- 1College of Desert Control Science and Engineering, Inner Mongolia Agricultural University, Hohhot, China
- 2Key Laboratory of Desert Ecosystem Conservation and Restoration, State Forestry and Grassland Administration of China, Hohhot, China
- 3Inner Mongolia Yiji Biotechnology Co., Ltd., Hohhot, China
- 4Inner Mongolia Mengnong Quinoa Industry Research Institute, Hohhot, China
The sub-mountain plains area at the northern foothills of the Yinshan Mountains in Inner Mongolia is an important grain producing area in the cold and arid regions of China, but due to climatic and environmental factors, it has resulted in a fragile ecological environment, degradation of arable land, and serious soil erosion. Quinoa (Chenopodium quinoa Willd.) is a hardy, cold and saline tolerant crop with much higher protein content than other crops. Therefore, it has begun to be grown on a large scale in the region in recent years. In order to investigate the distribution characteristics of soil aggregates and organic carbon (SOC) in quinoa fields in ecologically fragile areas, four quinoa fields with different soil textures, namely, sandy soil, sandy loam, loamy soil and clay loam, were selected for this experiment, and the mass percentages of different soil aggregate particle sizes and the organic carbon content of each particle size were determined before and after quinoa planting, and the organic carbon mineralisation in soil aggregates of quinoa fields with different textures was analysed through indoor organic carbon mineralisation cultures. The dynamic characteristics were analysed. The results showed that the stability of soil aggregates was enhanced after planting quinoa, and the highest mass percentage of aggregates with >2 mm particle size was 27.95% in clay loam soil. Planting quinoa in loamy soil had the most obvious effect on promoting the formation of soil macroaggregates, while the organic carbon content increased the most. The organic carbon (SOC) content of soil aggregates of all grain sizes increased by 0.45–3.09 g kg-1 compared to the pre-sowing period, with the fastest rate and greatest intensity of organic carbon mineralisation in aggregates of 2–0.25 mm grain size. The study showed that growing quinoa in loamy soils improves soil structure, slows down the rate of soil organic carbon mineralization, and increases soil carbon sequestration capacity.
Introduction
Soil is the largest terrestrial carbon reservoir and plays a crucial role in controlling regional carbon balance and global climate change. Large-scale farming activities can have a large impact on both the physical and chemical properties of soils, and in the process of agricultural production, due to long-term irrational planning, monoculture structure, weathering, and mechanical crushing, soil agglomeration is reduced, and surface cover often exists in the form of a mixture of sandy soils, resulting in the degradation of sandy textural structure and soil ecological environment (Weidhuner et al., 2020).
Quinoa (Chenopodium quinoa Willd.) is a crop that grows and develops normally on infertile soils and is tolerant to cold, drought (Espíndola Canedo et al., 1988), and salinity (González and Gallardo, 1992).Compared with other crops, quinoa seeds have more amino acids, proteins, and other nutrients that are beneficial to the human body, so quinoa is widely cultivated in areas with poor living conditions with high economic value (Vilcacundo and Hernández-Ledesma, 2016), and in the last century, some countries in Europe began to introduce cultivation and selection of good varieties (Rodríguez Gómez et al., 2021). China in 1987 after the successful introduction of many pilot cultivation began to be widely planted in suitable areas, the northern foothills of the Yinshan Mountains in China is a cold arid, and semi-arid region, and the climate environment is suitable for the growth and development of quinoa, as of 2022, quinoa planted in the Inner Mongolia Autonomous Region, the total area of more than 100,000 acres, becoming one of the largest quinoa planting area in China, of which the northern foothills of the Yinshan Mountains area planted more than 40,000 acres. Inner Mongolia Yinshan northern foothills region crop growth conditions are poor, cold climate, and soil fertility is weak, by the climate, the local soil scouring and nutrient loss is serious, as well as the rough management of arable land, etc., resulting in soil organic carbon and soil fertility there are large differences. Soil texture, as a heavy environmental factor for crop growth, has an important influence on the migration, transformation, and accumulation of organic carbon in arable land.
Soil aggregates are the basic units of soil structure, providing habitat and activity for soil microorganisms and driving material exchange and energy flow in terrestrial ecosystems.The increase in soil organic matter content contributes to the formation of aggregates, which can enhance the stability of soil structure (Cong et al., 2016). Carbon is an important constituent element in soil ecosystems, which directly affects soil fertility, crop quality, and yield, and is closely related to global climate change, soil nutrient cycling and energy conversion, and greenhouse gas emissions (Sierra et al., 2021). About 90% of the organic carbon in the topsoil of terrestrial ecosystems is located in soil aggregates, indicating a close correlation between the formation of aggregates and soil carbon sequestration and mineralization (Li et al., 2023a). In recent years, a great deal of research has been carried out at home and abroad on the relationship between soil organic matter and the formation of aggregates. The results have proved that organic and inorganic complexes are the material basis for the formation of soil water-stable aggregates and that mineral particles existing in the colloidal state in the soil and humus in the organic matter are the most active parts of the soil (Ritschel and Totsche, 2019). Humic substances, in particular, are highly susceptible to combining with mineral particles in loose, tight, and stable junction states to form organic-inorganic complexes on their surfaces, forming microaggregates, while small microaggregates combine in various ways to form aggregates of various particle sizes (Ndzelu et al., 2021).
The mineralization of organic carbon in soil is a complex and slow natural process, and the amount and intensity of CO2 released from mineralization by microbial decomposition can reflect the soil quality status and evaluate the impact of environmental factors or anthropogenic changes on it. The mineralization process of soil organic carbon is affected by a variety of factors, such as the chemical composition of soil organic matter, the existence of state soil microbial population composition, the activity of soil physicochemical properties, etc. Complex conditions lead to different environments under the content of organic carbon there is a great deal of difference, which leads to a greater difference in the characteristics of the mineralization also exists (Liu et al., 2018). It was found that the porosity, oxygen, and water fluxes varied greatly among aggregates with different grain sizes, which implied that the SOC mineralization rate and CO2 release from soil aggregates with different grain sizes might be different. Therefore, it is of great scientific significance to investigate the characteristics of SOC mineralization in aggregates of different particle sizes for a deeper understanding of the soil carbon cycle in microenvironments.
