- 1College of Geology and Environment, Xi’an University of Science and Technology, Xi’an, Shaanxi, China
- 2Shaanxi Provincial Key Laboratory of Geological Support for Coal Green Exploitation, Xi’an, Shaanxi China
- 3Key Laboratory of Coal Resources Exploration and Comprehensive Utilization, Ministry of Land and Resources, Xi’an, Shaanxi China
- 4Postdoctoral Research Station of Geological Resource and Geological Engineering, Xi’an University of Science and Technology, Xi’an, Shaanxi, China
With the development of industry and the popularization of automobiles, a large number of tires are produced globally every year, and the tire rubber powder contaminants (TRPC) generated by the friction with the ground during the driving of automobiles. Then the TRPC settled into the soil through atmospheric circulation, causing soil pollution and altering the properties of arable land. This paper focused on the impact of TRPC on the physical properties of loess, dealing with the effect of TRPC on the electrical resistivity, thermal conductivity, and ultrasonic velocity of loess. Conclusion can be drawn as follows: (1) resistivity of loess increased with the increase of TRPC content; (2) thermal conductivity of loess decreased with the increase of TRPC content; (3) ultrasonic wave velocity of loess decreased with the increase of TRPC content; (4) soil resistivity decreased with the increase of water content, thermal conductivity of soil increased with the increase of water content, and ultrasonic wave velocity showed an increasing then decreasing trend with the increase of water content. This paper analyzed the reasons for the change in the physical properties of loess from two aspects: the inherent properties of TRPC and water, and the microstructure changes of loess due to the addition of TRPC and water. This research findings could provide valuable reference for pollutant treatment, agricultural irrigation, soil structure assessment, geological hazard early warning and to rise widespread social attention to TRPC-contaminated soil.
1 Introduction
With the rapid development of the global economy, various ecological issues have gradually emerged. Land, as the primary habitat for human activities, is affected by pollutants such as industrial and agricultural waste, fertilizers, pesticides, sedimentation, and acid rain, becoming a convergence point for various pollutants (Solís-Ramos et al., 2021; Zeng et al., 2019). Soil, as a key role in the ecosystem, connects rocks, water, and the atmosphere. Therefore, soil pollution is directly related to air and water pollution (Zhang et al., 2023). Soil pollution threatens biodiversity directly and is closely related to food safety and human health (Münzel et al., 2024; Simpson, 2023). Therefore, soil environmental pollution has become a global environmental and public health issue (Xia et al., 2024).
In response to soil pollution issues, many investigators have studied the impact of different pollutants on soil, including heavy metals, petroleum hydrocarbons, antibiotics, microplastics, etc (Hanif et al., 2024; Mendes et al., 2024; Wu et al., 2024; Wang et al., 2024; Wan et al., 2019; Xu et al., 2023; Zhang et al., 2024). With the rapid development of economy and society, new pollutants are produced continuously. In response to soil pollution, researchers have proposed various remediation methods, such as plant remediation, microbial remediation, animal remediation technology, soil leaching technology and other treatment techniques (Hidalgo et al., 2024; Kwon et al., 2023; Sharma et al., 2024; Yu et al., 2024).
With the development of socio-economics, a large number of cars have emerged, resulting in a large number of waste tires. It is estimated that about 1.5 billion new tires are produced continuously globally, with about two-thirds of the tires reaching the end of their service life (Mohajerani et al., 2020). In addition to the waste tire itself as a pollutant, during the operation of vehicles, tires continuously wear and produce small black rubber particles. These black rubber particle pollutants can enter the soil through atmospheric deposition and rainwater runoff. Due to the small size of these rubber particles, they are more likely to diffuse and migrate in soil, changing the internal structure of the soil and affecting its physical properties significantly. Ahmed and Faizan (2024) comprehensively investigated the basic properties of waste tire rubber powder through various characterization techniques, including moisture content, specific gravity, physical structure, elemental composition, thermal stability, crystallization properties, functional groups and covalent bonds, which provides a valuable basis for further in-depth study of tire powder.
In order to reduce the environmental pollution of waste tires, many investigators have done in-depth research on the recycling of waste tires (Alsheyab et al., 2023; AbdelAleem and Hassan, 2022; Alaloul et al., 2020; Khan and Faizan, 2023; Naseem et al., 2019; Saad et al., 2024). However, these researches mainly focus on the influence of TRPC on the physical and mechanical properties of composite materials. To date, the impact of TRPC on the basic physical properties of soil has not been widely studied, especially the influence of tire powder content on soil resistivity, thermal conductivity, and ultrasonic wave velocity. Soil resistivity values have important applications in predicting soil moisture content, soil salinity, compaction, saturation, and the degree of freezing and thawing (Gunnink and El-Jayyousi, 1993; Kalinski and Kelly, 1993; McCarter, 1984; Shea and Luthin, 1961). Thermal conductivity modifies soil-atmosphere heat exchange and temperature, subsequently impacting plant growth and microbial activity, is directly related to agricultural production (Jia et al., 2019; Dong et al., 2015). Ultrasonic velocity, as a non-destructive exploration technology, will directly affect the soil assessment, including soil stability and soil structure (Huang et al., 2015; Xu and Shi, 2004; Xu et al., 2015).
