- 1Department of Zoology, Poznan University of Life Sciences, Poznan, Poland
- 2Department of Animal Physiology, Biochemistry and Biostructure, Poznan University of Life Sciences, Poznan, Poland
Due to their high sensitivity to hormonal agents, in recent years, amphibians have been proposed as bioindicators for the presence of endocrine-disrupting compounds (EDCs) in the environment. EDCs are a chemically diverse group of compounds, mainly of anthropogenic origin, that can interfere with hormone receptors. The escalating global environmental pollution with endocrine-disrupting compounds (EDCs) poses a significant threat to wildlife and human health. Amphibians are at high risk of exposure to EDCs in the environment, as they produce eggs without a protective shell, possess highly permeable skin, and most of them undergo an aquatic life phase, where they are chronically exposed to EDCs in the water. This exposure raises concerns about the contribution of EDCs to the dramatic decline of amphibian populations and underscores the necessity of environmental-relevant studies in this area. Despite the critical need, amphibians have attracted relatively little research focus in this regard. Although above 200 experimental studies on the topic of EDCs and sex, reproduction, and related traits in amphibians have been conducted, a significant portion of them rely on model species from the Xenopus genus, which do not fully represent the diverse group of amphibians. Additionally, these studies often use strict laboratory conditions that fail to mimic natural exposure scenarios. Our extensive review highlights the research gaps and emphasizes the importance of incorporating a broader range of amphibian species to understand the true impact of EDCs. We advocate for more studies in environmentally relevant settings and the use of native species to enhance the ecological validity of findings.
1 Introduction
Amphibians have often been used in experiments concerning the impact of hormonal agents on reproductive parameters (Kloas et al., 2024). The most notable example includes the use of frogs from the Xenopus genus in the creation and validation of pregnancy tests (Wallingford, 2022). Due to their high sensitivity to hormonal agents, in recent years, amphibians have also been proposed as possible bioindicators of the presence of endocrine-disrupting compounds (EDCs) in the environment (Lambert et al., 2016). EDCs are concerning, mostly human-generated, exogenous substances that mimic the action of endogenous hormones (European Commission, 1996). EDCs are a chemically diverse group that includes mainly synthetic compounds present in, e.g., pesticides, plasticizers, surfactants, drugs, and personal care products. EDCs can interact with hormone receptors, or by other mechanisms, target estrogenic, androgenic, and thyroid pathways of action (for the review see La Merrill et al., 2020). These compounds are found in significant quantities in soil, atmosphere, and surface waters worldwide (Ankley et al., 2018; Annamalai and Namasivayam, 2015; Caliman and Gavrilescu, 2009; Singh et al., 2023).
Amphibians are at high risk of exposure to EDCs in the environment, as they possess highly permeable skin and produce eggs without a protective shell (Wells, 2010). Moreover, most of them undergo an aquatic life phase during embryonic and larval development, where they are chronically exposed to all compounds, such as EDCs, in the surrounding water. In the context of the development of body systems and sex differentiation taking place at this time, they are extremely susceptible to the potential harmful effects of EDCs (Huang et al., 2005; Kloas et al., 1999; Kloas, 2002). Because of this, amphibians can act as excellent bioindicators of EDCs’ presence in the environment (Lambert et al., 2016), which is important from the perspective of biomonitoring and human health, especially in a comprehensive approach, such as One Health. Studies on the impact of EDCs are crucial also because EDCs are suggested to contribute to the worldwide dramatic decline of amphibian populations, underlining the urgent need for more studies with environmental-relevant settings on this topic (Kloas et al., 2024; Luedtke et al., 2023; Salla et al., 2024a).
In comparison to other groups of vertebrates, particularly fish, amphibians are still an understudied group of animals in the context of endocrine disruptors (Johnson et al., 2017; Kloas and Lutz, 2006; Kloas et al., 2024). It is important to remember that limiting research to only selected taxa carries significant risks, with the most glaring example being the thalidomide scandal, which would not have had such disastrous effects had it been tested on a wider range of species. Thalidomide, a drug prescribed to pregnant women in the late 1950s and early 1960s, caused severe birth defects in thousands of children because its teratogenic effects were not detected in the limited animal models initially used for testing (Swaters et al., 2022). Apart from studying the impact of EDCs on different groups of vertebrates, the diversity of species should also be taken into account.
In the case of amphibians, some authors suggested, that popular ecotoxicological protocols, such as The Frog Embryo Teratogenesis Assay-Xenopus (FETAX) used by American Society of Testing and Materials (Dumont et al., 1983; Testing and Materials, 1998) for studying potential endocrine-disrupting effects of industrial substances are limited due to the use of the single species from the Xenopus genus. The good example is the ongoing discussion on the safety of pesticide atrazine (Hayes et al., 2002; Hoskins et al., 2019; LaFiandra et al., 2008; Spolyarich et al., 2010; Storrs and Semlitsch, 2008), which in the studies on Xenopus spp. and other amphibians produced different results. Species diversity in ecotoxicological studies is crucial when thinking about how these findings might apply to different ecosystems and the environment as a whole (Luedtke et al., 2023). The current study was designed as a literature review to explore research gaps in studies on the impact of endocrine-disrupting compounds (EDCs) on the amphibian reproductive system. A primary focus was placed on examining the diversity of species involved in existing research and identifying possible, correlated issues.
2 Methods of literature search
We extensively reviewed the studies published by the end of 2024 on the topic of EDCs and their impact on sex and sex-related traits of amphibians. As sex-related traits, we understood the sex accessory structures: genital ducts and derivatives of these structures; and the secondary sexual characteristics: all differences between the sexes resulting from sexual maturation, other than gonads and sex accessory structures (Sever and Staub, 2011). In the search, we also included the impact of EDCs on hormone signaling, expression of reproduction-related genes, sexually dimorphic behavior, mating, and fertility of amphibians. We focused on experimental studies. Analysis of research focused on EDCs and thyroid-dependent functions was beyond the scope of this review.
We used two electronic databases for the search: Web of Science Core Collection® and Google Scholar. In the database Web of Science Core Collection® the following Boolean search string was used: TS=(amphibia* OR frog* OR toad* OR newt* OR salamander* OR tadpole* OR caecilian* OR anura* OR caudata* OR urodela* OR apoda*) AND TS=(sex* OR gonad* OR test* OR ovar* OR hormone* OR vitellogenin OR reproduct* OR fertilit* OR oocyt* OR sperm* OR breeding* OR behaviour* OR nuptial pad* OR gene*) AND TI=(“endocrine disruptor*`” OR endocrine* OR EDC* OR pollutant* OR pharm* OR estro* OR *estra* OR andro* OR *estradiol* OR EE2* OR *gestagen* OR progesterone* OR levonorgestrel* OR diethylstilbestrol* OR “aromatase inhibitor*” OR flutamide* OR finasteride* OR *phenol* OR *paraben* OR *cide* OR *azine* OR methoxychlor* OR chlorpyrifos* OR organochlorine* OR *dichlorodiphenyl* OR DDD* OR DDE* OR DDT* OR azocyclotin* OR glyphosate* OR Roundup* OR butachlor* OR *phosphate* OR thiophanate* OR triadimefon* OR *azole* OR vinclozolin* OR thiophanate* OR tamoxifen* OR pyrimethanil* OR tributyltin* OR triphenyltin* OR fludioxonil* OR metaxyl* OR MAXIM OR triclosan* OR fertilizer* OR nitrate* OR ammonium* OR “heavy metal*” OR arsenic* OR chromium* OR cadmium* OR lead* OR surfactant* OR detergent* OR plasticizer* OR “flame retardant*” OR TBBPA* OR TCBPA* OR *phenyl* OR PCB* OR PBDE* OR phthalate* OR styrene* OR *chlor* OR benzene* OR “UV filter*” OR camphor* OR 4-MBC* OR 3-BC* OR trenbolone* OR microcystin* OR MCLR* OR *acid* OR salt*).
A similar strategy of search strategy was used in Google Scholar. The keywords for the search were chosen based on a preliminary literature review and updated each time a new type of EDC or potential EDC was encountered during the search. To select potentially useful studies, the titles and abstracts of 3,450 articles found were first scanned for those remotely relevant to the topic. 757 potentially relevant studies were identified. Next, after reading the full papers, we were left with 241 works, which we included in the review (Figure 1). Studies were chosen if they fulfilled the inclusion criteria: (a) the publication was written in English; (b) the study was experimental and (c) the study provided data on the impact of the exposition of amphibians or amphibian tissue on the chemical compound on sex and/or at least one, defined by us, sex-related trait. The journal or date of publication of the paper was not relevant. Unpublished results from Master’s and PhD Dissertation papers were not included.
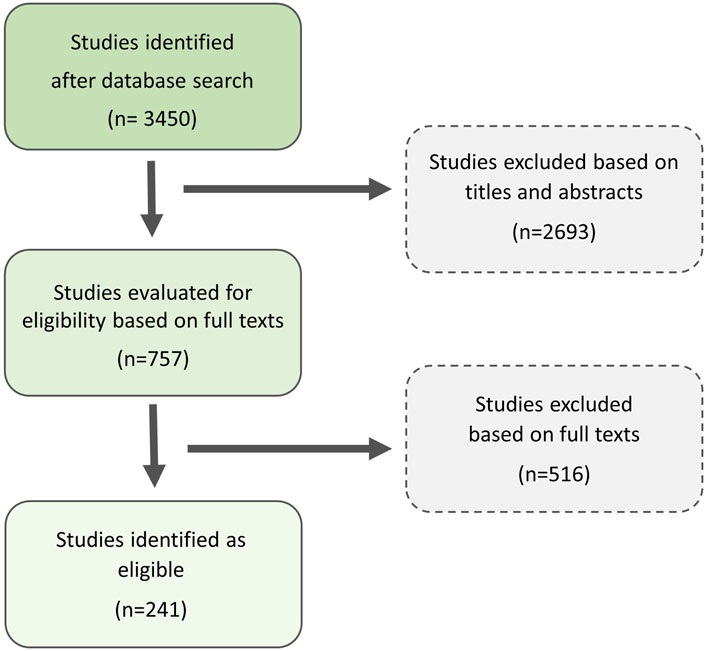
Figure 1. Scheme of literature search approach based on two electronic databases for the search: Web of Science Core Collection® and Google Scholar.
The nomenclature of species mentioned in the article follows Frost (2024), with some modifications. A full list of English common and Latin names of all mentioned species, as well as their taxonomy, can be found in the Supporting Information (Supplementary Table S5). For describing changes within the reproductive system caused by EDCs, we use the nomenclature proposed by Lutz et al. (2008).
3 Results
We identified 241 experimental studies on the impact of EDCs on sex and sex-related traits of amphibians, from which 214 were in vivo studies. The majority of the experimental works were performed on developmental, aquatic forms of amphibians (see Table 1). In the Supplementary Information, detailed information about found experiments with waterborne exposition (90,19% of all in vivo studies) of amphibians to EDCs, including time of exposition, life stages, concentrations of EDCs, and the most significant results can be found (Supplementary Table S1). Among in vivo experiments, we identified only two articles in which the compound was ingested by animals (Tang et al., 2020; Ziková et al., 2013). In some, mostly older studies, amphibians were exposed to EDCs via injections or intraperitoneal implants (9,81% of all in vivo studies), and we consider them to be generally less environmentally relevant. Full lists of studies with these routes of exposition to EDCs (Supplementary Table S2) as well as in vitro and ex vivo studies (Supplementary Table S3), and species used in them, are included in the Supplementary Information (Supplementary Data Sheet S1). Moreover, in the Supplementary Information, we attach a table with abbreviations and IUPAC (International Union of Pure and Applied Chemistry) nomenclature of reviewed EDCs. (Supplementary Table S4). The most significant, recognized effects of EDCs on sex and sex-related traits of amphibians in the researched literature are presented in Figure 2.
