- Department of Crop Science, College of Agricultural Sciences, São Paulo State University (UNESP), Botucatu, Brazil
Thermomagnesium (TM), a byproduct of Ni ore mining, can be processed as a clean alternative to conventional fertilizers as a source of magnesium (Mg) and silicon (Si) for agriculture. TM positively impacts soil properties and provides nutrients that are available for uptake by plants; however, information on the effects of TM on plant physiology in cropping systems is limited. This study aimed to evaluate the impact of increasing doses of TM on crop yield; soil chemical attributes; and leaf contents of Mg, Si, reducing sugars, sucrose, and starch in a soybean-maize crop rotation system. The study was performed under rainfed conditions during three consecutive crop seasons in 2018/2019 (soybean), 2019 (maize), and 2019/2020 (soybean). Six TM doses (0, 350, 700, 1050, 1400, 1750 kg ha−1) with four replicates were applied prior to the first season. Responses to the application of TM were observed up to the highest doses (1,400 and 1750 kg ha−1), with increases in soil concentrations of Mg and Si, soil pH, leaf pigments, gas exchange parameters, and carbohydrate concentrations but decreases in starch content. The increases in photosynthetic rates and carbohydrate partitioning led to increases in the weight of 100 grains (W100G) and grain yield (GY). W100G increased by 11% in soybean at a TM dose of 1,050 kg ha−1 and 23% in maize at a TM dose of 1,400 kg ha−1 dose. For both crops, the greatest increases in GY were obtained at a TM dose of 1,050 kg ha−1, with increases of 1,068 and 3,658 kg ha−1 for soybean and maize, respectively, compared with the control. Therefore, TM can be used in agricultural systems as a viable source of Mg and Si and as soil acidity amendment to promote sustainable agriculture.
Introduction
The use of nickel (Ni) in batteries and catalysts has greatly increased waste generation from Ni ore mining and led to environmental contamination. One strategy for reducing the negative environmental and economic impacts of the mining industry is to process the byproduct of Ni ore mining for use as a clean alternative to conventional fertilizers in agriculture (Dalmora et al., 2020). Studies have shown that byproducts with a low negative impact on soil can be environmental liabilities when used to supply nutrients (Dalmora et al., 2020; Moretti et al., 2020b), but information on the effects of such byproducts on plant physiology in cropping systems is limited.
Thermomagnesium (TM, MgSiO3) is a byproduct produced via a thermic process of Ni extraction and is a potential source of magnesium (Mg) and silicon (Si) for crops. Mg is a macronutrient for crop growth due to its functions in photosynthesis, enzyme activation, and nucleic acid and protein synthesis (Marschner, 2012). Limiting levels of Mg greatly inhibits crop development and yields by decreasing photosynthetic carbon metabolism and CO2 fixation, which leads to reduced transport and accumulation of carbohydrates in leaves (Farhat et al., 2016; Tränkner and Jaghdani, 2019). Mg also plays key roles in carbon partitioning, starch synthesis, triose phosphate transport, and sucrose production (Ceylan et al., 2016), and thus adequate Mg levels enhance the physiological efficiency of crops (Chen et al., 2018).
In contrast to Mg, Si is nonessential for plants but enhances resistance to insects and diseases (Ma and Yamaji, 2006). Si is an environmentally friendly element that improves water-use efficiency (Gao et al., 2004), photosynthesis (Barbosa et al., 2015), and plant metabolism, mainly in Si-accumulating plant species (Farooq et al., 2019; Kumaraswamy et al., 2021). In metal-contaminated soils, Si boosts the activities of non-enzymatic antioxidants, such as glutathione, non-protein thiols, and ascorbic acid (Adrees et al., 2015; Hussain et al., 2021). Thus, Si availability can alleviate the effects of abiotic and biotic stresses in plants, especially under drought (Moradtalab et al., 2018; Ning et al., 2020) and Mg-deficient conditions (Hosseini et al., 2019) such as those found in the Brazilian Cerrado and African Savanna.
In tropical regions, extensive areas are cultivated with soybean-maize crop rotation systems, which require adequate nutrient supplies (Castro and Crusciol, 2013). Recent studies have shown that adequate supplies of Mg and Si can increase crop yields (Hosseini et al., 2019; Li et al., 2020; Wang et al., 2020) and primary results of TM benefits on crop nutrition tested under controlled climate conditions have also indicated the usage possibility (Crusciol et al., 2019; Moretti et al., 2020c; Bossolani et al., 2021b). Nevertheless, information about TM effectiveness as a source of Mg and Si, its residual effect, and appropriate dosages under field conditions in soybean-maize rotation systems, is limited. Accordingly, we hypothesized that the direct or residual effect of TM application can enhance soil pH condition, crop nutrition, metabolism, and yields, due to the soil acidity amendment capacity of TM, and the effect of Mg and Si on plant physiology, gas exchange, and carbohydrate transport. To test these hypotheses, the effects of applying a range of TM doses in a soybean-maize crop rotation system on Mg and Si concentrations in the soil and leaves, soil pH, photosynthetic pigment concentrations, gas exchange parameters, and soybean and maize yields were evaluated.
Materials and Methods
Thermomagnesium Processing
TM was produced by melting Ni ore in the presence of water in an electric furnace, which granulates nonmetallic elements such as silicon dioxide (SiO2), magnesium oxide (MgO), and ferric oxide (Fe2O3) (Moretti et al., 2020b). The ore was then crushed to alter its granulometry, followed by calcination in a rotary kiln to remove all moisture, including chemically bound water. The calcined ore was subsequently charged in an electric furnace and reduced at a high temperature, which removed O from Fe and Ni oxides to produce an iron-nickel alloy (Fe–Ni) containing approximately 20% Ni, as well as a large amount of byproduct (Moretti et al., 2021). The byproduct was immediately cooled from 1,600°C to room temperature under pressure using several water jets. The solidified MgSiO3-rich byproduct did not exhibit granulometric uniformity and thus underwent a forming process. First, the byproduct was dried in a rotary dryer at a beneficiation plant. After drying, a uniform particle size distribution (100 mesh size, ∼150 µm) was obtained by sieving, and the mineralogical phases of the byproduct were determined by X-ray diffraction (XRD) in a Philips X-ray diffractometer (Moretti et al., 2021). The crystalline phases were identified by comparing the sample to the PDF2 Database of the International Center for Diffraction Data and the Inorganic Crystal Structure Database (ICSD; Figure 1). The values of the crystalline phases were calculated following the method of Rietveld using the standard ICSD crystalline structures and internal fluorite (CaF2) to determine the amorphous phase. According to the maximum limits of potentially toxic elements [PTEs; arsenic (As), cadmium (Cd), chromium (Cr), lead (Pb), nickel (Ni), mercury (Hg), and selenium (Se)] in soil remineralizers allowed by the Brazilian National Environmental Council, as established by Annexe I of the Normative Instruction SDA n. 27 (Brazil, 2008), TM does not represent an environmental risk and can be safely reused in agriculture.
