- 1College of Geographical Sciences, Qinghai Normal University, Xining, China
- 2Qinghai Province Key Laboratory of Physical Geography and Environmental Process, College of Geographical Science, Qinghai Normal University, Xining, China
- 3Key Laboratory of Tibetan Plateau Land Surface Processes and Ecological Conservation (Ministry of Education), Qinghai Normal University, Xining, China
- 4National Positioning Observation and Research Station of Qinghai Lake Wetland Ecosystem in Qinghai, National Forestry and Grassland Administration, Haibei, China
- 5Qinghai Sanjiangyuan Nationalities Middle School, Xining, China
Photovoltaic (PV) park construction has a profound impact on the regional microclimate and ecosystem carbon cycle. Meanwhile, biological soil crusts (BSCs) also play a crucial role in the carbon and nitrogen cycles of desert ecosystems. However, the response mechanism of algal BSC microbial communities to PV panel construction is still poorly elucidated. In this study, high-throughput sequencing was utilized to explore the mechanism underlying the effects of PV panel construction on algal BSC microbial communities in the Talatan PV Base. The results showed that the predominant bacterial phyla of algal BSCs in desert grassland were Actinobacteriota and Proteobacteria. PV panel construction changed the structure of algal BSC bacterial communities, which significantly increased the relative abundance of Proteobacteria and enhanced the interconnectivity between bacterial species. Nevertheless, the response of bacterial community diversity to the construction of PV panels was not significant (P > 0.05). PV panel construction altered the microenvironment by significantly increasing temperatures under the panels while significantly reducing soil carbon stocks. In addition, temperature and carbon content were the most important factors influencing the structure and diversity of algal BSC bacterial communities in alpine desert grasslands. In summary, the installation of photovoltaic panels led to a notable elevation in the temperature underneath them. This temperature increase, in turn, enhanced the stability of the bacterial communities within the algal BSC in alpine desert grasslands. Simultaneously, it significantly reduced the carbon storage capacity in these grasslands, thus exerting a dual-edged impact on the ecological environment of the region.
1 Introduction
Grassland ecosystems are widely regarded as among the ecosystems most sensitive to climate change (Piao et al., 2012; Chen et al., 2013). They play a pivotal role in soil and water conservation, as well as climate regulation (Wang et al., 2020; Che et al., 2018). The Qinghai-Tibetan Plateau (QTP) stands as a globally significant ecological region. Alpine grassland ecosystems cover approximately 60% of its territory (Dong et al., 2022; Dong et al., 2010; Zhao et al., 2017). Characterized by cold and humid habitats, the ecosystems in this region are fragile and extremely sensitive to environmental changes (Zeng et al., 2013; Ganjurjav et al., 2016a; Ganjurjav et al., 2016b; He et al., 2016). Desert steppes, being one of the major land cover types of QTP grasslands, have a critical influence the plateau’s climate regulation and water conservation (Gao et al., 2016). Furthermore, their unique regionality renders them highly vulnerable to external environmental disturbances (Chang et al., 2020). In recent decades, due to climate change, improper grazing management, and other human activities, at least one-third of the alpine grasslands on the QTP have undergone degradation (Liu et al., 2020; Wang and Wesche, 2016; Qiu, 2016; Yang and Sun, 2021). This degradation has had a direct impact on the carbon and nitrogen cycling processes in alpine grassland ecosystems (Ding et al., 2016).
Biological soil crusts (BSCs) represent complex assemblages of surface soil microorganisms. These remarkable entities act as the primary catalysts for the biogeochemical cycling of carbon, nitrogen, and phosphorus within dryland ecosystems, while also playing a pivotal role in soil and water conservation (Eldridge et al., 2020; Li et al., 2021). Consequently, they are fundamental to maintaining the fertility and stability of drylands (Weber et al., 2016). As an important reservoir of organic carbon and nitrogen in desert soils, BSCs are vital to the carbon and nitrogen cycles of desert ecosystems (Yan et al., 2013; Zhao et al., 2016). It is estimated that BSCs cover approximately 12% of the global terrestrial surface area and contribute 15% of global terrestrial net primary productivity (Elbert et al., 2012; Rodriguez-Caballero et al., 2018). In addition, they provide ecosystem services that influence biogeochemical fluxes on a global scale (Grote et al., 2010; Castillo-Monroy et al., 2011; Elbert et al., 2012; Weber et al., 2015; Mogul et al., 2017; Maier et al., 2018). The microorganisms inhabiting in BSCs perform several important ecological functions, such as fixing atmospheric carbon and nitrogen, and also producing polymeric substances such as exopolysaccharides and glycoproteins. These substances, especially the latter, can enhance soil aggregate stability by binding together soil minerals particles. As a result, they significantly mitigate soil erodibility (Bowker et al., 2008; Mager and Thomas, 2011).
Photovoltaic (PV) power generation has emerged as one of the most favored clean energy sources globally. Characterized by its inexhaustible nature and non - polluting properties (Dhar et al., 2020; Armstrong et al., 2016), it holds great promise as a viable solution for humanity’s transition towards a low - carbon future. Natural grasslands, boasting abundant light resources and relatively low land costs, have become prime locations for the establishment of PV power plants. Nevertheless, PV parks, with their dark, sunlight - absorbing panels covering a significant portion of the soil surface, can substantially modify the original surface topography of the local area. This, in turn, exerts far - reaching effects on the local microclimate, the ecosystem carbon cycle, biodiversity, soil weathering processes, and the flow of energy within the ecosystem (Armstrong et al., 2014; Salamanca et al., 2016). Moreover, the construction areas of PV facilities not only disrupt the original characteristics of plant communities but also damage the soil structure, thereby causing harm to the native ecosystems (Xu et al., 2008). The rapid expansion of the PV industry has led to an increasingly profound impact on terrestrial ecosystems. In addition, the installation of PV panels can exacerbate the uneven accumulation of atmospheric precipitation (Liu et al., 2019). Considering the far - reaching influence of climate change on various ecosystem processes (Watson et al., 2020; HMa et al., 2020), it is of utmost importance to comprehensively explore the impacts of PV construction on soil microbial communities. This exploration is essential for better understanding the complex ecological consequences of PV development and for formulating more sustainable strategies for the integration of clean energy and ecosystem conservation.
Currently, the climate and environmental impact of PV power plants is of great concern. But the mechanism of how PV park construction affects BSC microbial communities is barely studied. Understanding how PV panel construction influences the dynamic changes of algal BSC microbial communities in alpine desert steppes is vital for keeping grassland ecosystem functions. Our study focuses on China’s largest PV base in Talatan, Gonghe Basin, northeastern QTP. We used high - throughput sequencing to analyze the 16S rRNA of bacterial communities and measured soil biogeochemical properties. The study aims to address the following questions: (1) how microbial community characteristics in alpine desert grasslands respond to PV panel construction? (2) how PV - construction - induced soil property changes impact microbial communities in these grasslands? (3) how the microbial community assembled and what the changes will occur in their interaction networks due to PV panel constructions? By comparing microbial community responses in areas with different PV panel installations, we aim to uncover how PV panel construction impacts microbial community diversity, structure, function, assembly, and interaction networks in alpine desert grasslands. This research will offer theoretical support for dealing with global climate change and protecting desert grassland ecosystems.
2 Materials and methods
2.1 Study area profile
The study area was located in Talatan, Gonghe County, Qinghai Province in the northeastern region of the QTP. Its geographical coordinates range from 98°54′–101°22′E in longitude and 35°46′–37°10′N in latitude. Characterized by a gourd - shaped topography, it is broad in the east and narrow in the west. This area is known as the “throat of the QTP”. The average annual precipitation in Talatan is 303 mm, with precipitation being unevenly distributed both within a year and across different years. Regionally, precipitation is higher in southeast Talatan, and lower in the northwest. The average annual evaporation reaches 1,800 mm, and the annual sunshine duration of 2,772.2 h. Spring has the most sunshine hours, followed by summer and autumn, with winter having the least. The climate is mainly arid and semi-arid, with an average annual temperature of 4.1°C and an average annual frost-free period of 91 days. The average annual number of windy days is 207. Under the influence of the northwest wind, quicksand invades in the southeast direction. The soil in this area is mainly chestnut soil, and the thickness of the soil development layer generally ranges from 50 to 70 cm. The dominant vegetation species mainly consist of Achnatherum splendens (Trin.) Nevski, Stipa breviflora Griseb, Orinus thoroldii (Stapf ex Hemsl.) Bor, Leymus secalinus (Georgi) Tzvelev, Artemisia frigida Willd, among others.
