- 1Department of Applied Physics, Gipuzkoa Engineering Faculty, University of the Basque Country UPV/EHU, Donostia, Spain
- 2School of Dual Engineering, Machine Tool Institute (IMH), Elgoibar, Spain
Integrated STEM education (iSTEM) has become an innovative educational strategy that combines Science, Technology, Engineering, and Mathematics to address contemporary scientific and technical challenges. Yet, the variety of interpretations of the theoretical foundations of iSTEM education makes its understanding, analysis, and translation into classroom reality a complicated process. This article explores the foundations of iSTEM education by analyzing key systematic reviews that identify and describe the most widely agreed upon principles, with five: integration, real-world problems, inquiry, design, and cooperative work. Even so, the explanations in the literature reviewed do not clarify the relationship between them. Debate arises about their hierarchy and relative importance in the design of Teaching-Learning Sequences. The review of this study highlights that integration and the use of real-world problems are fundamental pillars, while other principles, such as inquiry and design, vary according to the disciplinary approach. The article also addresses the impact of problem choice on teaching strategies to understand the relationship between the principles of “real-world problem,” “design,” and “inquiry.” Thus, epistemological differences between Science, Mathematics, and Engineering determine how an iSTEM problem is defined and solved, with Science and Engineering leading the way in problem formulation due to their wide use in the literature. This allows for the analysis of Design-Based Learning (DBL) and Inquiry-Based Learning (IBL) as the main teaching approaches and presents a range of problem types to be used in the classroom depending on the objective pursued. Finally, this study highlights the need to connect the theoretical foundations with their practical application in actual teaching-learning sequences, providing a framework for future research to enrich the understanding and application of iSTEM education.
1 Introduction
Integrated STEM education is one of the educational approaches to science and technology teaching and learning that has received the most attention in recent years (Kayan-Fadlelmula et al., 2022; Halawa et al., 2024). Firstly, the literature in this field uses a variety of terms, including integrated STEM education, iSTEM education, STEM education, and STEAM education, among others. To clarify the distinction between individual disciplines and an integrated educational approach, this study will use the term integrated STEM education or iSTEM. Following the explanations of Honey et al. (2014) and Martín-Páez et al. (2019), in this article we understand iSTEM education as the interdisciplinary educational approach that connects at least two of the disciplines that create the acronym (Science, Technology, Engineering and Mathematics) using a real world problem. Thus, one of the most important factors of integrated STEM education is the opportunity to develop interdisciplinary projects, giving students an idea of the connection of what they are learning with reality and with other subjects (Millar, 2016).
The growth of iSTEM educational proposals is driven by various motivations. On the one hand, the economic progress of countries through the training of professionals in the sector (Vázquez-Alonso and Manassero-Mas, 2015; Levrini et al., 2017; Millar, 2020; Mejias et al., 2021). Its purpose is to equip students with the necessary knowledge for their professional future and foster the critical thinking required in today’s labor market (Kayan-Fadlelmula et al., 2022; Zhou et al., 2022). Another important reason is to promote literacy in these disciplines among students (Holmlund et al., 2018; Thibaut et al., 2018). This is essential to develop generations with scientific-technological knowledge and skills to solve socio-scientific problems of everyday life (Bybee, 2010; Delahunty and Kimbell, 2021). Likewise, numerous studies in iSTEM education have shown the positive impact of this educational approach on students’ attitudes, motivation and performance, particularly in minority groups and female students (Honey et al., 2014; Aguilera and Ortiz-Revilla, 2021; Al-Mutawah et al., 2021; De Loof et al., 2021; Jackson et al., 2021).
Although iSTEM education originated in the 1990s, it has been gaining presence in recent years as evidenced by the growing number of research and publications in this field (Gil-Doménech et al., 2020; Marín-Marín et al., 2021; Tas and Bolat, 2022; Zhan et al., 2022). The main publication topics according to the most recent bibliometric analyses are equity, iSTEM education’s impact on both general students and minority groups, teacher education, and students’ learning and skills development (Marín-Marín et al., 2021; Zhan et al., 2022). This field of research is accompanied by ongoing debates about the theoretical foundations of iSTEM education, as the variety of perspectives and interpretations complicates understanding, comparing studies, and achieving consistent classroom application (Bybee, 2013; English, 2017; Chu et al., 2019; Yata et al., 2020; Roehrig et al., 2021; Ortiz-Revilla et al., 2022). In the literature there are several articles on definitions and levels of integration aiming to advance on the problem of heterogeneity within the field (Gresnigt et al., 2014; English, 2016; Kelley and Knowles, 2016; Martín-Páez et al., 2019; Perales Palacios and Aguilera, 2020; Al-Mutawah et al., 2021; Dare et al., 2021; Spikic et al., 2022; Zhou et al., 2022). However, following Portillo-Blanco et al.’s (2024) systematic review of the different theoretical frameworks published in the literature reveals that the presence of these articles has also been increasing in recent years and that the diversity of explanations reflects the need to create a minimum consensus around the foundations of integrated STEM education (Aguilera and Ortiz-Revilla, 2021; Portillo-Blanco et al., 2024).
This article is a critical literature review that aims to analyze the theoretical ambiguities around iSTEM principles, clarify their relationships, and build bridges between the theoretical perspective and its practical translation. A critical literature review not only describes the state of the art but analyses, critiques, and synthesizes key studies to develop a new theoretical framework or innovative perspective (Torraco, 2005; Snyder, 2019). Moreover, it does not follow a strict methodology but requires an in-depth analysis of the relevant content to substantiate the proposal (Whittemore and Knafl, 2005; Grant and Booth, 2009).
Based on this approach, this article presents an analysis of iSTEM principles and their interrelationships, starting with the examination of two systematic reviews. Throughout the text, each section raises new questions that drive a progressive analysis, ultimately leading to the construction of an interrelational model of iSTEM principles.
Systematic reviews analyzing the theoretical frameworks published to date have aimed to move towards a more homogeneous consensus model of iSTEM education. This article, firstly, compares two systematic reviews of iSTEM principles, looking for points of consensus and discussion in their conclusions. As will be seen in the following sections, these reviews present various principles independently and without developing the relationship between them. This raises a key question: How do these principles interrelate in educational practice?
Secondly, to address the previous question, the article seeks to clarify the relationships between the principles and the impact they have on the decisions that are made when developing an iSTEM Teaching-Learning Sequence (TLS). To this end, in section 3, a reflection is presented based on literature of a possible hierarchical organization of the principles using the common characteristics of the variety of definitions of iSTEM education. This analysis exposes a certain order of importance among the principles and emerges as first principal common characteristics the integration and the real-world problems.
Thirdly, in Section 4, we begin with a reflection on the importance of considering the selection of real-world problems as the main common characteristic and its impact on the other principles and the design of the TLS. To clarify this issue, we use the common elements of the epistemology of iSTEM disciplines to develop a general problem-solving structure in iSTEM (Sections 4.2 and 4.3). Finally, in section 4.4, a last review of the literature allows us to offer a set of examples that facilitate the understanding of the proposal presented. All this concludes in a proposed model of iSTEM principles that establishes relationships between principles that until now had been explained independently, thus offering a new perspective for translating theory into educational practice.
2 Principles of iSTEM education: insights from the systematic reviews underpinning this study
To establish the starting point of this study, a search for systematic reviews published to date was conducted in Web of Science, ERIC, and Scopus, aiming to find articles that had “systematic review” and “STEM” on their title and “framework” or “principle” on their abstract. It was decided to search only for systematic reviews because they offer a comprehensive and structured analysis of the existing literature, synthesizing findings from multiple studies with methodological rigor, providing an evidence-based and holistic view of the topic of interest, minimizing biases, and optimizing the quality of the information gathered (Page et al., 2021). After analyzing the results of these searches, the articles by Thibaut et al. (2018) and Portillo-Blanco et al. (2024) were selected. The reason for proceeding from these two systematic reviews is that they both conclude their studies with a set of iSTEM theoretical principles created from the analysis of a collection of previous research. This section is aimed at explaining the most important points of these two articles in order to facilitate the understanding of the discussion that follows.
On the one hand, the review by Thibaut et al. (2018) examines 23 iSTEM education articles for the secondary education level that incorporate three disciplines that make up the acronym and describe teaching practices. Thus, they analyzed the underlying educational theories and listed the identified teaching practices of each of the articles. They then rearranged the instructional practices’ list and grouped based on their similarities, leading to nine different categories. In their results, they conclude that iSTEM education is framed by social-constructivist learning theory and that the instructional practices are composed of nine principles: (1) integration of STEM content, (2) focus on problems, (3) inquiry, (4) design, (5) teamwork, (6) student-centered, (7) hands-on, (8) assessment, and (9) twenty first century skills. However, it is worth noting that, according to their analysis the first five principles bring together the four others because of the characteristics they share. Which concludes their iSTEM education model firstly as framed by social-constructivist learning theory and constituted by five principles: (1) integration of STEM content, (2) focus on problems, (3) inquiry, (4) design, (5) teamwork.
On the other hand, Portillo-Blanco et al. (2024) carry out an analysis of theoretical articles looking for the principles and characteristics of integrated STEM education described in each of them, in order to find the points of consensus and discussion. In contrast to the previous systematic review, Portillo-Blanco et al. (2024) analyzed 27 studies focused on the theoretical foundation of iSTEM education. This systematic review shows the wide variety of interpretations of the iSTEM theoretical framework in the literature and examines the key ideas of each of them in grouping into principles according to their shared characteristics. They start identifying 16 different principles and report that according to the frequency of appearance in the articles included in the review, five principles are the most common: (1) integration, (2) the use of real-world problems, (3) design, (4) inquiry, and (5) teamwork. The fact that the frequency of occurrence is high in the theoretical articles on iSTEM shows that these are the principles on which there is the greatest consensus in the literature so far. However, this does not mean that they are the only ones, as additional principles are also worth mentioning: (6) student-centered, (7) hands-on, (8) twenty first century skills, (9) evaluation, (10) modeling, (11) STEM practices, (12) mathematical thinking, (13) technology literacy, and (14) STEM career. Based on their explanations, principles 6-11 are aligned with the Big Five because of their shared characteristics, leaving the last three (12-14) as principles that add new insights to the big five model.
