- 1Hydrobiogeochemistry and Pollution Control Laboratory, Department of Environmental Sciences, Jahangirnagar University, Dhaka, Bangladesh
- 2Department of Environmental Sciences, Jahangirnagar University, Dhaka, Bangladesh
Occurrences and exposure to high levels of microbial bioaerosols such as pathogenic bacteria, fungi, fungal spores, and viruses can be linked to the deterioration of the environment and public health. This study aimed to review the results available for the unusual bioaerosol distribution scenario in the Asian regions. The amount of bioaerosol load and their environmental behavior in the atmosphere is heavily influenced by air pollution such as haze, fog, dust, and particulate matter (PM) and thus strongly affect the air quality index (AQI). Human factors such as heavy traffic, overcrowds, and biomass burning also affected the prevalence or occurrences of bioaerosols in the atmosphere. Seasonal/temporal and diurnal variation was significantly observed from these studies and in the case of South Asia, post-monsoon and winter months were incredibly concentrated with pathogenic bioaerosols. Many human infections, for example, pneumonia, tuberculosis, brucellosis, anthrax, and query fever (Q-fever), are linked to pathogenic bacterial bioaerosols. Respiratory diseases such as asthma and chronic pulmonary obstructiveness are related to fungal bioaerosols, spores, and viral infections. To facilitate the testing and monitoring appraisal of airborne bioaerosols, artificial intelligence, deep neural networks, and machine learning can be used to develop real-time PCR-based bioaerosol sensors. Moreover, mobile apps and compatible electronic gadgets can be developed for the city dwellers to real-time monitor the concentration of bioaerosols in the air they are breathing.
Introduction
In urban areas, global anthropogenic air pollution is pervasive (Groulx et al., 2018). The rapid urbanization, industrial activities, substantial energy consumption, and economic growth are indicated by a significant increase in traffic and the worsening air quality in the developing countries (Lu et al., 2018; Yan et al., 2019). High ambient particulate matter (PM) exposure can be responsible for unintended human health effects (Lu et al., 2018). Repeated hazy days can be caused by fine particulate matter (PM2.5) in China, and many other Asian megacities have caused substantial environmental and public health crises (Xie et al., 2018). Subsequently, World Air Quality Report 2019 stated the accretion of airborne PM and its atmospheric and environmental disturbances around the Asian and South Asian countries (IQAir 2020). Yet in 2020, lockdown actions to reduce COVID-19 pandemic across many Asian megacities have resulted in a substantial shift in air pollution.
When a consortium of airborne microorganisms of numerous sizes and types are present in the air in association with PMs, it is called airborne particles or bioaerosols (Caruana, 2011). It combines solids and semi-solids material in association with biotic and abiotic pieces with sizes ranging from 0.001 nm to 100 μm (Humbal et al., 2018). Bioaerosols may comprise 15–25% of PM by mass combined with living or dead bacteria, fungi, viruses, secondary metabolites, pollens, and dust (Ghosh et al., 2015; Samake et al., 2017). The study of airborne bioaerosols has gained significant interest in recent years (Fatahinia et al., 2018). Bioaerosols can result in infectious diseases, respiratory and chronic health issues (Valdez-Castillo and Arriaga 2021). Considering the recent COVID-19 pandemic, bioaerosols might have a significant role to play in the transmission of coronavirus (Noorimotlagh et al., 2020). A growing quantity of data implying that the airborne microbial portion of the particulate matter is responsible for critically escalating adverse effects on public health (Gandolfi et al., 2013).
Bacteria contribute significantly to the occurrence and composition of airborne aerosols formed by seasonal changes induced by the atmospheric and local terrestrial environment (Bowers et al., 2012). Furthermore, concentrations of cultured bacteria and fungi showed a strong connection to the AQI. When AQI <200, the concentration of airborne fungi gradually increased while AQI>200 increased airborne bacteria (Yan et al., 2019). Chemical composition, along with microbiological compositions, is significant in identifying the relationships between PM and AQI and, thus, the microorganism residing in them (Gandolfi et al., 2013). However, the question is why bioaerosols increase with air pollution remaining unclear. One hypothesis may be that high or extreme pollution levels primarily affect the diversity and composition of bioaerosols (Fan et al., 2019). The viability of the microbes in the bioaerosols depends on the surrounding environment, climate, and atmospheric chemistry, in conjunction with microbial concentrations (Gong et al., 2020). Therefore, knowing the microbiological structure and composition of PM is necessary to identify the mode of disease transmission and the possible human health impact (Wang et al., 2019). South Asia and many other Asian megacities are currently undergoing rapid development in urban and industrial sectors and eventually deteriorating the air quality. Thus, this review aims to identify bioaerosols in relationship with PM reported from Asian megacities and their impact on human health.
Review Methodology
Electronic databases (PubMed central) and grey literature (Google Scholar) and bibliographies were searched for studies reporting bioaerosols and PM in Asian cities from 2010 to 2021. From the Pubmed and Google Scholar search, articles were exported to Endnote and indexed. Duplicates were removed. The exclusion has been rendered in two phases: title and summary, then complete text using Ryaan (https://rayyan.qcri.org/). Two reviewers checked both abstracts and titles. An independent review by two authors had reported more inconsistencies. Full-text copies were then retrieved for inclusion in the study and likewise reviewed.
Bioaerosol Research in the Environment of Asian Megacities
Bioaerosols play a significant role in the earth’s environment by interacting with the atmosphere, biosphere, micro-climate, and human health (Fröhlich-Nowoisky et al., 2016). While airborne microorganisms are omnipresent in the ambient near-surface, the spatiotemporal shifting aspects of their communities vary due to anthropogenic activities (Bowers et al., 2012). The exchanges may shape the variation of airborne microbes with the surrounding matrice of particles such as topsoils, soots, and dust that might inhibit or strengthen environmental growth and potency (e.g., resistance to alteration in seasonal and ecological parameters, temperature, humidity, and others.) (Groulx et al., 2018). The level of atmospheric pollutants impacts the entire structure of the bacterial community, including bacterial pathogens and ammonia-oxidizing microorganisms, than fungi (Fan et al., 2019). The prevailing harsh environment of minimal nutrition, dryness, and severe radiative heat in the ambiance and seasonal variations in the concentration of air pollutants shapes the community composition of microbes (Fan et al., 2019; Wei et al., 2019). PM and bioaerosols can both modify the oxidative capability of the harmful compounds and their toxicity present therein (Samake et al., 2017). The contribution of PM and airborne bioaerosols are, therefore, intrinsically interlinked. Table 1 indicates the list of research done in Asian cities' outdoor environments identified from 2010 to 2020. Approximately 54% of studies found were conducted in China, followed by India and Japan.
Detection of Airborne Bioaerosols
Detection and recognition of natural bioaerosols is an analytic task that requires many approaches (Caruana, 2011). The extreme-low biomass nature of the atmosphere represents an inherent analytical challenge confounded by temporal fluctuations in community structure (Luhung et al., 2021). Consequently, environmental sampling and quantitative analysis of bioaerosols are conducted using several fundamental and applied technical disciplines that vary from microbial plating, physical-chemical assays, molecular techniques, etc., each having individual targets, expectations, and terminology (Šantl et al., 2019; King et al., 2020). Bioaerosols can vary spatially and temporally depending on the air pollution level, PM, AQI, dust storms, haze or fogs, aerodynamics, social gatherings, and crowding and pollution source such as biomass burning. The development of standardized methods for detecting and quantitating bioaerosols has become an important issue (King et al., 2020).
Broadly defined, the sampling of bioaerosols and particulate matters is simultaneously done using particle counters, dust collectors, high-volume air sampler with filters or impactors, aerosol samplers, and total suspended particulate (TSP) sampler with filters. Table 2 displays various environmental surveillance of bioaerosols associated with particulate matters and other airborne pathogens. The benefit of passive sampling using electrostatic dust fall collectors (EDCs) increases the sampling period for a long time (Liebers et al., 2012). The cyclonic aerosol sampler was efficient for collecting bacterial samples, while the filter-type showed similar efficacy in collecting airborne fungal samples. Each has a different level of efficiency for sampling a sizable sum of microbes. Filter samplers operate under low temperatures, whereas cyclonic aerosol samplers have a temperature limit during the sampling period. Wang et al. (2019) showed that the cyclonic aerosol sampler was more efficient in collecting more genera than the high-volume air sampler with filters.