The northern foothills of Yinshan Mountain are an important ecological barrier in northern China and have an important position in China’s ecological environment construction and global change research. Environmental problems such as land degradation, soil erosion of arable land, and nutrient decline should not be ignored. At present, the research on soil organic carbon mineralization focuses on the macroscopic study of different utilization modes of soils, such as forest land (Dong et al., 2022; Lazicki et al., 2023; Dong et al., 2022; Lazicki et al., 2023), grassland (He et al., 2022; He et al., 2022), and arable land, etc. Some scholars have studied the soil aggregate and organic carbon content of arable land in terms of cultivation modes (Li et al., 2024), planting modes, and different crops, but there are fewer studies on the formation and distribution of soil aggregates and organic carbon mineralization in arable land with different soil textures in the northern foothills of the Yinshan Mountain region. Based on this study, this study selected four quinoa fields with different soil textures from cultivated land in the northern foothills of the Yinshan Mountains, all of which are large planting areas with important impacts on the soil environment of local cultivated land. From the soil aggregates before and after planting quinoa for 2 years, the structural and organic carbon changes in quinoa fields with different soil textures were analysed. Combined with the results of mineralisation culture of the soil after planting quinoa, we will further understand the characteristics of soil organic carbon mineralisation after extensive planting of quinoa in this area, provide theoretical basis for quinoa planting in arid and semi-arid areas in the northern foothills of the Yinshan Mountains, and contribute to the healthy and sustainable development of agriculture.
Materials and methods
Study area and patch characteristics
The Wuchuan region, situated in the northern foothills of the Yinshan Mountains in Inner Mongolia, represents an intermediate zone in the transition from the Yinshan Mountains to the Mongolian high plains. The southern portion of the region is characterized by low to medium mountainous terrain, while the central and northern regions predominantly exhibit low hill and undulating hill terrain. The region’s vegetation is primarily mountain grassland on the sun-exposed slopes and summer green broad-leaved and coniferous forests on the shady slopes. The climate is classified as a mesothermal continental monsoon climate. The region is traversed by four inland and outland rivers. The inland river is part of the Tabu River system, while the outland river is part of the Dahei River system. The average annual temperature is 3.0°C, with an annual minimum temperature of −37.0°C and an annual maximum temperature of 36.2°C. The frost-free period is about 124 days, and the daily maximum and low temperatures and precipitation during the reproductive period (May-September) of quinoa in 2022–2023 are shown in Figure 1. The main soil type in the study area is chestnut-calcium soil, with an organic matter content of 16.35 g kg-1, a total nitrogen content of 1.23 g kg-1, an alkaline dissolved nitrogen of 55.29 mg kg-1, plant available phosphorus 9.7 mg kg-1, and plant available potassium 99.67 g kg-1.
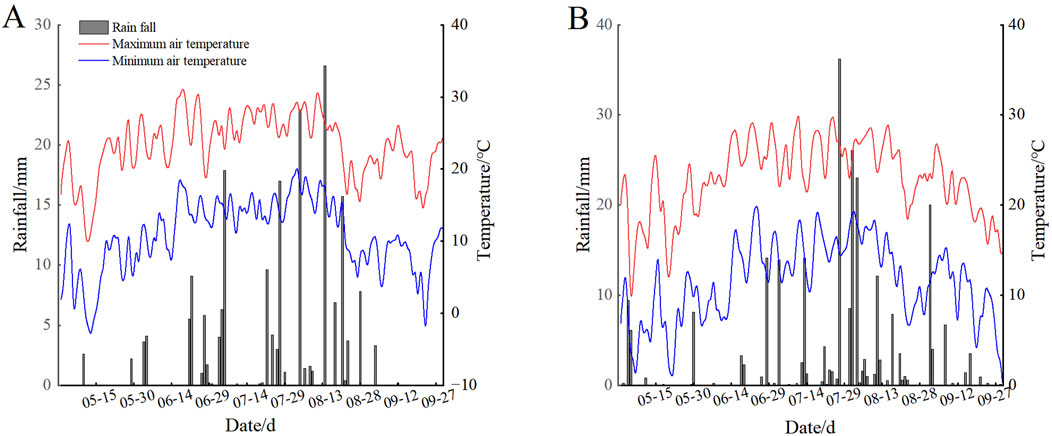
Figure 1. Daily maximum and minimum temperatures and precipitation during the quinoa growing season. 2022 (A), 2023 (B).
Experimental design and sample collection
In the study area, four sample plots of farmland with different soil textures were selected, and all the previous crops were potatoes, and the profile of the sample plots is shown in Table 1, and quinoa was planted in 2022 and 2023 for two consecutive years, and the variety was Meng quinoa No. 6, and all the plots were finely leveled before sowing, and spot seeding was carried out by using a two-row mulching planter, and the sowing depth was debugged to be about 3 cm before sowing, and the spacing of the plants was 20 cm and the spacing of rows was 50 cm, and the fertilizers were applied before spot seeding. The fertilizer applied was bio-organic fertilizer (organic matter ≥60%, effective number of live bacteria ≥0.2 billion/g), 15 g per hole, and quinoa was fertilized with chelated drip diammonium water-soluble fertilizer (N-P2O5-K2O, total nutrients ≥64.0%) in the flowering and filling period at 150 kg hm-2. Timely weeding, disease prevention, and pest control during the whole life cycle to ensure the normal growth and development of quinoa.
Soil samples were collected before quinoa sowing in 2022 and after harvesting in 2023, respectively, from sample plots of farmland with 2 years of continuous quinoa cropping in different soil textures, and the “Z” sampling method was adopted on each sample plot, with five points selected from each quinoa planting area, and soil profiles were dug vertically, and stratified sampling was carried out using the “ring-knife method”. The soil profile was dug out vertically, and stratified sampling was carried out using the “ring knife method”. Soil samples were collected from 0 to 20 cm, dried naturally indoors, and broken into 1 cm pieces after removing plant roots, stones, and other foreign objects, and then sieved through an 8 mm sieve and set aside. All experiments were repeated three times.
