024). For example, soil and microbial biomass P content initially decreased and then increased, while the N:P ratio exhibited the opposite trend with increasing altitude on Dinghu Mountain (He et al., 2016) and Wuyi Mountain (Zhu et al., 2024). Therefore, further studies are needed to better understand the general patterns of soil and microbial biomass stoichiometry along altitudinal gradients and identify the important factors influencing these patterns.
To date, most studies on the altitudinal patterns of soil and microbial biomass C, N, and P stoichiometry have primarily focused on the topsoil (He et al., 2016; Chen et al., 2024; Zhu et al., 2024), with relatively few studies on the subsoil (Zuo et al., 2021; Huang et al., 2023; Wu et al., 2024). Soil less than 20 cm in depth is usually regarded as topsoil. The subsoil, which lies below 20 cm in depth, stores more than 50% of the total global soil C storage (Rumpel and Kögel-Knabner, 2011). The significantly different soil environment conditions—such as temperature, moisture, porosity, and pH—in the topsoil and subsoil lead to variations in soil nutrients and microbial biomass (Jobbágy and Jackson, 2000; Chen et al., 2022). Soil nutrients and microbial biomass in different soil layers may respond differently to altitude. In the topsoil, soil P content increases with increasing altitude, while soil N content increases in the subsoil (Wu et al., 2024). In contrast, microorganisms remain relatively stable at different altitudes owing to low subsoil nutrient availability (Zuo et al., 2021). However, some studies have indicated consistent responses of soil and microbial biomass nutrients to altitude across different soil layers (Zhao et al., 2019; Huang et al., 2023). Although previous studies have elucidated the responses of soil nutrients and microbial biomass to altitude in different soil layers, research focusing on subtropical mountain ecosystems remains limited. Therefore, using an altitudinal gradient to examine soil nutrients and microbial biomass responses to environmental changes while considering soil layer variations can provide a more comprehensive understanding of nutrient dynamics and ecosystem functioning in subtropical mountain ecosystems.
Daming Mountain has the typical monsoon evergreen broad-leaved forests found in southern China. These forests are relatively well-preserved, with high plant diversity and characteristic vertical zonation (Li J. L. et al., 2023; Li Y. F. et al., 2023). Previous studies have explored soil properties along the altitudinal gradient on this mountain. For example, Zhao et al. (2013) found that soil organic matter increased with altitude across different soil layers (0–20, 20–40, and 40–60 cm). In contrast, total N and P contents exhibited a pattern of initial decrease followed by an increase in the middle and lower soil layers. This pattern was mainly attributed to variations in vegetation types, temperature, and precipitation at different altitudes. However, research on the stoichiometry of C, N, and P in microbial biomass across soil layers, as well as their relationships to soil properties, remains limited.
Therefore, in the present study, we established forest plots along altitudinal gradients on Daming Mountain and analyzed the C, N, and P stoichiometric characteristics of soil and microbial biomass across altitudinal gradients in different soil layers. We aimed to (1) examine the altitudinal patterns of C, N, and P contents and their ratios in soil and microbial biomass across different soil layers and (2) identify the dominant factors influencing the C, N, and P stoichiometric characteristics of microbial biomass. The findings will enhance our understanding of the mechanisms and ecological implications of soil and microbial biomass C, N, and P stoichiometric characteristics responses to changes in altitude. This study also offers a theoretical basis for determining the soil C, N, and P cycling processes in subtropical mountain ecosystems and their regulatory mechanisms.
2 Materials and methods
2.1 Study area
The study area was located in Daming Mountain, Nanning City, Guangxi, South China (23°27′–23°32′N, 108°22′–108°27′E) (Figure 1). The Tropic of Cancer crosses the mountain, indicating that the area is located in a subtropical monsoon climate zone. The region has an annual average temperature of 15.1°C, with a maximum temperature of 21.9°C and a minimum temperature of 5.8°C. The annual average precipitation is 2,630.3 mm, with approximately 50% of the total occurring from June to August (Hu et al., 2025). The average annual sunshine duration ranges from 1,295.4 to 1,665.1 h, and the annual average relative humidity is 90%. The altitude ranges from 115 to 1,760 m. The zonal vegetation is characterized by south subtropical monsoon evergreen broad-leaved forests, with dominant species including Clethra bodinieri, Engelhardia roxburghiana, Rhodoleia championii, Symplocos lancifolia, and Machilus thunbergii. The soil types are predominantly red and yellow soil, with the soil pH between 3.9 and 4.7 (Li J. L. et al., 2023; Li Y. F. et al., 2023; Hu et al., 2025).
2.2 Sample collection and analysis
In July 2023, eight altitudinal gradients were selected from 300 to 1,400 m (Figure 1), with the location, slope aspect, slope degree, forest types, soil types, and dominant tree taxa from each altitude summarized in Table 1. At each altitude, three 20 × 20 m plots were established, with a minimum distance of 100 m between plots, totaling 24 plots. Soil samples were collected from each plot using the five-point method from three layers: 0–20 cm (topsoil), 20–40 cm (sub-topsoil), and 40–60 cm (subsoil) in depth. Soil samples from the same layer were mixed uniformly to form a single composite sample, comprising a total of 72 samples (24 plots × 3 soil layers). The collected soil samples were transported back to the laboratory in a 4°C cooler. Plant roots and stones were removed, and the samples were sieved through a 2 mm mesh. Each soil layer sample was divided into two portions. One portion (fresh soil) was used for measuring the microbial biomass C, N, and P contents, while the other portion was air-dried for determining soil C, N, and P contents and physicochemical properties. Intact soil samples were collected from each plot using a ring cutter to measure soil water content (SWC) and bulk density (SBD). In addition, soil moisture and temperature data loggers were installed in each plot to monitor soil temperature (ST).
ST was measured at a soil depth of 8 cm using TMS-3 data loggers (TOMST s.r.o., Prague, Czech Republic) (Wild et al., 2019). SWC was determined using the oven-drying method, while SBD was measured using the ring knife method. Soil pH was measured with a pH meter (soil:water ratio of 1:2.5) (Bao, 2000). Soil organic carbon (SOC) content was measured using the acidified dichromate (K2Cr2O7-H2SO4) oxidation-external heating method (Nelson and Sommers, 1982). Soil total nitrogen (STN) content was determined using the micro-Kjeldahl digestion method (Bremner, 1996). Available nitrogen (AN) content was extracted with 2 M KCl solution and then measured using a continuous flow analyzer (San++System, Skalar, Holland) (Keeney and Nelson, 1982). Soil total phosphorus (STP) content was determined using the molybdenum-antimony anti-colorimetric method (Olsen and Sommers, 1982). Available phosphorus (AP) content was determined using ultraviolet spectrophotometry after extraction with NaHCO3 solution (Olsen et al., 1954). Soil microbial biomass carbon (MBC), nitrogen (MBN), and phosphorus (MBP) were measured using the chloroform fumigation-extraction method (Brookes et al., 1982; Vance et al., 1987).
2.3 Data analysis
Before analysis, data normality and homogeneity of variance were tested using the Kolmogorov–Smirnov and Levene’s tests, respectively. A one-way analysis of variance (ANOVA) and post hoc Duncan’s test were performed to examine the differences in C, N, and P stoichiometric characteristics of soil and microbial biomass and physicochemical properties across different soil layers. Regression analysis was conducted to evaluate the altitudinal patterns of soil and microbial biomass C, N, and P stoichiometric characteristics and physicochemical properties. Specifically, quadratic regression models were applied to assess the relationships between altitude and each variable, with altitude serving as the independent variable and the stoichiometric characteristics or physicochemical properties as dependent variables. Pearson’s correlation analysis was used to determine the relationships between soil and microbial biomass C, N, and P stoichiometric characteristics and physicochemical properties. The correlation coefficients (r) were calculated to quantify the strength and direction of these relationships. Redundancy analysis (RDA) was used to identify the primary factors influencing the C, N, and P stoichiometric characteristics of soil microbial biomass. Statistical analyses were conducted with SPSS 26.0 (IBM, Chicago, IL, USA). Regression, correlation, and redundancy analyses were performed using the “stats,” “psych,” and “vegan” packages in R (R Core Team, version 4.3.1), respectively.
3 Results
3.1 Responses of soil physicochemical properties to altitude and soil depth
With increasing altitude, AN did not change significantly across all layers, whereas AP decreased first and then increased significantly in all layers. In the 0–20 cm layer, SWC and SBD increased and decreased significantly, respectively. Soil pH increased significantly with increasing altitude in the 20–40 and 40–60 cm layers (Figure 2). Additionally, ST decreased significantly with increasing altitude (Supplementary Figure S1).