Therefore, this study aims to explore the impact of TRPC on the basic physical properties of loess. This study investigates the thermal insulation performance, electrical conductivity, and ultrasonic wave velocity of the soil with different TRPC content and different moisture content, analyzes the impact of the TRPC itself on the physical properties of the mixed soil, discusses the mechanism of changing the microstructure of the soil, and thus explains the reasons for the changes in thermal conductivity, resistivity, and ultrasonic wave velocity of the mixed soil. It is hoped that these studies can provide valuable references for geophysical analysis, agricultural irrigation, ultrasonic non-destructive testing technology, and environmental protection, and raise awareness of TRPC-contaminated soil.
2 Sample preparation and experimental procedures
2.1 Sample preparation
The soil samples were collected from Quaternary loess in the suburbs of Xi’an City, Shaanxi Province. The particle size of TRPC is 150 mesh number. According to the geotechnical test method standard (GB/T 50123–2019), laboratory physical experiments on loess were carried out. The basic physical parameters of the soil are shown in Table 1.
The dried loess was ground into a grinder to a size of smaller than 2 mm. Then the loess powder was dried in an oven at 110°C for 24 h. The TRPC powder was dried in an oven at 40°C for 24 h. Then, both of them were sealed with plastic wrap and cooled to room temperature. High-temperature drying and plastic wrap sealing were both to eliminate the influence of natural moisture content on the experiment (Li et al., 2022). The RO-treated water was used in this experiment, which can eliminate the influence of impurities in water on the experiment. Firstly, the corresponding dried soil powder, purified water, dried TRPC were weighed according to the experimental design. Then, the loess powder and TRPC were mixed uniformity, corresponding purified water was added and intensely stirred. The mixtures then were sealed in sealable bags and were stored in a laboratory glassware dryer. After 24 h storage at room temperature, soil samples were compacted using a hydraulic jack. Then, the prepared samples were sealed with multiple layers of cling film and were placed in a glassware dryer for testing (Sun and Lü, 2019).
This experiment aimed to study the influence of TRPC on the physical properties of loess with different moisture contents. Six groups (0%, 0.5%, 1%, 2%, 3%, 4%) of TRPC contents and five groups (6%, 10%, 14%, 18%, 22%) of moisture contents were set up respectively. The dry density of all samples was 1.45 g/cm3. The samples were made into cylinders with a hydraulic jack and the size of all samples was 39.1 mm × 46 mm (diam × h). According to the design, 30 groups soil samples were prepared, with two parallel samples in each group, for a total of 60 soil samples, which are listed in Table 2. Among them, the TRPC content refers to the ratio of the weight of TRPC to the weight of dried soil sample. During the sample preparation process, the mass error of each cylindrical sample must be controlled within the range of ±0.1%, and the height error of each cylindrical sample must be controlled within the range of ±0.5%. The sample preparation process needs to be operated carefully to ensure the quality and precision of the samples (Lv et al., 2024).
2.2 Experimental process
The experimental process of this study is showed Figure 1. Based on the quick and accurate measurement of the transient plane source method (TPS), it has now become a relatively common method for measuring thermal conductivity (Al-Ajlan, 2006; Wang et al., 2020). In this experiment, the thermal conductivity meter of model TC3000E in the XIATECH series was used to measure the thermal conductivity at room temperature. The LCR digital bridge tester is based on the principle of the automatic balance bridge method and combines modern digital technology to achieve fast, high-precision, and high-stability resistance value measurement. In this experiment, the TH2816A wideband LCR digital bridge measuring instrument was used to measure the resistance of soil samples. The resistivity was calculated by Formula (1) based on the resistance value at an excitation frequency of 100 kHz. The ultrasonic detector evaluates the internal structure of the material by emitting and receiving ultrasonic waves and analyzing the propagation speed of waves in the material. In this experiment, the RSM-SY6(C) non-metallic acoustic detector of Zhongyan Technology was used to detect the wave velocity of soil samples and analyzed the difference between them. For each soil sample, all the above measurements were performed at least three times, and the average value was used to the final value of the corresponding sample.