2.2 Sample collection
The layout of the field plots is presented in Supplementary Figure S1. In November 2023, during the wilting period, soil samples were collected. A 3,000 - meter sampling line was established between the areas (Supplementary Figure S2) and directly under the panels (Supplementary Figure S3) within the PV panel - constructed regions of the desert grasslands, as well as in the non - PV panel - constructed areas (natural control, Supplementary Figure S4). Algal BSCs were collected from nine sites along the sampling line, with each site located 500 m apart and the samples from these sites were then mixed. Samples in each site were collected using the five - point sampling method from the soil layer of 0–5 cm depth for experimental analysis. The samples were named GJCZ (algal BSCs between panels), GXCZ (algal BSCs under panels), and FCZ (natural control) according to the grouping. The experiment incorporated three treatments, each with five replicates. Consequently, a total of 15 soil algal BSC samples were collected. All samples were divided into two portions. They were stored in ice packs and promptly transported back to the laboratory. One portion of the samples was refrigerated at 4°C for the determination of physicochemical properties, while the other portion was stored at −80°C for DNA extraction.
2.3 Determination of soil physicochemical properties
pH was measured using a 1:2.5 soil-deionized water mixture (AZ8601, AZ Instrument, China). Total carbon (TC) and total nitrogen (TN) content was quantified using an elemental analyzer (Vario EL III, Elementar). Dissolved organic carbon (DOC) was extracted from unfumigated algal BSCs, and soil organic carbon (SOC) was determined by the exothermic (170°C–180°C) dichromate oxidation method and ferrous ammonium sulfate titration method. Soil available nitrogen [NH4+-N (AN) and NO3–-N (NN)] was determined using a Foss FIAstar 5000 automatic flow injection analyzer (Foss, Denmark) and calculated based on soil dry weight. Soil temperature (Tem) and moisture (Moi) were measured using a dedicated Zhengda soil temperature/moisture (water content) detector (ZD-1608, China).
2.4 DNA extraction and polymerase chain reaction (PCR)
Microbial DNA of algal BSCs was extracted using the E. Z.N.A.® (Omega Biotek, Norcross, GA, USA) kit. The V3–V4 hypervariable region of the bacterial 16S rRNA gene was amplified with a thermocycler PCR system (GeneAmp 9700, ABI, USA) using the specific primers 341F (5′-CCTAYGGGRBGCASCAG-3′) and 806R (5′-GGACTACNNGGGGTATCTAAT-3′). Purified amplicons were pooled in equal amounts and paired-end sequencing was performed on the Illumina MiSeq platform. The raw data were analyzed using QIIME software (v 1.9.0). Using the denoising algorithm (DADA2 method) of the QIIME2 pipeline, the raw reads of all samples were subjected to quality control, denoising, ligation and chimera removal to define the amplicon sequence variants (ASVs) (Han et al., 2024).
2.5 Statistical analysis
Data analyses were conducted using the R software (version 4.3.2). Alpha diversity indices including the ACE, Chao1, Simpson, and Shannon indices, were calculated based on Amplicon Sequence Variant (ASV) using the MicrobiotaProcess package. One-way analysis of variance (ANOVA) was performed to determine if there were significant differences between groups. Principal component analysis (PCA) was carried out using the PCA tools package. Non-metric multidimensional scaling (NMDS), Adonis results were calculated based on the Bray-Curtis distance using the vegan package, in order to verify the grouping validity. Functional taxa of algal BSC microorganisms were predicted by FAPROTAX (Liang et al., 2020). The circlize package was then utilized to create circus plots for a clear visualization of the functional taxonomic data.
For further exploration, we plotted ASV distribution maps using the UpSetR package. To analyze the relationships between environmental factors and microbial communities, we plotted mantel correlation heatmaps with the linkET package. The psych package was used to calculate the interspecies correlation. For a more in - depth analysis of the relationships between microbial species, we created network diagrams using Gephi software, version 1.0. To comprehensively evaluate the specific impacts of PV panel construction and physicochemical factors on the diversity and structure of algal BSC bacterial communities, we carried out hierarchical partitioning of the specific effects. This was achieved through a generalized linear mixed - effects model, using the rdacca. hp package in R. This allowed us to precisely assess the relative contributions of different factors to the observed variations in the microbial communities.
3 Results
3.1 Analysis of differences in physicochemical properties of algal BSCs in different areas of PV panel construction
PV panel construction caused significant differences in the physicochemical properties of algal BSCs (Figure 1). AN content increased by 1.3-fold (from 33.47 mg/kg to 43.53 mg/kg), while temperature (Tem) increased by 1.6-fold (from 8.12°C to 13.06°C), with AN and Tem showing consistent trends. DOC, NN and TC contents were all highest between the panels and lowest under the panels. Compared with the non-PV area, the contents of the three properties had increased by 1.1-fold (from 30.62 mg/kg to 32.31 mg/kg), 1.2-fold (from 184.84 mg/kg to 217.30 mg/kg) and 1.1-fold (from 26.62 g/kg to 28.53 g/kg), and decreased by 1.4-fold (from 30.62 mg/kg to 22.36 mg/kg), 1.2-fold (from 184.84 mg/kg to 151.56 mg/kg) and 1.5-fold (from 26.62 g/kg to 47.43 g/kg), respectively. TN content was highest in the non-PV area, with a 2.3-fold decrease (from 2.29 to 1.01 g/kg) between the panels and a 1.2-fold decrease (from 2.29 to 1.99 g/kg) under the panels. In addition, moisture (Moi), pH and SOC of algal BSCs did not respond significantly to PV panel construction.
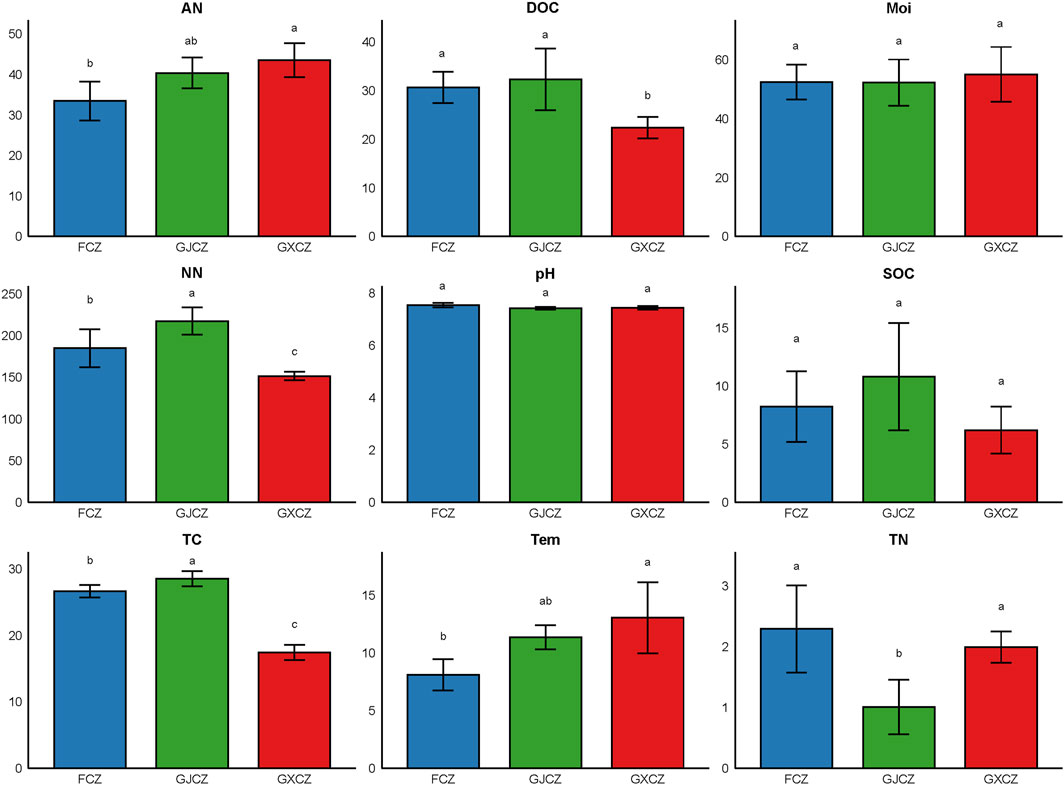
Figure 1. Physicochemical properties of algal BSCs in different areas of PV panel construction. Note: TN, total nitrogen of algal BSCs; TC, total carbon of algal BSCs; SOC, soil organic carbon of algal BSCs; NN, nitrate nitrogen; AN, ammonium nitrogen; Moi, moisture of algal BSCs; pH, pH value of algal BSCs; DOC, dissolved organic carbon of algal BSCs; Tem, temperature of algal BSCs. Different lowercase letters for the same factor indicate significant differences (P < 0.05) between different areas of PV panel construction.
