- 1Saint Loup Research Institute, Saint-Loup-Lamairé, France
- 2ECOSYS Unit, AgroParisTech, Université Paris-Saclay, Thiverval-Grignon, France
- 3General and Theoretical Ecology, University of Bremen, Bremen, Germany
- 4HEPIA, University of Applied Sciences and Arts of Western Switzerland, Jussy, Switzerland
- 5Soil and Crop Sciences Section, School of Integrative Plant Science, Cornell University, Ithaca, NY, United States
- 6Department of Biosystems Engineering, University of Costa Rica, San José, Costa Rica
Over the last two decades, the sequestration of carbon in soils has often been advocated as a solution to mitigate the steady increase in the concentration of CO2 in the atmosphere, one of the most commonly mentioned causes of climate change. A large body of literature, as well as sustained efforts to attract funding for the research on soil organic matter, have focused on the soil carbon sequestration – climate change nexus. However, because CO2 is not the only greenhouse gas released by soils, and given the fact that the feasibility of large-scale carbon sequestration in soils remains controversial, this approach does not appear optimal to convince policy makers to invest in soils. In this perspective article, we argue that a far better strategy revolves around the effect of climate change on functions/services that soils render. In particular, since climatologists forecast less frequent but more intense rainfall events in the future, which may lead to food shortages, catastrophic flooding, and soil erosion if soils are not able to cope, a more suitable focus of the research would be to increase soil organic matter content so as to strengthen the water regulation function of soils. The different conceptual and methodological shifts that this new focus will require are discussed in detail.
Background – Re-Storing Carbon in Soils
Over the last two decades, soil scientists have addressed a number of challenges associated with soil organic matter (SOM) in the context of climate change. In particular, various authors have argued that since soils often exhibit a sizeable carbon deficit relative to historical levels 50 or 60 years ago, there is a significant potential for them to re-store large amounts of carbon, and thereby, in principle, contribute to decelerating climate change or even halting it (e.g., Paustian et al., 1997, 2000; Swift, 2001; Kimble et al., 2002; Lal, 2004). This theme of the possible sequestration of carbon in soils, also referred to as the “recarbonization” of soils (Food and Drug Organization [FAO], 2019), and especially the controversial question of precisely how much additional C storage one can expect realistically, have since become a major focus of the research on SOM, resulting in scores of conferences every year and a plethora of scholarly articles on the topic (e.g., Lal et al., 2015; Johannes et al., 2017; Torres-Sallan et al., 2017; Poulton et al., 2018; Schiefer et al., 2018; Smith et al., 2018; Chen et al., 2019; Keel et al., 2019; Gessesse et al., 2020; Loisel et al., 2019; Harvey, 2020; Hairiah et al., 2020; Kogut and Semenov, 2020; Moinet et al., 2020; Wiesmeier et al., 2020).
The emphasis on carbon re-storing is understandable, given two key trends that have manifested in recent years. The first is that the public and political discourse on climate change has revolved predominantly around the concentration of CO2 in the atmosphere, which has the advantage of being a simple enough metric to grasp. In this context, it is hardly surprising that researchers seeking funds to work on SOM have used as a sales pitch the reduction in atmospheric CO2 that might result from carbon storage in soils (Minasny et al., 2017; Lal, 2018). The second trend is related to the development of carbon markets, which present great attractiveness for financial institutions and governments, and have received exponentially increasing attention in recent years (e.g., Abdul-Salam et al., 2019). Some view the inclusion of soil carbon sequestration efforts in this context as a cunning strategy not only to support agriculture economically and make it more sustainable in the long run, but also to incentivize farmers and land owners to preserve soil resources.
As long as, to meet political and economic expectations, research by SOM specialists remains fixated almost single-mindedly on the carbon storage – atmospheric CO2 nexus, as seems to be the case now, we run the risk of missing important aspects of the climate change problem. Greenhouse gases (GHGs) include other chemical compounds than just CO2, some of which are far more potent than the latter. Methane (CH4) is estimated to have a Global Warming Potential (GWP) that is between 28 and 36 times that of CO2 over 100 years, whereas nitrous oxide (N2O) has a GWP between 265 and 298 over a similar 100-year timescale. Lugato et al. (2018) have argued that efforts to increase carbon storage in soils may result in the release in the atmosphere of significant amounts of N2O, an observation that is also supported by the fact that soil rich in C (for example, organic soils) tend to give rise to the highest N2O emissions (Pärn et al., 2018). Therefore, the net mitigating effect of soil carbon sequestration may be less than expected, yet unfortunately, there is no consensus at the moment on the issue. Several meta-analyses have evaluated how different C accrual strategies affect N2O emissions, but the results are not conclusive. For instance, the effect of adding organic amendments on N2O emissions is very dependent on the degree of stabilization of the material as well as many other factors (Chen et al., 2013; Cayuela et al., 2017). Reduced tillage, another strategy that has been promoted to store C, has been found to affect N2O emissions in different ways and may sometimes be associated to increased use of environmentally detrimental herbicides. In a meta-analysis comparing more than forty studies, van Kessel et al. (2013) concluded that N2O emissions relative to production (yield-scaled emissions) increased more than 50% in studies that had reduced tillage for less than 10 years, while in long-term studies a reduction of 27% was observed. The use of cover crops is also widely acknowledged as an approach to increase SOC. Basche et al. (2014) found great variability between studies, with an increase in emissions in 60% of the cases and a decrease in 40%. Their conclusion is that, when studies of a year or more are considered, the impact of cover crops on N2O emissions is negligible, but they stress the need for more long-term field studies to get robust conclusions.
The connection of soil carbon with GHG emissions gets even more murky when one looks not just at soils, but at entire agricultural systems. For example, the conversion of an agricultural field to a pasture may result in an increase in carbon storage in the subsurface over a certain period of time, before the system reaches a new equilibrium. However, as pointed out, e.g., by White et al. (2018), as soon as one puts grazing animals on that pasture, CH4 emissions tend to increase drastically, leading to a net GHG abatement that can be less than predicted solely on the basis of soil carbon storage.