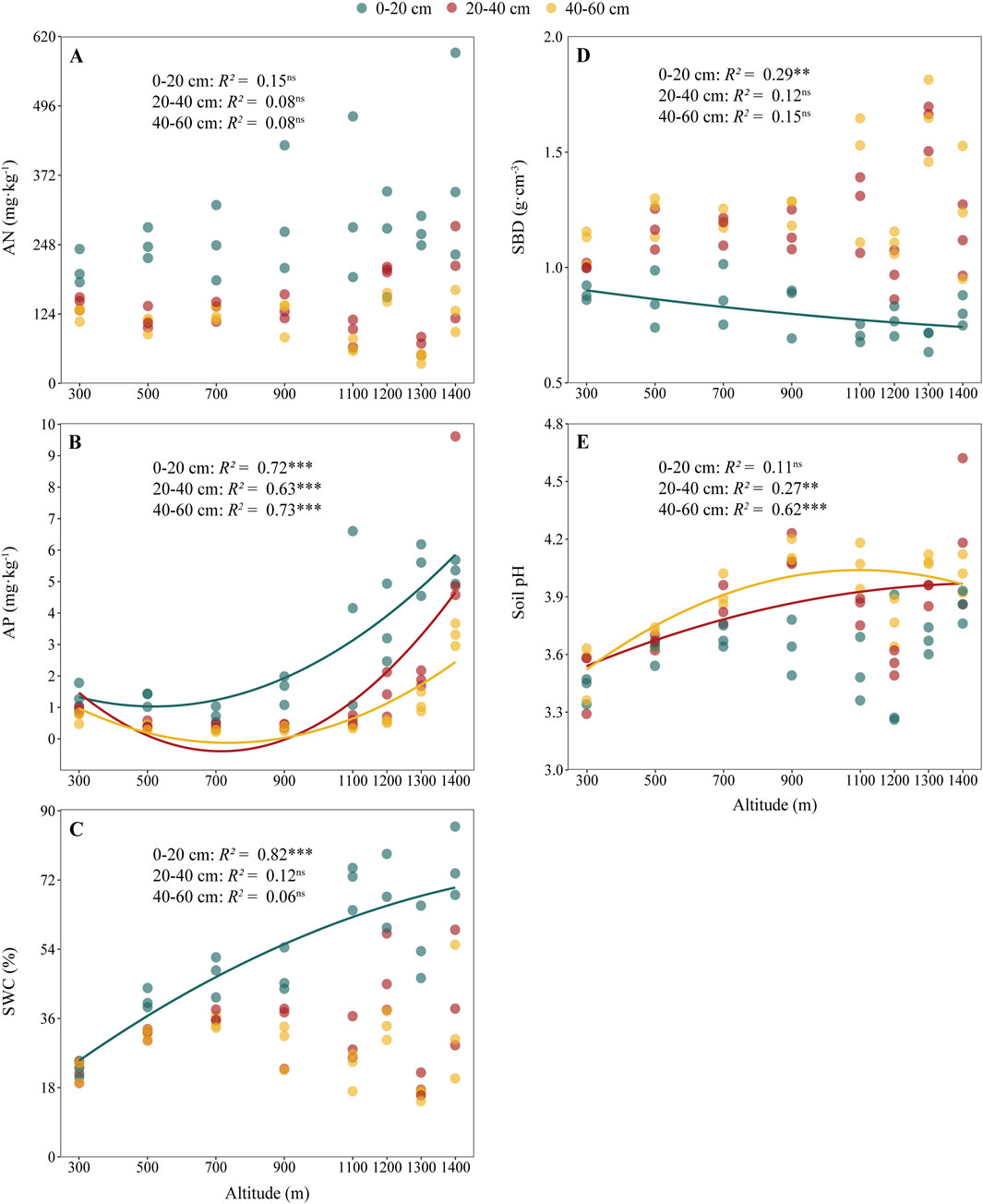
Figure 2. Variations in soil physicochemical properties at different altitudes. (A) AN, available nitrogen; (B) AP, available phosphorous; (C) SWC, soil water content; (D) SBD, soil bulk density; (E) Soil pH. nsp > 0.05, **p < 0.01, ***p < 0.001.
With increasing soil layer, AN, AP, and SWC decreased significantly, whereas SBD and pH increased significantly (Table 2). The highest AN, AP, and SWC were noted in the 0–20 cm layer, with mean values of 279.95 ± 20.56, 2.9 ± 0.42 mg kg−1, and 53.49 ± 3.63%, respectively. The highest SBD and pH were observed in the 40–60 cm layer, with mean values of 1.28 ± 0.04 g cm−3 and 3.9 ± 0.05, respectively.
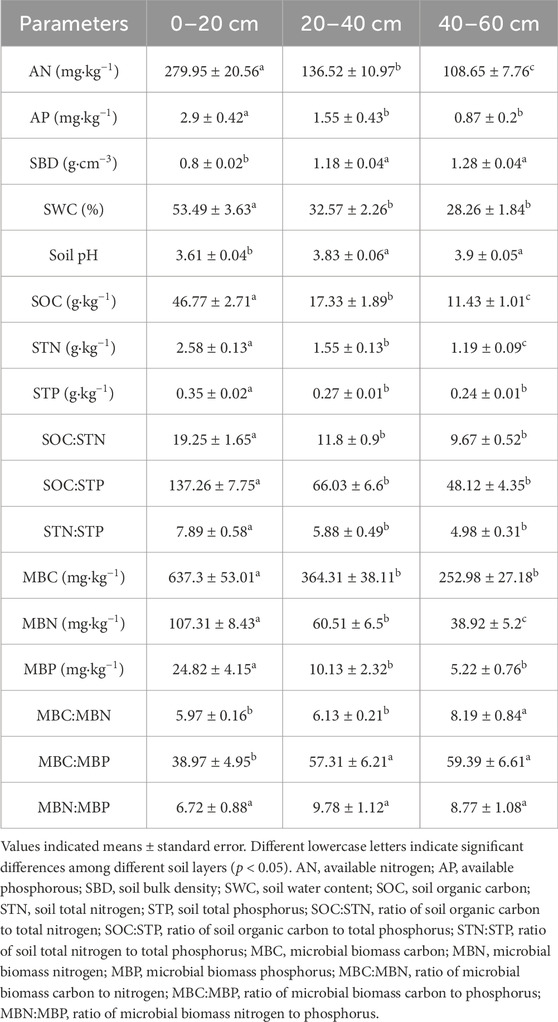
Table 2. Differences in soil and microbial biomass C, N, and P stoichiometric characteristics and physicochemical properties across different soil layers.
3.2 Responses of soil C, N, and P stoichiometric characteristics to altitude and soil depth
STN, the SOC:STP ratio, and the STN:STP ratio did not change significantly with increasing altitude across all soil layers. In the 0–20 cm layer, SOC and the SOC:STN ratio initially increased and then decreased significantly with altitude. With increasing altitude, STP in the 40–60 cm layer decreased, while the SOC:STN ratio in the 20–40 cm layer increased significantly (Figure 3).
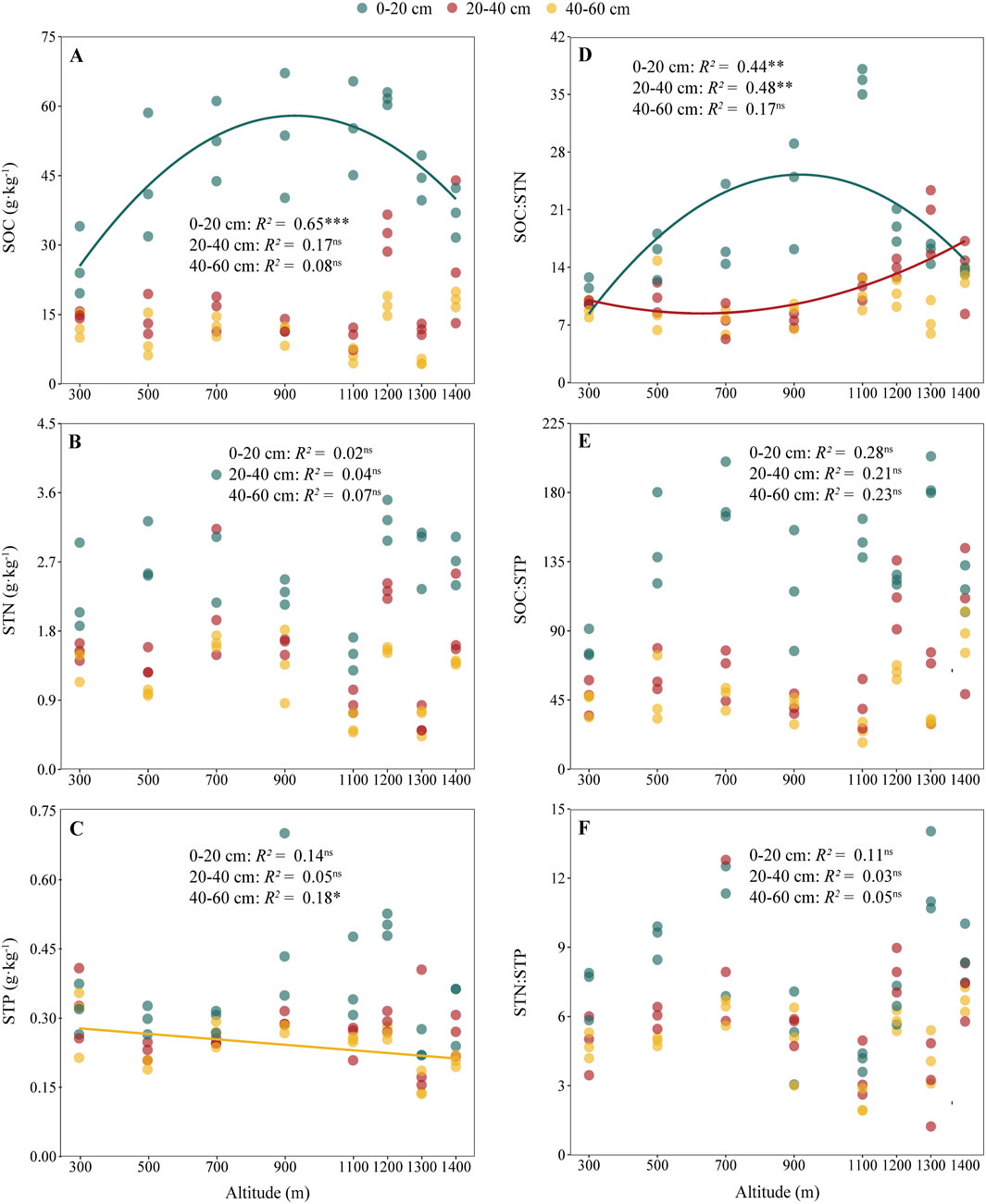
Figure 3. Variations in soil C, N, and P contents and their ratios at different altitudes. (A) SOC, soil organic carbon; (B) STN, soil total nitrogen; (C) STP, soil total phosphorus; (D) SOC:STN, ratio of soil organic carbon to total nitrogen; (E) SOC:STP, ratio of soil organic carbon to total phosphorus; (F) STN:STP, ratio of soil total nitrogen to total phosphorus. nsp > 0.05, *p < 0.05, **p < 0.01, ***p < 0.001.