3.2 Diversity analysis of algal crust bacterial communities in different areas of PV panel construction
The rarefaction curves of individual samples reaching a plateau indicated that the sequencing depths were sufficient (Figure 2a). The impact of PV panel construction on the Alpha diversity of the bacterial communities was relatively small (Figure 2b, P > 0.05). Within the context of PV panel construction, the richness of microbial species increased under the panels and decreased between the panels; the species evenness of microorganisms was the highest in the non-PV area and decreased in the presence of PV panel construction (Figure 2b). PCA revealed that the heterogeneity of algal BSCs in desert grasslands was relatively large, and the bacterial communities of algal BSCs were affected by PV panel construction (Figure 2c). Grouping validity was further verified by NMDS, Adonis and Anosim, which showed that the construction of PV panels led to significant differences in bacterial communities (P < 0.05; Figure 2d).
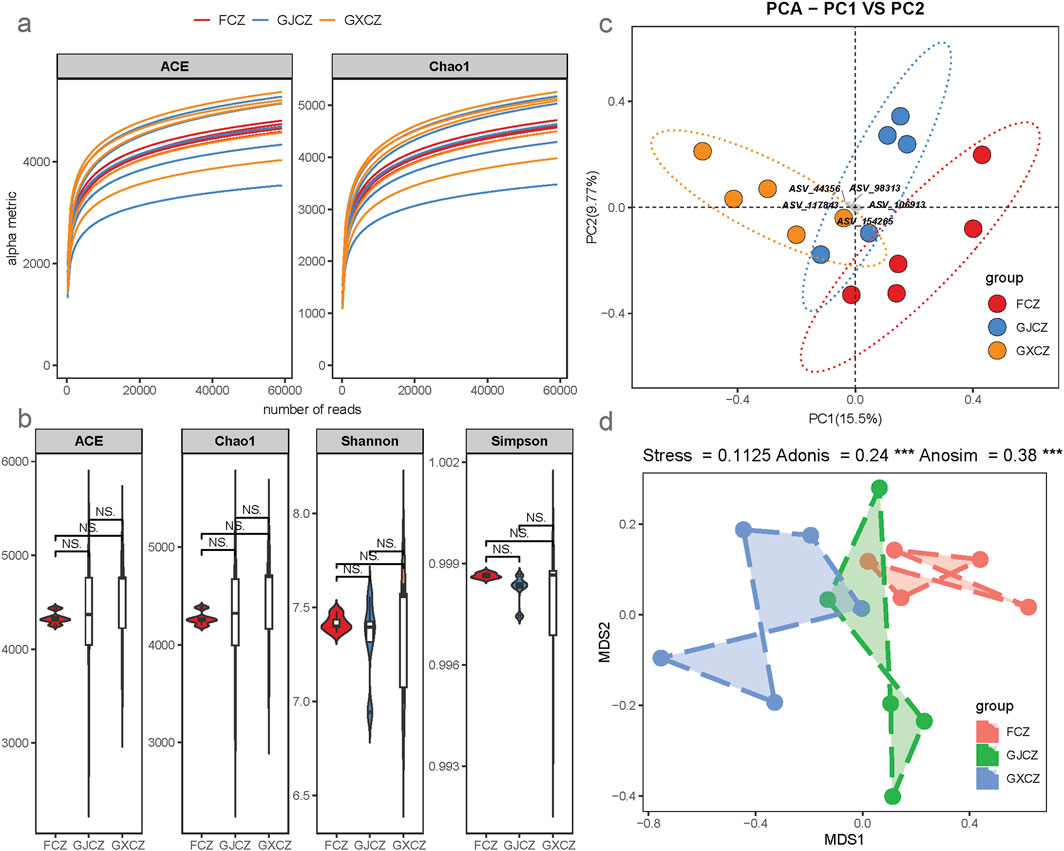
Figure 2. Characteristics of algal BSC bacterial diversity in different areas of PV panel construction; (a) sample dilution curves; (b) Alpha diversity indices; (c) PCA, principal component analysis; (d) Analysis of variance between groups. Note: NS indicates P > 0.05.
3.3 Analysis of bacterial community composition and differences of algal BSCs in different areas of PV panel construction
The dominant bacterial phyla of algal BSCs in desert grasslands (Figure 3) included Actinobacteriota (29.70%), Proteobacteria (17.86%), Chloroflexi (13.21%), Gemmatimonadota (11.36%) and Acidobacteriota (10.20%), which accounted for more than 70% of the total sequences. At the genus level, uncultivated bacteria accounted for the highest percentage in desert grasslands, with a relative abundance of 14.90%. Linear discriminant analysis effect size (LefSe) (Figure 4b) showed that 12 taxa were significantly affected by PV panel construction, and the number of differential taxa from the phylum to the genus level was 3, 3, 2, 2, 2 and 2, respectively. The differential taxa at the phylum and genus levels were all dominant taxa of desert grasslands. Among which, the phylum Proteobacteria and Myxococcota had the highest relative abundance under the panels (GXCZ), whereas the remaining taxa were more dominant under natural conditions (FCZ). In addition, the number of sequences obtained from the bacterial communities of the 15 samples ranged between 119,550 -140,701. Sequences were clustered into ASVs by the DADA2 method. The number of ASVs shared among the different groups was 2,408. The total number of ASVs for FCZ, GXCZ and GJCZ were 14,736, 15,652 and 14,873, respectively, while the number of unique ASVs were 10,575, 11,513 and 10,255, respectively (Figure 4a). The highest number of total ASVs and unique ASVs were found under the PV panel.
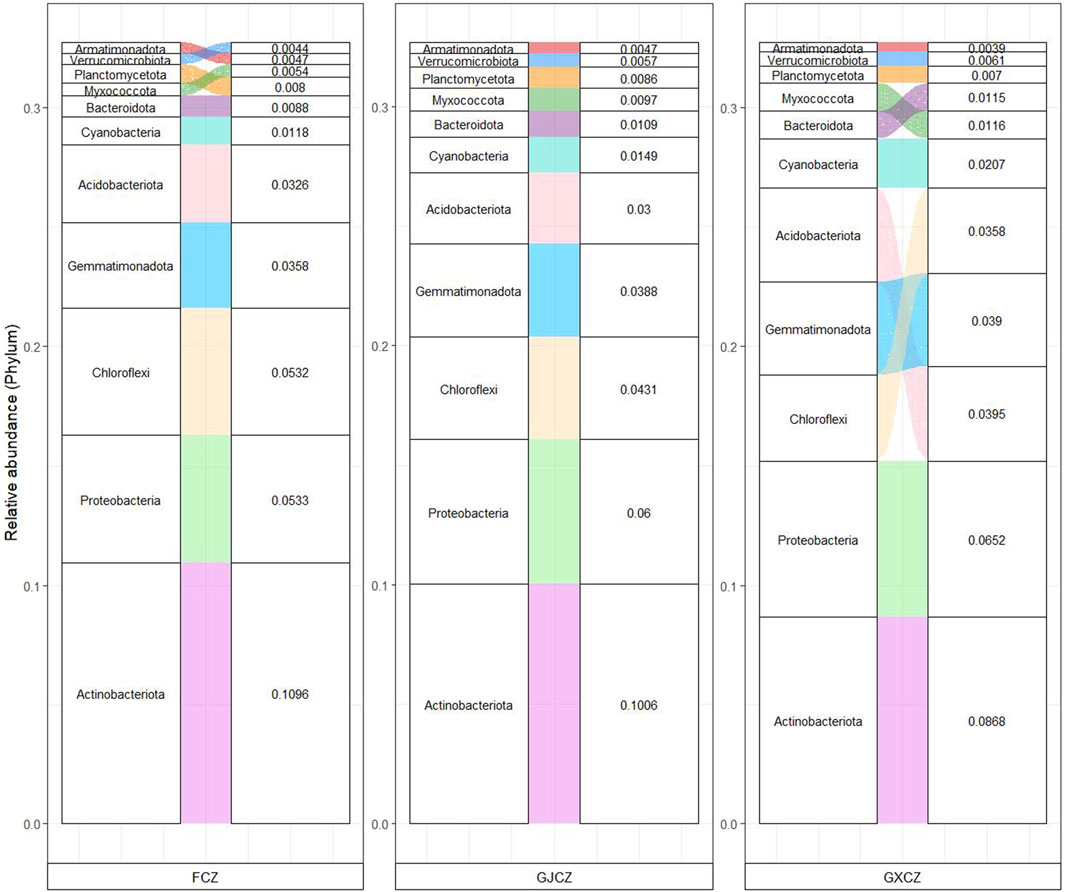
Figure 3. Phylum-level bacterial community composition of algal BSCs in different areas of PV panel construction.
