- 1Key Laboratory of Agro-Forestry Environmental Processes and Ecological Regulation of Hainan Province, College of Ecology and Environment, Hainan University, Haikou, China
- 2Rattan & Flower, Sanya Research Base, International Center for Bamboo and Rattan, Institute of Tropical Bamboo, Sanya, China
- 3State Key Laboratory of Grassland and Agro-Ecosystems, College of Ecology, Lanzhou University, Lanzhou, China
- 4College of Tropical Cops, Hainan University, Haikou, China
- 5Guangxi Agricultural Vocational and Technical University, Nanning, China
- 6National Ecological Research Station of Sanya Bamboo and Rattan Associated Forest Ecosystem, Sanya, China
Arbuscular mycorrhizal fungi play an important role in mediating plant-soil interactions across succession stages. However, AMF community dynamics which about the change of community composition and member activity remain unclear. To complete the gap knowledge about microbial community dynamics during restoration succession, soil AMF community composition was studied within a tropical forest ecosystem in the Ganshiling nature reserve using high throughput sequencing methods. The results revealed that soil AMF communities characteristics about speices diversity, species composition and microbial correlation network showed significant differences between shrubland (SC) and secondary forest ecosystems, but the same differences were not found between 40-year recovery secondary forest (SF40) and 60-year recovery secondary forest (SF60). Plant community dynamics were the key factor for regulating soil AMF communities among succession stages. An important biotic factor explaining variance in AMF community composition was root biomass. The correlation network analysis showed that although the nodes were similar among succession stages, the complexity of networks was significant higher in SF40 than in SC and SF60, suggesting that AMF communities were more active in SF40, which verified the hypothesis of intermediate disturbance hypothesis. This study provides new insights into AMF community dynamics and their driving factors across succession stages, as well as expanding knowledge of the ecological value of AMF for tropical forest restoration processes.
1 Introduction
Mycorrhizal plant-fungal symbiosis as mediators impact organic matter synthesized by plant photosynthesis to exchange with soil nutrients and increases the root surface 100 or 1,000 times by forming extraradical mycelia, causing water and nutrients to be more efficiently absorbed by roots (Finlay, 2008; Barea et al., 2011). Approximately 80% of plants can be symbiotically interacted with AMF, which forms mycelia within vegetation root cells and colonizes various ecosystems (de Oliveira Freitas et al., 2014). AMF promote host plant resistance (van der Heijden and Horton, 2009; Achatz and Rillig, 2014; Johnson and Gilbert, 2015) and play key roles in the terrestrial ecosystem.
In recent years, most research has paid attention to AMF community dynamics under restoration secondary succession and its influence factors. The host preference of AMF influences the composition of aboveground vegetation communities with secondary succession. Due to its host preference, AMF drives some host plant to benefit more than others (Janos, 1980). Nutrients and water are transferred between individual plants through an underground mycorrhizal established by AMF community, significantly increasing the competitive ability of specific hosts and altering host growth stability. As a result, the composition of above-ground vegetation changes (Janos, 1980; Kikvidze et al., 2010; Shi et al., 2016). Through mycelia formation in roots, AMF mediates plant-soil feedback across succession stages, altering the traits of host plant and improving the hosts’ efficiency for absorbing water and nutrients (Jansa et al., 2011; Jansa et al., 2019). As plant communities progress, AMF colonization is the major factor driving plant community dynamics during secondary succession (Zobel et al., 2014). Mycorrhizal colonization expands and/or complements plant root function: mycorrhizal expands the regional range of resource acquisition by increasing the contact area between roots and soil, and mycelial networks established by AMF enhance the soil nutrition and water acquisition capacity of plants. Host plants’ access to soil minerals and nutrients with low mobility is enhanced via mycelial networks (e.g., phosphorous, zinc, and copper; Jansa et al., 2011). At the same time, by increasing the input of litter and organic matter, AMF communities are influenced by improving soil properties along succession stages (Casazza et al., 2017; Winagraski et al., 2019).
In addition, the effect of AMF host preferences on plant community structure may induce change in AMF community dynamics. Some studies indicate that the variation of host plants also affects the soil properties, like soil moisture and nutrient availability (Jiao et al., 2011). The variations of soil properties determine the nutrient acquisition patterns of host plants under plant succession. Due to the demand relationship between plants and AMF, AMF community structure was indirectly affected by the availability of soil properties (van der Heijden et al., 2008; Casazza et al., 2017). Moreover, aboveground vegetation communities may direct influence AMF community structure through apoplast chemistry (Li et al., 2020).
AMF communities exhibit significant variation in compositional with plant succession ((Kruger et al., 2017). As succession proceeds, AMF community was composed of some compatible species populations in early succession stages to broad species composition. Meanwhile, several studies have demonstrated the prevalence of stochastic processes in AMF communities in mature ecosystems, favouring builds a broad species composition of AMF communities (Kruger et al., 2017). And with plant succession, increased habitat diversity drives the formation of AMF community patches, also leading to variation in AMF community composition (Walker and del Moral, 2003). In addition, with forest disturbance, AMF communities which are highly resilient and equally resistant had no significant change (Soteras et al., 2015). However, it has also been shown that land degradation affects the AMF community dynamics (Asmelash et al., 2016).
In tropical rainforest ecosystems, AMF symbiosis with aboveground vegetation has revealed their potential importance for biogeochemical cycling (Jansa et al., 2011; Parihar et al., 2020), although it is unclear how environmental factors relate to AMF across succession stages. The tropical forest in Ganshiling, Hainan Province was subjected to large-scale logging in the 1980s, making it an important study area for ecosystem succession (Qi et al., 2014). This study addresses the following two questions: i) to explore the change of AMF community composition and its response mechanism and ii) the most influential environmental factors in AMF community dynamic during restoration succession. Revealing AMF community dynamics in the Ganshiling area during restoration succession is of great significance in vegetation restoration, and it could provide insights in regional ecological protection and the sustainable management of forests. Therefore, the characteristics and influencing factors of soil AMF communities were investigated in different forest succession stages of Ganshiling.
2 Materials and methods
2.1 Study area
Ganshiling Natural Reserve served as the site of the study (109°37’∼109°41′E, 18°19’∼18°24′N), a typical tropical lowland rainforest from 50 to 681 m, and the slope is <50°. It is a typical lowland hilly landform with a tropical marine monsoon climate on Hainan Island, China. The annual average precipitation and temperature are 1800 mm and 25.4°C, respectively. The soil parent material was granite and the main plant species in this area include Hopea reticulata, Vatica mangachapoi, Koilodepas hainanense, Rhodomyrtus tomentosa, Melastoma sanguineum, Scleria elata and Blechnum orientale (Qi et al., 2014; Mao et al., 2021).