Where ρ is the resistivity (Ω·m) of the tested loess sample, Rv is the resistance (Ω) of the loess sample, S is the cross-sectional area (m2) of the sample, and L is the height (m) of the sample.
3 Experimental results and analysis
3.1 The influence of TRBC content on loess resistivity under different moisture contents
Figure 2 demonstrated a pronounced upward trend in soil resistivity with increasing TRPC content, indicating that TRPC inclusion impeded current conduction in the soil. As shown in Figure 2, when the moisture content was 22%, 18%, and 14%, the resistivity of the soil samples remained unchanged, indicating that the addition of TRPC has a negligible effect on the resistivity under higher moisture content. When the moisture content was 10%, the resistivity showed a slightly increasing trend with the increase of TRPC. And the resistivity of TRPC content at 2%, 3%, and 4% levels was 1.11 times, 1.56 times, and 1.82 times that of the unadded TRPC, respectively. When the moisture content was 6%, the resistivity remained unchanged at first and then increased significantly with the increase of TRPC. And the resistivity of TRPC content at 2% and 3% was 2.03 times and 2.82 times that of the unadded TRPC, respectively. Moreover, when the TRPC content increased to 4%, the resistivity was as high as 1,329.68 Ω m, which was 3.81 times that of the sample without TRPC.
From Figure 3, it can be seen that the resistivity decreased as the moisture content increased, indicating that the increase in moisture content was beneficial for the conduction of electricity. And the resistivity showed a rapid decrease trend followed by a slow decrease as the moisture content increased. As shown in Figure 3, when the moisture content increased from 6% to 10%, the resistivity decreased by 50%, 39.6%, 44.6%, 72.6%, 72.4%, and 76.1% respectively with the increase of TRPC. And during this stage, there was a great decline. When the moisture content increased from 10% to 14%, the resistivity decreased by an average of 48.3%. When the moisture content increased from 14% to 18%, the resistivity decreased by an average of 35.6%. And the resistivity decreased by an average of 18.4% when the moisture content increased from 18% to 22%. The reduction in resistivity became smaller gradually as the moisture content increased, which was similar to the research results of Zhang et al. (2014), Lyu et al. (2019).
3.2 The influence of TRPC content on thermal conductivity of loess under different moisture contents
As demonstrated in Figure 4, the thermal conductivity of the soil sample decreased steadily and slowly with the increase of TRPC content from the overall perspective, indicating that the addition of TRPC improved the insulation performance of the soil.
When the content of TRPC increased to 4%, the thermal conductivity decreased by 42.4%, 31.6%, 21.1%, 14.7%, and 8.0% with the moisture content increasing, indicating that the influence of TRPC on thermal conductivity was related to moisture content. The relationship between thermal conductivity and TRPC content were fitted as in Figure 4, and the fitting equations for thermal conductivity and TRPC content at different moisture contents were shown in Table 3. And the correlation coefficients R2 were high, indicating a high fitting degree. From the slope coefficient of the fitting equations, it can be seen that the influence of TRPC content on thermal conductivity linearly decreased with the increasing moisture content.
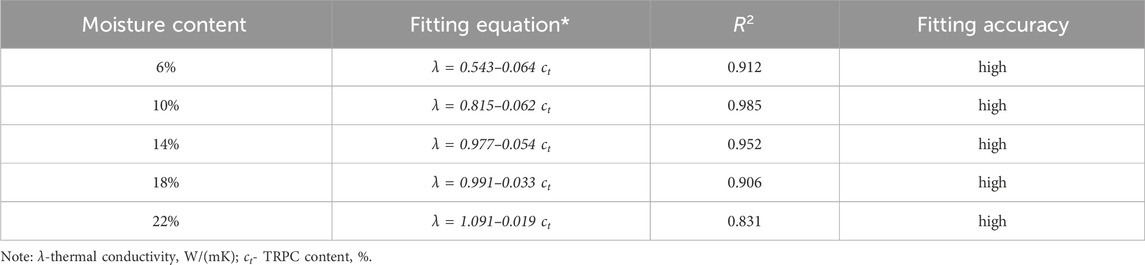
Table 3. The fitting relationship between thermal conductivity and TRPC content under different moisture content.
As illustrated in Figure 5, the thermal conductivity of the soil increased gradually with the increase of moisture content. When moisture content increased from 6% to 10%, the thermal conductivity increased by an average of 68.6%. When the moisture content increased from 10% to 18%, the thermal conductivity increased by an average of 33.2%. When the moisture content increased from 18% to 22%, the thermal conductivity increased by an average of 12.9%. It can be concluded that the influence of moisture content on thermal conductivity decreased gradually.