Perspective Shift: Restoring Soil Functions
Since the link between carbon sequestration in soils and the concentration of GHGs in the atmosphere is clearly not as easy to establish as was once thought, GHG reduction alone does not appear to be an appropriate driver to promote SOM research. A possible alternative approach to sell the research on soils to the general public would be to review in detail the various functions of soils and the crucial services they provide to human populations (cf Figure 1)1, to find out the extent to which each one of them could help mitigate climate change. However, our opinion is that a far better sales pitch for the research on SOM arises if we turn the looking glass around, and focus instead on the opposite perspective of the effects that climate change already has now, or is forecasted to increasingly have in the very near future, on the various soil functions on which society crucially depends. In this respect, the “4 per 1000 initiative,” launched by the French agriculture minister in 2015, included food security as one of its targets, even if, since, one could argue that it has been paid scarcely more than lip service in the related literature. Corbeels et al. (2019) opined recently that SOM researchers should focus on the production of food instead of on the release of GHGs. Undoubtedly, starvation and malnutrition are already fueling massive population exodus toward several industrialized countries, in particular from Sub-Saharan Africa to Europe and from Central America to the United States. In the longer run, the prospect of having to feed 9 or 10 billion people by the year 2050 is a daunting challenge, of which soil scientists ought to be constantly mindful. Our experience indicates that agro industries are very concerned by this problem. However, even though malnutrition has been a critical geopolitical issue for decades (e.g., de Castro, 1956), politicians appear to have demonstrated even less eagerness to tackle it seriously than they evince toward climate change. Therefore, one could argue that it would be wise for us to try to “sell” our research by emphasizing other soil functions/services, directly influenced by SOM content, that cause such societal concern at the moment that they would be more susceptible to incite politicians into action.
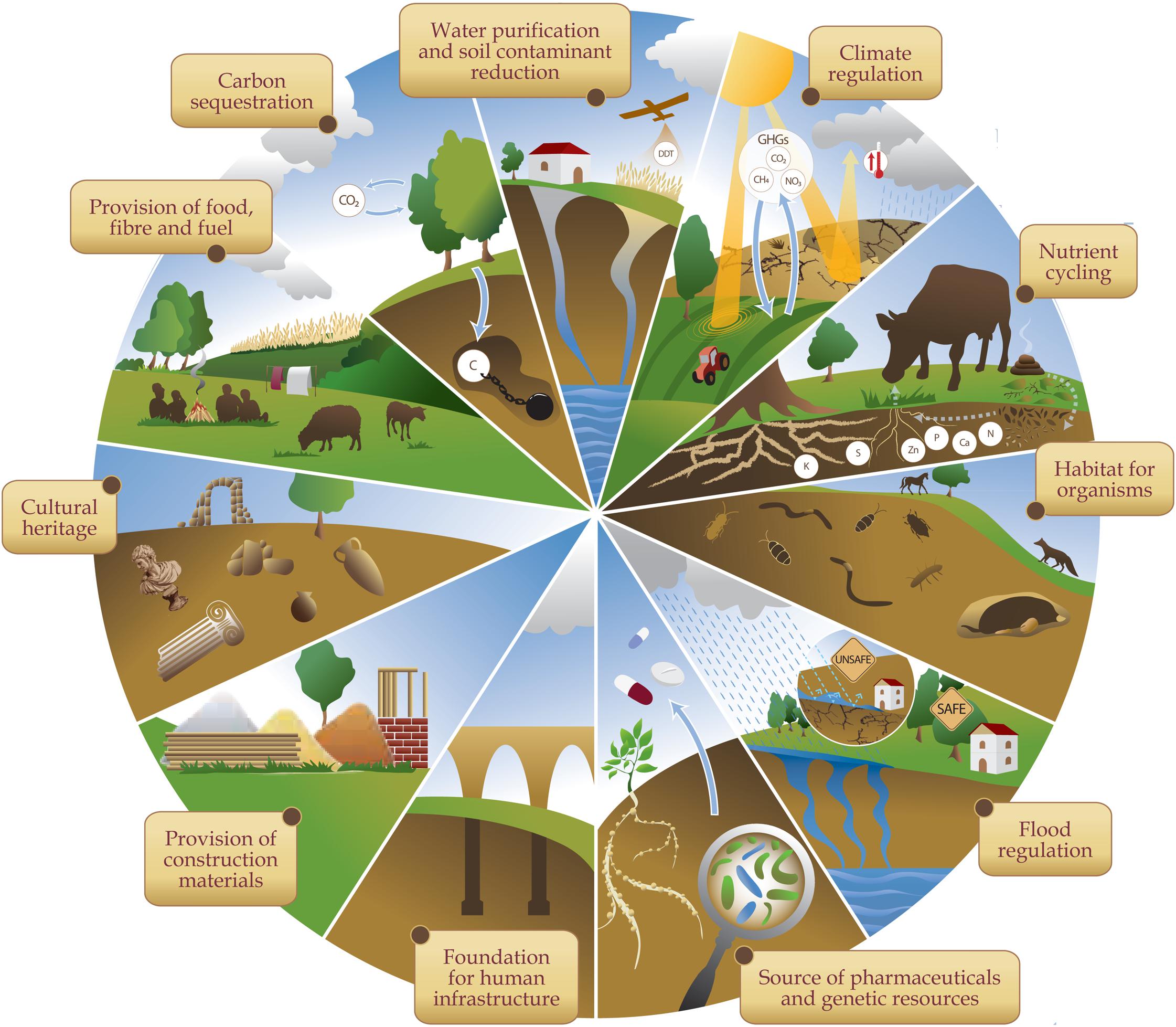
Figure 1. Schematic diagram of soil functions. This diagram is part of an uncommented “infographics” on soil functions put together by the FAO, with the subtitle “Soils deliver ecosystem services that enable life on earth.” This particular diagram considers “carbon sequestration” and “climate regulation” functions separately (Adapted from http://www.fao.org/resources/infographics/infographics-details/en/c/284478/).
The downward trends in SOM contents in various parts of the world have been amply documented in recent years, as well as the significant effect that rising temperature can have in that respect (e.g., Conant et al., 2011; Johnston and Sibly, 2018). To understand the potential emergencies we face in this context, one needs to start by considering the effects that decreases in organic matter content have on a number of key soil properties. One could go down the list of physical, (bio)chemical, and biological properties, and demonstrate that the loss of organic matter has a significant impact on virtually all of them. But to make our point, it suffices here to consider physical properties.
With few exceptions (e.g., Santos et al., 1989; Radulovich et al., 1992), organic matter plays a key role in binding mineral particles together in most soils, and in making their architecture resistant, in particular to raindrop impacts at the soil surface or to protracted ponding (e.g., Rabot et al., 2018). Surface horizons depleted in organic matter tend to disperse more easily during rainfall events, and even in some cases tend to form largely impermeable crusts (Bresson and Boiffin, 1990; Bielders and Baveye, 1995; Bielders et al., 1996; Obale-Ebanga, 2001; Janeau et al., 2003), both of which accelerate the occurrence of ponding and runoff. This process diminishes the flood regulation function of soils, and in some extreme cases of erosion, where dense subsurface horizons become exposed, drastically decreases their potential for food production. Upon drying, dispersed clayey soils often have a tendency to become cemented, making it difficult for plants to germinate and grow.