With the increase in soil layer, soil C, N, and P contents and their ratios all decreased significantly. The highest value was recorded in the 0–20 cm layer (Table 2). The mean values of SOC, STN, and STP were 46.77 ± 2.71, 2.58 ± 0.13, and 0.35 ± 0.02 g kg−1, respectively. The SOC:STN, SOC:STP, and STN:STP ratios were 19.25 ± 1.65, 137.26 ± 7.75, and 7.89 ± 0.58, respectively.
3.3 Responses of soil microbial biomass C, N, and P stoichiometric characteristics to altitude and soil depth
MBC and MBN exhibited no significant differences with increasing altitude across all soil layers. MBP in all layers and the MBC:MBN ratio in the 40–60 cm layer initially decreased and then increased significantly with altitude, whereas the MBC:MBP and MBN:MBP ratios exhibited an opposite trend across all layers (Figure 4).
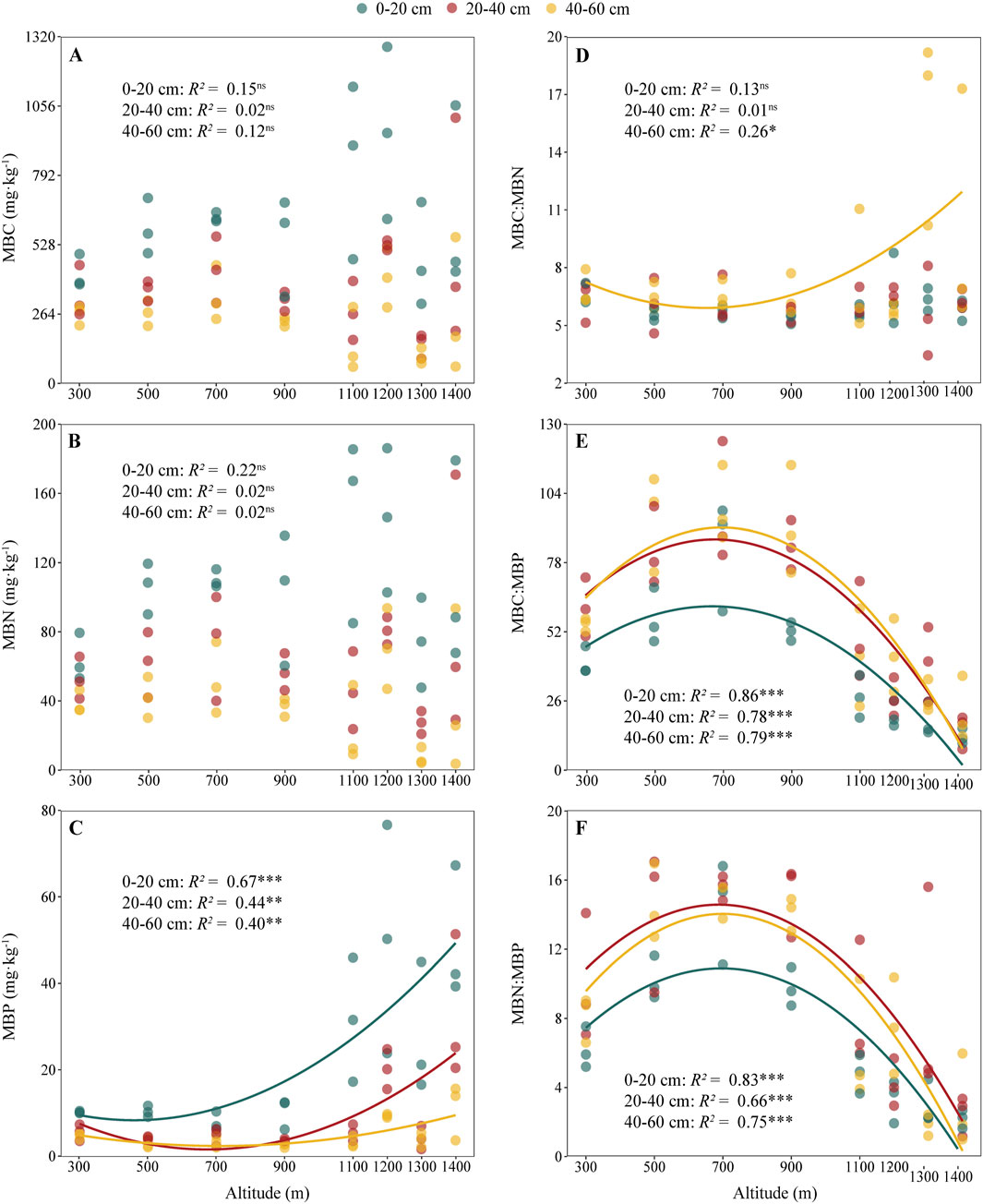
Figure 4. Variations in microbial biomass C, N, and P contents and their ratios at different altitudes. (A) MBC, microbial biomass carbon; (B) MBN, microbial biomassnitrogen; (C) MBP, microbial biomass phosphorus; (D) MBC:MBN, ratio of microbial biomass carbon to nitrogen; (E) MBC:MBP, ratio of microbial biomass carbon to phosphorus; (F) MBN:MBP, ratio of microbial biomass nitrogen to phosphorus. nsp > 0.05, *p < 0.05, **p < 0.01, ***p < 0.001.
As soil depth increased, soil microbial biomass C, N, and P contents decreased significantly while their ratios increased (Table 2). The highest MBC, MBN, and MBP values were observed in the 0–20 cm layer, with mean values of 637.3 ± 53.01, 107.31 ± 8.43, and 24.82 ± 4.15 mg kg−1, respectively. The highest MBC:MBN and MBC:MBP ratios were detected in the 40–60 cm layer, with mean values of 8.19 ± 0.84 and 59.39 ± 6.61, respectively. The highest mean value of MBN:MBP was 9.78 ± 1.12 observed in the 20–40 cm layer.
3.4 Relationships between soil properties and microbial biomass stoichiometry
Soil microbial C, N, and P contents were positively correlated with soil C, N, and P contents and their ratios, AN, AP, and SWC. However, they were negatively correlated with SBD and soil pH. MBP was significantly negatively correlated with ST. The MBC:MBP and MBN:MBP ratios were strongly positively correlated. These ratios were significantly negatively correlated with MBP, the SOC:STN ratio, the SOC:STP ratio, AP, and SWC and highly positively correlated with ST. The MBC:MBN ratio was significantly negatively correlated with MBC, MBN, the MBN:MBP ratio, AN, SWC, and soil C, N, and P contents, and significantly positively correlated with SBD and pH (Figure 5).
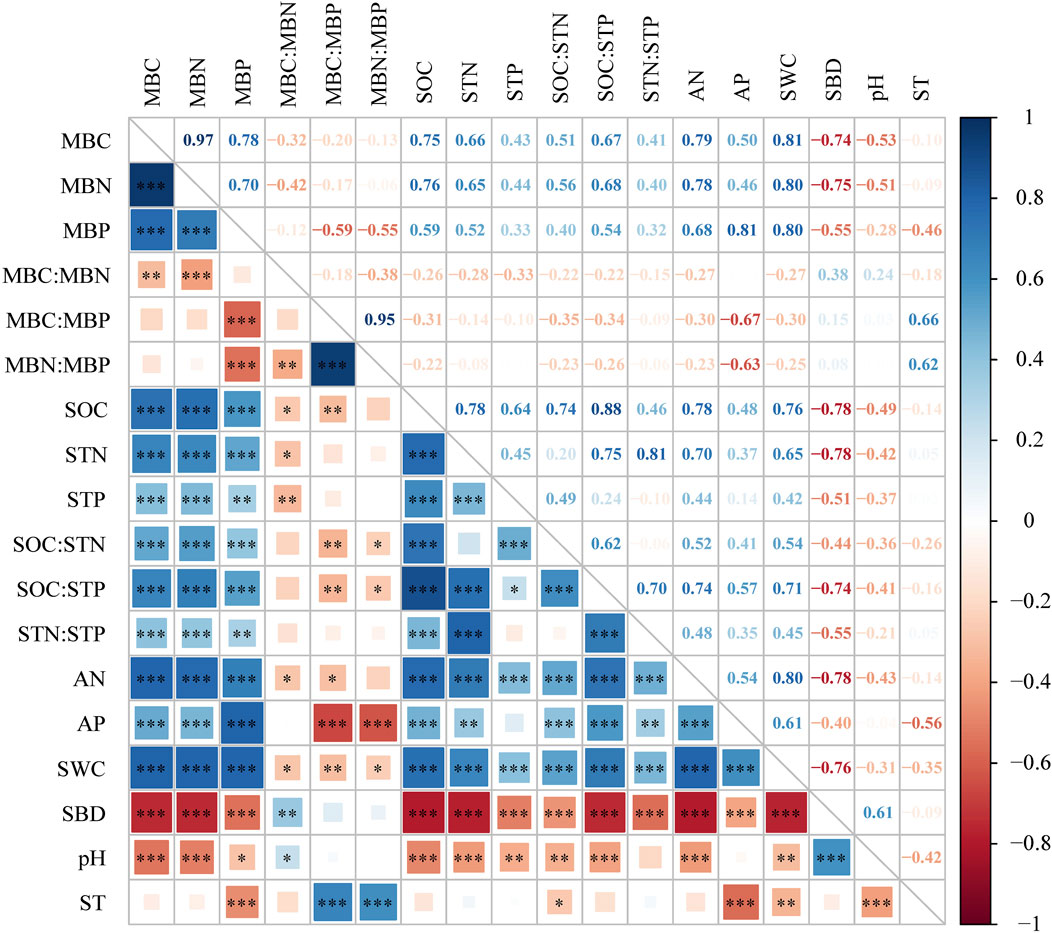
Figure 5. Correlation analysis between soil and microbial biomass C, N, and P stoichiometric characteristics and physicochemical properties. SOC, soil organic carbon; STN, soil total nitrogen; STP, soil total phosphorus; SOC:STN, ratio of soil organic carbon to total nitrogen; SOC:STP, ratio of soil organic carbon to total phosphorus; STN:STP, ratio of soil total nitrogen to total phosphorus; MBC: microbial biomass carbon; MBN: microbial biomass nitrogen; MBP, microbial biomass phosphorus; MBC:MBN, ratio of microbial biomass carbon to nitrogen; MBC:MBP, ratio of microbial biomass carbon to phosphorus; MBN:MBP, ratio of microbial biomass nitrogen to phosphorus; AN, available nitrogen; AP, available phosphorous; SWC, soil water content; SBD, soil bulk density; ST, soil temperature. *p < 0.05, **p < 0.01, ***p < 0.001.