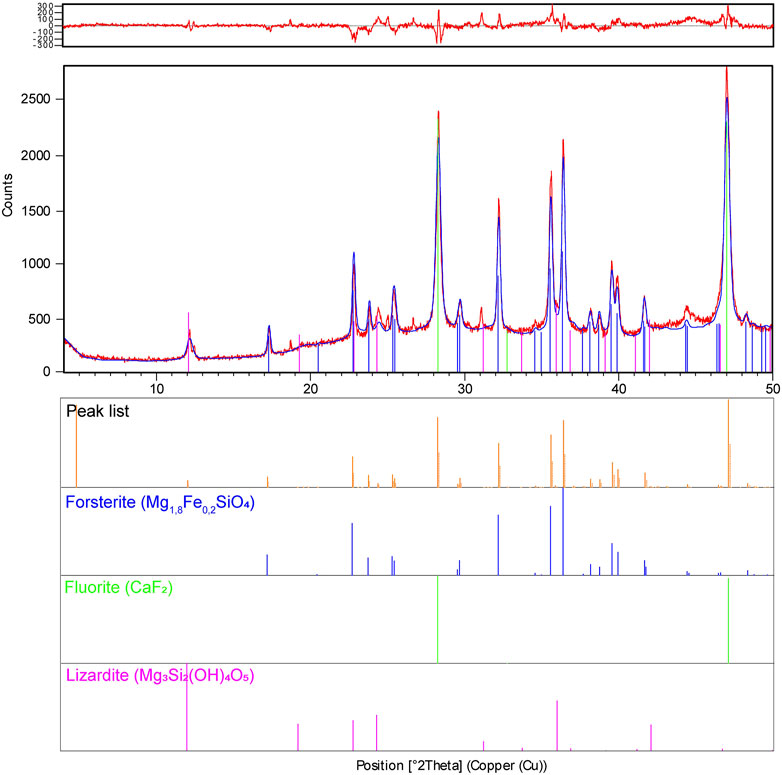
FIGURE 1. X-ray diffractogram for quantifying the mineralogical phases of Thermomagnesium from Ni ore mining. To calculate the amorphous material, 10% fluorite (ICDD 01-077-2093) was added.
Field Description and Experimental Design
The field experiment was conducted under rainfed conditions in Botucatu, São Paulo State, southeastern Brazil (48°25′39″ W, 22°49′51″ S, 790 m above sea level), during three consecutive crop seasons (growing season 2018/19 = soybean; off-season 2019 = maize; growing season 2019/20 = soybean) (Figure 2). The soil is classified as a Rhodic Hapludox (Soil Survey Staff, 2014), with 353, 169, and 478 g kg−1 of clay, silt, and sand, respectively. The climate according to the Köppen-Geiger climate classification system is Cwa, which corresponds to a humid subtropical zone with dry winters and hot summers (Alvares et al., 2013). The long-term (50-year average) annual maximum and minimum temperatures in this region are 26.1 and 15.3°C, respectively, and the average rainfall, maximum and minimum temperature in the experiment period is presented in Supplementary Figure S1.
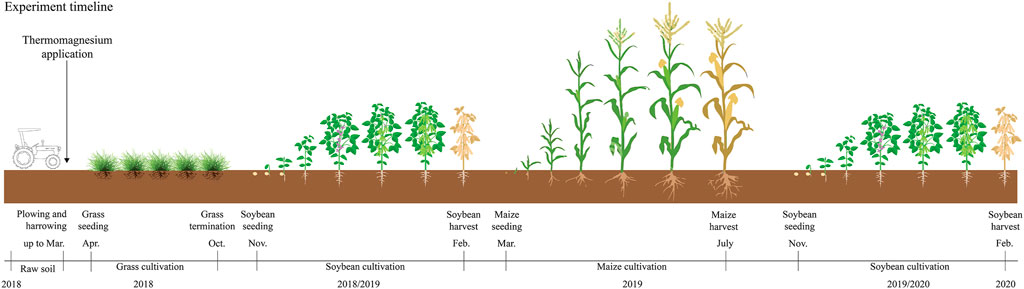
FIGURE 2. Schematic graphic representing the field experiment timeline and treatment application in the growing seasons.
Prior to the experiment, soil chemical characterization (0.0–0.2 m) was performed according to (Raij et al., 2001). The soil chemical characteristics were as follows: pH (0.01 mol L−1 CaCl2), 4.0; soil organic matter (SOM), 22 g kg−1; Presin, 28 mg kg−1; H + Al, 40 mmolc kg−1; exchangeable K, Ca and Mg, 1.5, 14, and 8 mmolc kg−1, respectively; S-SO42−, 3 mg kg−1; cation exchange capacity (CEC), 63,5 mmolc kg−1; and base saturation (BS), 38%.
The experimental design was a randomized complete block with six treatments and four replicates. The treatments comprised six TM doses: 0, 350 (SiO2, 168 and MgO, 98 kg ha−1), 700 (SiO2, 336 and MgO, 196 kg ha−1), 1,050 (SiO2, 504 and MgO, 294 kg ha−1), 1,400 (SiO2, 672 and MgO, 392 kg ha−1) and 1750 kg ha−1 (SiO2, 840 and MgO, 490 kg ha−1).
Crop Management Practices History
Before establishing the experiment, liming [2000 kg ha−1 as calcitic lime (530 g kg−1 of calcium oxide, CaO; 50 g kg−1 of magnesium oxide, MgO; and 78% calcium carbonate equivalents, %ECaCO3)] was performed to increase the base saturation (BS) of the topsoil (0.00–0.20-m depth) to 60% as proposed by (Cantarella et al., 1998). Soil amendments were incorporated via conventional tillage (one disk plow with a working depth of 200 mm and two leveling harrows with a working depth of 100 mm). The specified doses of lime and TM were applied simultaneously to the soil before tilling. Before the experiment, palisade grass (Urochloa brizantha cv. Marandu) was sown without fertilizer at 2.8 kg ha−1 of viable seeds. The cover crop was grown for approximately 8 months, from April to November 2018 (off-season), to provide straw for the cultivation of the subsequent crop (4 t ha−1 of dry matter on the soil surface).
Soybean (cultivar CD2728 IPRO; 300,000 plants ha−1; CODETEC®; Cascavel, Brazil) was sown under no-till. Each plot consisted of 10 rows spaced 0.45 m apart, with a total plot area of 45 m2. The soybean plants were fertilized with 300 kg ha−1 of 00–20–20 (60 kg ha−1 of P2O5 and 60 kg ha−1 of K2O) in both growing seasons (2018/19 and 2019/20). The seeds were inoculated 1 h before sowing by evenly coating with an appropriate amount of inoculant of a bacterial consortium of Bradyrhizobium japonicum (strain SEMIA 5079) and Bradyrhizobium diazoefficiens (strain SEMIA 5080) according to (Moretti et al., 2020a). Weeds, insects, and diseases were managed according to the recommendations of (EMBRAPA, 2020).
Maize (hybrid P3707VYH; 60,000 plants ha−1; DuPont Pioneer®, Johnston, IA, United States) was sown under no-till in the same plots in March 2019. Base fertilization was performed with 300 kg ha−1 of 08–28–16 (24 kg ha−1 of N, 84 kg ha−1 of P2O5, and 48 kg ha−1 of K2O). At the V5 maize phenological stage (Ritchie et al., 1993), N–K fertilizers were broadcast over the soil surface at 100 kg N ha−1 as ammonium sulfate and 20 kg K2O ha−1 as potassium chloride. Phytosanitary treatments were applied according to the needs of the maize.