The cultured and non-cultured-based methods can estimate airborne bioaerosols by different samplers. Culturable airborne microbes can be identified by colony-forming units (CFU/m3) by plate counts or microscopes. However, over 99% of airborne microbes in bioaerosols collected by different methods are not culturable. For example, Wang et al. (2019) identified five airborne bioaerosol methods and particulate matter detection that identifies concentration and size. Among the five techniques, Andersen’s six-stage impactor device or sampler can collect culturable bacteria and fungi in the air where the aerodynamic diameter varies between >0.7 and 10 µm (Yadav et al., 2015; Yan et al., 2019). Andersen’s six-stage impactor device is a culture-based approach that enhances the survival rate of culturable microorganisms (Wang et al., 2019).
Biological composition analysis of bioaerosol samples can be performed by molecular methods of bacterial 16S ribosomal DNA and fungal internal transcribed spacer region sequence, respectively, instead of microorganism culture (Wang et al., 2019; Luhung et al., 2021). Furthermore, PCR, quantitative/real-time PCR, reveals microbial cell numbers instead of total bacterial count assessment (Wei et al., 2019). In addition, it is essential to identify a device or method for on-site real-time-air sampling of bioaerosols. Many potential respiratory diseases can be prevented and deactivated by such devices. An automated bioaerosol-monitoring system developed by Cho et al. (2020) can provide a continuous real-time monitoring system with significant sensitivity. It uses a combination of units such as a wet-cyclone bioaerosol sampler, a thermal-lysis unit for extracting adenosine triphosphate (ATP), and an ATP-detection unit based on the immobilization of luciferase/luciferin for bioluminescence reactions, and a photomultiplier tube-based detector. The bioaerosol concentration reported from different studies with the declared variables is given in Table 3. The types of bioaerosols such as bacterial, fungal, and virus bioaerosols are discussed in the following subsections.
Bacterial Bioaerosols
Bacterial bioaerosols significantly change atmospheric chemistry and impact human health (Gong et al., 2020). The highest bacterial abundances, rates, and relative bacterial contributions to total aerosol loads are typically observed during the autumn and spring. This variability in bacterial cells represents a significant fraction (22% on average) of overall variations in PM concentrations of the near-surface aerosol particles of >0.5 µm. The massive- or severe-pollution levels vastly altered bacterial structure (Fan et al., 2019). For example, Li et al. (2018) reported enrichment of pathogenic bacteria Halomonas and Shewanella on hazy days in Beijing, particularly in the autumn and early winters.
Airborne bacteria principally occurred in the particles larger than 2.1 μm along with the total bioaerosols concentration, identified in Qingdao coastal region, China (Li et al., 2011) (Table 4). From massive traffic junctions of Dehradun city, India, airborne Micrococcus, Staphylococcus, and Bacillus bacterial species were identified in the percentage of 25.73, 17.98, and 13.8%, respectively (Madhwal et al., 2020). Furthermore, methicillin-resistant staphylococci (MRS) S. aureus (MRSA), coagulase-negative staphylococci (CNS) were identified in the airborne bioaerosols from India associated with global environmental health (Kumar and Goel, 2016).
Fungal Bioaerosols and Spores
Quantitative assessments of fungi and their spores in bioaerosols are crucial to comprehend human respiratory diseases in densely populated developing countries. The climatological parameters significantly control fungal bioaerosols load in the atmosphere, including ambient temperature, humidity content, precipitation, wind directions, and speed (Li et al., 2011; Priyamvada et al., 2017). Diurnal variation, presence of haze days in winter significantly affects the mycological population in PM2.5 samples. DNA proportion of fungi in the PM2.5 (RD/P) altered air quality and demonstrated a substantial adverse correlation with PM2.5 and temperature. Moreover, the fungal species richness of night samples was more significant than the day samples [119–537 > 71–198 OTUs (operational taxonomic units)]. Subsequently, the night-sample accounted for enriched fungal taxa compared to the day samples (29 taxa >9 taxa). Likewise, haze periods also affected species richness significantly, with 198 OTUs before haze incidence compared to the 76 OTUs after haze incidence. Moreover, 8.6% of the entire fungal population was pathogenic (Zeng et al., 2019).
Fatahinia et al. (2018) identified 1,240 fungal colonies of 24 genera from the atmospheric bioaerosols of Ahvaz, Iran. The humid environment registered for many airborne fungi (32.3%) than the industrial atmosphere (17%). Priyamvada et al. (2017) identified three fungal species from the ambient PM10 from tropical city Chennai, India, which were the most dominant allergenic fungi. Likewise, from the massive traffic junctions, Madhwal et al. (2020) also identified those genera from India (Table 4).
Airborne fungal spores are another bioaerosol in which peak concentrations were found in spring, followed by summer, winter, and autumn, respectively (Table 4). Ascospores were the highly dominant class identified from Taipei, Taiwan. The concentrations of Aspergillus/Penicillium spores were prevalent in the residential or commercial areas, whereas basidiospores were dominant in regions with shorter road lengths (Table 4) (Kallawicha et al., 2015). It is crucial to carry out the ecological diagnosis of respirational symptoms caused by the breathing of fungi and fungal spores in any metropolitan area (Fatahinia et al., 2018). However, considering the public health issues, this type of study is entirely inadequate to perceive the actual human health threat from fungal bioaerosol in Asian cities.
Viruses in Bioaerosols
Airborne viral stability and infectivity may be associated with the interaction with pollutants such as PM and may have public health implications for communicable infectious diseases. Ambient PM (PM2.5) in the polluted air interact with viral aerosols and alter their infectivity (Groulx et al., 2018). It is, therefore, perceivable that the threat of viral toxicity has been heightened considerably by the presence of PM. In their study, Groulx et al. (2018) further showed that when PM2.5 is treated with high-efficiency particulate air (HEPA)-filter, the infectivity of a specific virus is reduced.
The respiratory syncytial virus (RSV) is an airborne viral infection that showed an infection rate concerning PM. Dose-response, lag-time, cumulative effects of RSV infection positively correlated to the PM concentrations from January-May and October-December throughout the study period (Ye et al., 2016). RSV infection among infants and kids was positively correlated to atmospheric temperature and AQI (Ye et al., 2016). Very recently, it is proved that infective microdroplets (>5–10 µm) and aerosols (≤5 µm) of coronavirus in air-suspension can expose individuals as far as 2 m from the COVID-19 infected person (Guzman 2020; Morawska and Milton 2020; Prather et al., 2020a; Prather et al., 2020b). The airborne SARS-CoV-2 virus can stay longer in the atmosphere in bioaerosols and on inanimate objects increasing transmission to other people’s hands and noses. The study already reported that the COVID-19 could survive on any inert surface for several hours to days (Kampf et al., 2020). There is a suspicion that pollen bioaerosols can also affect coronavirus survival (Khaiwal et al., 2021). Data analysis on SARS-CoV-2 infection, airborne pollen, and meteorological factors such as humidity and temperature from 130 sites and 31 countries showed 44% infection rate variability (Damialis et al., 2021). Considering the recent incidence of coronavirus (COVID-19) pandemics, it shows that under suitable environmental conditions, simultaneous or co-exposure to SARS-CoV-2 (via other infected human carriers) and airborne pollen might promote viral infection. Therefore, we must detect their seasonal patterns of pollens and airborne viruses, including COVID-19, based on environmental factors. However, the drastic mutating nature of the virus makes it difficult to predict which mechanisms and ecological parameters affect its growth and prevalence.
Effects of Environmental Parameters on the Distribution of Bioaerosols
Different environmental variables like humidity, relative humidity, temperature, wind speed, wind direction, presence of sunlight, specifically UV light at day time and absence at night time, seasonal variations, presence of pollutants PM2.5-10, dust particles, along with the gases related to air quality, haze and fog, precipitation affect the concentration, structure, and taxonomy of bioaerosols. There is a strong seasonal correlation that increases bioaerosol loads in fall, early winter, spring, and PM (r = 0.75, p < 0.001) (Bowers et al., 2012). The ambient bioaerosols community structure, in turn, is being affected by the seasons and terrestrial environments (Bowers et al., 2012). It further affects the community structure of the indoor bioaerosols (Zhou et al., 2021). The microbial ecology of the atmospheric ecosystems is evident for this correlation, and the exploration requires in-depth analysis. In the following subsections, we have discussed these variables.
Bioaerosols in Association With PM
Many Asian megacities are seriously affected by particulate matter pollution such as PM2.5, PM10, and other air pollutants. COVID-19 lockdown resulted in a significant reduction in PM2.5, PM10, NO2, and CO in all the Indian regions (Singh et al., 2020). In Delhi, the concentrations of PM10 and PM2.5 have observed a >50% decrease compared to the pre-lockdown period (Mahato et al., 2020). For PM2.5, 40–70% reduction was observed during the first week of lockdown. However, O3 pollution remained high during the lockdown due to non-linear chemistry and dynamics under low aerosol loading (Dhaka et al., 2020). In China, similar results were also observed. The AQI in the locked-down cities was reduced to 19.84 points. PM2.5 was reduced by 14.07 μg m−3. In cities without lockdown, AQI was brought down by 6.34 points (PM2.5 down by 7.05 μg m−3) compared to 2019 (He et al., 2020).