As a corollary to the less stable architecture that results from the loss of organic matter, research has demonstrated that it also renders soils more vulnerable to compaction, which is increasingly regarded as one of the leading causes of soil degradation. This effect has become particularly acute in countries where mechanized agriculture is practiced and where, over the years, steadily heavier equipment has been used. In this respect, one might be tempted to think that the situation is not worrisome yet, and that it will take years for soils to get compacted to an extent that raises concern. But in some regions of the world, evidence to the contrary are in plain sight (Figure 2), and things are already now bordering on catastrophic, as soil researchers have repeatedly pointed out (Horn et al., 1995; Boivin et al., 2006; Gabarron-Galeote et al., 2019; Keller et al., 2019; Pöhlitz et al., 2020). Anecdotally, farmers one of us (P.C. B.) interviewed in the Picardie region in France (Northeast of Paris) have observed over the last two decades, as the organic matter content of their soils kept decreasing, that it became more and more difficult to grow their key cash crops, especially sugar beets. The reason is clearly due in part to increasing sensitivity of the soil to raindrop impacts, but predominantly to compaction by the hugely heavy “integral” machines now used (in the Fall, when the soils are wet) to harvest sugar beets. The biggest of these harvesters weigh more than 63 tons when their bunkers are full of beets. Many of the farmers in Picardie consider that they will no longer be able to grow sugar beets in a few (<10) years, at which point some of those who are old enough are planning to simply end their farming practice and retire.
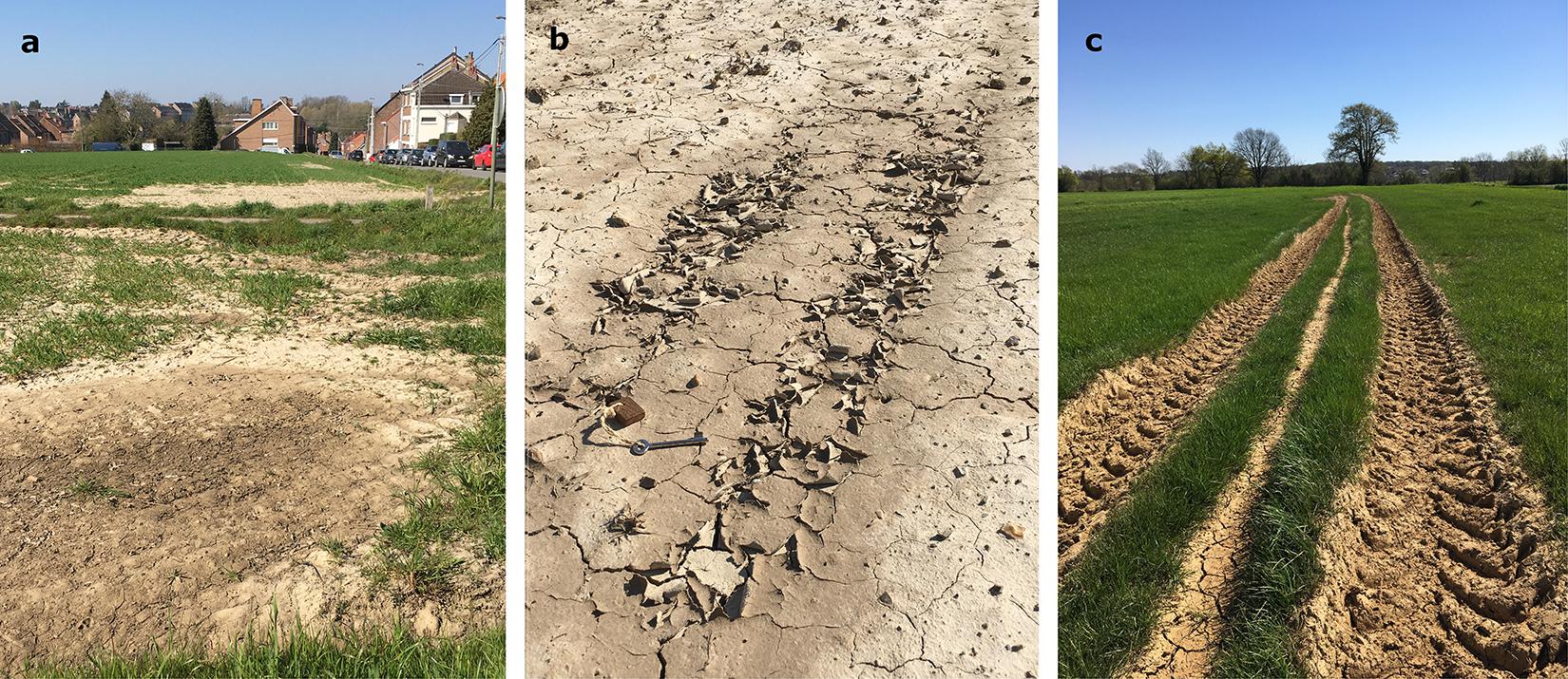
Figure 2. Example of soil degradation brought about by compaction. (a) Field in Braine-l’Alleud (Belgium) where the use of heavy machinery, including sugar beet harvesters, over the years has resulted in soil compaction, lack of drainage, and difficulty for crops to germinate, (b) in this same field, evidence of dispersion of the soil at the surface, which at this point (April 4, 2020) is as hard as concrete when dry, (c) field soil in Lamairé (Deux-Sèvres, France) where pesticide application using heavy machinery has resulted in intense compaction. At various places, the tractor tracks are 20 cm deep, and the soil is once again extremely hard.
These known facts about organic matter losses in soils take on significant urgency in the context of the ongoing climate change. One of the consistent predictions climate modelers have made over the last decade is that climate change will result in less frequent but more intense rainfall events in many parts of the world (e.g., Trenberth et al., 2003; Sun et al., 2007; Min et al., 2011; Ipcc, 2013; Intergovernmental Panel on Climate Change, 2014; Kendon et al., 2014; Berghuijs et al., 2017; Tang et al., 2019; Hess et al., 2020; Morán-Ordóñez et al., 2020; O’Donnell and Thorne, 2020). In North America, meteorological records indicate that heavy precipitation events have already increased in a large number of areas (Melillo et al., 2014). In the U.S. Midwest, for example, the quantity of precipitation occurring in the largest one percent of all daily events has increased by almost 40% over the last 60 years (Pryor et al., 2014). In the United Kingdom, modelers predicted a few years ago that heavy Summer downpours would become significantly more frequent in years to come (Kendon et al., 2014). A study carried out by the Met Office (2020) on Storm Desmond, which brought widespread flooding to Northern England and Southern Scotland in 2015, found such events have been made 59% more likely by climate change. This ongoing “rainfall intensification” process spells serious trouble in a number of ways on virtually all soils but especially so on soils that have lost a significant portion of their organic matter, because the problems that are already apparent in these soils now will get amplified in the future.