Crop Nutrition
Soybean plant nutritional status was evaluated at the R2 phenological stage (Fehr and Caviness, 1977) (full bloom) by collecting 20 leaves of the third node from the top of each plot (Ambrosano et al., 1997). Maize nutritional status was analyzed at the R1 phenological stage (Ritchie et al., 1993) (silking) by collecting the middle third of 20 ear leaves in each plot (Cantarella et al., 1997) and determined for Si (Korndörfer et al., 2004), nitrogen (N), phosphorus (P), potassium (K), calcium (Ca), Mg, sulfur (S), copper (Cu), iron (Fe), zinc (Zn), manganese (Mn), and boron (B) (Malavolta et al., 1997).
Photosynthetic Pigments
Chlorophyll a, chlorophyll b, total chlorophyll, and carotenoids concentrations were quantified in both growing seasons in fresh diagnostic leaves collected at the same time as the leaves collected for nutritional analysis. Plant tissues were stored in N,N-dimethylformamide (DMF) and analyzed using a spectrophotometric method (Malavolta et al., 1997).
Gas Exchange Parameters
Gas exchange measurements were performed using a model CIRAS-3 portable gas exchange device (PP Systems Inc., Amesbury, MA, United States). The following parameters were measured in the same leaf samples used for physiological and nutritional analysis: net photosynthetic rate (A, μmol CO2 m−2s−1), stomatal conductance (gs, mol H2O m−2s−1), internal CO2 (Ci, μmol mol−1) and transpiration (E, mmol H2O m−2s−1). In addition, water use efficiency [WUE; µmol CO2 (mmol H2O)−1], calculated by the A/E ratio, and carboxylation efficiency (dimensionless), calculated by the A/Ci ratio, were assessed. The system was calibrated with the following parameters: 380–400 mol−1 atmospheric CO2, 1,100 μmol quanta m−2s−1 of photosynthetically active radiation (PAR) supplied by LED lamps, 25–27°C leaf chamber temperature, and 60–70% relative humidity. Measurements were carried out under natural conditions on clear days between 10:00 a.m. and 12:00 p.m. with 12 readings per treatment (4 readings in each plot).
Carbohydrate Partitioning
Sugar fractionation by high-performance liquid chromatography (HPLC) was performed at the same time as the physiological and nutritional analyses. Sucrose and reducing sugars were calculated by referring to the peak areas of standards and multiplying by the dilution factor (Garcia et al., 2020). The starch concentration was determined by measuring the absorbance at 535 nm in a spectrophotometer (Nelson, 1944).
Agronomic Parameters and Grain Yield
In all growing seasons, plants were harvested at physiological maturity from a 16-m2 area in the center of each plot. Grain yield (kg ha−1) and hundred-grain weight (W100G) were converted to values on a dry weight basis by correcting for 13% moisture. Moisture content was determined using an automatic moisture meter (Gehaka G650i, Brazil).
Soil Chemical Analysis
After the second soybean harvest, which corresponded to 24 months after TM application, four soil subsamples from the 0.00–0.20 m layer were randomly collected from the useful area of each plot. For each plot, the subsamples were pooled, dried, sieved (2 mm), and analyzed for pH (0.01 mol L−1 CaCl2), Mg2+ (Raij et al., 2001), and Si (Korndörfer et al., 2004). The soil samples were also submitted for chromium (Cr) analysis to certify the absence of any concentration above the prevention value (75 mg kg−1) established by federal regulations (CETESB, 2005). The soil concentration of total Cr did not differ between treatments, and the average value was 28.2 mg kg−1, below the threshold for contamination. No trace concentrations of hexavalent chromium (Cr VI) were detected.
Statistical Analysis
Means were subjected to the outliers test followed by the Anderson-Darling normality test, and homogeneity was evaluated with Levene’s test. The means were subsequently subjected to individual analysis of variance (ANOVA) by the F test (p ≤ 0.05) and, when significant, analyzed using the modified t test [Fisher’s protected least significant difference (LSD) at p ≤ 0.05]. Heatmap analysis was performed by calculating the Pearson correlation coefficients (p ≤ 0.05), and only significant correlations are shown. Hierarchical clustering was constructed based on Gower’s distance with 1,000 bootstraps.
Results
Soil pH, Exchangeable Mg (Mg2+), and Si Availability in Soil and Leaf Concentrations of Mg and Si
Soil Mg2+ and Si concentrations increased as increasing TM doses (Figures 3A,B). The largest TM dose (1750 kg ha−1) increased the soil concentrations of Mg2+ and Si by 18 and 3.7 mmolc kg−1, respectively, compared with the control. In general, the leaf concentrations of Mg and Si in soybean (1st and 2nd growing seasons) and maize increased with the TM dose up to 700 kg ha−1 (Figures 3C,D). Soil pH varied between treatments and increased by approximately 1.2 unities up to 1,050 kg ha−1 of TM compared with the control (Figure 3E).
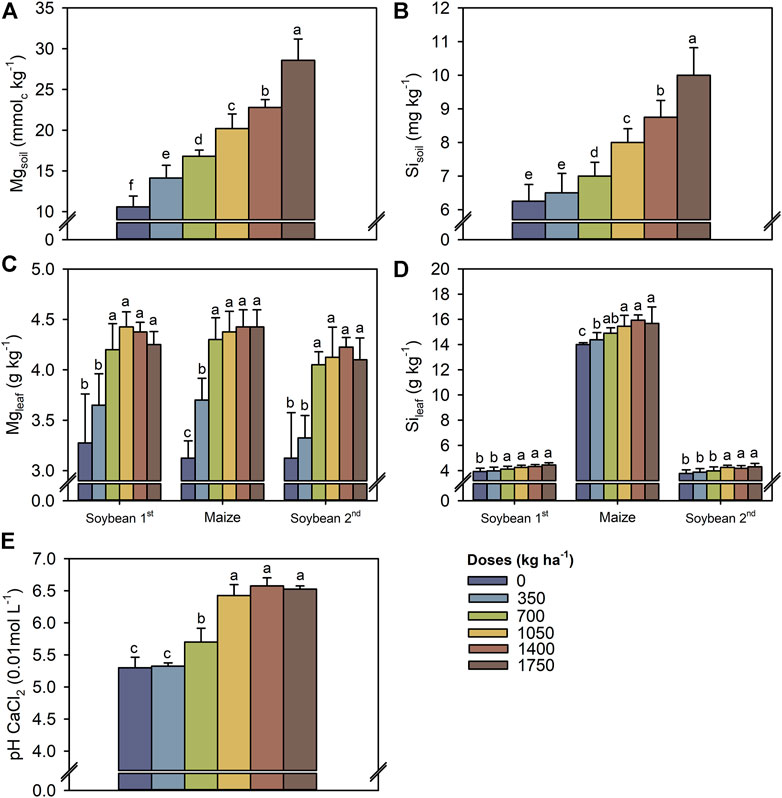
FIGURE 3. Exchangeable Mg2+ (A) and Si availability (B) in soil (0.0–0.2 m), leaf concentrations of Mg (C) and Si (D) in first soybean growing season, maize off-season and second soybean growing season, and soil pH (0.0–0.2 m) (E) as a function of the Thermomagnesium doses (kg ha−1). Different lower-case letters for each crop season indicate significant differences between treatments by Student’s t-test at p ≤ 0.05. The error bars express the standard error of the mean (n = 4).