Taking the results of the two systematic reviews into account, despite being published 6 years apart, both identify the same five fundamental principles in iSTEM education, widely recognized in the literature: (1) integration, (2) the use of real-world problems, (3) design, (4) inquiry, and (5) teamwork. Not only that, but they also coincide in some of the additional principles that collapse into the five main ones: (6) student-centered, (7) hands-on, (8) assessment/evaluation, and (9) twenty first century skills. Even so, exactly at the principle of evaluation the two reviews have somewhat different perspectives that we would like to underline in this article in order to remain faithful to their interpretations. In the case of Thibaut et al. (2018) assessment is considered a general aspect of any educational process and therefore does not need to be included in the five main principles. However, Portillo-Blanco et al. (2024) emphasize that proposals for educational practices in the classroom and in particular, proposed TLSs should contain an explicit section on the evaluation and the learning achieved by students. In this article, we understand a Teaching-Learning Sequence (TLS) as a product similar to a traditional curriculum unit package, but which includes carefully researched teaching-learning activities empirically adapted to the students’ reasoning. As well as a system for evaluating the learning achieved and the effectiveness of the educational material (Méheut and Psillos, 2004).
Figure 1 summarizes the principles defined in the two systematic reviews, with a brief explanation of each. The size of the circle refers to which are the most important in each of the reviews. Furthermore, following the color-coding in the figure, the blue circles (dark and light) are used for the principles that coincide in the two reviews, while the yellow circles are used to highlight novel ideas. On either side of the figure are two arrows indicating which principles are considered to fit into the top five according to the explanations of the two systematic reviews. In other words, the arrows indicate that the light blue principles fall within the dark blue principles. Also, three principles are left out, as they present new ideas not included in the Big Five principles’ model.
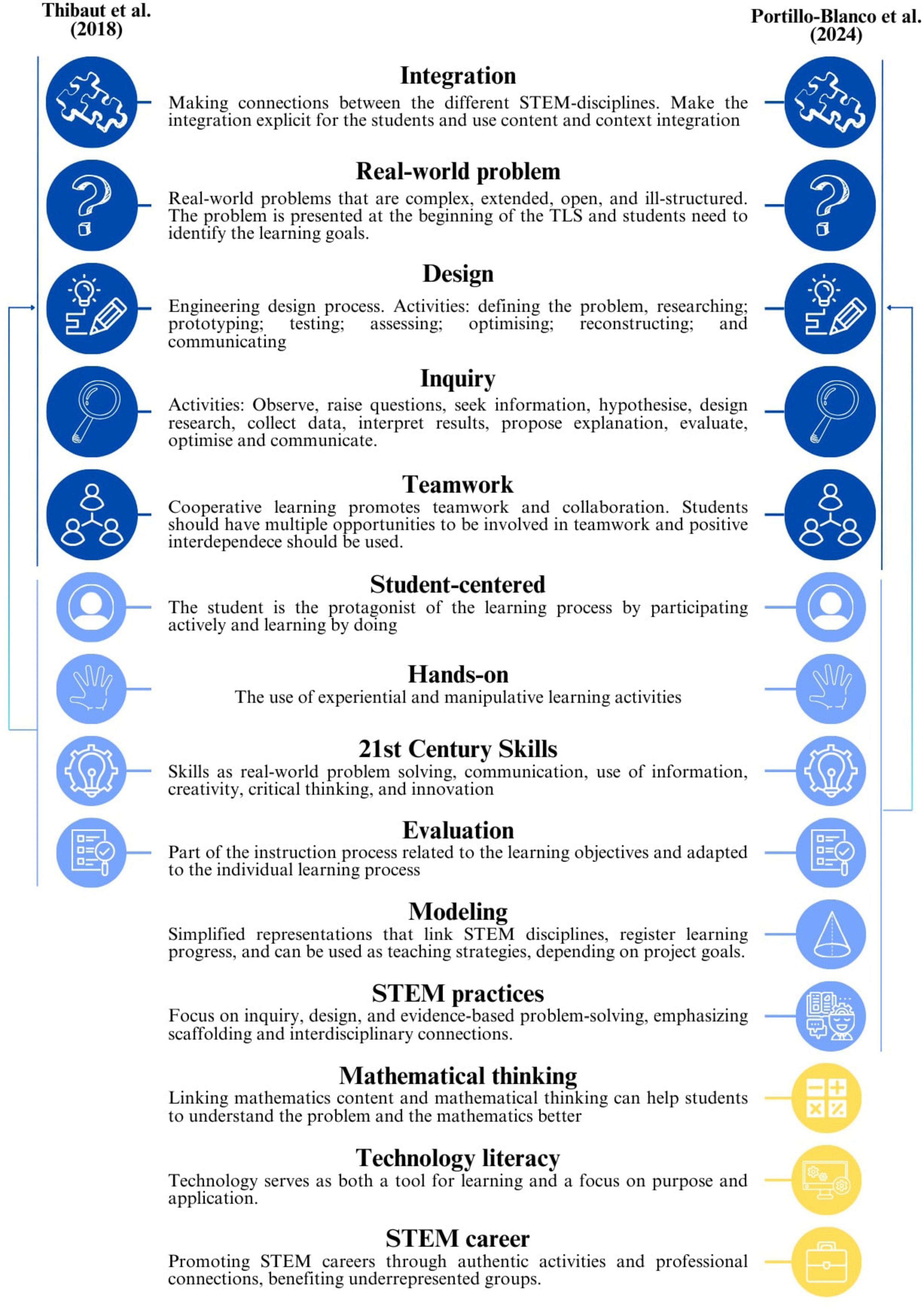
Figure 1. Summary of the principles identified in the reviews by Thibaut et al. (2018) and Portillo-Blanco et al. (2024).
The fact that two systematic reviews defining iSTEM education principles from different types of articles– Teaching-Learning Sequence designs in the case of Thibaut et al. (2018) and theoretical articles in the case of Portillo-Blanco et al. (2024) – reach similar conclusions reinforces the idea that these principles are key elements within iSTEM education. However, neither of the two reviews delves into analyzing or describing the relationship between the principles themselves. Thibaut et al. (2018) present a diagram in their paper where the five principles are shown at the same level, whereas Portillo-Blanco et al. (2024) do not explore this aspect but rather mention it as an area that should be further investigated. In fact, articles that develop theoretical models on iSTEM suffer from the same issue and tend to explain the established principles as independent modules (Kelley and Knowles, 2016; Al-Mutawah et al., 2021; Roehrig et al., 2021).
The lack of clarity regarding the interrelationship between the principles and their presentation as independent blocks raises the following questions: the two reviews agree on five principles, does this mean they are more important than the new principles added by the review of Portillo-Blanco et al. (2024)? Do the five principles with the greatest consensus have the same relevance in iSTEM education, or is there a hierarchical order among them? In the following section, we aim to analyze whether some principles are essential when designing an iSTEM TLS and to explore the implications of this potential hierarchy on instructional design.
3 Essential principles in integrated STEM education
The systematic reviews by Thibaut et al. (2018) and Portillo-Blanco et al. (2024) conclude that iSTEM education is composed of at least five principles: (1) integration, (2) the use of real-world problems, (3) design, (4) inquiry, and (5) teamwork. In addition, as can be seen in Figure 1, the most recent review of the two adds new principles that, although not as mentioned (smaller circumference size) in the theoretical frameworks published in recent years, promote new points of view to be taken into account when we talk about integrated STEM education. These include mathematical thinking, technological literacy, and STEM careers. Adding these three principles to the theoretical foundations allows us to underline the role of mathematics and technology disciplines within iSTEM and to emphasize the added value that can be generated by showing the reality of STEM jobs and the diversity of professionals that form them.
The central question of this section is whether all the principles identified in the reviews hold equal importance or if a hierarchy exists among them. The latter assumption suggests that some principles may be fundamental to iSTEM education, while others could be optional depending on the intended objectives. We define essential principles as theoretical concepts that are foundational for the implementation of an iSTEM TLS.
A possible initial response to the questions posed in this article would be to consider that the five most frequently mentioned principles constitute the fundamental and indispensable characteristics of an iSTEM educational approach. In other words, any TLS proposal in an iSTEM context should start with a real-world problem that integrates a given set of disciplines (variable according to the interpretation adopted). This problem should be addressed through activities designed with a design-based learning (DBL) approach and others focused on inquiry-based learning (IBL), all developed cooperatively. It is worth mentioning that DBL mostly reflects engineering methodology while IBL relies more on scientific methods (Kelley and Knowles, 2016; Hallstrom and Ankiewicz, 2023; Ong et al., 2023). Therefore, if these principles were mandatory, science and engineering would always be included.
Additionally, accepting that both design and inquiry are essential principles for developing a TLS iSTEM would require a dual teaching strategy that responds to each principle. This would increase the level of complexity of TLS designs to reflect the presence of both principles in activities and, at the same time, would make it difficult to find a real-world problem whose solution would always lead students to the development of the principles of inquiry and design. Moreover, this approach highlights the asymmetry of importance of the disciplines described in the articles in the field, as it leaves out the presence of mathematics and technology (English, 2016; Roehrig et al., 2021; Tytler et al., 2023). Figure 1 shows that these two principles are left out of the consensus of key principles because of the low frequency of mention, but this does not mean that their importance is minor but could be a reflection of the lack of research into real integration of mathematics and technology in iSTEM TLSs (Roehrig et al., 2021).
Examining the limitations of treating all five key principles as essential raises an alternative perspective: not all may be mandatory. This raises the question of whether each could be considered optional or, conversely, whether there is a hierarchy of importance among them. In the latter case, the principles would have varying degrees of mandatory status when designing a STEM teaching-learning sequence.