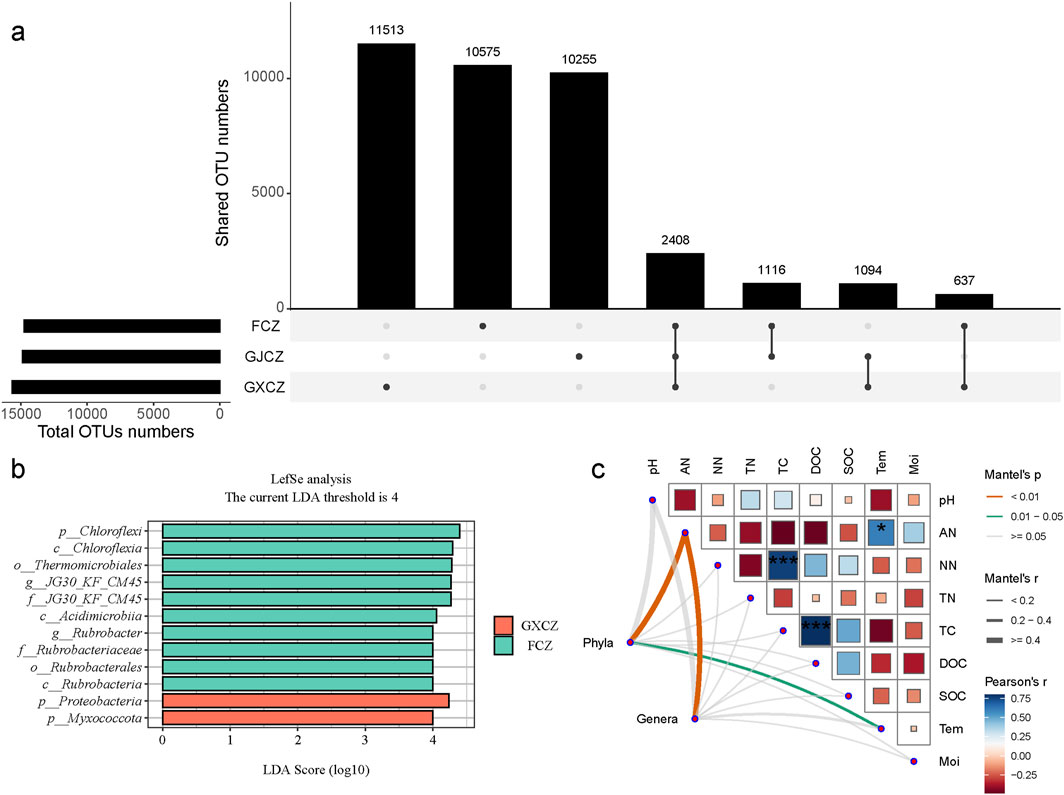
Figure 4. Differences in algal BSC bacterial communities in different areas of PV panel construction and correlation analysis with physicochemical factors; (a) ASV distribution map; (b) LEfSe differential taxa analysis; (c) Correlation analysis between bacterial community structure and physicochemical factors. Note: * indicates P < 0.05, *** indicates P < 0.001.
3.4 Correlation analysis between physicochemical properties of algal BSCs and bacterial community composition in different areas of PV panel construction
Mantel correlation analysis (Figure 4c) showed that dominant phyla were mainly and significantly influenced by AN and Tem. Conversely, the dominant genera were mainly and significantly affected by AN only. Environmental factors were mostly negatively correlated with each other, but this correlation was not significant. In addition, AN showed a significant positive correlation with Tem, while NN and DOC exhibited highly significant positive correlations with TC. In order to analyze the specific effects of environmental factors on bacterial communities, hierarchical partitioning analysis was performed using a generalized linear model (Figure 5). The results showed that PV panel construction, TC, DOC and Tem explained most of the variation in the bacterial community structure of desert grasslands. Community structure was strongly affected by PV construction, while TC was the most important environmental factor, followed by DOC and Tem (Figure 5a). Bacterial community diversity did not respond significantly to PV panel construction. Tem and SOC were the predominant drivers of community diversity, while Moi was also an important driver (Figure 5b).
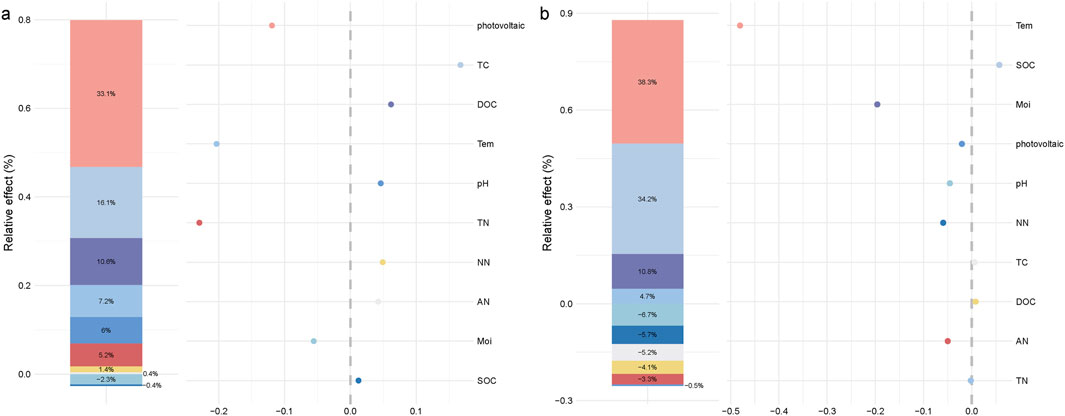
Figure 5. Hierarchical partitioning analysis of factors influencing the bacterial community structure and diversity of algal BSCs; (a) Hierarchical partitioning analysis of factors influencing bacterial community structure; (b) Hierarchical partitioning analysis of factors influencing bacterial community diversity.
3.5 Functional group analysis of algal BSC bacterial communities in different areas of PV panel construction
Functional taxonomic annotation of algal BSC bacteria in alpine grasslands (Figure 6a) revealed that the functions of the bacterial communities were primarily concentrated in several key areas. These included chemoheterotrophy (32.40%), aerobic_chemoheterotrophy (31.72%), phototrophy (5.38%), photoautotrophy (5.19%), oxygenic_photoautotrophy (5.12%), photosynthetic_cyanobacteria (5.12%), nitrate_reduction (4.76%), chloroplasts (1.94%), aromatic_compound_degradation (1.44%), and predatory_or_exoparasitic (1.08%). Collectively, these functions accounted for over 90% of the total sequences. Statistical analysis (Figure 6b) indicated that PV construction significantly changed the relative abundance of nitrate_reduction and chloroplasts. Regarding nitrate_reduction, it had the highest relative abundance in the non - PV area and the lowest under the PV panels, with a significant difference between these two conditions. The relative abundance between the panels was intermediate, and the differences were not significant. In contrast, the pattern for chloroplasts was the opposite. Reverse localization analysis showed that these two functional groups were mainly distributed among 18 genus - level taxa from eight phyla. Among them, only the chloroplasts of Cyanobacteria exhibited the chloroplast function (Figure 6c).