Leaf Pigments, Gas Exchange Parameters, and Leaf Sugar Fractions
The most significant increases in the concentrations of chlorophyll a, chlorophyll b, total chlorophyll, and carotenoids occurred at a TM dose of 1,400 kg ha−1 (Figures 4A–D). At this dose, the contents of chlorophyll a, chlorophyll b, total chlorophyll, and carotenoids increased by averages of 15, 35, 19, and 28%, respectively, compared with the control. Similar to the leaf pigments, A and gs values were highest in both crops at a TM dose of 1,400 kg ha−1 (Figures 5A,B). Conversely, Ci and E values decreased in both crops at a TM dose of 1,050 kg ha−1 compared with a dose of 700 kg ha−1 (Figures 5C,D). As a result of increasing A and reducing Ci and E, the A/Ci index and WUE were increased up to 1,400 kg ha−1 for both crops (Figures 5E,F).
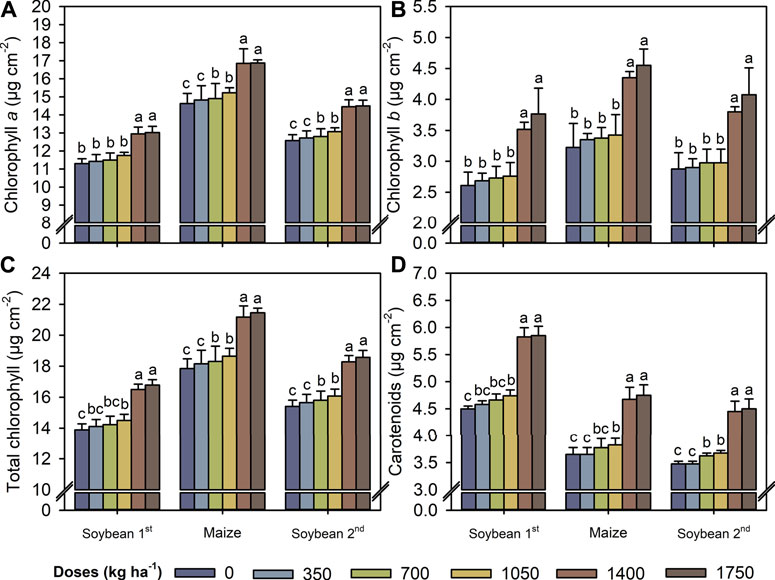
FIGURE 4. Chlorophyll a (A), chlorophyll b (B), total chlorophyll (C), and total carotenoids (D) contents in leaves of first soybean growing season, maize off-season, and second soybean growing season as a function of the Thermomagnesium doses (kg ha−1). Different lower-case letters for each crop season indicate significant differences between treatments by Student’s t-test at p ≤ 0.05. The error bars express the standard error of the mean (n = 4).
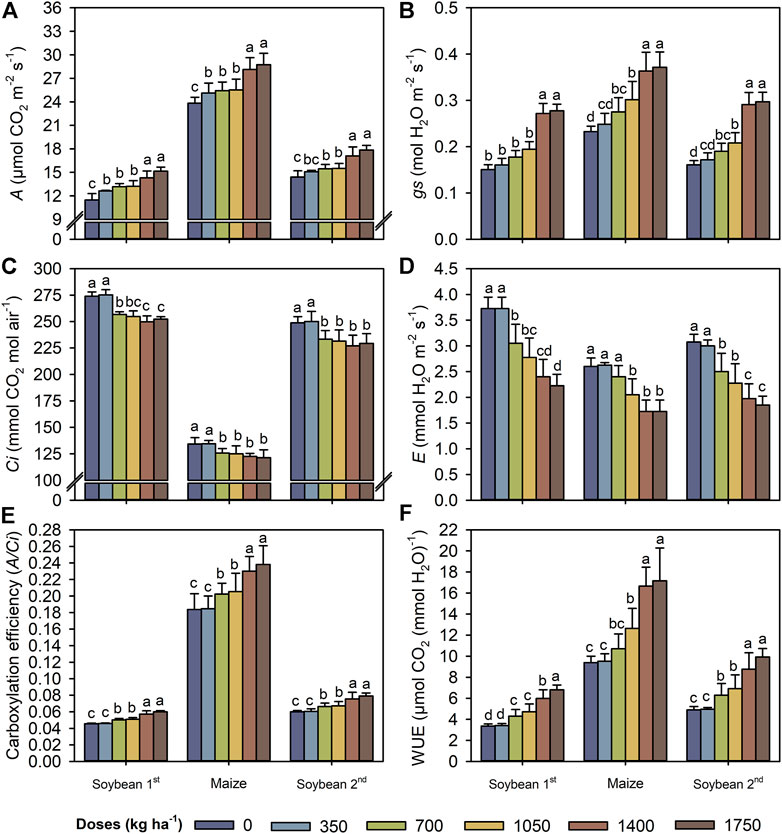
FIGURE 5. A—Net photosynthetic rate (A), gs - stomatal conductance (B), Ci—internal CO2 concentration in the substomatal chamber (C), E—transpiration (D), carboxylation efficiency (E), and WUE–water use efficiency (F) in leaves of first soybean growing season, maize off-season and second soybean growing season as a function of the Thermomagnesium doses (kg ha−1). Different lower-case letters for each crop season indicate significant differences between treatments by Student’s t-test at p ≤ 0.05. The error bars express the standard error of the mean (n = 4).
In both crops and growing seasons, the concentrations of RS and sucrose tended to increase at a TM dose of 1,050 kg ha−1, whereas the concentration of starch was reduced by increasing TM doses, especially by 1750 kg ha−1. Compared with the control, the average RS and sucrose concentrations increased by 40 and 30%, respectively, whereas the starch concentration decreased by 10% (Figures 6A–C).
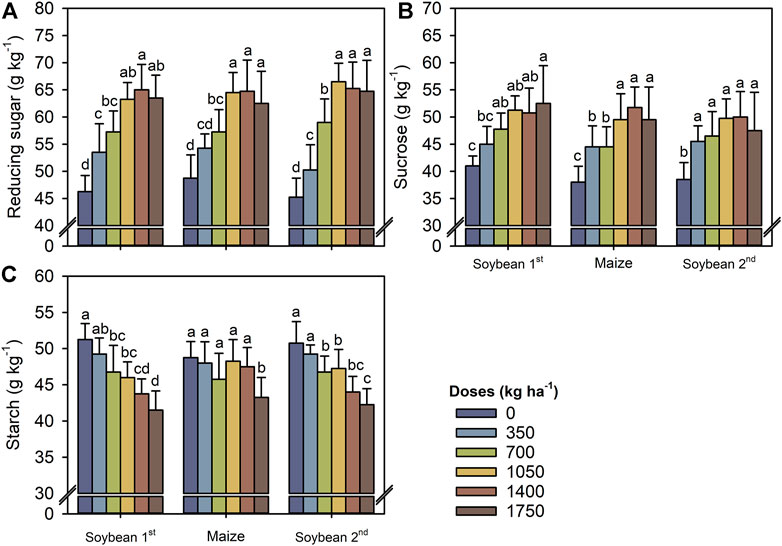
FIGURE 6. Reducing sugars (A), sucrose (B), and starch (C) in leaves of first soybean growing season, maize off-season, and second soybean growing season as a function of the Thermomagnesium doses (kg ha−1). Different lower-case letters for each crop season indicate significant differences between treatments by Student’s t-test at p ≤ 0.05. The error bars express the standard error of the mean (n = 4).