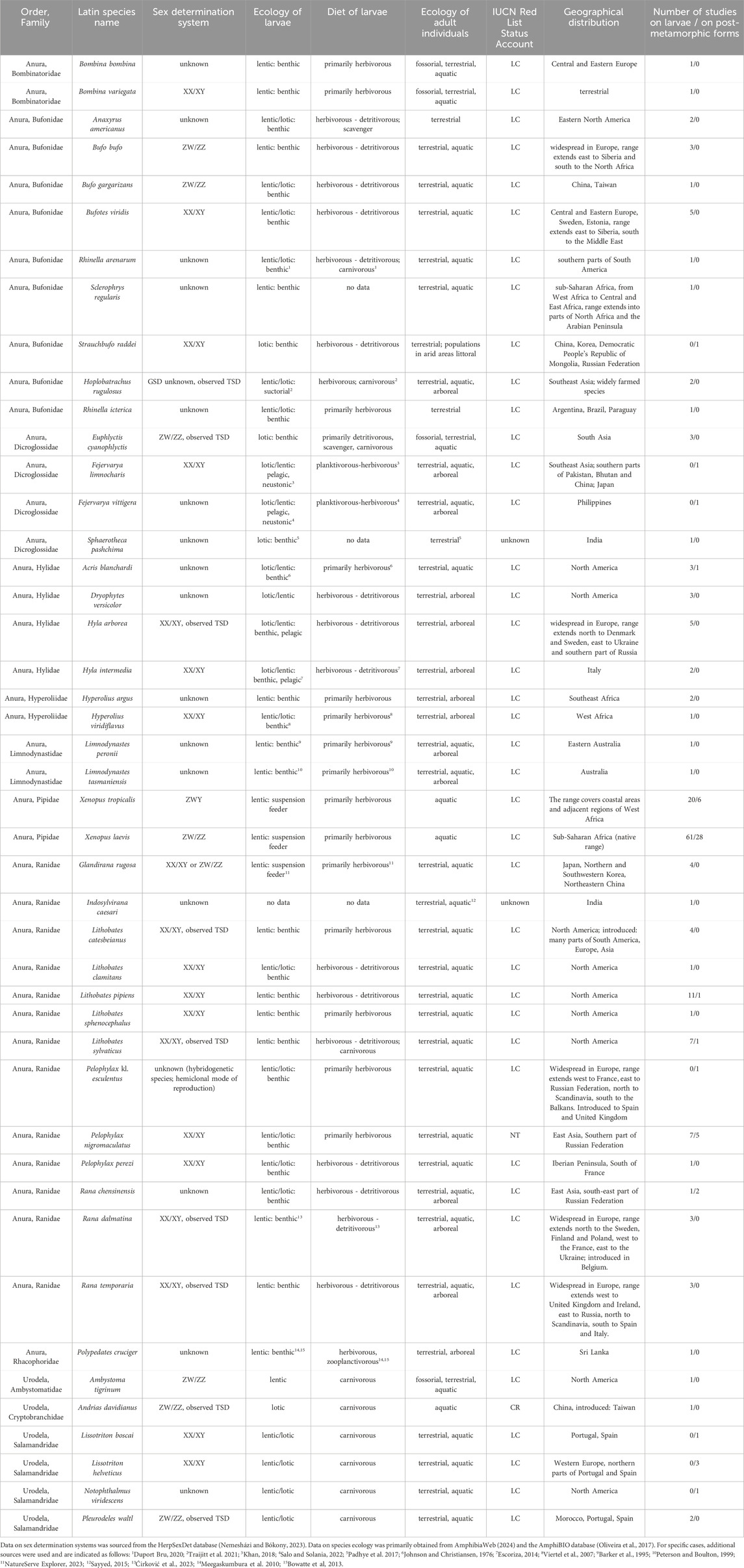
Table 1. Nomenclature, systematic position, sex determination system (GSD – genetic sex determination, TSD – temperature-dependent sex determination) and ecological traits of species of amphibians in experimental studies on the impact of endocrine-disrupting compounds on sex and sex-related traits with external exposition to compound. Please note that some studies used more than one species.
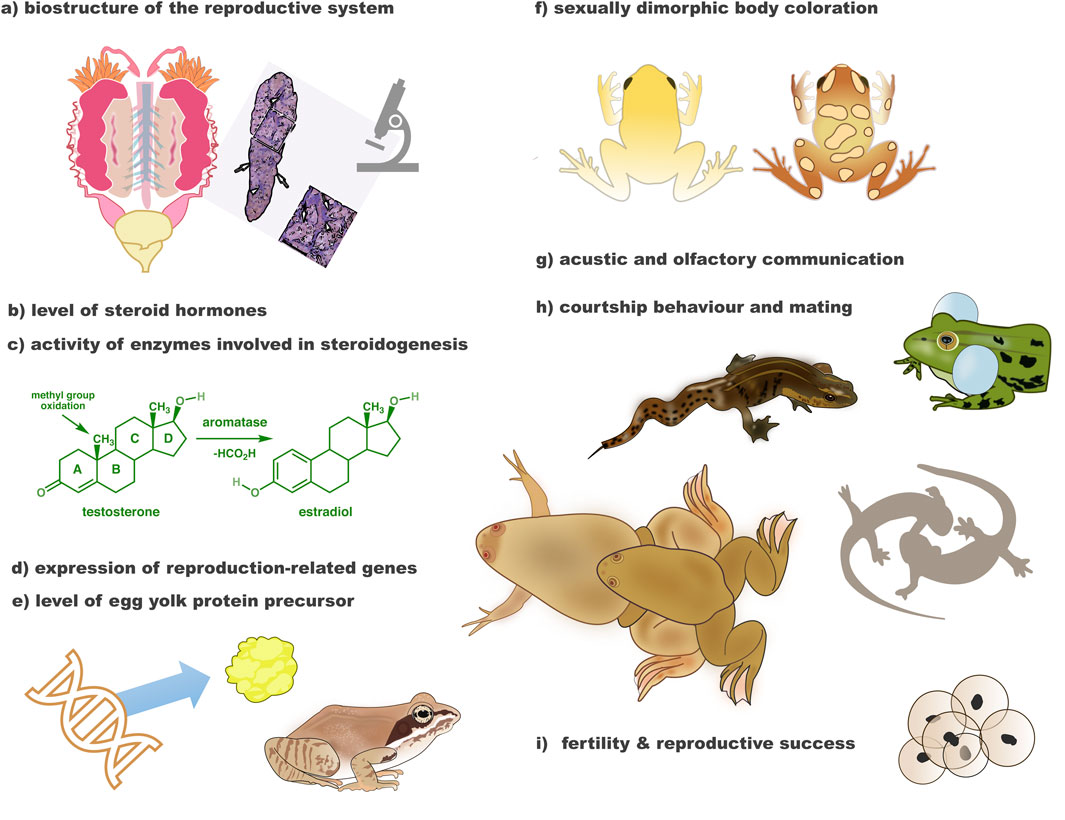
Figure 2. Endocrine-disrupting compounds affect many aspects of amphibian sex and reproduction. Among observed effects of EDCs in in vivo studies on amphibians were adverse impact on (morphology and/or histology) of the reproductive system (a), level of sex steroid hormones in plasma and/or body organs (b), activity of enzymes involved in steroidogenesis in liver and/or gonads (c), expression of sexually-dimorphic and other reproduction-related genes in liver, gonads and/or brain (d), level of egg yolk protein precursor–vitellogenin in liver and/or gonads (e), as well as sexually dimorphic external traits (f), sexual communication (g, h), fertility, and reproductive success (i).
We found that in vivo studies externally exposing amphibians to EDCs included 45 species (Table 1), constituting 0,52% of all species of amphibians known to date (31.12.2024). The two most frequently used species, from the same family Pipidae, account for almost half (49,78%) of all researched species (Figure 3).
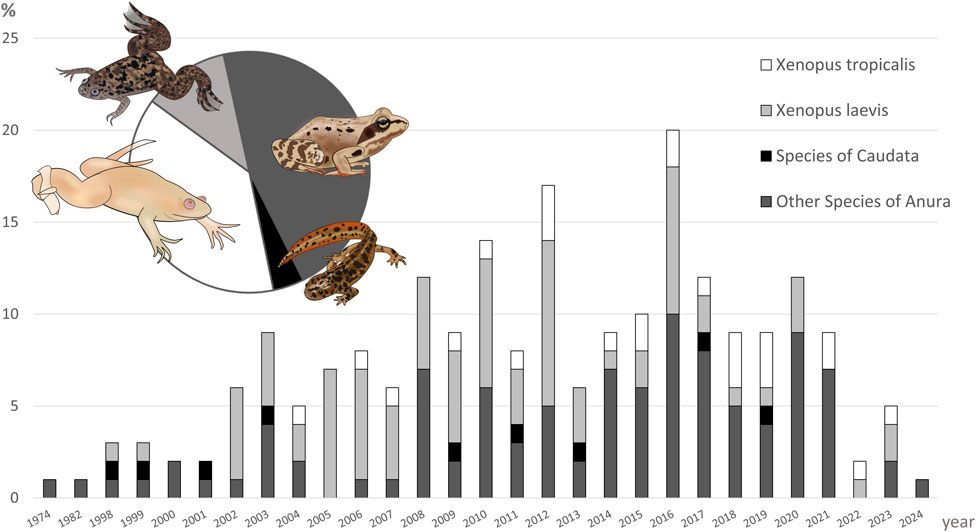
Figure 3. Annual trends in the number of amphibian taxa used in experimental studies on the impact of endocrine-disrupting compounds on sex and sex-related traits with the external exposition to compound. The y-axis represents the percentage of studies per year using particular taxa, while the x-axis the publication year (1974–2024). The pie chart illustrates the overall proportion of each taxonomic group used across all analyzed studies. Please note, that some studies used more than one species of amphibians.
4 Discussion: are model species the best choice?
4.1 The overreliance on Xenopus spp.
The African clawed frog X. laevis is still perceived as an ideal model for studying the effects of endocrine-disrupting compounds. It is due to its quick somatic and sexual development, the well-established knowledge about life stages, and available methods of genetic sex determination (Cong et al., 2006; Huang et al., 2005; Oka et al., 2006; Yoshimoto et al., 2008). It should be noted that Xenopus laevis is an evolutionarily conserved lineage, having diverged nearly 17.5 million years ago (Furman et al., 2015). While, for example, the American toad Anaxyrus americanus has existed for only 1.3 million years (Masta et al., 2002). The evolutionary conservation of X. laevis contributes to its utility as a model for human biology because it implies stability in its genetic and developmental pathways over millions of years. This stability makes it easier to study fundamental biological processes, such as cell division, gene regulation, and embryonic development, that are shared between humans and X. laevis. Its conserved nature ensures that findings in X. laevis are often applicable to humans, providing a reliable system for studying evolutionarily shared mechanisms (Blum and Ott, 2018; Gao and Shen, 2021; Nenni et al., 2019).
However, evolutionary conservation of X. laevis may limit its representativeness for amphibian biology. Its long divergence time means that X. laevis represents a unique and relatively ancient lineage compared to other amphibians, which may not well reflect the evolutionary and ecological traits of most of the contemporary species (Roelants et al., 2007). Another commonly used model closely related to X. laevis is the western clawed frog Xenopus tropicalis, which shares its advantages for toxicological research (Olmstead et al., 2010). Unfortunately, it does not add sufficient variance to account for responses to toxic substances (Cannatella, 2015; Robert and Cohen, 2011). Both species from the family Pipidae belong to the basal anurans (suborder Mesobatrachia), constituting less than 5% of currently known species (together with Archaeobatrachia), while most modern Anura belong to Neobatrachia. Therefore, the diversity of amphibians is still poorly represented in laboratory research (Wake and Koo, 2018).
The most commonly used species in ecotoxicology research are easy to obtain, common, and widely distributed (Schiesari et al., 2007), which is also evident in our study. Species bias in studies on endocrine disruptors may have significant consequences for the conservation of amphibians. The species most threatened by pollution with EDCs may be the ones difficult to obtain, living in areas with little interest and investment in ecotoxicological research (Schiesari et al., 2007). Another issue is the origin of animals used in the studies. Model species are usually obtained from laboratory populations with limited genetic variance and do not undergo natural selection like wild populations. They can also be a subject of unintentional selection for traits supporting survival in captivity conditions, of which researchers using such “domesticated” lines are not always aware. It has been shown that wild populations may develop tolerance to pollutants in the environment. Consequently, they can be less sensitive to the harmful effects of some toxicants than animals of the same species bred for generations in controlled, laboratory conditions (Romero-Blanco and Alonso, 2022).
4.2 Amphibians differ in sensitivity to EDCs
Results obtained from studies on non-model species often contradict those from studies on model ones. For example, significant differences in sensitivity of species to the effects of exogenous 17β-estradiol (E2) were found. E2 is widely present in surface waters, as it is excreted into the environment by humans and farm animals. In water-borne exposition studies, E2 was found to have a feminizing impact on morphology and histology of gonads in X. laevis (Hu et al., 2008; Lutz et al., 2008; Oka et al., 2006), the Indian skipper frog Euphlyctis cyanophlyctis (Phuge and Gramapurohit, 2015) and the Japanese wrinkled frog G. rugosa males (Ohtani et al., 2000; Oike et al., 2020). Moreover, exposition through the larval period to different concentrations of E2 caused female-biased to 100% female sex ratio in X. laevis (Bögi et al., 2002; Hu et al., 2008; Lutz et al., 2008; Oka et al., 2006), E. cyanophlyctis (Phuge and Gramapurohit, 2015), the common toad Bufo bufo (Petrini and Zaccanti, 1998), and Glandirana rugosa males (Ohtani et al., 2000; Oike et al., 2020), which suggested cases of sex reversal.
However, in the green frog Lithobates clamitans, injections with E2 for a long period did not significantly affect the sexual development of male and female tadpoles (Mintz et al., 1945). Also in the common reed frog Hyperolius viridiflavus exposition to E2 did not have any impact on gonadal morphology, even when a dose of E2 was very high (Richards, 1982), and in an outdoor study of the Blanchard’s cricket frog Acris blanchardi E2 was found to have minimal effect on sexual development (Windle et al., 2021). Another study comparing the impact of E2 on the X. laevis, the European fire-bellied toad Bombina bombina, the yellow-bellied toad Bombina variegata, the European tree frog Hyla arborea, and the European green toad Bufotes viridis, found significant differences in the extent of induced changes within gonads of these species (Piprek et al., 2012).