Taking moisture content and TRPC content as independent variables and thermal conductivity as the dependent variable for linear regression analysis, the regression equation was obtained as Formula (2).
where λ is the thermal conductivity, W/(m·K), w is the moisture content, and ct is the TRPC content. The value of R2 was 0.912, indicating a very high degree of fitting. The regression coefficient of moisture content was 3.669, indicating that moisture content had a significant positive impact on thermal conductivity. The regression coefficient of TRPC content was −4.589, indicating that TRPC content had a significant negative impact on thermal conductivity. This regression equation could provide reference value for the prediction of the thermal conductivity of soil in TRPC-contaminated areas.
3.3 The influence of TRPC content on ultrasonic wave of loess under different moisture contents
As shown in Figure 6, the ultrasonic velocity decreased with the increase of TRPC content overall. When the TRPC content increased to 4%, the decrease in velocity was 500 m/s, 550 m/s, 333 m/s, 133 m/s, and 126 m/s, respectively. When the moisture content was 6%, the wave velocity dropped sharply as the TRPC content increased to 2% and the decrease was 345 m/s, accounting for 69% of the total decrease. Then the wave velocity remained stable. When the moisture content was 10%, the wave velocity showed a slowly decreasing trend firstly, then rapidly decreasing, and finally slowly decreasing as the TRPC content increased. And there was a sharp decrease when TRPC content increased to 3%, accounting for 50% of the total decrease. When the water content was 14%, 18%, and 22%, the wave velocity showed a steadily and slowly decreasing trend. Therefore, the addition of TRPC blocked the propagation of ultrasonic wave overall, and the proportion of TRPC were negatively correlated the wave velocity.
From Figure 7, it can be seen that the ultrasonic wave velocity showed an increasing trend first and then decreased with the increasing moisture content. This indicated that the increasing moisture gradually changed the internal structure of the soil sample, thereby changing the propagation speed of ultrasonic wave. When the TRPC content was 0%, the wave velocity decreased steadily with the increase of moisture content, with a decrease of 191.5 m/s. When the TRPC content was 0.5%, 1%, the wave velocity increased slightly first and then steadily decreased with the increase of moisture content, and the turning point was 10% moisture content. When the TRPC content was 2%, 3%, the wave velocity increased rapidly first and then steadily decreased with the increase of moisture content, and the turning point was 14% moisture content. When the TRPC content was 4%, the wave velocity decreased slowly first and then increased rapidly and then steadily decreased with the increase of moisture content, and the turning point was 18% moisture content. It can be concluded that the influence degree of moisture content on ultrasonic wave velocity was closely related to the TRPC content.
4 Discussion
4.1 The influence of TRPC on resistivity, thermal conductivity, and wave velocity of loess
4.1.1 The influence of TRPC on electrical resistivity of loess
In three-phase soil containing TRPC, both TRPC and air are insulators, the content and connectivity of pore water determine the soil’s conductivity (Jiang et al., 2017). In soil containing TRPC, there are three types of electrical conduction pathways: double electric layer between soil particles; the solid-liquid path (double electric layer and water); and the continuous liquid water path (Lv et al., 2024).
As shown in Figure 8, when the moisture content of the mixed soil was very low, the addition of TRPC with high resistance and hydrophobic action blocked the double-layer conduction between soil particles. And the greater the content of TRPC, the greater the degree of impeding, leading more complex electrical conduction pathways within the soil sample. On the other hand, the addition of TRPC with equal weight but less specific gravity increased the compactness of the soil sample, which enhanced the connection between soil particles, thus facilitating the conduction of electricity (Shan et al., 2015). When the two sides are in balance, the resistivity remains constant. When the impeding factor predominates, the resistivity increases. This theory can explain some of the experimental results in Figure 2. When the moisture content was 6%, the balance state rapidly shifted to the state where the impeding factor dominated, and the resistivity increased rapidly and significantly. When the moisture content was 10%, the impeding factor gradually became dominant, and the resistivity increased steadily and slowly.
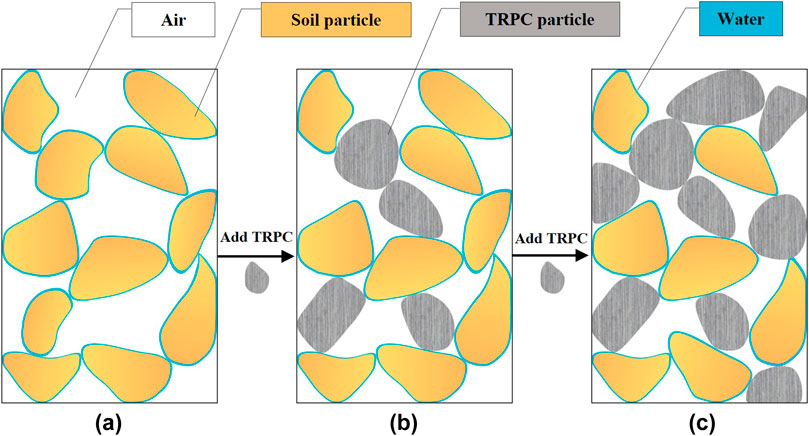
Figure 8. Effect of TRPC on soil internal structure with low moisture content. (a) No TRPC particle. (b) A few TRPC particles. (c) Many TRPC particles.