Measurement methods
The air-dried soil samples were weighed 50 g and placed in the uppermost layer of a set of sieves with sequential sizes of 2, 0.25, and 0.053 mm, and the test soil samples were sieved for aggregates by the dry sieve method, which was divided into four particle sizes, namely, coarse macro-aggregates (>2 mm), fine macro-aggregates (0.25–2 mm), micro-aggregates (0.053–0.25 mm), and powdered clay particles (<0.053 mm). The soil aggregates of different particle sizes were ground and sieved through a 100-mesh sieve, and the organic carbon content was determined by an external heating method using potassium dichromate. The recovered soil organic carbon ranged from 90.71% to 92.64% for >2mm, 89.60%–94.41% for 0.25–2 mm, 89.55%–94.25% for 0.053–25 mm, 88.09%–93.92% for 0.25–2 mm. The mass percent content of aggregates was calculated using the total weight of soil after sieving.
The formula for calculating soil bulk density is as Equation 1:
Where ρb is the soil bulk density (g·cm-3); m is the dry mass of the soil (g); and V is the volume of the ring blade (cm3).
The mass fraction of water-stable aggregates of each particle size was expressed as Wi (i = 1, 2, 3, 4, corresponding to >2mm, 0.25∼2mm, 0.053–0.25mm, and <0.053 mm aggregates of four particle sizes, respectively), and the stability of soil aggregates was evaluated by using the Mean Weighted Diameter (MWD), which was calculated by the following Formulas 2, 3:
Where M is the total weight of the soil (g); mi is the mass of each particle size aggregate (g); Xi is the average diameter of each particle size aggregate (mm); and Wi is the percentage content of each particle size aggregate (%).
Mineralization incubation tests were carried out using a constant temperature indoor incubation and determined by the alkali absorption method. Weigh 20.00 g of air-dried agglomerate samples of each particle size in 500 mL culture flasks. The moisture content of the entire soil and aggregates of various grain sizes was adjusted to 60% of the water holding capacity of the field with deionized water, then sealed and placed in the incubator at 25°C for 1 week to recover microbial activity, and then 25 mL absorber cups containing 10 mL of 0.1 mol·L-1NaOH were suspended in the culture flasks, sealed, and then dark incubated in the incubator at 25°C. Then, 25 mL absorber cups containing 10 mL of 0.1 mol·L-1NaOH solution were suspended in the culture flasks, sealed, and incubated in the dark at 25°C in a constant temperature incubator. Three parallel tests were set up for each culture device, and three soil-free blank controls were set up at the same time, and the measurements were carried out in the first, fourth, seventh, 10th, 14th, 18th, 22nd, and 28th d of the culture, and the alkali absorber cups were carefully replaced with tweezers during the measurements, and 1 mol L-1 BaCl2 solution 2 mL was added into the absorber cups, followed by two drops of phenolphthalein indicator until the red color disappeared. BaCl2 solution 2 mL, then add two drops of phenolphthalein indicator and titrate with 0.1 mol L-1 HCl (calibrated with borax before each titration) until the red color disappears. The amount of organic carbon mineralized in each particle size agglomerate during the incubation period was calculated based on the titration amount of HCl.
Mineralization of organic carbon in aggregates is calculated as in Equation 4:
Where Ct is the amount of organic carbon mineralized in the agglomerate (mg·kg-1); CHCl is the concentration of hydrochloric acid (mol·L-1); V0 is the volume of blank titration (mL); V1 is the volume of hydrochloric acid consumed (mL); MCO2 is the molar mass of CO2 (44 g mol-1); and M is the dry weight of the soil sample (g).
Rate of organic carbon mineralization in aggregates is calculated as in Equation 5:
Where: R is the rate of organic carbon mineralization of aggregates (mg·kg-1·d-1); Ct is the amount of organic carbon mineralization of aggregates during the incubation time (mg·kg-1); t is the number of incubation days (d).
Fitting the incubation time to the cumulative mineralization of organic carbon by means of a first-order kinetic Equation 6,
In the equation, Ct is the cumulative mineralized amount (g·kg-1) after incubation for t time, C0 denotes the amount of potentially mineralizable organic carbon (g·kg-1), k is the turnover constant of the organic carbon pools (d− 1), t denotes the number of days of incubation (d), and the half-turnover period T1∕2 = ln2/k.
Statistical analysis
Experimental data were analysed by one-way ANOVA, correlation analysis and Duncan’s test of significance (p < 0.05) using SPSS 22.0; first-order kinetic equations were fitted and plotted using Origin 2024.
Results
Particle size distribution of soil aggregates
Figure 2 shows the distribution of aggregate content by particle size after planting quinoa. The results indicate that planting quinoa significantly changes the distribution of soil aggregate particle size.The percentage of soil agglomerate mass in the Wuchuan area at the northern foot of Yinshan Mountain was mainly dominated by fine macro aggregates and micro aggregates, which could reach 60.79%–75.41% in the soil. The percentage of >0.25 mm particle size aggregates decreased by 4.46% after planting quinoa in sand and increased by 4.51%, 9.04%, and 9.47% in sandy loam, loamy soil, and clay loam, respectively. As shown in Table 2, after planting quinoa, the soil bulk weight was significantly reduced and the soil aggregation stability of loamy soil was significantly increased.
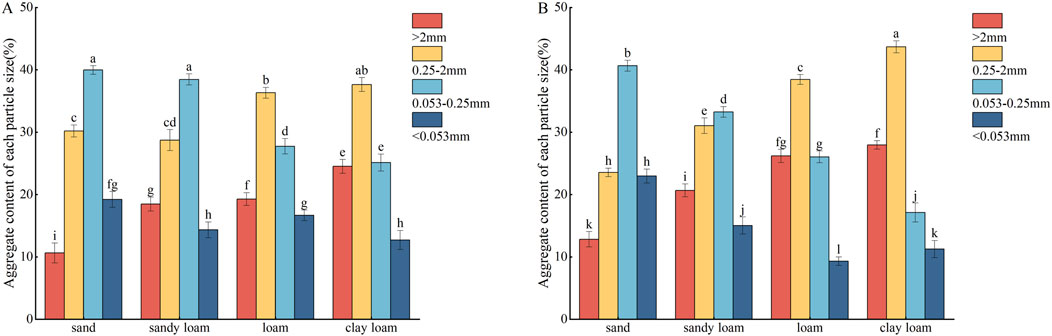
Figure 2. Particle size distribution of soil aggregates with different textures after planting quinoa. Pre-planting (A), Post-planting (B).
