- 1School of Economics and Management, Beijing University of Posts and Telecommunications, Beijing, China
- 2RCV College of Business Administration and International Study Programs, The University of Texas Rio Grande Valley, Edinburg, TX, United States
The transition from a carbon-intensive economy to a carbon-neutral one has become a critical global objective to address climate change. This study examines the relationship between Low-Carbon Economic Development (LCED) and Geopolitical Risk (GPR) in China by focusing on the challenges and opportunities they present. We use a qualitative analysis to identify that rising GPR, exacerbated by market instability, resource allocation conflicts, and trade disputes, significantly hinders LCED progress. However, these geopolitical tensions also act as a catalyst for accelerating the development of renewable energy, reducing reliance on traditional energy sources, and fostering low-carbon technologies. Furthermore, LCED can ameliorate GPR by decreasing dependence on energy imports, promoting international cooperation, and encouraging scientific innovation. These findings suggest actionable policy recommendations to support the global transition to a low-carbon economy. This research underscores the potential LCED has as a fundamental tool for resolving geopolitical tensions and uniting global efforts to combat climate change.
1 Introduction
1.1 The relationship between LCED and GPR worldwide
This study investigates the linkages between LCED and GPR. When facing up to address the global challenge of climate change, the transition towards a LCED experiences a growing appeal among countries (Ren et al., 2023a; Xing et al., 2023). While low-carbon economic transitions are seen as a pathway to environmental sustainability (Li et al., 2024; Zhao et al., 2023a), they are deeply enmeshed in the processes of national economic restructuring, industrial transformations, and regional energy resilience (Tong et al., 2023; Zheng et al., 2021). These impact the whole of the geopolitical arena for that matter (Hunjra et al., 2024). Low-energy, low-pollution, low-emission economic model accords with the consensus of the international community to combat global warming and achieve green development (Chen et al., 2024a; Meng et al., 2023). Some countries, previously strained in their geopolitical relationships, have managed to ease and improve them through cooperation in the low-carbon realm (Andrews-Speed et al., 2014). For instance, China and the United States released a “U.S.-China Joint Statement on Climate Change”, pledging to lower greenhouse gas emissions together (Jayaraman, 2014). The cooperation in this area has helped build the Paris Climate Accords of 2015 (Cheng, 2020) and has had a calming effect on the fraught geopolitical relations between two countries (Liu et al., 2023). Nonetheless, the intricacies of domestic policies required to develop a low-carbon economy are commonly finding ground in international policy disagreements and trade disputes (Ren et al., 2024; Böhringer et al., 2022). In other words, GPR is influenced by these deviations and conflicts as well as global interaction, government stability, and policy change (Caldara and Iacoviello, 2022).
For example, the European Union’s efforts to abate carbon leakage through the planned Carbon Border Adjustment Mechanism (CBAM) met with much opposition to the extent of aggravating global tension (Sun et al., 2023). In turn, GPR also influence energy strategies, economic paradigms, and global governance frameworks (Ugurlu-Yildirim and Ordu-Akkaya, 2022; Scheffran, 2023). In effect, they present a double challenge to the transition to a low-carbon economy. Over the past several decades, as efforts to reign in carbon dioxide (CO2) emissions have deepened, resource management has become a flashpoint for international tension and the basis for new geopolitical games (Shang and Luo, 2021; Blondeel et al., 2021; Zhang et al., 2021). Thus, the question “Is Low-Carbon Development Mitigating or Exacerbating Geopolitical Conflicts?” justifies an in-depth investigation. Furthermore, the March toward low-carbon economic growth goes well beyond traditional environmental and economic spheres, rising up to become a key driving force in the global geopolitical context.
1.2 The LCED and GPR in China
China offers an ideal substrate for studying the above issues. Firstly, from a geographic viewpoint, it is bordered by 14 nations via land and 8 additional countries by means of the sea, boasting the hold of vital international sea routes for trade like the South and East China Seas (Wang et al., 2021). This unique location exposes China to far more intricated geopolitical wrinkles over relative border and regional security issues (Zhao et al., 2021). Secondly, it is the largest importer of oil and natural gas and the largest emitter of CO2 in the world (Li et al., 2023a; Chen et al., 2022a; Huang et al., 2023). As such, its energy security and economic stability are exposed to the fluctuations of the global energy markets and geopolitics (Song et al., 2019; Li et al., 2020a) if chaos in the Middle East or some other international tension would affect China’s energy supply chain (Ren et al., 2023b). In order to decrease its dependence and reduce GPR, China has initiated a number of measures such as promoting low-carbon development, initiate energy mix, raise energy efficiency, develop renewables, and move towards sustainable economy (Xin-Gang and Ying, 2023; Qi et al., 2023a). Lastly, amidst the ever-shifting global geopolitical dynamics, China’s political and economic advancements have gained attention and resistance from the United States and its allies (Lippert et al., 2020). Scholars concur that China is balancing conflicting geopolitical environments (Almujeem, 2021; Rogelja and Tsimonis, 2020). Western countries use their advantages in energy and low-carbon technologies to increase R&D costs for low and middle-income countries through mechanisms like carbon taxes to maintain their global economic dominance (Nong et al., 2021). In contrast, China’s “30–60” vision—peaking CO2 emissions by 2030 and achieving carbon neutrality by 2060—demonstrates its commitment to adjust its energy structure and lead a new global energy revolution (Ren et al., 2022). By establishing its own standards for calculating carbon footprints and enhancing its CO2 discourse, China would improve its bargaining position internationally. It can also use the historical carbon debt of the developed countries as a bargaining chip (Zhao et al., 2022). This mixture of intertwined LCED and GPR confirm China as the ideal sample compared to the rest of the world for offering a unique insight into this linkage.
1.3 Theoretical analysis of the relationship between LCED and GPR
To deepen the understanding of the intricate interplay between Low-Carbon Economic Development (LCED) and Geopolitical Risk (GPR), this study introduces a conceptual model grounded in environmental economics and international relations theories. The “Double Dividend Hypothesis” in environmental economics (Bovenberg and De Mooij, 1994) suggests that policies promoting LCED have a dual benefit: not only do they reduce greenhouse gas emissions, but they also stimulate economic growth through innovation and efficient resource allocation. Specifically, technological innovation plays a crucial role in this process, as advancements in renewable energy, energy efficiency, and carbon capture technologies help mitigate GPR. By fostering energy independence and reducing reliance on volatile fossil fuel markets, LCED becomes a stabilizing force in geopolitics, helping to mitigate conflicts over energy resources. This process also includes energy security adjustments, wherein countries diversify their energy sources to ensure stability and security, reducing vulnerability to geopolitical tensions and external disruptions in energy supply chains. Furthermore, international cooperation in climate governance, such as agreements like the Paris Climate Accords, enhances diplomatic relations and contributes to easing tensions between nations with competing energy interests (Cheng, 2020). As nations work together to achieve shared environmental goals, the potential for conflicts driven by resource competition diminishes.
On the other hand, the “Complex Interdependence” theory in international relations (Keohane and Nye, 1973) underscores the interconnectedness of global economic and environmental systems. This interconnectedness often transforms competitive dynamics into cooperative relationships. For instance, nations investing in low-carbon technologies often form strategic partnerships, promoting technology sharing and resource pooling. These collaborations not only reduce the likelihood of conflict but also enhance the mutual benefits derived from shared innovation in green energy. However, this interaction is not entirely unidirectional. High levels of GPR can disrupt LCED by creating uncertainty in energy markets, delaying cross-border investment, and imposing barriers to the transfer of green technologies (Scheffran, 2023; Zhao et al., 2021). Moreover, geopolitical tensions can inadvertently accelerate LCED development, as countries strive to reduce dependence on external energy sources and invest in building resilient energy infrastructures. These adjustments, driven by geopolitical risks, push countries to innovate and enhance their energy security through technological and policy-driven strategies.
This conceptual model underscores the bidirectional and time-sensitive nature of LCED and GPR. It highlights the need for a comprehensive approach that integrates environmental and geopolitical strategies to achieve sustainable development while mitigating risks. Figures 1, 2 visually illustrate this dynamic, showing how LCED and GPR interact through mechanisms of mitigation, collaboration, and disruption. This framework provides a theoretical foundation for the subsequent analysis of China’s experience and policy implications.
1.4 Contribution of the study
This study offers the following contributions to the extant literature. While previous research has discussed the impact of LCED on GPR (Wang et al., 2023; Hunjra et al., 2024), LCED is a gradual and non-static process (Ding et al., 2023). Even though many countries have recently turned their attention to low-carbon development, there is a lack of research on the long-term effects of LCED on GPR. Our study fills this gap by exploring the bidirectional causality between China’s LCED and GPR over a ten-year period. It delves deeper into how the long-term implementation of its low-carbon economic policies has changed China’s position in the global energy market and how this shift has affected its political relations with other major energy producing and consuming countries. Secondly, to more accurately capture the time-varying features of the causal connection between the two, we use a monthly sub-sample rolling window causality test to unveil their changing interrelationships over the 2013–2023 period. We also adopted the GPR index proposed by Caldara and Iacoviello in 2017, which captures the intensity of geopolitical events, to more accurately measure the state of development of GPR. We chose the China Low Carbon Index, a representative sample of 40 companies' securities in the LCED sector, to more comprehensively reflect the overall performance of LCED. Through full-sample and sub-sample tests, we identified a bidirectional causal relationship between the two across several sub-periods. This signals that GPR have both advantageous and disadvantageous effects on LCED. In turn, LCED has a negative effect on GPR. Ultimately, as China vigorously pushes forward with its low-carbon transformation, the situation of geopolitical tensions has been alleviated. Finally, this study will provide strategic recommendations for policymakers to better understand the impact of China’s LCED on GPR and how to advance the LCED agenda globally while safeguarding the country’s energy security and economic interests.