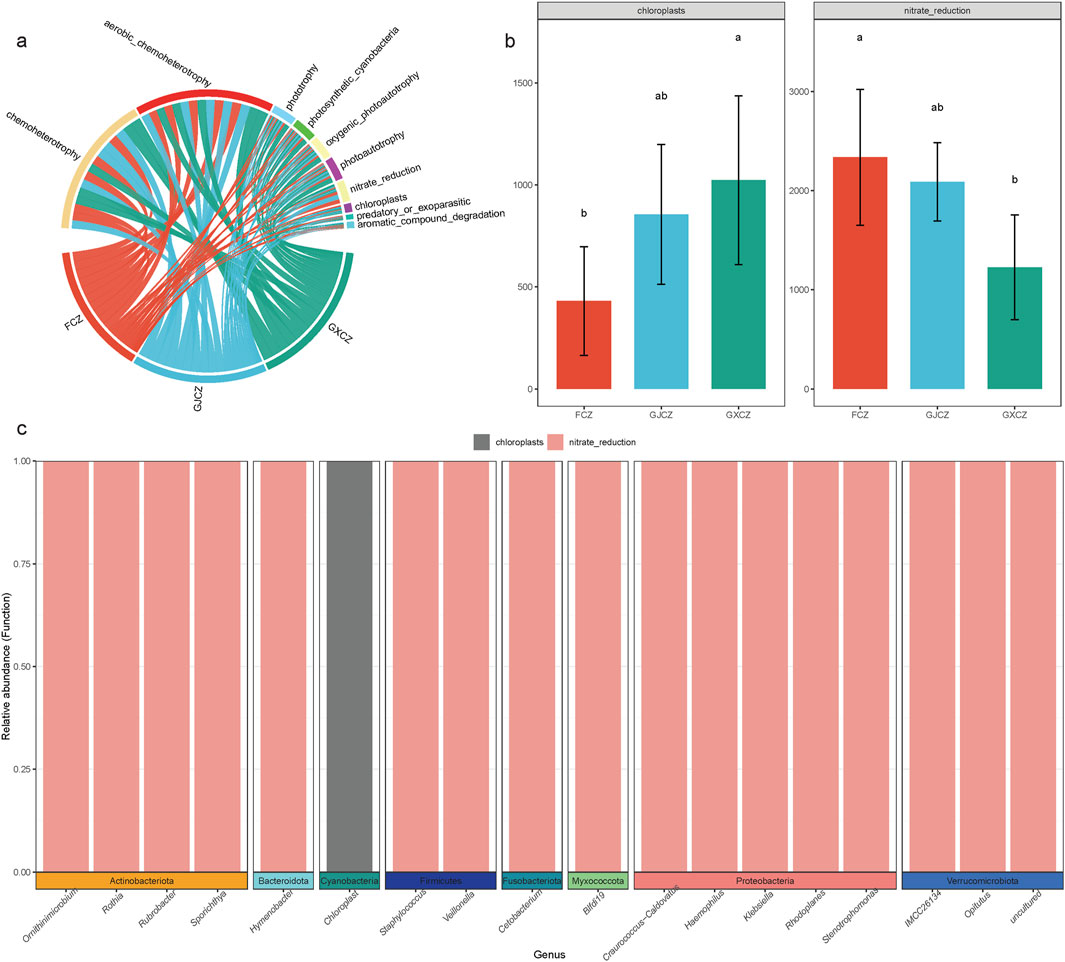
Figure 6. Functional groups of algal BSC bacteria in PV construction area; (a) Main functional groups of algal BSC bacteria; (b) Differential functional groups of algal BSC bacteria in different PV construction areas; (c) Algal BSC microbial taxa corresponding to differential functional groups. Note: ab indicates significance, the same letter indicates P > 0.05, and different letters indicate P < 0.05.
3.6 Network analysis of algal BSC bacterial communities in different areas of PV panel construction
The topological properties exhibited distinct trends among different treatments (Figure 7). PV panel construction led to an increase in the complexity of the bacterial network in algal BSCs under the panels, with tighter connectivity between species, whereas a decrease in network complexity was found between panels (Figure 7). This was manifested by a tighter connectivity among species. In contrast, a decrease in network complexity was observed between the panels. Specifically, the bacterial network under the panels was characterized by an augmentation in both the total number of nodes and edges. Between the panels, although the total number of nodes increased, the total number of edges decreased, especially a reduction in the number of negative correlations (Figure 7). Furthermore, the bacterial network under the panels showed an increase in average degree and modularization (Figure 7), suggesting that PV plate construction enhanced the interconnectivity between bacterial species. In the bacterial network of algal BSCs, the proportion of positive correlations increased from 47.98% (non-PV area) to 53.97% (between PV panels) and decreased to 50.29% (under PV panels).
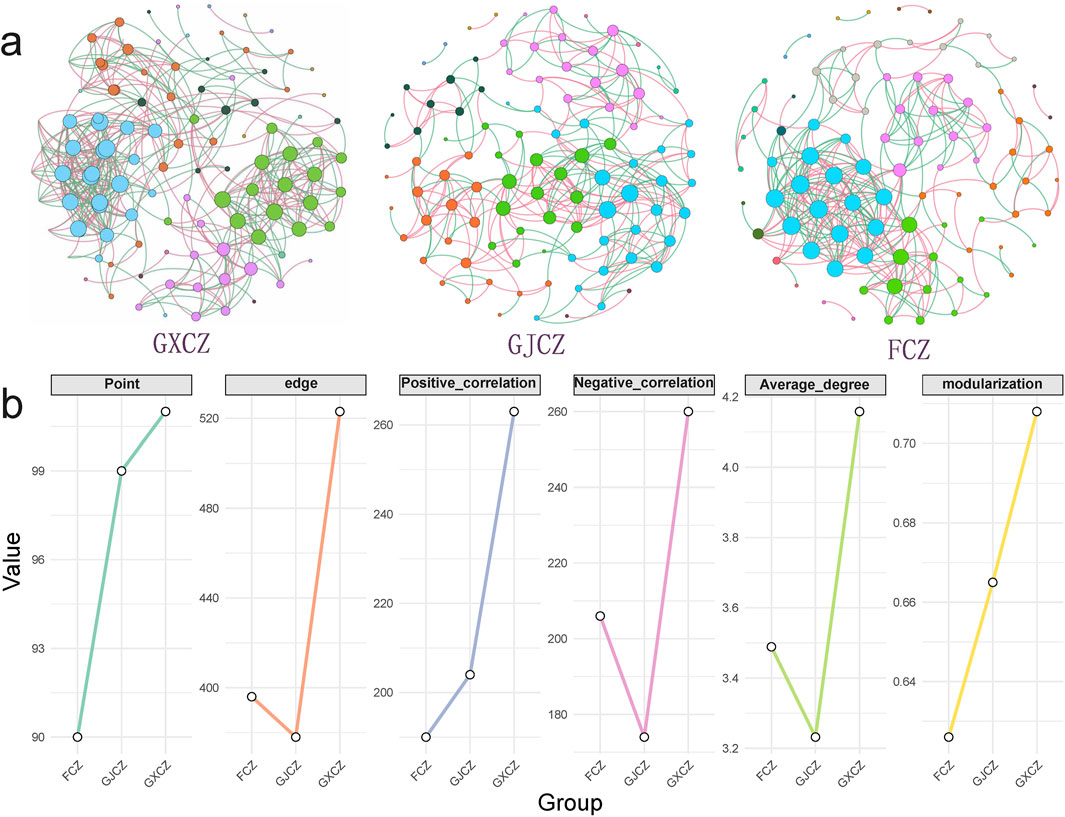
Figure 7. Bacterial network patterns (a) and main topological characteristics (b) of algal BSCs in different PV construction areas. Note: The size of the nodes indicates the degree. Node colors represent different modules. Edge colors indicate positive or negative correlation, with red for positive correlation and green for negative correlation. GXCZ, under PV panels; GJCZ, between PV panels; FCZ, non-PV construction area.
4 Discussion
4.1 Effect of PV panels on microbial diversity and structure of algal BSCs
The biodiversity and richness of microbial communities play a crucial role in maintaining the integrity, function, and long - term sustainability of soil ecosystems. However, they are often subject to changes due to environmental disturbances (Zhao et al., 2014). In this study, we observed distinct changes in microbial characteristics. Compared with the natural control, the richness of microbial species increased under the PV panels but decreased between the panels. The evenness of microbial species was highest in the area without PV panel construction and declined in the presence of PV panels. This change might be due to the redistribution of precipitation caused by the orientation of the PV panels. The results of previous studies on this topic are inconsistent. For example, Jia et al. (2024) investigated the bacterial community in the PV panel construction area of the Songnen Plain and found a decreasing trend in bacterial Alpha diversity indices under the PV panels, which contradicts our findings. In contrast, Wang et al. (2024) examined soil bacterial communities in PV arrays in western Jilin and showed that PV panel construction increased soil bacterial Alpha diversity indices, opposing the results of Jia et al. Bai et al. (2022) also conducted research in the PV panel construction area of the Songnen Plain. They found that PV panels increased bacterial richness under the panels while reducing the bacterial evenness index, which supports our findings.