Protracted dry spells lead to significant wind erosion of soils in dryland areas, representing 40% of the world’s land surface (Millennium Ecosystem Assessment, 2005) and 30% of the world population (UNCCD, 2014). This wind erosion gives rise to dust storms, which had not been seen since the 1930s but reappeared about a decade ago in various parts of the world (e.g., Baveye et al., 2011; McLeman et al., 2014; Barbosa and Olsson, 2019; Eekhout and De Vente, 2019; Middleton et al., 2019), and are forecasted to become even more frequent in the future. Beside dust storms, longer dry spells between rains could also cause severe water deficits for plants, e.g., crop failure in agricultural fields, and they could prolong the “hunger season” (the interval between two cropping seasons) in vulnerable and market-decoupled regions. Unless one builds massive numbers of artificial reservoirs, the water deficits will be impossible to compensate via irrigation since available groundwater or surface water resources are already severely overdrawn, causing a whole suite of environmental problems. In addition, more intense rainfall events generally mean quicker ponding at the soil surface and increased runoff, which in turn cause more severe soil erosion, an issue that has become critical in many countries (e.g., Nearing et al., 2004; Morán-Ordóñez et al., 2020; Wang et al., 2020). Beside via soil erosion, higher-intensity rainfalls may also have other effects on soil carbon storage, when the latter is increased through the incorporation of pyrolyzed organic material (e.g., agrichar or biochar). Physical modeling indeed shows that, under ponding conditions, agrichar or biochar particles have a tendency to rise to the soil surface because of their buoyancy, and to be transferred to runoff waters (e.g., Wang et al., 2013).
The higher runoff that one is likely to observe, either directly as a result of the higher rainfall intensity or indirectly, e.g., as a consequence of fires caused by drought (e.g., Candela et al., 2005; Papathanasiou et al., 2012), should also contribute to increase the frequency of severe floods and in particular “flashfloods,” at least in some parts of the world, causing significant damages to infrastructures, forcing 500 million people to flee their homes (UNCCD, 2014), and leading to the frequent loss of lives. The intensity and severity of the problem is heightened in many countries by the widespread sealing of soils, in particular because of urban sprawl (e.g., Miller and Hutchins, 2017; Skougaard Kaspersen et al., 2017; Rudd et al., 2020). In 2018, a team of researchers in Germany and the United States (Willner et al., 2018) concluded that in the next two decades, most of the United States, Central Europe, Northeast and West Africa, as well as large parts of India and Indonesia, will require intensive and onerous adaptation efforts, at least at double the current level, to keep high-end fluvial flood risk in check.
Future Research and Practice
In this context, the imminent threats associated with rainfall intensification, yield stagnation, and land degradation seem to us to constitute a far better argument to convince decision-makers to fund research on SOM, than the disputed connection between soil carbon sequestration and GHG emissions from soils. Such a shift of focus of the research will require changes in a number of respects.
First, the research will have to concentrate predominantly on the top portion of soil profiles. In the past decade, in part because plant roots often extend deep into soils (e.g., Baveye and Laba, 2015), some researchers have endeavored to estimate soil carbon stocks at depths between one and two meters (e.g., Baker et al., 2007; Rumpel and Kögel-Knabner, 2011; Börjesson et al., 2018). This makes sense from a carbon re-storing perspective, as does the premise that any form of carbon, regardless of how much it contributes to the development or maintenance of a suitable soil architecture, is acceptable as input to increase stocks. However, neither one of these perspectives is meaningful from the viewpoint of making soils rapidly more resilient to rain intensification or of regenerating soil functions at an acceptable level. In fact, the focus on carbon storage may lead to the adoption of oversimplified practices, e.g., deep plowing (Alcantara et al., 2016, 2017; Keel et al., 2019), that appear convincing to decision makers but turn out eventually to be very deleterious to soils functions.
Second, to make sure that soils and their functions are apprehended in all their inherent (and often daunting) complexity, research teams will have to be more interdisciplinary than they generally are at present. A break with tradition is direly needed in that respect (Baveye and Wander, 2019; Baveye, 2020). Clearly, soil physicists and soil fauna specialists, who at the moment tend to be only peripherally if at all associated with the research on soil carbon sequestration, will have to be intimately involved in the work that will need to be carried out to understand key processes at the microscale in soils. Apart from anecic earthworm burrows aiding water infiltration, little to nothing is known mechanistically about the interactions between soil fauna and physical or chemical soil characteristics. It has been known for a long time that grazing microarthropods stimulate microbial activity and thus speed up OM degradation (Uvarov, 1985). But it is not clear which species of either group are involved, if this phenomenon holds true in soils of different mineral makeup, and how this will impact plant growth, plant-microbe interactions, or SOM stocks over the longer term. Soil physicists, zoologists, microbiologists, (bio)chemists, as well as agronomists will have to collaborate closely to resolve such questions (Briones, 2014; Filser et al., 2016). In particular, measurements will need to be carried out, and experiments designed, to assess not merely the amount of carbon re-stored in soils at any given time, but also how and to what extent this stored carbon improves the hydrology of soils, which molecular compounds are most effective in this context, and which organisms are instrumental in the transformation of added organic residues into these molecular compounds. From a broader perspective, to determine how these microscale processes affect the capacity of soils to regulate the cycle of water, it will be necessary to monitor simultaneously the dynamics of SOM as well as the water regulation function of soils under different conditions. Research carried out recently by Chalhoub et al. (2020) shows for the first time how direct measurements of soil water regulation services can be carried out in the field. All that will be needed to move forward will be to combine these measurements with a detailed analysis of the composition and evolution of SOM.
The focus on functions and services of soils in SOM research will necessitate interdisciplinary collaboration of a different kind as well. Experience has shown that in situations related to soil use that require a change in human attitude, soil expertise is in general ineffective, unless it is complemented by assistance from sociologists or psychologists. In this case, not only will experts from these disciplines need to be involved in the research, to gain insight into the human side of things, but stakeholders themselves (in particular farmers) will also have to be intimately associated with the work or even lead the questioning it will encompass. To achieve effective agri-environmental management, one will need to reenergize the research on indicators of what constitutes proper management, and their data requirements (Burton and Schwarz, 2013). The motivation of farmers to increase the organic matter content of their soil is a key condition of success. Soil researchers must acknowledge that decision making occurs at farm scale under heavy pressure of the value chain, and that, compared to that pressure, pure soil-related technical considerations or norms are of little relevance to trigger change (Seguin et al., 2007; Amundson and Biardeau, 2018, 2019). In that context, our contacts with farmers suggest that increasing the soil productivity, and eventually maintaining it over the long haul, is a much stronger motivation for farmers at the moment than C sequestration. In any broadening of the scope to include other soil functions than just food production, farmers will have to be brought on board from the onset.
A third key change will also stem directly from the focus on functions and services, which by their very nature are very much affected by local conditions. As a result, the practical solutions that the research will try to identify will require intensive knowledge acquisition at the local scale, and will likely defy attempts at one-size-fits-all approaches imposed by global C markets.