This study is structured as follows: Section 2 unveils the existing research. Sections 3, 4 present the methodologies and data. In Section 5, the quantitative analyses are discussed. Section 6 sums up the conclusions and recommendations.
2 Literature review
The notion of a low-carbon economy (LCED) has gained widespread recognition as a critical strategy to mitigate greenhouse gas emissions and address the multifaceted challenges of climate change (Tong et al., 2020). However, a growing body of literature highlights the evolving conflicts of interest between LCED and geopolitical risk (GPR), which have become central to international competition (Rasoulinezhad et al., 2020; Feng et al., 2024; Safi et al., 2023). These geopolitical tensions are not limited to energy disputes but also encompass issues such as carbon pricing and the allocation of carbon emission rights, with implications for both international relations and economic strategies (Wang et al., 2022a). Consequently, the dynamic interplay between LCED and GPR has garnered significant attention from environmental economists and policymakers, who now recognize its complexity and relevance in shaping global sustainability (Ladislaw et al., 2015; Wang and Liu, 2015).
On one hand, the transition toward a low-carbon economy has prompted a shift in the geopolitical landscape, as countries strive to secure resources vital for green technologies, such as critical minerals used in batteries, wind energy, and photovoltaics. This resource competition has introduced new geopolitical risks, particularly in regions rich in minerals like lithium, cobalt, and rare earth elements (Cui et al., 2023). Nakano (2021) emphasizes that these materials are predominantly sourced from a small number of low- and middle-income countries, creating supply chain vulnerabilities and exacerbating geopolitical tensions. In fact, research by Bannon and Collier (2003) demonstrates that resource wealth can fuel geopolitical conflict, while Burgis (2015) highlights how mineral wealth in the Democratic Republic of Congo has led to armed conflict and long-term instability, further underscoring the link between resource dependence and GPR. Additionally, the “resource curse” hypothesis (Auty, 2002) provides valuable insight into how countries rich in natural resources are often plagued by political instability and rent-seeking behavior, which heightens their vulnerability to geopolitical crises.
However, there are contrasting views on the impact of the low-carbon transition on GPR. Several studies have suggested that the proactive development of renewable energy and the diversification of energy sources can mitigate geopolitical risks. For example, the transition to low-carbon energy can reduce a nation’s dependence on fossil fuel imports, making it less vulnerable to market fluctuations and geopolitical conflicts associated with resource scarcity (Newbery et al., 2018). Gozgor and Paramati (2022) argue that energy diversification enhances regional stability, while Siciliano et al. (2021) assert that renewable energy promotes energy self-sufficiency and strengthens international relations by reducing dependence on finite energy sources. This shift towards renewables is not just a strategic necessity for climate change mitigation but also a means to foster technological innovation, as evidenced by the Belt and Road Initiative, which has facilitated clean energy cooperation between China and regions like Central Asia and Southeast Asia (Liu et al., 2022; Xu et al., 2023). Such cooperation has not only contributed to technological advancements but has also enhanced regional energy security, offering a model for countries seeking to reduce their geopolitical risks through energy transition.
In contrast to the growing body of research emphasizing the complexity and variability of GPR, recent studies have focused on the temporal aspects of this relationship. The time-dependent variability of LCED’s impact on GPR has been largely overlooked in existing literature, particularly in the case of China. Research by Zhao et al. (2021), using the Nonlinear Cointegrating Autoregressive Distributed Lag (NARDL) approach, found that GPR’s impact on carbon pollution can behave differently across countries, such as those in the BRICS group. Similarly, Adams et al. (2020) pointed out that while geopolitical tensions may initially lead to a temporary increase in CO2 emissions, the long-term effects tend to favor green energy investment and technological advancement, thus eventually reducing emissions. This “investment effect” has been further supported by Sweidan (2021), who noted that changes in geopolitical risk could spur countries to increase their investments in green technologies, thus mitigating the negative impact on CO2 emissions.
From a theoretical perspective, the resource dependence theory (Pfeffer and Salancik, 2015) highlights the vulnerability of countries that rely heavily on finite natural resources, making them prone to external shocks and geopolitical conflicts. Such dependencies often lead to political alliances or trade-offs, further aggravating geopolitical tensions. Conversely, the low-carbon transition can reduce this dependence, helping countries avoid the pitfalls of the resource curse while promoting greater economic and political stability. As a global strategy, the low-carbon economy offers a unique opportunity to reshape GPR by diversifying energy supplies and promoting technological innovation, thereby contributing to both environmental and geopolitical security.
Despite these advances, three critical knowledge gaps persist. First, existing studies predominantly treat the LCED-GPR relationship as static, neglecting its time-dependent dynamics and country-specific heterogeneity. Second, the mechanisms through which short-term geopolitical conflicts translate into long-term low-carbon transitions remain underexplored, particularly in resource-dependent economies like China. Third, there is limited empirical evidence on how geopolitical risks influence green technology investments and their subsequent impact on carbon emissions. This paper addresses these gaps by (1) employing a dynamic analytical framework to capture temporal variations in the LCED-GPR nexus, (2) integrating regional case studies to reveal context-specific pathways, and (3) examining the role of geopolitical risks in driving green technology investments and their long-term effects on emissions reduction. Through this approach, we provide new insights into optimizing the geopolitical dividends of low-carbon transitions while mitigating associated risks.
3 Methodology
This study employs the rolling-window Granger causality test to investigate the dynamic and bidirectional relationship between LCED and GPR in China. By using a combination of bootstrap full-sample Granger causality test, parameter stability test and sub-sample rolling window causality test, we aim to uncover time-varying interactions and establish actionable insights. The methodological structure is designed to ensure statistical rigor and robustness.
3.1 Research hypothesis
The relationship between LCED and GPR is conceptualized within a feedback loop framework. On the one hand, GPR can disrupt LCED by creating economic and trade uncertainties, hindering investments in renewable energy and low-carbon technologies. On the other hand, advancements in LCED can reduce geopolitical tensions by fostering energy independence, technological innovation, and cross-border cooperation. To formalize this relationship, we test the following hypotheses:
H01: LCED does not Granger cause GPR.
If LCED influences GPR, it implies that low-carbon strategies, such as reducing reliance on fossil fuels or increasing renewable energy investments, have significant geopolitical implications, potentially alleviating tensions over resource allocation and energy security.
H02: GPR does not Granger cause LCED.
If GPR influences LCED, it suggests that geopolitical uncertainties, such as trade wars or resource conflicts, drive countries to accelerate their energy transitions to enhance resilience and reduce vulnerability.
3.2 Bootstrap full-sample causality test
Studies within the classical vector autoregression (VAR) framework present certain limitations. Firstly, the statistics used for testing Granger causality need to follow a specific asymptotic distribution law. Otherwise, the analysis results may be biased (Sims, 1980; Qin et al., 2023a; Chen et al., 2024b). The Residual Bootstrap (RB) technique was here adopted to circumvent this problem. This technique is suitable for Granger causality tests that do not conform to the standard normal distribution (Shukur and Mantalos, 2004). Additionally, in studies with insufficient sample sizes, using Monte Carlo simulations to optimize the Wald test method also introduces errors (Shukur and Mantalos, 2004). The Likelihood Ratio (LR) test proposed by Shukur and Mantalos in 2000 offers a more accurately correct the model when dealing with small to medium samples (Shukur and Mantalos, 2000). Therefore, this study employs the modified LR test based on RB technology to explore the correlation between LCED and GPR. The following Equation 1 shows the bivariate VAR (p) system for this study:
where the p-value is chosen according to the Schwartz Information Criterion (SIC), which indicates the optimal lag order. We denote the variable X as:
The error term
Where
3.3 Stability test of parameters
It is generally believed that the coefficients of the VAR model used in full-sample causality tests remain constant over the observation period (Su et al., 2020). In practice, however, these coefficients are often unstable, which may lead to biased results. To address this problem, Andrews (1993) and Plobberger (1994) proposed three methods: the Sup-F, Ave-F, and Exp-F. Among them, the Sup-F is mainly used to detect mutations in the model structure, while the Ave-F and Exp-F are used to assess the stability of the parameters. In addition, the LC test proposed by Nyblom (1989) helps to determine whether the coefficients exhibit random wandering. Due to the variation of coefficients over time, the relationship between LCED and GPR may also become unstable. In this case, using a full-sample approach for testing may not yield accurate results. Therefore, this study turns into a sub-sample approach to more accurately capture the evolution of the dynamic relationship between LCED and GPR.