Yield Parameters
Compared with the control, W100G increased by 11% for soybean (average of the two growing seasons) at a TM dose of 1,050 kg ha−1 and 23% for maize at a TM dose of 1,400 kg ha−1 (Figure 7A). Similarly, TM application increased the GY of both crops up to a dose of 1,050 kg ha−1 (Figure 7B). At a TM dose of 1,050 kg ha−1, the GY of soybean (average of the two growing seasons) and maize increased by 1,070 and 3,660 kg ha−1, respectively, compared with the control.
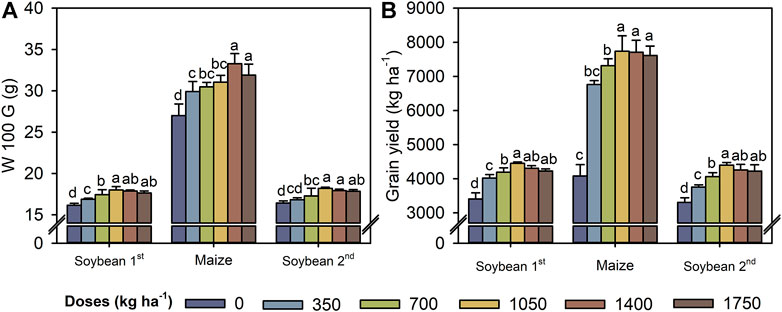
FIGURE 7. W100G - Hundred-grain weight (A), and grain yield (B) in the first soybean growing season, maize off-season, and second soybean growing season as a function of the Thermomagnesium doses (kg ha−1). Different lower-case letters for each crop season indicate significant differences between treatments by Student’s t-test at p ≤ 0.05. The error bars express the standard error of the mean (n = 4).
Pearson’s Correlation Analysis and Hierarchical Clustering of Treatments
The W100G and GY were positively correlated with the concentrations of Mg and Si in soil and leaves; soil pH; the concentrations of chlorophyll a, chlorophyll b, and total chlorophylls; A; gs; A/Ci and WUE; and concentrations of reducing sugars and sucrose. By contrast, W100G and GY were negatively correlated with the Ci and E. No correlations of starch and carotenoid concentrations with W100G and GY were observed (Figure 8A). According to the soil and leaf parameters, hierarchical clustering analysis distinguished three groups based on their similarities: group 1 comprised the treatments with 0 and 350 kg ha−1 of TM; group 2 comprised the treatments with 750 and 1,050 kg ha−1 of TM; and group 3 comprised the treatments with 1,400 and 1750 kg ha−1 of TM (Figure 8B).
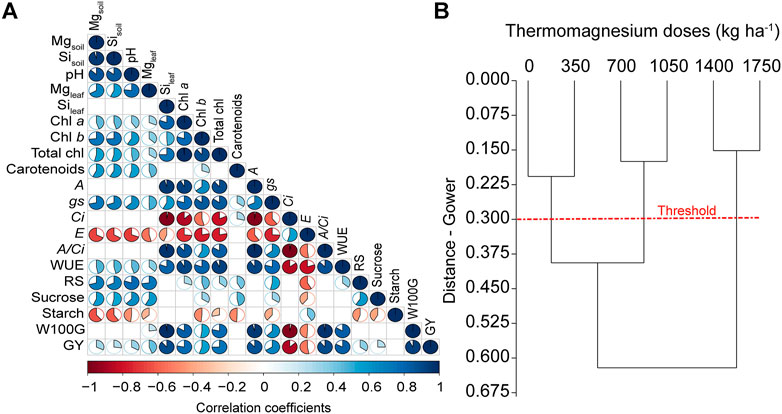
FIGURE 8. Heatmap of the Pearson correlation coefficients matrix (A) between soil, nutritional, physiological, biochemical, and agronomic parameters. Only significant correlations at p ≤ 0.05 are shown. Magnesium (Mg) and silicon (Si) in soil and leaves, soil pH, chlorophyll a (Chl a), chlorophyll b (Chl b), total chlorophyll (Total chl), carotenoids, net photosynthesis rate (A), stomatal conductance (gs), internal CO2 concentration in the substomatal chamber (Ci), transpiration (E), carboxylation efficiency (A/Ci), water use efficiency (WUE), reducing sugar (RS), sucrose, starch, hundred-grain weight (W100G) and grain yield (GY). Hierarchical clustering (B) was constructed based on Gower distances of similarity with 1,000 bootstraps.
Discussion
In the soil, the concentrations of Mg2+ and Si were weakly correlated with the yield parameters. Considering the most adequate dose of TM (1,050 kg ha−1), the critical levels of Mg2+ and Si in the soil were 20 mmolc Kg−1 and 8 mg kg−1, respectively. According to the critical level (>8mmolc Kg−1) proposed by the soil fertility recommendation bulletin of São Paulo State (Quaggio and van Raij, 1997), all treatments presented an adequate concentration of Mg2+. In the leaves, the critical level of Mg concentration was 4.4 g kg−1 for both crops, which is within the adequate ranges proposed for soybean (3.0–10 mg kg−1) and maize (1.5–5.0 mg kg−1) (Ambrosano et al., 1997; Cantarella et al., 1997). For Si, the critical levels were 4.2 g kg−1 in soybean (average of two growing seasons) and 15.5 g kg−1 in maize. Although an adequate official rate has not yet been established for leaf concentration of Si, all treatments were above the concentration range found in the literature, which is 1.0–3.5 g kg−1 in soybean, and 2.5–11.5 g kg−1 in maize (Korndörfer et al., 2004) and suggested well-nourished plants of Si, even in the control. The soil acidity was equally controlled by lime application prior to the experiment, however, after the subsequent cultivations, the soil pH decreased in the treatments under the lowest doses of TM and in the control. This result is attributed to the corrective nature and buffer capacity of TM due to its chemical composition and highlighted the residual effect of TM under field conditions. In contact with the soil moisture, MgO and SiO32− dissociates and both components are considered effective soil acidity amendments (Castro and Crusciol, 2013). Although soil pH can influence the nutrient dynamics, the acidity was not excessively high to the point of making the crop growing unfeasible, even in the control. According to the soil fertility recommendation bulletin of São Paulo State (Quaggio and van Raij, 1997), the soil acidity was classified as moderate (5.0–5.5) to slight (>5.5) with values ranging from 5.4 to 6.7. This range of soil pH can enhance the availabilities of K, Ca, and Mg by increasing the effective cation exchange capacity (CECe) and reducing their losses by leaching (Dinkecha and Tsegaye, 2017). Also, the concentration of Al3+ is practically null under soil pH (CaCl2) above approximately 5.0 and the predominant Al chemical species in this pH range are minor or non-toxic to plants (Antonangelo et al., 2017; Martins et al., 2020; de Campos et al., 2022). Considering the yield assessments, for both crops, the soil pH was positively correlated with GY and the value of 6.5 was the most adequate in this study.
The enhancements of soil chemical conditions evidenced by TM application initiated a cascade of physiological effects from the leaf photosynthetic pigments to the photosynthetic efficiency, carbohydrate synthesis, and positive yield responses of both crops. Several studies evidenced the positive effect of suitable soil chemical conditions on root growth and higher soil exploration, which alleviates water and nutrients limitation, especially in highly weathered acidic soils (Caires et al., 2015; Auler et al., 2019; Bossolani et al., 2021a). Although the leaf concentrations of Mg and Si were within the proposed ranges for both crops and all treatments, the two highest doses of TM had a direct effect on the biosynthesis of chlorophyll a, chlorophyll b, total chlorophyll, and carotenoids. The fundamental role of Mg as the central atom of the chlorophyll molecule and by activating carboxylation enzymes and carbohydrate metabolism (Marschner, 2012) can support this result, especially in agricultural systems under field conditions. Furthermore, Si can also induce the biosynthesis of photosynthetic pigments (Barbosa et al., 2015). The increases in the photosynthetic pigments, especially at the two highest TM doses (1,400 and 1750 kg ha−1), were positively correlated with photosynthetic parameters, indicating higher efficiency of the pathway from light absorption to electron excitation and carbohydrate synthesis.