As shown in Figure 9, when the moisture content of the mixed soil was high, the water in the soil sample was well connected, leading to good water electrical conduction paths. The addition of TRPC did not disrupt the water connectivity, but enhanced the water connectivity due to its less specific gravity. Therefore, the addition of TRPC had a minimal impact on soil’s resistivity under high moisture contents. As shown by the experimental results in Figure 2, when the moisture content was greater than 14%, the electrical resistivity increased slightly with the increase of TRPC, which verified the above theoretical analysis (Datsios et al., 2017).
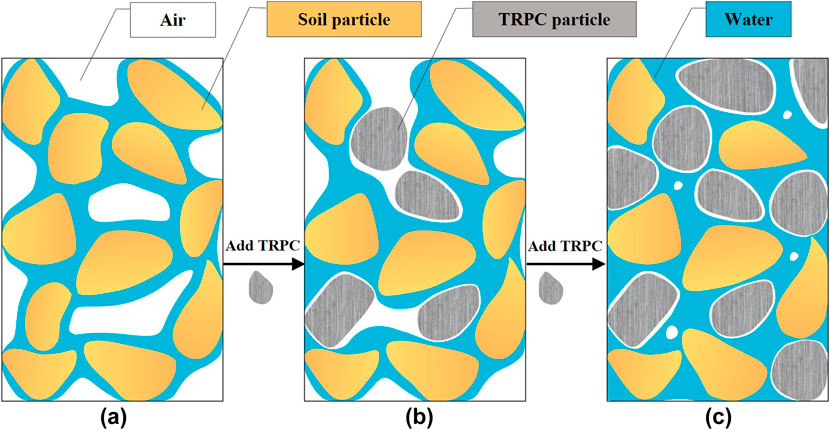
Figure 9. Effect of TRPC on soil internal structure with high moisture content. (a) No TRPC particle. (b) A few TRPC particles. (c) Many TRPC particles.
4.1.2 The influence of TRPC on thermal conductivity of loess
The soil’s three-phase structure determines that heat in soil can be conducted through solid particles, free water, bound water adsorbed on solid particles, and air (Shiozawa and Campbell, 1990). The thermal conductivity of different phases varies greatly, with orders of magnitude differences. The thermal conductivity of water is approximately 0.599 W/(m·K) at room temperature, the thermal conductivity of air is approximately 0.026 W/(m·K), and the thermal conductivity of soil minerals is greater than 3 W/(m·K), thus many scholars established heat transfer models through the proportion of phases (Brandon and Mitchell, 1989; Yun and Santamarina, 2008). From the above data and related studies, it can be seen that air hinders heat conduction, and heat conduction in soil mainly occurs through particles, and solid minerals are the most favorable heat-conducting component in the soil system (Gangadhara Rao and Singh, 1999; Tarnawski et al., 2002; Xiao et al., 2008).
The main component of TRPC is rubber, and the thermal conductivity range of rubber is 0.19–0.26 W/(m·K), which is much smaller than the thermal conductivity of soil minerals. Therefore, the addition of TRPC could reduce the heat conductivity of the mixed soil. On the other hand, the specific gravity of TRPC is approximately 0.42 (Olofinnade and Adeyinka, 2024), which is much smaller than the specific gravity of soil particles. So, adding TRPC with equal weight but less specific gravity could make soil sample denser, and the porosity of soil sample decreased with the increasing TRPC, as shown in Figures 8, 9. The decrease in porosity means that the volume of the least favorable heat-conducting air reduced, which is conducive to heat conduction in the soil sample (Côté and Konrad, 2005; Abu-Hamdeh and Reeder, 2000). Therefore, when the factor that is favorable to heat conduction dominates, the thermal conductivity of the soil sample shows an overall upward trend. On the contrary, when the factor that hinders the heat conduction dominates, the thermal conductivity of the soil sample decreases. When favorable factors and hindering factors reach the equilibrium state, the thermal conductivity is basically unchanged. As can be seen from Figure 4, the factors that hinder heat conduction occupied a dominant position, thus the thermal conductivity of the mixed soil showed an overall decreasing trend with the increase of TRPC content. In addition, the increase of the moisture content can weaken the influence of TRPC on the thermal conductivity, leading to the different reduction range of thermal conductivity in Figure 4.