3.4 Sub-sample rolling window causality test
Balcilar et al. (2010) proposed a rolling window method that allows a complete time series to be divided into multiple smaller sub-sequences. These sub-sequences can be rolled sequentially and accurately identify the time-varying characteristics between variables (Balcilar et al., 2010). Moreover, when performing rolling window estimation, a balance needs to be found between parameter accuracy and the representativeness of the sub-samples. Choosing an appropriate window size is crucial: a larger window improves estimation precision but may make the subsample less representative, whereas a smaller window has the opposite effect. Pesaran and Timmermann (2005) addressed the issue of parameter instability in their 2005 study by proposing a restriction on the minimum width of the window. In this study, we first set the total length of the time series to be T and the width of the rolling window to be r. Thus, the length of each sub-sequence is r, and the starting points of the sequence are, in order, r, r+1, … , t, yielding a total of T-r+1 time series. Next, for every sub-sample, we used the RB-based revised-LR technique in order to figure out their causal link. Finally, we summarized the LR statistics and p-values of these sub-samples in chronological order. The mean
4 Data
We selected monthly data from 2013.1 to 2023.12 to examine the correlation between China’s LCED and GPR. This time frame was selected because China has undertaken several of significant regulations and actions pertaining to climate change adaptation which have had profound impacts on global low-carbon development. These include the establishment of the National Low-Carbon Day (2013), the signing of the Paris Agreement (2016), the introduction of the “Two Carbon” target (2020), the launch of the national carbon market (2021), the release of the Glasgow Climate Agreement (2021), and the publication of the Green Finance Development Plan (2023). In this paper, we have chosen an index that reflects the performance of stocks of listed companies related to China’s LCED - CS Low Carbon Index. This LCED Index visualizes the changes in economic activities in this sector and better reflects China’s LCED (Chen et al., 2024c). In addition, China experienced a series of geopolitical changes and international relations adjustments during this period. In 2013, President Xi Jinping announced the “BRI” project, which strengthened China’s economic ties with the countries along the route but also triggered concerns in the West (Rolland, 2017). In 2014, China’s construction of islands in the South China Sea led to tensions with neighboring countries, particularly The Philippines and Vietnam (Rolland, 2017). The South China Sea triggered tensions with neighboring countries, especially disputes with The Philippines and Vietnam (Biedzynski, 2015). In 2018, the trade war between China and the U.S. increased transnational economic uncertainty (Park, 2020). In 2020, the outbreak of the global COVID-19 pandemic had profound impacts on the social, legal, economic, political, and technological dimensions around the world (Teng et al., 2020; Shaik et al., 2023). In 2021, the US military’s withdrawal from Afghanistan altered the geopolitical landscape of South and Central Asia, impacting China’s peripheral security environment (Manish and Kaushik, 2023). The escalation of the Russia-Ukraine conflict in 2022 heightened global geopolitical tensions, and simultaneously affected China’s energy security (Zhou and Lu, 2023). This paper uses the GPR Index, a measure that assesses the threat of terrorism, trade disputes, and political tensions (Caldara and Iacoviello, 2022). Higher values of the LCED and GPR indices imply better development of China’s low-carbon economy and higher geopolitical risk. The trend of LCED and GPR is shown below:
Figure 3 displays the fluctuations in the LCED and GPR indices over the period from 2013 to 2023. The relationship between LCED and GPR is marked by several significant phases, reflecting both internal and external dynamics:
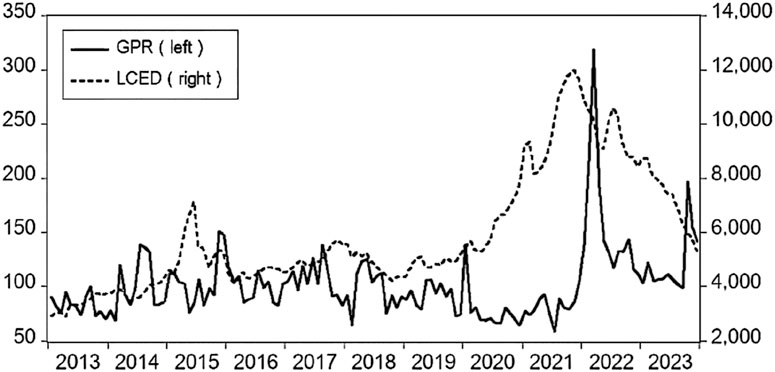
Figure 3. Trends of LCED and GPR. Notes: The dotted line on the right axis indicates the LCED trend while the solid line on the left axis represents the GPR trend. The x-axis represents years while and the y-axis represents the indexes of LCED and GPR.
2013–2015: A pivotal period for China as the country introduced the LCED policy, focusing on energy savings, CO2 emission reductions, and green energy development. The LCED index rose sharply from 2,915 to 7,133, indicating a positive response to these policies. However, this period also saw economic challenges, including a bear market, which caused the LCED index to fall to 4120 by 2015.
2016–2019: During this phase, the implementation of LCED policies matured, with a stabilizing effect on the LCED index. The LCED continued to show slow but steady growth as China’s low-carbon policies took root. In contrast, the GPR index saw some volatility, reflecting geopolitical tensions, particularly territorial disputes in the South China Sea, which increased GPR from 83 to 139.
2020–2021: The announcement of China’s carbon peak and carbon neutrality targets marked a new stage for LCED, driving a sharp rise in the LCED index. This was coupled with strong governmental support and market attention toward a carbon-neutral economy. At the same time, the GPR index fluctuated dramatically, with geopolitical events like North Korea’s nuclear tests and the COVID-19 pandemic further contributing to instability in global relations. These factors contributed to substantial shifts in GPR, culminating in a significant peak in 2021–2022.
2021–2023: Geopolitical factors, such as deteriorating Sino-American relations, the Russia-Ukraine conflict, and other global tensions, led to a sharp rise in GPR from 58 to 319, while the post-pandemic economic recovery drove a temporary resurgence in high-energy-consuming industries, negatively impacting the development of the low-carbon economy. This was reflected in a decline in the LCED index from 11,983 to 5,290, showing the negative impact of geopolitical instability on China’s green energy transition.
Table 1 provides the GPR and LCD descriptive statistics. These two have maximum values of 11,983.26 and 318.95, and minimum values of 2,900.190 and 58.42. Furthermore, the mean values of LCD vs GPR are 5,906.718 and 102.106, demonstrating the dramatic features of the chosen variables. Positive skewness reflects the right-skewed nature of LCD and GPR. Additionally, according to the Jarque-Bera test, these variables have a significant non-normal distribution. The use of Granger causality test based on VAR system causes a series of errors. Therefore, we used the RB-based revised-LR technique and sub-sampling technique to more accurately identify the changing transmission mechanism between LCD and GPR.
To ensure the robustness of the analysis, we conducted an outlier analysis on the LCED and GPR data. Outliers were identified using the Interquartile Range (IQR) method, where any data points falling below
5 Quantitative analyses and discussions
In this study, after taking first order differences for both LCD and GPR, we employed the ADF, PP, and KPSS tests to examine the stationarity of the two variables (Dickey and Fuller, 1981; Phillips and Perron, 1988; Kwiatkowski et al., 1992). From the test results shown in Table 2, we observe these two variables are essentially stationary. Therefore, we used LCED and GPR to construct a bivariate VAR model and examined the full-sample causal relationship between these two variables.
We set the optimal lag length to 6 based on SIC and the number of Bootstrap repetitions to 1,000. Table 3 below shows the results of the full-sample causal relationship between the two variables. We can observe that LCED and GPR do not significantly exhibit Granger causality. This represents that LCED cannot have any effect on GPR and vice versa. This outcome deviates from previous studies (Wang et al., 2022b; Borozan, 2024; Chen et al., 2024d).
Previous studies analyzed time series data under the assumption of no structural breaks and a single causal relationship (Balcilar et al., 2010). If structural mutations occur in the VAR (s) system, there must be an unstable Granger causality between LCED and GPR (Sun and Su, 2024). Thus, in order to address this issue, we examined the temporal stability of the LCED and GPR parameters in the aforementioned models through Sup-F, Ave-F, and Exp-F tests (Andrews, 1993; Andrews and Plobberger, 1994). Moreover, to determine if parameter changes follow a random walk, we employed the Lc test (Nyblom, 1989; Hansen, 2002). Table 4 demonstrates the results of the test.
The Sup-F test shows that the LCED, GPR and VAR (s) systems underwent structural changes at the 1% and 5% levels, respectively. Furthermore, the Exp-F and Ave-F tests proved that the parameters progressively alter with time. Meanwhile, the Lc test indicates that the original hypothesis is rejected at the 5% level. This means that the parameters do not follow a random walk process. These tests demonstrate that there is a constantly changing relationship between LCED and GPR. Therefore, this study employs a rolling window sub-sample method to accurately capture the time-varying relationship between LCED and GPR. We set the width for the rolling window to 241 months to improve the accuracy of the results. Figures 4–7 illustrate the rolling results of sub-samples from January 2015 to December 2023. From the test results, we observe whether the null hypothesis that LCED is not Granger cause of GPR or, conversely, GPR is not Granger cause of LCED is accepted or rejected at the 10% significance level. Additionally, we can the direction of their mutual influence.
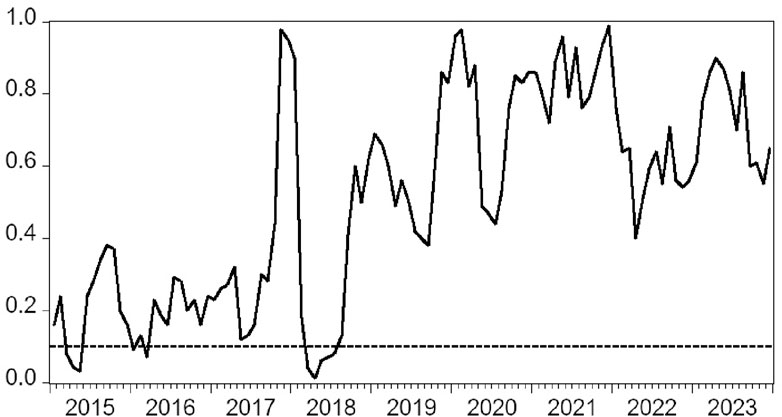
Figure 4. Examining the null hypothesis that LCED is not a Granger cause of GPR. Notes: The study uses 1000 bootstrap iterations to count p-values. The dashed line shows that the p-value is 0.1 and the solid line represents the Bootstrap p-values. The x-axis represents years while the y-axis represents the Bootstrap p-value.