The increasing number of bacterial colonies correlated to the PM concentrations has been identified (Amarloei et al., 2020). PM2.5 and PM10 are significant AQI that hosts a load of secondary pollutants, including airborne microorganisms. PM’s physical and chemical composition positively correlates with bacteria, especially pathogenic bacteria (Liu et al., 2018).
Ammonia (NH3) emission develops secondary inorganic aerosol with a dynamic relationship with the concentration of urban PM2.5. The contribution of ammonia in PM2.5 is often ignored and undervalued compared to SO2 and NOx (Wu et al., 2016). Fan et al. (2019) identified that ammonia-oxidizing microbes significantly cooccurred with the prevalent bacterial and fungal groups and potential pathogens. It further proves the role of ammonia in the development of PM2.5. Therefore, managing ammonia emission means minimizing PM2.5 pollution rather than other PM2.5 precursors, SO2, and NOx, for instance (Fan et al., 2019).
However, the stimuli of PM10 and temperature on bioaerosols contrasted with the hazing intensity (Gao et al., 2016). In winter, on heavy pollution days, hub bacteria in PM2.5 were abundant. In contrast, on less-pollution days in winter, hub fungi were dominant (Fan et al., 2019). However, Li et al. (2018) informed that in autumn and early winter haze days in Beijing, PM10 and relative humidity were the main reasons to alter the airborne bacterial load and community structure substantially. During the summer heatwaves, the size distribution of bioaerosol became single-mode while on average sunny days, the particle sizes of the total microbes showed two sets of distribution with one skewing at 1.1–2.1 μm and another at 4.7–7.0 μm (Dong et al., 2016) with average airborne fungi distributed towards 2.1–3.3 µm while bacteria skewed towards greater size (Gao et al., 2016). The tendency led to the development of a bigger size particle. Nevertheless, Lu et al. (2018) showed that the bacterial population structure in PM2.5, PM10, and total suspended particulate (TSP) did not very much.
The overall characteristics depending on the load and size distribution of culturable airborne microbes are related to several environmental factors. The factors are AQI (PM2.5-10), sampling times and seasons, diurnal temperature, relative humidity, dewpoints, wind pressure and speed, ground-level gases such as ozone, NOx, and SOx (Gao et al., 2016). Airborne microbe concentration had a substantial negative correlation with temperature and ozone concentration (Yan et al., 2019), while positive relationships with the AQI and ground-level SOx, NOx, CO concentration during the hazy days (Dong et al., 2016). Similar observations were found in the study of Yan et al. (2019). Gao et al. (2016) informed that the ratio of SOx/NOx showed a diurnal and chronological change in bioaerosol concentration (Gao et al., 2016). However, Lu et al. (2018) confirmed that atmospheric temperature, ozone, and NOx substantially affected bacterial communities compared to other atmospheric parameters (Liu et al., 2018; Lu et al., 2018). Consequently, the increased number of airborne microbes may affect the air quality on hazy days by different size distributions of bioaerosol (Liu et al., 2018; Fan et al., 2019), which might be abundant in pathogenic bacteria (Liu et al., 2018) mentioned in Table 4.
A Seasonal and Temporal Variation of Bioaerosols
The amount of bioaerosols present in winter and summer is comparable. For example, a study from the Seoul Metropolitan area confirmed significant fungal bioaerosols in winter compared to the very few bacterial bioaerosols (Uk Lee et al., 2016). Yan et al. (2019) further confirmed noteworthy differences in the concentration of culturable airborne bacteria and fungi in the winter season from Xinxiang, China (bacteria 4,595 ± 3410 CFU/m3 > fungi 6,358 ± 5032 CFU/m3). Again, precipitation types (snowfall and rainfall) may have different effects on bioaerosols. Snowfall in winter seizes bioaerosols, while rains in summer aerosolize microbes from the ground into the air through splashes and humidifying the immediate environments (Uk Lee et al., 2016).
Temperature affects the bacterial concentration while rainfall (1–4 mm) increases the fungal concentration in the environment (Rajput et al., 2017) (Figure 1). For tropical regions such as South Asia, the seasonal pattern of bioaerosol in the atmosphere is similar. During the monsoon and post-monsoon months, bacterial and fungal loads were the highest (Madhwal et al., 2020). Raindrop splashes caused microbial aerosolization on ground air and automobile movement, human microbial fluid from phlegm and coughs, lifted relative humidity in the air, and soil resuspension. Significant seasonality among the fungal bioaerosols in the tropical city of Chennai, India, was also confirmed (Priyamvada et al., 2017).
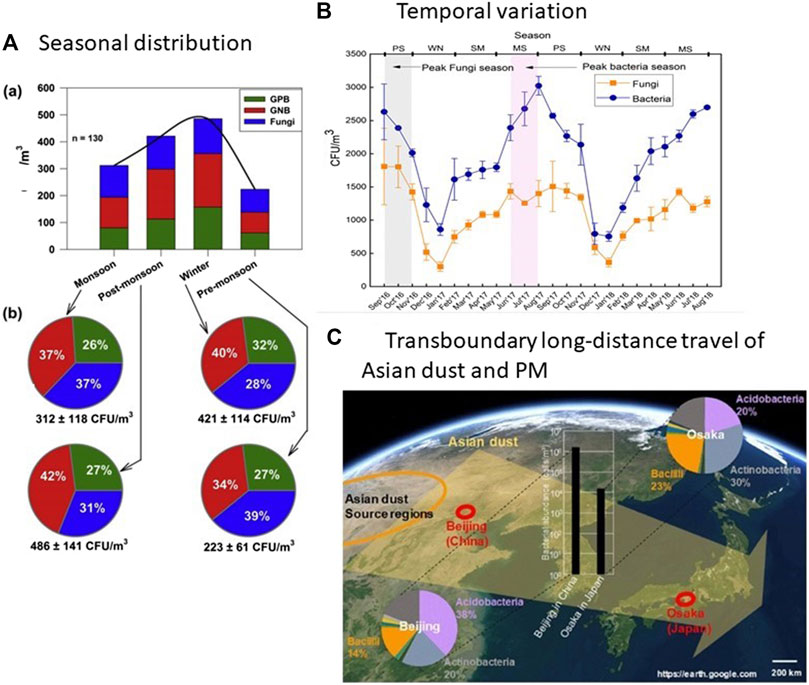
FIGURE 1. (A) Seasonal variability of total viable bioaerosols [Gram-positive bacteria (GPB)+ Gram-negative bacteria (GNB)+ Fungi] and percentage fractions of GPB, GNB, and Fungi colonies collected from biomass burning of Kanpur, India (Rajput et al., 2017). (B) Temporal variation in fungal and bacterial concentrations at a high traffic density junction in Dehradun city, India (PS: post-monsoon, WN: winter, SM: summer, MS: monsoon) (Madhwal et al., 2020). This seasonal pattern is suspected to be similar for all tropical South and South-East Asian cities. (C) Asian dust incidents and the impact on airborne bacterial populations at different distances from the source area to the downwind region (Park et al., 2018).
The maximum and minimum bioaerosol loads were documented at night time and mid-day, respectively, from the study of Yan et al. (2019). Temporal differences in bioaerosol explain the potential harms during hazy and non-hazy days as the UV radiation intensity varies (Gao et al., 2015). An example includes the rush-hour effect of PM10 on bioaerosol varies at different times of the day. For instance, in the morning rush hour, 9:00 am, people go to work, while at midday, people could go for lunch outside. At approximately 6:00 pm, another rush hour occurs when people return home (Gao et al., 2016). Moreover, during rush hours, transport crossings are among the most populated locations where travelers are likely to acquire airborne and transmitted microbial pathogens due to increased exposure levels. Traffic/human-induced roadside dust resuspension and microbial aerosolization from commuters were the primary sources of bioaerosol emissions (Madhwal et al., 2020).
Effect of Haze Days Versus Non-haze Days on Bioaerosols
The presence of haze days varies bioaerosol loads in the atmosphere. The severity of haze days significantly impacts atmospheric bioaerosols composition and community structure (Li et al., 2018). The principal component analysis suggested that haze-level, sampling period, and seasons influence the bioaerosol sizes. Among hazy and non-hazy days, the size distribution of bioaerosols (Gao et al., 2016) and relative abundances of pathogenic bacteria rarely varied (Lu et al., 2018). Moreover, airborne bacteria inclined towards the larger particles, while airborne fungi showed a Gaussian distribution (Gao et al., 2015). Besides, total microbes increased in the finer portion on haze days, while on foggy days, it inclined towards coarse portions. As discussed in earlier sections that the microbial load was the sharpest in the winter and at a low level in the summer (Dong et al., 2016). However, Li et al. found a clear recurring association with the haze events and airborne bacterial load from Beijing in autumn and early winter (Li et al., 2018). Nevertheless, winter haze events can be a potential source of fungal bioaerosol infection risk, and people should be careful about these days (Zeng et al., 2019).