The RDA revealed that the first two axes explained approximately 65.91% of the total variation (Figure 6). Environmental factors had a significant impact on microbial biomass C, N, and P stoichiometric characteristics. Moreover, SWC, AP, and AN were identified as the factors that explained the greatest amount of variance, accounting for 72.0%, 65.1%, and 64.3% of the variance, respectively (Supplementary Table S1).
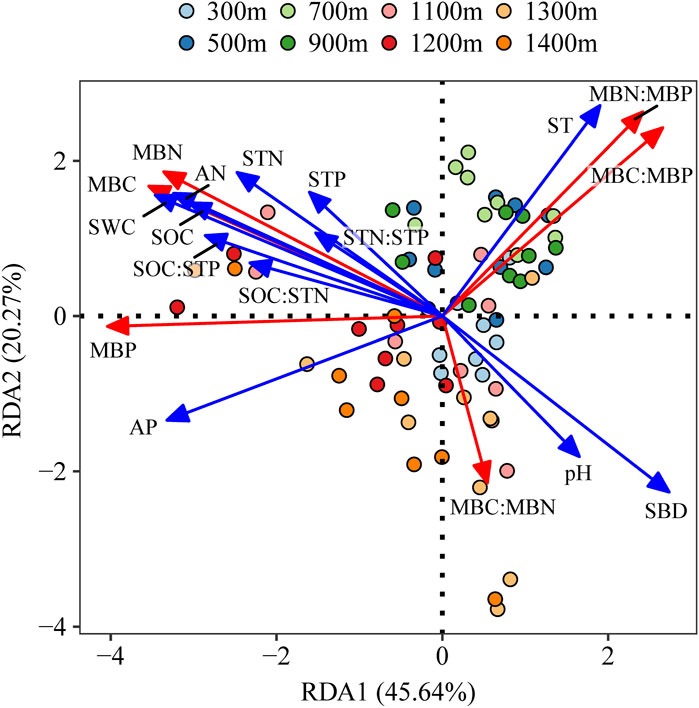
Figure 6. Redundancy analysis of soil microbial biomass C, N, and P stoichiometric characteristics with soil C, N, and P stoichiometric characteristics and physicochemical properties. The red and blue lines indicate response variables and explanatory variables, respectively. SOC, soil organic carbon; STN, soil total nitrogen; STP, soil total phosphorus; SOC:STN, ratio of soil organic carbon to total nitrogen; SOC:STP, ratio of soil organic carbon to total phosphorus; STN:STP, ratio of soil total nitrogen to total phosphorus; MBC, microbial biomass carbon; MBN, microbial biomass nitrogen; MBP, microbial biomass phosphorus; MBC:MBN, ratio of microbial biomass carbon to nitrogen; MBC:MBP, ratio of microbial biomass carbon to phosphorus; MBN:MBP, ratio of microbial biomass nitrogen to phosphorus; AN, available nitrogen; AP, available phosphorous; SWC, soil water content; SBD, soil bulk density; ST, soil temperature.
4 Discussion
4.1 Altitudinal patterns of soil C:N:P stoichiometry
Topography, climate, and biological factors interact along the altitudinal gradient to create high spatial variability in soil nutrients (Yu et al., 2018). In the present study, SOC in the 0–20 cm layer initially increased and then decreased significantly with increasing altitude. At lower altitudes (300–500 m), SOC increased with altitude, consistent with the findings of Zhao et al. (2013) in a similar altitude range on Daming Mountain. This pattern can be attributed to vegetation dynamics and climatic conditions across different altitude zones. In low-altitude areas, monsoon evergreen broadleaf forests dominate, characterized by high biomass production and substantial litter. However, the high temperatures in this zone accelerate microbial decomposition of organic matter, partially offsetting SOC accumulation. As altitude increases, the vegetation transitions to montane evergreen broadleaf forests, which maintain sufficient litter input. Cooler temperatures slow decomposition, resulting in peak SOC accumulation. In high-altitude areas, montane elfin forests prevail and are characterized by decreased plant biomass and litter (Feng et al., 2022; Li J. L. et al., 2023). The cooler temperatures at high-altitude areas further constrain microbial activity (Li S. et al., 2024), contributing to a net decrease in SOC. The role of litter input in SOC dynamics is further highlighted by a previous study on litter hydrological properties (Hu et al., 2025). Hu et al. (2025) demonstrated that litter mass and thickness initially increased with altitude, reaching a maximum at mid-altitudes (around 700 m), and then decreased at higher altitudes (up to 1,400 m). The similar altitudinal trends in litter, as reported by Hu et al. (2025), and in SOC, as observed in our study, suggest that litter input plays a critical role in regulating SOC accumulation along the altitudinal gradient.
In the present study, SOC, STN, and STP significantly decreased with increasing soil layers, consistent with previous findings (Qiao et al., 2020; Wang et al., 2021; Chen et al., 2022). The primary sources of soil C and N are litter inputs. After microbial decomposition, these nutrients tend to accumulate in the topsoil layer (Luo et al., 2020). Therefore, the response of topsoil SOC to altitude is stronger than that of deeper soil layers. The difference in the altitudinal trends of the SOC:STN ratio between the 0–20 and 20–40 cm layers further highlights distinct soil processes at different depths. In the 0–20 cm layer, the SOC:STN ratio is primarily influenced by variations in SOC, driven by higher organic matter inputs from litter and root exudates (Jobbágy and Jackson, 2000; Rumpel and Kögel-Knabner, 2011). Consequently, the altitudinal trends of SOC and the SOC:STN ratio in the 0–20 cm layer are similar, exhibiting an initial increase followed by a decrease with altitude. In contrast, the 20–40 cm layer exhibited a different SOC:STN dynamic, with a consistent increase along the altitudinal gradient. This pattern is likely attributed to slow organic matter decomposition, leaching of nitrogen from the surface, and reduced root activity in the deeper layer (Fontaine et al., 2007; Yamashita et al., 2010; Iversen et al., 2017). We found that although STP decreased with increasing soil layer, its variation range was small (0.24–0.35 g kg−1), similar to a previous study (0.28–0.38 g kg−1) (Qiao et al., 2020). This is because soil P is a sedimentary element primarily derived from rock weathering with a low migration rate (Smeck, 1985). As SWC increased with altitude, STP was leached to deeper soil layers, causing a significant reduction in STP in the 40–60 cm layer with increasing altitude.
The soil C, N, and P stoichiometric ratio is a key index to estimate soil quality (Jiang et al., 2024). A soil C:N ratio of less 25:1 likely indicates sufficient soil N and a high degree of organic matter mineralization (Paul, 2007). In our study, the decreased SOC:STN (19.25) ratio in the topsoil suggests sufficient C and N contents, which may promote microbial activity and organic matter decomposition. High microbial use efficiency of organic matter can enhance nutrient availability and support plant growth, particularly in ecosystems with sufficient soil nutrients (Prommer et al., 2020; Duan et al., 2023). However, the relationship between microbial efficiency and plant growth can vary depending on soil types, plant species, and local environmental conditions, potentially influencing nutrient cycling and microbial activity (Muhammad et al., 2025). The soil C:P ratio is commonly used to assess the potential of microorganisms for P release or uptake during the mineralization of organic matter. A low soil C:P ratio generally promotes microorganisms to release P during the decomposing of organic matter and increases soil P availability. The soil N:P ratio serves as a diagnostic indicator of N saturation and is often used to determine the threshold for nutrient limitation. (Jiang et al., 2019). In our study, the SOC:STP (137.26) and STN:STP (7.89) ratios in the topsoil were both higher than the global terrestrial (72.0, 5.9) (Cleveland and Liptzin, 2007), Chinese tropical and subtropical (78.0, 6.4) (Tian et al., 2010), and the Guangxi forest averages (69.60, 5.53) (Lu et al., 2023). These high C:P and N:P ratios indicate P limitation for soil microorganisms during organic matter decomposition, reflecting low P availability. Moreover, this conclusion is further supported by the low levels of AP (0.87–2.9 mg kg-1) and TP (0.24–0.35 g kg-1), classified as Grade VI (<3 mg kg-1) and Grade V (0.2–0.4 g kg-1), respectively, according to the soil nutrient grading standards of China’s second soil census (NSCO, 1992). The results align with previous studies in tropical and subtropical regions, which also indicated P limitation (Mo et al., 2019; van Breugel et al., 2019; Zhu et al., 2024). However, some studies in these regions have indicated N limitation (Hui et al., 2021; Chen et al., 2024). These differences may be influenced by differences in vegetation types and environmental conditions.