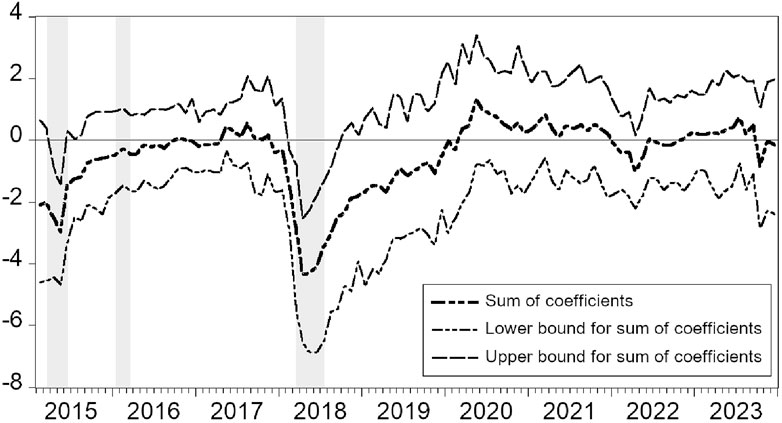
Figure 5. Coefficients of the influence from LCED to GPR. Notes: The period where NUC exhibits significant Granger causality to LCED which is represented by the shadow bars. The x-axis represents years while the y-axis represents the Sum of Rolling-Window Coefficients.
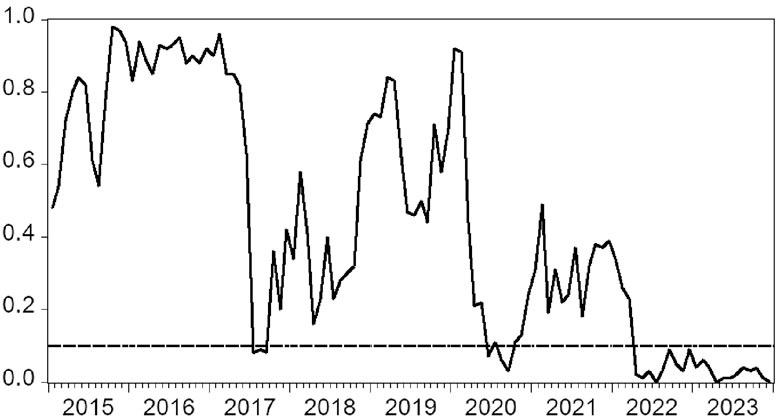
Figure 6. Examining the null hypothesis that GPR is not a Granger cause of LCED. Notes: The study uses 1,000 bootstrap iterations to count the p-values. The dashed line shows the p-value of 0.1 while the solid line represents the bootstrap p-values. The x-axis represents year while the y-axis represents the Bootstrap p-value.
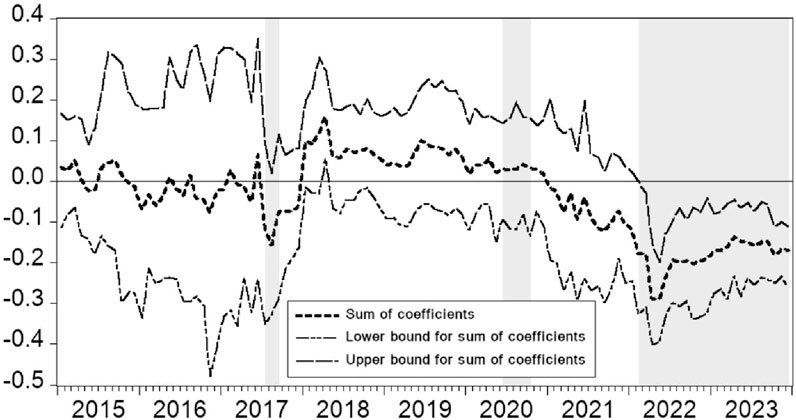
Figure 7. Coefficients of the influence from GPR to LCED. Notes: The period where NUC exhibits significant Granger causality to LCED which is represented by the shadow bar. The x-axis represents years while the y-axis represents the Sum of Rolling-Window Coefficients.
Figures 4, 5 indicate the original hypothesis that LCED does not Granger cause GPR is rejected at the 10% significance level from March 2015 to May 2015; from December 2015 to March 2016; and from March 2018 to July 2018, respectively. In all these periods, the influence of LCED on GPR is negative.
From March to May 2015, LCED grew significantly from 4,918 to 7,133, while GPR declined from 112 to 76. This period underscores how advancements in renewable energy and international cooperation in climate policy can mitigate geopolitical risks. First, building on the foundation of the 2014 “U.S.-China Joint Statement on Climate Change,” China made further commitments to reduce greenhouse gas emissions. In March 2015, multiple high-level meetings between the two nations deepened collaboration on climate change, which not only contributed to achieving global climate goals but also alleviated tensions in their bilateral relations (Lu and Zhu, 2022). This highlights how shared environmental objectives can serve as a platform for reducing geopolitical risks. Second, China substantially increased its investment in renewable energy. As reported by Sun (2020), the Inner Mongolia Autonomous Region launched several large-scale wind power projects during this period. These projects helped to diversify China’s energy mix and reduce dependence on coal and other traditional energy sources, thereby mitigating risks associated with global energy market volatility. By decreasing reliance on fossil fuels imported from geopolitically unstable regions, such investments significantly enhance energy security and reduce vulnerabilities to external shocks. Third, China’s leadership in establishing the Asian Infrastructure Investment Bank (AIIB) in 2015 further promoted green and sustainable infrastructure development across the region (Morris, 2023). A portion of the AIIB’s funds was allocated to renewable energy projects, fostering regional cooperation in low-carbon economic development and reducing tensions over energy resource competition. Additionally, China’s 863 Program (National High Technology Research and Development Program) accelerated the development of clean technology projects such as electric vehicles, battery storage, and smart grids (Su et al., 2015). For instance, the launch of BYD’s electric vehicle in April 2015 marked a significant milestone for the global electric vehicle industry, showcasing how technological advancements can reduce carbon footprints and contribute to global climate goals (Wang et al., 2017). These developments not only bolster China’s leadership in green technologies but also reduce reliance on fossil fuel-based energy, which has historically been a source of geopolitical conflict. These examples illustrate how LCED can act as a stabilizing force in the global geopolitical landscape by addressing underlying dependencies that fuel conflicts. However, they also highlight the importance of sustained investment and international cooperation to ensure that such progress remains resilient to geopolitical shocks.
Between December 2015 and March 2016, China’s low-carbon economy was further developed. This was mainly due to China’s active participation in climate change issues in the international arena. At the 2015 Paris Climate Change Conference, China worked with other countries to promote the Paris Agreement (Zhao et al., 2023b). This spirit of cooperation has enhanced trust with major economies and to some extent reduced the issue of geopolitical tensions arising from climate change. On this basis, China continues to participate in and promote international climate cooperation. In 2016, China launched collaboration initiatives in developing countries, including 1,000 climate change assistance slots, 100 coping and adapting programs, and 10 carbon-free demonstration areas. Additionally, China continued to advance international collaboration in sustainable energy and the construction of low-carbon smart cities (Weigel and Demissie, 2021). Furthermore, China and the nations along the BRI have inked several agreements for green energy cooperation (Cheng and Wang, 2023). For example, China’s green energy projects with Kazakhstan have promoted the development of renewable energy in Kazakhstan (Yesmurzayeva and Mrzabayeva, 2023). This has not only increased the energy autonomy of these countries but also reduced geopolitical tensions arising from energy dependence.
In contrast to the previous two periods, from March 2018 to July 2018, LCD and GPR exhibited completely opposite trends. This means that the growth of China’s green economy was hindered, leading to a rise in geopolitical risk. This was primarily due to the China-U.S. trade war. Following American taxes on Chinese commodities, China responded by imposing duties on American goods. This move led to the blocking of cooperation between the two countries in new energy technologies and clean energy equipment (Pepe et al., 2023). For example, the U.S. tariffs on Chinese photovoltaic (PV) products have made Chinese PV firms less competitive internationally and hindered the development of clean energy globally. In addition, the trade war has disrupted supply chains in both countries (Sullivan, 2022). It has also increased production costs and market uncertainty especially in the production of clean energy equipment and supply chain lines. The investment climate has also deteriorated, with international investors’ confidence in the markets of both countries declining. GPR were exacerbated by growing political tensions between the two countries. Meanwhile, in the first half of 2018, international oil prices experienced significant fluctuations causing a profound impact on China (Khan et al., 2021). The high costs and risks associated with finding alternative sources of oil made difficult for China to reduce its dependence on fossil fuels in the short term. This not only increased the difficulty of transitioning to renewable energy but also slowed the pace of developing a low-carbon economy. Furthermore, the economic pressures and energy supply risks associated with high oil prices have led to increased competition for China in the international energy market, as well as increased geopolitical tensions with other energy-importing countries (Gong et al., 2022).
Figures 6, 7 show the p-values and the direction of GPR’s influence on LCD. From July 2017 to September 2017; June to October 2020; and February 2022 to December 2023, the original hypothesis that GPR does not Granger lead to LCED is rejected at the 10% significance level. Only during June to October 2020 is the effect of GPR on LCED positive while in the other two periods the effect of GPR on LCED is negative.
China’s declining GPR in 2017 provided favorable conditions for the LCED. Reduced GPR usually mean more stable international relations. This facilitates the country’s focus on promoting internal economic transformation and sustainable development policies. A stable geopolitical environment bolstered the confidence of domestic and foreign investors in the Chinese market, leading to a large inflow of capital into the renewable energy sector (Guo and Shi, 2024). Between July and September 2017, several large-scale wind power and photovoltaic (PV) projects received financial support and accelerated construction (Sun, 2020). Additionally, the Chinese government expanded its backing for low-carbon technologies and renewable energy, such as wind energy, solar energy, and electric vehicles (Guilhot, 2022). The Chinese government further clarified subsidy standards and application processes to ensure the availability of funds and the smooth advancement of projects (Wu et al., 2022). For example, the National Energy Administration (NEA) issued the “2017 Photovoltaic Power Generation Construction Implementation Plan,” which provides clear subsidy support for new PV power generation projects (Li et al., 2020b). Local governments have also introduced purchase subsidy policies and charging infrastructure construction plans for electric vehicles, which have driven the rapid growth of the new energy vehicle market.