Bioaerosols in Association With Dust Storms and Asian Dust Events
Dust storms are a major global environmental problem, especially in Asian cities, due to high Asian monsoon variability. The deserts of the Middle East and Central and North East Asia are the primary sources of dust storm events in Asia. Dust from these areas can be distributed worldwide all year round with different concentrations and occurrences (Soleimani et al., 2020). Amarloei et al. (2020), from Ilam city, Iran, identified that increased dust storm events are correlated to the PM2.5 and PM10 concentrations in the warm and dry months, subsequently increasing the bioaerosols. The chiefly detected bacterial colonies by Amarloei et al. (2020) isolated from AD events are given in Table 4. Asian dust events, also known as Kosa (Kobayashi et al., 2015), contain Aeolian dust particles. Aeolian dust is a crucial component impacting the atmosphere in dry and semiarid environments, which intimately affects the global biogeochemical and carbon cycle and energy exchange (Zhang et al., 2020). Aeolian dust particles are a critical media for the long-range transport of microorganisms (Maki et al., 2019). Usually, the bioaerosols are transported freely or attached to dust particles suspended in the atmosphere (Aalismail et al., 2020). Strong seasonal winds cause Aeolian dust from arid and semiarid Mongolia and northern China (Yoo et al., 2019). East Asian countries China, Japan, and Korea are disturbed mainly by Aeolian dust and the bioaerosols by air currents through the westerly winds (Kobayashi et al., 2015; Park et al., 2018). During the spring, the transit of air masses over continental and marine surfaces brings Kosa or Aeolian bioaerosols (Maki et al., 2019) that affect environments, public health, and agricultural production in the windward regions (Kobayashi et al., 2015).
Maki et al. (2019) reported that airborne bacterial diversity increased from the Chinese desert to the Japanese shore with dust scatterings and bacterial taxa, including desert microbial communities and pathogens related to humans and plants. During the travel of Aeolian air mass, it is also influenced by the local landforms, and microbial dispersal occurs even at high altitudes. The study further showed that on non-dust event days, the bioaerosols are usually dominated from the nearby shores.
Acute AD days raise bacterial richness 1000-fold times compared to the non-AD days. Prevalent bacterial phyla in the same AD dust events differed between regions. Aerosol bacterial communities closer to the dust origin region (Beijing, China) are altered by Aeolian dust highly than their outlying analogous areas (Osaka, Japan) is showed in Figure 1C (Park et al., 2018). As the 16S ribosomal RNA gene quantity increased, bacterial diversity expanded considerably into AD samples, and the PM10 concentration was associated positively (Yoo et al., 2019). AD samples are usually abundant with the Bacillus species (almost 23.8%) than the non-AD models (almost 13.3%).
Effects of Aerodynamics and the Human Crowd on Bioaerosol
Aerodynamic size variation is a significant parameter in evaluating bioaerosol exposure during hazy and non-hazy days. The temporal variation of aerodynamic diameter (dg) of microbial aerosol with PM2.5 (AQI) varied from morning 9:00 to evening 21:00, suggesting their depositional pattern would fluctuate during a day (Gao et al., 2015). Moreover, climatological aspects, PM with dg of ≤10 μm, and altitude had sizable interactions per loads of fungal spore in the atmosphere (Kallawicha et al., 2015). Origin-tracking analysis by Lu et al. (2018) indicated that the airborne microbes could be transported to an entire city or region by airflow and aerodynamic characteristics.
The circulation of microbial aerosols associated with mass people such as fair, political meetings; religious gatherings is a less-researched area. A substantial number of airborne microbial aerosols were found throughout a trade fair period in central India contrasted to the no-fair period and no-fair sites. Furthermore, the antibiotic-resistant bacterial proportion was higher throughout the trade fair period and continued even after-fair period (Yadav et al., 2015). Considering the recent COVID-19 pandemic, the application of social distancing, home-isolation, and uses of face masks can decrease human transmission of the virus bioaerosols (Li et al., 2020).
Biomass Burning Effect on Bioaerosols
Biomass burning sources are firing grasslands, pine needles, forest woods, and hays during the harvest seasons. The crop harvest seasons are marked by open biomass burning and subsequently elevated PM2.5 and other air pollutants. The broader range of particle-spectrum indicated growth of similar community structure for bacteria and fungi in PM1.0 and PM2.5 (Table 4) with a slight profusion in PM2.5 (Wei et al., 2019). Biomass burning often originates locally or from cross-regional and transboundary airborne transportation by air masses. Urban haze days in Shanghai were traced from four regional transport sources: biomass burning (28%), pollen (25%), spores (24%), and emissions from fuel combustion (23%) (Li et al., 2016). In winter, biomass burning tracers peaked, while fungal spore tracers peaked in summer (Xu et al., 2019).
From Indo-Gangetic Plain Kanpur, India, the concentration of PM number associated with the finer portion of aerosol throughout the year while the high abundance of Gram-negative bacteria (GNB) dominated during large-scale biomass burning (Rajput et al., 2017). Peatland fire from Indonesian tropical peatlands is another haze and PM2.5 pollution (Uda et al., 2019) in Southeast Asian countries. Every dry season is marked by peatland fires in Sumatra and Kalimantan Islands, leading to the transport of heavy smoke and haze that impacts public wellbeing, visual clarity, and the local climate of Indonesia, Malaysia, and Singapore (Fujii et al., 2017; Uda et al., 2019). Although loads of bioaerosols from peatland fire were not found during this review, we think this research requires in-depth exploration. Again, in South Asian countries, roadside wastes and biomass burning are common practices of waste management. The generation of PM and the associated bioaerosols should be vividly explored in aerodynamics, seasonal, and spatial distribution.
Public Health Effects and Deactivation/Inactivation of Bioaerosols
The replication and dispersion of airborne bacteria, fungi, spores, viruses, and other bioparticles in different environments can transmit diseases to animals, plants, and humans (Fröhlich-Nowoisky et al., 2016). The effective cooccurrence and co-elimination mode of pathogenic microbes in PM2.5 aggravates air pollution on public health and the ecosystem despite low concentrations (Fan et al., 2019; Wang et al., 2019; Amarloei et al., 2020). Aerodynamics of PM and size characteristics precede it to transfer over spaces from the source of their origin, which is an essential pathway of spreading diseases in different locations (Wang et al., 2019). Besides, smoke particles from biomass burning and microbial aerosols are likely to form ice nuclei and condense cloud nuclei. Subsequently, it can influence biochemical cycles and public health (Wei et al., 2019). An example includes Gram-negative bacterial endotoxins exposure to public health during large-scale biomass burning (Rajput et al., 2017). A substantial seasonal rise of asthma exasperation and emergency medical department visit was found in Kyoto, Japan, in association with the ambient coarse particles, proteins, and endotoxin (Khan et al., 2018). It was observed that in the springtime, protein concentration in fine ambient particles increased while endotoxin concentrations in both fine and coarse particles rise in the autumn, and hence the risk of asthma increases.
The risk of human exposure to airborne pathogenic bacterial bioaerosol has primarily been related to air inhalation. (Lu et al., 2018; Yan et al., 2019). Many deadly diseases, such as pneumonia, tuberculosis, brucellosis, anthrax, and Q-fever, are connected to bacterial bioaerosols (Uk Lee et al., 2016). Moreover, frequent causes of asthma, respiratory allergies, hypersensitive rhinitis and chronic obstructive pulmonary disease (COPD), and others are associated with the airborne fungal pathogenic exposure owing to their omnipresence (Kallawicha et al., 2015; Uk Lee et al., 2016; Priyamvada et al., 2017). A. fumigatus is implicated in numerous wellbeing difficulties in people compromised by immune deficiencies or bronchopulmonary aspergillosis. S. chartarum, on the other hand, colonize in damps and produce mycotoxins. Fungal bioaerosols considerably influence the oxidative ability and chemical composition of the ambient PM in the presence of A. fumigatus spores (Samake et al., 2017). Also, bioaerosols accelerate reactive oxygen species (ROS) in human lungs in response to different microorganisms and concentrations. For example, fungal spores generated up to10 times more ROS than bacterial cells (Samake et al., 2017).
The microbial organisms associated with the desert dust and AD events may cause several diseases such as respirational, cardiopulmonary, and heart diseases (Soleimani et al., 2020). Moreover, the systematic characterization of airborne bacterial communities in Asian dust (AD) samples identified the presence of B. cereus that poses risks to public health (Yoo et al., 2019).