One way to examine this possible hierarchy is to examine the definitions of integrated STEM education in the literature. After all, a definition is “a precise statement of the essential nature of a thing” (Oxford English Dictionary, n.d.), which implies that the most important elements of iSTEM education should be reflected in its definition. However, as we will see, this task is complicated by the lack of consensus on what constitutes STEM education (Bybee, 2013; Martín-Páez et al., 2019).
The first common feature in the definitions of iSTEM in the literature is the integration of the disciplines that make up the acronym. However, depending on the authors this integration it is interpreted differently concerning the number of disciplines to be integrated. Several articles define iSTEM education as the integration of two or more disciplines in a real-world problem context (Sanders, 2009; Honey et al., 2014; Marrero et al., 2014; Moore et al., 2014; Lamb et al., 2015; Kelley and Knowles, 2016; Siekmann and Korbel, 2016; Nadelson and Seifert, 2017; Holmlund et al., 2018; Spikic et al., 2022; Halawa et al., 2024). Furthermore, Honey et al. (2014), Marrero et al. (2014), and Moore et al. (2014) specify that this integration can occur in a class, a specific subject, or even an entire subject. In contrast, there is another group of studies that define iSTEM education as the integration of the four disciplines (Burrows et al., 2018; Bozkurt et al., 2019; Toma, 2019; Couso and Simarro, 2020; De Loof et al., 2021). Likewise, Merrill and Daugherty (2009) understands the integration of disciplines as a new STEM meta-discipline with an integrated approach where content is undivided, providing dynamic and fluid instruction.
Therefore, despite discussions on the specifics of disciplinary integration, definitions mostly agree on the need for integration and contextualization in real-world problems. If we combine the fact that these two elements are the most frequently repeated in the definitions with the fact that they are also the principles that most often appear in the theoretical frameworks reviewed by Portillo-Blanco et al. (2024), we could assume that they are essential principles in the theoretical foundation of iSTEM education. This means that both integration and real-world problems would be at the top of the hierarchy of principles, and in its application in the classroom, an iSTEM TLS must integrate disciplines by proposing a real-world problem.
In turn, some studies propose an approach that integrates, to a greater or lesser extent, conceptual and procedural content from Science, Technology, Engineering, and Mathematics (Honey et al., 2014; Marrero et al., 2014; Baran et al., 2016; Bautista et al., 2016; Nadelson and Seifert, 2017; Martín-Páez et al., 2019; Spikic et al., 2022). Within the epistemological aspects of problem-solving, some definitions highlight the importance of engineering design in integrated STEM education (Sanders and Wells, 2006; Sanders, 2009; Shaughnessy, 2013; Berland and Steingut, 2016; Moore et al., 2020; Zhou et al., 2022). In contrast, few emphasize the use of mathematics and technology (Sanders and Wells, 2006; Shaughnessy, 2013) as well as inquiry as a specific practice (Moore and Smith, 2014).
The iSTEM education consensus models of Thibaut et al. (2018) and Portillo-Blanco et al. (2024) define inquiry and design principles among the top five and knowing they are not elements that appear with great frequency in the definitions suggests that they are not at the same level of importance as integration and real-world problems. This implies that both principles could be considered optional to some extent. The results of the systematic review by Halawa et al. (2024) shed light on this issue, since after analyzing the teaching strategies used in 229 articles on iSTEM education, they found that Design-Based Learning and Inquiry-Based Learning appeared independently. Therefore, whether one or both of these principles are used in iSTEM TLSs indicates that neither is strictly mandatory. The key to this idea lies in the impact that design and inquiry have on the definition of the problem to be solved since it must lead to the execution of the activities that make up each principle. Thus, their optional nature broadens the variety of problems defined and the resolution processes, allowing the use of one, the other, or both.
Although systematic reviews outline additional principles, iSTEM education definitions fail to clarify their significance, as they are not explicitly discussed. This could indicate that the principles of cooperative work and STEM careers may hold a lower hierarchical status within iSTEM education.
The ideas presented in this first analysis reflect that not all principles have the same weight and that integration and the context of the real-world problem are the key principles that define an iSTEM educational proposal, while the rest could be optional depending on the objectives to be achieved with the design of the iSTEM teaching-learning sequences. Due to the polysemy of the wording of both principles, it is worth specifying the meaning with which they are used in the bibliography.
The principle of “integration” is understood as the union of two or more disciplines in which certain learning objectives are shared while maintaining discipline-specific objectives (Thibaut et al., 2018; Hallstrom and Ankiewicz, 2023). Integration can be of either content, procedures, or skills and can take place through content or context (Falloon et al., 2020; Al-Mutawah et al., 2021; Roehrig et al., 2021; Smith et al., 2022).
The principle of “real-world problems” means fostering connections between different STEM disciplines in the problem, ensuring that the integration is clear and explicit to students. This involves purposefully combining content and contexts (Fan et al., 2021). The problem definition directly influences the activities and teaching approaches used in TLSs. To delve further into this perspective, it would be interesting to analyze the different types of problems proposed in the literature and the approach to solving them, but we encounter an obstacle marked by the polysemy of the terms used in the articles (Portillo-Blanco et al., 2024). Characterizing the different types of problems that can be used to initiate an iSTEM teaching-learning sequence and the impact they have on teaching approaches is a key step in determining the relationship of the principles to each other and facilitating the translation of the theoretical underpinning into the practice of sequence design. In the following section, we will therefore focus on the latter problem.
4 Key elements of iSTEM TLS design: from real-world problems to implementation
4.1 Selecting the initial problem: influence on teaching approaches
The literature suggests that an iSTEM TLS starts from the presentation of a problem in a real-world context. Following the idea that knowledge arises from confrontation with problems, and these problems are presented in the form of questions that challenge the known (Bachelard, 1993), different types of problems will lead to the construction of different knowledge. This underlines the importance of analyzing how epistemological differences between disciplines influence problem definition and problem-solving.
Falloon et al. (2020) in their theoretical framework on iSTEM education explain that the input to the problem that contextualizes a TLS can be driven by any of the disciplines that form it, allowing the level of involvement of each discipline to be modified according to the objectives to be achieved. That statement introduces a new perspective on problem definition, raising the question: How does the choice of a real-world problem vary depending on the leading discipline?
4.2 Epistemological perspectives on real-world problems in iSTEM
To answer the question posed, we must address the epistemological differences between the disciplines that form the acronym. Considering that epistemology, or the nature of the disciplines, encompasses the set of practices, methodologies, goals and values, knowledge, and social norms that characterize them and that must be recognized when teaching those disciplines (Erduran and Dagher, 2014), analyzing their differences will help us understand the diversity of problems that can be used in iSTEM education. These epistemological differences determine both the types of questions each discipline addresses and their influence on iSTEM TLS activities and objectives. Understanding this link is crucial to designing initial problems that maximize the learning potential of each discipline in an integrated context.
The epistemic differences between STEM disciplines and the relationship between them have been the subject of study in multiple investigations (Couso and Simarro, 2020; McComas and Burgin, 2020; Ortiz-Revilla et al., 2020; Pleasants, 2020; Reynante et al., 2020). Concerning scientific epistemology, in general terms, its objective is to study and develop knowledge about the natural world, describing reality through models, so they answer questions related to “why”, “what happens” or “how can we explain something” (Couso and Simarro, 2020; McComas and Burgin, 2020; Pleasants, 2020; Reynante et al., 2020). As for engineering and technology, their purpose is to manipulate the environment to meet specific needs, asking themselves questions such as “What can be built or improved?” To do so, they employ problem-oriented design processes (McComas and Burgin, 2020; Reynante et al., 2020). In this regard, mathematics focuses on producing generalizations and explanations by studying patterns and relationships between quantities, numbers, and space (McComas and Burgin, 2020; Reynante et al., 2020). This discipline has as its main objective to demonstrate or solve questions in order to generate knowledge (McComas and Burgin, 2020).
At this point, it should be noted that, although the differences between science, mathematics, and engineering/technology are well defined, in the acronym of integrated STEM education engineering and technology are presented as separate disciplines, although the literature reflects a wide debate on their relationship. Some articles defend that they are the same (McComas and Burgin, 2020), while others say that technology is within engineering and is the result of its activity (Quinn et al., 2020), or, on the contrary, that technology is a discipline of its own and that engineering is the part focused on design (García-Carmona, 2023). For the question that concerns us in this article, we will consider them as a whole and refer to engineering when talking about both the teaching approach and the real-world problem design for the context of a TLS iSTEM.
4.3 Structuring problem-solving in iSTEM education
As we have just discussed, the STEM disciplines have different epistemological goals and approaches, but they all share methodological characteristics in structuring the problem-solving process. These common methodological features are particularly interesting since they define a model of STEM activity based on three main stages in problem-solving: (A) defining the scope and understanding of the problem; (B) generating solution proposals; and (C) evaluating the solution. Figure 2 shows the three main stages of the iSTEM activity model, which we will explain below. Likewise, they are concretized with two general characteristics in the resolution process: (1) the process as a reflective practice; and (2) the process as an iterative process. All this is accompanied by decision-making with dialog in teamwork and with the rest of the teams (National Research Council, 2012; Dasgupta et al., 2019; Purzer and Quintana-Cifuentes, 2019; Ortiz-Revilla et al., 2020). Below, we explain each of the stages of STEM activity in Figure 2.