Under the influence of PV panel construction, the dominant bacterial phyla in BSCs were Actinobacteriota and Proteobacteria. This result is consistent with previous studies (Jia et al., 2024; Bai et al., 2022; Ding and Liu, 2021). Actinobacteria is a highly abundant bacterial phylum. It can utilize carbon and nitrogen substances such as lignin, hemicellulose, protein, and cellulose to grow rapidly and regulate the soil nutrient balance (Dai et al., 2018; Liu et al., 2021). Proteobacteria has been reported to be significantly enriched in disturbed environments. Their extensive biodegradation and metabolic properties enable them to utilize a wide range of carbon sources and quickly adapt to various habitats (Xiao et al., 2021; Sinkko et al., 2013). These characteristics further explain their high abundance in our study. The LEfSe analysis indicated that the relative abundance of three phyla changed significantly. The relative abundance of Proteobacteria and Myxococcota increased significantly, while that of Chloroflexi decreased significantly under the PV panels. This suggests that PV panel construction had a significant impact on the bacterial community structure of BSCs. Co-occurrence network analyses can reveal potential co-existence patterns, showing how microorganisms interact not only between paired taxa but also across different domains (Faust, 2021; Neu et al., 2021). In alpine desert grasslands, PV panel construction led to an increase in the complexity of the bacterial network in algal BSCs under the panels, with closer connectivity between species. In contrast, the network complexity decreased between the panels. This finding implies that PV panel construction enhances the stability of the microbial community under the panels but reduces it between the panels (Yuan et al., 2021). Moreover, it offers an effective approach for in - depth analysis of how the development of BSCs affects the structure of bacterial communities.
4.2 Correlation analysis of algal BSC microbial communities and environmental factors in the presence of PV panel construction
Microbial community changes are propelled by a complex interplay of multiple environmental factors in the habitat. Even a minor alteration in any single environmental factor can, to a certain degree, reshape the soil bacterial community (Li et al., 2017; Burns et al., 2013). In this study, we observed that PV panel construction brought about substantial changes to the soil microenvironment. Specifically, there were statistically significant differences in soil Available Nitrogen (AN), Nitrate Nitrogen (NN), Total Carbon (TC), Soil Organic Carbon (SOC), and Temperature (Tem) under the PV panels. Among these, the levels of NN, TC, and SOC decreased. This reduction might be attributed to the removal of top - soil during the construction of PV arrays, which disrupted the soil texture and subsequently led to changes in soil nutrient content (Choi et al., 2020).
Hierarchical partitioning analysis based on a generalized linear model revealed that TC, SOC, and Tem were the key determinants of the BSC bacterial community structure in alpine desert grasslands. Meanwhile, Tem, SOC, and Moisture (Moi) were the primary drivers of bacterial community diversity. These findings deviate from those of previous research. For instance, Zu et al. (2016) concluded that soil pH was the most reliable predictor of Alpha diversity. Jia et al. (2024) conducted a study in the PV construction area on the Songnen Plain and found that pH, salinity, and Total Nitrogen (TN) were significant factors influencing bacterial community composition. Wang et al. (2024) also explored the effects of PV arrays on the composition and diversity of soil bacterial communities in the Songnen Plain and identified soil pH, TC, TN, and SOC as the main factors affecting soil bacterial communities. However, Zhang et al. (2022), in their study of grassland soil microbial communities, did not find pH to be a crucial factor. Instead, they posited that high SOC enhances substrate availability, while soil moisture dictates soil texture, oxygen availability, and soil connectivity. These two factors can significantly impact microbial communities by altering nutrient availability.
Moreover, fluctuations in precipitation and temperature typically influence soil microbial communities by directly or indirectly modifying the growth and activities of soil microorganisms (Bardgett et al., 2008; Ren et al., 2018). The above results imply that the impact of PV panel construction on alpine desert grasslands differs somewhat from that in other regions. This discrepancy may stem from changes in certain key influencing factors within the ecosystem, such as precipitation, soil erosion, surface runoff, vegetation characteristics, and wind speed, in PV panel construction areas (Barron-Gafford et al., 2019; Moscatelli et al., 2022).
5 Conclusion
This study, centered on the Taratan PV construction park, comprehensively elucidated the underlying mechanism of how PV construction impacts the bacterial communities within algal Biological Soil Crusts (BSCs) in alpine desert grasslands. Notably, the diversity of the bacterial community did not exhibit a significant response to PV panel construction. However, the installation of PV panels significantly elevated the relative abundance of the phylum Proteobacteria and remarkably enhanced the interconnectivity among bacterial species. This enhancement played a crucial role in contributing to the stability of the alpine desert grassland ecosystem. Moreover, PV panel construction led to a significant increase in temperature and a significant reduction in soil carbon stock. These two factors, temperature and soil carbon stock, were identified as the two most crucial and significant elements influencing the structure and diversity of algal BSC bacterial communities in alpine desert grasslands. In summary, the construction of PV panels augmented the stability of algal BSC bacterial communities while decreasing the carbon stock in alpine desert steppes. This research offers novel insights into the regulatory mechanisms of PV panel construction on microorganisms within the alpine desert grassland ecosystem, thereby providing valuable theoretical support for a more in - depth understanding of the ecological impacts of PV development in such unique environments.
Data availability statement
The datasets presented in this study can be found in online repositories. The names of the repository/repositories and accession number(s) can be found below: https://www.ncbi.nlm.nih.gov/, PRJNA1178580.
Author contributions
DQ: Writing – original draft, Investigation, Writing – review and editing. XZ: Data curation, Investigation, Writing – review and editing. NZ: Data curation, Investigation, Writing – review and editing. ZY: Data curation, Writing – review and editing. YC: Software, Writing – review and editing. KC: Conceptualization, Funding acquisition, Writing – review and editing.
Funding
The author(s) declare that financial support was received for the research and/or publication of this article. This research was funded by the National Natural Science Foundation of China (42461018) and the Qinghai Province science and technology plan (2023-ZJ-905T).
Conflict of interest
The authors declare that the research was conducted in the absence of any commercial or financial relationships that could be construed as a potential conflict of interest.
Generative AI statement
The author(s) declare that no Generative AI was used in the creation of this manuscript.
Publisher’s note
All claims expressed in this article are solely those of the authors and do not necessarily represent those of their affiliated organizations, or those of the publisher, the editors and the reviewers. Any product that may be evaluated in this article, or claim that may be made by its manufacturer, is not guaranteed or endorsed by the publisher.
Supplementary material
The Supplementary Material for this article can be found online at: https://www.frontiersin.org/articles/10.3389/fenvs.2025.1552071/full#supplementary-material
SUPPLEMENTARY FIGURE S1 | Field map of the sample plot.
SUPPLEMENTARY FIGURE S2 | Photovoltaic panel between the panels.
SUPPLEMENTARY FIGURE S3 | Photovoltaic panel under the plate.
SUPPLEMENTARY FIGURE S4 | Non-photovoltaic panel construction area.