Children are susceptible to airborne pathogens and allergens (Shin et al., 2015). The annual RSV-prevalence among children was 10%, of which infants under 6.5 months represented 80% of all patients (Ye et al., 2016). Biodefense approaches, which include assessing, detecting, monitoring, responding to biological threats, can be established to avert/lessen the apparent exposure of bioaerosols. Displaying hourly or daily AQI level in color-code in public screens, endorsing alternative travel routes, face masks, prevention of spitting and littering in public places, and above all, maintaining environmental and personal hygiene through strict laws are required for the commuters’ safety plan (Madhwal et al., 2020). COVID-19 lockdown shows that Indian cities with poor air quality correlated with higher COVID-19 cases and deaths. Conversely, low mortality was reported in cities with better air quality (Naqvi et al., 2021). The SARS-CoV-2 viral disease COVID-19 infectivity rate is higher in many developing and developed countries as the unaware population is not using masks properly and not maintaining social distancing. Wearing face masks in public is associated with a decline in the daily COVID-19 growth rate in many US states (Lyu, and Wehby 2020), requiring general public guidance (Leung et al., 2020).
Subsequently, mechanisms to deactivate or inactivate bioaerosols containing airborne bacteria, fungi, fungal spores, and virus bioaerosols are pressing research issues to protect the public at the hospitals, offices, homes, and institutional environments. Photocatalysis using titanium dioxide (TiO2) is a significant research area of bioaerosol deactivation or inactivation. Biological waste management processes are often the primary sources of bioaerosols in the cities (Valdez-Castillo et al., 2019; Valdez-Castillo et al., 2021). Valdez-Castillo and his colleagues (2019), in their earlier study, showed that photocatalytic systems based on Perlite-supported ZnO and TiO2 significantly inactivated fungal/bacterial emissions up to 70%. In their later study (Valdez-Castillo et al., 2021), involving photocatalysis with ZnO and TiO2 immobilized onto Poraver glass beads proved to inactivate 78% bioaerosols during the first two-hour. Both of these processes by bacteria and fungi were inactivated by cell death. In another study (Valdez-Castillo and Arriaga 2021), a photocatalytic system could entirely inactivate bioaerosols in indoor environments.
The application of nanotechnology can significantly contribute to the efficiency improvement of bioaerosol inactivation. Recent research on visible-light-activated (VLA) antimicrobial air filters equipped with TiO2–crystal violet nanocomposites shows <99.9% bioaerosol inactivation (Heo et al., 2021). Application of Iron Oxide nanowire-based filter significantly inactivated airborne bacteria. The inactivation of bacteria is related to the combined effect of the OH radicals, electroporation, and Joule heating that disrupts the bacterial cell walls. A single filter can capture 52% of the bacterial cells and inactivate them. The filter also has a long life (Wang et al., 2018). The application of plasma technology is also a novel approach to inactivate many bioaerosols, including SARS-COV-2 RNA, in hospitals and other indoor environments (Bisag et al., 2020).
Future Research Perspectives
It is evident that PM from urban development works, industrial activities, heavy traffic pollution, roadside wastes, and biomass burning, massive human crowds can increase the bioaerosols in the atmosphere (Figure 2). It is, therefore, essential to carry out regular bioaerosol assessments in the cities of developing countries, particularly in Asian cities, when the pollution levels are high. The literature review found that the higher prevalence of bioaerosols occurs in fall, early winter, late winter, and spring. Seasonally these are the tipping times for bioaerosols concentration in the outdoor environments. However, the seasonal effect of post-monsoon and pre-monsoon on bioaerosol load in tropical countries are still less-explored issues that need research.
• In many developing countries, detection and laboratory facilities are non-existent, leading to unreported cases of bioaerosols. For example, in Bangladesh, the exact bioaerosols concentration in the atmosphere is unknown. However, the hospital admission data on respiratory affected people show a drastically increasing trend in 2019–2020, according to the Ministry of Health and Family Welfare which was reported in the local Bengali daily Kalerkantha (Maruf, 2020) compared to the previous years (Figure 3). In February 2020, alone, 5,21,384 persons were affected in the November-March season of 2019–2020, which is considered winter months and post-winter months and possibly by airborne transmission. However, lack of research and enough evidence-based research is a pressing issue to infer exposure to airborne pathogenic microbes related to microbial infections such as asthma, allergy, or other respiratory diseases in the urban, industrial and institutional environment in relation to PM.
• Integrated research should be focused on rapid airborne bioaerosol detection, monitoring, early warning, and inactivation or deactivation of bioaerosol is required in association with PM.
• Since the research of airborne bioaerosol is integrated research, a network of environmental scientists, microbiologists, medical microbiologists, biochemists, computer engineers, and researchers from information technology can develop integrated ecological sensors for bioaerosols detection technologies. Kit-based rapid detection research and methods should be focused on detecting the nature of the bioaerosols in the urban atmosphere that may pose a serious public health threat. Fluorescence techniques can be used for the rapid detection of bioaerosols. In addition, research on low-cost real-time quantitative PCR (RT-PCR and qPCR) should be developed to identify which phylum is in the air.
• Artificial intelligence, deep neural networks, and machine learning can develop and test real-time bioaerosols sensors. Mobile apps can be created for city dwellers to inform them about the concentration of bioaerosols in the air, particularly of the pathogenic and allergens type. Similarly, like AQI a global real-time bioaerosol sensors and detection technologies can be developed to alarm the global real-time scenario of bioaerosols. Further exploration should focus on the uses and consequences of the internet of things (IoT) technologies for calculating the concentrations of bioaerosols and maps in real-time.
• For public warning, precaution and communication, and policymaking on bioaerosols, effective health policy research is needed for public health safety.
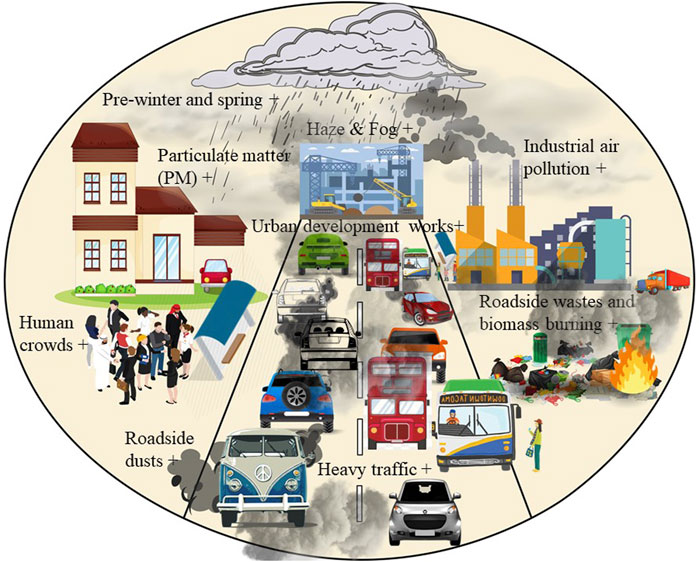
FIGURE 2. Factors influencing bioaerosols load (indicated by +) in association with the development of particulate matter (>2.5–10 µm) in the outdoor urban/industrial environments of developing countries. This is suspected to be similar for all tropical South Asian and Southeast Asian cities.
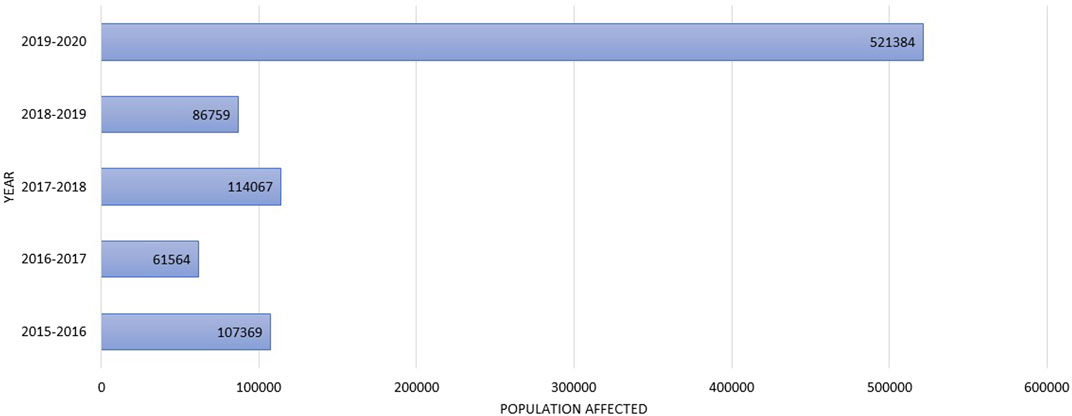
FIGURE 3. The affected population by seasonal flu, coughing, and fever reported from 2015 to 2020 November-March data according to the Ministry of Health and family welfare, Bangladesh.