2.2 Sampling collection and environmental variables
Three succession stages in Ganshiling Natural Reserve were studied: 1) the shrub forest (SC; 109°39’∼109°41′E, 18°22’∼18°23′N), was subjected to logging and formed by artificial disturbance such as construction of nature reserve, and the main plant species include: Aporosa dioica and Olea hainanensis; 2) the 40 years recovery secondary forest after logging ceased in 1979 (SF40; 109°39’∼109°40′E, 18°21’∼18°23′N), and the main plant species include: Garcinia oblongifolia and Vatica mangachapoi; and 3) the 60 years recovery secondary forest after logging ceased in 1959 (SF60; 109°39’∼109°40′E, 18°22’∼18°23′N), and the main plant species include: Vatica mangachapoi and Hopea reticulata. Detailed information of each sample were shown in Supplementary Table S1.
In August 2019, five soil samples of 0–10 cm depth were obtained in each plot from three plots of 900 m2 (30 m × 30 m) in each succession stage. These samples were then divided into portions intended for AMF community (stored at −80°C) and soil property assessment. The soil moisture was measured by gravimetric method (Blake, 1965). A 2 mm screen sieve was used to sieve air-dried soil samples for analyzing soil pH, soil organic carbon (SOC), total nitrogen (TN), and total phosphorus (TP), as well as soil stoichiometric characteristics (e.g., C/N ratio, C/P ratio, and N/P ratio). In order to measure soil pH, soil suspensions were mixed with distilled water. An analysis of the soil TN and TP was conducted using sulfuric acid and potassium sulfate after digestion with sulfuric acid, and then followed with the protocol described by Kjeldahl (Bremner, 1960; Taylor, 2000).
At the same time, vegetation surveys and root samples were conducted on sample plots to obtain aboveground plant community characteristics. Using gravimetric method to measure root biomass. And the vegetation survey data were used to calculate the Shannon-Wiener index, Margalef index and Pielous index.
2.3 DNA extraction and amplicon sequencing
Using the MIO-BIO PowerSoil DNA Isolation Kit (MO BIO Laboratories, Carlsbad, CA, United States), soil DNA was extracted from 250 mg soil samples. The NanoDrop ND-2000 Spectrophotometer (Thermo Fisher Scientific, Waltham, MA, United States) was used to analyze and quantify DNA, which was prepared at a standard concentration for PCR amplification.
For PCR amplification, AMV4.5NF (5′-AAGCTCGTAGTTGAATTTCG-3′) and AMDGR (5′-CCCAACTATCCCTATTAATCAT-3′) were used as primers (Lumini et al., 2010). An AMF library was constructed using two-step PCR amplification. Target fragments were amplified using specific primers. Later, an Illumina adapter sequence, a primer pad, and a barcode were added into both the forward and reverse target fragments. A 50 ml reaction volume was used for PCR of each sample, comprising 10 ml of 5x buffer, 1 ml of dNTP (10 m/M), 1 U of Phusion DNA High-Fidelity polymerase (New England Biolabs, Ipswich, MA, United States), 1 ml of forward and reverse phasing primers, and 5–50 ng of target sequencing. The reaction volume was supplemented to 50 μl with RNase free ultrapure water. An ABI9700 PCR amplifier (Applied Biosystems, Waltham, MA, United States) was used to amplify AMF. Amplification products were kept warm at 10°C. With the assistance of an AxyPrepDNA Gel Recovery Kit (Axygen Scientific, Corning, NY, United States), electrophoresis was used on an agarose gel to pool and purify PCR products. FTC 3000TM real-time PCR (Fungyln Biotech, Richmond Hill, Ontario, Canada) was used to quantify purified DNA and mixed to an equal molar ratio to perform the second PCR amplification. When the second PCR amplification, the reaction volume change to 40 μl: 5x buffer (8 μl), dNTP (1 μl, 10 m/M), Phusion High-Fidelity polymerase (0.8 U, New England Biolabs, Ipswich, MA, United States), both forward and reverse phasing primer (1 μl), respectively, and DNA template (5 μl) and RNase free ultrapure water (5 μl).
Using different barcodes to identity sequences of different samples in the parallel sequencing test, the sequence with a complete barcode was regarded as a valid sequence and extracted to a dataset stored in the FASTQ format. Quality assurance and splicing was performed on the valid sequences, the specific process for which follows: 1) using the Trimmomatic program, poorly overlapped and low-quality sequences (quality score <20, window size <5) were removed (Bolger et al., 2014); 2) a merged pair of reads as a sequence was used to refer to the overlap relationship of PEreads (>10 bp) by the FLASH program (Magoc and Salzberg, 2011); and 3) merge sequences were screened according to error ratio (<0.2). By using mothur V.1.39.5, quality control of merge reads was performed by removing sequences that were ambiguous, homologous, or chimeras generated during PCR amplification (Schloss et al., 2009). Analysis of species information and operational taxonomic units (OTUs) clustering was based on the clean tags. OTUs were selected using UPARSE with a cutoff of 97% similarity (Edgar, 2013). The dataset was screened for chimeric reads using UCHIME in reference database mode (Edgar et al., 2011). Through USEARCH_global, all sequences were compared to OTU representative sequences to obtain the OTU table. The identity of OTU taxonomy was determined by using Blast to compared representative sequences with the Maarjam database (Zhang et al., 2000; Opik et al., 2010). The taxonomy of OTU was obtained for species which had the highest similar identity with OTU representative sequences in the Maarjam database, except for uncultivated environmental information. Some OTUs without species information were marked ‘Unidentified’. The library preparation and sequencing were performed by TinyGene Bio-Tech (Shanghai, China). Raw sequence data has been submitted to NCBI’s Sequence Read Archives (accession no. PRJNA884298).
2.4 Bioinformatic and environmental correlation analyses
In order to explore the AMF community dynamics, the change in distribution and composition of AMF were analyzed. Furthermore, the impact of environmental factor on AMF community dynamics were explored.
For bioinformation analysis, some OTUs that the classification results could not identify specific genus, and <5 sequences were not used. The species diversity was calculated in R package “vegan”. The non-metric multidimensional (NMDS) and principal coordinate analysis (PCoA) were analyzed using Bray-Crutis dissimilarity with R. The Venn diagram was used to demonstrate the distribution of OTUs during succession stages by R version 4.1.2.
Based on this dataset, the genus level was used to explore AMF composition differences which had the clearest taxonomy annotations. To explored the AMF community difference across groups, the OTU level was used for analysis. The top 10 relative abundances under genera and OTUs were selected for multi-group differential analysis.
Using R package ‘microbiomeMarker’, the linear discriminant analysis effect size (LEfSe) and linear discriminant analysis (LDA) were performed (Segata et al., 2011). Screening the OTUs which it frequency of occurrence >70% in samples for the following calculation. According to Spearman’s correlation coefficient (p-value ≤0.05), the dominant OTUs was selected to construct microbial network. The microbial network was using selected dominant OTUs as nodes and its strong correlations as edges. The screened of dominant OTUs and visualize data preparation were completed by R, and the microbial network visualized with Gephi (Sirova et al., 2018; Nauta et al., 2020).