In complete contrast to the previous cycle, the rise in GPR from June to October 2020 has instead led to further LCED growth. This means that despite the rise in geopolitical risk due to global uncertainty due to the intensifying trade war between the US and China, and the global COVID-19 outbreak, it also promoted the development of China’s low-carbon economy to some extent. The uncertain international situation spurred China to accelerate the localization and diversification of its supply chains, particularly in low-carbon technologies and materials (Panwar et al., 2022). This strategic pivot aimed to reduce reliance on external instability while enhancing domestic capacities. First, China prioritized the development and utilization of its domestic rare earth resources, which are critical for renewable energy technologies such as wind turbines and electric vehicles. By introducing supportive policies for rare earth mining and processing enterprises, China secured key material supplies essential for its energy transition (Li et al., 2023b). Second, the localization of the battery materials industry chain became a focus. Substantial investments in lithium and cobalt production facilitated domestic production of key battery components, enhancing the autonomy and resilience of China’s new energy vehicle supply chain (Melin et al., 2021). Moreover, the Chinese government’s “dual circulation” development strategy, introduced during this period, emphasized mutual reinforcement between domestic and international markets (Tan et al., 2023). This strategy prioritized internal economic development, directing more resources toward low-carbon technologies and renewable energy, thereby accelerating the growth of related industries. Additionally, China’s international commitments, such as President Xi Jinping’s September 2020 pledge at the United Nations General Assembly to achieve carbon neutrality by 2060, provided further impetus. This commitment not only demonstrated China’s determination to combat climate change but also catalyzed domestic policy innovation and market demand for low-carbon infrastructure (Han et al., 2021; Tong et al., 2023). These developments underscore how heightened GPR can act as a catalyst for low-carbon investments, as countries seek to strengthen domestic resilience and reduce dependence on volatile global supply chains.
From 2022 to 2023, GPR has had a negative impact on LCED. We analyze it in three time periods. From February 2022 to April 2022, GPR sharply increased from 139 to 319, while LCD declines from 10,921 to 9,152. It stems largely from the expansion of the Russia-Ukraine conflict in February 2022. The conflict was not an only military concern in Europe but also had global effects on the political and economic grounds. The global energy market went into chaos, pushing oil and gas prices to the extreme legible limit (Balsalobre-Lorente, 2023). Being a big importer of global energy, China is confronted with the challenge of sporadic energy supply and prices, which in the short term, compels it to turn back more to conventional fossil fuels in order to guarantee energy security (Liu, 2024). The challenges and uncertainties for China to promote LCED were thus exacerbated. From April to July, international focus on the Russia-Ukraine conflict waned (Chen et al., 2022b). Oil and gas prices started to slip, and the energy market slowly stabilized. This to some extent reduced the uncertainty of energy supply in China and also re-configured the external environment for a low-carbon economy. In this context, the NEA released a series of policies to facilitate low-carbon economic development, including the “14th Five-Year Plan for a Modern Energy System,” which further supports investment and construction of wind and photovoltaic power plants (Yang et al., 2023). However, from July 2022 to December 2023, the GPR rose again, and the LCED declined. There are three reasons behind this phenomenon. First, in August 2022, U.S. Speaker of the House visited Taiwan. This act was regarded by China as a serious interference in China’s internal affairs, which led to a further deterioration of the U.S.-China relations (Al Obaidy, 2023). Moreover, China held extensive military drills across the Taiwan Strait, intensifying geopolitical tensions. Second, between 2022 and 2023, China and its neighbors’ territorial disputes in the South China Sea grew more intense. Countries like The Philippines sought international arbitration. Additionally, the U.S. and its allies conducted multiple “freedom of navigation” operations, sending warships and aircraft through disputed waters in the South China Sea, further escalating tensions (Murphy and Turek, 2024). Deteriorating diplomatic relations have hindered international cooperation on low-carbon technologies and environmental policies. As a result, multinational corporations and international organizations have delayed or cancelled investments in low-carbon projects in China. Moreover, tensions in the South China Sea have also affected the stability of global supply chains, increasing production costs and project implementation difficulties in low-carbon industries (Qin et al., 2023b). Finally, the U.S. and its allies imposed multiple technological blockades on China, particularly in high-tech products, advanced manufacturing, and new energy technologies (Piao, 2023). These sanctions include export bans on semiconductors and high-end chips, restrictions on communications equipment, and export restrictions on key manufacturing equipment, affecting China’s development of 5G networks and smart grids. For new energy technologies, the U.S. has restricted exports of solar energy, electric vehicle batteries, and wind power technologies, and put pressure on key raw material supply chains, increasing production costs (Canuto, 2023). These sanctions have significantly hindered China’s progress in low-carbon technology R&D, industry chain stabilization and international cooperation. Consequently, the development of the low-carbon economy experienced a downward trend during these 2 years.
6 Conclusion and recommendations
In this paper, we investigated the relationship between Low-Carbon Economic Development (LCED) and Geopolitical Risk (GPR) in China, emphasizing the dynamic interplay between these two factors. We first tested the relationship between the two using the full sample Granger causality test, followed by parameter stability tests that highlighted the continuously evolving nature of this relationship. To capture these dynamic effects more accurately, we applied a sub-sample rolling window causality estimation. The results revealed that LCED negatively impacts GPR, suggesting that the development of a low-carbon economy can reduce geopolitical risks. Conversely, GPR influences LCED in both positive and negative ways: while instability in the geopolitical environment can hinder LCED progress, rising geopolitical risks can also spur nations to reduce their dependency on external instability by accelerating the transition to a low-carbon economy.
This study not only sheds light on the domestic context of China’s low-carbon transition but also offers valuable insights for broader global transitions. The bidirectional relationship between LCED and GPR underscores the significance of reducing geopolitical dependencies to build resilient and sustainable economic systems. In the context of the global transition to circular economies and decarbonization, these findings carry substantial policy implications. Specifically, reducing reliance on finite and geopolitically sensitive resources such as rare earth elements and lithium is crucial. By promoting resource efficiency through recycling, reuse, and sustainable management, countries can mitigate tensions arising from the global competition for these materials. This aligns with the principles of a circular economy and offers a path toward greater global cooperation. For developing nations, China’s strategies—such as its investments in renewable energy, the localization of supply chains, and energy diversification—serve as important models for decarbonization pathways. By prioritizing renewable energy and building local industries for low-carbon technologies, nations can reduce their vulnerability to external shocks and improve their energy security. For example, China’s “dual circulation” strategy and Belt and Road Initiative (BRI) showcase how a combination of international cooperation and domestic policy alignment can drive low-carbon transitions while promoting regional stability. China’s commitment to carbon neutrality by 2060 further provides a compelling example for other developing nations, demonstrating how clear, long-term commitments can attract investment, incentivize technological innovation, and contribute to global climate goals. In conclusion, the findings from this study emphasize the broader geopolitical and economic implications of low-carbon transitions. By reducing geopolitical dependencies, fostering international cooperation, and aligning energy policies with sustainability goals, countries can pave the way for a more resilient, secure, and environmentally stable global economy. This research not only contributes to the understanding of LCED and GPR in the context of China but also offers valuable lessons for the ongoing global transition to sustainable development, offering pathways for mitigating geopolitical tensions while advancing climate goals.
The above-mentioned findings suggest the following policy recommendations. Firstly, governments should focus on diversifying the supply of energy and lowering reliance on a single energy source. To that end, they can increase investment in and development of renewable energy, especially renewable energy sources such as solar, wind and hydropower. In addition, countries should strengthen international cooperation on low-carbon technology and environmental policies through multilateral platforms and bilateral cooperation mechanisms. This will not only show China’s optimistic approach to combating climate change but also garner support and understanding from the international community to mitigate geopolitical conflicts. Secondly, at the corporate level, companies should implement a low-carbon supply chain management system and reduce their carbon footprint by selecting low-carbon suppliers and optimizing the supply chain structure. At the same time, ensuring the diversity and resilience of the supply chain can also effectively mitigate the potential threats due to the escalation of GPR. Finally, social organizations should encourage the public to take an active role in carbon-free initiatives, such as energy conservation and green travel. Governments can also support the implementation of low-carbon public behaviors through policy incentives, such as tax incentives and subsidies. Promoting the development of low-carbon economies within regions through community participation and local government support can enhance local resilience and mitigate the negative impacts of escalating GPR.
This study reveals the complex relationship between China’s Low-Carbon Economic Development (LCED) and Geopolitical Risk (GPR), but several limitations warrant further exploration. First, while the study utilizes existing LCED and GPR indices, these may not fully capture the impact of non-market factors, such as policy uncertainty or social dynamics, on their interaction. Future research could expand this framework by incorporating a broader set of indicators, including regional low-carbon project data, socio-economic variables, and other geopolitical or environmental factors, to uncover more nuanced and dynamic relationships at different scales and levels. Additionally, environmental variables such as climate-related risks (e.g., natural disasters or extreme weather events) could offer further insights into how environmental changes influence both LCED and GPR. Second, while the study is grounded in China’s experience, the generalizability of the findings to other countries or regions may be limited due to contextual differences in geopolitical and economic conditions. Future studies could explore the applicability of China’s low-carbon transition model to other regions, particularly emerging economies or those with varying levels of development. This research could provide tailored policy recommendations that consider local challenges, resource endowments, and geopolitical contexts, thereby fostering more context-specific strategies for global decarbonization efforts. Third, as the world transitions to low-carbon and circular economies, understanding the interplay between LCED and GPR is crucial for crafting more inclusive and resilient energy policies. This understanding not only helps reduce resource dependence and mitigate supply chain vulnerabilities but also promotes global low-carbon economic development through strengthened international cooperation, technology sharing, and policy alignment. In particular, enhancing cooperation on technological innovation, energy diversification, and cross-border green investments can contribute to achieving sustainable geopolitical stability, providing pathways for countries to jointly address both environmental and geopolitical challenges. Furthermore, integrating additional variables, such as regional trade dynamics, security risks related to energy supply chains, or the role of multinational corporations in the low-carbon transition, could help further illuminate the broader economic and geopolitical impacts of LCED. As future research continues to develop in this field, it will be crucial to incorporate these multidimensional factors to gain a deeper understanding of the forces shaping the global low-carbon economy.