Soil organic carbon concentration in soil aggregate sizes
Planting quinoa affected the distribution of organic carbon in soil aggregates in different grain sizes (Figure 3). There were significant differences (p < 0.05) in the content of organic carbon in aggregates of different grain sizes in different soil textures. The content of organic carbon in clay loam soil was higher than that in other soil textures and increased by 19.74%–28.08% compared with that before planting. After planting quinoa, the organic carbon content of 0.25–2 mm size aggregates increased in all soil textures. In loamy soils, the organic carbon content of 0.053–0.25 mm size aggregates decreased from 13.38 g·kg-1–12.06 g kg-1 compared to the pre-planting, but in sand, it increased by 1.21 g kg-1. The most significant increase in organic carbon content was observed in <0.053 mm size aggregates after planting quinoa.
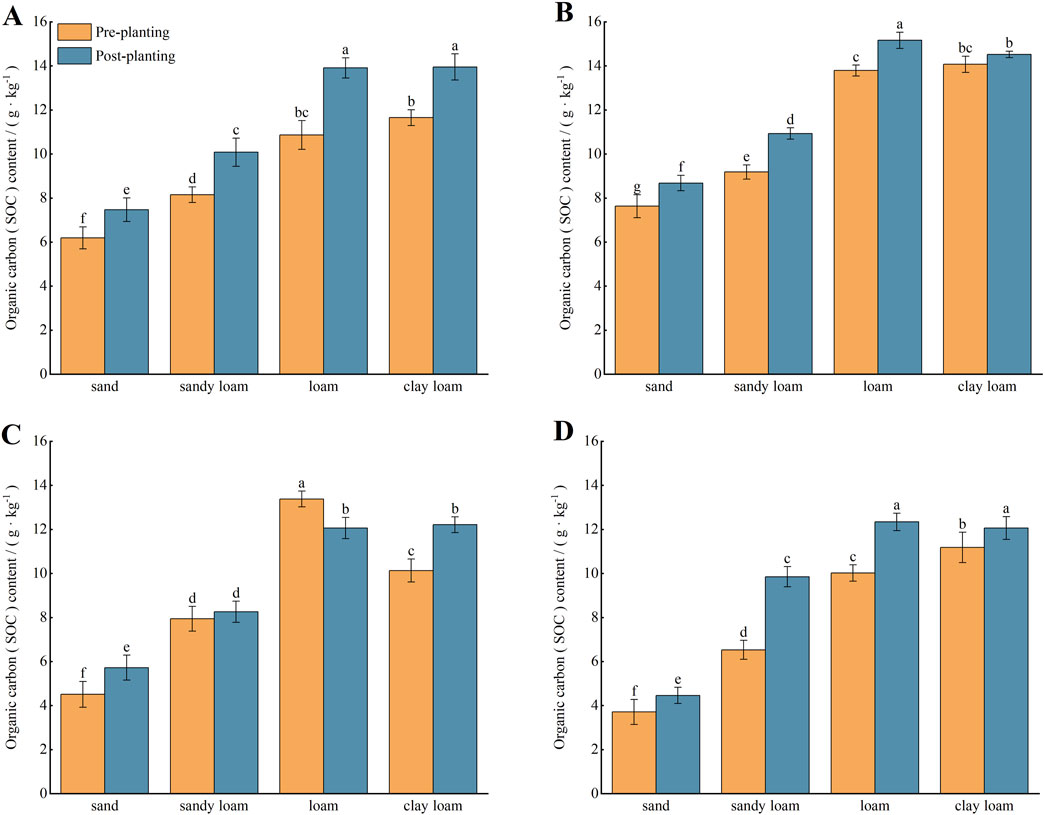
Figure 3. Organic carbon content of soil aggregates with different textures after planting quinoa. >2 mm (A), 0.25–2 mm (B), 0.053–0.25 mm (C), <0.053 mm (D).
Changes in soil organic carbon mineralisation
From Figure 4, the results showed that the rate of organic carbon mineralization of aggregates in quinoa fields of all four soil textures tended to decrease with the increase of incubation time, with the overall performance of clay loam > loam > sandy loam > sand. Comparison of the organic carbon mineralisation rates of soils with different particle size aggregates during the incubation period showed that: the difference in the organic carbon mineralisation rates of soils with >0.25 mm particle size aggregates was large, and the organic carbon sequestration capacity of the soils was weak; the difference in the organic carbon mineralisation rates of soils with <0.25 mm particle size aggregates was small, and the organic carbon sequestration capacity of the soils was relatively strong. During the incubation period, the rates of organic carbon mineralization in quinoa fields with four soil textures ranged from 7.14 to 89.44 mg kg-1 ·d-1 for sand, from 11.99 to 94.44 mg kg-1 ·d-1 for sandy loam, from 12.71 to 122.66 mg kg-1 ·d-1 for loam and from 14.16 to 138.23 mg kg-1 ·d-1 for clay loam,. The soil organic carbon mineralization rate had a similar rule of change specifically manifested in two stages, the soil organic carbon mineralisation rate decreased rapidly in the first 4 days of incubation, and the mineralisation rate gradually levelled off after 4 days.