By using R version 4.1.2, the mantel test, two-factor correlation heatmap and distance-based redundancy analysis (db-RDA) was analyzed. The significances of environmental factors with AMF were tested using Monte Carlo permutation ANOVA (Tamminen et al., 2018).
3 Result
3.1 The distribution of soil AMF community across succession stages
Among alpha diversity indices, the Chao1, ACE, and phylogenetic diversity (PD) of the soil AMF community showed significant differences between shrubland and secondary forest (p < 0.05). These indices showed an increasing trend with succession. Compared with other succession stages, SF60 group had the highest Chao1, ACE, and PD (Table 1). Conversely, Simpson and Shannon-Wiener indices showed no significant differences across succession stages (Table 1).
NMDS, PCoA, Venn, differential, and beta diversity analyses revealed significant differences in soil AMF communities between shrubland and secondary forest, but not between SF40 and SF60 (Figure 1A; Supplementary Figures S2, S3).
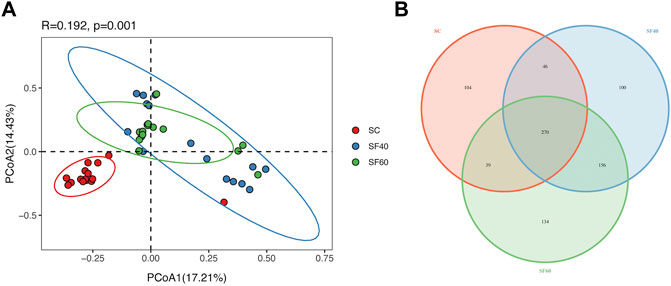
FIGURE 1. Principal coordinate analysis (PCoA) of AMF community (A) in succession. Point represent samples with points in the same colour representing samples of the same group. OTUs represent common or unique OTUs to a group in the Venn diagram (B). SC, shrub forest; SF40, the 40a secondary forest; SF60, the 60a secondary forest.
The Venn diagram showed that there were 270 shared OTUs in different groups, and the number of shared OTUs between SC and SF40 and between SC and SF60 were 46 and 39, respectively. However, the number of shared OTUs between SF40 and SF60 was 156 (Figure 1B). Kuklospora and Redeckera were just present in SC, and Diversispora were only present in secondary forests, respectively. In summary, AMF community distribution was influenced by succession, and the differences between shrubland and secondary forest were especially obvious.
3.2 The composition of soil AMF community
As succession stages progressed, the composition of soil AMF community changed. As a result, Paraglomus and Glomus emerged as dominant genera, with Paraglomus exhibiting higher relative abundances in SF40. In contrast, the higher relative abundance of Glomus and Claroideoglomus was present in SC and SF60 (Figure 2A).
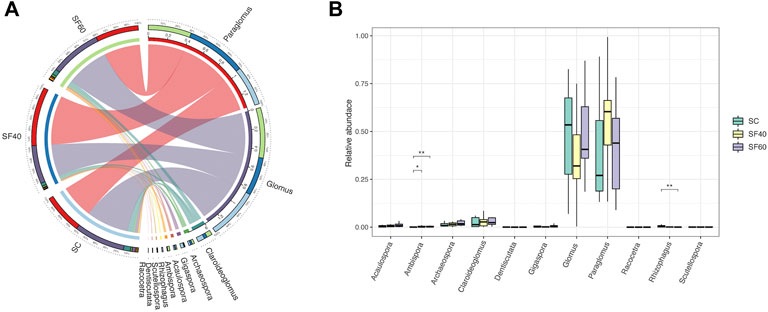
FIGURE 2. The Circos diagram (A) represents the AMF community’s contribution in succession across groups based on genera. Each colour represented a gene and the columnar size shows the relative abundance. The AMF contribution was combined with different colour columnar. The significant test of genera differences relative to abundance in multi-groups (B), which is analysed using Wilcoxon rank-sum test. * (p < 0.05); ** (p < 0.01); SC, shrub forest; SF40, the 40a secondary forest; SF60, the 60a secondary forest.
At the genera level, the multi-group differential analysis showed the variation of Paraglomus and Glomus were non-significant during succession. In addition, Ambispora and Rhizophagus were differential genera in succession, and Ambispora was significantly less abundant in shrubland than in secondary forest. In addition, Rhizophagus had the highest relative abundance in shrubland, and showed a decreasing trend with succession (Figure 2B).
At the OTUs level, the multi-group differential analysis showed that most of the Top 10 OTUs belonged to Paraglomus and Glomus, and only OTU15 belonged to Claroideoglomus. Multi-group differential analysis showed that the relative abundance of OTU1 and OTU4 increased along succession stages. The relative abundances of OTU5, OTU6, and OTU72 were greater in SC than in SF40 and SF60. OTU1, OTU2 and OTU3 were higher in SF40 than in SC and SF60 (Figure 3).
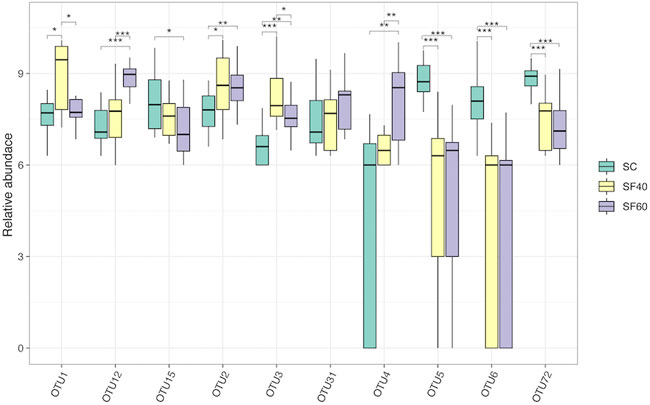
FIGURE 3. The significant test of difference of the top10 OTUs relative abundance in multi-group. The significant difference is analysed using analyzed by Wilcoxon rank-sum test. * (p < 0.05); ** (p < 0.01); *** (p < 0.001); SC, shrub forest; SF40, the 40a secondary forest; SF60, the 60a secondary forest.
LEfSe analysis showed that Rhizophagus and Geosiphon were specific genus in SC, but Ambispora and Diversispora were specific genus in SF60 (Figure 4).