Data availability statement
The raw data supporting the conclusions of this article will be made available by the authors, without undue reservation.
Author contributions
YC: Writing–review and editing. JL: Writing–original draft. JO: Conceptualization, Formal Analysis, Supervision, Writing–review and editing. WS: Data curation, Methodology, Resources, Writing–original draft.
Funding
The author(s) declare financial support was received for the research, authorship, and/or publication of this article. This research was supported by the National Natural Science Foundation of China (72172018) and the Innovative Talent Exchange Program for Foreign Experts (DL202310701L).
Conflict of interest
The authors declare that the research was conducted in the absence of any commercial or financial relationships that could be construed as a potential conflict of interest.
Generative AI statement
The author(s) declare that no Generative AI was used in the creation of this manuscript.
Publisher’s note
All claims expressed in this article are solely those of the authors and do not necessarily represent those of their affiliated organizations, or those of the publisher, the editors and the reviewers. Any product that may be evaluated in this article, or claim that may be made by its manufacturer, is not guaranteed or endorsed by the publisher.
Supplementary material
The Supplementary Material for this article can be found online at: https://www.frontiersin.org/articles/10.3389/fenvs.2025.1524329/full#supplementary-material
Footnotes
1This paper also uses rolling-window widths of 20-, 28- and 32- months to explore causality. The results do not change significantly which confirm their robustness.
References
Adams, S., Adedoyin, F., Olaniran, E., and Bekun, F. V. (2020). Energy consumption, economic policy uncertainty and carbon emissions; causality evidence from resource-rich economies. Econ. Analysis Policy 68, 179–190. doi:10.1016/j.eap.2020.09.012
Almujeem, N. S. (2021). GCC countries’ geoeconomic significance to China’s geopolitical ends. Rev. Econ. Political Sci. 6 (4), 348–363. doi:10.1108/reps-11-2019-0152
Al Obaidy, L. I. M. (2023). The Chinese vision of US rapprochement with taiwan in the administration of president joe biden. Political Sci. J. (65). doi:10.30907/jcopolicy.vi65.642
Andrews, D. W. (1993). Tests for parameter instability and structural change with unknown change point. Econ. J. Econ. Soc. 61, 821–856. doi:10.2307/2951764
Andrews, D. W., and Ploberger, W. (1994). Optimal tests when a nuisance parameter is present only under the alternative. Econ. J. Econ. Soc. 62, 1383–1414. doi:10.2307/2951753
Andrews-Speed, P., van der Linde, C., and Keramidas, K. (2014). Conflict and cooperation over access to energy: implications for a low-carbon future. Futures 58, 103–114. doi:10.1016/j.futures.2013.12.007
Auty, R. (2002). Sustaining development in mineral economies: the resource curse thesis. United Kingdom: Routledge.
Balcilar, M., Ozdemir, Z. A., and Arslanturk, Y. (2010). Economic growth and energy consumption causal nexus viewed through a bootstrap rolling window. Energy Econ. 32 (6), 1398–1410. doi:10.1016/j.eneco.2010.05.015
Balsalobre-Lorente, D., Sinha, A., and Murshed, M. (2023). Russia-Ukraine conflict sentiments and energy market returns in G7 countries: discovering the unexplored dynamics. Energy Econ. 125, 106847. doi:10.1016/j.eneco.2023.106847
I. Bannon, and P. Collier (2003). Natural resources and violent conflict: options and actions (United States: World Bank Publications).
Biedzynski, J. (2015). “Asia's cauldron: the South China sea and the end of A stable pacific,” in Journal of third world studies. Editor R. D. Kaplan, 32, 317–318.
Blondeel, M., Bradshaw, M. J., Bridge, G., and Kuzemko, C. (2021). The geopolitics of energy system transformation: a review. Geogr. Compass 15 (7), e12580. doi:10.1111/gec3.12580
Böhringer, C., Fischer, C., Rosendahl, K. E., and Rutherford, T. F. (2022). Potential impacts and challenges of border carbon adjustments. Nat. Clim. Change 12 (1), 22–29. doi:10.1038/s41558-021-01250-z
Borozan, D. (2024). Do geopolitical and energy security risks influence carbon dioxide emissions? Empirical evidence from European Union countries. J. Clean. Prod. 439, 140834. doi:10.1016/j.jclepro.2024.140834
Bovenberg, A. L., and De Mooij, R. A. (1994). Environmental levies and distortionary taxation. Am. Econ. Rev. 84 (4), 1085–1089.
Burgis, T. (2015). The looting machine: warlords, tycoons, smugglers, and the systematic theft of africa's wealth. New York: PublicAffairs.
Caldara, D., and Iacoviello, M. (2022). Measuring geopolitical risk. Am. Econ. Rev. 112 (4), 1–66. doi:10.17016/ifdp.2018.1222
Canuto, O. (2023). A tale of two technology wars: semiconductors and clean energy (No. 2025). Moroccan: Policy Center for the New South.
Chen, B., Dai, Y., Ye, J., Xu, H., Huang, Y., and Bie, P. (2022a). Evaluating energy efficiency from the perspective of clean energy and carbon emission. Front. Environ. Sci. 10, 1002572. doi:10.3389/fenvs.2022.1002572
Chen, L., Gozgor, G., Lau, C. K. M., Mahalik, M. K., Rather, K. N., and Soliman, A. M. (2024a). The impact of geopolitical risk on CO2 emissions inequality: evidence from 38 developed and developing economies. J. Environ. Manag. 349, 119345. doi:10.1016/j.jenvman.2023.119345
Chen, Y., Li, B., Zhang, D., and Zhang, Y. (2022b). The Russia-Ukraine conflict, crude oil price, and transportation industry yield. energy 30, 88–95. doi:10.54691/bcpbm.v30i.2406
Chen, Y., Li, Q., and Liu, J. (2024b). Innovating sustainability: VQA-based AI for carbon neutrality challenges. J. Organ. End User Comput. (JOEUC) 36 (1), 1–22. doi:10.4018/joeuc.337606
Chen, Y., Zhang, R., Lyu, J., and Hou, Y. (2024d). AI and Nuclear: a perfect intersection of danger and potential? Energy Econ. 133, 107506. doi:10.1016/j.eneco.2024.107506
Chen, Y., Zhang, R., Lyu, J., and Ma, X. (2024c). The butterfly effect of cloud computing on the low-carbon economy. Technol. Forecast. Soc. Change 204, 123433. doi:10.1016/j.techfore.2024.123433
Cheng, F. T. (2020). China and the United States’ critical roles in tackling climate change and shaping the international regimes. International Relations 8 (04), 173–187.
Cheng, S., and Wang, B. (2023). Impact of the Belt and Road Initiative on China's overseas renewable energy development finance: effects and features. Renew. Energy 206, 1036–1048. doi:10.1016/j.renene.2023.02.101
Cui, L., Yue, S., Nghiem, X. H., and Duan, M. (2023). Exploring the risk and economic vulnerability of global energy supply chain interruption in the context of Russo-Ukrainian war. Resour. Policy 81, 103373. doi:10.1016/j.resourpol.2023.103373
Dickey, D. A., and Fuller, W. A. (1981). Likelihood ratio statistics for autoregressive time series with a unit root. Econ. J. Econ. Soc. 49, 1057–1072. doi:10.2307/1912517
Ding, T., Li, H., Tan, R., and Zhao, X. (2023). How does geopolitical risk affect carbon emissions? An empirical study from the perspective of mineral resources extraction in OECD countries. Resour. Policy 85, 103983. doi:10.1016/j.resourpol.2023.103983
Feng, Y. S., Ling, M. J., and Gao, J. (2024). Interplay multifractal dynamics among carbon trading market, geopolitical risk and economic policy uncertainty. Singapore: Fluctuation and Noise Letters. 2450029.
Gong, X., Sun, Y., and Du, Z. (2022). Geopolitical risk and China's oil security. Energy Policy 163, 112856. doi:10.1016/j.enpol.2022.112856
Gozgor, G., and Paramati, S. R. (2022). Does energy diversification cause an economic slowdown? Evidence from a newly constructed energy diversification index. Energy Econ. 109, 105970. doi:10.1016/j.eneco.2022.105970
Guilhot, L. (2022). An analysis of China's energy policy from 1981 to 2020: transitioning towards to a diversified and low-carbon energy system. Energy Policy 162, 112806. doi:10.1016/j.enpol.2022.112806
Guo, P., and Shi, J. (2024). Geopolitical risks, investor sentiment and industry stock market volatility in China: evidence from a quantile regression approach. North Am. J. Econ. Finance 72, 102139. doi:10.1016/j.najef.2024.102139
Han, P., Zeng, N., Zhang, W., Cai, Q., Yang, R., Yao, B., et al. (2021). Decreasing emissions and increasing sink capacity to support China in achieving carbon neutrality before 2060. arXiv Prepr. arXiv:2102.10871. doi:10.48550/arXiv.2102.10871
Hanson, B. E. (2002). Tests for parameter instability in regressions with I(1) processes. J. Bus. and Econ. Statistics 20 (1), 45–59. doi:10.1198/073500102753410381
Huang, Z., Xie, X., and Zhang, T. (2023). Medium-and long-term energy demand of China and energy transition pathway toward carbon neutrality. Strategic Study Chin. Acad. Eng. 24 (6), 8–18. doi:10.15302/j-sscae-2022.06.002
Hunjra, A. I., Azam, M., Verhoeven, P., Taskin, D., and Dai, J. (2024). The impact of geopolitical risk, institutional governance, and green finance on attaining net-zero carbon emission. J. Environ. Manag. 359, 120927. doi:10.1016/j.jenvman.2024.120927
Jayaraman, T. (2014). Climate change and development: implications of the US–China Joint announcement on climate change. Rev. Agrar. Stud. 4 (2), 84–92. doi:10.25003/ras.04.02.0004
Keohane, R. O., and Nye Jr, J. S. (1973). Power and interdependence. Survival 15 (4), 158–165. doi:10.1080/00396337308441409
Khan, K., Su, C. W., Umar, M., and Yue, X. G. (2021). Do crude oil price bubbles occur? Resour. Policy 71, 101936. doi:10.1016/j.resourpol.2020.101936
Kwiatkowski, D., Phillips, P. C., Schmidt, P., and Shin, Y. (1992). Testing the null hypothesis of stationarity against the alternative of a unit root: how sure are we that economic time series have a unit root? J. Econ. 54 (1-3), 159–178. doi:10.1016/0304-4076(92)90104-y
Ladislaw, S. O., Leed, M., and Walton, M. A. (2015). New energy, new geopolitics: balancing stability and leverage. United States: Rowman and Littlefield.