Improvements in the photosynthetic apparatus were directly reflected in the results of the gas exchange analysis, which were mainly evidenced by the greater values A and gs, and the lower values Ci and E. The A/Ci index is an indicator of the diffusion and immobilization of CO2, and the A/E indicates the WUE (Lawson and Flexas, 2020). In summary, the higher the A and the lower the Ci and E, the higher the efficiencies of carboxylation (transforming CO2 into carbon chains) and WUE (losing less water during the gas exchange process). The results of gas exchange analysis were also highly positive (A, gs, A/Ci, and WUE) and negative (Ci and E) correlated with W100G and GY.
The dynamics of leaf concentrations of RS, sucrose, and starch were also indicatives of the Mg effect since non-structural carbohydrates are tightly controlled by intracellular Mg concentrations (Cakmak and Kirkby, 2008). The continuous demand for Mg by plants for carbohydrates synthesis and the transport was well evidenced by the tendency of increasing RS and sucrose and decreasing starch concentration in the leaves as increasing doses of TM. These results reflect positive feedback between high consumption and synthesis of reducing sugars and sucrose (Rodrigues et al., 2021). In the presence of an adequate Mg supply, sucrose is continually synthesized and transported during the day and resynthesized during the night via maltose from starch degradation (Moretti et al., 2021), thereby decreasing the starch concentration, as observed.
Pearson’s correlation analysis revealed positive correlations of soil and leaf concentrations of Mg, sucrose content, and RS content with GY, as well as a negative correlation between starch content with GY. These correlations were expected due to the interaction of suitable plant nutrition with the synthesis and transport of carbohydrates to grains to promote increments in W100G. W100G and GY were highly correlated with each other regardless of crop and growing season, and both increased with the TM dose up to a dose of 1,050 kg ha−1. Considering all factors, cluster analysis grouped the treatments into three groups based on similarity: 0 and 350 kg ha−1 of TM; 700 and 1,050 kg ha−1 of TM; and 1,400 and 1750 kg ha−1 of TM. This grouping is reasonable because although the yield parameters responded to increasing TM dose only up to 1,050 kg ha−1, the photosynthetic parameters and the synthesis of chlorophyll and carbohydrates were stimulated up to the highest TM dose. This result evidenced that either the leaf concentrations of Si and Mg or the yield of both crops reached their limits mainly based on the local climate condition and soil attributes. As lime is also an Mg source and all treatments were limed before the experiment, the positive variation in the TM fertilized plots indicated direct and indirect effects, either as a source of Mg and Si or as soil acidity amendment, especially for soils of low chemical fertility. These results provide agronomic support for TM fertilization under field conditions, but further research on cost-effectiveness that considers issues such as availability, transport, and applicability is needed.
Conclusion
The residual effect of Thermomagnesium (TM) application on Magnesium (Mg) and Silicon (Si) fertilization and soil acidity amendment was well evidenced after 24 months. The dose of 700 kg ha−1 of TM was sufficient to reach the maximum leaf concentration of Magnesium (Mg) and Silicon (Si), whereas 1,050 kg ha−1 of TM buffered the soil pH efficiently. In the soil, the concentrations of Mg and Si increased with increasing doses of TM. Our results also indicated that TM can improve plant physiological parameters in agricultural systems. Although positive physiological responses of soybean and maize plants were obtained by TM application up to the highest dose, the yield responded only up to a TM dose of 1,050 kg ha−1. Accordingly, the application of this mining byproduct on agricultural systems as a source of Mg and Si and as soil acidity amendment can be considered a viable option (Table 1) (Souza et al., 2007).
Data Availability Statement
The raw data supporting the conclusion of this article will be made available by the authors, without undue reservation.
Author Contributions
CC, JB, and LuM designed the experiment. CM, JB, and LuM obtained and process the data. MC and JB analysed the data. CC, MC, LeM, and LuM wrote the manuscript with the contributions of all co-authors. All authors contributed to the article and approved the submitted version.
Conflict of Interest
The authors declare that the research was conducted in the absence of any commercial or financial relationships that could be construed as a potential conflict of interest.
Publisher’s Note
All claims expressed in this article are solely those of the authors and do not necessarily represent those of their affiliated organizations, or those of the publisher, the editors and the reviewers. Any product that may be evaluated in this article, or claim that may be made by its manufacturer, is not guaranteed or endorsed by the publisher.
Acknowledgments
The National Council for Scientific and Technological Development (CNPq) is acknowledged for the “Excellence in Research” award given to the first and eighth authors. In addition, we would like to thank Anglo American, Brazil, for their support.
Supplementary Material
The Supplementary Material for this article can be found online at: https://www.frontiersin.org/articles/10.3389/fenvs.2022.880613/full#supplementary-material
References
Adrees, M., Ali, S., Rizwan, M., Zia-ur-Rehman, M., Ibrahim, M., Abbas, F., et al. (2015). Mechanisms of Silicon-Mediated Alleviation of Heavy Metal Toxicity in Plants: A Review. Ecotoxicol. Environ. Saf. 119, 186–197. doi:10.1016/j.ecoenv.2015.05.011
Alvares, C. A., Stape, J. L., Sentelhas, P. C., de Moraes Gonçalves, J. L., and Sparovek, G. (2013). Köppen's Climate Classification Map for Brazil. Meteorol. Z. 22, 711–728. doi:10.1127/0941-2948/2013/0507
Ambrosano, E. J., Tanaka, R. T., Mascarenhas, H. A. A., van Raij, B., Quaggio, J. A., and Cantarella, H. (1997). “Leguminosas e oleaginosas,” in Recomendações de adubação e calagem para o Estado de São Paulo. Editors B. van Raij, H. Cantarella, J. A. Quaggio, and A. M. C. Furlani (Campinas, Brazil: Instituto Agronômico), 187–203.
Antonangelo, J. A., Ferrari Neto, J., Crusciol, C. A. C., and Alleoni, L. R. F. (2017). Lime and Calcium-Magnesium Silicate in the Ionic Speciation of an Oxisol. Sci. Agric. (Piracicaba, Braz.) 74, 317–333. doi:10.1590/1678-992X-2016-0372
Auler, A. C., Caires, E. F., Pires, L. F., Galetto, S. L., Romaniw, J., and Charnobay, A. C. (2019). Lime Effects in a No-Tillage System on Inceptisols in Southern Brazil. Geoderma Reg. 16, e00206. doi:10.1016/j.geodrs.2019.e00206
Barbosa, M. A. M., da Silva, M. H. L., Viana, G. D. M., Ferreira, T. R., Souza, C. L. F. de. C., Lobato, E. M. S. G., et al. (2015). Beneficial Repercussion of Silicon (Si) Application on Photosynthetic Pigments in Maize Plants. Aust. J. Crop Sci. 9, 1113–1118. (Accessed March 22, 2022). doi:10.3316/INFORMIT.773682362533050
Bossolani, J. W., Crusciol, C. A. C., Garcia, A., Moretti, L. G., Portugal, J. R., Rodrigues, V. A., et al. (2021a). Long-Term Lime and Phosphogypsum Amended-Soils Alleviates the Field Drought Effects on Carbon and Antioxidative Metabolism of Maize by Improving Soil Fertility and Root Growth. Front. Plant Sci. 12, 1437. doi:10.3389/fpls.2021.650296
Bossolani, J. W., Moretti, L. G., Portugal, J. R., Rossi, R., and Crusciol, C. A. C. (2021b). Thermomagnesium: A By-Product of Ni Ore Mining as a Clean Fertilizer Source for Maize. Agronomy 11, 525. doi:10.3390/AGRONOMY11030525
Brazil, (2008). Normative Instruction SDA/MAPA 27/2006. Brasília, Brazil: Minist. Agric. Livest. Supply. Available at: http://www.mma.gov.br/estruturas/ascom_boletins/_arquivos/03042008_regulamentaprocedimentos.pdf.