4.1.3 The influence of TRPC on wave velocity of loess
The addition of TRPC changed the internal skeleton structure of the soil sample. As shown in Figure 8, when the moisture content was low, the primary propagation path of ultrasonic wave in the soil sample changed progressively from “soil particle + air” to “soil particle + air + TRPC particle.” As shown in Figure 9, when the moisture content was high, the primary propagation path of ultrasonic wave in the soil sample changed from the initial “soil particle + air + water” to “soil particle + air + water + TRPC particle,” and finally to “soil particle + water + TRPC particle.”
On one hand, due to the different acoustic impedance of each component, wave reflection and transmission produce at the interface when ultrasonic wave pass through the interface, and the transmitted wave would appear scattering, interference and diffraction, leading the propagation path of the ultrasonic wave in the mixed soil more complicated with increasing TRPC, resulting in a decrease in wave velocity (Song et al., 2019). In addition, the hydrophobicity of TRPC hindered the formation and connection of the soil aggregates, making a more complicated propagation path of wave in the soil sample, resulting a decrease of propagation (Olofinnade and Adeyinka, 2024). On the other hand, since the propagation speed of ultrasonic wave in air is about 340 m/s, which is much lower than that in solids and liquids, the addition of TRPC with equal weight but smaller specific gravity reduces the proportion of air and thus increases the wave velocity in the mixed soil (Olofinnade and Adeyinka, 2024). Therefore, when the preventing factors dominated, the ultrasonic wave speed decreased. Conversely, when the promoting factors dominated, the ultrasonic wave speed increased.
From Figure 6, the addition of TRPC hindered the propagation of ultrasonic wave. When the moisture content was 6%, the ultrasonic wave velocity dropped sharply when the content of TRPC was 2%, and the effect of hindering the wave conduction took an absolutely dominant position. When the moisture content was 10%, the ultrasonic wave velocity dropped sharply when the content of TRPC was 3%, and the effect of hindering the wave conduction took an absolutely dominant position. With the increase of the moisture content, the ultrasonic wave velocity showed a slow and stable downward trend with the addition of TRPC. This conclusion was consistent with the conclusion obtained by Song Kun. Song et al. (2019) have shown that the proportion of lump rocks in soil-rock mixed soil was negatively correlated with sound velocity.
4.2 The influence of moisture content on resistivity, thermal conductivity, and wave velocity of loess
4.2.1 The influence of moisture content on the resistivity of loess
As shown in Figure 10, with the increase of moisture content, the form of water in the soil gradually changes from initial bound water to a combination of bound water and capillary water, and finally to free water. As shown in Figure 10a, when the moisture content was low (such as the experimental moisture content of 6%), the soil particles adsorbed water molecules to form double electric layer. For loess containing clay particles, the double electric layer formed by the interaction between soil particles and water played a crucial role in soil conduction (Hasan et al., 2021). Therefore, the double electric layer structure was the main electrical conduction path in the soil sample in the case of low moisture content. As shown in Figure 10b, with the increase of moisture content (such as the experimental moisture content of 10%), the thickness of the double electric layer gradually increased, enhancing the contact between soil particles and promoting the conduction of electric current, resulting in a decrease in soil resistivity (Hasan et al., 2021). During this time, the change of moisture had a great influence on the resistivity of soil sample. As shown in Figure 10b, capillary water began to exist and the water connectivity further increased with the increasing moisture, resulting in a further decrease in soil resistivity. The trend of soil resistivity change slowed down is due to the stronger conductivity of adsorbed water compared with capillary water (Mojid et al., 2007). As shown in Figure 10c, when the moisture content reached 18% or above, most of the pores in soil sample were filled with free water, and connected conductive paths were formed in soil. The resistivity decreased at a smaller rate because the conductivity of gravity water was lower than that of adsorbed water and capillary water (Bery and Ismail, 2018; Fagbemigun et al., 2021; Pozdnyakov et al., 2006). In addition, as shown in Figure 10, the saturation of the soil sample increased with the increasing moisture content, and the air obstructing current conduction decreased with the increasing saturation, leading more smoother conduction path of current, thus reducing the soil resistivity (Vincent et al., 2021). Therefore, the soil resistivity decreased rapidly with increasing moisture content at first, and then remained stabilized.