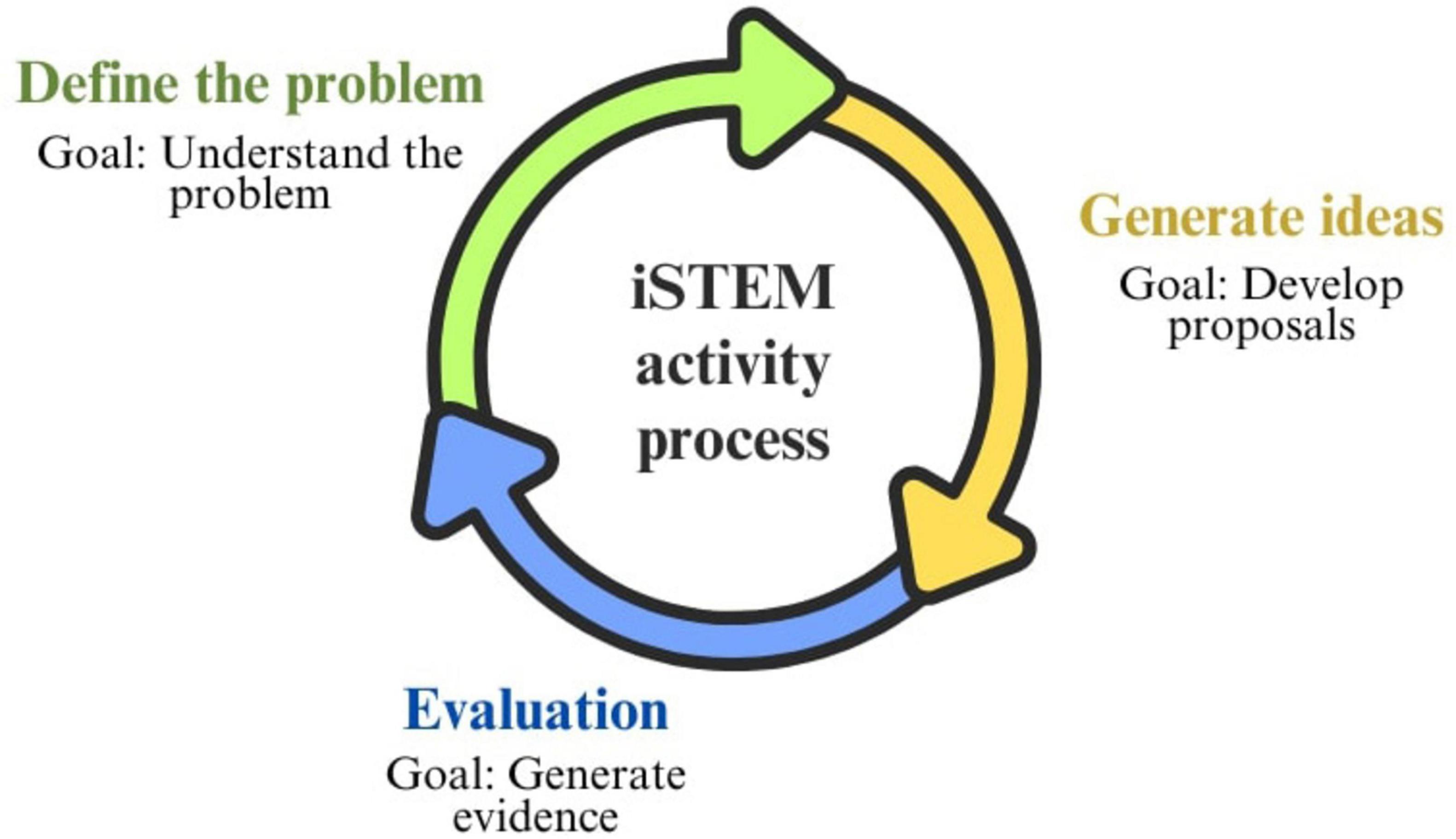
Figure 2. A model of STEM activity integrating the contributions of National Research Council (2012), Ortiz-Revilla et al. (2020), Osborne (2014) and Purzer and Quintana-Cifuentes (2019).
A. Defining the problem and understanding the challenge: The ability to formulate precise questions is essential for solving iSTEM problems. Depending on the challenge to be solved, it will be necessary to gather information about the context, problem, existing solutions, etc. Additionally, it involves determining design requirements when necessary.
B. Generating ideas, proposals, and hypotheses: At this stage, ideas are represented through sketches, drawings, and early-stage prototypes. In addition, proposals are presented that can be contrasted by different means (experiments, prototypes.) to simulate possible solutions.
C. Evaluating the process and results to assess the effectiveness of the solution: This step includes essential elements such as analyzing, visualizing, and providing evidence to argue the evaluation. Aspects such as performing experiments, simulations, data banks as evidence, etc., are also worked on.
These three stages are not only essential for structuring iSTEM learning but also allow problem-solving to be adapted to the different objectives and characteristics of each discipline. As we have explained in the model in Figure 2, their characteristics show flexibility in the resolution process, which leads to considering different paths depending on the context and characteristics of the problem posed. This flexibility explains the possibilities of using various approaches in STEM education (Purzer and Quintana-Cifuentes, 2019; Portillo-Blanco et al., 2024). In other words, there is no single approach to applying the STEM activity model in the classroom.
4.4 Epistemological approaches to problem-posing and problem-solving in iSTEM education
The analysis of problem-solving structures in the previous section has shown that common epistemic features across STEM disciplines allow for the formulation of a general problem-solving process. However, this shared framework is also flexible enough to accommodate epistemic differences between the disciplines themselves, leaving room for specific goals and methodologies. Thus, while the overall process is common, it is also adaptable. Based on this structure, the following section explores epistemological approaches to problem posing and problem solving, with an emphasis on how each discipline uniquely contributes to problem definition and problem solving in iSTEM education.
In this context, there is no single way to introduce a problem. The problem-solving process and related practices depend on the chosen approach, as each discipline has distinct epistemological objectives and methodologies (Falloon et al., 2020). As explained above, and because most articles on iSTEM education focus on engineering and not on the technology approach, in this article they are treated in a unified way. This leads us to explore three epistemological approaches to problem definition and analysis: science, engineering, and mathematics.
The importance placed on mathematics compared to science and engineering within integrated STEM education has been much lower (English, 2013; Shaughnessy, 2013; Maass et al., 2019; Mayes, 2019). Mathematics has been presented as a tool for developing knowledge in other disciplines, using content already learned (Tytler, 2020; Goos et al., 2023). Therefore, recent studies aim to address this imbalance by promoting mathematical modeling as an effective approach to integrating mathematics into iSTEM (Doğan et al., 2019; English, 2023; Goos et al., 2023). The examples presented in these articles follow both inquiry and design problems, where to solve the problem it is necessary to translate the real-life situation into mathematical language or representations (Doğan et al., 2019; English, 2023). Although the introduction to the real-world problem could be made from mathematics, the literature reflects that its presence at the moment is more of a tool for understanding or solving the problem, proposing modeling as a strategy.
Therefore, although it would be possible to define the problem from any of the disciplines that make up the acronym, both the analysis of the reality of the iSTEM TLSs and the epistemology lead us to establish two main pillars for this task: science and engineering. Returning to the epistemology of both, the main methodology of science is inquiry, while the practice of engineering is described through design. It is true that when epistemology describes science, the focus is on basic science, but applied science is far from what is described, considering that it is the application of existing knowledge to solve social problems (Roll-Hansen, 2017; Pleasants, 2020; Reynante et al., 2020; Shaw, 2022). This process of solving concrete societal problems shares many characteristics with the epistemology of engineering, making design the working methodology of applied science (Alza, 2017).
Addressing real-world problems through science (basic or applied) and engineering aligns with Halawa et al. (2024) systematic review findings. In their analysis of teaching-learning sequences in the iSTEM context, the authors conclude that the teaching strategies used in such projects are Design-Based Learning (DBL), Inquiry-Based Learning (IBL), and Problem/Project-Based Learning (PBL). IBL focuses on activities investigating scientific phenomena and generating new knowledge through experimentation and data interpretation.
On the other hand, DBL is based on engineering design, with key activities such as conceptualization, testing, and evaluation, focused on solving specific problems. Finally, Halawa et al. (2024) describe PBL as a process for addressing complex real-world problems, structured around engineering design and composed of multiple subproblems and solutions (Capraro and Slough, 2013; Wilson, 2021). The main difference between PBL and DBL lies in the open-ended nature of the problems posed in engineering designs. In summary, these strategies highlight two fundamental axes of teaching that coincide with the principles of iSTEM education: inquiry and design. Below, as an example, we provide a detailed description of some of the STEM sequence proposals with a DBL and PBL approach, mentioned in the literature.
4.4.1 Design-based learning in iSTEM education contexts
The epistemology of engineering design aims to create solutions that address specific needs while considering constraints that shape both the problem and the problem-solving process (McComas and Burgin, 2020; Reynante et al., 2020). This epistemological approach is reflected in the iSTEM education design principle (see Figure 1). However, recent contributions from engineering epistemology show that the sequence of activities focused on product development and engineering design activity is varied and flexible, responding to different types of problems (Figueiredo, 2008; Purzer et al., 2022). Different problem-solving approaches are used depending on the type of problem formulation. In particular, Purzer et al. (2022) propose a variable DBL approach for six types of problem formulation:
1. User-Centered Design (UCD) proposes to solve problems by prioritizing the understanding of the needs of users or clients and their context through observations, interviews, and other sources. It involves students in all phases of the design cycle.
2. Design-Build-Test (DBT) Focuses on building and testing prototypes to validate design concepts. It starts with clearly defined requirements and criteria, leaving aside the collection of user information.
3. Engineering Science (ENS) Analyses a designed artifact or system through a research question, intending to generate technological knowledge through controlled experiments and manipulation of specific variables.
4. Engineering Optimization (OPT) Seeks to improve suboptimal systems using clear metrics and a mathematical approach to optimize the design and evaluate the best outcome according to different criteria.
5. Engineering Analysis (EAN) Focuses on the analysis of data and mathematical models to develop evidence-based predictions and recommendations, without the need to build physical prototypes.
6. Reverse Engineering (REV) focuses on understanding an existing system or artifact through processes such as analyzing its performance, redesigning, modernizing or repairing it.