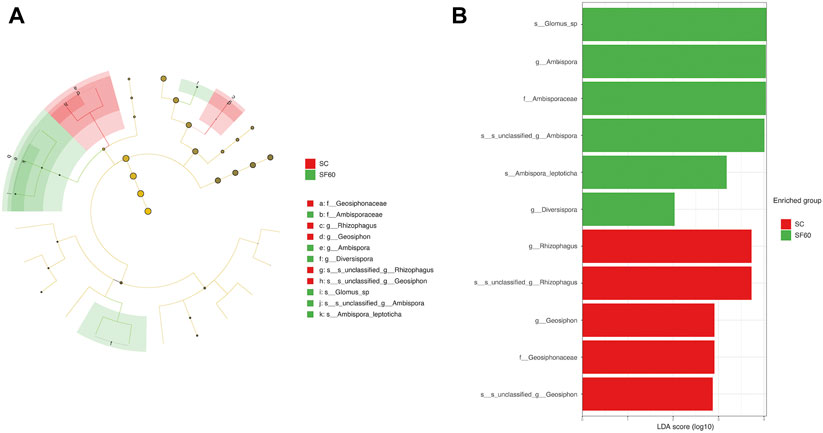
FIGURE 4. Linear discriminant analysis effect size (LEfSe) analysis in AMF composition across succession. Phylum to species is represented by the cladogram circle (A). Points at different taxonomy levels represent microbial groups within that level, and their diameters represent relative abundances. Similarly colored enriched points are represented in a group. The yellow point represents microbes with no significant differences. The microbial groups with LDA score >2 are in the histogram (B). SC, shrub forest; SF40, the 40a secondary forest; SF60, the 60a secondary forest.
3.3 Correlation network construction of AMF community
The correlation network analysis showed AMF community co-occurrence patterns were significantly different between succession stages. Although the nodes were similar among the three groups, the edges were significantly higher in SF40 (nodes = 54, edges = 272) than in SC (nodes = 56, edges = 145) and SF60 (nodes = 53, edges = 170). Furthermore, the SF40 network had higher network topological properties (average degree, network diameter, and clustering coefficient) than SC and SF60. Although most of modes in these networks were similar, the interaction between nodes were significantly different among groups (Figure 5).
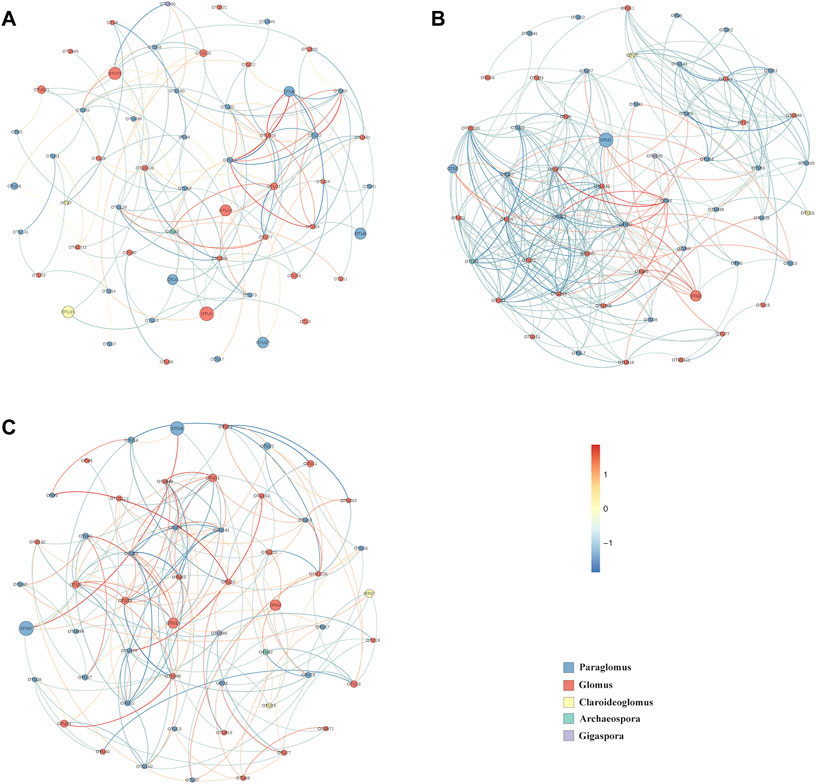
FIGURE 5. The correlation network of AMF community in SC (A), SF40 (B) and SF60 (C). Strong and significant correlations (Spearman’s r > 0.5) indicate a connection. Positive correlations are represented by red edges, while blue edges represent negative correlations. The size of the nodes represents OTUs abundance. Nodes represented by the same colour belong to the same genera.
3.4 Relationship between environmental factors and AMF community
In the Mantel test, aboveground plants community characteristics were the major factor in varieties of soil AMF community composition (r2 = 0.608, p = 0.004; Table 2). Likewise, the db-RDA analyses also showed that the Margalef index, Shannon-Wiener index, Pielou index, and root biomass of aboveground vegetation were drivers in changes of AMF community composition (Figure 6). In addition, the soil moisture, SOC, and total N—which belong to abiotic factors—also affect AMF community composition significantly (Supplementary Table S2). The composition of AMF communities could be explained by 54.2% of these factors. Among them, root biomass was the key factors which influenced AMF community composition (r2 = 0.789, p = 0.012; Figure 6; Supplementary Table S4).
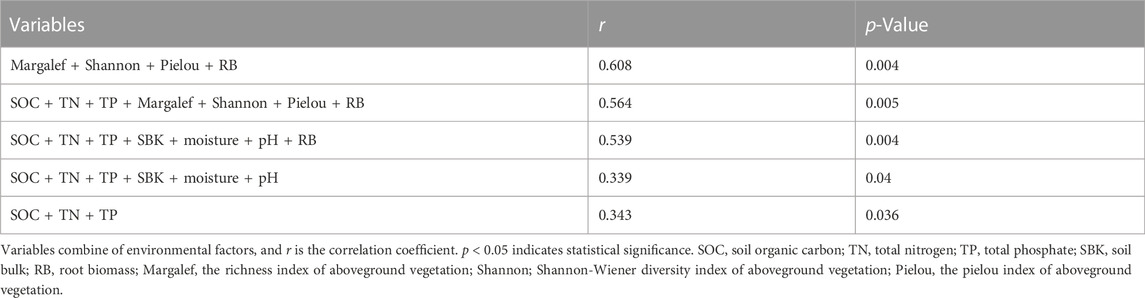
TABLE 2. Mantel test on the correlations between the relative abundance of OTUs and biotic or abiotic variables.
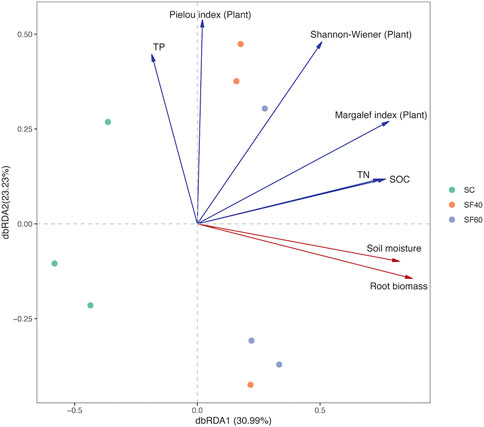
FIGURE 6. The db-RDA analysis based on Bray-Crutis distance in OTU levels. Relationships between AMF community and environment plotted. Each point represents a plot with the same colour in each group. SOC, soil organic carbon; TN, total nitrogen; TP, total phosphate; RB, root biomass.