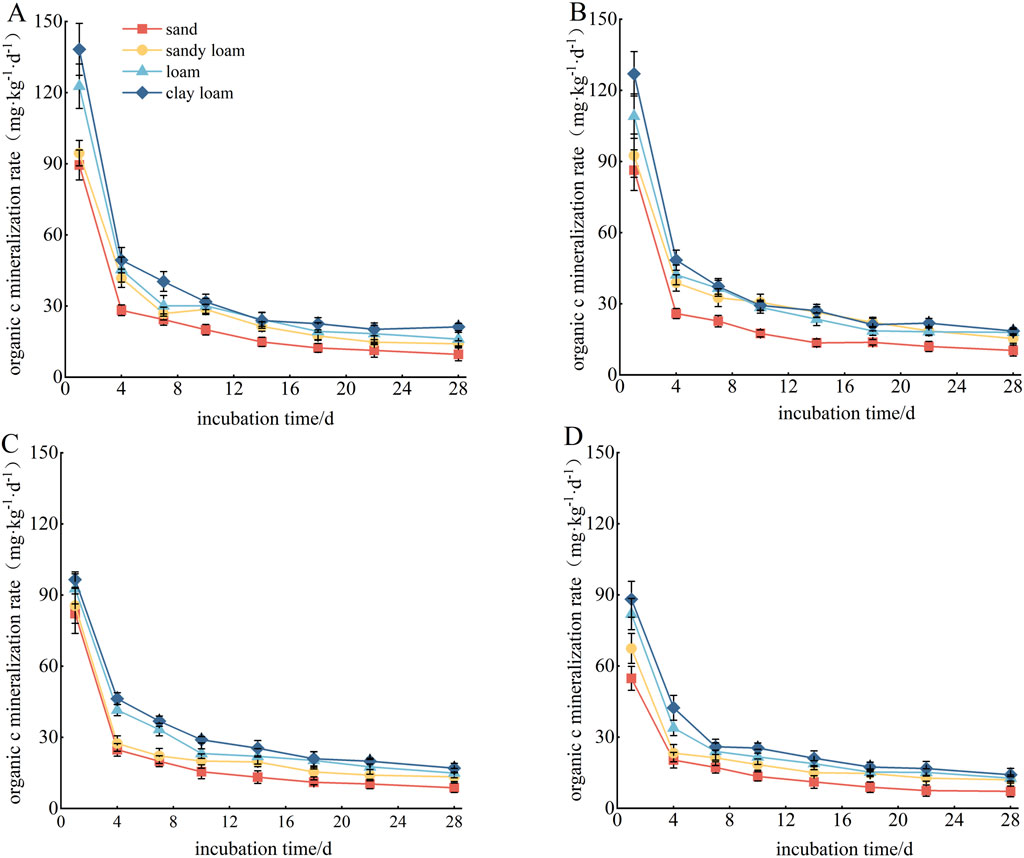
Figure 4. Changes in the rate of organic carbon mineralization in soil aggregates of quinoa fields with different soil textures. >2 mm (A), 0.25–2 mm (B), 0.053–0.25 mm (C), <0.053 mm (D).
Cumulative soil organic carbon mineralization
The cumulative mineralization of organic carbon (CM) in quinoa field soils of different soil textures, for each particle size aggregate at 28 days of incubation, is shown in Figure 5. For the same soil texture, the cumulative mineralisation of organic carbon in aggregates of different soil textures was the highest in clay loam and the lowest in sandy loam. Among them, the cumulative mineralisation of large agglomerates was the largest. The cumulative mineralisation of organic carbon in clay loam with >2 mm agglomerates was 120.41% higher than that in sandy loam, 50.31% higher than that in sandy loam and 31.84% higher than that in loam.
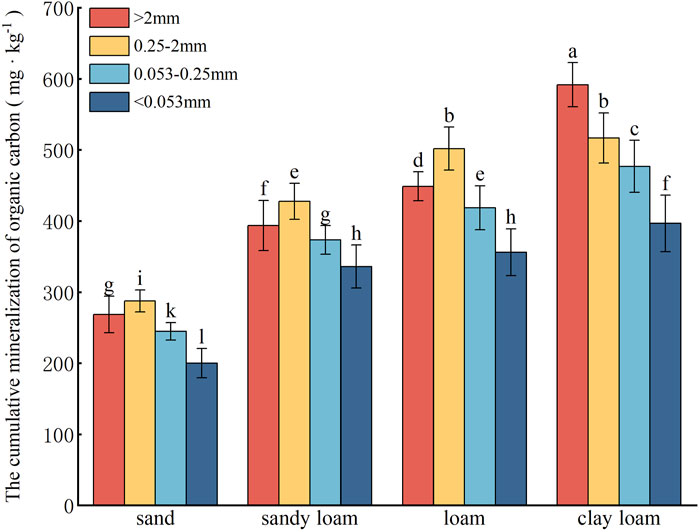
Figure 5. Cumulative mineralization of organic carbon in aggregates of different soil textures incubated for 28 d.
Soil organic carbon mineralization parameters
The cumulative mineralization of soil organic carbon fitted to the first-order kinetic model presented in Table 3. The R2 value of the fitted equations was greater than 0.8. The potential mineralisable organic carbon content of aggregates with different particle sizes was as follows: coarse macroaggregates (>2 mm) > fine macroaggregates (0.25–2 mm) > microaggregates (0.053–0.25 mm) > powdery clay particles (<0. 053 mm). The potential mineralisable organic carbon content of the same grain size in different soil textures showed clay loam > loam > sandy loam > sand. Compared to sandy loam, the potentially mineralisable organic carbon content in >2 mm particle size aggregates in quinoa fields with clay loam texture was 134.26% higher, 2–0.25 mm 82.19% higher, 0.25–0.053 mm 102.95% higher, and <0.053 mm 123.20% higher. The daily turnover rate constants of the organic carbon pools of each aggregate in quinoa fields with different soil textures ranged from 0.065 to 0.164, and the half-turnover periods ranged from 2.501 to 3.426. The C0/SOC was able to better reflect the strength of the soil’s carbon sequestration capacity, and the higher the value, the greater the mineralization capacity and the weaker the capacity of organic carbon sequestration. The overall size of C0/SOC was clay loam > loam > sandy loam > sand, and the sand content of sand was higher than that of clay loam, which indicated that the carbon sequestration capacity of the four soils gradually weakened with the increase of sand.
Correlation between soil aggregate particle size and organic carbon
Correlation analysis of each aggregate particle size and organic carbon, as shown in Figure 6, MWD was significantly positively correlated with >2 mm particle size aggregates, and significantly negatively correlated with 0.053–0.25 mm particle size aggregates. The larger the mass proportion of >2 mm particle size aggregates, the better the stability of aggregates and the more organic carbon content.
Discussion
Effect of quinoa on soil aggregates in arable land
Quinoa has rich genetic diversity, a large genetic coefficient of variation, growth, and development are influenced by the environment, and the same variety in different environments phenotypic traits will show large differences (Maestro-Gaitán et al., 2023). Meng quinoa No. 6 has full grain, and excellent quality characteristics, so in the northern foothills of the Yinshan Mountains in Inner Mongolia quinoa is the main planting varieties that are widely planted. Following the planting of quinoa, the mass ratio of coarse and fine macroaggregates in loam and clay loam soils was found to be greater than that of microaggregates and clayey silt particles. Additionally, the soil structure was observed to be optimal. The differences in above-ground development and root growth among quinoa varieties result in variations in soil structure and nutrient changes following the planting of quinoa. The interaction mechanism between the quinoa root system and soil aggregate formation and structural changes requires further experimental investigation.