Although not specifically developed for iSTEM education, Purzer et al. (2022) introduce a range of design processes and problem types that help explain the variety of iSTEM teaching approaches. Furthermore, although it is created from the epistemology of engineering, as claimed above, design also responds to applied science. In this way, these six types can be adapted to applied science contexts to increase the variety of proposals. The literature in iSTEM education provides examples that illustrate the different kinds of problems and design processes. For example, Ortiz Revilla (2020) proposes a TLS that seeks to design the best lighting system for the study room. To solve this problem, an analysis of the characteristics of the study room must be carried out, so we could classify it within the UCD type of Purzer et al. (2022).
Another example is the proposal by Domènech-Casal (2019) in which the objective is to design and make an economic proposal for a house for certain families with specific construction requirements. In this case, the design specifications are given, so it belongs to the second type of problem: DBT. Finally, an example of TLS based on the analysis of evidence and proof is that of Domènech-Casal et al. (2018) in which the students must perform an expert appraisal of a mobile accident using the data collected in the accident. In this case, we are dealing with an EAN-type problem, where instead of designing an artifact, the analysis and interpretation of the results are carried out.
4.4.2 Inquiry-based learning in iSTEM education contexts
Inquiry-based learning focuses on the investigation of scientific phenomena to generate knowledge (Couso and Simarro, 2020; Reynante et al., 2020). Thus, problem formulation in IBL focuses on understanding ‘why’ or ‘how’ rather than solving a specific problem, offering a different perspective from the approaches discussed in the previous section (Reynante et al., 2020; Shaw, 2022).
In the inquiry principle of the Portillo-Blanco et al. (2024) review, the following activities are proposed: Observing, posing questions, seeking information, formulating hypotheses, designing investigations, collecting data, interpreting results, proposing explanations, evaluating, optimizing, and communicating. Examples of TLSs that are characterized by being mostly inquiry-based are the proposal by Dufranc et al. (2024) where the question “What would the world be like without bees and other pollinators?” or the proposal by Crippen and Archambault (2012) on how we could survive 30 years from now. Similarly, López-Banet et al. (2021) propose an iSTEM TLS that combines inquiry and modeling to evaluate the accuracy of claims in a chewing gum advertisement.
IBL as an initial problem formulation enables the creation of problems driven by curiosity and a desire for deeper understanding, allowing iSTEM education to extend beyond addressing immediate societal needs (basic science epistemological approach). Moreover, this does not mean that design and inquiry cannot be united in the same sequence, since to tackle a design problem, students must construct knowledge and inquiry is a good way to do so (Yata et al., 2020; Fan et al., 2021).
5 Discussion
Integrated STEM education (iSTEM) presents a promising, albeit complex, theoretical framework for addressing STEM education challenges. However, the diversity of its foundations often makes it difficult to understand, apply, and adapt to educational practice. This article aims to analyze the essential characteristics of iSTEM education, exploring its fundamental principles and reflecting on the hierarchical relationship between them. It responds to a need in the iSTEM education literature to move away from presenting these principles as independent blocks (Kelley and Knowles, 2016; Roehrig et al., 2021; Spikic et al., 2022) and instead create bridges between theoretical foundations and the application into the classroom (McLure et al., 2022; Spikic et al., 2022).
We began this study by analyzing two systematic reviews (Thibaut et al., 2018; Portillo-Blanco et al., 2024), which converged on the formulation of five key principles underpinning iSTEM education: (1) integration, (2) real-world problems, (3) design, (4) inquiry, and (5) teamwork. Additionally, Portillo-Blanco et al. (2024) expanded on this consensus by adding the principles of mathematical thinking, technological literacy, and STEM careers. Given this framework of agreed-upon principles, the central question posed was whether all principles hold equal importance in designing an iSTEM TLS or if there is a hierarchy among them.
To address this question, we analyzed existing definitions of iSTEM education in the literature, as the essential elements of a concept tend to be reflected in its definition. Based on their high frequency of mention, we concluded that the principles of integration and real-world problems appear to be core elements in iSTEM’s foundation. Regarding the other principles, definition analysis does not clarify their hierarchical position beyond indicating that they seem less essential than the first two. At this point, Halawa et al.’s (2024) review about teaching strategies highlights the independent and complementary use of design and inquiry in iSTEM TLSs, suggesting that these two principles may be optional.
As a next step, we focused on the principle of real-world problems, analyzing the impact of problem selection on the other principles. Following Falloon et al.’s (2020) interpretation and accepting the premise that problem introduction can originate from any discipline; we conducted an epistemological analysis to examine similarities and differences. The shared epistemological aspects of the problem-solving process revealed the possibility of structuring an iSTEM problem-solving cycle consisting of three phases: problem definition, idea generation, and evaluation. This cycle provides a common structural framework that is flexible enough to accommodate the specific characteristics of problem-solving in each discipline, allowing for a variety of initial scenarios depending on the objectives and disciplines involved.
The epistemological analysis, combined with the realities of iSTEM TLSs in the literature, showed that mathematics primarily serves as a tool for solving scientific or engineering problems. Meanwhile, when a problem is introduced through science or engineering, its resolution involves inquiry and design. These findings align with the principles defined by Thibaut et al. (2018) and Portillo-Blanco et al. (2024) as well as with Halawa et al. (2024) results on the use of design-based learning (DBL) and inquiry-based learning (IBL). Moreover, the analysis of definitions revealed a lack of consensus regarding the inclusion of these two elements, and when combined with Halawa et al.’s (2024) findings—where either one or both strategies are used—it reinforces the idea that design and inquiry, while important, may be optional or complementary principles. Similarly, these two teaching approaches follow the three-phase iSTEM activity process, where, after defining both the design and inquiry problem, they can either design a solution and evaluate it according to the design criteria or generate an investigation and evaluate the evidence from the data.
All these findings led us to propose a model illustrating the relationship between iSTEM education’s fundamental principles, as presented in Figure 3. Integration and the use of real-world problems appear at the top of the model, as the literature analysis indicates strong consensus on their necessity in designing an iSTEM TLS. Furthermore, the premise that problem definition can originate from any discipline, combined with the analysis of epistemic similarities, enabled us to develop a structured iSTEM problem-solving cycle applicable to all cases. Based on that, depending on the type of problem and its objectives, a teaching approach based on design, inquiry, or a combination of both may be used, with mathematical modeling serving as a tool. That is, while maintaining the same iSTEM activity structure, the TLS can be developed based on design-based learning, inquiry-based learning, or a combination of both, depending on the requirements of the problem. An example of the latter is the use of inquiry to develop the knowledge needed for an engineering design problem. This is why, in Figure 3, both principles are linked by “and/or.”
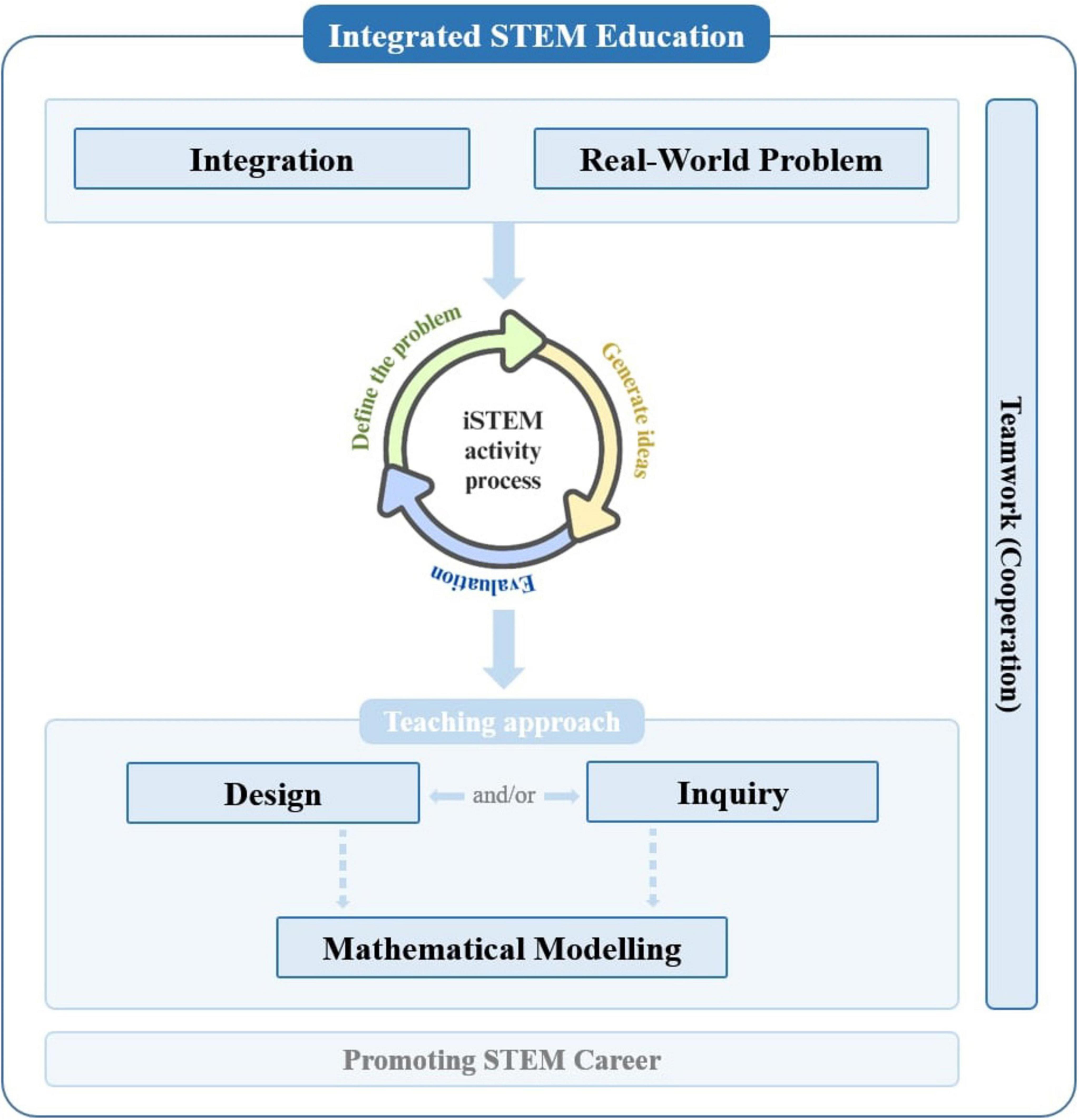
Figure 3. Diagram of the organization of the iSTEM education principles summarizing the analysis carried out in this study.