Concluding Remarks
This review is aimed to identify the current bioaerosol scenario in the Asian regions. The environmental quality of Asian megacities is composed of rapid industrialization, faster urbanization, and development, followed by very high PM pollution. In turn, PM, AQI, and the presence of associated gases significantly contribute to the abundances of airborne bioaerosols concentration during the hazy and foggy days, and the aerodynamics controls their floating behavior. Moreover, dense crowds, heavy traffic density, uncontrolled biomass burning also regulates the prevalence of bioaerosols in different concentrations in the outdoor atmosphere and the occurrences of human transmission. Subsequently, pathogenic bioaerosols in outdoor environments are responsible for various allergens and respiratory diseases among children and adults, and the response is not identical. For public health safety, it is essential to detect and control the pathogenic bioaerosol loads, along with the spatiotemporal distribution in Asian megacities. Particular emphasis should be given to developing on-site automated bioaerosol-monitoring systems and bioaerosol deactivation or inactivation mechanisms. Although, in this review, we have emphasized bioaerosol formation and detection in the outdoor environment, to protect indoor spaces such as homes, offices, schools, and hospitals, should also be brought under strict and continuous monitoring of bioaerosols. Research should be more focused on devising bioaerosol’s deactivation/inactivation mechanism.
Author Contributions
MS planned, reviewed, and prepared the manuscript. MMR and SMT reviewed independently. All authors commented and contributed to the final manuscript preparation.
Conflict of Interest
The authors declare that the research was conducted in the absence of any commercial or financial relationships that could be construed as a potential conflict of interest.
Publisher’s Note
All claims expressed in this article are solely those of the authors and do not necessarily represent those of their affiliated organizations, or those of the publisher, the editors, and the reviewers. Any product that may be evaluated in this article, or claim that may be made by its manufacturer, is not guaranteed or endorsed by the publisher.
Acknowledgments
The authors would like to acknowledge all the authors cited in the references.
References
Aalismail, N. A., Díaz-Rúa, R., Ngugi, D. K., Cusack, M., and Duarte, C. M. (2020). Aeolian Prokaryotic Communities of the Global Dust Belt over the Red Sea. Front. Microbiol. 11, 538476. doi:10.3389/fmicb.2020.538476
Amarloei, A., Fazlzadeh, M., Jafari, A. J., Zarei, A., and Mazloomi, S. (2020). Particulate Matters and Bioaerosols during Middle East Dust Storms Events in Ilam, Iran. Microchem. J. 152, 104280. doi:10.1016/j.microc.2019.104280
Bisag, A., Isabelli, P., Laurita, R., Bucci, C., Capelli, F., Dirani, G., et al. (2020). Cold Atmospheric Plasma Inactivation of Aerosolized Microdroplets Containing Bacteria and Purified SARS‐CoV‐2 RNA to Contrast Airborne Indoor Transmission. Plasma Process. Polym. 17 (10), 2000154. doi:10.1002/ppap.202000154
Bowers, R. M., Mccubbin, I. B., Hallar, A. G., and Fierer, N. (2012). Seasonal Variability in Airborne Bacterial Communities at a High-Elevation Site. Atmos. Environ. 50, 41–49. doi:10.1016/j.atmosenv.2012.01.005
Caruana, D. J. (2011). Detection and Analysis of Airborne Particles of Biological Origin: Present and Future. Analyst 136 (22), 4641–4652. doi:10.1039/c1an15506g
Cho, Y. S., Kim, H. R., Ko, H. S., Jeong, S. B., Chan Kim, B., and Jung, J. H. (2020). Continuous Surveillance of Bioaerosols On-Site Using an Automated Bioaerosol-Monitoring System. ACS Sens. 5 (2), 395–403. doi:10.1021/acssensors.9b02001
Damialis, A., Gilles, S., Sofiev, M., Sofieva, V., Kolek, F., Bayr, D., et al. (2021). Higher Airborne Pollen Concentrations Correlated with Increased SARS-CoV-2 Infection Rates, as Evidenced from 31 Countries across the globe. Proc. Natl. Acad. Sci. 118 (12), e2019034118. doi:10.1073/pnas.2019034118
Dhaka, S. K., Kumar, V., Panwar, V., Dimri, A. P., Singh, N., Patra, P. K., et al. (2020). PM 2.5 Diminution and Haze Events over Delhi during the COVID-19 Lockdown Period: an Interplay between the Baseline Pollution and Meteorology. Sci. Rep. 10 (1), 1–8. doi:10.1038/s41598-020-70179-8
Dong, L., Qi, J., Shao, C., Zhong, X., Gao, D., Cao, W., et al. (2016). Concentration and Size Distribution of Total Airborne Microbes in Hazy and Foggy Weather. Sci. Total Environ. 541, 1011–1018. doi:10.1016/j.scitotenv.2015.10.001
Fan, X.-Y., Gao, J.-F., Pan, K.-L., Li, D.-C., Dai, H.-H., and Li, X. (2019). More Obvious Air Pollution Impacts on Variations in Bacteria Than Fungi and Their Co-occurrences with Ammonia-Oxidizing Microorganisms in PM2.5. Environ. Pollut. 251, 668–680. doi:10.1016/j.envpol.2019.05.004
Fatahinia, M., Zarei-Mahmoudabadi, A., Shokri, H., and Ghaymi, H. (2018). Monitoring of Mycoflora in Outdoor Air of Different Localities of Ahvaz, Iran. J. de Mycologie Médicale 28 (1), 87–93. doi:10.1016/j.mycmed.2017.12.002
Fröhlich-Nowoisky, J., Kampf, C. J., Weber, B., Huffman, J. A., Pöhlker, C., Andreae, M. O., et al. (2016). Bioaerosols in the Earth System: Climate, Health, and Ecosystem Interactions. Atmos. Res. 182, 346–376. doi:10.1016/j.atmosres.2016.07.018
Fujii, Y., Tohno, S., Amil, N., and Latif, M. T. (2017). Quantitative Assessment of Source Contributions to PM2.5 on the West Coast of Peninsular Malaysia to Determine the burden of Indonesian Peatland Fire. Atmos. Environ. 171, 111–117. doi:10.1016/j.atmosenv.2017.10.009
Gandolfi, I., Bertolini, V., Ambrosini, R., Bestetti, G., and Franzetti, A. (2013). Unravelling the Bacterial Diversity in the Atmosphere. Appl. Microbiol. Biotechnol. 97 (11), 4727–4736. doi:10.1007/s00253-013-4901-2
Gao, M., Qiu, T., Jia, R., Han, M., Song, Y., and Wang, X. (2015). Concentration and Size Distribution of Viable Bioaerosols during Non-haze and Haze Days in Beijing. Environ. Sci. Pollut. Res. 22 (6), 4359–4368. doi:10.1007/s11356-014-3675-0
Gao, M., Yan, X., Qiu, T., Han, M., and Wang, X. (2016). Variation of Correlations between Factors and Culturable Airborne Bacteria and Fungi. Atmos. Environ. 128, 10–19. doi:10.1016/j.atmosenv.2015.12.008
Ghosh, B., Lal, H., and Srivastava, A. (2015). Review of Bioaerosols in Indoor Environment with Special Reference to Sampling, Analysis and Control Mechanisms. Environ. Int. 85, 254–272. doi:10.1016/j.envint.2015.09.018
Gong, J., Qi, J., E, B., Yin, Y., and Gao, D. (2020). Concentration, Viability and Size Distribution of Bacteria in Atmospheric Bioaerosols under Different Types of Pollution. Environ. Pollut. 257, 113485. doi:10.1016/j.envpol.2019.113485
Groulx, N., Urch, B., Duchaine, C., Mubareka, S., and Scott, J. A. (2018). The Pollution Particulate Concentrator (PoPCon): A Platform to Investigate the Effects of Particulate Air Pollutants on Viral Infectivity. Sci. Total Environ. 628-629, 1101–1107. doi:10.1016/j.scitotenv.2018.02.118
Guzman, M. I. (2020). An Overview of the Effect of Bioaerosol Size in Coronavirus Disease 2019 Transmission. Int. J. Health Plan. Manag. 36, 257. doi:10.1002/hpm.3095
He, G., Pan, Y., and Tanaka, T. (2020). The Short-Term Impacts of COVID-19 Lockdown on Urban Air Pollution in China. Nat. Sustain. 3 (12), 1005–1011. doi:10.1038/s41893-020-0581-y
Heo, K. J., Jeong, S. B., Shin, J., Hwang, G. B., Ko, H. S., Kim, Y., et al. (2021). Water-Repellent TiO2-Organic Dye-Based Air Filters for Efficient Visible-Light-Activated Photochemical Inactivation against Bioaerosols. Nano Lett. 21 (4), 1576–1583. doi:10.1021/acs.nanolett.0c03173
Humbal, C., Gautam, S., and Trivedi, U. (2018). A Review on Recent Progress in Observations, and Health Effects of Bioaerosols. Environ. Int. 118, 189–193. doi:10.1016/j.envint.2018.05.053
IQAir (2020). Air Quality index (AQI) and PM2.5 Air Pollution. Available at: https://www.iqair.com/bangladesh (Accessed March 1, 2020).