The two-factor correlation heatmap of network attributes and environmental factors showed that C/N ratio significantly correlated to Harmonic geodesic distance (HD), network transitivity (Trans), and average clustering coefficient (avgCC), while root biomass (RB) and Margalef significantly correlated with total nodes (Figure 7).
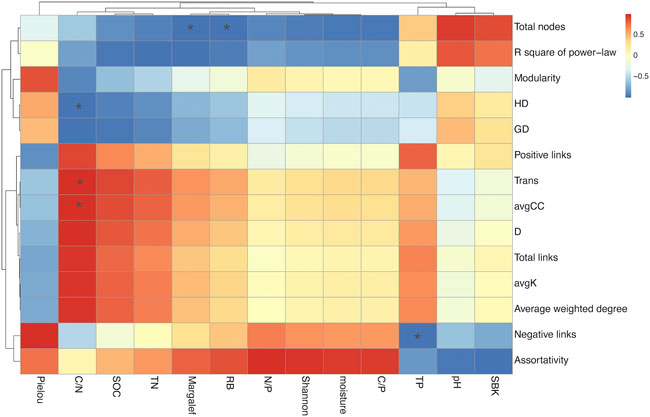
FIGURE 7. The two-factors correlation heatmap. The plot illustrates the relationship between AMF microbial network attributes and environmental factors. * (p < 0.05); HD, Harmonic geodesic distance; GD, Average path distance; Trans, Transitivity; avgCC, Average clustering coefficient; D, Density; avgK, Average degree; SOC, soil organic carbon; TN, total nitrogen; TP, total phosphate; RB, root biomass.
4 Discussions
4.1 Characteristics of AMF community among the forest succession
With succession, the dominant vegetation gradually evolves from shrubs to trees, and it showed an increase trend in vegetation community complexity. Consistent with the variation of aboveground vegetation communities, AMF species richness and spectral diversity were increased during succession stages in this study (Table 1), and it could be confirmed that the AMF is closely related to host plants, which have host preferences (Janos, 1980; Xu T. et al., 2016; Li et al., 2020). It could be further found that the composition of AMF communities is highly similar in higher taxonomy levels during restoration succession, and it contrasted to vegetation communities composition had significant differences with succession (Leal et al., 2013; Reyes et al., 2019). For example, AMF community composition was similar at the genera level during succession in this study (Figure 2B), and the differences in AMF community composition were significant at the OTU level (Figure 3). In this study, Paraglomus and Glomus dominated in AMF community during succession, which benefits from its biological characteristics that have wide biogeographic distributions and vegetation cover (Figure 2A) (Lovelock et al., 2003). This study demonstrates that the importance of OTUs varied significantly between groups. This dynamic is associated with changes in the AMF niche and functionality at the OTUs level and it confirms that the host preference of AMF community was reflected in changes to the species importance and many coexisting microorganisms have functional redundancy at the OTU level (Louca et al., 2018). The niche analysis of keystone microbial taxa at different successional stages proof that the niche of these keystone taxa at each stage was clearly differentiated (Supplementary Figure S5). In generally, AMF functional redundancy and niche changes are likely response mechanisms to help AMF adapt the habitat changes during succession which consistent with previous studies (Varela-Cervero et al., 2015; Alguacil et al., 2016).
4.2 Influencing factors of AMF community
This study showed solid correlation between plant communities and soil AMF communities (r2 = 0.608, p = 0.001; Table 2). As an important factor, the host plants could affect the AMF community dynamic by altering the microhabitat (McGuire et al., 2012; Chai et al., 2019). With the change in vegetation community, the host plant reshapes the AMF community composition by secreting apoplast chemistry and root exudates (Goldmann et al., 2015; Hanif et al., 2019; Li et al., 2020). This effect further impacts the uptake of nutrients and water by vegetation (McGuire et al., 2012; Nakayama et al., 2019). In this study, the root biomass also has the strongest correlation with soil AMF community (r2 = 0.789, p = 0.012; Figure 6; Supplementary Table S4) because microbial activity and growth could be stimulated by roots (Wang et al., 2017). By altering root turnover and/or root exudation, the change in root biomass may affect resource availability for the soil AMF community (Philippot et al., 2013). As well as influencing soil microbial community nutrient uptake, root exudates act as signaling molecules and attractants, as well as inhibitors or repellents (Baetz and Martinoia, 2014). Therefore, the soil AMF community might be regulated by changes in root biomass during succession (Eisenhauer et al., 2017).
In addition, soil properties and AMF community composition correlated strongly (Figure 6). Decomposition rates of organic matter and nutrient cycling were directly influenced by soil properties, which contributed to a diverse community of soil AMF (Xu X. et al., 2016). The alternations in AMF community also react upon nutrient cycling (Phillips et al., 2013). As an important environmental factor, the soil moisture drive for soil AMF community characteristics (r2 = 0.689, p = 0.036; Figure 6; Supplementary Table S4). There is a direct relationship between soil moisture and nutrient effectiveness, which could impact on the soil microbial diversity and abundance through affect microbial nutrient uptake, and it could further affect in soil AMF community composition (Deepika and Kothamasi, 2015; Peng et al., 2021). In addition, soil moisture had clear legacy effects on soil properties, the nutrient uptake of plants, the formation of AMF, and mycorrhizal responsiveness, which would alter the synergistic relationship between AMF and host plants (Cavagnaro, 2016).
4.3 Influence factors of AMF correlation network
This study’s results showed that the most complex network occurs in SF40 (Figure 5). In the Ganshiling Nature Reserve, the aboveground vegetation suffered extensive deforestation in the 1980s and gradually recovered to become a tropical lowland rainforest after successive anthropogenic disturbances. With increasing restoration years, the intensity of disturbance to the successional stages which were selected for this study varied. With further explored, it could be found that SF40 suffer intermediate disturbance frequency compared with other. According to intermediate-disturbance hypothesis (IDH), the SF40 with intermediate disturbance frequency could keep the most active AMF community. Moderate ecological scale disturbances keep off interspecific competition and provide supportive environment for microbial species which about both early and late succession to survival (Grime, 1973; George et al., 2019).
Moreover, consistent with existing research, the two-factor correlation heatmap showed that changes in AMF community structure were closely related to soil C/N ratios (Figure 7; Wan et al., 2014). It is well established that the Trans and avgCC positively correlated with AMF community activity and HD showed negative correlation with it. In general, a significant positive correlation between change in soil C/N ratio and AMF community activity as succession progressed. Soil C/N ratio responds to soil nutrient status by influencing nutrient mineralization and organic matter decomposition. It has been shown that the soil C/N ratio affects microbial biomass and activity by altering the nutrient effectiveness and quality of the soil substrate in which microorganisms survive (Demoling et al., 2007).
5 Conclusion
Across the succession stages of restoration, significant differences were observed in soil AMF community structure in this study. Moreover, the results not only support that the variation of soil AMF community structure was much higher at the OTU than at the genera, but also confirmed that plant community characteristics are more closely related to the soil AMF community than soil properties.