The growth and development of quinoa in the northern foothills of the Yinshan Mountains in Inner Mongolia are subjected to saline and drought stress, and the special root structure and diverse rhizobial microbial community enable it to survive under unfavorable conditions (Varma, 2021). Crop root stubble is one of the main carbon source factors for the variation of soil organic carbon content in agricultural fields. Applying organic and chemical fertilizers has been observed to increase the amount of quinoa stubble and promote an increase in root secretions. This, in turn, has been shown to increase the input of soil organic carbon and facilitate the accumulation of soil organic matter and nutrient enrichment (Zhao et al., 2016). The cementing material produced by the inter-root microbial metabolism of quinoa can bind with small soil particles and promote the formation of large aggregates, which is also conducive to the accumulation of organic matter. Soil organic matter and other nutrient contents were significantly higher after planting quinoa than after planting different crops, and the planting of quinoa treatment showed the most significant increase in soil enzyme activities, indicating that quinoa has some advantages over other crops for soil improvement and nutrient sequestration (Wieme et al., 2020). These results may be related to the continuous release of organic acids, sugars, and amino acids into the inter-root zone during the growth of quinoa roots. Numerous studies have demonstrated that for annual plants, approximately 40% of the photosynthetic products transported to the root system are released into the inter-root zone, which in turn affects the soil’s physicochemical properties.
In this study, Meng quinoa No. Six was planted in the experimental plots for two consecutive years, but in actual agricultural production, the year-round continuous cultivation of a single crop will lead to a decline in soil fertility, which is not conducive to the growth and development of the crop. Different planting modes of crops also have different effects on the soil environment, and the introduction of quinoa cultivation and related research in China is relatively short, and the effects of rotating or intercropping quinoa with other crops on the soil need to be further explored.
Distribution of organic carbon in quinoa field clusters of different soil textures
Crop cultivation exerted a considerable influence on the dispersion of soil aggregates; however, the distribution of soil aggregates was not influenced by soil depth. The growth of the quinoa root system will disturb the soil and affect the formation of soil aggregates (Dai et al., 2024). In this study, we found that soil water-stable aggregates in the 0–20 cm soil layer accounted for the largest proportion of fine and large aggregates after planting quinoa, and soil aggregate stability was affected accordingly. The primary factors influencing soil aggregate formation in agricultural fields are tillage activities and soil texture. Studies have shown that the fertility status of agricultural soils on a regional scale depends on soil texture and is regulated by macroaggregates (Jordan et al., 1996). With the increase of soil clay content, the content of macroaggregates and organic carbon increased significantly, and the content of micro aggregates decreased significantly, which is consistent with the results of this study after planting quinoa in sand and clay loam soils.
In this study, the organic carbon content increased significantly after planting quinoa. The reason for this phenomenon may be that, after the withering of deciduous weeds, organic matter is produced by shallow surface decay, and plant residues and the underground rhizomes of quinoa are conducive to promoting the accumulation of soil organic matter. Studies have shown that soil nutrients are correlated with crop nitrogen uptake into the soil, which in turn affects crop protein content [96]. Quinoa protein content is much higher than other crops, so there may be a special way of nitrogen utilization, which in turn affects soil nutrients. The interaction effect between quinoa and soil nutrients needs to be further explored to clarify its mechanism of action.
Characteristics of organic carbon mineralisation in soil aggregates from quinoa fields
The formation of aggregates is the result of the interaction between soil particles and cementing substances. The quantity and nature of these substances influence the stability of the aggregates. Fragmentation of soil aggregates leads to the decomposition of organic matter, while ideal aggregate composition promotes the sequestration of soil organic matter (Krause et al., 2020; Li et al., 2023a). The physical protection effect of soil aggregates is favorable to soil organic carbon sequestration, >2 mm and 0.25–2 mm particle size aggregates have higher content of organic carbon and carbon fractions, and crop cultivation mainly affects the accumulation of organic carbon in coarse and fine aggregates, which is consistent with the trend of changes in the distribution of aggregates in different soil textures after quinoa cultivation in this study.The study showed that the application of organic fertilizers increased the organic carbon content of aggregates of all grain sizes significantly higher than that of chemical fertilizers, and the application of organic fertilizers increased the organic carbon content of aggregates of 0.25–2 mm grain size significantly higher than that of other grain sizes under the same conditions of different grain sizes (Zhao et al., 2021). However, in this experiment, the test did not show this result, which may be because in this experiment, only bio-organic fertilizer was applied as a base fertilizer before quinoa planting, and no organic fertilizer was used during the growth and development process, and chemical fertilizer was used for follow-up.
Organic carbon is composed of two distinct categories: those that are readily mineralizable and those that are less so. The decomposed organic carbon that was incubated indoors for 28 days predominantly fell into the readily mineralizable category. The mineralization of soil organic carbon is closely related to aggregates, and the size of aggregates can affect the mineralization rate of soil organic carbon, which in turn affects the cumulative mineralization of soil organic carbon (Li et al., 2023b). Due to the low stability of 2–0.25 mm aggregates, which contain more reactive organic carbon and are easy to decompose, resulting in a higher rate of organic carbon mineralization, and therefore a higher cumulative mineralization. This study further found that the cumulative mineralization of organic carbon in clay loam soil planted with quinoa was significantly higher than that in other soil textures. Although sandy soil has good permeability, the high content of sand is not conducive to the formation of aggregates, which affects the microbial species and activity and thus the mineralization rate of soil organic carbon. Variables such as soil moisture, temperature, and the length of the incubation period can affect the mineralization of organic carbon. In this study, we used a short incubation period of 28 days with constant soil moisture and temperature, which may not reflect long-term or variable conditions but may provide some reference for the study of the soil environment of quinoa fields.