More examples of differences in sensitivity to EDCs can be found in studies using a bacteriostatic compound triclosan (TSC), widely present in the environment as a residue from, e.g., personal care products. In the dark-spotted frog Pelophylax nigromaculatus, exposure to TSC during larval development caused a male-biased sex ratio, abnormalities within the gonads, and changed expression of reproduction-related genes (Chen et al., 2018). In comparison, TCS did not significantly impact sexual development and gonadal differentiation in X. laevis (Fort et al., 2017) or the expression of reproduction-related genes in adult X. tropicalis (Regnault et al., 2016).
Significant differences in sensitivity between species were also noted in other studies, exposing amphibians to a synthetic estrogen ethinyloestradiol (Tamschick et al., 2016b; Tamschick et al., 2016c), plasticizer bisphenol A (Tamschick et al., 2016a), nonylphenol, compound widely used in surfactants and detergents (Mackenzie et al., 2003) and a fungicide agent ethylenethiourea (Phuge et al., 2021).
Differences in sensitivity to EDCs between species could be largely due to various sex determination and differentiation mechanisms, as well as physiological and ecological differences, which we discuss below.
4.3 Differences in sex determination and differentiation in amphibians
The sexual determination system for most species of amphibians is still unknown. The limited data we have, however, show a huge variety of sex determination systems in this group of vertebrates (Nakamura, 2009). In this context, it becomes obvious that studying the effects of EDCs on only model species (such as X. laevis and X. tropicalis) is not enough to identify the true impact of these compounds on a huge variety of modern amphibian forms.
4.3.1 Genetic sex determination
Although it is generally accepted that the sex of amphibians is determined genetically, for most amphibian species, morphologically distinguishable sex chromosomes are not identified. Until now, the sex chromosome system has been recognized only for about 2% of the species (Bullejos et al., 2024). Data on sex-determining genes and gene regulatory networks involved in sexual differentiation are also limited (Nakamura, 2009; Ruiz-Garciá et al., 2021; Kuhl et al., 2024).
No universal sex-specific loci in amphibians were found, and at this moment, genetic determination of sex is possible only for a few species (Olmstead et al., 2010; Yoshimoto et al., 2008). It is particularly striking when considering the diversity of this taxonomic group. To date (31.12.2024), 8,816 amphibian species have been identified, and new species are discovered every year AmphibiaWeb (2024).
However, even this limited knowledge about genetic sex determination systems in amphibians shows a huge variability and plasticity within the group (Dufresnes and Crochet, 2022; Ma and Veltsos, 2021). The male (XX/XY) or female (ZZ/ZW) heterogametes in amphibian species can be found, as well as novel systems of chromosomal sex determination. Examples are the 00/W0 sex chromosome system in the Hochstetter’s frog Leiopelma hochstetteri (Green, 1988), or the multiple sex chromosomes (=XXAA∼/XXAY ∼ type) system in the Puerto Cabello robber frog Strabomantis biporcatus (Schmid et al., 1992). Notably, in some phylogenetic lines of amphibians, the events of switching chromosomal sex determination systems occurred (Evans et al., 2012).
Variations of sex chromosomes have been identified even within a single species. Xenopus tropicalis is an interesting example, as in this species, three homomorphic sex chromosomes (Y > W > Z) were identified. As a result, males of X. tropicalis can possess ZZ, YZ, or YW chromosome combinations, while in females, ZW or WW chromosome sets can be found. Additionally, both sexes can be either heterogametic or homogametic. It should be taken into account in the studies on EDCs, as depending on the chromosome sets and types of gametes of parents, the sex ratio obtained in the offspring may differ from the conventionally assumed 1:1 (Furman et al., 2020; Roco et al., 2015). Another, somewhat extreme example, is the Japanese wrinkled frog G. rugosa the 6 geographic variants, with both XX/XY and ZZ/ZW sex chromosome systems, were found (Miura, 2007; Ogata et al., 2003; Oike et al., 2017; Mawaribuchi et al., 2023).
There are pieces of evidence that the sex determination system may impact the sensitivity of amphibians to hormonal compounds. Glandirana rugosa frogs from populations with novel chromosome sex determination systems were found to have lower sensitivity to hormonally induced gonadal sex reversal and the associated gene expression pattern (Miura et al., 2016). In theory, they could be less prone to the impact of EDCs. Thus, switching sex determination systems can have serious consequences for the survival of amphibian populations. According to the theory of “asymmetrical sex reversal”, homogametic sexes are more prone to phenotypical sex reversal and require weaker stimuli to undergo it (Nemesházi and Bókony, 2022).
4.3.2 Impact of steroidal sex hormones
Steroidal hormones play a crucial role in sex determination in amphibians (Hayes, 1998; Nakamura, 2013; Zaborski, 1986). They are also involved in the development and maintenance of sex accessory structures and secondary sexual characteristics in these animals (Harvey and Propper, 1997; Iwasawa and Kobayashi, 1974; Norris, 2011a). EDCs mimic steroidal hormone effects in sex determination, making amphibians extremely sensitive to these compounds. However, the role of steroidal hormones in sex determination and differentiation varies among species (Mali and Gramapurohit, 2016), which can be another reason for a species-specific sensitivity to the endocrine-disrupting compounds in the environment.
4.3.3 Impact of temperature on the sex of amphibians
The available evidence suggests that no amphibian species has exclusively temperature-dependent sex determination, although some of them retain sensitivity to it (Hayes, 1998; Nakamura, 2009; Ruiz-Garciá et al., 2021). Examples are some Urodela species: the Edough ribbed newt Pleurodeles poireti (Dournon et al., 1984), the iberian ribbed newt Pleurodeles waltl (Dournon et al., 1990; Zaborski, 1986), as well as Anura: the wood frog Lithobates sylvaticus (Lambert et al., 2018), the Dybowski’s frog Rana dybowskii (Xu et al., 2022), the agile frog Rana dalmatina and the chinese edible frog Hoplobatrachus rugulosus (Mikó et al., 2021; Tang et al., 2020). For such species, outcomes of exposure to EDCs may differ depending on the interacting temperature. In the environment, amphibians are simultaneously subjected to many factors that can shape their phenotypical sex. In this context, the potential role of temperature in sex determination should not be overlooked.
4.3.4 Type of gonadal differentiation
In addition to a huge variety of sex determination systems, amphibian species differ in patterns of gonadal differentiation and rate of sexual development. It may significantly impact their sensitivity to endocrine disruptors, the time frame in which EDCs can affect their phenotypical sex, and the outcome of exposure to EDCs (e.g., feminization, masculinization, or none).
In amphibians can be found bipotential gonads. The differentiation of them may be categorized into three types. In most species, bipotential gonads develop directly into ovaries or testes (differentiated type). In some anuran species, however, the timing of differentiation of gonads in males and females is different, with testes developing from bipotential gonads much later than ovaries (semi-differentiated type). In this type of differentiation, some authors mistakenly recognize bipotential gonads in males as ovaries (explained by Ogielska, 2009). In rare cases, another type of gonadal differentiation can be found, in which in genetic males and females bipotential gonads first develop into ovaries, and then, in males, transform into testis (undifferentiated type) (Gramapurohit et al., 2000; Ogielska, 2009; Tanimura and Iwasawa, 1989).
Importantly, even populations of the same species may differ in the timing/type of gonadal differentiation (differentiated vs. semi-differentiated type) (Rodrigues et al., 2015). Studies on Urodela suggest that they undergo direct differentiation from bipotential gonad into ovary or testis (Dumond et al., 2008), although knowledge on gonadal differentiation in this group of amphibians is scarce.
4.3.5 Rate of gonadal differentiation
Amphibians also differ in the timing and rate of gonadal development and differentiation (for the review see Ogielska and Kotusz, 2004), with some having extremely slow gonadal differentiation in comparison to others (Piazza et al., 2023). The effects of EDCs on sex and sex-related traits may strongly depend on the type and timing of gonadal differentiation in species. Xenopus laevis, with direct and synchronized differentiation of bipotential gonad into ovaries or testis, may not be universally representative of the whole group of amphibians.
Data from experimental studies show that species with short ovarian development time, e.g., the Southern leopard frog Lithobates sphenocephalus (Storrs and Semlitsch, 2008), are more susceptible to the impact of EDCs than others. Species with quick sexual differentiation during the aquatic phase of life can be more prone to adverse effects of EDCs in surrounding water than species with slow sexual differentiation, extended to the post-metamorphic, terrestrial life stages. Worth noting, temperature can also have a significant impact on the rate of gonadal differentiation (Burraco et al., 2023).
4.3.6 Mixed-sex amphibians: norm in some species?
In many studies in which amphibians were exposed to EDCs, the presence of mixed-sex gonads (which contain both ovarian and testicular tissue) (Howe et al., 2004), intersex gonads (both testes and ovaries as separate structures in one individual) (Hayes et al., 2002), testicular oocytes (oocytes within testicular tissue) (Jeganathan and Rajakaruna, 2021) or completely sex reversed individuals (e.g., genetic males with regular, female gonads) was observed (Oka et al., 2006). However, there is a question: To what extent are these gonadal changes a natural phenomenon?
For example, mixed-sex individuals and testicular oocytes were observed in both experimental and control groups in several studies regarding impact of pesticide atrazine (Brande-Lavridsen et al., 2008; Coady et al., 2004; Jooste et al., 2005; Orton et al., 2006; Preez et al., 2008; Storrs-Méndez and Semlitsch, 2010) or EE2 (Brande-Lavridsen et al., 2008). Existences of testicular oocytes, mixed-sex or sex-reversed individuals in amphibian populations come also from field studies (Alho et al., 2010; Bókony et al., 2021; Lambert et al., 2019; Nemesházi et al., 2020; Preez et al., 2009; Reeder et al., 1998; Xu et al., 2022). Especially testicular oocytes, in many studies regarded as a pathology, may, in fact, be a natural phenomenon in some species or populations (Preez et al., 2009; Storrs-Méndez and Semlitsch, 2010). In some cases, observations of abnormal gonads in control groups could be, however, due to mistakes in experimental design (Hayes, 2005).
Some studies suggest that mixed-sex or intersex stages of gonads may be expected for some species (with semi-differentiated or undifferentiated type of gonadal development). Especially for toads (Bufonidae), not all cases of mixed-sex or intersex individuals should be perceived as anomalies. In Bufonidae rudimental hermaphroditism can be found. Adult males have Bidder’s organs–undeveloped ovaries - located anterior to the testes, in which oogenesis is still active, but oocytes do not reach maturity or are reduced. The exact function of the Bidder’s organ is unknown (Petrini and Zaccanti, 1998). Additionally, differentiation of the bipotential gonads into testes in toads is delayed (retarded type of differentiation) (Storrs-Méndez and Semlitsch, 2010). As we underlined in the above section, Differences in sex determination and differentiation in amphibians, to accurately identify mixed-sex and intersex individuals, comprehensive knowledge of gonadal development, timing, and subsequent stages in a particular species is crucial.
4.4 Ecological differences
4.4.1 Aquatic vs. biphasic species
Apart from genetic differences between taxa, species also differ in ecology (Table 1), which can impact the main routes of exposure to EDCs in the environment. Most of our knowledge about the impact of EDCs on amphibians comes from studies with aqueous exposure (Slaby et al., 2019). The widely used standardized amphibian toxicity test, FETAX, using X. laevis, is based on exposure via water (Testing and Materials, 1998). However, the water environment may not be the primary route of exposure to pollutants for most species of amphibians (Brühl et al., 2013; Purucker et al., 2023). Xenopus spp., as fully aquatic species, do not represent the majority of amphibians with biphasic life cycles, which transition between aquatic and terrestrial habitats.
This transition exposes animals to additional stressors, such as pesticide contamination on land, different temperatures, and greater exposure to UV light (Nolan et al., 2023). Moreover, biphasic amphibians by bioaccumulation during the aquatic stage can transfer pollutants such as microplastics from the aquatic environment to the terrestrial one, thus contributing to the dissemination of them in the ecosystem (Park and Kidd, 2005; Szkudlarek et al., 2023). While the Xenopus spp. remain a convenient laboratory organism, its life history fails to capture the complexity of biphasic species’ exposure to environmental stressors and processes occurring during the transition from the aquatic stage to the terrestrial one. To obtain a more accurate understanding of the impact of EDCs on amphibians, ecotoxicology studies must incorporate biphasic species (Nolan et al., 2023).
4.4.2 Terrestrial exposure
Terrestrial stage of life may be particularly problematic for amphibians living in agricultural areas, where they are exposed to significant quantities of endocrine-disrupting pesticides and fertilizers via contact with polluted soil and overspraying (Brühl et al., 2011; Churko et al., 2024; Cruz-Santiago et al., 2023; Fryday and Thompson, 2017). Pesticides, such as atrazine, may be absorbed by the highly permeable skin of amphibians, especially on the ventral side, and accumulate in the body (Purucker et al., 2023; Quaranta et al., 2009). This has been confirmed in the studies on, e.g., Anaxyrus americanus (Méndez et al., 2009), the juvenile barking tree frog Dryophytes gratiosus, and the southern leopard frog Lithobates sphenocephala (Belden et al., 2010).
However, species differ in their risk of exposure due to behavioral and ecological traits (Lenhardt et al., 2015). Nocturnal or fossorial species that remain underground during the day may avoid direct contact with contaminated surfaces, unlike diurnal, surface-active species (Adams et al., 2021; Belden et al., 2010).