References
Armstrong, A., Ostle, N. J., and Whitaker, J. (2016). Solar park microclimate and vegetation management effects on grassland carbon cycling. Environ. Res. Lett. 11, 074016. doi:10.1088/1748-9326/11/7/074016
Armstrong, A., Waldron, S., Whitaker, J., and Ostle, N. J. (2014). Wind farm and solar park effects on plant soil carbon cycling: uncertain impacts of changes in ground level microclimate. Glob. change boil 20, 1699–1706. doi:10.1111/gcb.12437
Bai, Z., Jia, A., Bai, Z., Qu, S., Zhang, M., Kong, L., et al. (2022). Photovoltaic panels have altered grassland plant biodiversity and soil microbial diversity. Front. Microbiol. 13, 1065899. doi:10.3389/fmicb.2022.1065899
Bardgett, R. D., Freeman, C., and Ostle, N. J. (2008). Microbial contributions to climate change through carbon cycle feedbacks. ISME J. 2, 805–814. doi:10.1038/ismej.2008.58
Barron-Gafford, G. A., Pavao-Zuckerman, M. A., Minor, R. L., Sutter, L. F., Barnett-Moreno, I., Blackett, D. T., et al. (2019). Agrivoltaics provide mutual benefits across the food-energy-water nexus in drylands. Nat. Sustain 2, 848–855. doi:10.1038/s41893-019-0364-5
Bowker, M. A., Belnap, J., Chaudhary, V. B., and Johnson, N. C. (2008). Revisiting classic water erosion models in drylands: the strong impact of biological soil crusts. Soil Biol. biochem. 40, 2309–2316. doi:10.1016/j.soilbio.2008.05.008
Burns, R. G., Deforest, J., Marxsen, J., Sinsabaugh, R., Stromberger, M. E., Wallenstein, M., et al. (2013). Soil enzymes in a changing environment: current knowledge and future directions. Soil Biol. Biochem. 58, 216–234. doi:10.1016/j.soilbio.2012.11.009
Castillo-Monroy, A. P., Bowker, M. A., Maestre, F. T., Rodríguez-Echeverría, S., Martinez, I., Barraza-Zepeda, C. E., et al. (2011). Relationships between biological soil crusts, bacterial diversity and abundance, and ecosystem functioning: insights from a semi-arid Mediterranean environment. J. Veg. Sci. 22, 165–174. doi:10.1111/j.1654-1103.2010.01236.x
Chang, R., Luo, Y., and Zhu, R. (2020). Simulated local climatic impacts of large-scale photovoltaics over the barren area of Qinghai, China. Renew. energy 145, 478–489. doi:10.1016/j.renene.2019.06.059
Che, R., Qin, J., Tahmasbian, I., Wang, F., Zhou, S., Xu, Z., et al. (2018). Litter amendment rather than phosphorus can dramatically change inorganic nitrogen pools in a degraded grassland soil by affecting nitrogen-cycling microbes. Soil Biol. biochem. 120, 145–152. doi:10.1016/j.soilbio.2018.02.006
Chen, H., Zhu, Q. A., Peng, C. H., Wu, N., Wang, Y. F., Fang, X. Q., et al. (2013). The impacts of climate change and human activities on biogeochemical cycles on the Qinghai-Tibetan plateau. Glob. Chang. Biol. 19, 2940–2955. doi:10.1111/gcb.12277
Choi, C. S., Cagle, A. E., Macknick, J., Bloom, D. E., Caplan, J. S., and Ravi, S. (2020). Effects of revegetation on soil physical and chemical properties in solar photovoltaic infrastructure. Front. Environ. Sci. 8, 140. doi:10.3389/fenvs.2020.00140
Dai, Z., Su, W., Chen, H., Barberán, A., Zhao, H., Yu, M., et al. (2018). Long-term nitrogen fertilization decreases bacterial diversity and favors the growth of Actinobacteria and Proteobacteria in agro-ecosystems across the globe. Glob. Chang. Biol. 24, 3452–3461. doi:10.1111/gcb.14163
Dhar, A., Naeth, M. A., Jennings, P. D., and Gamal El-Din, M. (2020). Perspectives on environmental impacts and a land reclamation strategy for solar and wind energy systems. Sci. Total Environ. 718, 134602. doi:10.1016/j.scitotenv.2019.134602
Ding, C. X., and Liu, Y. (2021). Effects of solar photovoltaic park construction on soil microbial community in alpine desert of Qinghai-Tibetan Plateau. Acta Agrestia Sin. 29, 1061–1069. doi:10.11733/j.issn.1007-0435.2021.05.023
Ding, J. Z., Li, F., Yang, G. B., Chen, L. Y., Zhang, B. B., Liu, L., et al. (2016). The permafrost carbon inventory on the Tibetan Plateau: a new evaluation using deep sediment cores. Glob. Chang. Biol. 22, 2688–2701. doi:10.1111/gcb.13257
Dong, S., Wen, L., Zhu, L., and Li, X. (2010). Implication of coupled natural and human systems in sustainable rangeland ecosystem management in HKH region. Front. Earth Sci. China 4, 42–50. doi:10.1007/s11707-010-0010-z
Dong, S. K., Shang, Z. H., Gao, J. X., and Boone, R. (2022). Enhancing the ecological services of the Qinghai-Tibetan Plateau’s grasslands through sustainable restoration and management in era of global change. Agric. Ecosyst. Environ. 326, 107756. doi:10.1016/j.agee.2021.107756
Elbert, W., Weber, B., Burrows, S., Steinkamp, J., Büdel, B., Andreae, M. O., et al. (2012). Contribution of cryptogamic covers to the global cycles of carbon and nitrogen. Nat. Geosci. 5, 459–462. doi:10.1038/ngeo1486
Eldridge, D. J., Reed, S. C., Travers, S. K., Bowker, M. A., Maestre, F. T., Ding, J., et al. (2020). The pervasive and multifaceted influence of biocrusts on water in the world's drylands. Glob. Chang. Biol. 26, 6003–6014. doi:10.1111/gcb.15232
Faust, K. (2021). Open challenges for microbial network construction and analysis. ISME J. 15, 3111–3118. doi:10.1038/s41396-021-01027-4
Ganjurjav, H., Gao, Q., Gornish, E. S., Schwartz, M. W., Liang, Y., Cao, X., et al. (2016a). Differential response of alpine steppe and alpine meadow to climate warming in the central Qinghai–Tibetan Plateau. Agric. For. Meteorol 223, 233–240. doi:10.1016/j.agrformet.2016.03.017
Ganjurjav, H., Gao, Q., Schwartz, M. W., Zhu, W., Liang, Y., Li, Y., et al. (2016b). Complex responses of spring vegetation growth to climate in a moisture-limited alpine meadow. Sci. Rep. 6, 23356–23410. doi:10.1038/srep23356
Gao, X. Q., Yang, L. W., and Lv, F. (2016). Observational study on the impact of the large solar farm on air tempera-ture and humidity in desert areas of Golmud. Acta Energiae Solaris Sin. 11, 2909–2915. doi:10.19912/j.0254-0096.2016.11.027
Grote, E. E., Belnap, J., Housman, D. C., and Sparks, J. P. (2010). Carbon exchange in biological soil crust communities under differential temperatures and soil water contents: implications for global change. Glob. Change Biol. 16, 2763–2774. doi:10.1111/j.1365-2486.2010.02201.x
Han, L., Chen, L., Feng, Y., Kuzyakov, Y., Chen, Q., Zhang, S., et al. (2024). Microplastics alter soil structure and microbial community composition. Environ. Int. 185, 108508. doi:10.1016/j.envint.2024.108508
He, D., Xiang, X., He, J. S., Wang, C., Cao, G., Adams, J., et al. (2016). Composition of the soil fungal community is more sensitive to phosphorus than nitrogen addition in the alpine meadow on the Qinghai-Tibetan Plateau. Biol. Fertil. Soils 52, 1059–1072. doi:10.1007/s00374-016-1142-4
Hma, W., Li, J., Gao, Y., Xing, F., Sun, S., Zhang, T., et al. (2020). Responses of soil extracellular enzyme activities and microbial community properties to interaction between nitrogen addition and increased precipitation in asemi-arid grassland ecosystem. Sci. Total Environ. 703, 134691. doi:10.1016/j.scitotenv.2019.134691
Jia, A., Bai, Z., Gong, L., Li, H., and Wang, M. (2024). Effects of organic fertilizer addition to vegetation and soil bacterial communities in saline-alkali-degraded grassland with photovoltaic panels. Plants (Basel) 13, 1491. doi:10.3390/plants13111491
Li, X., Hui, R., Tan, H., Zhao, Y., Liu, R., and Song, N. (2021). Biocrust research in China: recent progress and application in land degradation control. Front. Plant Sci. 12, 751521. doi:10.3389/fpls.2021.751521
Li, Y., Adams, J., Shi, Y., Wang, H., He, J. S., and Chu, H. (2017). Distinct soil microbial communities in habitats of differing soil water balance on the Tibetan Plateau. Sci. Rep. 7, 46407. doi:10.1038/srep46407
Liang, S., Deng, J., Jiang, Y., Wu, S., Zhou, Y., and Zhu, W. (2020). Functional distribution of BacterialCommunity under different land use PatternsBased on FaProTax function prediction. J. Env. Stud. 29, 1245–1261. doi:10.15244/pjoes/108510
Liu, M., Zhang, Z., Sun, J., Li, Y., Liu, Y., Liyew Berihun, M., et al. (2020). Restoration efficiency of short-term grazing exclusion is the highest at the stage shifting from light to moderate degradation at Zoige, Tibetan Plateau. Ecol. Indic. 114, 106323. doi:10.1016/j.ecolind.2020.106323