Kallawicha, K., Tsai, Y.-J., Chuang, Y.-C., Lung, S.-C. C., Wu, C.-D., Chen, T.-H., et al. (2015). The Spatiotemporal Distributions and Determinants of Ambient Fungal Spores in the Greater Taipei Area. Environ. Pollut. 204, 173–180. doi:10.1016/j.envpol.2015.04.020
Kampf, G., Todt, D., Pfaender, S., and Steinmann, E. (2020). Persistence of Coronaviruses on Inanimate Surfaces and Their Inactivation with Biocidal Agents. J. Hosp. Infect. 104, 246–251. doi:10.1016/j.jhin.2020.01.022
Khaiwal, R., Goyal, A., and Mor, S. (2021). Does Airborne Pollen Influence COVID-19 Outbreak?. Sustain. Cities Soc. 70, 102887. doi:10.1016/j.scs.2021.102887
Khan, M. S., Coulibaly, S., Matsumoto, T., Yano, Y., Miura, M., Nagasaka, Y., et al. (2018). Association of Airborne Particles, Protein, and Endotoxin with Emergency Department Visits for Asthma in Kyoto, Japan. Environ. Health Prev. Med. 23 (1), 41. doi:10.1186/s12199-018-0731-2
King, M. D., Lacey, R. E., Pak, H., Fearing, A., Ramos, G., Baig, T., et al. (2020). Assays and Enumeration of Bioaerosols-Traditional Approaches to Modern Practices. Aerosol Sci. Tech. 54 (5), 611–633. doi:10.1080/02786826.2020.1723789
Kobayashi, F., Maki, T., Kakikawa, M., Yamada, M., Puspitasari, F., and Iwasaka, Y. (2015). Bioprocess of Kosa Bioaerosols: Effect of Ultraviolet Radiation on Airborne Bacteria within Kosa (Asian Dust). J. Biosci. Bioeng. 119 (5), 570–579. doi:10.1016/j.jbiosc.2014.10.015
Kumar, P., and Goel, A. K. (2016). Prevalence of Methicillin Resistant Staphylococcal Bioaerosols in and Around Residential Houses in an Urban Area in Central India. J. Pathog. 2016, 7163615. doi:10.1155/2016/7163615
Leung, C. C., Lam, T. H., and Cheng, K. K. (2020). Mass Masking in the COVID-19 Epidemic: People Need Guidance. Lancet 395 (10228), 945. doi:10.1016/s0140-6736(20)30520-1
Li, M., Qi, J., Zhang, H., Huang, S., Li, L., and Gao, D. (2011). Concentration and Size Distribution of Bioaerosols in an Outdoor Environment in the Qingdao Coastal Region. Sci. Total Environ. 409 (19), 3812–3819. doi:10.1016/j.scitotenv.2011.06.001
Li, W., Yang, J., Zhang, D., Li, B., Wang, E., and Yuan, H. (2018). Concentration and Community of Airborne Bacteria in Response to Cyclical Haze Events during the Fall and Midwinter in Beijing, China. Front. Microbiol. 9, 1741. doi:10.3389/fmicb.2018.01741
Li, X., Jiang, L., Hoa, L. P., Lyu, Y., Xu, T., Yang, X., et al. (2016). Size Distribution of Particle-phase Sugar and Nitrophenol Tracers during Severe Urban Haze Episodes in Shanghai. Atmos. Environ. 145, 115–127. doi:10.1016/j.atmosenv.2016.09.030
Li, Y., Zhang, R., Zhao, J., and Molina, M. J. (2020). Understanding Transmission and Intervention for the COVID-19 Pandemic in the United States. Sci. Total Environ. 748, 141560. doi:10.1016/j.scitotenv.2020.141560
Liebers, V., Van Kampen, V., Bunger, J., Duser, M., Stubel, H., Bruning, T., et al. (2012). Assessment of Airborne Exposure to Endotoxin and Pyrogenic Active Dust Using Electrostatic Dustfall Collectors (EDCs). J. Toxicol. Environ. Health A. 75 (8-10), 501–507. doi:10.1080/15287394.2012.674919
Liu, H., Zhang, X., Zhang, H., Yao, X., Zhou, M., Wang, J., et al. (2018). Effect of Air Pollution on the Total Bacteria and Pathogenic Bacteria in Different Sizes of Particulate Matter. Environ. Pollut. 233, 483–493. doi:10.1016/j.envpol.2017.10.070
Lu, R., Li, Y., Li, W., Xie, Z., Fan, C., Liu, P., et al. (2018). Bacterial Community Structure in Atmospheric Particulate Matters of Different Sizes during the Haze Days in Xi'an, China. Sci. Total Environ. 637-638, 244–252. doi:10.1016/j.scitotenv.2018.05.006
Lyu, W., and Wehby, G. L. (2020). Community Use of Face Masks and COVID-19: Evidence from A Natural Experiment of State Mandates in the US. Health Aff. 39 (8), 1419–1425. doi:10.1377/hlthaff.2020.00818
Madhwal, S., Prabhu, V., Sundriyal, S., and Shridhar, V. (2020). Ambient Bioaerosol Distribution and Associated Health Risks at a High Traffic Density junction at Dehradun City, India. Environ. Monit. Assess. 192 (3), 196. doi:10.1007/s10661-020-8158-9
Mahato, S., Pal, S., and Ghosh, K. G. (2020). Effect of Lockdown amid COVID-19 Pandemic on Air Quality of the Megacity Delhi, India. Sci. Total Environ. 730, 139086. doi:10.1016/j.scitotenv.2020.139086
Maki, T., Lee, K. C., Kawai, K., Onishi, K., Hong, C. S., Kurosaki, Y., et al. (2019). Aeolian Dispersal of Bacteria Associated with Desert Dust and Anthropogenic Particles over continental and Oceanic Surfaces. J. Geophys. Res. Atmos. 124 (10), 5579–5588. doi:10.1029/2018jd029597
Maruf, T. (2020). Flue Coughing and Fever in Every House. Kalerkantha- Bengali newspaper. Archived from the original on 18 February 2020. doi:10.23919/acc45564.2020.9148018
Morawska, L., and Milton, D. K. (2020). It Is Time to Address Airborne Transmission of Coronavirus Disease 2019 (COVID-19). Clin. Infect. Dis. 71 (9), 2311–2313. doi:10.1093/cid/ciaa939
Naqvi, H. R., Datta, M., Mutreja, G., Siddiqui, M. A., Naqvi, D. F., and Naqvi, A. R. (2021). Improved Air Quality and Associated Mortalities in India under COVID-19 Lockdown. Environ. Pollut. 268, 115691. doi:10.1016/j.envpol.2020.115691
Noorimotlagh, Z., Jaafarzadeh, N., Martínez, S. S., and Mirzaee, S. A. (2020). A Systematic Review of Possible Airborne Transmission of the COVID-19 Virus (SARS-CoV-2) in the Indoor Air Environment. Environ. Res. 193, 110612. doi:10.1016/j.envres.2020.110612
Park, J., Li, P.-F., Ichijo, T., Nasu, M., and Yamaguchi, N. (2018). Effects of Asian Dust Events on Atmospheric Bacterial Communities at Different Distances Downwind of the Source Region. J. Environ. Sci. 72, 133–139. doi:10.1016/j.jes.2017.12.019
Prather, K. A., Marr, L. C., Schooley, R. T., McDiarmid, M. A., Wilson, M. E., and Milton, D. K. (2020a). Airborne Transmission of SARS-CoV-2. Science 370 (6514), 303–304. doi:10.1126/science.abf0521
Prather, K. A., Wang, C. C., and Schooley, R. T. (2020b). Reducing Transmission of SARS-CoV-2. Science 368 (6498), 1422–1424. doi:10.1126/science.abc6197
Priyamvada, H., Singh, R. K., Akila, M., Ravikrishna, R., Verma, R. S., and Gunthe, S. S. (2017). Seasonal Variation of the Dominant Allergenic Fungal Aerosols - One Year Study from Southern Indian Region. Sci. Rep. 7 (1), 11171. doi:10.1038/s41598-017-11727-7
Rajput, P., Anjum, M. H., and Gupta, T. (2017). One Year Record of Bioaerosols and Particles Concentration in Indo-Gangetic Plain: Implications of Biomass Burning Emissions to High-Level of Endotoxin Exposure. Environ. Pollut. 224, 98–106. doi:10.1016/j.envpol.2017.01.045