C0/SOC value represents the proportion of organic carbon consumed by The decomposition of soil organic carbon mineralization in the soil can be used to assess the strength of the soil’s carbon sequestration capacity. A lower proportion indicates a stronger capacity, while a higher proportion indicates a weaker capacity (Hu et al., 2024). In this study, under the same soil texture, the C0 and C0/SOC values of small aggregates were lower (p < 0.05), indicating that small aggregates had a stronger carbon sequestration capacity, which was due to the differences in the cementing substances in the agglomeration process of aggregates, which resulted in varying degrees of physical protection of organic carbon. The large-size aggregates are easily decomposed transiently cemented organic matter, while the microaggregates are mostly persistent cement. Macroaggregates contain a greater proportion of readily decomposable reactive organic carbon, whereas microaggregates comprise a higher concentration of highly humified inert organic carbon (Song et al., 2024). Considering from the perspective of particle size, microbial sources of humic substances are more and combine with sticky particles, organic carbon in small-sized aggregates is mainly chemically protected and decomposes slowly, which is conducive to long-term preservation, while organic carbon in large-sized aggregates is mainly physically protected, and plant-sourced organic carbon is more and turns over more quickly, and is sensitive to the environment (Qiang et al., 2022).The quantity of CO₂ lost due to the mineralization (respiration) of organic carbon in soil aggregates is primarily derived from the decomposition of organic carbon within large aggregates. Consequently, the mechanism of CO₂ production by soil respiration is subject to variation by the composition of soil aggregates.In this study, except for the quinoa field in clay loam soil, the k value of the organic carbon pool of 2–0.25 mm aggregates was the highest (p < 0.05) under the same cropping pattern. This may be attributed to the substantial quantity of organic carbon in an easily oxidizable state that has aggregated in the particle group of large aggregates measuring 2–0.25 mm. Consequently, the organic carbon present in these aggregates exhibits reduced stability and a more rapid turnover.
Conclusion
In the northern foothills of the Yinshan Mountains in Inner Mongolia, growing quinoa changed the soil aggregate structure and reduced soil bulk density. Among the four soil textures (sand, sandy loam, loam and clay loam), planting quinoa in loamy soil had the most obvious effect on promoting the formation of soil macroagglomerates, while the increase in organic carbon content was also the greatest. In actual agricultural production, priority can be given to planting quinoa in loamy soils, which is conducive to the improvement of soil structure and the accumulation of organic carbon, and to the sustainable development of the northern foothills of the Yinshan Mountains in Inner Mongolia.
Data availability statement
The original contributions presented in the study are included in the article/supplementary material, further inquiries can be directed to the corresponding author.
Author contributions
SL: Conceptualization, Data curation, Formal Analysis, Investigation, Methodology, Validation, Writing–original draft, Writing–review and editing. RL: Conceptualization, Funding acquisition, Investigation, Methodology, Project administration, Resources, Supervision, Validation, Writing–review and editing. ZG: Funding acquisition, Investigation, Project administration, Supervision, Validation, Writing–review and editing. SW: Funding acquisition, Investigation, Resources, Supervision, Validation, Writing–review and editing.
Funding
The author(s) declare that financial support was received for the research, authorship, and/or publication of this article. This research was funded by Hohhot Application Technology Research and Development Fund Project (2022-Agriculture-5); Central Leading Local Science and Technology Development Fund Project (2022ZY0138); Oat and Quinoa Industry Technology Innovation Promotion System in Inner Mongolia Autonomous Region; Hohhot Application Technology Research and Development Fund Project (2023-Agriculture-1).
Conflict of interest
Author ZG was employed by Inner Mongolia Yiji Biotechnology Co. Ltd.
The remaining authors declare that the research was conducted in the absence of any commercial or financial relationships that could be construed as a potential conflict of interest.
Publisher’s note
All claims expressed in this article are solely those of the authors and do not necessarily represent those of their affiliated organizations, or those of the publisher, the editors and the reviewers. Any product that may be evaluated in this article, or claim that may be made by its manufacturer, is not guaranteed or endorsed by the publisher.
References
Cong, P., Ouyang, Z., Hou, R., and Han, D. (2016). Effects of application of microbial fertilizer on aggregation and aggregate-associated carbon in saline soils. Soil Tillage Res. 168, 33–41. doi:10.1016/j.still.2016.12.005
Dai, W., Feng, G., Huang, Y., Adeli, A., and Jenkins, J. N. (2024). Influence of cover crops on soil aggregate stability, size distribution and related factors in a no-till field. Soil Tillage Res. 244, 106197. doi:10.1016/j.still.2024.106197
Dong, L., Fan, J., Li, J., Zhang, Y., Liu, Y., Wu, J., et al. (2022). Forests have a higher soil C sequestration benefit due to lower C mineralization efficiency: evidence from the central loess plateau case. Agric. Ecosyst. and Environ. 339, 108144. doi:10.1016/j.agee.2022.108144
Espíndola canedo, G., Rodríguez, O., and Luis, J. (1988). Respuestas fisiológicas y del rendimiento de la quinua (chenopodium quinoa willd) a deficit hídrico. Agrociencia (74), 297–312.
González, J. A., and Gallardo, M. G. (1992). Efecto de algunos factores ambientales sobre la germinacion de chenopodium quinoa willd y sus posibilidades de cultivo en algunas zonas de la provincia de tucuman (argentina), 55–64. Lilloa.