Similarly, habitat use—particularly the proportion of time spent in terrestrial versus aquatic environments—modulates the likelihood of EDC exposure (Swanson et al., 2018). In this context, an interesting question is how the risk of exposure to EDCs differs between species with various overwintering strategies of adult forms. For example, species wintering in polluted water bodies in bottom sediment, where EDCs are accumulated (Fuentes et al., 2014; Swanson et al., 2018), are potentially more prone to exposure to EDCs than the species wintering on land.
4.4.3 Skin structure and physiology
Species ecology is closely tied to interspecific differences in skin structure and physiology, which influence both exposure to, and sensitivity to EDCs. Amphibian skin is not only the principal organ for respiration and osmoregulation but also the main route of chemical uptake from the environment (Quaranta et al., 2009). It varies in permeability, keratinization, glandular structure, and lipid content depending on species, developmental stage, and habitat (Hillyard et al., 2008). For example, toads living in arid regions have thicker, more keratinized skin to reduce water loss (Akat et al., 2022), which may also limit absorption of waterborne or soil-based pollutants.
In aquatic larvae, the skin is typically thin and highly vascularized, optimized for cutaneous respiration but also highly vulnerable to aqueous contaminants (Duellman and Trueb, 1994; Fox, 1977). As in some species larvae metamorphose into terrestrial juveniles or adults, keratinization increases to prevent desiccation—yet dermal absorption, especially of lipophilic compounds, remains a key exposure route (Akat et al., 2022). These physiological traits, coupled with their dual aquatic–terrestrial lifestyle, make amphibians sensitive bioindicators of environmental contamination (Kloas et al., 2006), but also demand a broader, more species-diverse approach in toxicological research.
Additionally, it is important to note that some EDCs are airborne (Annamalai and Namasivayam, 2015) and may reach amphibians through less-studied routes such as inhalation or direct dermal absorption from the atmosphere. This pathway remains largely unexplored in amphibians and its potential significance should not be overlooked. To our knowledge, the inhalation or dermal absorption of airborne EDCs and their effects have not yet been studied in amphibians, representing a critical gap in ecotoxicological research.
4.4.4 Feeding strategies
Additionally, it is worth considering the ecological and physiological differences in relation to feeding strategies. Notably, Xenopus spp. tadpoles are highly specialized filter feeders, primarily consuming suspended organic particles and zooplankton from the water column (Wassersug, 1996). In contrast, the majority of anuran tadpoles feed by grazing on biofilms and scraping detritus or algae from submerged surfaces using keratinized mouthparts (McDiarmid and Altig, 2000). The key differences in larval feeding strategies are summarized in Table 1.
These differences in feeding modes can substantially affect the route and intensity of EDCs exposure, as shown in fish (Fan et al., 2019; Müller et al., 2020). Filter-feeding larvae may be particularly vulnerable to dissolved or particle-bound EDCs suspended in the water, while surface-grazing tadpoles may ingest pollutants accumulated in periphyton or sediment layers. To our best knowledge, this hypothesis has not been tested yet.
Similar considerations apply to adult amphibians. Species feeding on aquatic invertebrates may remain more vulnerable to waterborne or sediment-associated pollutants (Veseli et al., 2022) whereas terrestrial-feeding species may primarily encounter EDCs present in, e.g., soil-dwelling invertebrates (Kwak and An, 2021). Moreover, trophic level may influence susceptibility: animals feeding on vertebrates or high trophic-level invertebrates may experience biomagnification of certain lipophilic EDCs (Mizukawa et al., 2009; Previšić et al., 2021; Ruhí et al., 2016).
Altogether, both the habitat and dietary preferences of amphibians—during larval and adult stages—contribute to interspecific variation in EDCs exposure and likely influence also physiological susceptibility to chemicals. These ecological and behavioral factors should be taken into account when assessing the environmental risks posed by EDCs across different species.
4.5 Species with unique life history traits
Among anuran amphibians, unique species with direct development, without an intermediate, morphologically distinct, free-living larval phase can be found (Callery et al., 2001). These direct developers, e.g., the Puerto Rican tree frog, Eleutherodactylus coqui, still undergo a thyroid hormone-dependent, rapid metamorphosis before hatching. It is interesting how EDCs present in the environment can influence the somatic and sexual development of such species. Are they less or more prone to the effects of endocrine disruptors?
The risk and consequences of exposure to EDCs are also unknown for Caecilians. Only about 5% of all described species of Caecilians have been studied in the topic of reproductive biology (Gomes et al., 2012). Very little is known about sex determination and gonadal development (Serrano-Perez and Ramírez-Pinilla, 2021), and almost nothing about the hormonal system in Caecilians (Brun et al., 2023; Norris, 2011b). Based on the study of two species, the Tenmalai caecilian Gegeneophis ramaswamii and Gegeneophis sp. (unspecified species), it is stated that Caecilians represent male heterogamety (XX/XY) (Govindappa and Venkatachalaiah, 2005). Because of the fossoriality of these animals, it is hard to observe them in nature and study and identify the environmental risks they are prone to.
Interestingly, some species of Caecilians and Urodela are ovoviviparous, or viviparous, with “pregnancy” controlled by hormones (Exbrayat, 1992). We already know that for many hydrophobic EDCs, the primary exposure route may be via maternal transfer (Kadokami et al., 2004; Liu et al., 2020). Field studies suggest that some EDCs can be transferred to eggs in oviparous amphibians (Du et al., 2019; Guan et al., 2024; Hopkins et al., 2006; Kadokami et al., 2004; Wu et al., 2009). However, to the best of our knowledge, there is currently no data on the impact of EDC on embryos in ovoviviparous and viviparous amphibians. Some clues may come from the study on reptiles (Squamata), where gravid females of northern watersnakes Nerodia sipedon, exposure to atrazine by ingestion altered the sex ratio of offspring, skewing it toward males (Neuman-Lee et al., 2014).
Worth noting, in all known oviparous species of Caecilians, as well as some species of Urodela, such as Pseudoeurycea juarezi, and Anura, such as the Rosenberg’s treefrog Boana rosenbergi, parental care of offspring can be found (Gomes et al., 2012). It can be suspected that all these processes are, to some extent, controlled by reproductive hormones and possibly can be disrupted by external disruptors.
4.6 Differences in study design
The ecological characteristics of species significantly shape the optimal design of experimental setups to deliver truly environmentally relevant data. In in vivo waterborne exposure experiments, methodologies vary, including the flow-through water system, which maintains relatively stable concentrations of contaminants and effectively removes their metabolites or degradation products (Pickford et al., 2003). In contrast, the static renewal system allows for the accumulation of these substances within the water tank, potentially influencing experimental outcomes, especially if these by-products possess endocrine-disrupting properties (Hayes et al., 2003). Flow-through systems may more accurately mimic the environmental conditions of species that inhabit flowing waters. However, this setup might not be entirely suitable for species residing in stagnant water bodies, for example, ephemeral ponds, like puddles or phytotelmata. Moreover, natural aquatic environments often contain sediments and organic matter that can bind significant amounts of EDCs and their metabolites, thereby reducing their concentration in the water, which suggests that the real exposure levels to EDCs for amphibians in wild conditions could differ from those in controlled settings (Fuentes et al., 2014). Worth noting, the impact of the presence of sediments in water tanks may vary between amphibian species with benthic or pelagic specialized larvae (Table 1).
For investigating the complicated interactions between contaminants, their metabolic and degradation by-products, and various environmental factors, outdoor mesocosms offer a viable solution (Windle et al., 2021). Despite the challenges associated with their use in ecotoxicological studies, mesocosms provide conditions that closely resemble those in natural static water bodies. They incorporate multiple ecosystem components and account for additional stressors such as temperature (Tang et al., 2020), UV light (Croteau et al., 2009), pathogens (Paetow et al., 2023; Salla et al., 2024b) or predators (LaFiandra et al., 2008). Even more realistic conditions than mesocosms offer experimental lakes (Park and Kidd, 2005), which are, though, not a widely available solution.
5 Summary
In conclusion, amphibians are notably vulnerable to endocrine-disrupting compounds (EDCs), which pose a significant threat to their survival and highlight their utility as sensitive bioindicators of environmental pollution. Almost half of the EDCs studies focus on just two amphibian model species, failing to adequately represent the group’s diversity. This is particularly true for Caecilians and Urodela, where data on EDC impacts are scarce.
As shown in the previous study of Schiesari et al. (2007), there is a significant biogeographic bias in the general field of ecotoxicology of amphibians. The vast majority of research in this field uses few, common species with the least-concern threat status, primarily found in temperate zones of the northern hemisphere (Table 1). This bias may be due to the concentration of ecotoxicological researchers in these regions and the reliance on traditional, generic ecological risk-assessment methodologies. It should be noted, however, that our review took into account only publications written in English, which could potentially create a research bias (Chowdhury et al., 2022; Konno et al., 2020).
Nevertheless, our review underlines the need to recognize the natural diversity within amphibians in ecotoxicological studies. Alternative model species, representative of diverse taxonomic groups, should be considered (Salla et al., 2024b; Wagner and Viertel, 2017). Whenever possible, the effects of EDCs should be examined on regionally occurring species, under conditions that closely mimic their natural habitats. In studies on native species, non-invasive methods of sex identification and assessing endocrine disruptions are particularly useful (Orton et al., 2023) but still require development. Studies using non-model species in complex experimental setups may be challenging. However, intentional variations in ecotoxicological experiments may help gain a realistic view of the EDC’s impact on amphibians in nature (Mikó et al., 2017; Romero-Blanco and Alonso, 2022) and contribute to their protection.
Author contributions
MF: Conceptualization, Investigation, Visualization, Writing – original draft, Writing – review and editing. MK: Conceptualization, Writing – review and editing. KS: Supervision, Writing – review and editing. PT: Conceptualization, Methodology, Supervision, Writing – review and editing.
Funding
The author(s) declare that financial support was received for the research and/or publication of this article. This work was supported by the National Science Centre research grant no UMO 2023/49/N/NZ8/01103. The publication was partly financed by the Polish Minister of Science and Higher Education as part of the Strategy of the Poznan University of Life Sciences for 2024-2026 in the field of improving scientific research and development work in priority research areas.
Acknowledgments
We would like to thank Mikołaj Liebelt, MSE for consultation on the nomenclature and chemical properties of endocrine-disrupting compounds.
Conflict of interest
The authors declare that the research was conducted in the absence of any commercial or financial relationships that could be construed as a potential conflict of interest.
Generative AI statement
The author(s) declare that Generative AI was used in the creation of this manuscript. Language editing assistance was provided using OpenAI’s ChatGPT-4. No AI-generated content or ideas were included in the manuscript.
Publisher’s note
All claims expressed in this article are solely those of the authors and do not necessarily represent those of their affiliated organizations, or those of the publisher, the editors and the reviewers. Any product that may be evaluated in this article, or claim that may be made by its manufacturer, is not guaranteed or endorsed by the publisher.
Supplementary material
The Supplementary Material for this article can be found online at: https://www.frontiersin.org/articles/10.3389/fenvs.2025.1556788/full#supplementary-material
SUPPLEMENTARY TABLE S1 | Summary of the in vivo studies on the impact of endocrine-disrupting compounds on sex and sex-related traits in amphibians with waterborne exposition, including the type of compound, species studied, developmental stage, time of exposition and the most significant results.
SUPPLEMENTARY TABLE S2 | Summary of the in vivo studies on the impact of endocrine-disrupting compounds on sex and sex-related traits of amphibians with alternative routes of exposition.
SUPPLEMENTARY TABLE S3 | Summary of the in vitro and ex vivo studies on the impact of endocrine-disrupting compounds on sex and sex-related traits of amphibians.
SUPPLEMENTARY TABLE S4 | Nomenclature of all chemical compounds and commercial products mentioned in the manuscript and Supplementary Information.
SUPPLEMENTARY TABLE S5 | Nomenclature and taxonomy of all species of amphibians mentioned in the manuscript and in the Supplementary Information.
SUPPLEMENTARY DATA SHEET S1 | List of references cited only in the Supplementary Materials.
References
Adams, E., Leeb, C., and Brühl, C. A. (2021). Pesticide exposure affects reproductive capacity of common toads (Bufo bufo) in a viticultural landscape. Ecotoxicology 30 (2), 213–223. doi:10.1007/S10646-020-02335-9
Akat, E., Yenmiş, M., Pombal, M. A., Molist, P., Megías, M., Arman, S., et al. (2022). Comparison of vertebrate skin structure at class level: a review. Anatomical Rec. 305 (12), 3543–3608. doi:10.1002/ar.24908
Alho, J. S., Matsuba, C., and Merilä, J. (2010). Sex reversal and primary sex ratios in the common frog (Rana temporaria). Mol. Ecol. 19 (9), 1763–1773. doi:10.1111/J.1365-294X.2010.04607.X
Ankley, G. T., Coady, K. K., Gross, M., Holbech, H., Levine, S. L., Maack, G., et al. (2018). A critical review of the environmental occurrence and potential effects in aquatic vertebrates of the potent androgen receptor agonist 17β-trenbolone. Environ. Toxicol. Chem. 37 (8), 2064–2078. doi:10.1002/ETC.4163
Annamalai, J., and Namasivayam, V. (2015). Endocrine disrupting chemicals in the atmosphere: their effects on humans and wildlife. Environ. Int. 76, 78–97. doi:10.1016/J.ENVINT.2014.12.006
AmphibiaWeb, (2024). AmphibiaWeb. Available online at: https://amphibiaweb.org/(Accessed: December 31, 2024).