Even though relatively profound changes will be required in research programs focusing on the effect of climate change on key soil functions, that does not mean that traditional research ought to stop altogether on carbon storage in soils or on practical approaches currently being investigated to sequester carbon. Cover crops, relay- or intercropping, conservation agriculture practices, and a return to mixed forms of agriculture (including both crop production and animal husbandry), which are currently advocated in various areas as convenient ways to incorporate more fresh plant residues and nitrogen in soils, are potentially useful also to restore the flood-regulation function of soils or their capacity to produce food. Undoubtedly, new approaches, which have not yet been developed or tested, will be needed as well, and will likely differ from region to region.
Author Contributions
All authors contributed equally and substantially to the discussion that led to this perspective manuscript. PCB wrote a first draft of the manuscript, integrated editing inputs from all the other co-authors, and submitted the final version with their approval.
Conflict of Interest
The authors declare that the research was conducted in the absence of any commercial or financial relationships that could be construed as a potential conflict of interest.
Acknowledgments
Sincere gratitude is expressed to Dr. María Luz Cayuela (Spain) for providing several useful leads to the literature on N2O emissions from soils, and for general comments on the manuscript.
Footnotes
- ^ As defined here, soil functions are the benefits that nature in general, including human populations, derive from soils. Soil services refer specifically to the subset of benefits that humans derive from soils (see e.g., Baveye et al., 2016; Vogel et al., 2018, 2019).
References
Abdul-Salam, Y., Hawes, C., Roberts, D., and Young, M. (2019). The economics of alternative crop production systems in the context of farmer participation in carbon trading markets. Agroecol. Sustain. Food Syst. 43, 67–91. doi: 10.1080/21683565.2018.1537986
Alcantara, V., Don, A., Vesterdal, L., Well, R., and Nieder, R. (2017). Stability of buried carbon in deep-ploughed forest and cropland soils - implications for carbon stocks. Sci. Rep. 7:5511.
Alcantara, V., Don, A., Well, R., and Nieder, R. (2016). Deep ploughing increases agricultural soil organic matter stocks. Glob. Change. Biol. 22, 2939–2956. doi: 10.1111/gcb.13289
Amundson, R., and Biardeau, L. (2018). Opinion: Soil carbon sequestration is an elusive climate mitigation tool. Proc. Natl. Acad. Sci. U.S.A. 115, 11652–11656. doi: 10.1073/pnas.1815901115
Amundson, R., and Biardeau, L. (2019). Reply to Loisel et al.: Soil in climate mitigation and adaptation. Proc. Natl. Acad. Sci. U.S.A. 116, 10213. doi: 10.1073/pnas.1905360116
Baker, J. M., Ochsner, T. E., Venterea, R. T., and Griffis, T. J. (2007). Tillage and soil carbon sequestration—What do we really know? Agric. Ecosyst. Environ. 118, 1–5. doi: 10.1016/j.agee.2006.05.014
Barbosa, H., and Olsson, L. (2019). Climate Change and Land: An IPCC Special Report on Climate Change, Desertification, Land Degradation, Sustainable Land Management, Food Security, and Greenhouse Gas Fluxes in Terrestrial Ecosystems. Geneva: IPCC.
Basche, A. D., Miguez, F. E., Kaspar, T. C., and Castellano, M. J. (2014). Do cover crops increase or decrease nitrous oxide emissions? A meta-analysis. J. Soil Water Conserv. 69, 471–482. doi: 10.2489/jswc.69.6.471
Baveye, P. C. (2020). Bypass and hyperbole in soil research: Worrisome practices critically reviewed through examples. Eur. J. Soil Sci. doi: 10.1111/ejss.12941
CrossRef Full Text Online ahead of print | Google Scholar
Baveye, P. C., Baveye, J., and Gowdy, J. (2016). Soil ‘ecosystem’ services and natural capital: Critical appraisal of research on uncertain ground. Front. Environ. Sci. 4:41. doi: 10.3389/fenvs.2016.00041
Baveye, P. C., and Laba, M. (2015). Moving away from the geostatistical lamppost: Why, where, and how does the spatial heterogeneity of soils matter? Ecol. Modell. 298, 24–38. doi: 10.1016/j.ecolmodel.2014.03.018
Baveye, P. C., Rangel, D., Jacobson, A. R., Laba, M., Darnault, C., Otten, W., et al. (2011). From Dust Bowl to Dust Bowl: Soils are still very much a frontier of science. Soil Sci. Soc. Am. J. 75, 2037–2048. doi: 10.2136/sssaj2011.0145
Baveye, P. C., and Wander, M. (2019). The (bio)chemistry of soil humus and humic substances: Why is the “new view” still considered novel after more than 80 years? Front. Environ. Sci. 7:27. doi: 10.3389/fenvs.2019.00027
Berghuijs, W., Aalbers, E., Larsen, J., Trancoso, R., and Woods, R. (2017). Recent changes in extreme floods across multiple continents. Environ. Res. Lett. 12, 114035. doi: 10.1088/1748-9326/aa8847
Bielders, C. L., and Baveye, P. (1995). Processes of structural crust formation on coarse-textured soils. Eur. J. Soil Sci. 46, 221–232. doi: 10.1111/j.1365-2389.1995.tb01830.x
Bielders, C. L., Baveye, P., Wilding, L. P., Drees, L. R., and Valentin, C. (1996). Tillage-induced spatial distribution of surface crusts on a sandy Paleustult from Togo. Soil Sci. Soc. Am. J. 60, 843–855. doi: 10.2136/sssaj1996.03615995006000030024x
Boivin, P., Schäffer, B., Temgoua, E., Gratier, M., and Steinman, G. (2006). Assessment of soil compaction using soil shrinkage modelling: Experimental data and perspectives. Soil Tillage Res. 88, 65–79. doi: 10.1016/j.still.2005.04.008
Börjesson, G., Bolinder, M. A., Kirchmann, H., and Kätterer, T. (2018). Organic carbon stocks in topsoil and subsoil in long-term ley and cereal monoculture rotations. Biol. Fertil. Soils 54, 549–558. doi: 10.1007/s00374-018-1281-x
Bresson, L.-M., and Boiffin, J. (1990). Morphological characterization of soil crust development stages on an experimental field. Geoderma 47, 301–325. doi: 10.1016/0016-7061(90)90035-8
Briones, M. J. I. (2014). Soil fauna and soil functions: a jigsaw puzzle. Front. Environ. Sci. 2:7. doi: 10.3389/fenvs.2014.00007
Burton, R. J. F., and Schwarz, G. (2013). Result-oriented agri-environmental schemes in Europe and their potential for promoting behavioural change. Land Use Policy 30, 628–641. doi: 10.1016/j.landusepol.2012.05.002