Liu, Q., Xie, S., Zhao, X., Liu, Y., Xing, Y., Dao, J., et al. (2021). Drought sensitivity of sugarcane cultivars shapes rhizosphere bacterial community patterns in response to water stress. Front. Microbiol. 12, 732989. doi:10.3389/fmicb.2021.732989
Liu, Y., Zhang, R. Q., Huang, Z., Cheng, Z., López-Vicente, M., Ma, X., et al. (2019). Solar photovoltaic panels significantly promote vegetation recovery by modifying the soil surface microhabitats in an arid sandy ecosystem. Land Degrad. Dev. 30, 2177–2186. doi:10.1002/ldr.3408
Mager, D. M., and Thomas, A. D. (2011). Extracellular polysaccharides from cyanobacterial soil crusts: a review of their role in dryland soil processes. J. Arid. Environ. 75, 91–97. doi:10.1016/j.jaridenv.2010.10.001
Maier, S., Tamm, A., Wu, D., Caesar, J., Grube, M., and Weber, B. (2018). Photoautotrophic organisms control microbial abundance, diversity, and physiology in different types of biological soil crusts. ISME J. 12, 1032–1046. doi:10.1038/s41396-018-0062-8
Mogul, R., Vaishampayan, P., Bashir, M., McKay, C. P., Schubert, K., Bornaccorsi, R., et al. (2017). Microbial community and biochemical dynamics of biological soil crusts across a gradient of surface coverage in the central Mojave desert. Front. Microbiol. 8, 1974. doi:10.3389/fmicb.2017.01974
Moscatelli, M. C., Marabottini, R., Massaccesi, L., and Marinari, S. (2022). Soil properties changes after seven years of ground mounted photovoltaic panels in Central Italy coastal area. Geoderma Reg. 29, e00500. doi:10.1016/j.geodrs.2022.e00500
Neu, A. T., Allen, E. E., and Roy, K. (2021). Defining and quantifying the core microbiome: challenges and prospects. Proc. Natl. Acad. Sci. 118, e2104429118. doi:10.1073/pnas.2104429118
Piao, S. L., Tan, K., Nan, H. J., Ciais, P., Fang, J. Y., Wang, T., et al. (2012). Impacts of climate and CO2 changes on the vegetation growth and carbon balance of Qinghai-Tibetan grasslands over the past five decades. Glob. Planet Change 98-99, 73–80. doi:10.1016/j.gloplacha.2012.08.009
Qiu, J. (2016). Trouble in Tibet: rapid changes in Tibetan grasslands are threatening Asia’s main water supply and the livelihood of nomads. Nature 529, 142–146. doi:10.1038/529142a
Ren, C. J., Chen, J., Lu, X. J., Doughty, R., Zhao, F. Z., Zhong, Z. K., et al. (2018). Responses of soil total microbial biomass and community compositions to rainfall reductions. Soil Biol. Biochem. 116, 4–10. doi:10.1016/j.soilbio.2017.09.028
Rodriguez-Caballero, E., Belnap, J., Burkhard, B., Crutzen, P. J., Andreae, M. O., Pschl, U., et al. (2018). Dryland photoautotrophic soil surface communities endangered by global change. Nat. Geosci. 11, 185–189. doi:10.1038/s41561-018-0072-1
Salamanca, F., Georgescu, M., Mahalov, A., Moustaoui, M., and Martilli, A. (2016). Citywide impacts of cool roof and rooftop solar photovoltaic deployment on near-surface air temperature and cooling energy demand. Boundary-lay Meteorol. 161, 203–221. doi:10.1007/s10546-016-0160-y
Sinkko, H., Lukkari, K., Sihvonen, L. M., Sivonen, K., Leivuori, M., Rantanen, M., et al. (2013). Bacteria contribute to sediment nutrient release and reflect progressed eutrophication-driven hypoxia in an organic-rich continental sea. PLoS ONE 8, e67061. doi:10.1371/journal.pone.0067061
Wang, J., Wang, X., Liu, G., Wu, Y., and Zhang, C. (2020). Fencing as an effective approach for restoration of alpine meadows: evidence from nutrient limitation of soil microbes. Geoderma 363, 114148. doi:10.1016/j.geoderma.2019.114148
Wang, S. W., Wang, S. T., and Li, Y. (2024). Effects of photovoltaic arrays on soil bacterial community composition and diversity in the Songnen Plain. J. Northeast Normal Univ. Nat. Sci. Ed. 56, 107–114. doi:10.16163/j.cnki.dslkxb202311270004
Wang, Y., and Wesche, K. (2016). Vegetation and soil responses to livestock grazing in Central Asian grasslands: a review of Chinese literature. Biodivers. Conserv. 25, 2401–2420. doi:10.1007/s10531-015-1034-1
Watson, S. C., Preston, J., Beaumont, N. J., and Watson, G. J. (2020). Assessing the natural capital value of water quality and climate regulation intemperate marine systems using a EUNIS biotope classification approach. Sci. total Environ. 744, 140688. doi:10.1016/j.scitotenv.2020.140688
Weber, B., Büdel, B., and Belnap, J. (2016). Biological soil crusts as an organizing principle in drylands. Spring Int. Publ. 226, 3–13. doi:10.1007/978-3-319-30214-0_1
Weber, B., Wu, D., Tamm, A., Ruckteschler, N., Rodriguez-Caballero, E., Steinkamp, J., et al. (2015). Biological soil crusts accelerate the nitrogen cycle through large NO and HONO emissions in drylands. Proc. Natl. Acad. Sci. U. S. A. 112, 15384–15389. doi:10.1073/pnas.1515818112
Xiao, E. Z., Wang, Y. Q., Xiao, T. F., Sun, W. M., Deng, J. M., Jiang, S. M., et al. (2021). Microbial community responses to land-use types and its ecological roles in mining area. Sci. Total Environ. 775, 145753. doi:10.1016/j.scitotenv.2021.145753
Xu, S. L., Chen, L. D., Chen, C., Huang, Y., and Zhang, D. G. (2008). Impact of pipeline construction on agricultural soil nutrients in the Ji-Ning section of the West-East gas pipeline project. J. Agro-Environ Sci. 27, 627–635. doi:10.3321/j.issn:1672-2043.2008.02.042
Yan, G. S., Xin, R. L., Ying, W. C., Zhi, S. Z., and Yan, L. (2013). Carbon fixation of cyanobacterial–algal crusts after desert fixation and its implication to soil organic carbon accumulation in desert. Land Degrad. 24, 342–349. doi:10.1002/ldr.1131
Yang, C., and Sun, J. (2021). Impact of soil degradation on plant communities in an overgrazed Tibetan alpine meadow. J. Arid. Environ. 193, 104586. doi:10.1016/j.jaridenv.2021.104586
Yuan, M. M., Guo, X., Wu, L., Zhang, Y., Xiao, N., Ning, D., et al. (2021). Climate warming enhances microbial network complexity and stability. Nat. Clim. Chang. 11, 343–348. doi:10.1038/s41558-021-00989-9
Zeng, C., Zhang, F., Wang, Q., Chen, Y., and Joswiak, D. R. (2013). Impact of alpine meadow degradation on soil hydraulic properties over the Qinghai-Tibetan Plateau. J. Hydrol. 478, 148–156. doi:10.1016/j.jhydrol.2012.11.058
Zhang, B., Xue, K., Zhou, S., Wang, K., Liu, W., Xu, C., et al. (2022). Environmental selection overturns the decay relationship of soil prokaryotic community over geographic distance across grassland biotas. ELife 11, e70164. doi:10.7554/eLife.70164
Zhao, J., Zhang, R., Xue, C., Xun, W., Sun, L., Xu, Y., et al. (2014). Pyrosequencing reveals contrasting soil bacterial diversity and community structure of two main winter wheat cropping systems in China. Microb. Ecol. 67, 443–453. doi:10.1007/s00248-013-0322-0
Zhao, Y., Zhang, Z., Hu, Y., and Chen, Y. (2016). The seasonal and successional variations of carbon release from biological soil crust-covered soil. J. Arid. Environ. 127, 148–153. doi:10.1016/j.jaridenv.2015.11.012
Zhao, Z., Dong, S., Jiang, X., Liu, S., Ji, H., Li, Y., et al. (2017). Effects of warming and nitrogen deposition on CH4, CO2 and N2O emissions in alpine grassland ecosystems of the Qinghai-Tibetan Plateau. Sci. Total Environ. 592, 565–572. doi:10.1016/j.scitotenv.2017.03.082
Keywords: Qinghai-Tibetan plateau, biological soil crust, carbon cycle, photovoltaic parks, climate change
Citation: Qi D, Zhao X, Zhang N, Yang Z, Chen Y and Chen K (2025) Mechanistic insights into the influences of photovoltaic panel construction on algal crust microbial communities in alpine desert grasslands. Front. Environ. Sci. 13:1552071. doi: 10.3389/fenvs.2025.1552071
Received: 27 December 2024; Accepted: 14 April 2025;
Published: 29 April 2025.
Edited by:
Zhi-kun Chen, Xi’an Botanical Garden of Shaanxi Province, ChinaReviewed by:
Yi Wang, Chinese Academy of Sciences (CAS), ChinaYang Zhao, Chinese Academy of Sciences (CAS), China
Copyright © 2025 Qi, Zhao, Zhang, Yang, Chen and Chen. This is an open-access article distributed under the terms of the Creative Commons Attribution License (CC BY). The use, distribution or reproduction in other forums is permitted, provided the original author(s) and the copyright owner(s) are credited and that the original publication in this journal is cited, in accordance with accepted academic practice. No use, distribution or reproduction is permitted which does not comply with these terms.
*Correspondence: Kelong Chen, Y2tsNzgxM0AxNjMuY29t