Belden, J., McMurry, S., Smith, L., and Reilley, P. (2010). Acute toxicity of fungicide formulations to amphibians at environmentally relevant concentrations. Environ. Toxicol. Chem. 29 (11), 2477–2480. doi:10.1002/ETC.297
Blum, M., and Ott, T. (2018). Xenopus: an undervalued model organism to study and model human genetic disease. Cells Tissues Organs 205 (5–6), 303–313. doi:10.1159/000490898
Bögi, C., Levy, G., Lutz, I., and Kloas, W. (2002). Functional genomics and sexual differentiation in amphibians. Comp. Biochem. Physiology Part B 133 (4), 559–570. doi:10.1016/s1096-4959(02)00162-8
Bókony, V., Ujhegyi, N., Mikó, Z., Erös, R., Hettyey, A., Vili, N., et al. (2021). Sex reversal and performance in fitness-related traits during early life in agile frogs. Front. Ecol. Evol. 9, 745752. doi:10.3389/fevo.2021.745752
Bowatte, G., Perera, P., Senevirathne, G., Meegaskumbura, S., and Meegaskumbura, M. (2013). Tadpoles as dengue mosquito (Aedes aegypti) egg predators. Biol. Control 67 (3), 469–474. doi:10.1016/j.biocontrol.2013.10.005
Brande-Lavridsen, N., Christensen-Dalsgaard, J., and Korsgaard, B. (2008). Effects of prochloraz and ethinylestradiol on sexual development in Rana temporaria. J. Exp. Zoology Part A Ecol. Genet. Physiology 309A (7), 389–398. doi:10.1002/JEZ.462
Brühl, C. A., Pieper, S., and Weber, B. (2011). Amphibians at risk? Susceptibility of terrestrial amphibian life stages to pesticides. Environ. Toxicol. Chem. 30 (11), 2465–2472. doi:10.1002/ETC.650
Brühl, C. A., Schmidt, T., Pieper, S., and Alscher, A. (2013). Terrestrial pesticide exposure of amphibians: an underestimated cause of global decline? Sci. Rep. 3 (1), 1135. doi:10.1038/srep01135
Brun, C., Measey, J., Exbrayat, J. M., and Raquet, M. (2023). Detection of the hormone receptors controlling the female genital tract throughout the sexual cycle of an oviparous caecilian Amphibian, boulengerula taitana. Asian J. Res. Animal Veterinary Sci. 6 (4), 425–440. doi:10.9734/ajravs/2023/v6i4271
Bullejos, M., Ruiz-García, A., and Roco, Á. S. (2024). “Sex determination and gonadal differentiation in amphibians”. in Hormones and Reproduction of Vertebrates 2, 1–31. doi:10.1016/B978-0-443-16020-2.00012-7
Burraco, P., Torres-Montoro, J. C., and Gomez-Mestre, I. (2023). Larval plastic responses to warming and desiccation delay gonadal maturation in postmetamorphic spadefoot toads. Evolution 77 (12), 2687–2695. doi:10.1093/evolut/qpad180
Caliman, F. A., and Gavrilescu, M. (2009). Pharmaceuticals, personal care products and endocrine disrupting agents in the environment—a review. Clean. - Soil, Air, Water 37 (4–5), 277–303. doi:10.1002/clen.200900038
Callery, E. M., Fang, H., and Elinson, R. P. (2001). Frogs without polliwogs: evolution of anuran direct development. BioEssays 23 (3), 233–241. doi:10.1002/1521-1878(200103)23:3<233::AID-BIES1033>3.0.CO;2-Q
Cannatella, D. (2015). Xenopus in space and time: fossils, node calibrations, tip-dating, and paleobiogeography. Cytogenet. Genome Res. 145 (3–4), 283–301. doi:10.1159/000438910
Chen, J., Meng, T., Li, Y., Gao, K., and Qin, Z. (2018). Effects of triclosan on gonadal differentiation and development in the frog Pelophylax nigromaculatus. J. Environ. Sci. 64, 157–165. doi:10.1016/j.jes.2017.05.040
Chowdhury, S., Gonzalez, K., Aytekin, M. Ç. K., Baek, S.-Y., Bełcik, M., Bertolino, S., et al. (2022). Growth of non-English-language literature on biodiversity conservation. Conservation Biol. J. Soc. Conservation Biol. 36 (4), e13883. doi:10.1111/cobi.13883
Churko, G., Haibach, G., Bär, M., Szerencsits, E., and Schmidt, B. R. (2024). Use of agricultural fields by Natterjack toads: implications for pesticide exposure. Agric. Ecosyst. and Environ. 369, 109049. doi:10.1016/j.agee.2024.109049
Ćirković, G., Mitrović, A., Ajtic, R., Halami, P. M., and G, S. K. (2023). In vitro fermentation of glycosaminoglycans from mackerel fish waste and its role in modulating the antioxidant status and gut microbiota of high fat diet-fed C57BL/6 mice. Food Funct, Bufo Bufotes species Differ. localities Serbia 14, 7130–7145. doi:10.1039/d2fo03603g
Coady, K. K., Murphy, M. B., Villeneuve, D. L., Hecker, M., Jones, P. D., Carr, J. A., et al. (2004). Effects of atrazine on metamorphosis, growth, and gonadal development in the green frog (Rana clamitans). J. Toxicol. Environ. Health 67 (12), 941–957. doi:10.1080/15287390490443722
Cong, L., Qin, Z. F., Jing, X. N., Yang, L., Zhou, J. M., and Xu, X. B. (2006). Xenopus laevis is a potential alternative model animal species to study reproductive toxicity of phytoestrogens. Aquat. Toxicol. 77 (3), 250–256. doi:10.1016/j.aquatox.2005.12.014
Croteau, M. C., Davidson, M., Duarte-Guterman, P., Wade, M., Popesku, J. T., Wiens, S., et al. (2009). Assessment of thyroid system disruption in Rana pipiens tadpoles chronically exposed to UVB radiation and 4-tert-octylphenol. Aquat. Toxicol. 95 (2), 81–92. doi:10.1016/J.AQUATOX.2009.05.013
Cruz-Santiago, O., Flores-Ramírez, R., Ilizaliturri-Hernández, C. A., Méndez-Rodríguez, K. B., Cilia-López, V. G., Espinosa-Reyes, G., et al. (2023). Levels of persistent organic pollutants in cane toads (Rhinella marina) differ among sites with varying industrial and agricultural activities. Environ. Toxicol. Pharmacol. 103, 104280. doi:10.1016/j.etap.2023.104280
Dournon, C., Guillet, F., Boucher, D., and Lacroix, J. C. (1984). Cytogenetic and genetic evidence of male sexual inversion by heat treatment in the newt Pleurodeles poireti. Chromosoma 90, 261–264. doi:10.1007/BF00287033
Dournon, C., Houillon, C., and Pieau, C. (1990). Temperature sex-reversal in amphibians and reptiles. Int. J. Dev. Biol. 34 (1), 81–92. doi:10.1387/IJDB.2393628
Du, X., Yuan, B., Zhou, Y., Zheng, Z., Wu, Y., Qiu, Y., et al. (2019). Tissue-specific accumulation, sexual difference, and maternal transfer of chlorinated paraffins in black-spotted frogs. Environ. Sci. Technol. 53 (9), 4739–4746. doi:10.1021/acs.est.8b06350
Duellman, W. E., and Trueb, L. (1994). Sex chromosomes as supergenes of speciation: why amphibians defy the rules? Biology of amphibians. Baltimore, MD: JHU Press
Dufresnes, C., and Crochet, P. A. (2022). Sex chromosomes as supergenes of speciation: why amphibians defy the rules? Philosophical Trans. R. Soc. B 377, 20210202. doi:10.1098/RSTB.2021.0202
Dumond, H., Kuntz, S., Chesnel, A., Ko, C.-I., Wallacides, A., Chardard, D., et al. (2008). Sexual development of the urodele amphibian Pleurodeles waltl. Sex. Dev. 2, 104–114. doi:10.1159/000129695
Dumont, J. N., Schultz, T. W., Buchanan, M. V., and Kao, G. L. (1983). “Frog embryo Teratogenesis assay: Xenopus (FETAX) — a short-term assay applicable to complex environmental mixtures,” in Short-term bioassays in the analysis of complex environmental mixtures III. Editors M. D. Waters, S. S. Sandhu, J. Lewtas, L. Claxton, N. Chernoff, and S. Nesnow (Springer US), 393–405. doi:10.1007/978-1-4613-3611-2_27
Escoriza, D. (2014). Predation of Hyla intermedia egg-clutches by tadpoles of Discoglossus pictus in Sicily. Herpetol. Notes 7, 575–576.
European Commission, (1996). European workshop on the impact of endocrine disrupters on human health and wildlife, 17549. Weybridge, United Kingdom: DG XII Weybridge Environment and Climate Research Programme Report Europe.
Evans, B. J., Pyron, R. A., and Wiens, J. J. (2012). “Polyploidization and sex chromosome evolution in amphibians,” in Polyploidy and genome evolution (Springer), 385–410. doi:10.1007/978-3-642-31442-1_18
Exbrayat, J. M. (1992). Reproduction et organes endocrines chez les femelles d’un Amphibien Gymnophione vivipare, Typhlonectes compressicaudus. Bull. La Société Herpétologique Fr. 64, 37–50.
Fan, J.-J., Wang, S., Tang, J.-P., Zhao, J.-L., Wang, L., Wang, J.-X., et al. (2019). Bioaccumulation of endocrine disrupting compounds in fish with different feeding habits along the largest subtropical river, China. Environ. Pollut. 247, 999–1008. doi:10.1016/j.envpol.2019.01.113
Fort, D. J., Mathis, M. B., Pawlowski, S., Wolf, J. C., Peter, R., and Champ, S. (2017). Effect of triclosan on anuran development and growth in a larval amphibian growth and development assay. J. Appl. Toxicol. 37 (10), 1182–1194. doi:10.1002/jat.3474
Fox, H. (1977). The anuran tadpole skin: changes occurring in it during metamorphosis and some comparisons with that of the adult. Symposia Zoological Soc. Lond. 39, 269–289.