Candela, A., Aronica, G., and Santoro, M. (2005). Effects of forest fires on flood frequency curves in a mediterranean catchment. Hydrol. Sci. J. 50, null–206. doi: 10.1623/hysj.50.2.193.61795
Cayuela, M. L., Aguilera, E., Sanz-Cobena, A., Adams, D. C., Abalos, D., Barton, L., et al. (2017). Direct nitrous oxide emissions in Mediterranean climate cropping systems: Emission factors based on a meta-analysis of available measurement data. Agric. Ecosyst. Environ. 238, 25–35. doi: 10.1016/j.agee.2016.10.006
Chalhoub, M., Gabrielle, B., Tournebize, J., Chaumont, C., Maugis, P., Girardin, C., et al. (2020). Direct measurement of selected soil services in a drained agricultural field: Methodology development and case study in Saclay (France). Ecosyst. Serv. 42:101088. doi: 10.1016/j.ecoser.2020.101088
Chen, H., Li, X., Hu, F., and Shi, W. (2013). Soil nitrous oxide emissions following crop residue addition: a meta-analysis. Glob. Change Biol. 19, 2956–2964. doi: 10.1111/gcb.12274
Chen, S., Arrouays, D., Angers, D. A., Chenu, C., Barré, P., Martin, M. P., et al. (2019). National estimation of soil organic carbon storage potential for arable soils: A data-driven approach coupled with carbon-landscape zones. Sci. Total Environ. 666, 355–367. doi: 10.1016/j.scitotenv.2019.02.249
Conant, R. T., Ryan, M. G., Agren, G. I., Birge, H. E., Davidson, E. A., Eliasson, P. E., et al. (2011). Temperature and soil organic matter decomposition rates - synthesis of current knowledge and a way forward. Glob. Change Biol. 17, 3392–3404. doi: 10.1111/j.1365-2486.2011.02496.x
Corbeels, M., Cardinael, R., Naudin, K., Guibert, H., and Torquebiau, E. (2019). The 4 per 1000 goal and soil carbon storage under agroforestry and conservation agriculture systems in Sub-Saharan Africa. Soil Tillage Res. 188, 16–26. doi: 10.1016/j.still.2018.02.015
Eekhout, J. P. C., and De Vente, J. (2019). How soil erosion model conceptualization affects soil loss projections under climate change. Prog. Phys. Geogr. Earth Environ. 44, 212–232. doi: 10.1177/0309133319871937
Filser, J., Faber, J. H., Tiunov, A. V., Brussaard, L., Frouz, J., De Deyn, G., et al. (2016). Soil fauna: Key to new carbon models. Soil 2, 565–582. doi: 10.5194/soil-2-565-2016
Food and Drug Organization [FAO] (2019). Recarbonization of global soils - A tool to support the implementation of the Koronivia Joint Work on Agriculture. Rome: FAO.
Gabarron-Galeote, M. A., Hannam, J. A., Mayr, T., and Jarvis, P. J. (2019). BEETSOIL: A decision support tool for forecasting the impact of soil conditions on sugar beet harvest. Soil Tillage Res. 191, 131–141. doi: 10.1016/j.still.2019.04.001
Gessesse, T. A., Khamzina, A., Gebresamuel, G., and Amelung, W. (2020). Terrestrial carbon stocks following 15 years of integrated watershed management intervention in semi-arid Ethiopia. Catena 190, 104543. doi: 10.1016/j.catena.2020.104543
Hairiah, K., van Noordwijk, M., Sari, R. R., Saputra, D. D., Widianto, Suprayogo, D., et al. (2020). Soil carbon stocks in Indonesian (agro) forest transitions: Compaction conceals lower carbon concentrations in standard accounting. Agric. Ecosyst. Environ. 294:106879. doi: 10.1016/j.agee.2020.106879
Harvey, C. (2020). Soils Store Huge Amounts of Carbon, Warming May Unleash it. Available at: https://www.scientificamerican.com/article/soils-store-huge-amounts-of-carbon-warming-may-unleash-it/ (aceessed June 21, 2020).
Hess, L. J. T., Hinckley, E.-L. S., Robertson, G. P., and Matson, P. A. (2020). Rainfall intensification increases nitrate leaching from tilled but not no-till cropping systems in the U.S. midwest. Agric. Ecosyst. Environ. 290:106747. doi: 10.1016/j.agee.2019.106747
Horn, R., Domżżał, H., Słowińska-Jurkiewicz, A., and van Ouwerkerk, C. (1995). Soil compaction processes and their effects on the structure of arable soils and the environment. Soil Compaction Environ. 35, 23–36. doi: 10.1016/0167-1987(95)00479-C
Intergovernmental Panel on Climate Change (2014). Climate Change 2013 – The Physical Science Basis: Working Group I Contribution to the Fifth Assessment Report of the Intergovernmental Panel on Climate Change. Cambridge: Cambridge University Press, doi: 10.1017/CBO9781107415324
Ipcc. (2013). “Climate change 2013: the physical science basis,” in Contribution of Working Group I to the Fifth Assessment Report of the Intergovernmental Panel on Climate Change, eds T. F. Stocker, D. Qin, G.-K. Plattner, M. Tignor, S. K. Allen, J. Boschung, et al. (Cambridge: Cambridge University Press).
Janeau, J. L., Bricquet, J. P., Planchon, O., and Valentin, C. (2003). Soil crusting and infiltration on steep slopes in Northern Thailand. Eur. J. Soil Sci. 54, 543–554. doi: 10.1046/j.1365-2389.2003.00494.x
Johannes, A., Matter, A., Schulin, R., Weisskopf, P., Baveye, P. C., and Boivin, P. (2017). Optimal organic carbon values for soil structure quality of arable soils: Does clay content matter?”. Geoderma 302, 14–21. doi: 10.1016/j.geoderma.2017.04.021
Johnston, A. S. A., and Sibly, R. (2018). The influence of soil communities on the temperature sensitivity of soil respiration. Nat. Ecol. Evol. 2, 1597–1602. doi: 10.1038/s41559-018-0648-6
Keel, S. G., Anken, T., Büchi, L., Chervet, A., Fliessbach, A., Flisch, R., et al. (2019). Loss of soil organic carbon in Swiss long-term agricultural experiments over a wide range of management practices. Agric. Ecosyst. Environ. 286, 106654. doi: 10.1016/j.agee.2019.106654
Keller, T., Sandin, M., Colombi, T., Horn, R., and Or, D. (2019). Historical increase in agricultural machinery weights enhanced soil stress levels and adversely affected soil functioning. Soil Tillage Res. 194:104293. doi: 10.1016/j.still.2019.104293
Kendon, E. J., Roberts, N. M., Fowler, H. J., Roberts, M. J., Chan, S. C., and Senior, C. A. (2014). Heavier summer downpours with climate change revealed by weather forecast resolution model. Nat. Clim. Change 4, 570–576. doi: 10.1038/nclimate2258
Kimble, J. M., Lal, R., and Follett, R. F. (2002). Agricultural Practices and Policies for Carbon Sequestration in Soil. Boca Ratonm: CRC Press, 2002.