He, X., Sheng, M., Wang, L., Zhang, S., and Luo, N. (2022). Effects on soil organic carbon accumulation and mineralization of long-term vegetation restoration in Southwest China karst. Ecol. Indic. 145, 109622. doi:10.1016/j.ecolind.2022.109622
Hu, L., Liu, X., Xie, Y., Zeng, Y., Ou, H., Yu, Y., et al. (2024). Bamboo charcoal application altered the mineralization process of soil organic carbon in different succession stages of karst forest land. Front. Environ. Sci. 12. doi:10.3389/fenvs.2024.1411122
Jordan, D., Bruce, R. R., and Coleman, D. C. (1996). Nitrogen availability to grain sorghum from organic and inorganic sources on sandy and clayey soil surfaces in a greenhouse pot study. Biol. Fertil. Soils 21, 271. doi:10.1007/s003740050060
Krause, L., Klumpp, E., Nofz, I., Missong, A., Amelung, W., and Siebers, N. (2020). Colloidal iron and organic carbon control soil aggregate formation and stability in arable Luvisols. Geoderma 374, 114421. doi:10.1016/j.geoderma.2020.114421
Lazicki, P., Lee, J., Mengistu, A., and Jagadamma, S. (2023). Drought, heat, and management interact to affect soil carbon and nitrogen losses in a temperate, humid climate. Appl. Soil Ecol. 189, 104947. doi:10.1016/j.apsoil.2023.104947
Li, J., Chen, P., Li, Z., Li, L., Zhang, R., Hu, W., et al. (2023a). Soil aggregate-associated organic carbon mineralization and its driving factors in rhizosphere soil. Soil Biol. Biochem. 186, 109182. doi:10.1016/j.soilbio.2023.109182
Li, J., Chen, P., Li, Z., Li, L., Zhang, R., Hu, W., et al. (2023b). Soil aggregate-associated organic carbon mineralization and its driving factors in rhizosphere soil. Soil Biol. Biochem. 186, 109182. doi:10.1016/j.soilbio.2023.109182
Li, M., Li, X., Shi, Y., Jiang, Y., Xue, R., and Zhang, Q. (2024). Soil enzyme activity mediated organic carbon mineralization due to soil erosion in long gentle sloping farmland in the black soil region. Sci. Total Environ. 929, 172417. doi:10.1016/j.scitotenv.2024.172417
Liu, L., Wang, H., and Dai, W. (2018). Characteristics of soil organic carbon mineralization and influence factor analysis of natural Larix olgensis forest at different ages. J. For. Res. 30, 1495–1506. doi:10.1007/s11676-018-0724-4
Maestro-gaitán, I., Granado-Rodríguez, S., Redondo-Nieto, M., Battaglia, A., Poza-Viejo, L., Matías, J., et al. (2023). Unveiling changes in rhizosphere-associated bacteria linked to the genotype and water stress in quinoa. Microb. Biotechnol. 16, 2326–2344. doi:10.1111/1751-7915.14337
Ndzelu, B. S., Dou, S., Zhang, X., Zhang, Y., Ma, R., and Liu, X. (2021). Tillage effects on humus composition and humic acid structural characteristics in soil aggregate-size fractions. Soil Tillage Res. 213, 105090. doi:10.1016/j.still.2021.105090
Qiang, S., Xu, Y., Jun, M., Yu, L., Xiaori, H., Wenfu, C., et al. (2022). Long-term effects of straw and straw-derived biochar on humic substances and aggregate-associated humic substances in Brown earth soil. Front. Environ. Sci. 10. doi:10.3389/fenvs.2022.899935
Ritschel, T., and Totsche, K. U. (2019). Modeling the formation of soil microaggregates. Comput. and Geosciences 127, 36–43. doi:10.1016/j.cageo.2019.02.010
Rodríguez gómez, M. J., Matías Prieto, J., Cruz Sobrado, V., and Calvo Magro, P. (2021). Nutritional characterization of six quinoa (Chenopodium quinoa Willd) varieties cultivated in Southern Europe. J. Food Compos. Analysis 99, 103876. doi:10.1016/j.jfca.2021.103876
Sierra, C. A., Crow, S. E., Heimann, M., Metzler, H., and Schulze, E. (2021). The climate benefit of carbon sequestration. Biogeosciences 18, 1029–1048. doi:10.5194/bg-18-1029-2021
Song, X., Yuan, Z., Fang, C., Hu, Z., Li, F., Sardans, J., et al. (2024). The formation of humic acid and micro-aggregates facilitated long-time soil organic carbon sequestration after Medicago sativa L. introduction on abandoned farmlands. Geoderma 445, 116889. doi:10.1016/j.geoderma.2024.116889
Varma, A. (2021). Biology and biotechnology of quinoa: super grain for food security. Singapore: Springer.
Vilcacundo, R., and Hernández-Ledesma, B. (2016). Nutritional and biological value of quinoa (Chenopodium quinoa Willd.). Curr. Opin. Food Sci. 14, 1–6. doi:10.1016/j.cofs.2016.11.007
Weidhuner, A., Hanauer, A., Krausz, R., Crittenden, S. J., Gage, K., and Sadeghpour, A. (2020). Tillage impacts on soil aggregation and aggregate-associated carbon and nitrogen after 49 years. Soil Tillage Res. 208, 104878. doi:10.1016/j.still.2020.104878
Wieme, R. A., Reganold, J. P., Crowder, D. W., Murphy, K. M., and Carpenter-Boggs, L. A. (2020). Productivity and soil quality of organic forage, quinoa, and grain cropping systems in the dryland Pacific Northwest, USA. Agric. Ecosyst. and Environ. 293, 106838. doi:10.1016/j.agee.2020.106838
Zhao, B., Zhang, J., Yu, Y., Karlen, D. L., and Hao, X. (2016). Crop residue management and fertilization effects on soil organic matter and associated biological properties. Environ. Sci. Pollut. Res. 23, 17581–17591. doi:10.1007/s11356-016-6927-3
Keywords: northern Yinshan Mountains, quinoa, soil aggregates, organic carbon mineralization, kinetic equations
Citation: Lv S, Liu R, Guo Z and Wang S (2024) Characteristics of soil aggregate distribution and organic carbon mineralization in quinoa fields with different soil textures in the northern of the Yinshan Mountains in inner Mongolia. Front. Environ. Sci. 12:1494983. doi: 10.3389/fenvs.2024.1494983
Received: 11 September 2024; Accepted: 18 November 2024;
Published: 29 November 2024.
Edited by:
Wakene Negassa, The James Hutton Institute, United KingdomReviewed by:
Haojie Liu, University of Rostock, GermanyAna Cruz Morillo Coronado, Teacher Universidad Pedagogica y Tecnologica de Colombia, Colombia
Copyright © 2024 Lv, Liu, Guo and Wang. This is an open-access article distributed under the terms of the Creative Commons Attribution License (CC BY). The use, distribution or reproduction in other forums is permitted, provided the original author(s) and the copyright owner(s) are credited and that the original publication in this journal is cited, in accordance with accepted academic practice. No use, distribution or reproduction is permitted which does not comply with these terms.
*Correspondence: Ruixiang Liu, bGl1cnVpeEAxMjYuY29t