Frost, D. R. (2024). Amphibian Species of the World: an Online Reference. Version 6.2 (Date of access: 31.12.2024). Electronic Database accessible at https://amphibiansoftheworld.amnh.org/index.php. (New York, United States: American Museum of Natural History). doi:10.5531/db.vz.0001
Fryday, S., and Thompson, H. (2017). Toxicity of pesticides to aquatic and terrestrial life stages of amphibians and occurrence, habitat use and exposure of amphibian species in agricultural environments. EFSA Support. Publ. 9 (9), 343E. doi:10.2903/SP.EFSA.2012.EN-343
Fuentes, L., Moore, L. J., Rodgers, J. H., Bowerman, W. W., Yarrow, G. K., and Chao, W. Y. (2014). Role of sediments in modifying the toxicity of two Roundup formulations to six species of larval anurans. Environ. Toxicol. Chem. 33 (11), 2616–2620. doi:10.1002/ETC.2725
Furman, B. L. S., Bewick, A. J., Harrison, T. L., Greenbaum, E., Gvoždík, V., Kusamba, C., et al. (2015). Pan-African phylogeography of a model organism, the African clawed frog “Xenopus laevis.”. Mol. Ecol. 24 (4), 909–925. doi:10.1111/mec.13076
Furman, B. L. S., Cauret, C. M. S., Knytl, M., Song, X.-Y., Premachandra, T., Ofori-Boateng, C., et al. (2020). A frog with three sex chromosomes that co-mingle together in nature: Xenopus tropicalis has a degenerate W and a Y that evolved from a Z chromosome. PLOS Genet. 16 (11), e1009121. doi:10.1371/journal.pgen.1009121
Gao, J., and Shen, W. (2021). Xenopus in revealing developmental toxicity and modeling human diseases. Environ. Pollut. 268, 115809. doi:10.1016/j.envpol.2020.115809
Gomes, A. D., Moreira, R. G., Navas, C. A., Antoniazzi, M. M., and Jared, C. (2012). Review of the reproductive biology of caecilians (Amphibia, Gymnophiona). S. Am. J. Herpetology 7 (3), 191–202. doi:10.2994/057.007.0301
Govindappa, V., and Venkatachalaiah, G. (2005). Karyology of two species of Caecilians (Caeciliidae: gymnophiona): evolution through tandem fusion and sex chromosome dimorphism. Caryologia 58 (2), 140–151. doi:10.1080/00087114.2005.10589444
Gramapurohit, N. P., Shanbhag, B. A., and Saidapur, S. K. (2000). Pattern of gonadal sex differentiation, development, and onset of steroidogenesis in the frog, Rana curtipes. General Comp. Endocrinol. 119 (3), 256–264. doi:10.1006/GCEN.2000.7513
Green, D. M. (1988). Cytogenetics of the endemic New Zealand frog, Leiopelma hochstetteri: extraordinary supernumerary chromosome variation and a unique sex-chromosome system. Chromosoma 97 (1), 55–70. doi:10.1007/bf00331795
Guan, K.-L., Luo, X.-J., Zhu, C.-H., Chen, X., Chen, P.-P., Guo, J., et al. (2024). Tissue-specific distribution and maternal transfer of persistent organic halogenated pollutants in frogs. Environ. Toxicol. Chem. 43, 1557–1568. doi:10.1002/etc.5882
Harvey, L. A., and Propper, C. R. (1997). Effects of androgens on male sexual behavior and secondary sex characters in the explosively breeding spadefoot toad, Scaphiopus couchii. Hormones Behav. 31 (1), 89–96. doi:10.1006/HBEH.1997.1354
Hayes, T. (2005). Comment on “gonadal development of larval male Xenopus laevis exposed to atrazine in outdoor microcosms.”. Environ. Sci. Technol. 39 (19), 7757–7758. doi:10.1021/ES051099I
Hayes, T., Haston, K., Tsui, M., Hoang, A., Haeffele, C., and Vonk, A. (2003). Atrazine-induced hermaphroditism at 0.1 ppb in American leopard frogs (Rana pipiens): laboratory and field evidence. Environ. Health Perspect. 111 (4), 568–575. doi:10.1289/ehp.5932
Hayes, T. B. (1998). Sex determination and primary sex differentiation in amphibians: genetic and developmental mechanisms. J. Exp. Zoology 281 (5), 373–399. doi:10.1002/(SICI)1097-010X(19980801)281:5<373::AID-JEZ4>3.0.CO;2-L
Hayes, T. B., Case, P., Chui, S., Chung, D., Haeffele, C., Haston, K., et al. (2006). Pesticide mixtures, endocrine disruption, and amphibian declines: are we underestimating the impact? Environ. Health Perspect. 114 (Suppl. 1), 40–50. doi:10.1289/ehp.8051
Hayes, T. B., Collins, A., Lee, M., Mendoza, M., Noriega, N., Stuart, A. A., et al. (2002). Hermaphroditic, demasculinized frogs after exposure to the herbicide atrazine at low ecologically relevant doses. Proc. Natl. Acad. Sci. 99 (8), 5476–5480. doi:10.1073/PNAS.082121499
Hillyard, S. D., Møbjerg, N., Tanaka, S., and Larsen, E. H. (2008). Osmotic and ion regulation in amphibians. in Osmotic and ionic regulation (Boca Raton, FL: CRC Press), 367–441. doi:10.1201/9780849380525-9
Hopkins, W. A., DuRant, S. E., Staub, B. P., Rowe, C. L., and Jackson, B. P. (2006). Reproduction, embryonic development, and maternal transfer of contaminants in the Amphibian Gastrophryne carolinensis. Environ. Health Perspect. 114 (5), 661–666. doi:10.1289/EHP.8457
Hoskins, T. D., Dellapina, M., Papoulias, D. M., and Boone, M. D. (2019). Effects of larval atrazine exposure in mesocosms on Blanchard’s cricket frogs (Acris blanchardi) reared through overwintering and to reproductive age. Chemosphere 220, 845–857. doi:10.1016/j.chemosphere.2018.12.112
Howe, C. M., Berrill, M., Pauli, B. D., Helbing, C. C., Werry, K., and Veldhoen, N. (2004). Toxicity of glyphosate-based pesticides to four North American frog species. Environ. Toxicol. Chem. 23 (8), 1928–1938. doi:10.1897/03-71
Hu, F., Smith, E. E., and Carr, J. A. (2008). Effects of larval exposure to estradiol on spermatogenesis and in vitro gonadal steroid secretion in African clawed frogs, Xenopus laevis. General Comp. Endocrinol. 155 (1), 190–200. doi:10.1016/J.YGCEN.2007.04.015
Huang, Y.-W., Matthews, J. B., Fertuck, K. C., and Zacharewski, T. R. (2005). Use of Xenopus laevis as a model for investigating in vitro and in vivo endocrine disruption in amphibians. Environ. Toxicol. Chem. 24 (8), 2002–2009. doi:10.1897/04-378r1.1
Iwasawa, H., and Kobayashi, M. (1974). Effects of testosterone and estradiol on the development of sexual characters in young Rana nigromaculata. Biol. Reproduction 11 (4), 398–405. doi:10.1095/BIOLREPROD11.4.398
Jeganathan, P., and Rajakaruna, R. S. (2021). Testicular oocyte development and other toxic effects in the common hourglass tree frog exposed to three commercial insecticide formulations. Ceylon J. Sci. 50 (3), 249–259. doi:10.4038/cjs.v50i3.7906
Johnson, B. K., and Christiansen, J. L. (1976). The food and food habits of Blanchard's cricket frog, Acris crepitans blanchardi (Amphibia, Anura, hylidae), in Iowa. Iowa. J. Herpetology 10, 63. doi:10.2307/1562786
Johnson, M. S., Aubee, C., Salice, C. J., Leigh, K. B., Liu, E., Pott, U., et al. (2017). A review of ecological risk assessment methods for amphibians: comparative assessment of testing methodologies and available data. Integr. Environ. Assess. Manag. 13 (4), 601–613. doi:10.1002/ieam.1881
Jooste, A. M., Preez, L. H. D., Carr, J. A., Giesy, J. P., Gross, T. S., Kendall, R. J., et al. (2005). Gonadal development of larval male Xenopus laevis exposed to atrazine in outdoor microcosms. Environ. Sci. Technol. 39 (14), 5255–5261. doi:10.1021/es048134q
Kadokami, K., Takeishi, M., Kuramoto, M., and Ono, Y. (2004). Maternal transfer of organochlorine pesticides, polychlorinated dibenzo-p-dioxins, dibenzofurans, and coplanar polychlorinated biphenyls in frogs to their eggs. Chemosphere 57 (5), 383–389. doi:10.1016/j.chemosphere.2004.06.014
Khan, M. (2018). Ecology and breeding habits of Fejervarya frogs, with notes on morphology and feeding habits of their tadpoles.
Kloas, W. (2002). Amphibians as a model for the study of endocrine disruptors. Int. Rev. Cytol. 216, 1–57. doi:10.1016/s0074-7696(02)16002-5
Kloas, W., and Lutz, I. (2006). Amphibians as model to study endocrine disrupters. J. Chromatogr. A 1130 (1), 16–27. doi:10.1016/j.chroma.2006.04.001
Kloas, W., Lutz, I., and Einspanier, R. (1999). Amphibians as a model to study endocrine disruptors: II. Estrogenic activity of environmental chemicals in vitro and in vivo. Sci. Total Environ. 225 (1–2), 59–68. doi:10.1016/s0048-9697(99)80017-5
Kloas, W., Stöck, M., Lutz, I., and Ziková-Kloas, A. (2024). Endocrine disruption in teleosts and amphibians is mediated by anthropogenic and natural environmental factors: implications for risk assessment. Philosophical Trans. R. Soc. B Biol. Sci. 379 (1898), 20220505. doi:10.1098/rstb.2022.0505
Konno, K., Akasaka, M., Koshida, C., Katayama, N., Osada, N., Spake, R., et al. (2020). Ignoring non-English-language studies may bias ecological meta-analyses. Ecol. Evol. 10 (13), 6373–6384. doi:10.1002/ece3.6368
Kuhl, H., Tan, W. H., Klopp, C., Kleiner, W., Koyun, B., Ciorpac, M., et al. (2024). A candidate sex determination locus in amphibians which evolved by structural variation between X- and Y-chromosomes. Nat. Commun. 15 (1), 4781. doi:10.1038/s41467-024-49025-2
Kwak, J. I., and An, Y.-J. (2021). Assessing potential indicator of endocrine-disrupting property of chemicals using soil invertebrates. Comp. Biochem. Physiology Part C Toxicol. and Pharmacol. 245, 109036. doi:10.1016/j.cbpc.2021.109036
LaFiandra, E. M., Babbitt, K. J., and Sower, S. A. (2008). Effects of atrazine on anuran development are altered by the presence of a nonlethal predator. J. Toxicol. Environ. Health - Part A Curr. Issues 71 (8), 505–511. doi:10.1080/15287390801907442
Lambert, M. R., Giller, G. S. J., Skelly, D. K., and Bribiescas, R. G. (2016). Septic systems, but not sanitary sewer lines, are associated with elevated estradiol in male frog metamorphs from suburban ponds. General Comp. Endocrinol. 232, 109–114. doi:10.1016/J.YGCEN.2016.01.009
Lambert, M. R., Smylie, M. S., Roman, A. J., Freidenburg, L. K., and Skelly, D. K. (2018). Sexual and somatic development of wood frog tadpoles along a thermal gradient. J. Exp. Zoology. Part A, Ecol. Integr. Physiology 329 (2), 72–79. doi:10.1002/JEZ.2172
Lambert, M. R., Tran, T., Kilian, A., Ezaz, T., and Skelly, D. K. (2019). Molecular evidence for sex reversal in wild populations of green frogs (Rana clamitans). PeerJ 7, e6449. doi:10.7717/PEERJ.6449
La Merrill, M. A., Vandenberg, L. N., Smith, M. T., Goodson, W., Browne, P., Patisaul, H. B., et al. (2020). Consensus on the key characteristics of endocrine-disrupting chemicals as a basis for hazard identification. Nat. Rev. Endocrinol. 16 (1), 45–57. doi:10.1038/s41574-019-0273-8
Lenhardt, P. P., Brühl, C. A., and Berger, G. (2015). Temporal coincidence of amphibian migration and pesticide applications on arable fields in spring. Basic Appl. Ecol. 16 (1), 54–63. doi:10.1016/j.baae.2014.10.005
Liu, Y. E., Luo, X. J., Guan, K. L., Huang, C. C., Zhu, C. Y., Qi, X. M., et al. (2020). Legacy and emerging organophosphorus flame retardants and plasticizers in frogs: sex difference and parental transfer. Environ. Pollut. 266, 115336. doi:10.1016/J.ENVPOL.2020.115336
Luedtke, J. A., Chanson, J., Neam, K., Hobin, L., Maciel, A. O., Catenazzi, A., et al. (2023). Ongoing declines for the world’s amphibians in the face of emerging threats. Nature 622 (7982), 308–314. doi:10.1038/s41586-023-06578-4
Lutz, I., Kloas, W., Springer, T. A., Holden, L. R., Wolf, J. C., Krueger, H. O., et al. (2008). Development, standardization and refinement of procedures for evaluating effects of endocrine active compounds on development and sexual differentiation of Xenopus laevis. Anal. Bioanal. Chem. 390, 2031–2048. doi:10.1007/S00216-008-1973-4
Ma, W. J., and Veltsos, P. (2021). The diversity and evolution of sex chromosomes in frogs. Genes 12 (4), 483. doi:10.3390/GENES12040483
Mackenzie, C. A., Berrill, M., Metcalfe, C., and Pauli, B. D. (2003). Gonadal differentiation in frogs exposed to estrogenic and antiestrogenic compounds. Environ. Toxicol. Chem. An Int. J. 22 (10), 2466–2475. doi:10.1897/02-173
Mali, P. V., and Gramapurohit, N. P. (2016). Are sex steroids essential for gonadal differentiation of the ornate frog, Microhyla ornata? General Comp. Endocrinol. 233, 63–72. doi:10.1016/J.YGCEN.2016.05.019
Masta, S. E., Sullivan, B. K., Lamb, T., and Routman, E. J. (2002). Molecular systematics, hybridization, and phylogeography of the Bufo americanus complex in Eastern North America. Mol. Phylogenetics Evol. 24 (2), 302–314. doi:10.1016/s1055-7903(02)00216-6
Mawaribuchi, S., Ito, M., Ogata, M., Yoshimura, Y., and Miura, I. (2023). Parallel evolution of sex-linked genes across XX/XY and ZZ/ZW sex chromosome systems in the Frog Glandirana rugosa. Genes 14 (2), 257. doi:10.3390/genes14020257
R. W. McDiarmid, and R. Altig (2000). Tadpoles: the biology of Anuran larvae (University of Chicago Press). Available online at: https://press.uchicago.edu/ucp/books/book/chicago/T/bo3643050.html.