Kogut, B. M., and Semenov, V. M. (2020). Estimation of soil saturation with organic carbon. Dokuchaev Soil Bull. 102, 103–124. doi: 10.19047/0136-1694-2020-102-103-124
Lal, R. (2004). Soil carbon sequestration impacts on global climate change and food security. Science 304:1623. doi: 10.1126/science.1097396
Lal, R. (2018). Digging deeper: A holistic perspective of factors affecting soil organic carbon sequestration in agroecosystems. Glob. Change Biol. 24, 3285–3301. doi: 10.1111/gcb.14054
Lal, R., Negassa, W., and Lorenz, K. (2015). Carbon sequestration in soil. Environ. Change Issues 15, 79–86. doi: 10.1016/j.cosust.2015.09.002
Loisel, J., Casellas Connors, J. P., Hugelius, G., Harden, J. W., and Morgan, C. F. (2019). Soils can help mitigate CO2 emissions, despite the challenges. Proc. Natl. Acad. Sci. U.S.A. 116, 10211–10212. doi: 10.1073/pnas.1900444116
Lugato, E., Leip, A., and Jones, A. (2018). Mitigation potential of soil carbon management overestimated by neglecting N2O emissions. Nat. Clim. Chang. 8, 219–223. doi: 10.1038/s41558-018-0087-z
McLeman, R. A., Dupre, J., Ford, L. B., Ford, J., Gajewski, K., and Marchildon, G. (2014). What we learned from the Dust Bowl: Lessons in science, policy, and adaptation. Popul. Environ. 35, 417–440. doi: 10.1007/s11111-013-0190-z
Melillo, J. M., Richmond, T. C., and Yohe, G. W. (eds) (2014). “Climate change impacts in the United States: The third national climate assessment,” in Proceedings of the 2014 National Climate Assessment. U.S. Global Change Research Program (Washington, DC: USGCRP).
Met Office (2020). Record Breaking Rainfall. Available online at: https://www.metoffice.gov.uk/about-us/press-office/news/weather-and-climate/2020/2020-winter-february-stats (accessed August 29, 2020).
Middleton, N., Tozer, P., and Tozer, B. (2019). Sand and dust storms: underrated natural hazards. Disasters 43, 390–409. doi: 10.1111/disa.12320
Miller, J. D., and Hutchins, M. (2017). The impacts of urbanisation and climate change on Urban flooding and urban water quality: A review of the evidence concerning the United Kingdom. J. Hydrol. 12, 345–362. doi: 10.1016/j.ejrh.2017.06.006
Min, S.-K., Zhang, X., Zwiers, F. W., and Hegerl, G. C. (2011). Human contribution to more-intense precipitation extremes. Nature 470, 378–381. doi: 10.1038/nature09763
Minasny, B., Malone, B. P., McBratney, A. B., Angers, D. A., Arrouays, D., Chambers, A., et al. (2017). Soil carbon 4 per mille. Geoderma 292, 59–86. doi: 10.1016/j.geoderma.2017.01.002
Moinet, G. Y. K., Moinet, M., Hunt, J. E., Rumpel, C., Chabbi, A., and Millard, P. (2020). Temperature sensitivity of decomposition decreases with increasing soil organic matter stability. Sci. Total Environ. 704:135460. doi: 10.1016/j.scitotenv.2019.135460
Morán-Ordóñez, A., Duane, A., Gil-Tena, A., De Cáceres, M., Aquilué, N., Guerra, C. A., et al. (2020). Future impact of climate extremes in the mediterranean: soil erosion projections when fire and extreme rainfall meet. Land Degrad. Dev. doi: 10.1002/ldr.3694
CrossRef Full Text Online ahead of print | Google Scholar
Nearing, M. A., Pruski, F. F., and O’Neal, M. R. (2004). Expected climate change impacts on soil erosion rates: A review. J. Soil Water Conserv. 59, 43–50.
Obale-Ebanga, F. (2001). Impacts of Agricultural Land use Histories on Soil Organic Matter Dynamics and Related Properties of Savannah soils in North Cameroon. Amsterdam: Universiteit van Amsterdam.
O’Donnell, E. C., and Thorne, C. R. (2020). Drivers of future urban flood risk. Phil. Trans. R. Soc. A 378, 20190216. doi: 10.1098/rsta.2019.0216
Papathanasiou, C., Alonistioti, D., Kasella, A., Makropoulos, C., and Mimikou, M. (2012). The impact of forest fires on the vulnerability of periurban catchments to flood events (The case of the Eastern Attica region). Glob. Nest J. 14, 294–302. doi: 10.30955/gnj.000877
Pärn, J., Verhoeven, J. T. A., Butterbach-Bahl, K., Dise, N. B., Ullah, S., Aasa, A., et al. (2018). Nitrogen-rich organic soils under warm well-drained conditions are global nitrous oxide emission hotspots. Nat. Commun. 9:11351.
Paustian, K., Andrén, O., Janzen, H. H., Lal, R., Smith, P., Tian, G., et al. (1997). Agricultural soils as a sink to mitigate CO2 emissions. Soil Use Manag. 13, 230–244. doi: 10.1111/j.1475-2743.1997.tb00594.x
Paustian, K., Six, J., Elliott, E. T., and Hunt, H. W. (2000). Management options for reducing CO2 emissions from agricultural soils. Biogeochemistry 48, 147–163. doi: 10.1023/A:1006271331703
Pöhlitz, J., Rücknagel, J., Schlüter, S., Vogel, H.-J., and Christen, O. (2020). Estimation of critical stress ranges to preserve soil functions for differently textured soils. Soil Tillage Res. 200:104637. doi: 10.1016/j.still.2020.104637
Poulton, P., Johnston, J., Macdonald, A., White, R., and Powlson, D. (2018). Major limitations to achieving ‘4 per 1000’ increases in soil organic carbon stock in temperate regions: evidence from long-term experiments at rothamsted research, United Kingdom. Glob. Change Biol. 24, 2563–2584. doi: 10.1111/gcb.14066
Pryor, S. C., Scavia, D., Downer, C., Gaden, M., Iverson, L., and Nordstrom, R. (2014). “Midwest,” in Climate Change Impacts in the United States: The Third National Climate Assessment. US Global Change Research Program, ed. J. M. Melillo (Washington, DC: USGCRP), 418–440. doi: 10.7930/J0J1012N
Rabot, E., Wiesmeier, M., Schlüter, S., and Vogel, H.-J. (2018). Soil structure as an indicator of soil functions: a review. Geoderma 314, 122–137. doi: 10.1016/j.geoderma.2017.11.009
Radulovich, R., Sollins, P., Baveye, P., and Solórzano, E. (1992). Bypass water flow through unsaturated microaggregated tropical soils.”. Soil Sci. Soc. Am. J. 56, 721–726. doi: 10.2136/sssaj1992.03615995005600030008x
Rudd, A. C., Kay, A. L., Wells, S. C., Aldridge, T., Cole, S. J., Kendon, E. J., et al. (2020). Investigating potential future changes in surface water flooding hazard and impact. Hydrol. Process. 34, 139–149. doi: 10.1002/hyp.13572
Rumpel, C., and Kögel-Knabner, I. (2011). Deep soil organic matter—a key but poorly understood component of terrestrial C cycle. Plant and Soil 338, 143–158. doi: 10.1007/s11104-010-0391-5
Santos, M. C. D., Ribeiro, M. R., and Mermut, A. R. (1989). Submicroscopy of clay microaggregates in an oxisol from Pernambuco, Brazil. Soil Sci. Soc. Am. J. 53, 1895–1901. doi: 10.2136/sssaj1989.03615995005300060047x
Schiefer, J., Lair, G. J., Lüthgens, C., Wild, E. M., Steier, P., and Blum, W. E. H. (2018). The increase of soil organic carbon as proposed by the ‘4/1000 Initiative’ is strongly limited by the status of soil development - A case study along a substrate age gradient in Central Europe. Sci. Total Environ. 628– 629, 840–847.