As for the role of mathematics, the literature shows its use as a tool for science and engineering. Although this does not rule out the possibility of developing a TLS iSTEM with mathematical thinking at its core, this critical review has found no evidence to place it in a different position within the model. Similarly, although technological literacy was a principle proposed by Portillo-Blanco et al. (2024), our epistemological analysis has led us to unify engineering with technology, incorporating this principle within engineering design.
Finally, although not central to this study, we emphasize the importance of teamwork and cooperation in iSTEM education and highlight the value of showcasing STEM careers to strengthen the connection between the classroom and the professional field. On the one hand, there is strong consensus in the literature regarding the importance of group work in iSTEM TLS, as it applies to any teaching strategy that may be used. For this reason, in this model, it is considered a transversal principle and is represented vertically, spanning the entire structure. On the other hand, although the STEM careers principle does not yet carry significant weight in the literature, it offers a novel perspective not present in the other principles. Thus, while an iSTEM TLS can be developed without explicitly linking classroom learning to real-world STEM careers, this principle has been placed at the bottom of the model and in a less striking color to acknowledge its relevance without positioning it at the same level of importance as the others.
The novelty of this work lies in establishing relationships among iSTEM education principles, which have previously been explained independently in the literature, and in linking theoretical perspectives with practical applications in TLSs. Additionally, we propose an approach that increases the variety of problems that can be used, decentralizing the reliance on engineering and design in iSTEM projects (Fan et al., 2021; Roehrig et al., 2021; Fang et al., 2023) and incorporating the possibility of inquiry-driven problems with a scientific epistemological foundation. Lastly, we underscore the importance of incorporating mathematical modeling into the framework of iSTEM principles to highlight its significance in iSTEM education (Doğan et al., 2019; English, 2023).
The novelty of this work lies in establishing relationships among iSTEM education principles, which have previously been explained independently in the literature, and in linking theoretical perspectives with practical applications in TLSs. Additionally, we propose an approach that increases the variety of problems that can be used, decentralizing the reliance on engineering and design in iSTEM projects (Fan et al., 2021; Roehrig et al., 2021; Fang et al., 2023) and incorporating the possibility of inquiry-driven problems with a scientific epistemological foundation. Lastly, we underscore the importance of incorporating mathematical modeling into the framework of iSTEM principles to highlight its significance in iSTEM education (Doğan et al., 2019; English, 2023).
6 Conclusion
This study proposes a framework that advances the clarification of relationships among the fundamental principles of iSTEM education. Based on the analysis of two systematic reviews (Thibaut et al., 2018; Portillo-Blanco et al., 2024), five key principles have been identified: (1) integration, (2) real-world problems, (3) design, (4) inquiry, and (5) teamwork. However, the lack of literature on the interrelation of these principles and their translation into iSTEM TLS design has sparked a debate that this work aims to clarify.
The results suggest that integration and the use of real-world problems are the fundamental pillars of iSTEM education, as they appear repeatedly in theoretical definitions and the reviewed literature. On the other hand, design and inquiry seem to play a more flexible role, being used optionally or complementarily depending on the nature of the problem posed. In this regard, Halawa et al.’s (2024) review confirms that these teaching approaches can be applied independently or in combination, reinforcing their adaptable nature within iSTEM. Additionally, epistemological analysis has highlighted the variety and impact of initial problem formulation on the application of design and inquiry principles through their respective teaching strategies when solving the problem. All these findings have enabled us to propose a relational model of the principles underpinning iSTEM education, adding a new theoretical perspective to the field.
So far, the framework proposed in this study serves as an initial theoretical construct aimed at bridging the gap between the theoretical foundations of iSTEM and their application in TLS design. Empirical research and teaching practice are needed to assess its usefulness and determine how it can be effectively applied. Therefore, future studies could focus on a detailed analysis of how integration and problem formulation decisions influence teaching strategies. Educators and researchers can leverage this framework as a practical and accessible guide for identifying and differentiating STEM design processes.
Author contributions
AP-B: Conceptualization, Data curation, Formal Analysis, Writing – original draft, Writing – review & editing. JG: Conceptualization, Funding acquisition, Supervision, Writing – review & editing. KZ: Conceptualization, Funding acquisition, Supervision, Writing – review & editing.
Funding
The author(s) declare that financial support was received for the research and/or publication of this article. This research was funded by the Spanish government MINECO\FEDER (grant no. PID2019-105172RB-I00) and the APC was founded by the Basque Country Government (Ikasgaraia Research Group IT1637/22).
Conflict of interest
The authors declare that the research was conducted in the absence of any commercial or financial relationships that could be construed as a potential conflict of interest.
Publisher’s note
All claims expressed in this article are solely those of the authors and do not necessarily represent those of their affiliated organizations, or those of the publisher, the editors and the reviewers. Any product that may be evaluated in this article, or claim that may be made by its manufacturer, is not guaranteed or endorsed by the publisher.
References
Aguilera, D., and Ortiz-Revilla, J. (2021). STEM vs. STEAM education and student creativity: A systematic literature review. Educ. Sci. 11:331. doi: 10.3390/educsci11070331
Al-Mutawah, M. A., Alghazo, Y. M., Mahmoud, E. Y., Preji, N., and Thomas, R. (2021). Designing a need-based integrated “STEAM” framework for primary schools in bahrain. Int. J. Educ. Pract. 9, 602–612. doi: 10.18488/journal.61.2021.93.602.612
Baran, E., Bilici, S. C., Mesutoglu, C., and Ocak, C. (2016). Moving STEM beyond schools: Students’ perceptions about an out-of-school STEM education program. Int. J. Educ. Math. Sci. Technol. 4, 9–19. doi: 10.18404/ijemst.71338
Bautista, A., Tan, L. S., Ponnusamy, L. D., and Yau, X. (2016). Curriculum integration in arts education: Connecting multiple art forms through the idea of ‘space.’. J. Curric. Stud. 48, 610–629. doi: 10.1080/00220272.2015.1089940
Berland, L. K., and Steingut, R. (2016). Explaining variation in student efforts towards using math and science knowledge in engineering contexts. Int. J. Sci. Educ. 38, 2742–2761. doi: 10.1080/09500693.2016.1260179
Bozkurt, A., Ucar, H., Durak, G., and Idin, S. (2019). The current state of the art in STEM research: A systematic review study. Cypriot J. Educ. Sci. 14, 374–383. doi: 10.18844/cjes.v14i3.3447
Burrows, A., Lockwood, M., Borowczak, M., Janak, E., and Barber, B. (2018). Integrated STEM: Focus on informal education and community collaboration through engineering. Educ. Sci. 8:4. doi: 10.3390/educsci8010004
Bybee, R. W. (2013). The Case for STEM Education: Challenges and Opportunities. Arlington, VI: National Science Teachers Association - NSTA Press.
Capraro, R. M., and Slough, S. W. (2013). “Why PBL? Why STEM? Why now? an Introduction to STEM project-based learning,” in STEM Project-Based Learning: An Integrated Science, Technology, Engineering, and Mathematics (STEM) Approach, eds R. M. Capraro, M. M. Capraro, and J. R. Morgan (Rotterdam: SensePublishers), 1–5. doi: 10.1007/978-94-6209-143-6_1
Chu, H.-E., Martin, S. N., and Park, J. (2019). A theoretical framework for developing an intercultural STEAM program for Australian and Korean students to enhance science teaching and learning. Int. J. Sci. Math. Educ. 17, 1251–1266. doi: 10.1007/s10763-018-9922-y
Couso, D., and Simarro, C. (2020). “STEM education through the epistemological lens: Unveiling the challenge of STEM Transdisciplinarity,” in Handbook of Research on STEM Education, eds C. C. Johnson, M. J. Mohr-Schroeder, and T. J. Moore (Milton Park: Routledge), 17–28.
Crippen, K. J., and Archambault, L. (2012). Scaffolded inquiry-based instruction with technology: A signature pedagogy for STEM education. Comput. Schools 29, 157–173. doi: 10.1080/07380569.2012.658733
Dare, E. A., Hiwatig, B., Karatithamkul, K., Ellis, J. A., Roehrig, G. H., Ring-Whalen, E. A., et al. (2021). “Improving STEM education: The design and development of a K-12 STEM observation protocol (STEM-OP),” in Proceedings of the ASEE Annual Conference proceedings, (ASEE).
Dasgupta, C., Magana, A. J., and Vieira, C. (2019). Investigating the affordances of a CAD enabled learning environment for promoting integrated STEM learning. Comput. Educ. 129, 122–142. doi: 10.1016/j.compedu.2018.10.014
De Loof, H., Boeve-de Pauw, J., and Van Petegem, P. (2021). Engaging students with integrated STEM education: A happy marriage or a failed engagement? Int. J. Sci. Math. Educ. 20, 1291–1313. doi: 10.1007/s10763-021-10159-0
Delahunty, T., and Kimbell, R. (2021). (Re)framing a philosophical and epistemological framework for teaching and learning in STEM: Emerging pedagogies for complexity. Br. Educ. Res. J. 47, 742–769. doi: 10.1002/berj.3706
Doğan, M. F., Gürbüz, R., Çavuş-Erdem, Z., and Şahin, S. (2019). Using mathematical modeling for integrating STEM disciplines: A theoretical framework. Turkish J. Comp. Math. Educ. 10, 628–653. doi: 10.16949/turkbilmat.502007
Domènech-Casal, J. (2019). Proyecto ABP casa: Áreas, porcentajes, proporciones y componentes éticas del contexto immobiliario. Uno: Revista de Didáctica de las Matematicas 71–76.