Li, H., Lin, H., Tan, Q., Wu, P., Wang, C., De, G., et al. (2020a). Research on the policy route of China’s distributed photovoltaic power generation. Energy Rep. 6, 254–263. doi:10.1016/j.egyr.2019.12.027
Li, H., Bhatti, Z., Abbas, Q., Ahmad, I., Iqbal, N., and Aziz, B. (2024). Measuring low carbon energy, economic and environmental sustainability performance of BRICS. Singap. Econ. Rev. 69 (01), 357–376. doi:10.1142/s0217590820500617
Li, J., Wang, L., Lin, X., and Qu, S. (2020b). Analysis of China’s energy security evaluation system: based on the energy security data from 30 provinces from 2010 to 2016. Energy 198, 117346. doi:10.1016/j.energy.2020.117346
Li, T., Yu, L., Ma, Y., Duan, T., Huang, W., Zhou, Y., et al. (2023a). Carbon emissions of 5G mobile networks in China. Nat. Sustain. 6 (12), 1620–1631. doi:10.1038/s41893-023-01206-5
Li, Z., Meng, Q., Zhang, L., Lobont, O. R., and Shen, Y. (2023b). How do rare earth prices respond to economic and geopolitical factors? Resour. Policy 85, 103853. doi:10.1016/j.resourpol.2023.103853
Lippert, B., Perthes, V., and Politik-Swp-Deutsches, S. W. (2020). Strategic rivalry between United States and China: causes, trajectories, and implications for Europe.
Liu, F., Su, C. W., Qin, M., and Lobonţ, O. R. (2023). Winner or loser? The bidirectional impact between geopolitical risk and energy transition from the renewable energy perspective. Energy 283, 129174. doi:10.1016/j.energy.2023.129174
Liu, Y., Fan, X., and Bao, X. (2022). Economic optimization of new energy technologies in the context of low carbon economy. Energy Rep. 8, 11899–11909. doi:10.1016/j.egyr.2022.09.006
Liu, Z. (2024). The impact and challenges of the Russia-Ukraine war on energy strategy transformation. J. Educ. Humanit. Soc. Sci. 28, 34–39. doi:10.54097/kfqs0m81
Lu, Z., and Zhu, Z. (2022). “The complexity of US-China relationship: examination through their cooperation on climate change,” in 2021 international conference on public art and human development (ICPAHD 2021) (Netherlands: Atlantis Press), 1058–1062.
Manish, , and Kaushik, P. (2023). China’s interests in Afghanistan: an assessment post US withdrawal. China Rep. 59 (1), 58–79. doi:10.1177/00094455231152902
Melin, H. E., Rajaeifar, M. A., Ku, A. Y., Kendall, A., Harper, G., and Heidrich, O. (2021). Global implications of the EU battery regulation. Science 373 (6553), 384–387. doi:10.1126/science.abh1416
Meng, B., Liu, Y., Gao, Y., Li, M., Wang, Z., Xue, J., et al. (2023). Developing countries’ responsibilities for CO2 emissions in value chains are larger and growing faster than those of developed countries. One Earth 6 (2), 167–181. doi:10.1016/j.oneear.2023.01.006
Morris, D. (2023). “Asian infrastructure Investment Bank,” in The palgrave encyclopedia of global security studies (Cham: Springer International Publishing), 86–95.
Murphy, A. M., and Turek, A. (2024). “The United States and the South China sea,” in Security dynamics in the South China sea (England: Routledge), 241–263.
Nakano, J. (2021). The geopolitics of critical minerals supply chains. Washington, DC, USA: Center for Strategic and International Studies.
Newbery, D., Pollitt, M. G., Ritz, R. A., and Strielkowski, W. (2018). Market design for a high-renewables European electricity system. Renew. Sustain. Energy Rev. 91, 695–707. doi:10.1016/j.rser.2018.04.025
Nong, D., Simshauser, P., and Nguyen, D. B. (2021). Greenhouse gas emissions vs CO2 emissions: comparative analysis of a global carbon tax. Appl. Energy 298, 117223. doi:10.1016/j.apenergy.2021.117223
Nyblom, J. (1989). Testing for the constancy of parameters over time. J. Am. Stat. Assoc. 84 (405), 223–230. doi:10.2307/2289867
Panwar, R., Pinkse, J., and De Marchi, V. (2022). The future of global supply chains in a post-COVID-19 world. Calif. Manag. Rev. 64 (2), 5–23. doi:10.1177/00081256211073355
Park, S. (2020). Trade conflict between the US and China: what are the impacts on the Chinese economy. Int. Organ. Res. J. 15 (2), 213–235. doi:10.17323/1996-7845-2020-02-10
Pepe, J. M., Grinschgl, J., and Westphal, K. (2023). “US-China rivalry and its impact on the energy transformation: difficult cooperation fraught with dilemmas,” in Handbook on the Geopolitics of the Energy Transition. Edward Elgar Publishing, 107–107.
Pesaran, M. H., and Timmermann, A. (2005). Small sample properties of forecasts from autoregressive models under structural breaks. J. Econ. 129 (1-2), 183–217. doi:10.1016/j.jeconom.2004.09.007
Pfeffer, J., and Salancik, G. (2015). External control of organizations—resource dependence perspective. Organ. Behav. 2, 355–370. doi:10.4324/9781315702001
Phillips, P. C., and Perron, P. (1988). Testing for a unit root in time series regression. biometrika 75 (2), 335–346. doi:10.2307/2336182
Piao, L. (2023). China in 2022: political power, zero-COVID policy, and economic slump. Asian Surv. 63 (2), 175–185. doi:10.1525/as.2023.63.2.175
Qi, Y., Liu, T., and Jing, L. (2023). China's energy transition towards carbon neutrality with minimum cost. J. Clean. Prod. 388, 135904. doi:10.1016/j.jclepro.2023.135904
Qin, M., Su, C. W., Lobonţ, O. R., and Umar, M. (2023a). Blockchain: a carbon-neutral facilitator or an environmental destroyer? Int. Rev. Econ. and Finance 86, 604–615. doi:10.1016/j.iref.2023.04.004
Qin, M., Su, C. W., Umar, M., Lobonţ, O. R., and Manta, A. G. (2023b). Are climate and geopolitics the challenges to sustainable development? Novel evidence from the global supply chain. Econ. Analysis Policy 77, 748–763. doi:10.1016/j.eap.2023.01.002
Rasoulinezhad, E., Taghizadeh-Hesary, F., Sung, J., and Panthamit, N. (2020). Geopolitical risk and energy transition in Russia: evidence from ARDL bounds testing method. Sustainability 12 (7), 2689. doi:10.3390/su12072689
Ren, X., An, Y., and Jin, C. (2023b). The asymmetric effect of geopolitical risk on China's crude oil prices: new evidence from a QARDL approach. Finance Res. Lett. 53, 103637. doi:10.1016/j.frl.2023.103637
Ren, X., An, Y., Jin, C., and Yan, C. (2024). Weathering the policy storm: how climate strategy volatility shapes corporate total factor productivity. Energy Econ. 134, 107553. doi:10.1016/j.eneco.2024.107553
Ren, X., Li, J., He, F., and Lucey, B. (2023a). Impact of climate policy uncertainty on traditional energy and green markets: evidence from time-varying granger tests. Renew. Sustain. Energy Rev. 173, 113058. doi:10.1016/j.rser.2022.113058
Ren, X., Zhang, X., Yan, C., and Gozgor, G. (2022). Climate policy uncertainty and firm-level total factor productivity: evidence from China. Energy Econ. 113, 106209. doi:10.1016/j.eneco.2022.106209
Rogelja, I., and Tsimonis, K. (2020). Narrating the China threat: securitising Chinese economic presence in Europe. Chin. J. Int. Polit. 13 (1), 103–133. doi:10.1093/cjip/poz019
Rolland, N. (2017). China's Eurasian century? Political and strategic implications of the belt and road initiative. Natl. Bureau Asian Res.