Seguin, B., Arrouays, D., Balesdent, J., Soussana, J.-F., Bondeau, A., Smith, P., et al. (2007). Moderating the impact of agriculture on climate. Agric. Forest Meteorol. 142, 278–287. doi: 10.1016/j.agrformet.2006.07.012
Skougaard Kaspersen, P., Høegh Ravn, N., Arnbjerg-Nielsen, K., Madsen, H., and Drews, M. (2017). Comparison of the impacts of urban development and climate change on exposing European cities to pluvial flooding. Hydrol. Earth Syst. Sci. 21, 4131–4147. doi: 10.5194/hess-21-4131-2017
Smith, P., Lutfalla, S., Riley, W. J., Torn, M. S., Schmidt, M. W. I., and Soussana, J.-F. (2018). The changing faces of soil organic matter research. Eur. J. Soil Sci. 69, 23–30. doi: 10.1111/ejss.12500
Sun, Y., Solomon, S., Dai, A., and Portmann, R. W. (2007). How often will it rain? J. Clim. 20, 4801–4818. doi: 10.1175/JCLI4263.1
Tang, F. H. M., Riley, W. J., and Maggi, F. (2019). Hourly and daily rainfall intensification causes opposing effects on C and N emissions, storage, and leaching in dry and wet grasslands. Biogeochemistry 144, 197–214. doi: 10.1007/s10533-019-00580-7
Torres-Sallan, G., Schulte, R. P. O., Lanigan, G. J., Byrne, K. A., Reidy, B., Simó, I., et al. (2017). Clay illuviation provides a long-term sink for C sequestration in subsoils. Sci. Rep. 7:45635. doi: 10.1038/srep45635
Trenberth, K. E., Dai, A., Rasmussen, R. M., and Parsons, D. B. (2003). The changing character of precipitation. Bull. Am. Meteorol. Soc. 84, 1205–1218. doi: 10.1175/BAMS-84-9-1205
Uvarov, A. V. (1985). “Energetic evaluation of the role of soil invertebrates in the process of plant remains decomposition,” in Proceedings of the 9th International Colloquium on Soil Zoology, ed. B. R. Striganova (Moscow: Moscow Nauka).
van Kessel, C., Venterea, R., Six, J., Adviento-Borbe, M. A., Linquist, B., and van Groenigen, K. J. (2013). Climate, duration, and N placement determine N2O emissions in reduced tillage systems: A meta-analysis. Glob. Change Biol. 19, 33–44. doi: 10.1111/j.1365-2486.2012.02779.x
Vogel, H.-J., Bartke, S., Daedlow, K., Helming, K., Kögel-Knabner, I., Lang, B., et al. (2018). A systemic approach for modeling soil functions. Soil 4, 83–92. doi: 10.5194/soil-4-83-2018
Vogel, H.-J., Eberhardt, E., Franko, U., Lang, B., Ließ, M., Weller, U., et al. (2019). Quantitative evaluation of soil functions: Potential and state. Front. Environ. Sci. 7:164. doi: 10.3389/fenvs.2019.00164
Wang, C., Walter, M. T., and Parlange, J.-Y. (2013). Modeling simple experiments of biochar erosion from soil. J. Hydrol. 499, 140–145. doi: 10.1016/j.jhydrol.2013.06.055
Wang, L., Zhang, F., Fu, S., Shi, X., Chen, Y., Jagirani, M. D., et al. (2020). Assessment of soil erosion risk and its response to climate change in the mid-Yarlung Tsangpo river region. Environ. Sci. Pollut. Res. 27, 607–621. doi: 10.1007/s11356-019-06738-y
White, R. E., Davidson, B., Lam, S. K., and Chen, D. (2018). A critique of the paper “Soil carbon 4 per mille” by Minasny et al. (2017). Geoderma 309, 115–117. doi: 10.1016/j.geoderma.2017.05.025
Wiesmeier, M., Mayer, S., Burmeister, F., Hübner, R., and Kögel-Knabner, I. (2020). Feasibility of the 4 per 1000 initiative in Bavaria: A reality check of agricultural soil management and carbon sequestration scenarios. Geoderma 369, 114333. doi: 10.1016/j.geoderma.2020.114333
Keywords: carbon sequestration, rainfall pattern, soil architecture, infiltration, flooding, erosion, runoff
Citation: Baveye PC, Schnee LS, Boivin P, Laba M and Radulovich R (2020) Soil Organic Matter Research and Climate Change: Merely Re-storing Carbon Versus Restoring Soil Functions. Front. Environ. Sci. 8:579904. doi: 10.3389/fenvs.2020.579904
Received: 03 July 2020; Accepted: 17 August 2020;
Published: 10 September 2020.
Edited by:
Sonoko D. Bellingrath-Kimura, Leibniz Centre for Agricultural Landscape Research (ZALF), GermanyReviewed by:
Masayuki Kawahigashi, Tokyo Metropolitan University, JapanAung Zaw Oo, National Institute for Agro-Environmental Sciences, Japan
Copyright © 2020 Baveye, Schnee, Boivin, Laba and Radulovich. This is an open-access article distributed under the terms of the Creative Commons Attribution License (CC BY). The use, distribution or reproduction in other forums is permitted, provided the original author(s) and the copyright owner(s) are credited and that the original publication in this journal is cited, in accordance with accepted academic practice. No use, distribution or reproduction is permitted which does not comply with these terms.
*Correspondence: Philippe C. Baveye, UGhpbGlwcGUuQmF2ZXllQEFncm9QYXJpc1RlY2guZnI=; YmF2ZXllLnJwaUBnbWFpbC5jb20=
†This article is dedicated to the memory of Professor Ricardo Radulovich, who passed away on July 25, 2020, after a short fight against cancer. Professor Radulovich is best known worldwide for his pioneering vision of how seafarming could help alleviate the food security challenge in coming decades, but we are very grateful that occasionally he would still accept enthusiastically to share his experience of soils, as he did during the writing of this article