4.2 Altitudinal patterns of soil microbial C:N:P stoichiometry
In the present study, MBP generally increased with increasing altitude, primarily due to two reasons. First, as altitude increases, temperature decreases, and soil microorganisms can compensate for reduced activity under low-temperature conditions by increasing microbial biomass P content. This supports the temperature compensation hypothesis (Reich and Oleksyn, 2004; Li et al., 2014). Second, in mid-to-low-altitude areas, the dominant soil type is red soil, characterized by high acidity and abundant iron and aluminum oxides. These properties promote the formation of stable P compounds, reducing P availability. As altitude increases, the soil transitions to yellow and meadow soils, accompanied by increased pH and decreased iron and aluminum oxide content. This shift reduces P fixation and enhances P availability (Spohn and Widdig, 2017; Fan et al., 2019; Bai et al., 2021; Li J. L. et al., 2023). Consequently, both soil pH and AP increased with altitude. The mineralization of organic P is mainly driven by MBP (McGill and Cole, 1981; Li W. N. et al., 2024), which explains the consistent trends of MBP and AP along the altitudinal gradient. Moreover, SWC changes significantly influence microbial activity and community structure (Cesarz et al., 2022). In this study, SWC increased with altitude and was positively correlated with MBP and AP. This suggests that SWC may play a role in regulating microbial activity and P mineralization. However, both excessive and insufficient SWC can limit microbial biomass and activity (Wei et al., 2017; Gao et al., 2024), indicating that the relationship between SWC and MBP is complex and context-dependent. Therefore, while our results suggest a potential link between SWC and microbial P dynamics, further research is needed to clarify the underlying mechanisms.
We observed that MBC, MBN, and MBP significantly decreased with increasing soil layer, consistent with the trend of soil nutrients. This is mainly because litter, fine roots, and animal residues are primarily distributed in the topsoil, providing a substrate for microbial activity. Moreover, the favorable water, temperature, and aeration conditions in the topsoil further promote microbial growth and reproduction, leading to the accumulation of microbial biomass in the surface layer (Bahram et al., 2018; Jiang et al., 2021). As soil depth increases, the availability of nutrients and the oxygen transported by roots decreases, worsening the microbial habitat and limiting microbial biomass distribution. Consequently, the microbial biomass in the subsoil is lower than that in the topsoil (Zhou and Wang, 2015; Wu et al., 2024).
Soil microbial C:N:P stoichiometry is crucial for determining soil nutrient balance and material cycling (Hu et al., 2020). In our study, the MBC:MBP and MBN:MBP ratios initially increased and then decreased with increasing altitude, exhibiting an overall downward trend. This is primarily attributed to the changes in MBP with altitude. The observed trend indicates that P limitation is more pronounced in mid-to-low-altitude regions (300–900 m), which aligns with the findings of He et al. (2016), who reported P limitation at 500–700 m. Our findings also align with those of Zhu et al. (2024), who identified the strongest P limitation at 900 m. The MBC:MBN ratio typically reflects the N supply capacity in the soil. In our study, the MBC:MBN (5.97) ratio in the topsoil was lower than the global tropical or subtropical average (9.7) (Gao et al., 2022) and the Chinese forests average (8.3) (Xue et al., 2019), indicating higher N availability in the study area. The MBC:MBP ratio is commonly used to evaluate the effectiveness of P use, with a deceased ratio indicating higher P availability. The MBN:MBP ratio can be used to reflect the degree of plant demand for N and P (Hartman and Richardson, 2013). In this study, the MBC:MBP (38.97) ratio in the topsoil was similar to the global tropical or subtropical average (38.0) (Gao et al., 2022) but lower than the average of Chinese forests (54.2) (Xue et al., 2019). The MBN:MBP (6.72) ratio in the topsoil was higher than the global tropical or subtropical average (5.3) (Gao et al., 2022) and the Chinese forests average (6.0) (Xue et al., 2019). These findings indicate that soil microorganisms and plant growth are in a P-limited state in the study area, leading to a tendency for soil microorganisms to assimilate AP and compete with plants for its content.
4.3 Factors controlling soil microbial C:N:P stoichiometry
During the decomposition of plant and animal residues by soil microorganisms, C, N, and P elements are simultaneously released. These elements are also required for the growth and metabolism of soil microorganisms, leading to a coupled relationship between soil nutrient cycling and microbial metabolic processes. This coupling causes the changes in the contents and ratios of C, N, and P to be somewhat synchronous (Khan et al., 2024). This explains our observation of significant correlations between the C, N, and P contents and their ratios in soil and microbial biomass. Specifically, soil microbial biomass C, N, and P contents exhibited a significant positive correlation with soil C, N, and P contents and their ratios. This indicates that soil microbial biomass can be a crucial indicator of soil fertility and effectively characterize the intensity of organic matter metabolism (Hu et al., 2020). However, soil microbial biomass C, N, and P stoichiometric ratios were mostly significantly negatively correlated or not correlated with soil C, N, and P contents and their ratios. This is likely related to microbial metabolic constraints and nutrient competition under P-limited conditions. In the study area, the scarcity of available P in the soil may have driven microorganisms to increase their P assimilation efficiency to sustain growth and metabolic activities, leading to a relative increase in MBP. However, owing to the limited availability of P, microorganisms may reduce their utilization of other nutrients (e.g., C and N), resulting in a relative decrease in MBC and MBN (Wang et al., 2024). This could explain the negative or non-significant correlations between microbial biomass stoichiometric ratios and soil nutrients and stoichiometric ratios (Luo et al., 2024). In addition, P limitation may further regulate microbial demand for C, N, and P by influencing metabolic pathways and enzyme activities. This further contributes to the complexity of the relationship between microbial and soil stoichiometry (Cui et al., 2024).
In the present study, soil microbial biomass C, N, and P contents were significantly positively correlated with soil AN, AP, and SWC. These factors were also the main factors influencing the stoichiometric characteristics of soil microbial biomass C, N, and P. Owing to the significant influence of AN on AP (Zhang et al., 2004), in long-term P-limited subtropical ecosystems, adding N to acidic soils can stimulate soil microorganisms to secrete more phosphatases to obtain AP (Marklein and Houlton, 2012; Fan et al., 2018). As discussed above, SWC is important for microbial activity and P mineralization, likely by enhancing microbial metabolism and enzyme production (Lei et al., 2023). Therefore, adequate water content and N-enriched soils could facilitate the mineralization of P by soil microorganisms. SBD exhibited a significant negative correlation with soil and microbial biomass C, N, and P stoichiometric characteristics. This is primarily because an increased SBD reduces soil porosity and decreases aeration and water infiltration. It is also detrimental to microbial growth and metabolic activities, inhibiting the decomposition of soil nutrients (Zhu et al., 2012).
5 Conclusion
Based on a field investigation conducted at eight altitudes of Daming Mountain, we demonstrated that soil and microbial biomass C, N, and P stoichiometric characteristics exhibited significant variations across different altitudes and soil layers. With increasing altitude, soil C, N, and P stoichiometric characteristics varied across different soil layers. MBP initially decreased and then increased across all soil layers, whereas the MBC:MBP and MBN:MBP ratios exhibited the opposite trends. We identified AN, AP, and SWC as primary factors affecting soil microbial biomass C, N, and P stoichiometric characteristics. Moreover, P emerged as a limiting factor for soil microbial growth in the study area, with this limitation being more pronounced at mid-to-low altitudes. Therefore, it is necessary to apply P fertilizer strategically, especially in mid-to-low-altitude areas, to improve the soil nutrient supply and ensure P availability in forest management on Daming Mountain. These findings are important for understanding soil nutrient balance and limiting factors in subtropical mountain ecosystems. This study offers insights for informing soil management and the conservation and sustainable development of forest ecosystems in the region.
Data availability statement
The raw data supporting the conclusion of this article will be made available by the authors, without undue reservation.
Author contributions
CH: Conceptualization, Investigation, Data curation, Formal analysis, Methodology, Software, Writing – original draft. ZZ: Conceptualization, Funding acquisition, Supervision, Writing – review & editing. YL: Funding acquisition, Writing – review & editing. GH: Funding acquisition, Writing – review & editing, Conceptualization, Investigation, Supervision.
Funding
The author(s) declare that financial support was received for the research and/or publication of this article. This study was supported by the Special Funding for Guangxi Bagui Young Top Talents Program (to ZZ), the Guangxi Natural Science Foundation (2021GXNSFFA196005), and the National Natural Science Foundation of China (31960275).
Conflict of interest
The authors declare that the research was conducted in the absence of any commercial or financial relationships that could be construed as a potential conflict of interest.
Generative AI statement
The authors declare that no Generative AI was used in the creation of this manuscript.
Publisher’s note
All claims expressed in this article are solely those of the authors and do not necessarily represent those of their affiliated organizations, or those of the publisher, the editors and the reviewers. Any product that may be evaluated in this article, or claim that may be made by its manufacturer, is not guaranteed or endorsed by the publisher.
Supplementary material
The Supplementary Material for this article can be found online at: https://www.frontiersin.org/articles/10.3389/feart.2025.1569387/full#supplementary-material
References
Bahram, M., Hildebrand, F., Forslund, S. K., Anderson, J. L., Soudzilovskaia, N. A., Bodegom, P. M., et al. (2018). Structure and function of the global topsoil microbiome. Nature 560, 233–237. doi:10.1038/s41586-018-0386-6
Bai, J. Y., Zong, M. M., Li, S. Y., Li, H. X., Duan, C. Q., Feng, Y., et al. (2021). Nitrogen, water content, phosphorus and active iron jointly regulate soil organic carbon in tropical acid red soil forest. Eur. J. Soil Sci. 72, 446–459. doi:10.1111/ejss.12966
Beniston, M. (2003). Climatic change in mountain regions: a review of possible impacts. Clim. Change 59, 5–31. doi:10.1023/a:1024458411589
Bremner, J. M. (1996). Nitrogen-total in Methods of Soil Analysis. Part 3. Chemical Methods. Editors D. L. Sparks, A. L. Page, P. A. Helmke, R. H. Loeppert, P. N. Soltanpour, M. A. Tabatabaiet al. (Madison, WI: SSSA Press), 1085–1121.