Caires, E. F., Haliski, A., Bini, A. R., and Scharr, D. A. (2015). Surface Liming and Nitrogen Fertilization for Crop Grain Production under No-Till Management in Brazil. Eur. J. Agron. 66, 41–53. doi:10.1016/j.eja.2015.02.008
Cakmak, I., and Kirkby, E. A. (2008). Role of Magnesium in Carbon Partitioning and Alleviating Photooxidative Damage. Physiol. Plant. 133, 692–704. doi:10.1111/j.1399-3054.2007.01042.x
Cantarella, H., van Raij, B., and Camargo, C. E. O. (1997). “Adubação de cereais,” in Recomendações de adubação e calagem para o Estado de São Paulo. Editors B. van Raij, H. Cantarella, J. A. Quaggio, and A. M. C. Furlani (Brazil: Instituto Agronômico, Campinas), 43–50. Boletim 100.
Cantarella, H., van Raij, B., and Quaggio, J. A. (1998). Soil and Plant Analyses for Lime and Fertilizer Recommendations in Brazil. Commun. Soil Sci. Plant Analysis 29, 1691–1706. doi:10.1080/00103629809370060
Castro, G. S. A., and Crusciol, C. A. C. (2013). Effects of Superficial Liming and Silicate Application on Soil Fertility and Crop Yield under Rotation. Geoderma 195-196, 234–242. doi:10.1016/j.geoderma.2012.12.006
CETESB (2005). Valores Orientadores para Solos e Águas Subterrâneas no Estado de São Paulo. São Paulo. Available at: https://cetesb.sp.gov.br/areas-contaminadas/wp-content/uploads/sites/17/2020/12/2001-Relatório-de-Estabelecimento-de-Valores-Orientadores-para-Solos-e-Águas-Subterrâneas-no-Estado-de-São-Paulo.pdf (Accessed January 26, 2022).
Ceylan, Y., Kutman, U. B., Mengutay, M., and Cakmak, I. (2016). Magnesium Applications to Growth Medium and Foliage Affect the Starch Distribution, Increase the Grain Size and Improve the Seed Germination in Wheat. Plant Soil 406, 145–156. doi:10.1007/s11104-016-2871-8
Chen, Z. C., Peng, W. T., Li, J., and Liao, H. (2018). Functional Dissection and Transport Mechanism of Magnesium in Plants. Seminars Cell & Dev. Biol. 74, 142–152. doi:10.1016/j.semcdb.2017.08.005
Crusciol, C. A. C., Moretti, L. G., Bossolani, J. W., Moreira, A., Micheri, P. H., and Rossi, R. (2019). Can Dunite Promote Physiological Changes, Magnesium Nutrition and Increased Corn Grain Yield? Commun. Soil Sci. Plant Analysis 50, 2343–2353. doi:10.1080/00103624.2019.1659304
Dalmora, A. C., Ramos, C. G., Plata, L. G., da Costa, M. L., Kautzmann, R. M., and Oliveira, L. F. S. (2020). Understanding the Mobility of Potential Nutrients in Rock Mining By-Products: An Opportunity for More Sustainable Agriculture and Mining. Sci. Total Environ. 710, 136240. doi:10.1016/J.SCITOTENV.2019.136240
de Campos, M., Penn, C. J., Gonzalez, J. M., and Alexandre Costa Crusciol, C. (2022). Effectiveness of Deep Lime Placement and Tillage Systems on Aluminum Fractions and Soil Chemical Attributes in Sugarcane Cultivation. Geoderma 407, 115545. doi:10.1016/J.GEODERMA.2021.115545
Dinkecha, K., and Tsegaye, D. (2017). Effects of Liming on Physicochemical Properties and Nutrient Availability of Acidic Soils in Welmera Woreda, Central Highlands of Ethiopia. Biochem. Mol. Biol. 2, 102–109. doi:10.11648/j.bmb.20170206.16
EMBRAPA, (2020). Tecnologias de produção de soja, Sistemas de Produção 17. Londrina, Brazil: Londrina Embrapa Soja. Available at: https://www.infoteca.cnptia.embrapa.br/infoteca/bitstream/doc/1123928/1/SP-17-2020-online-1.pdf
Farhat, N., Elkhouni, A., Zorrig, W., Smaoui, A., Abdelly, C., and Rabhi, M. (2016). Effects of Magnesium Deficiency on Photosynthesis and Carbohydrate Partitioning. Acta Physiol. Plant. 38, 1–10. doi:10.1007/S11738-016-2165-Z
Farooq, M. A., Niazi, A. K., Akhtar, J., SaifullahFarooq, M., Farooq, M., Souri, Z., et al. (2019). Acquiring Control: The Evolution of ROS-Induced Oxidative Stress and Redox Signaling Pathways in Plant Stress Responses. Plant Physiology Biochem. 141, 353–369. doi:10.1016/j.plaphy.2019.04.039
Fehr, W. R., and Caviness, C. E. (1977). “Stages of Soybean Development,”. Iowa State Univ. Coop. Ext. Serv. Spec. Rep., 80.
Gao, X., Zou, C., Wang, L., and Zhang, F. (2005). Silicon Improves Water Use Efficiency in Maize Plants. J. Plant Nutr. 27, 1457–1470. doi:10.1081/PLN-200025865
Garcia, A., Crusciol, C. A. C., McCray, J. M., Nascimento, C. A. C., Martello, J. M., de Siqueira, G. F., et al. (2020). Magnesium as a Promoter of Technological Quality in Sugarcane. J. Soil Sci. Plant Nutr. 20, 19–30. doi:10.1007/S42729-019-00096-X/TABLES/6
Hosseini, S. A., Rad, S. N., Ali, N., and Yvin, J.-C. (2019). The Ameliorative Effect of Silicon on Maize Plants Grown in Mg-Deficient Conditions. Int. J. Mol. Sci. 20, 969. doi:10.3390/ijms20040969
Hussain, S., Mumtaz, M., Manzoor, S., Shuxian, L., Ahmed, I., Skalicky, M., et al. (2021). Foliar Application of Silicon Improves Growth of Soybean by Enhancing Carbon Metabolism under Shading Conditions. Plant Physiology Biochem. 159, 43–52. doi:10.1016/j.plaphy.2020.11.053
Korndörfer, G. H., Pereira, H. S., and Nolla, A. (2004). “Análise de silício: solo, planta e fertilizante,”. Uberlândia: GPSi-ICIAG-UFU (Boletim Técnico, 2) (Uberlândia, Brazil: Instituto de Ciências Agrárias, Universidade Federal de Uberlândia), 34.