Meegaskumbura, M., Boyagoda, S., Bowatte, G., Manamendra-Arachchi, K., Pethiyagoda, R., Hanken, J., et al. (2010). Taruga (Anura: rhacophoridae), a new genus of foam-nesting tree frogs endemic to Sri Lanka. J. Sci. Bio. Sci. 39, 75–94. doi:10.4038/cjsbs.v39i2.2995
Méndez, S. I. S., Tillitt, D. E., Rittenhouse, T. A. G., and Semlitsch, R. D. (2009). Behavioral response and kinetics of terrestrial atrazine exposure in American toads (Bufo americanus). Archives Environ. Contam. Toxicol. 57 (3), 590–597. doi:10.1007/S00244-009-9292-0
Mikó, Z., Nemesházi, E., Ujhegyi, N., Verebélyi, V., Ujszegi, J., Kásler, A., et al. (2021). Sex reversal and ontogeny under climate change and chemical pollution: are there interactions between the effects of elevated temperature and a xenoestrogen on early development in agile frogs? Environ. Pollut. 285, 117464. doi:10.1016/J.ENVPOL.2021.117464
Mikó, Z., Ujszegi, J., Gál, Z., and Hettyey, A. (2017). Standardize or diversify experimental conditions in ecotoxicology? A case study on herbicide toxicity to larvae of two anuran amphibians. Archives Environ. Contam. Toxicol. 73 (4), 562–569. doi:10.1007/s00244-017-0427-4
Mintz, B., Foote, C. L., and Witschi, E. (1945). Quantitative studies on response of sex characters of differentiated Rana clamitans larvae to injected androgens and estrogens. Endocrinology 37 (4), 286–296. doi:10.1210/ENDO-37-4-286
Miura, I. (2007). An evolutionary witness: the frog Rana rugosa underwent change of heterogametic sex from XY male to ZW female. Sex. Dev. 1 (6), 323–331. doi:10.1159/000111764
Miura, I., Ohtani, H., Ogata, M., and Ezaz, T. (2016). Evolutionary changes in sensitivity to hormonally induced gonadal sex reversal in a frog species. Sex. Dev. 10 (2), 79–90. doi:10.1159/000445848
Mizukawa, K., Takada, H., Takeuchi, I., Ikemoto, T., Omori, K., and Tsuchiya, K. (2009). Bioconcentration and biomagnification of polybrominated diphenyl ethers (PBDEs) through lower-trophic-level coastal marine food web. Mar. Pollut. Bull. 58 (8), 1217–1224. doi:10.1016/j.marpolbul.2009.03.008
Müller, A.-K., Markert, N., Leser, K., Kämpfer, D., Crawford, S. E., Schäffer, A., et al. (2020). Assessing endocrine disruption in freshwater fish species from a “hotspot” for estrogenic activity in sediment. Environ. Pollut. 257, 113636. doi:10.1016/j.envpol.2019.113636
Nakamura, M. (2009). Sex determination in amphibians. Seminars Cell Dev. Biol. 20, 271–282. doi:10.1016/j.semcdb.2008.10.003
Nakamura, M. (2013). Is a sex-determining gene(s) necessary for sex-determination in amphibians? Steroid hormones may be the key factor. Sex. Dev. 7 (1–3), 104–114. doi:10.1159/000339661
NatureServe Explorer (2023). Glandirana rugosa. Available online at: https://explorer.natureserve.org/Taxon/ELEMENT_GLOBAL.2.100244/Glandirana_rugosa (Accessed January 3, 2025).
Nemesházi, E., and Bókony, V. (2022). Asymmetrical sex reversal: does the type of heterogamety predict propensity for sex reversal? BioEssays 44 (7), 2200039. doi:10.1002/BIES.202200039
Nemesházi, E., and Bókony, V. (2023). HerpSexDet: the herpetological database of sex determination and sex reversal. Scientific Data 10, 377. doi:10.1038/s41597-023-02268-y
Nemesházi, E., Gál, Z., Ujhegyi, N., Verebélyi, V., Mikó, Z., Üveges, B., et al. (2020). Novel genetic sex markers reveal high frequency of sex reversal in wild populations of the agile frog (Rana dalmatina) associated with anthropogenic land use. Mol. Ecol. 29 (19), 3607–3621. doi:10.1111/mec.15596
Nenni, M. J., Fisher, M. E., James-Zorn, C., Pells, T. J., Ponferrada, V., Chu, S., et al. (2019). Xenbase: facilitating the use of Xenopus to model human disease. Front. Physiology 10, 154. doi:10.3389/fphys.2019.00154
Neuman-Lee, L. A., Gaines, K. F., Baumgartner, K. A., Voorhees, J. R., Novak, J. M., and Mullin, S. J. (2014). Assessing multiple endpoints of atrazine ingestion on gravid northern watersnakes (Nerodia sipedon) and their offspring. Environ. Toxicol. 29 (9), 1072–1082. doi:10.1002/TOX.21837
Nolan, N., Hayward, M.W., Klop-Toker, K., Mahony, M., Lemckert, F., and Callen, A. (2023). Complex organisms must deal with complex threats: how does amphibian conservation deal with biphasic life cycles?. Animals 13 (10), 1634. doi:10.3390/ani13101634
Norris, D. O. (2011a). Endocrine disruption of reproduction in Amphibians. Hormones Reproduction Vertebrates - 2, 203–211. doi:10.1016/B978-0-12-374931-4.10011-2
Norris, D. O. (2011b). Hormones and reproductive patterns in urodele and gymnophionid amphibians. Hormones Reproduction Vertebrates 2, 187–202. doi:10.1016/B978-0-12-374931-4.10010-0
Ogata, M., Ohtani, H., Igarashi, T., Hasegawa, Y., Ichikawa, Y., and Miura, I. (2003). Change of the heterogametic sex from male to female in the frog. Genetics 164 (2), 613–620. doi:10.1093/GENETICS/164.2.613
Ogielska, M. (2009). “The undifferentiated Amphibian gonad,” in Reproduction of Amphibians. 1st Edition (Boca Raton, FL: CRC Press), 1–33.
Ogielska, M., and Kotusz, A. (2004). Pattern and rate of ovary differentiation with reference to somatic development in anuran amphibians. J. Morphol. 259 (1), 41–54. doi:10.1002/jmor.10162
Ohtani, H., Miura, I., and Ichikawa, Y. (2000). Effects of dibutyl phthalate as an environmental endocrine disruptor on gonadal sex differentiation of genetic males of the frog Rana rugosa. Environ. Health Perspect. 108 (12), 1189–1193. doi:10.1289/EHP.001081189
Oike, A., Nakamura, Y., Yasumasu, S., Ito, E., and Nakamura, M. (2020). A threshold dosage of estrogen for male-to-female sex reversal in the Glandirana rugosa frog. J. Exp. Zoology Part A Ecol. Integr. Physiology 333 (9), 652–659. doi:10.1002/JEZ.2408
Oike, A., Watanabe, K., Min, M. S., Tojo, K., Kumagai, M., Kimoto, Y., et al. (2017). Origin of sex chromosomes in six groups of Rana rugosa frogs inferred from a sex-linked DNA marker. J. Exp. Zoology Part A Ecol. Integr. Physiology 327 (7), 444–452. doi:10.1002/JEZ.2130
Oka, T., Mitsui, N., Hinago, M., Miyahara, M., Fujii, T., Tooi, O., et al. (2006). All ZZ male Xenopus laevis provides a clear sex-reversal test for feminizing endocrine disruptors. Ecotoxicol. Environ. Saf. 63 (2), 236–243. doi:10.1016/J.ECOENV.2005.07.018
Oliveira, B. F., São-Pedro, V. A., Santos-Barrera, G., Penone, C., and Costa, G. C. (2017). AmphiBIO, a global database for amphibian ecological traits. Sci. Data 4 (1), 170123. doi:10.1038/sdata.2017.123
Olmstead, A. W., Lindberg-Livingston, A., and Degitz, S. J. (2010). Genotyping sex in the amphibian, Xenopus (Silurana) tropicalis, for endocrine disruptor bioassays. Aquat. Toxicol. 98 (1), 60–66. doi:10.1016/j.aquatox.2010.01.012
Orton, F., Carr, J. A., and Handy, R. D. (2006). Effects of nitrate and atrazine on larval development and sexual differentiation in the northern leopard frog Rana pipiens. Environ. Toxicol. Chem. 25 (1), 65–71. doi:10.1897/05-136r.1
Orton, F., Roberts-Rhodes, B., Whatley, C., and Tyler, C. R. (2023). A review of non-destructive biomonitoring techniques to assess the impacts of pollution on reproductive health in frogs and toads. Ecotoxicol. Environ. Saf. 262, 115163. doi:10.1016/J.ECOENV.2023.115163
Padhye, A., Dahanukar, N., Sulakhe, S., Dandekar, N., Limaye, S., and Jamdade, K. (2017). Sphaerotheca pashchima, a new species of burrowing frog (Anura: dicroglossidae) from western India. J. Threat. Taxa 9 (6), 10286. doi:10.11609/jott.2877.9.6.10286-10296
Paetow, L. J., Cue, R. I., Pauli, B. D., and Marcogliese, D. J. (2023). Effects of herbicides and the chytrid fungus batrachochytrium dendrobatidis on the growth, development and survival of larval American toads (Anaxyrus americanus). Ecotoxicol. Environ. Saf. 259, 115021. doi:10.1016/J.ECOENV.2023.115021
Park, B. J., and Kidd, K. (2005). Effects of the synthetic estrogen ethinylestradiol on early life stages of mink frogs and green frogs in the wild and in situ. Environ. Toxicol. Chem. 24 (8), 2027–2036. doi:10.1897/04-227R.1
Peterson, C. G., and Boulton, A. J. (1999). Stream permanence influences microalgal food availability to grazing tadpoles in arid-zone springs. Oecologia 118 (3), 340–352. doi:10.1007/s004420050735
Petrini, S., and Zaccanti, F. (1998). The effects of aromatase and 5 α-reductase inhibitors, antiandrogen, and sex steroids on Bidder’s organs development and gonadal differentiation in Bufo bufo tadpoles. J. Exp. Zoology 280 (3), 245–259. doi:10.1002/(sici)1097-010x(19980215)280:3<245::aid-jez6>3.0.co;2-n
Phuge, S., Sequeira, A., and Pandit, R. (2021). Effect of ethylenethiourea on metamorphosis and ovary development: a comparative study of three larval frogs. J. Exp. Zoology Part A Ecol. Integr. Physiology 335 (5), 469–476. doi:10.1002/jez.2464
Phuge, S. K., and Gramapurohit, N. P. (2015). Sex hormones alter sex ratios in the Indian skipper frog, Euphlyctis cyanophlyctis: determining sensitive stages for gonadal sex reversal. General Comp. Endocrinol. 220, 70–77. doi:10.1016/J.YGCEN.2014.04.030
Piazza, Y. G., Czuchlej, S. C., Gómez, M. L., and Meijide, F. J. (2023). Gonadal morphogenesis in the South American toad Rhinella Arenarum (Anura, Bufonidae) unveils an extremely delayed rate of sex differentiation. J. Morphol. 284 (8), e21611. doi:10.1002/JMOR.21611
Pickford, D. B., Hetheridge, M. J., Caunter, J. E., Hall, A. T., and Hutchinson, T. H. (2003). Assessing chronic toxicity of bisphenol A to larvae of the African clawed frog (Xenopus laevis) in a flow-through exposure system. Chemosphere 53 (3), 223–235. doi:10.1016/S0045-6535(03)00308-4
Piprek, R. P., Pecio, A., Kubiak, J. Z., and Szymura, J. M. (2012). Differential effects of testosterone and 17β-estradiol on gonadal development in five anuran species. Reproduction 144 (2), 257–267. doi:10.1530/REP-12-0048
Preez, L. H. D., Kunene, N., Everson, G. J., Carr, J. A., Giesy, J. P., Gross, T. S., et al. (2008). Reproduction, larval growth, and reproductive development in African clawed frogs (Xenopus laevis) exposed to atrazine. Chemosphere 71 (3), 546–552. doi:10.1016/j.chemosphere.2007.09.051
Preez, L. H. D., Kunene, N., Hanner, R., Giesy, J. P., Solomon, K. R., Hosmer, A., et al. (2009). Population-specific incidence of testicular ovarian follicles in Xenopus laevis from South Africa: a potential issue in endocrine testing. Aquat. Toxicol. 95 (1), 10–16. doi:10.1016/j.aquatox.2009.07.018
Previšić, A., Vilenica, M., Vučković, N., Petrović, M., and Rožman, M. (2021). Aquatic insects transfer pharmaceuticals and endocrine disruptors from aquatic to terrestrial ecosystems. Environ. Sci. and Technol. 55 (6), 3736–3746. doi:10.1021/acs.est.0c07609
Purucker, S. T., Snyder, M. N., Glinski, D. A., Van Meter, R. J., Garber, K., Chelsvig, E. A., et al. (2023). Estimating dermal contact soil exposure for amphibians. Integr. Environ. Assess. Manag. 19 (1), 9–16. doi:10.1002/ieam.4619
Quaranta, A., Bellantuono, V., Cassano, G., and Lippe, C. (2009). Why amphibians are more sensitive than mammals to xenobiotics. PLoS ONE 4 (11), e7699. doi:10.1371/JOURNAL.PONE.0007699
Reeder, A. L., Foley, G. L., Nichols, D. K., Hansen, L. G., Wikoff, B., Faeh, S., et al. (1998). Forms and prevalence of intersexuality and effects of environmental contaminants on sexuality in cricket frogs (Acris crepitans). Environ. Health Perspect. 106 (5), 261–266. doi:10.1289/EHP.98106261
Regnault, C., Willison, J., Veyrenc, S., Airieau, A., Méresse, P., Fortier, M., et al. (2016). Metabolic and immune impairments induced by the endocrine disruptors benzo[a]pyrene and triclosan in Xenopus tropicalis. Chemosphere 155, 519–527. doi:10.1016/j.chemosphere.2016.04.047
Richards, C. M. (1982). The alteration of chromatophore expression by sex hormones in the Kenyan reed frog, Hyperolius viridiflavus. General Comp. Endocrinol. 46 (1), 59–67. doi:10.1016/0016-6480(82)90163-0
Robert, J., and Cohen, N. (2011). The genus Xenopus as a multispecies model for evolutionary and comparative immunobiology of the 21st century. Dev. Comp. Immunol. 35 (9), 916–923. doi:10.1016/j.dci.2011.01.014
Roco, Á. S., Olmstead, A. W., Degitz, S. J., Amano, T., Zimmerman, L. B., and Bullejos, M. (2015). Coexistence of Y, W, and Z sex chromosomes in Xenopus tropicalis. Proc. Natl. Acad. Sci. U. S. A. 112 (34), E4752–E4761. doi:10.1073/PNAS.1505291112
Rodrigues, N., Vuille, Y., Loman, J., and Perrin, N. (2015). Sex-chromosome differentiation and ‘sex races’ in the common frog (Rana temporaria). Proc. R. Soc. B Biol. Sci. 282 (1806), 20142726. doi:10.1098/RSPB.2014.2726
Roelants, K., Gower, D. J., Wilkinson, M., Loader, S. P., Biju, S. D., Guillaume, K., et al. (2007). Global patterns of diversification in the history of modern amphibians. Proc. Natl. Acad. Sci. 104 (3), 887–892. doi:10.1073/pnas.0608378104
Romero-Blanco, A., and Alonso, Á. (2022). Laboratory versus wild populations: the importance of population origin in aquatic ecotoxicology. Environ. Sci. Pollut. Res. 29 (16), 22798–22808. doi:10.1007/s11356-021-17370-0
Ruhí, A., Acuña, V., Barceló, D., Huerta, B., Mor, J.-R., Rodríguez-Mozaz, S., et al. (2016). Bioaccumulation and trophic magnification of pharmaceuticals and endocrine disruptors in a Mediterranean river food web. Sci. Total Environ. 540, 250–259. doi:10.1016/j.scitotenv.2015.06.009
Ruiz-Garciá, A., Roco, Á. S., and Bullejos, M. (2021). Sex differentiation in amphibians: effect of temperature and its influence on sex reversal. Sex. Dev. 15 (1–3), 157–167. doi:10.1159/000515220
Salla, R. F., Costa, M. J., Abdalla, F. C., Oliveira, C. R., Tsukada, E., Boeing, G. A. N. S., et al. (2024b). Estrogen contamination increases vulnerability of amphibians to the deadly chytrid fungus. Sci. Total Environ. 917, 170337. doi:10.1016/j.scitotenv.2024.170337
Salla, R. F., Oliveira, F. N., Jacintho, J. C., Cirqueira, F., Tsukada, E., Vieira, L. G., et al. (2024a). Microplastics and TiO2 nanoparticles mixture as an emerging threat to amphibians: a case study on bullfrog embryos. Environ. Pollut. 346, 123624. doi:10.1016/j.envpol.2024.123624
Salo, J. V. B., and Solania, C. L. (2022). Preliminary survey and diet analysis of anurans in the riparian zone of Calayagon Watershed, Agusan Del Norte, Philippines. J. Bioresour. Manag. 9 (1), 2.