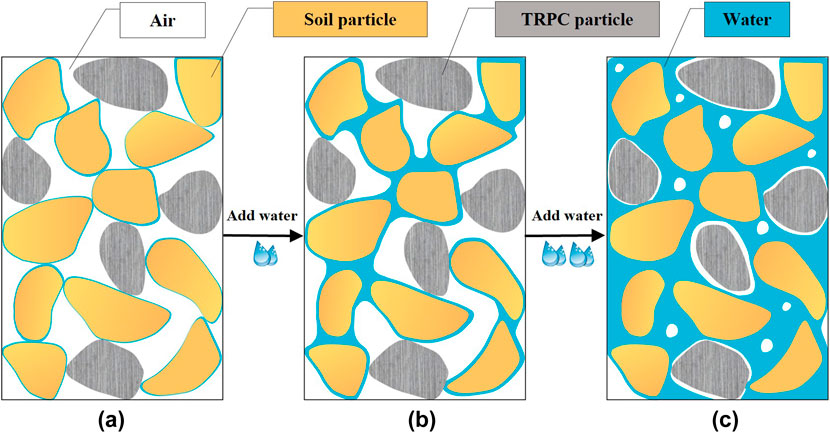
Figure 10. Effect of moisture content on soil internal structure. (a) Thin bound water. (b) Thick bound water and capillary water. (c) Connected free water.
4.2.2 The influence of moisture content on thermal conductivity
The thermal conductivity of the three-phase structure of the soil sample is: solid phase > liquid phase > gas phase. When the dry density of the soil sample remained constant, the saturation of soil increased with the increasing moisture content, leading the reduction of air that impeded heat conduction, thus improving the soil sample’s thermal conductivity (Côté and Konrad, 2005). As shown in Figure 10, the thickness of the bound water increased with the moisture content increasing, and the pores were gradually filled with capillary and free water. The water molecules in the bound water film are oriented and arranged in a line around the surface of the soil particle. They do not have the properties of ordinary water and their properties are close to solid, while the properties of capillary water and free water are close to ordinary water (Li et al., 2017). Therefore, the thickness of bound water increased significantly in the early stage of the increase in moisture content, and the increase in thermal conductivity was large in this stage. The increase rate of thickness of bound water slowed down as the moisture content increased to a certain extent, and the increase in thermal conductivity also slowed down in this stage. When the thickness of bound water no longer increased, free water began to fill the pores with the increasing moisture content. Since the density of free water is lower than that of bound water, free water of the same weight can reduce the porosity more effectively than bound water, and expel more air that hinders heat conduction. Therefore, the increase rate of the thermal conductivity was enhanced when the water content was greater than 18%. The above analysis can explain the experimental data in Figure 5.
4.2.3 The influence of moisture content on ultrasonic velocity
The ultrasonic velocity of each component in the soil sample is: minerals > bound water > free water > air. When the moisture content was very low, the bound water film around the soil particles was very thin, and the soil particles were mainly connected in an interlocking manner, as shown in Figure 10a. As the moisture content increased gradually, the thickness of the bound water film increased, and the connection type of soil particles changed from interlocking to cementation, as shown in Figure 10b, which was not conducive to wave propagation. On the other hand, the saturation of soil increased with the increasing moisture content, reducing the amount of air that impeded wave propagation, promoting wave propagation. Therefore, when the effect of promoting wave propagation was dominant, the wave propagation velocity increased; conversely, when the effect of preventing the wave propagation was dominant, the wave propagation velocity decreased (Li et al., 2017).
As can be seen from Figures 6, 7, when the moisture content was low, the wave velocity was sensitive to the change of moisture content, indicating that the internal structure of soil changed greatly with the change of moisture content. On the contrary, when the moisture content was high, the wave velocity was not sensitive to the change of moisture content, indicating that the internal structure of soil tended to be relatively stable.
4.3 The relationship between resistivity, thermal conductivity and ultrasonic velocity of loess
In summary, with the increase of TRPC, the resistivity of loess showed an overall upward trend, the thermal conductivity of loess showed an overall downward trend, and the ultrasonic velocity of loess showed an overall downward trend. In other words, the addition of TRPC was not conducive to the conduction of electric current, the transfer of heat, and the propagation of ultrasonic wave. Figure 11 showed the changes of thermal conductivity, resistivity, and ultrasonic velocity with TRPC content when the moisture content was 10%. The influence of TRPC on soil physical properties can be explained from two aspects: (1) the physical properties of TRPC itself, (2) the internal microstructure of the soil changed by the addition of TRPC. The combined effect of these two aspects changed the relevant physical properties of the soil.
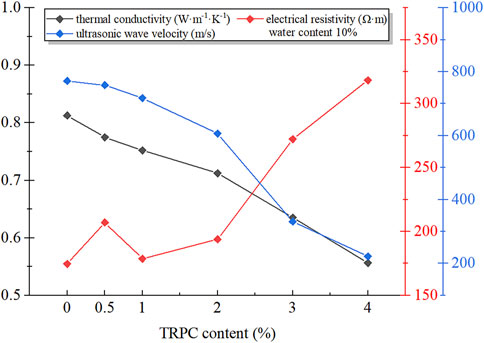
Figure 11. Variation of thermal conductivity, electrical resistivity and ultrasonic wave velocity with TRPC content.