Safi, A., Haseeb, M., Islam, M., and Umar, M. (2023). Can sustainable resource management overcome geopolitical risk? Resour. Policy 87, 104270. doi:10.1016/j.resourpol.2023.104270
Scheffran, J. (2023). Limits to the Anthropocene: geopolitical conflict or cooperative governance? Front. Political Sci. 5, 1190610. doi:10.3389/fpos.2023.1190610
Shaik, M., Jamil, S. A., Hawaldar, I. T., Sahabuddin, M., Rabbani, M. R., and Atif, M. (2023). Impact of geo-political risk on stocks, oil, and gold returns during GFC, COVID-19, and Russian–Ukraine War. Cogent Econ. and Finance 11 (1), 2190213. doi:10.1080/23322039.2023.2190213
Shang, M., and Luo, J. (2021). The tapio decoupling principle and key strategies for changing factors of Chinese urban carbon footprint based on cloud computing. Int. J. Environ. Res. Public Health 18 (4), 2101. doi:10.3390/ijerph18042101
Shukur, G., and Mantalos, P. (2000). A simple investigation of the Granger-causality test in integrated-cointegrated VAR systems. J. Appl. Statistics 27 (8), 1021–1031. doi:10.1080/02664760050173346
Shukur, G., and Mantalos, P. (2004). Size and power of the RESET test as applied to systems of equations: a bootstrap approach. J. Mod. Appl. Stat. methods 3, 370–385. doi:10.22237/jmasm/1099267800
Siciliano, G., Wallbott, L., Urban, F., Dang, A. N., and Lederer, M. (2021). Low-carbon energy, sustainable development, and justice: towards a just energy transition for the society and the environment. Sustain. Dev. 29 (6), 1049–1061. doi:10.1002/sd.2193
Song, Y., Zhang, M., and Sun, R. (2019). Using a new aggregated indicator to evaluate China's energy security. Energy Policy 132, 167–174. doi:10.1016/j.enpol.2019.05.036
Su, C. W., Qin, M., Tao, R., Shao, X. F., Albu, L. L., and Umar, M. (2020). Can Bitcoin hedge the risks of geopolitical events? Technol. Forecast. Soc. Change 159, 120182. doi:10.1016/j.techfore.2020.120182
Su, Y., Zhang, P., and Su, Y. (2015). An overview of biofuels policies and industrialization in the major biofuel producing countries. Renew. Sustain. Energy Rev. 50, 991–1003. doi:10.1016/j.rser.2015.04.032
Sullivan, B. (2022). Exogenous supply chain shocks and onshoring: an analysis of the trump trade war and the US-China bilateral trade relationship.
Sun, T. T., and Su, C. W. (2024). How is geopolitical risk associated with food prices? Int. J. Emerg. Mark. doi:10.1108/ijoem-01-2023-0004
Sun, X., Mi, Z., Cheng, L., Coffman, D. M., and Liu, Y. (2023). The carbon border adjustment mechanism is inefficient in addressing carbon leakage and results in unfair welfare losses. Fundam. Res. 4, 660–670. doi:10.1016/j.fmre.2023.02.026
Sun, Y. (2020). The achievement, significance and future prospect of China's renewable energy initiative. Int. J. Energy Res. 44 (15), 12209–12244. doi:10.1002/er.5243
Sweidan, O. D. (2021). The geopolitical risk effect on the US renewable energy deployment. J. Clean. Prod. 293, 126189. doi:10.1016/j.jclepro.2021.126189
Tan, J., Wang, F., and Wang, R. (2023). Exploring the role of “double cycle” and industrial structure in high-quality economic development in China. SAGE Open 13 (2), 21582440231180181. doi:10.1177/21582440231180181
Teng, Z., Wei, Z., Qiu, Y., Tan, Y., Chen, J., Tang, H., et al. (2020). Psychological status and fatigue of frontline staff two months after the COVID-19 pandemic outbreak in China: A cross-sectional study. J. Affect. Disord. 275, 247–252.
Tong, T., Ortiz, J., and Wang, H. (2023). The pathway to the natural gas industry: financial development, coal consumption, and carbon dioxide emissions in PR China. Sci. Prog. 106 (1), 00368504231160643. doi:10.1177/00368504231160643
Tong, T., Ortiz, J., Xu, C., and Li, F. (2020). Economic growth, energy consumption, and carbon dioxide emissions in the E7 countries: a bootstrap ARDL bound test. Energy, Sustain. Soc. 10 (1), 20–17. doi:10.1186/s13705-020-00253-6
Ugurlu-Yildirim, E., and Ordu-Akkaya, B. M. (2022). Does the impact of geopolitical risk reduce with the financial structure of an economy? A perspective from market vs. bank-based emerging economies. Eurasian Econ. Rev. 12 (4), 681–703. doi:10.1007/s40822-022-00219-3
Wang, F., Chun, W., and Cui, Y. (2022a). Urban water resources allocation and low-carbon economic development based on soft computing. Environ. Technol. and Innovation 28, 102292. doi:10.1016/j.eti.2022.102292
Wang, H., Niu, Y., Gapich, A., and Strielkowski, W. (2023). Natural resources extractions and carbon neutrality: the role of geopolitical risk. Resour. Policy 83, 103577. doi:10.1016/j.resourpol.2023.103577
Wang, K. H., Kan, J. M., Jiang, C. F., and Su, C. W. (2022b). Is geopolitical risk powerful enough to affect carbon dioxide emissions? Evidence from China. Sustainability 14 (13), 7867. doi:10.3390/su14137867
Wang, K. H., Su, C. W., and Umar, M. (2021). Geopolitical risk and crude oil security: a Chinese perspective. Energy 219, 119555. doi:10.1016/j.energy.2020.119555
Wang, W., and Liu, Y. (2015). Geopolitics of global climate change and energy security. Chin. J. Popul. Resour. Environ. 13 (2), 119–126. doi:10.1080/10042857.2015.1017907
Wang, Y., Sperling, D., Tal, G., and Fang, H. (2017). China's electric car surge. Energy Policy 102, 486–490. doi:10.1016/j.enpol.2016.12.034
Weigel, M., and Demissie, A. (2021). Achieving the SDGs in Africa through south-south cooperation on climate change with China. Palgrave Handb. Dev. Coop. Achiev. 2030 Agenda 605, 605–623. doi:10.1007/978-3-030-57938-8_28
Wu, Z., Fan, X., Zhu, B., Xia, J., Zhang, L., and Wang, P. (2022). Do government subsidies improve innovation investment for new energy firms: a quasi-natural experiment of China's listed companies. Technol. Forecast. Soc. Change 175, 121418. doi:10.1016/j.techfore.2021.121418
Xing, Z., Huang, J., and Wang, J. (2023). Unleashing the potential: exploring the nexus between low-carbon digital economy and regional economic-social development in China. J. Clean. Prod. 413, 137552. doi:10.1016/j.jclepro.2023.137552
Xin-Gang, Z., and Ying, Z. (2023). Can China’s renewable energy industry policy support the low-carbon energy transition effectively? Environ. Sci. Pollut. Res. 30 (11), 29525–29549. doi:10.1007/s11356-022-24324-7
Xu, A., Wang, W., and Zhu, Y. (2023). Does smart city pilot policy reduce CO2 emissions from industrial firms? Insights from China. J. Innovation and Knowl. 8 (3), 100367. doi:10.1016/j.jik.2023.100367
Yang, L., Gao, X., and Li, Z. (2023). “An overview of the policies and models of integrated development for solar and wind power generation in China,” in Research in cold and arid regions.
Yesmurzayeva, A., and Mrzabayeva, R. Z. (2023). The transition to green energy: a comprehensive market review of financing, investment, and policy for a sustainable future. Научный журнал «Вестник НАН РК» 404 (4), 481–496. doi:10.32014/2023.2518-1467.564
Zhang, L., Wang, Q., and Zhang, M. (2021). Environmental regulation and CO2 emissions: based on strategic interaction of environmental governance. Ecol. Complex. 45, 100893. doi:10.1016/j.ecocom.2020.100893
Zhao, S., Zhang, L., An, H., Peng, L., Zhou, H., and Hu, F. (2023a). Has China's low-carbon strategy pushed forward the digital transformation of manufacturing enterprises? Evidence from the low-carbon city pilot policy. Environ. impact Assess. Rev. 102, 107184. doi:10.1016/j.eiar.2023.107184
Zhao, W., Zhong, R., Sohail, S., Majeed, M. T., and Ullah, S. (2021). Geopolitical risks, energy consumption, and CO2 emissions in BRICS: an asymmetric analysis. Environ. Sci. Pollut. Res. 28, 39668–39679. doi:10.1007/s11356-021-13505-5
Zhao, X., Jiang, M., and Zhou, Y. (2023b). The impact of the digital economy on carbon emission intensity: evidence from China. Front. Environ. Sci. 11, 1119246. doi:10.3389/fenvs.2023.1119246
Zhao, X., Ma, X., Chen, B., Shang, Y., and Song, M. (2022). Challenges toward carbon neutrality in China: strategies and countermeasures. Resour. Conservation Recycl. 176, 105959. doi:10.1016/j.resconrec.2021.105959
Zheng, J., Shao, X., Liu, W., Kong, J., and Zuo, G. (2021). The impact of the pilot program on industrial structure upgrading in low-carbon cities. J. Clean. Prod. 290, 125868. doi:10.1016/j.jclepro.2021.125868
Keywords: low-carbon economic development, geopolitical risk, time-dependent interrelationships, China, granger causality test
Citation: Chen Y, Lyu J, Ortiz J and Song W (2025) China’s readiness for transitioning to a low-carbon economy: mitigant and catalyst factors for a geopolitical conflict. Front. Environ. Sci. 13:1524329. doi: 10.3389/fenvs.2025.1524329
Received: 07 November 2024; Accepted: 10 February 2025;
Published: 28 February 2025.
Edited by:
Le Wen, University of Auckland, New ZealandReviewed by:
Irina Georgescu, Bucharest Academy of Economic Studies, RomaniaMohamed R. Abonazel, Cairo University, Egypt
Copyright © 2025 Chen, Lyu, Ortiz and Song. This is an open-access article distributed under the terms of the Creative Commons Attribution License (CC BY). The use, distribution or reproduction in other forums is permitted, provided the original author(s) and the copyright owner(s) are credited and that the original publication in this journal is cited, in accordance with accepted academic practice. No use, distribution or reproduction is permitted which does not comply with these terms.
*Correspondence: Jaime Ortiz, amFpbWUub3J0aXpAdXRyZ3YuZWR1