Samake, A., Uzu, G., Martins, J. M. F., Calas, A., Vince, E., Parat, S., et al. (2017). The Unexpected Role of Bioaerosols in the Oxidative Potential of PM. Sci. Rep. 7 (1), 10978. doi:10.1038/s41598-017-11178-0
Šantl, T. T., Sikoparija, B., Maki, T., Carotenuto, F., Amato, P., Yao, M., et al. (2019). Bioaerosol Field Measurements: Challenges and Perspectives in Outdoor Studies. Aerosol Sci. Tech. 54, 1–27. doi:10.1080/02786826.2019.1676395
Shin, S. K., Kim, J., Ha, S. M., Oh, H. S., Chun, J., Sohn, J., et al. (2015). Metagenomic Insights into the Bioaerosols in the Indoor and Outdoor Environments of Childcare Facilities. PLoS One 10 (5), e0126960. doi:10.1371/journal.pone.0126960
Singh, V., Singh, S., Biswal, A., Kesarkar, A. P., Mor, S., and Ravindra, K. (2020). Diurnal and Temporal Changes in Air Pollution during COVID-19 Strict Lockdown over Different Regions of India. Environ. Pollut. 266, 115368. doi:10.1016/j.envpol.2020.115368
Soleimani, Z., Teymouri, P., Darvishi Boloorani, A., Mesdaghinia, A., Middleton, N., and Griffin, D. W. (2020). An Overview of Bioaerosol Load and Health Impacts Associated with Dust Storms: A Focus on the Middle East. Atmos. Environ. 223, 117187. doi:10.1016/j.atmosenv.2019.117187
Uda, S. K., Hein, L., and Atmoko, D. (2019). Assessing the Health Impacts of Peatland Fires: a Case Study for Central Kalimantan, Indonesia. Environ. Sci. Pollut. Res. 26 (30), 31315–31327. doi:10.1007/s11356-019-06264-x
Uk Lee, B., Lee, G., and Joon Heo, K. (2016). Concentration of Culturable Bioaerosols during winter. J. Aerosol Sci. 94, 1–8. doi:10.1016/j.jaerosci.2015.12.002
Valdez-Castillo, M., and Arriaga, S. (2021). Response of Bioaerosol Cells to Photocatalytic Inactivation with ZnO and TiO 2 Impregnated onto Perlite and Poraver Carriers. Front. Environ. Sci. Eng. 15 (3), 1–13. doi:10.1007/s11783-020-1335-9
Valdez-Castillo, M., Saucedo-Lucero, J. O., and Arriaga, S. (2019). Photocatalytic Inactivation of Airborne Microorganisms in Continuous Flow Using Perlite-Supported ZnO and TiO2. Chem. Eng. J. 374, 914–923. doi:10.1016/j.cej.2019.05.231
Valdez-Castillo, M., Saucedo-Lucero, J. O., Villalobos-Romero, K. L., Pérez-Rodriguez, F., and Arriaga, S. (2021). Steady-state Operation of a Biofilter Coupled with Photocatalytic Control of Bacterial Bioaerosol Emissions. Environ. Sci. Pollut. Res. 28 (11), 13970–13980. doi:10.1007/s11356-020-11583-5
Wang, D., Zhu, B., He, X., Zhu, Z., Hutchins, G., Xu, P., et al. (2018). Iron Oxide Nanowire-Based Filter for Inactivation of Airborne Bacteria. Environ. Sci. Nano 5 (5), 1096–1106. doi:10.1039/c8en00133b
Wang, Z., Li, J., Qian, L., Liu, L., Qian, J., Lu, B., et al. (2019). Composition and Distribution Analysis of Bioaerosols under Different Environmental Conditions. J. Vis. Exp. 143, e58795. doi:10.3791/58795
Wei, M., Xu, C., Xu, X., Zhu, C., Li, J., and Lv, G. (2019). Size Distribution of Bioaerosols from Biomass Burning Emissions: Characteristics of Bacterial and Fungal Communities in Submicron (PM1.0) and fine (PM2.5) Particles. Ecotoxicol. Environ. Saf. 171, 37–46. doi:10.1016/j.ecoenv.2018.12.026
Wu, Y., Gu, B., Erisman, J. W., Reis, S., Fang, Y., Lu, X., et al. (2016). PM2.5 Pollution Is Substantially Affected by Ammonia Emissions in China. Environ. Pollut. 218, 86–94. doi:10.1016/j.envpol.2016.08.027
Xie, Z., Fan, C., Lu, R., Liu, P., Wang, B., Du, S., et al. (2018). Characteristics of Ambient Bioaerosols during Haze Episodes in China: A Review. Environ. Pollut. 243 (Pt B), 1930–1942. doi:10.1016/j.envpol.2018.09.051
Xu, J., Jia, C., He, J., Xu, H., Tang, Y.-T., Ji, D., et al. (2019). Biomass Burning and Fungal Spores as Sources of fine Aerosols in Yangtze River Delta, China - Using Multiple Organic Tracers to Understand Variability, Correlations and Origins. Environ. Pollut. 251, 155–165. doi:10.1016/j.envpol.2019.04.090
Yadav, J., Kumar, A., Mahor, P., Goel, A. K., Chaudhary, H. S., Yadava, P. K., et al. (2015). Distribution of Airborne Microbes and Antibiotic Susceptibility Pattern of Bacteria during Gwalior Trade Fair, Central India. J. Formos. Med. Assoc. 114 (7), 639–646. doi:10.1016/j.jfma.2013.04.006
Yan, X., Qiu, D., Zheng, S., Yang, J., Sun, H., Wei, Y., et al. (2019). Distribution Characteristics and Noncarcinogenic Risk Assessment of Culturable Airborne Bacteria and Fungi during winter in Xinxiang, China. Environ. Sci. Pollut. Res. 26 (36), 36698–36709. doi:10.1007/s11356-019-06720-8
Ye, Q., Fu, J.-f., Mao, J.-h., and Shang, S.-q. (2016). Haze Is a Risk Factor Contributing to the Rapid Spread of Respiratory Syncytial Virus in Children. Environ. Sci. Pollut. Res. 23 (20), 20178–20185. doi:10.1007/s11356-016-7228-6
Yoo, K., Han, I., Ko, K. S., Lee, T. K., Yoo, H., Khan, M. I., et al. (2019). Bacillus-dominant Airborne Bacterial Communities Identified during Asian Dust Events. Microb. Ecol. 78 (3), 677–687. doi:10.1007/s00248-019-01348-0
Zeng, X., Kong, S., Zheng, S., Cheng, Y., Wu, F., Niu, Z., et al. (2019). Variation of Airborne DNA Mass Ratio and Fungal Diversity in fine Particles with Day-Night Difference during an Entire winter Haze Evolution Process of Central China. Sci. Total Environ. 694, 133802. doi:10.1016/j.scitotenv.2019.133802
Zhang, X.-X., Claiborn, C., Lei, J.-Q., Vaughan, J., Wu, S.-X., Li, S.-Y., et al. (2020). Aeolian Dust in Central Asia: Spatial Distribution and Temporal Variability. Atmos. Environ. 238, 117734. doi:10.1016/j.atmosenv.2020.117734
Keywords: particulate matter, airborne bioaerosols, human health, PM2.5, seasonal variation, inactivation
Citation: Shammi M, Rahman MM and Tareq SM (2021) Distribution of Bioaerosols in Association With Particulate Matter: A Review on Emerging Public Health Threat in Asian Megacities. Front. Environ. Sci. 9:698215. doi: 10.3389/fenvs.2021.698215
Received: 21 April 2021; Accepted: 26 July 2021;
Published: 20 August 2021.
Edited by:
Anabela Cachada, University of Porto, PortugalReviewed by:
Sonia Arriaga, Instituto Potosino de Investigación Científica y Tecnológica (IPICYT), MexicoVijay Shridhar, Doon University, India
Copyright © 2021 Shammi, Rahman and Tareq. This is an open-access article distributed under the terms of the Creative Commons Attribution License (CC BY). The use, distribution or reproduction in other forums is permitted, provided the original author(s) and the copyright owner(s) are credited and that the original publication in this journal is cited, in accordance with accepted academic practice. No use, distribution or reproduction is permitted which does not comply with these terms.
*Correspondence: Mashura Shammi, bWFzaHVyYTkyNkBqdW5pdi5lZHU=; orcid.org/0000-0001-5449-4761