Domènech-Casal, J., Gasco, J., Royo, P., and Vilches, S. (2018). Proyecto CRASH: enseñando cinemática y dinámica en el contexto del análisis pericial de accidentes. Rev. Eureka sobre Enseñanza Divulgación Ciencias 15, 2103–2103. doi: 10.25267/Rev_Eureka_ensen_divulg_cienc.2018.v15.i2.2103
Dufranc, I. M. R. G., Cal, E. S., Terceño, E. M. G., Centeno, A. A., González, A. M., Rodríguez, M. A., et al. (2024). Mucho Más que Abejas. Servicio de Publicaciones e Imagen Institucional - Libros en Acceso Abierto. Burgos: Universidad de Burgos, doi: 10.36443/9788418465642
English, L. (2013). Modelling as a vehicle for philosophical inquiry in the mathematics curriculum. Anal. Teach. Philos. Praxis 34, 46–57.
English, L. D. (2016). STEM education K-12: Perspectives on integration. Int. J. STEM Educ. 3:3. doi: 10.1186/s40594-016-0036-1
English, L. D. (2017). Advancing elementary and middle school STEM education. Int. J. Sci. Math. Educ. 15, 5–24. doi: 10.1007/s10763-017-9802-x
English, L. D. (2023). Ways of thinking in STEM-based problem solving. ZDM Math. Educ. 55, 1219–1230. doi: 10.1007/s11858-023-01474-7
Erduran, S., and Dagher, Z. R. (2014). Reconceptualizing the Nature of Science for Science Education: Scientific Knowledge, Practices and Other Family Categories. Dordrecht: Springer Netherlands, doi: 10.1007/978-94-017-9057-4
Falloon, G., Hatzigianni, M., Bower, M., Forbes, A., and Stevenson, M. (2020). Understanding K-12 STEM education: A framework for developing STEM literacy. J. Sci. Educ. Technol. 29, 369–385. doi: 10.1007/s10956-020-09823-x
Fan, S. C., Yu, K. C., and Lin, K. Y. (2021). A framework for implementing an engineering-focused STEM curriculum. Int. J. Sci. Math. Educ. 19, 1523–1541. doi: 10.1007/s10763-020-10129-y
Fang, S.-C., Yang, K.-L., and Fan, S.-C. (2023). A conceptual framework for assessing transdisciplinary STEM practices. Res. Sci. Technol. Educ. 43, 272–293. doi: 10.1080/02635143.2023.2264781
Figueiredo, A. D. (2008). Toward an Epistemology of Engineering. Available online at: https://papers.ssrn.com/abstract=1314224 (accessed January 8, 2025).
García-Carmona, A. (2023). Integración de la ingeniería en la educación científico-tecnológica desde un prisma CTS. Enseñanza Ciencias. Rev. Invest. Experiencias Didácticas 41, 25–41. doi: 10.5565/rev/ensciencias.5611
Gil-Doménech, D., Berbegal-Mirabent, J., and Merigó, J. M. (2020). “STEM education: A bibliometric overview,” in Modelling and Simulation in Management Sciences, eds J. C. Ferrer-Comalat, S. Linares-Mustarós, J. M. Merigó, and J. Kacprzyk (Cham: Springer International Publishing), 193–205. doi: 10.1007/978-3-030-15413-4_15
Goos, M., Carreira, S., and Namukasa, I. K. (2023). Mathematics and interdisciplinary STEM education: Recent developments and future directions. ZDM Math. Educ. 55, 1199–1217. doi: 10.1007/s11858-023-01533-z
Grant, M. J., and Booth, A. (2009). A typology of reviews: An analysis of 14 review types and associated methodologies. Health Inf. Libraries J. 26, 91–108. doi: 10.1111/j.1471-1842.2009.00848.x
Gresnigt, R., Taconis, R., van Keulen, H., Gravemeijer, K., and Baartman, L. (2014). Promoting science and technology in primary education: A review of integrated curricula. Stud. Sci. Educ. 50, 47–84. doi: 10.1080/03057267.2013.877694
Halawa, S., Lin, T.-C., and Hsu, Y.-S. (2024). Exploring instructional design in K-12 STEM education: A systematic literature review. Int. J. STEM Educ. 11:43. doi: 10.1186/s40594-024-00503-5
Hallstrom, J., and Ankiewicz, P. (2023). Design as the basis for integrated STEM education: A philosophical framework. Front. Educ. 8:1078313. doi: 10.3389/feduc.2023.1078313
Holmlund, T. D., Lesseig, K., and Slavit, D. (2018). Making sense of “STEM education” in K-12 contexts. Int. J. Stem Educ. 5:11. doi: 10.1186/s40594-018-0127-2
Honey, M., The National Academy of Engineering (NAE), and National Research Council (NRC). (2014). A Descriptive Framework for Integrated STEM Education, eds M. Honey, G. Pearson, and H. Schweingruber (Ottawa: National Research Council).
Jackson, C., Mohr-Schroeder, M. J., Bush, S. B., Maiorca, C., Roberts, T., Yost, C., et al. (2021). Equity-oriented conceptual framework for K-12 STEM literacy. Int. J. Stem Educ. 8:38. doi: 10.1186/s40594-021-00294-z
Kayan-Fadlelmula, F., Sellami, A., Abdelkader, N., and Umer, S. (2022). A systematic review of STEM education research in the GCC countries: Trends, gaps and barriers. Int. J. Stem Educ. 9:2. doi: 10.1186/s40594-021-00319-7
Kelley, T. R., and Knowles, J. G. (2016). A conceptual framework for integrated STEM education. Int. J. Stem Educ. 3:11. doi: 10.1186/s40594-016-0046-z
Lamb, R., Akmal, T., and Petrie, K. (2015). Development of a cognition-priming model describing learning in a STEM classroom. J. Res. Sci. Teach. 52, 410–437. doi: 10.1002/tea.21200
Levrini, O., De Ambrosis, A., Hemmer, S., Laherto, A., Malgieri, M., Pantano, O., et al. (2017). Understanding first-year students’ curiosity and interest about physics - Lessons learned from the HOPE project. Eur. J. Phys. 38:025701. doi: 10.1088/1361-6404/38/2/025701
López-Banet, L., Perales, F.-J., and Jimenez-Liso, M. R. (2021). STEAM views from a need: The case of the chewing gum and pH sensopill (Miradas STEAM desde la necesidad: El caso de la sensopíldora chicles y pH). J. Study Educ. Dev. 44, 909–941. doi: 10.1080/02103702.2021.1927505
Maass, K., Geiger, V., Ariza, M. R., and Goos, M. (2019). The role of mathematics in interdisciplinary STEM education. ZDM Math. Educ. 51, 869–884. doi: 10.1007/s11858-019-01100-5
Marín-Marín, J.-A., Moreno-Guerrero, A.-J., Dúo-Terrón, P., and López-Belmonte, J. (2021). STEAM in education: A bibliometric analysis of performance and co-words in Web of Science. IJ Stem Ed. 8:41. doi: 10.1186/s40594-021-00296-x
Marrero, M. E., Gunning, A. M., and Germain-Williams, T. (2014). What is STEM Education? Global Education Review 1. Available online at: https://ger.mercy.edu/index.php/ger/article/view/135 (accessed December 13, 2024).
Martín-Páez, T., Aguilera, D., Perales-Palacios, F. J., and Vílchez-González, J. M. (2019). What are we talking about when we talk about STEM education? A review of literature. Sci. Educ. 103, 799–822. doi: 10.1002/sce.21522
Mayes, R. (2019). “Quantitative reasoning and its rôle in interdisciplinarity,” in Interdisciplinary Mathematics Education: The State of the Art and Beyond, eds B. Doig, J. Williams, D. Swanson, R. Borromeo Ferri, and P. Drake (Cham: Springer International Publishing), 113–133. doi: 10.1007/978-3-030-11066-6_8
McComas, W. F., and Burgin, S. R. (2020). A critique of “STEM” Education: Revolution-in-the-making, passing fad, or instructional imperative? Sci. Educ. 29, 805–829. doi: 10.1007/s11191-020-00138-2
McLure, F. I., Tang, K. S., and Williams, P. J. (2022). What do integrated STEM projects look like in middle school and high school classrooms? A systematic literature review of empirical studies of iSTEM projects. Int. J. Stem Educ. 9:73. doi: 10.1186/s40594-022-00390-8
Méheut, M., and Psillos, D. (2004). Teaching–learning sequences: Aims and tools for science education research. Int. J. Sci. Educ. 26, 515–535. doi: 10.1080/09500690310001614762
Mejias, S., Thompson, N., Sedas, R. M., Rosin, M., Soep, E., Peppler, K., et al. (2021). The trouble with STEAM and why we use it anyway. Sci. Educ. 105, 209–231. doi: 10.1002/sce.21605
Merrill, C., and Daugherty, J. (2009). “The future of TE masters degrees: STEM,” in Paper Presented at the Meeting of the International Technology Education Association, (Louisville, KY).
Millar, V. (2016). Interdisciplinary curriculum reform in the changing university. Teach. High. Educ. 21, 471–483. doi: 10.1080/13562517.2016.1155549
Millar, V. (2020). Trends, issues and possibilities for an interdisciplinary STEM curriculum. Sci. Educ. 29, 929–948. doi: 10.1007/s11191-020-00144-4
Moore, T. J., Glancy, A. W., Tank, K. M., Kersten, J. A., Smith, K. A., and Stohlmann, M. S. (2014). A framework for quality K-12 engineering education: Research and development. J. Pre College Eng. Educ. Res. 4, 1–13. doi: 10.7771/2157-9288.1069
Moore, T. J., Johnston, A. C., and Glancy, A. W. (2020). “STEM integration: A synthesis of conceptual frameworks and definitions,” in Handbook of Research on STEM Education, eds C. C. Johnson, M. J. Mohr-Schroeder, and T. J. Moore (Milton Park: Routledge).