Brookes, P. (2001). The soil microbial biomass: concept, measurement and applications in soil ecosystem research. Microbes Environ. 16, 131–140. doi:10.1264/jsme2.2001.131
Brookes, P. C., Powlson, D. S., and Jenkinson, D. S. (1982). Measurement of microbial biomass phosphorus in soil. Soil Biol. biochem. 14, 319–329. doi:10.1016/0038-0717(82)90001-3
Cesarz, S., Craven, D., Auge, H., Bruelheide, H., Castagneyrol, B., Gutknecht, J., et al. (2022). Tree diversity effects on soil microbial biomass and respiration are context dependent across forest diversity experiments. Glob. Ecol. Biogeogr. 31, 872–885. doi:10.1111/geb.13461
Chang, E. H., Chen, T. H., Tian, G. L., and Chiu, C. Y. (2016). The effect of altitudinal gradient on soil microbial community activity and structure in moso bamboo plantations. Appl. Soil Ecol. 98, 213–220. doi:10.1016/j.apsoil.2015.10.018
Chen, X., Feng, J. G., Ding, Z. J., Tang, M., and Zhu, B. (2022). Changes in soil total, microbial and enzymatic C-N-P contents and stoichiometry with depth and latitude in forest ecosystems. Sci. Total Environ. 816, 151583. doi:10.1016/j.scitotenv.2021.151583
Chen, Z. T., Jiang, Z. K., Li, Q. Y., Tan, Y. B., Yan, P. B., and Arif, M. (2024). Examining the stoichiometry of C:N:P:K in the dynamics of foliar-litter-soil within dominant tree species across different altitudes in southern China. Glob. Ecol. Conserv. 51, e02885. doi:10.1016/j.gecco.2024.e02885
Christmann, T., and Menor, I. O. (2021). A synthesis and future research directions for tropical mountain ecosystem restoration. Sci. Rep. 11, 23948. doi:10.1038/s41598-021-03205-y
Cleveland, C. C., and Liptzin, D. (2007). C:N:P stoichiometry in soil: is there a “Redfield ratio” for the microbial biomass? Biogeochemistry 85, 235–252. doi:10.1007/s10533-007-9132-0
Cui, Y. X., Moorhead, D. L., Peng, S. S., Sinsabaugh, R. L., and Peñuelas, J. (2024). Predicting microbial nutrient limitations from a stoichiometry-based threshold framework. Innov. Geosci. 2, 100048. doi:10.59717/j.xinn-geo.2024.100048
Duan, P. P., Fu, R. T., Nottingham, A. T., Domeignoz-Horta, L. A., Yang, X. Y., Du, H., et al. (2023). Tree species diversity increases soil microbial carbon use efficiency in a subtropical forest. Glob. Change Biol. 29, 7131–7144. doi:10.1111/gcb.16971
Elser, J. J., Fagan, W. F., Denno, R. F., Dobberfuhl, D. R., Folarin, A., Huberty, A., et al. (2000a). Nutritional constraints in terrestrial and freshwater food webs. Nature 408, 578–580. doi:10.1038/35046058
Elser, J. J., Sterner, R. W., Gorokhova, E., Fagan, W. F., Markow, T. A., Cotner, J. B., et al. (2000b). Biological stoichiometry from genes to ecosystems. Ecol. Lett. 3, 540–550. doi:10.1111/j.1461-0248.2000.00185.x
Falkowski, P. G., Fenchel, T., and Delong, E. F. (2008). The microbial engines that drive Earth's biogeochemical cycles. Science 320, 1034–1039. doi:10.1126/science.1153213
Fan, Y. X., Lin, F., Yang, L. M., Zhong, X. J., Wang, M. H., Zhou, J. C., et al. (2018). Decreased soil organic P fraction associated with ectomycorrhizal fungal activity to meet increased P demand under N application in a subtropical forest ecosystem. Biol. Fertil. Soils 54, 149–161. doi:10.1007/s00374-017-1251-8
Fan, Y. X., Zhong, X. J., Lin, F., Liu, C. C., Yang, L. M., Wang, M. H., et al. (2019). Responses of soil phosphorus fractions after nitrogen addition in a subtropical forest ecosystem: insights from decreased Fe and Al oxides and increased plant roots. Geoderma 337, 246–255. doi:10.1016/j.geoderma.2018.09.028
Feng, J. G., He, K. Y., Zhang, Q. F., Han, M. G., and Zhu, B. (2022). Changes in plant inputs alter soil carbon and microbial communities in forest ecosystems. Glob. Change Biol. 28, 3426–3440. doi:10.1111/gcb.16107
Feng, W. L., Yang, J. L., Xu, L. G., and Zhang, G. L. (2024). The spatial variations and driving factors of C, N, P stoichiometric characteristics of plant and soil in the terrestrial ecosystem. Sci. Total Environ. 951, 175543. doi:10.1016/j.scitotenv.2024.175543
Fontaine, S., Barot, S., Barré, P., Bdioui, N., Mary, B., and Rumpel, C. (2007). Stability of organic carbon in deep soil layers controlled by fresh carbon supply. Nature 450, 277–280. doi:10.1038/nature06275
Gao, D. C., Bai, E., Wang, S. Y., Zong, S. W., Liu, Z. P., Fan, X. L., et al. (2022). Three-dimensional mapping of carbon, nitrogen, and phosphorus in soil microbial biomass and their stoichiometry at the global scale. Glob. Change Biol. 28, 6728–6740. doi:10.1111/gcb.16374
Gao, Y. J., Tariq, A., Zeng, F. J., Sardans, J., Graciano, C., Li, X. Y., et al. (2024). Soil microbial functional profiles of P-cycling reveal drought-induced constraints on P-transformation in a hyper-arid desert ecosystem. Sci. Total Environ. 925, 171767. doi:10.1016/j.scitotenv.2024.171767
Hartman, W. H., and Richardson, C. J. (2013). Differential nutrient limitation of soil microbial biomass and metabolic quotients (qCO2): is there a biological stoichiometry of soil microbes? PLoS One 8, e57127. doi:10.1371/journal.pone.0057127
He, X. J., Hou, E. Q., Liu, Y., and Wen, D. Z. (2016). Altitudinal patterns and controls of plant and soil nutrient concentrations and stoichiometry in subtropical China. Sci. Rep. 6, 24261. doi:10.1038/srep24261
He, X. J., Hou, E. Q., Veen, G. F., Ellwood, M. D. F., Dijkstra, P., Sui, X. H., et al. (2020). Soil microbial biomass increases along elevational gradients in the tropics and subtropics but not elsewhere. Glob. Ecol. Biogeogr. 29, 345–354. doi:10.1111/geb.13017
Hobbie, S. E., Nadelhoffer, K. J., and Högberg, P. (2002). A synthesis: the role of nutrients as constraints on carbon balances in boreal and arctic regions. Plant Soil 242, 163–170. doi:10.1023/a:1019670731128
Hou, Y. H., He, K. Y., Chen, Y., Zhao, J. X., Hu, H. F., and Zhu, B. (2021). Changes of soil organic matter stability along altitudinal gradients in Tibetan alpine grassland. Plant Soil 458, 21–40. doi:10.1007/s11104-019-04351-z
Hu, G., Huo, C. L., Hu, C., Zhong, C. F., Chen, S. Y., Xu, C. H., et al. (2025). Elevational patterns of hydrological properties of forest litter layers in Daming Mountain, southern China. Glob. Ecol. Conserv. 58, e03510. doi:10.1016/j.gecco.2025.e03510
Hu, M. J., Peñuelas, J., Sardans, J., Yang, X. Y., Tong, C., Zou, S. Q., et al. (2020). Shifts in microbial biomass C/N/P stoichiometry and bacterial community composition in subtropical estuarine tidal marshes along a gradient of freshwater-oligohaline water. Ecosystems 23, 1265–1280. doi:10.1007/s10021-019-00468-5
Huang, W. J., Liu, C., Liu, Y., Huang, B., Li, D. Q., and Yuan, Z. J. (2023). Soil ecological stoichiometry and its influencing factors at different elevations in nanling mountains. Ecol. Environ. Sci. 32, 80–89. doi:10.16258/j.cnki.1674-5906.2023.01.009
Hui, D. F., Yang, X. T., Deng, Q., Liu, Q., Wang, X., Yang, H., et al. (2021). Soil C:N:P stoichiometry in tropical forests on Hainan Island of China: spatial and vertical variations. Catena 201, 105228. doi:10.1016/j.catena.2021.105228
Iversen, C. M., Mccormack, M. L., Powell, A. S., Blackwood, C. B., Freschet, G. T., Kattge, J., et al. (2017). A global Fine-Root Ecology Database to address below-ground challenges in plant ecology. New Phytol. 215, 15–26. doi:10.1111/nph.14486
Jia, C. J., Wu, G., Lu, Y., Qin, H. L., Jiang, K., Huang, W. H., et al. (2023). Stoichiometry characteristics of C, N, and P of soil profiles along an altitude gradient in luofu mountain, guangdong. Pol. J. Environ. Stud. 32, 2669–2677. doi:10.15244/pjoes/161373
Jiang, L., He, Z. S., Liu, J. F., Xing, C., Gu, X. G., Wei, C. S., et al. (2019). Elevation gradient altered soil C, N, and P stoichiometry of Pinus taiwanensis forest on daiyun mountain. Forests 10, 1089. doi:10.3390/f10121089
Jiang, W. T., Gong, L., Yang, L. H., He, S. P., and Liu, X. H. (2021). Dynamics in C, N, and P stoichiometry and microbial biomass following soil depth and vegetation types in low mountain and hill region of China. Sci. Rep. 11, 19631. doi:10.1038/s41598-021-99075-5
Jiang, X. Y., Wang, X. J., Qiao, Y. Q., Cao, Y., Jiao, Y., Yang, A., et al. (2024). Atmospheric nitrogen deposition affects forest plant and soil system carbon:nitrogen:phosphorus stoichiometric flexibility: a meta-analysis. For. Ecosyst. 11, 100192. doi:10.1016/j.fecs.2024.100192
Jobbágy, E. G., and Jackson, R. B. (2000). The vertical distribution of soil organic carbon and its relation to climate and vegetation. Ecol. Appl. 10, 423–436. doi:10.2307/2641104
Keeney, D. R., and Nelson, D. W. (1982). Nitrogen in organic forms in Methods of soil analysis. Part 2. Chemical and microbial properties. Editors A. L. Page, R. H. Miller, and D. R. Keeney (Madison, WI: ASA Press), 643–698.