Root biomass mostly explained the variation in AMF communities during succession stages. Moreover, soil C/N ratio was positively correlated with AMF microbial network complexity significantly. Overall, this study provided theoretical data relevant to ecological restoration and the sustainable development of tropical forests. The study contributed to the development of knowledge about AMF communities in the tropical forest ecosystem of Hainan.
Data availability statement
The datasets presented in this study can be found in online repositories. The names of the repository/repositories and accession number(s) can be found below: https://www.ncbi.nlm.nih.gov/sra/, PRJNA884298.
Author contributions
The study was conceived and designed by SM and HM. The lab work and the laboratory analysis of YJ, GX, and XW were assisted by these individuals. SM wrote the main manuscript text. Insightful discussions were contributed by QY, HY, TH, and WL. All authors reviewed the manuscript.
Funding
Support for this study is provided jointly by the Natural Science Foundation of Hainan province (2019RC012); Supported by the Foundation Research Funds of the Central Non-profit International Center for Bamboo and Rattan (1632020022); Supported by Special Foundation of China Postdoctoral Science (2019T120073); Supported by China Postdoctoral Science Foundation (2018M631414).
Acknowledgments
We are grateful to many staff members who were involved in the maintenance of the field plots and the collection of samples, but who are not listed as coauthors. Additionally, we would like to thank Prof. Hongwei Zhao for his valuable suggestions during the revision process.
Conflict of interest
The authors declare that the research was conducted in the absence of any commercial or financial relationships that could be construed as a potential conflict of interest.
Publisher’s note
All claims expressed in this article are solely those of the authors and do not necessarily represent those of their affiliated organizations, or those of the publisher, the editors and the reviewers. Any product that may be evaluated in this article, or claim that may be made by its manufacturer, is not guaranteed or endorsed by the publisher.
Supplementary material
The Supplementary Material for this article can be found online at: https://www.frontiersin.org/articles/10.3389/fenvs.2022.1110655/full#supplementary-material
References
Achatz, M., and Rillig, M. C. (2014). Arbuscular mycorrhizal fungal hyphae enhance transport of the allelochemical juglone in the field. Soil Biol. Biochem. 78, 76–82. doi:10.1016/j.soilbio.2014.07.008
Alguacil, M. D. M., Torres, M. P., Montesinos-Navarro, A., and Roldan, A. (2016). Soil characteristics driving arbuscular mycorrhizal fungal communities in semiarid mediterranean soils. Appl. Environ. Microbiol. 82 (11), 3348–3356. doi:10.1128/aem.03982-15
Asmelash, F., Bekele, T., and Birhane, E. (2016). The potential role of arbuscular mycorrhizal fungi in the restoration of degraded lands. Front. Microbiol. 7, 1095. doi:10.3389/fmicb.2016.01095
Baetz, U., and Martinoia, E. (2014). Root exudates: The hidden part of plant defense. Trends Plant Sci. 19 (2), 90–98. doi:10.1016/j.tplants.2013.11.006
Barea, J. M., Palenzuela, J., Cornejo, P., Sánchez-Castro, I., Navarro-Fernández, C., Lopéz-García, A., et al. (2011). Ecological and functional roles of mycorrhizas in semi-arid ecosystems of Southeast Spain. J. Arid Environ. 75 (12), 1292–1301. doi:10.1016/j.jaridenv.2011.06.001
Bolger, A. M., Lohse, M., and Usadel, B. (2014). Trimmomatic: A flexible trimmer for Illumina sequence data. Bioinformatics 30 (15), 2114–2120. doi:10.1093/bioinformatics/btu170
Bremner, J. G. M. (1960). Determination of nitrogen in soil by the Kjeldahl method. J. Agric. Sci. 55, 11–33. doi:10.1017/s0021859600021572
Casazza, G., Lumini, E., Ercole, E., Dovana, F., Guerrina, M., Arnulfo, A., et al. (2017). The abundance and diversity of arbuscular mycorrhizal fungi are linked to the soil chemistry of screes and to slope in the Alpic paleo-endemic Berardia subacaulis. PLoS One 12 (2), e0171866. doi:10.1371/journal.pone.0171866
Cavagnaro, T. R. (2016). Soil moisture legacy effects: Impacts on soil nutrients, plants and mycorrhizal responsiveness. Soil Biol. Biochem. 95, 173–179. doi:10.1016/j.soilbio.2015.12.016
Chai, Y., Cao, Y., Yue, M., Tian, T., Yin, Q., Dang, H., et al. (2019). Soil abiotic properties and plant functional traits mediate associations between soil microbial and plant communities during a secondary forest succession on the loess plateau. Front. Microbiol. 10, 895. doi:10.3389/fmicb.2019.00895
de Oliveira Freitas, R., Buscardo, E., Nagy, L., dos Santos Maciel, A. B., Carrenho, R., and Luizao, R. C. (2014). Arbuscular mycorrhizal fungal communities along a pedo-hydrological gradient in a Central Amazonian terra firme forest. Mycorrhiza 24 (1), 21–32. doi:10.1007/s00572-013-0507-x
Deepika, S., and Kothamasi, D. (2015). Soil moisture--a regulator of arbuscular mycorrhizal fungal community assembly and symbiotic phosphorus uptake. Mycorrhiza 25 (1), 67–75. doi:10.1007/s00572-014-0596-1
Demoling, F., Figueroa, D., and Bååth, E. (2007). Comparison of factors limiting bacterial growth in different soils. Soil Biol. Biochem. 39 (10), 2485–2495. doi:10.1016/j.soilbio.2007.05.002
Edgar, R. C., Haas, B. J., Clemente, J. C., Quince, C., and Knight, R. (2011). UCHIME improves sensitivity and speed of chimera detection. Bioinformatics 27 (16), 2194–2200. doi:10.1093/bioinformatics/btr381
Edgar, R. C. (2013). Uparse: Highly accurate OTU sequences from microbial amplicon reads. Nat. Methods 10 (10), 996–998. doi:10.1038/nmeth.2604
Eisenhauer, N., Lanoue, A., Strecker, T., Scheu, S., Steinauer, K., Thakur, M. P., et al. (2017). Root biomass and exudates link plant diversity with soil bacterial and fungal biomass. Sci. Rep. 7, 44641. doi:10.1038/srep44641
Finlay, R. D. (2008). Ecological aspects of mycorrhizal symbiosis: With special emphasis on the functional diversity of interactions involving the extraradical mycelium. J. Exp. Bot. 59 (5), 1115–1126. doi:10.1093/jxb/ern059
George, P. B. L., Lallias, D., Creer, S., Seaton, F. M., Kenny, J. G., Eccles, R. M., et al. (2019). Divergent national-scale trends of microbial and animal biodiversity revealed across diverse temperate soil ecosystems. Nat. Commun. 10 (1), 1107. doi:10.1038/s41467-019-09031-1
Goldmann, K., Schoning, I., Buscot, F., and Wubet, T. (2015). Forest management type influences diversity and community composition of soil fungi across temperate forest ecosystems. Front. Microbiol. 6, 1300. doi:10.3389/fmicb.2015.01300
Grime, J. P. (1973). Control of species density in herbaceous vegetation. J. Environ. Manag. 1, 151–167.