Sayyed, A. (2015). Breeding and metamorphosis of indosylvirana caesari Maharashtra golden-backed frog (ranidae) from satara, Maharashtra, India. IRCF Reptiles and Amphibians 22, 160–163. doi:10.17161/randa.v22i4.14075
Schiesari, L., Grillitsch, B., and Grillitsch, H. (2007). Biogeographic biases in research and their consequences for linking amphibian declines to pollution. Conservation Biol. J. Soc. Conservation Biol. 21 (2), 465–471. doi:10.1111/j.1523-1739.2006.00616.x
Schmid, M., Steinlein, C., and Feichtinger, W. (1992). Chromosome banding in Amphibia. XVII. First demonstration of multiple sex chromosomes in amphibians: Eleutherodactylus maussi (Anura, leptodactylidae). Chromosoma 101 (5–6), 284–292. doi:10.1007/BF00346007
Serrano-Perez, C. A., and Ramírez-Pinilla, M. P. (2021). Morphology and histology of the male reproductive tract of Caecilia thompsoni (amphibia: gymnophiona). Anat. Rec. Hob. N.J. 2007 304 (5), 1119–1135. doi:10.1002/ar.24527
Sever, D. M., and Staub, N. L. (2011). Hormones, sex accessory structures, and secondary sexual characteristics in amphibians. Hormones Reproduction Vertebrates 2, 83–98. doi:10.1016/B978-0-12-374931-4.10005-7
Singh, A., Singh, G., Singh, P., and Mishra, V. K. (2023). Overview of sources, fate, and impact of endocrine disrupting compounds in environment and assessment of their regulatory policies across different continents. Total Environ. Res. Themes 7, 100071. doi:10.1016/j.totert.2023.100071
Slaby, S., Marin, M., Marchand, G., and Lemiere, S. (2019). Exposures to chemical contaminants: what can we learn from reproduction and development endpoints in the amphibian toxicology literature? Environ. Pollut. 248, 478–495. doi:10.1016/J.ENVPOL.2019.02.014
Spolyarich, N., Hyne, R., Wilson, S., Palmer, C., and Byrne, M. (2010). Growth, development and sex ratios of Spotted Marsh Frog (Limnodynastes tasmaniensis) larvae exposed to atrazine and a herbicide mixture. Chemosphere 78 (7), 807–813. doi:10.1016/J.CHEMOSPHERE.2009.11.048
Storrs, S. I., and Semlitsch, R. D. (2008). Variation in somatic and ovarian development: predicting susceptibility of amphibians to estrogenic contaminants. General Comp. Endocrinol. 156 (3), 524–530. doi:10.1016/j.ygcen.2008.03.001
Storrs-Méndez, S. I., and Semlitsch, R. D. (2010). Intersex gonads in frogs: understanding the time course of natural development and role of endocrine disruptors. J. Exp. Zoology Part B Mol. Dev. Evol. 314 (1), 57–66. doi:10.1002/jez.b.21310
Swanson, J. E., Muths, E., Pierce, C. L., Dinsmore, S. J., Vandever, M. W., Hladik, M. L., et al. (2018). Exploring the amphibian exposome in an agricultural landscape using telemetry and passive sampling. Sci. Rep. 8 (1), 10045–10110. doi:10.1038/s41598-018-28132-3
Swaters, D., van Veen, A., van Meurs, W., Turner, J. E., and Ritskes-Hoitinga, M. (2022). A history of regulatory animal testing: what can we learn? Altern. Laboratory Animals ATLA 50 (5), 322–329. doi:10.1177/02611929221118001
Szkudlarek, M., Najbar, B., and Jankowiak, Ł. (2023). Microplastics pollution in larvae of toads, frogs and newts in anthropopressure gradient. Ecol. Indic. 155, 110971. doi:10.1016/j.ecolind.2023.11097
Tamschick, S., Rozenblut-Kościsty, B., Ogielska, M., Kekenj, D., Gajewski, F., Krüger, A., et al. (2016a). The plasticizer bisphenol A affects somatic and sexual development, but differently in pipid, hylid and bufonid anurans. Environ. Pollut. 216, 282–291. doi:10.1016/j.envpol.2016.05.091
Tamschick, S., Rozenblut-Kościsty, B., Ogielska, M., Lehmann, A., Lymberakis, P., Hoffmann, F., et al. (2016b). Sex reversal assessments reveal different vulnerability to endocrine disruption between deeply diverged anuran lineages. Sci. Rep. 6 (1), 1–8. doi:10.1038/srep23825
Tamschick, S., Rozenblut-Kościsty, B., Ogielska, M., Lehmann, A., Lymberakis, P., Hoffmann, F., et al. (2016c). Impaired gonadal and somatic development corroborate vulnerability differences to the synthetic estrogen ethinylestradiol among deeply diverged anuran lineages. Aquat. Toxicol. 177, 503–514. doi:10.1016/J.AQUATOX.2016.07.001
Tang, Y., Chen, Z. Q., Lin, Y. F., Chen, J. Y., Ding, G. H., and Ji, X. (2020). The combined effects of temperature and aromatase inhibitor on metamorphosis, growth, locomotion, and sex ratio of tiger frog (Hoplobatrachus rugulosus) tadpoles. PeerJ 2020 (3), e8834. doi:10.7717/peerj.8834
Tanimura, A., and Iwasawa, H. (1989). Origin of somatic cells and histogenesis in the primordial gonad of the Japanese tree frog Rhacophorus arboreus. Anat. Embryology 180 (2), 165–173. doi:10.1007/bf00309768
Testing, A. S. for and Materials. (1998). Standard guide for conducting the frog embryo Teratogenesis assay-Xenopus (fetax).
Traijitt, T., Kitana, N., Khonsue, W., and Kitana, J. (2021). Chronological changes in the somatic development of Hoplobatrachus rugulosus (wiegmann, 1834) (Anura: dicroglossidae).
Veseli, M., Rožman, M., Vilenica, M., Petrović, M., and Previšić, A. (2022). Bioaccumulation and bioamplification of pharmaceuticals and endocrine disruptors in aquatic insects. Sci. Total Environ. 838, 156208. doi:10.1016/j.scitotenv.2022.156208
Viertel, B., Lötters, S., Baumgart, A., Oberst, M., Eisenbeis, G., and Veith, M. (2007). Larval morphology of reed frogs, Hyperolius kivuensis and H viridiflavus, from western Kenya (Amphibia, Hyperoliidae). Revue Suisse de Zoologie; Ann. de La Société Zoologique Suisse Du Muséum d’histoire Naturelle de Genève 114, 825–837. doi:10.5962/bhl.part.80417
Wagner, N., and Viertel, B. (2017). “The use of aquatic life-stages of European Amphibians in toxicological studies,” in Ecotoxicology and genotoxicology: non-traditional aquatic models Editor M. L. Larramendy 33rd ed. (London, United Kingdom: The Royal Society of Chemistry), 371–406. doi:10.1039/9781782629887-00371
Wake, D. B., and Koo, M. S. (2018). Amphibians. Curr. Biol. CB 28 (21), R1237–R1241. doi:10.1016/j.cub.2018.09.028
Wallingford, J. B. (2022). A quick history of Xenopus: “The Humble Batrachian.”. in Xenopus. (Boca Raton, FL: CRC Press), 3–12. doi:10.1201/9781003050230-2
Wells, K. D. (2010). The ecology and behavior of Amphibians. University of Chicago Press. Available online at: https://press.uchicago.edu/ucp/books/book/chicago/E/bo5298950.html.
Wells, K. D. (2010). The ecology and behavior of Amphibians. University of Chicago Press. Available online at: https://press.uchicago.edu/ucp/books/book/chicago/E/bo5298950.html.
Windle, S., McMurry, S., Brain, R., Maul, J., Pickford, D. B., Wolf, J., et al. (2021). Evaluating a developmental endocrine toxicity assay for Blanchard’s cricket frog (Acris blanchardi) in outdoor enclosures. Sci. Total Environ. 767, 145444. doi:10.1016/J.SCITOTENV.2021.145444
Wu, J. P., Luo, X. J., Zhang, Y., Chen, S. J., Mai, B. X., Guan, Y. T., et al. (2009). Residues of polybrominated diphenyl ethers in frogs (Rana limnocharis) from a contaminated site, South China: tissue distribution, biomagnification, and maternal transfer. Environ. Sci. Technol. 43 (14), 5212–5217. doi:10.1021/es901103y
Xu, Y., Du, Z., Liu, J., Su, H., Ning, F., Cui, S., et al. (2022). Male heterogametic sex determination in Rana dybowskii based on sex-linked molecular markers. Integr. Zool. 17 (1), 105–114. Article 1. doi:10.1111/1749-4877.12577
Yoshimoto, S., Okada, E., Umemoto, H., Tamura, K., Uno, Y., Nishida-Umehara, C., et al. (2008). A W-linked DM-domain gene, DM-W, participates in primary ovary development in Xenopus laevis. Proc. Natl. Acad. Sci. U. S. A. 105 (7), 2469–2474. doi:10.1073/PNAS.0712244105
Zaborski, P. (1986). Temperature and estrogen dependent changes of sex phenotype and H-Y antigen expression in gonads of a newt. Prog. Clin. Biol. Res. 217A, 163–169.
Keywords: EDC, environmental pollution, model species, ecotoxicology, one health
Citation: Frątczak M, Kaczmarski M, Szkudelska K and Tryjanowski P (2025) Assessing species bias in amphibian research on endocrine disruptors: beyond Xenopus laevis. Front. Environ. Sci. 13:1556788. doi: 10.3389/fenvs.2025.1556788
Received: 07 January 2025; Accepted: 03 April 2025;
Published: 14 May 2025.
Edited by:
Nsikak U. Benson, Topfaith University, NigeriaReviewed by:
Kelsey Hauser, Noblis, Inc., United StatesTyrone Hayes, University of California, Berkeley, United States
Copyright © 2025 Frątczak, Kaczmarski, Szkudelska and Tryjanowski. This is an open-access article distributed under the terms of the Creative Commons Attribution License (CC BY). The use, distribution or reproduction in other forums is permitted, provided the original author(s) and the copyright owner(s) are credited and that the original publication in this journal is cited, in accordance with accepted academic practice. No use, distribution or reproduction is permitted which does not comply with these terms.
*Correspondence: Martyna Frątczak, bWFydHluYS5mcmF0Y3pha0B1cC5wb3puYW4ucGw=