Khan, A., Li, T. Y., He, B. H., and Song, J. H. (2024). Fertilization and cultivation management alleviate microbial nitrogen limitation in purple soil sloping farmland: evidence from ecoenzymatic stoichiometry. Ecol. Eng. 207, 107347. doi:10.1016/j.ecoleng.2024.107347
Koerselman, W., and Meuleman, A. F. M. (1996). The vegetation N:P ratio: a new tool to detect the nature of nutrient limitation. J. Appl. Ecol. 33, 1441–1450. doi:10.2307/2404783
Körner, C. (2007). The use of 'altitude' in ecological research. Trends Ecol. Evol. 22, 569–574. doi:10.1016/j.tree.2007.09.006
Lei, J. J., Cao, Y. X., Wang, J., Chen, Y. Z., Peng, Y. Y., Shao, Q. W., et al. (2023). Soil nutrients, enzyme activities, and microbial communities along a chronosequence of Chinese fir plantations in subtropical China. Plants-Basel 12, 1931. doi:10.3390/plants12101931
Li, J. L., Luo, Y. H., Song, X. Y., Jiang, D. D., He, Q. P., Bai, A. Q., et al. (2023). Effects of the dominate plant families on elevation gradient pattern of community structure in a subtropical forest. Forests 14, 1860. doi:10.3390/f14091860
Li, P., Yang, Y. H., Han, W. X., and Fang, J. Y. (2014). Global patterns of soil microbial nitrogen and phosphorus stoichiometry in forest ecosystems. Glob. Ecol. Biogeogr. 23, 979–987. doi:10.1111/geb.12190
Li, S., Delgado-Baquerizo, M., Ding, J. X., Hu, H., Huang, W. G., Sun, Y. S., et al. (2024). Intrinsic microbial temperature sensitivity and soil organic carbon decomposition in response to climate change. Glob. Change Biol. 30, e17395. doi:10.1111/gcb.17395
Li, W. N., Ullah, S., Liu, F., Deng, F. C., Han, X. M., Huang, S. D., et al. (2024). Synergistic variation of rhizosphere soil phosphorus availability and microbial diversity with stand age in plantations of the endangered tree species Parashorea chinensis. Front. Plant Sci. 15, 1372634. doi:10.3389/fpls.2024.1372634
Li, Y., Wu, J. S., Liu, S. L., Shen, J. L., Huang, D. Y., Su, Y. R., et al. (2012). Is the C:N:P stoichiometry in soil and soil microbial biomass related to the landscape and land use in southern subtropical China? Glob. Biogeochem. Cycle 26, 14. doi:10.1029/2012gb004399
Li, Y. F., Ye, S. M., Luo, Y. H., Yu, S. F., and Zhang, G. Q. (2023). Relationship between species diversity and tree size in natural forests around the Tropic of Cancer. J. For. Res. 34, 1735–1745. doi:10.1007/s11676-023-01616-3
Li, Z. Y., Qiu, X. R., Sun, Y., Liu, S. N., Hu, H. L., Xie, J. L., et al. (2021). C:N:P stoichiometry responses to 10 years of nitrogen addition differ across soil components and plant organs in a subtropical Pleioblastus amarus forest. Sci. Total Environ. 796, 148925. doi:10.1016/j.scitotenv.2021.148925
Liu, D. P., Zheng, D. X., Xu, Y. Y., Chen, Y. F., Wang, H. S., Wang, K., et al. (2021). Changes in the stoichiometry of Castanopsis fargesii along an elevation gradient in a Chinese subtropical forest. PeerJ 9, e11553. doi:10.7717/peerj.11553
Lu, M. Z., Zeng, F. P., Lv, S. W., Zhang, H., Zeng, Z. X., Peng, W. X., et al. (2023). Soil C:N:P stoichiometry and its influencing factors in forest ecosystems in southern China. Front. For. Glob. Change 6, 13. doi:10.3389/ffgc.2023.1142933
Luo, X. Z., Hou, E. Q., Chen, J. Q., Li, J., Zhang, L. L., Zang, X. W., et al. (2020). Dynamics of carbon, nitrogen, and phosphorus stocks and stoichiometry resulting from conversion of primary broadleaf forest to plantation and secondary forest in subtropical China. Catena 193, 104606. doi:10.1016/j.catena.2020.104606
Luo, X. Z., Zhang, L. L., Li, Z., Zhang, R., Le Roux, J. J., Reynolds, J. K., et al. (2024). Adaptation mechanisms of soil microbial community to stoichiometric imbalances caused by forest conversion. Appl. Soil Ecol. 201, 105529. doi:10.1016/j.apsoil.2024.105529
Marklein, A. R., and Houlton, B. Z. (2012). Nitrogen inputs accelerate phosphorus cycling rates across a wide variety of terrestrial ecosystems. New Phytol. 193, 696–704. doi:10.1111/j.1469-8137.2011.03967.x
Mcgill, W. B., and Cole, C. V. (1981). Comparative aspects of cycling of organic C, N, S and P through soil organic matter. Geoderma 26, 267–286. doi:10.1016/0016-7061(81)90024-0
Mo, Q. F., Li, Z. A., Sayer, E. J., Lambers, H., Li, Y. W., Zou, B., et al. (2019). Foliar phosphorus fractions reveal how tropical plants maintain photosynthetic rates despite low soil phosphorus availability. Funct. Ecol. 33, 503–513. doi:10.1111/1365-2435.13252
Muhammad, M., Wahab, A., Waheed, A., Hakeem, K. R., Mohamed, H. I., Basit, A., et al. (2025). Navigating climate change: exploring the dynamics between plant-soil microbiomes and their impact on plant growth and productivity. Glob. Change Biol. 31, e70057. doi:10.1111/gcb.70057
Nandy, S., Saranya, M., and Srinet, R. (2022). Spatio-temporal variability of water use efficiency and its drivers in major forest formations in India. Remote Sens. Environ. 269, 112791. doi:10.1016/j.rse.2021.112791
Nelson, D. W., and Sommers, L. E. (1982). Total carbon, organic carbon, and organic matter in Methods of Soil Analysis. Part2. Chemical and Microbiological Properties. Editors A. L. Page, R. H. Miller, and D. R. Keeney (Madison, WI: ASA Press), 539–577.
NSCO (National Soil Census Office) (1992). Techniques of Soil Census in China. Beijing: Agricultural Press.
Olsen, S. R., Cole, C. V., Watanbe, F. S., and Dean, L. A. (1954). Estimation of available phosphorus in soils by extraction with sodium bicarbonate. Washington: USDA Press.
Olsen, S. R., and Sommers, L. E. (1982). Phosphorus in Methods of Soil Analysis, Part 2, Chemical and Microbiological Properties. Editors A. L. Page, R. H. Miller, and D. R. Keeney (Madison, WI: ASA Press), 403–430.