Hanif, M. A., Guo, Z., Moniruzzaman, M., He, D., Yu, Q., Rao, X., et al. (2019). Plant taxonomic diversity better explains soil fungal and bacterial diversity than functional diversity in restored forest ecosystems. Plants (Basel) 8 (11), 479. doi:10.3390/plants8110479
Janos, D. P. (1980). Vesicular-Arbuscular mycorrhizae affect lowland tropical rain forest plant growth. Ecology 61 (1), 151–162. doi:10.2307/1937165
Jansa, J., Finlay, R., Wallander, H., Smith, F. A., and Smith, S. E. (2011). “Role of mycorrhizal symbioses in phosphorus cycling,” in Phosphorus in action (Berlin, Heidelberg: Springer), 26, 137–168.
Jansa, J., Forczek, S. T., Rozmoš, M., Püschel, D., Bukovská, P., and Hršelová, H. (2019). Arbuscular mycorrhiza and soil organic nitrogen: Network of players and interactions. Chem. Biol. Technol. Agric. 6 (1), 10. doi:10.1186/s40538-019-0147-2
Jiao, F., Wen, Z.-M., and An, S.-S. (2011). Changes in soil properties across a chronosequence of vegetation restoration on the Loess Plateau of China. Catena 86 (2), 110–116. doi:10.1016/j.catena.2011.03.001
Johnson, D., and Gilbert, L. (2015). Interplant signalling through hyphal networks. New Phytol. 205 (4), 1448–1453. doi:10.1111/nph.13115
Kikvidze, Z., Armas, C., Fukuda, K., Martínez-García, L. B., Miyata, M., Oda-Tanaka, A., et al. (2010). The role of arbuscular mycorrhizae in primary succession: Differences and similarities across habitats. Web Ecol. 10 (1), 50–57. doi:10.5194/we-10-50-2010
Kruger, C., Kohout, P., Janouskova, M., Puschel, D., Frouz, J., and Rydlova, J. (2017). Plant communities rather than soil properties structure arbuscular mycorrhizal fungal communities along primary succession on a mine spoil. Front. Microbiol. 8, 719. doi:10.3389/fmicb.2017.00719
Leal, P. L., Siqueira, J. O., and Stürmer, S. L. (2013). Switch of tropical Amazon forest to pasture affects taxonomic composition but not species abundance and diversity of arbuscular mycorrhizal fungal community. Appl. Soil Ecol. 71, 72–80. doi:10.1016/j.apsoil.2013.05.010
Li, S., Huang, X., Shen, J., Xu, F., and Su, J. (2020). Effects of plant diversity and soil properties on soil fungal community structure with secondary succession in the Pinus yunnanensis forest. Geoderma 379, 114646. doi:10.1016/j.geoderma.2020.114646
Louca, S., Polz, M. F., Mazel, F., Albright, M. B. N., Huber, J. A., O'Connor, M. I., et al. (2018). Function and functional redundancy in microbial systems. Nat. Ecol. Evol. 2 (6), 936–943. doi:10.1038/s41559-018-0519-1
Lovelock, C. E., Andersen, K., and Morton, J. B. (2003). Arbuscular mycorrhizal communities in tropical forests are affected by host tree species and environment. Oecologia 135 (2), 268–279. doi:10.1007/s00442-002-1166-3
Lumini, E., Orgiazzi, A., Borriello, R., Bonfante, P., and Bianciotto, V. (2010). Disclosing arbuscular mycorrhizal fungal biodiversity in soil through a land-use gradient using a pyrosequencing approach. Environ. Microbiol. 12 (8), 2165–2179. doi:10.1111/j.1462-2920.2009.02099.x
Magoc, T., and Salzberg, S. L. (2011). Flash: Fast length adjustment of short reads to improve genome assemblies. Bioinformatics 27 (21), 2957–2963. doi:10.1093/bioinformatics/btr507
Mao, H., Liu, W., Yang, Q., Yao, H., Liu, G., and Yang, H. (2021). Characteristics of root biomass in the tropical lowland rain forest at different succession stages in ganshiling natural reserve, hainan Island. J. Trop. Biol. 12 (2), 176–184. doi:10.15886/j.cnki.rdswxb.2021.02.006
McGuire, K. L., Fierer, N., Bateman, C., Treseder, K. K., and Turner, B. L. (2012). Fungal community composition in neotropical rain forests: The influence of tree diversity and precipitation. Microb. Ecol. 63 (4), 804–812. doi:10.1007/s00248-011-9973-x
Nakayama, M., Imamura, S., Taniguchi, T., and Tateno, R. (2019). Does conversion from natural forest to plantation affect fungal and bacterial biodiversity, community structure, and co-occurrence networks in the organic horizon and mineral soil? For. Ecol. Manag. 446, 238–250. doi:10.1016/j.foreco.2019.05.042
Nauta, J. F., Hummel, Y. M., Tromp, J., Ouwerkerk, W., van der Meer, P., Jin, X., et al. (2020). Concentric vs. eccentric remodelling in heart failure with reduced ejection fraction: Clinical characteristics, pathophysiology and response to treatment. Eur. J. Heart Fail. 22 (7), 1147–1155. doi:10.1002/ejhf.1632
Opik, M., Vanatoa, A., Vanatoa, E., Moora, M., Davison, J., Kalwij, J. M., et al. (2010). The online database MaarjAM reveals global and ecosystemic distribution patterns in arbuscular mycorrhizal fungi (Glomeromycota). New Phytol. 188 (1), 223–241. doi:10.1111/j.1469-8137.2010.03334.x
Parihar, M., Rakshit, A., Meena, V. S., Gupta, V. K., Rana, K., Choudhary, M., et al. (2020). The potential of arbuscular mycorrhizal fungi in C cycling: A review. Archives Microbiol. 202 (7), 1581–1596. doi:10.1007/s00203-020-01915-x
Peng, X., Tamura, K., Asano, M., Takano, A., Kawagoe, M., and Kamijo, T. (2021). Changes in soil physical and chemical properties during vegetation succession on miyake-jima Island. Forests 12 (11), 1435. doi:10.3390/f12111435
Philippot, L., Raaijmakers, J. M., Lemanceau, P., and van der Putten, W. H. (2013). Going back to the roots: The microbial ecology of the rhizosphere. Nat. Rev. Microbiol. 11 (11), 789–799. doi:10.1038/nrmicro3109
Phillips, R. P., Brzostek, E., and Midgley, M. G. (2013). The mycorrhizal-associated nutrient economy: A new framework for predicting carbon-nutrient couplings in temperate forests. New Phytol. 199 (1), 41–51. doi:10.1111/nph.12221
Qi, L., Liang, C., Mao, C., Qin, X., Fan, S., Du, W., et al. (2014). Species composition and geographic elements of the tropical lowland secondary rain forest of Ganshiling, Hainan Island, China. Chin. J. Ecol. 33 (4), 922–929. doi:10.13292/j.1000-4890.2014.0091