Kumaraswamy, R. V., Saharan, V., Kumari, S., Chandra Choudhary, R., Pal, A., Sharma, S. S., et al. (2021). Chitosan-silicon Nanofertilizer to Enhance Plant Growth and Yield in Maize (Zea mays L.). Plant Physiology Biochem. 159, 53–66. doi:10.1016/j.plaphy.2020.11.054
Lawson, T., and Flexas, J. (2020). Fuelling Life: Recent Advances in Photosynthesis Research. Plant J. 101, 753–755. doi:10.1111/tpj.14698
Li, J., Yokosho, K., Liu, S., Cao, H. R., Yamaji, N., Zhu, X. G., et al. (2020). Diel Magnesium Fluctuations in Chloroplasts Contribute to Photosynthesis in Rice. Nat. Plants 6, 848–859. doi:10.1038/s41477-020-0686-3
Ma, J. F., and Yamaji, N. (2006). Silicon Uptake and Accumulation in Higher Plants. Trends Plant Sci. 11, 392–397. doi:10.1016/j.tplants.2006.06.007
Malavolta, E., Vitti, G. C., and Oliveira, S. A. (1997). Avaliação do estado nutricional das plantas: princípios e aplicações. 2nd ed. Piracicaba, Brazil: POTAFOS.
Martins, A. P., Denardin, L. G. d. O., Tiecher, T., Borin, J. B. M., Schaidhauer, W., Anghinoni, I., et al. (2020). Nine-year Impact of Grazing Management on Soil Acidity and Aluminum Speciation and Fractionation in a Long-Term No-Till Integrated Crop-Livestock System in the Subtropics. Geoderma 359, 113986. doi:10.1016/j.geoderma.2019.113986
Moradtalab, N., Weinmann, M., Walker, F., Höglinger, B., Ludewig, U., and Neumann, G. (2018). Silicon Improves Chilling Tolerance during Early Growth of Maize by Effects on Micronutrient Homeostasis and Hormonal Balances. Front. Plant Sci. 9, 420. doi:10.3389/fpls.2018.00420
Moretti, L. G., Crusciol, C. A. C., Bossolani, J. W., Garcia, A., Rossi, R., and Moreira, A. (2021). Thermomagnesium as a Fertilizer for Soybean: Carbohydrate Metabolism, Silicon-Magnesium Fertilizer, and Grain Yield. J. Plant Nutr. 44, 2108–2122. doi:10.1080/01904167.2021.1889599
Moretti, L. G., Crusciol, C. A. C., Bossolani, J. W., Momesso, L., Garcia, A., Kuramae, E. E., et al. (2020a). Bacterial Consortium and Microbial Metabolites Increase Grain Quality and Soybean Yield. J. Soil Sci. Plant Nutr. 20, 1923–1934. doi:10.1007/s42729-020-00263-5
Moretti, L. G., Crusciol, C. A. C., Bossolani, J. W., Rossi, R., and Moreira, A. (2020b). Agricultural Repurposing of Nickel Slag Residue. J. Plant Nutr. 44, 1141–1150. doi:10.1080/01904167.2020.1845385
Moretti, L. G., Crusciol, C. A. C., Bossolani, J. W., Rossi, R., and Moreira, A. (2020c). Agricultural Repurposing of Nickel Slag Residue. J. Plant Nutr. 44, 1141–1150. doi:10.1080/01904167.2020.1845385
Nelson, N. (1944). A PHOTOMETRIC ADAPTATION OF THE SOMOGYI METHOD FOR THE DETERMINATION OF GLUCOSE. J. Biol. Chem. 153, 375–380. doi:10.1016/s0021-9258(18)71980-7
Ning, D., Qin, A., Liu, Z., Duan, A., Xiao, J., Zhang, J., et al. (2020). Silicon-mediated Physiological and Agronomic Responses of Maize to Drought Stress Imposed at the Vegetative and Reproductive Stages. Agronomy 10, 1136. doi:10.3390/agronomy10081136
Quaggio, J. A., and van Raij, B. (1997). “Correção da acidez Do solo,” in Recomendações de adubação e calagem para o Estado de São Paulo. Editors B. van Raij, H. Cantarella, J. A. Quaggio, and A. M. C. Furlani (Campinas, Brazil: Instituto Agronômico), 14–19.
Raij, B. v., Andrade, J. C., Cantarella, H., and Quaggio, J. A. (2001). Análise química para avaliação da fertilidade de solos tropicais. Available at: www.iac.br (Accessed August 4, 2020).
Ritchie, S. W., Hanway, J. J., and Benson, G. O. (1993). How a Corn Plant Develops. Special Report N. 48. Ames, IA, USA: Iowa State University of Science and Technology. Coop. Ext. Serv.
Rodrigues, V. A., Crusciol, C. A. C., Bossolani, J. W., Moretti, L. G., Portugal, J. R., Mundt, T. T., et al. (2021). Magnesium Foliar Supplementation Increases Grain Yield of Soybean and Maize by Improving Photosynthetic Carbon Metabolism and Antioxidant Metabolism. Plants 10, 797. doi:10.3390/PLANTS10040797
Soil Survey Staff (2014). Keys to Soil Taxonomy. 12th ed. Washington, DC, USA: USDA - Natural Resources Conservation Service. doi:10.1007/978-1-4020-3995-9_269
Souza, D. M., Miranda, L. N., and Oliveira, S. A. (2007). “Acidez Do solo e sua correção,” in Fertilidade Do Solo. Editors R. F. Novais, V. H. Alvarez V, N. Barros, R. L. F. Fontes, R. B. Cantarutti, and J. C. L. Neves (Viçosa: Sociedade Brasileira de Ciência do Solo), 205–274.
Tränkner, M., and Jamali Jaghdani, S. (2019). Minimum Magnesium Concentrations for Photosynthetic Efficiency in Wheat and Sunflower Seedlings. Plant Physiology Biochem. 144, 234–243. doi:10.1016/j.plaphy.2019.09.040
Keywords: byproduct, soil remineralizer, crop nutrition, environmental safety, cropping systems
Citation: Crusciol CAC, de Campos M, Momesso L, Bossolani JW, Moretti LG, Portugal JR, de Melo CVCB and Calonego JC (2022) Nickel Ore Mining Waste as a Promising Source of Magnesium and Silicon for a Smart-Agricultural Management. Front. Environ. Sci. 10:880613. doi: 10.3389/fenvs.2022.880613
Received: 22 February 2022; Accepted: 19 April 2022;
Published: 03 May 2022.
Edited by:
Eduardo Guimarães Couto, Federal University of Mato Grosso, BrazilReviewed by:
Tiago Osório Ferreira, University of São Paulo, BrazilDirceu Mattos Jr, Instituto Agronômico de Campinas (IAC), Brazil
Copyright © 2022 Crusciol, de Campos, Momesso, Bossolani, Moretti, Portugal, de Melo and Calonego. This is an open-access article distributed under the terms of the Creative Commons Attribution License (CC BY). The use, distribution or reproduction in other forums is permitted, provided the original author(s) and the copyright owner(s) are credited and that the original publication in this journal is cited, in accordance with accepted academic practice. No use, distribution or reproduction is permitted which does not comply with these terms.
*Correspondence: Carlos A. C. Crusciol, Y2FybG9zLmNydXNjaW9sQHVuZXNwLmJy
†These authors have contributed equally to this work