Pan, Y. L., Fang, F., and Tang, H. P. (2021). Patterns and internal stability of carbon, nitrogen, and phosphorus in soils and soil microbial biomass in terrestrial ecosystems in China: a data synthesis. Forests 12, 1544. doi:10.3390/f12111544
Prommer, J., Walker, T. W. N., Wanek, W., Braun, J., Zezula, D., Hu, Y. T., et al. (2020). Increased microbial growth, biomass, and turnover drive soil organic carbon accumulation at higher plant diversity. Glob. Change Biol. 26, 669–681. doi:10.1111/gcb.14777
Qiao, Y., Wang, J., Liu, H. M., Huang, K., Yang, Q. S., Lu, R. L., et al. (2020). Depth-dependent soil C-N-P stoichiometry in a mature subtropical broadleaf forest. Geoderma 370, 114357. doi:10.1016/j.geoderma.2020.114357
Rahbek, C., Borregaard, M. K., Colwell, R. K., Dalsgaard, B., Holt, B. G., Morueta-Holme, N., et al. (2019). Humboldt's enigma: what causes global patterns of mountain biodiversity? Science 365, 1108–1113. doi:10.1126/science.aax0149
Reich, P. B., and Oleksyn, J. (2004). Global patterns of plant leaf N and P in relation to temperature and latitude. Proc. Natl. Acad. Sci. U. S. A. 101, 11001–11006. doi:10.1073/pnas.0403588101
Rumpel, C., and Kögel-Knabner, I. (2011). Deep soil organic matter-a key but poorly understood component of terrestrial C cycle. Plant Soil 338, 143–158. doi:10.1007/s11104-010-0391-5
Schindler, D. W. (2003). Ecological stoichiometry: the biology of elements from molecules to the biosphere. Nature 423, 225–226. doi:10.1038/423225b
Smeck, N. E. (1985). Phosphorus dynamics in soils and landscapes. Geoderma 36, 185–199. doi:10.1016/0016-7061(85)90001-1
Spohn, M., and Widdig, M. (2017). Turnover of carbon and phosphorus in the microbial biomass depending on phosphorus availability. Soil Biol. biochem. 113, 53–59. doi:10.1016/j.soilbio.2017.05.017
Tian, H. Q., Chen, G. S., Zhang, C., Melillo, J. M., and Hall, C. a. S. (2010). Pattern and variation of C:N:P ratios in China's soils: a synthesis of observational data. Biogeochemistry 98, 139–151. doi:10.1007/s10533-009-9382-0
Tong, R., Wu, T. G., Jiang, B., Wang, Z. G., Xie, B. L., and Zhou, B. Z. (2023). Soil carbon, nitrogen, and phosphorus stoichiometry and its influencing factors in Chinese fir plantations across subtropical China. Front. For. Glob. Change 5, 8. doi:10.3389/ffgc.2022.1086328
Van Breugel, M., Craven, D., Lai, H. R., Baillon, M., Turner, B. L., and Hall, J. S. (2019). Soil nutrients and dispersal limitation shape compositional variation in secondary tropical forests across multiple scales. J. Ecol. 107, 566–581. doi:10.1111/1365-2745.13126
Vance, E. D., Brookes, P. C., and Jenkinson, D. S. (1987). An extraction method for measuring soil microbial biomass C. Soil Biol. biochem. 19, 703–707. doi:10.1016/0038-0717(87)90052-6
Wan, L. F., Liu, G. H., Cheng, H., Yang, S. S., Shen, Y., and Su, X. K. (2023). Global warming changes biomass and C:N:P stoichiometry of different components in terrestrial ecosystems. Glob. Change Biol. 29, 7102–7116. doi:10.1111/gcb.16986
Wang, A., Wang, X., Tognetti, R., Lei, J. P., Pan, H. L., Liu, X. L., et al. (2018). Elevation alters carbon and nutrient concentrations and stoichiometry in Quercus aquifolioides in southwestern China. Sci. Total Environ. 622, 1463–1475. doi:10.1016/j.scitotenv.2017.12.070
Wang, H. C., Wang, H., Crowther, T. W., Isobe, K., Reich, P. B., Tateno, R., et al. (2024). Metagenomic insights into inhibition of soil microbial carbon metabolism by phosphorus limitation during vegetation succession. ISME Commun. 4, ycae128. doi:10.1093/ismeco/ycae128
Wang, Z. C., He, G. X., Hou, Z. H., Luo, Z., Chen, S. X., Lu, J., et al. (2021). Soil C:N:P stoichiometry of typical coniferous (Cunninghamia lanceolata) and/or evergreen broadleaved (Phoebe bournei) plantations in south China. For. Ecol. Manage. 486, 118974. doi:10.1016/j.foreco.2021.118974
Wei, X. M., Hu, Y. J., Peng, P. Q., Zhu, Z. K., Atere, C. T., O'donnell, A. G., et al. (2017). Effect of P stoichiometry on the abundance of nitrogen-cycle genes in phosphorus-limited paddy soil. Biol. Fertil. Soils 53, 767–776. doi:10.1007/s00374-017-1221-1
Wild, J., Kopecky, M., Macek, M., Sanda, M., Jankovec, J., and Haase, T. (2019). Climate at ecologically relevant scales: a new temperature and soil moisture logger for long-term microclimate measurement. Agric. For. Meteorol. 268, 40–47. doi:10.1016/j.agrformet.2018.12.018
Wu, G. P., Su, Y. G., Wang, J. J., Lin, S. N., Huang, Z. Y., and Huang, G. (2024). Elevational patterns of microbial carbon use efficiency in a subtropical mountain forest. Biol. Fertil. Soils 60, 5–15. doi:10.1007/s00374-022-01694-1
Xiong, X. L., Lyu, M. K., Deng, C., Li, X. J., Lu, Y. M., Lin, W. S., et al. (2022). Carbon and nitrogen availability drives seasonal variation in soil microbial communities along an elevation gradient. Forests 13, 1657. doi:10.3390/f13101657
Xue, H. L., Lan, X., Liang, H. G., and Zhang, Q. (2019). Characteristics and environmental factors of stoichiometric homeostasis of soil microbial biomass carbon, nitrogen and phosphorus in China. Sustainability 11, 2804. doi:10.3390/su11102804
Yamashita, N., Ohta, S., Sase, H., Luangjame, J., Visaratana, T., Kievuttinon, B., et al. (2010). Seasonal and spatial variation of nitrogen dynamics in the litter and surface soil layers on a tropical dry evergreen forest slope. For. Ecol. Manage. 259, 1502–1512. doi:10.1016/j.foreco.2010.01.026
Yu, Z. P., Wang, M. H., Huang, Z. Q., Lin, T. C., Vadeboncoeur, M. A., Searle, E. B., et al. (2018). Temporal changes in soil C-N-P stoichiometry over the past 60 years across subtropical China. Glob. Change Biol. 24, 1308–1320. doi:10.1111/gcb.13939
Zhang, D. D., Wu, B. Y., Li, J. S., and Cheng, X. L. (2023). Soil microbial relative resource limitation exhibited contrasting seasonal patterns along an elevational gradient in Yulong Snow Mountain. Funct. Ecol. 37, 1328–1338. doi:10.1111/1365-2435.14297
Zhang, F. C., Kang, S. Z., Zhang, J. H., Zhang, R. D., and Li, F. S. (2004). Nitrogen fertilization on uptake of soil inorganic phosphorus fractions in the wheat root zone. Soil Sci. Soc. Am. J. 68, 1890–1895. doi:10.2136/sssaj2004.1890
Zhang, J. Q., Shen, X. J., Wang, Y. J., Jiang, M., and Lu, X. G. (2021). Effects of forest changes on summer surface temperature in changbai mountain, China. Forests 12, 1551. doi:10.3390/f12111551
Zhao, P. P., Zhou, J. C., Lin, K. M., Lin, W. S., Yuan, P., Zeng, X. M., et al. (2019). Effects of different altitudes on soil microbial biomass and enzyme activities in Pinus taiwanensis forests on Daiyun Mountain,Fujian Province. Acta Ecol. Sin. 39, 2676–2686. doi:10.5846/stxb201805111043
Zhao, Y., Zhou, R. J. M., Yu, Q., and Zhao, L. (2025). Revealing the contribution of mountain ecosystem services research to sustainable development goals: a systematic and grounded theory driven review. J. Environ. Manage. 373, 123452. doi:10.1016/j.jenvman.2024.123452
Zhao, Z. G., Qin, X. D., Wei, P., Guo, J. J., Zeng, J., and Xu, J. M. (2013). Vertical variation of soil chemical properties in natural forests of Erythrophleum fordii in Damingshan Moutain, Guangxi. J. Cent. South Univ. For. Technol. 33, 101–105. doi:10.14067/j.cnki.1673-923x.2013.12.011
Zhou, Z. H., and Wang, C. K. (2015). Soil resources and climate jointly drive variations in microbial biomass carbon and nitrogen in China's forest ecosystems. Biogeosciences 12. doi:10.5194/bgd-12-11191-2015
Zhu, D. H., Zhou, Y., Peng, S. H., Hua, W. P., Luo, B., and Hui, D. F. (2024). Impacts of altitude on plant green leaf, fresh litter, and soil stoichiometry in subtropical forests. Front. For. Glob. Change 7, 12. doi:10.3389/ffgc.2024.1331623
Zhu, H. H., He, X. Y., Wang, K. L., Su, Y. R., and Wu, J. S. (2012). Interactions of vegetation succession, soil bio-chemical properties and microbial communities in a Karst ecosystem. Eur. J. Soil Biol. 51, 1–7. doi:10.1016/j.ejsobi.2012.03.003
Zuo, Y. P., Zhang, H. J., Li, J. P., Yao, X. D., Chen, X. Y., Zeng, H., et al. (2021). The effect of soil depth on temperature sensitivity of extracellular enzyme activity decreased with elevation: evidence from mountain grassland belts. Sci. Total Environ. 777, 146136. doi:10.1016/j.scitotenv.2021.146136
Keywords: altitude, nutrient limitation, soil microbial biomass, ecological stoichiometry, Daming Mountain
Citation: Huo C, Zhang Z, Luo Y and Hu G (2025) Altitudinal patterns of soil and microbial C:N:P stoichiometry in subtropical forests in Daming Mountain, South China. Front. Earth Sci. 13:1569387. doi: 10.3389/feart.2025.1569387
Received: 31 January 2025; Accepted: 27 March 2025;
Published: 07 April 2025.
Edited by:
Ji Liu, Chinese Academy of Sciences (CAS), ChinaReviewed by:
Xiangjin Shen, Chinese Academy of Sciences (CAS), ChinaYamin Jiang, Hainan University, China
Zhonglin Xu, Xinjiang University, China
Copyright © 2025 Huo, Zhang, Luo and Hu. This is an open-access article distributed under the terms of the Creative Commons Attribution License (CC BY). The use, distribution or reproduction in other forums is permitted, provided the original author(s) and the copyright owner(s) are credited and that the original publication in this journal is cited, in accordance with accepted academic practice. No use, distribution or reproduction is permitted which does not comply with these terms.
*Correspondence: Yinghua Luo, bGlsaWFjZWFlbHVvQDE2My5jb20=; Gang Hu, aHVnYW5nQG5ubnUuZWR1LmNu
†These authors have contributed equally to this work