Reyes, H. A., Ferreira, P. F. A., Silva, L. C., da Costa, M. G., Nobre, C. P., and Gehring, C. (2019). Arbuscular mycorrhizal fungi along secondary forest succession at the eastern periphery of Amazonia: Seasonal variability and impacts of soil fertility. Appl. Soil Ecol. 136, 1–10. doi:10.1016/j.apsoil.2018.12.013
Schloss, P. D., Westcott, S. L., Ryabin, T., Hall, J. R., Hartmann, M., Hollister, E. B., et al. (2009). Introducing mothur: Open-source, platform-independent, community-supported software for describing and comparing microbial communities. Appl. Environ. Microbiol. 75 (23), 7537–7541. doi:10.1128/aem.01541-09
Segata, N., Izard, J., Waldron, L., Gevers, D., Miropolsky, L., Garrett, W. S., et al. (2011). Metagenomic biomarker discovery and explanation. Genome Biol. 12 (6), R60. doi:10.1186/gb-2011-12-6-r60
Shi, N.-N., Gao, C., Zheng, Y., and Guo, L.-D. (2016). Arbuscular mycorrhizal fungus identity and diversity influence subtropical tree competition. Fungal Ecol. 20, 115–123. doi:10.1016/j.funeco.2015.12.007
Sirova, D., Barta, J., Simek, K., Posch, T., Pech, J., Stone, J., et al. (2018). Hunters or farmers? Microbiome characteristics help elucidate the diet composition in an aquatic carnivorous plant. Microbiome 6 (1), 225. doi:10.1186/s40168-018-0600-7
Soteras, F., Grilli, G., Cofre, M. N., Marro, N., and Becerra, A. (2015). Arbuscular mycorrhizal fungal composition in high montane forests with different disturbance histories in central Argentina. Appl. Soil Ecol. 85, 30–37. doi:10.1016/j.apsoil.2014.09.004
Tamminen, M., Betz, A., Pereira, A. L., Thali, M., Matthews, B., Suter, M. J., et al. (2018). Proteome evolution under non-substitutable resource limitation. Nat. Commun. 9 (1), 4650. doi:10.1038/s41467-018-07106-z
Taylor, M. D. (2000). Determination of total phosphorus in soil using simple Kjeldahl digestion. Commun. Soil Sci. Plant Analysis 31, 2665–2670. doi:10.1080/00103620009370616
van der Heijden, M. G. A., and Horton, T. R. (2009). Socialism in soil? The importance of mycorrhizal fungal networks for facilitation in natural ecosystems. J. Ecol. 97 (6), 1139–1150. doi:10.1111/j.1365-2745.2009.01570.x
van der Heijden, M. G., Bardgett, R. D., and van Straalen, N. M. (2008). The unseen majority: Soil microbes as drivers of plant diversity and productivity in terrestrial ecosystems. Ecol. Lett. 11 (3), 296–310. doi:10.1111/j.1461-0248.2007.01139.x
Varela-Cervero, S., Vasar, M., Davison, J., Barea, J. M., Opik, M., and Azcon-Aguilar, C. (2015). The composition of arbuscular mycorrhizal fungal communities differs among the roots, spores and extraradical mycelia associated with five Mediterranean plant species. Environ. Microbiol. 17 (8), 2882–2895. doi:10.1111/1462-2920.12810
Walker, L. R., and del Moral, R. (2003). Primary succession and ecosystem rehabilitation. Cambridge, UK: Cambridge University Press.
Wan, X., Huang, Z., He, Z., Yu, Z., Wang, M., Davis, M. R., et al. (2014). Soil C:N ratio is the major determinant of soil microbial community structure in subtropical coniferous and broadleaf forest plantations. Plant Soil 387 (1-2), 103–116. doi:10.1007/s11104-014-2277-4
Wang, X.-Y., Ge, Y., and Wang, J. (2017). Positive effects of plant diversity on soil microbial biomass and activity are associated with more root biomass production. J. Plant Interact. 12 (1), 533–541. doi:10.1080/17429145.2017.1400123
Winagraski, E., Kaschuk, G., Monteiro, P. H. R., Auer, C. G., and Higa, A. R. (2019). Diversity of arbuscular mycorrhizal fungi in forest ecosystems of Brazil: A review. Cerne 25 (1), 25–35. doi:10.1590/01047760201925012592
Xu, T., Veresoglou, S. D., Chen, Y., Rillig, M. C., Xiang, D., Ondrej, D., et al. (2016). Plant community, geographic distance and abiotic factors play different roles in predicting AMF biogeography at the regional scale in northern China. Environ. Microbiol. Rep. 8 (6), 1048–1057. doi:10.1111/1758-2229.12485
Xu, X., Shi, Z., Li, D., Rey, A., Ruan, H., Craine, J. M., et al. (2016). Soil properties control decomposition of soil organic carbon: Results from data-assimilation analysis. Geoderma 262, 235–242. doi:10.1016/j.geoderma.2015.08.038
Zhang, Z., Schwartz, S., Wagner, L., and Miller, W. (2000). A greedy algorithm for aligning DNA sequences. J. Comput. Biol. 7 (1-2), 203–214. doi:10.1089/10665270050081478
Keywords: arbuscular mycorrhizal fungi (AMF), microbial community dynamic, root biomass, secondary succession, tropical forest ecosystems
Citation: Mai S, Mao H, Jiang Y, Huang T, Yang Q, Xing G, Wang X, Yang H and Liu W (2023) Characteristics of the soil arbuscular mycorrhizal fungal community along succession stages in tropical forest and its driving factors. Front. Environ. Sci. 10:1110655. doi: 10.3389/fenvs.2022.1110655
Received: 29 November 2022; Accepted: 29 December 2022;
Published: 11 January 2023.
Edited by:
Xiang Liu, Lanzhou University, ChinaReviewed by:
Xinwei Guo, Chinese Academy of Tropical Agricultural Sciences, ChinaJie Chen, Chinese Academy of Forestry, China
Copyright © 2023 Mai, Mao, Jiang, Huang, Yang, Xing, Wang, Yang and Liu. This is an open-access article distributed under the terms of the Creative Commons Attribution License (CC BY). The use, distribution or reproduction in other forums is permitted, provided the original author(s) and the copyright owner(s) are credited and that the original publication in this journal is cited, in accordance with accepted academic practice. No use, distribution or reproduction is permitted which does not comply with these terms.
*Correspondence: Huai Yang, eWFuZ2h1YWlAaWNici5hYy5jbg==; Wenjie Liu, bGl1d2pAaGFpbmFudS5lZHUuY24=
†These authors contributed equally to this work and share first authorship