Moore, T., and Smith, K. (2014). Advancing the state of the art of STEM integration. J. STEM Educ. 15, 5–10.
Nadelson, L. S., and Seifert, A. L. (2017). Integrated STEM defined: Contexts, challenges, and the future. J. Educ. Res. 110, 221–223. doi: 10.1080/00220671.2017.1289775
National Research Council. (2012). A Framework for K-12 Science Education: Practices, Crosscutting Concepts, and Core Ideas. Washington, DC: The National Academies Press.
Ong, Y. S., Koh, J., Tan, A.-L., and Ng, Y. S. (2023). Developing an integrated STEM classroom observation protocol using the productive disciplinary engagement framework. Res. Sci. Educ. doi: 10.1007/s11165-023-10110-z Online ahead of print.
Ortiz Revilla, J. (2020). El desarrollo competencial en la Educación Primaria: Efectos de una propuesta STEAM integrada. Doctoral dissertation. University of Burgos. Institutional Repository of the University of Burgos. doi: 10.36443/10259/5521
Ortiz-Revilla, J., Adúriz-Bravo, A., and Greca, I. M. (2020). A Framework for epistemological discussion on integrated STEM education. Sci. Educ. 29, 857–880. doi: 10.1007/s11191-020-00131-9
Ortiz-Revilla, J., Greca, I. M., and Arriassecq, I. (2022). A theoretical framework for integrated STEM education. Sci. Educ. 31, 383–404. doi: 10.1007/s11191-021-00242-x
Osborne, J. (2014). Teaching scientific practices: Meeting the challenge of change. J. Sci. Teach. Educ. 25, 177–196. doi: 10.1007/s10972-014-9384-1
Oxford English Dictionary. (n.d.). definition - Quick Search Results | Oxford English Dictionary. Available online at: https://www.oed.com/search/dictionary/?scope=Entries&q=definition (accessed January 7, 2025)
Page, M. J., McKenzie, J. E., Bossuyt, P. M., Boutron, I., Hoffmann, T. C., Mulrow, C. D., et al. (2021). The PRISMA 2020 statement: An updated guideline for reporting systematic reviews. BMJ 372:n71. doi: 10.1136/bmj.n71
Perales Palacios, F. J., and Aguilera, D. (2020). Ciencia-tecnologıa-sociedad vs. STEM: ¿evolución, revolución o disyunción? Ápice. Rev. Educ. Cientıfica 4, 1–15. doi: 10.17979/arec.2020.4.1.5826
Pleasants, J. (2020). Inquiring into the nature of STEM problems: Implications for pre-college education. Sci. Educ. 29, 831–855. doi: 10.1007/s11191-020-00135-5
Portillo-Blanco, A., Deprez, H., De Cock, M., Guisasola, J., and Zuza, K. (2024). A systematic literature review of integrated STEM education: Uncovering consensus and diversity in principles and characteristics. Educ. Sci. 14:1028. doi: 10.3390/educsci14091028
Purzer, Ş, Quintana-Cifuentes, J., and Menekse, M. (2022). The honeycomb of engineering framework: Philosophy of engineering guiding precollege engineering education. J. Eng. Educ. 111, 19–39. doi: 10.1002/jee.20441
Purzer, S., and Quintana-Cifuentes, J. P. (2019). Integrating engineering in K-12 science education: Spelling out the pedagogical, epistemological, and methodological arguments. Discip. Interdiscip. Sci. Educ. Res. 1:13. doi: 10.1186/s43031-019-0010-0
Quinn, C. M., Reid, J. W., and Gardner, G. E. (2020). S + T + M = E as a convergent model for the nature of STEM. Sci. Educ. 29, 881–898. doi: 10.1007/s11191-020-00130-w
Reynante, B. M., Selbach-Allen, M. E., and Pimentel, D. R. (2020). Exploring the promises and perils of integrated STEM through disciplinary practices and epistemologies. Sci. Educ. 29, 785–803. doi: 10.1007/s11191-020-00121-x
Roehrig, G. H., Dare, E. A., Ellis, J. A., and Ring-Whalen, E. (2021). Beyond the basics: A detailed conceptual framework of integrated STEM. Discip. Interdscip. Sci. Educ. Res 3:11. doi: 10.1186/s43031-021-00041-y
Roll-Hansen, N. (2017). A historical perspective on the distinction between basic and applied science. J. General Philos. Sci. 48, 535–551. doi: 10.1007/s10838-017-9362-3
Sanders, M. (2009). “STEM, STEM education, STEMmania,” in Paper Presented at the Technology Education Association of Pennsylvania Conference, 20–26, (Pennsylvania).
Sanders, M., and Wells, J. (2006). Integrative STEM Education Course Syllabi & Instructional Materials: STEM Education Foundations. Blacksburg, VA: Virginia Tech.
Shaughnessy, J. (2013). Mathematics in a STEM context. Math. Teach. Middle School 18:324. doi: 10.5951/mathteacmiddscho.18.6.0324
Shaw, J. (2022). Revisiting the basic/applied science distinction: The significance of urgent science for science funding policy. J. Gen. Philos. Sci. 53, 477–499. doi: 10.1007/s10838-021-09575-1
Siekmann, G., and Korbel, P. (2016). Defining “STEM” Skills: Review and Synthesis of the Literature. Support Document 1. Adelaide, SA: National Centre for Vocational Education Research Ltd.
Smith, K., Maynard, N., Berry, A., Stephenson, T., Spiteri, T., Corrigan, D., et al. (2022). Principles of problem-based learning (PBL) in STEM education: Using expert wisdom and research to frame educational practice. Educ. Sci. 12:728. doi: 10.3390/educsci12100728
Snyder, H. (2019). Literature review as a research methodology: An overview and guidelines. J. Bus. Res. 104, 333–339. doi: 10.1016/j.jbusres.2019.07.039
Spikic, S., Van Passel, W., Deprez, H., and De Meester, J. (2022). Measuring and activating iSTEM key principles among student teachers in STEM. Educ. Sci. 13:12. doi: 10.3390/educsci13010012
Tas, N., and Bolat, Y. I. (2022). An examination of the studies on STEM in education: A bibliometric mapping analysis. Int. J. Technol. Educ. Sci. 6, 477–494. doi: 10.46328/ijtes.401
Thibaut, L., Ceuppens, S., De Loof, H., De Meester, J., Goovaerts, L., Struyf, A., et al. (2018). Integrated STEM education: A systematic review of instructional practices in secondary education. Eur. J. STEM Educ. 3:2. doi: 10.20897/ejsteme/85525
Toma, R. B. (2019). STEM Education in Elementary Grades: Design of An Effective Framework for Improving Attitudews Toward School Science. Burgos: Universidad de Burgos.
Torraco, R. J. (2005). Writing integrative literature reviews: Guidelines and examples. Hum. Resour. Dev. Rev. 4, 356–367. doi: 10.1177/1534484305278283
Tytler, R. (2020). “STEM education for the twenty-first century,” in Integrated Approaches to STEM Education: An International Perspective, eds J. Anderson and Y. Li (Cham: Springer International Publishing), 21–43. doi: 10.1007/978-3-030-52229-2_3
Tytler, R., Anderson, J., and Williams, G. (2023). Exploring a framework for integrated STEM: Challenges and benefits for promoting engagement in learning mathematics. ZDM Math. Educ. 55, 1299–1313. doi: 10.1007/s11858-023-01519-x
Vázquez-Alonso, Á, andManassero-Mas, M.-A. (2015). La elección de estudios superiores coentífico-técnicos: Análisis de algunos factores determinantes en seis países. Rev. Eur. Sobre Enseñanza Divulgación Ciencias 12, 264–277. doi: 10498/17251
Whittemore, R., and Knafl, K. (2005). The integrative review: Updated methodology. J. Adv. Nurs. 52, 546–553. doi: 10.1111/j.1365-2648.2005.03621.x
Wilson, K. (2021). Exploring the challenges and enablers of implementing a STEM project-based learning programme in a diverse junior secondary context. Int. J. Sci. Math. Educ. 19, 881–897. doi: 10.1007/s10763-020-10103-8
Yata, C., Ohtani, T., and Isobe, M. (2020). Conceptual framework of STEM based on japanese subject principles. Int. J. Stem Educ. 7:12. doi: 10.1186/s40594-020-00205-8
Zhan, Z., Shen, W., Xu, Z., Niu, S., and You, G. (2022). A bibliometric analysis of the global landscape on STEM education (2004-2021): Towards global distribution, subject integration, and research trends. Asia Pac. J. Innov. Entrepreneurship 16, 171–203. doi: 10.1108/APJIE-08-2022-0090
Keywords: integrated STEM education, iSTEM education, features of iSTEM teaching-learning sequence, teaching approach, design-based learning, inquiry-based learning
Citation: Portillo-Blanco A, Guisasola J and Zuza K (2025) Integrated STEM education: addressing theoretical ambiguities and practical applications. Front. Educ. 10:1568885. doi: 10.3389/feduc.2025.1568885
Received: 30 January 2025; Accepted: 04 April 2025;
Published: 23 April 2025.
Edited by:
Zahid Pranjol, University of Sussex, United KingdomReviewed by:
Eleni Kolokouri, University of Ioannina, GreeceStanley M. Lo, University of California, San Diego, United States
Copyright © 2025 Portillo-Blanco, Guisasola and Zuza. This is an open-access article distributed under the terms of the Creative Commons Attribution License (CC BY). The use, distribution or reproduction in other forums is permitted, provided the original author(s) and the copyright owner(s) are credited and that the original publication in this journal is cited, in accordance with accepted academic practice. No use, distribution or reproduction is permitted which does not comply with these terms.
*Correspondence: Ane Portillo-Blanco, YW5lLnBvcnRpbGxvQGVodS5ldXM=