With the increase of moisture content, the resistivity of loess showed an overall downward trend, the thermal conductivity showed an overall upward trend, and the ultrasonic velocity showed a trend of first rising and then decreasing, which indicated that the increase of moisture content was conducive to the conduction of current and the transfer of heat. Figure 12 depicted the changes in thermal conductivity, resistivity, and ultrasonic wave velocity with moisture content when the TRPC content was 2%. The influence of moisture content on soil physical properties can be interpreted from two aspects: one was the physical properties of water itself, and the other was the changed microstructure of soil at varying water contents. The combined effect of these two aspects altered the relevant physical properties of the soil.
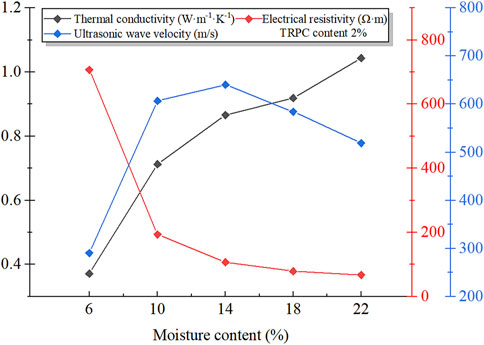
Figure 12. Variation of thermal conductivity, electrical resistivity and ultrasonic wave velocity with moisture content.
At present, there are a few studies on the effect of TRPC on soil physical properties. Most of the studies are limited to indoor tests, lacking field experiments. One single particle size and no field experiments were the main limitations of this study. In future research, in-depth investigations can be carried out from the following aspects: the effects of TRPC on different soil types, the influence of TRPC on soil under different climatic conditions, the effects of TRPC with different particle size, the long-term effects of TRPC on soil, and the mechanical properties of soil influenced by TRPC.
5 Conclusion
This paper took loess containing TRPC as the research subject and investigated the impact of TRPC content on the resistivity, thermal conductivity, and ultrasonic velocity of loess under different moisture content conditions. It explored the mechanism of the change of internal microstructure of the soil due to the addition of TRPC, explained the reasons for the changes in the macroscopic physical properties due to the alteration of the loess microstructure, and the conclusions can be drawn as follows.
(1) The resistivity of loess increased with the increase of TRPC content, especially when the water content was less than 14%. This was mainly because the addition of hydrophobic TRPC broke the connection between some soil particles, destroying the double electric layer structure current conduction path. And the disruption degree increased with increasing TRPC.
(2) The thermal conductivity of loess decreased with the increase of TRPC content. This was mainly because the thermal conductivity of TRPC was low than soil particles. The heat conduction path gradually changed from “soil particle + soil particle” to “soil particle + TRPC particle” with the increasing TRPC, so the thermal conductivity gradually decreased.
(3) The wave velocity decreased with the increase of TRPC content. This was mainly due to the interfacial effect. When ultrasonic waves propagated from one medium (soil particle) to another medium (TRPC), the waves would appear reflection, transmission, diffraction, and interference due to the different acoustic impedance, leading to more complicated propagation path and decreasing wave velocity.
(4) The increase of moisture content was beneficial to the electric conduction and heat transfer of loess. The wave velocity increased first and then decreased with the increase of moisture content. This was mainly related to the existence form of water in the soil, and closely related to the porosity of the soil sample.
Data availability statement
The raw data supporting the conclusion of this article will be made available by the authors, without undue reservation.
Author contributions
SL: Conceptualization, Data curation, Formal Analysis, Investigation, Project administration, Resources, Software, Validation, Visualization, Writing–original draft, Writing–review and editing. QS: Formal Analysis, Methodology, Supervision, Writing–review and editing. PM: Data curation, Investigation, Methodology, Writing–review and editing. RL: Data curation, Formal Analysis, Investigation, Writing–review and editing. HZ: Formal Analysis, Methodology, Supervision, Writing–review and editing. JN: Conceptualization, Funding acquisition, Supervision, Writing–review and editing.
Funding
The author(s) declare that financial support was received for the research and/or publication of this article. This research was supported by the National Natural Science Foundation of China (Program No. 42407268).
Conflict of interest
The authors declare that the research was conducted in the absence of any commercial or financial relationships that could be construed as a potential conflict of interest.
Generative AI statement
The author(s) declare that no Generative AI was used in the creation of this manuscript.
Publisher’s note
All claims expressed in this article are solely those of the authors and do not necessarily represent those of their affiliated organizations, or those of the publisher, the editors and the reviewers. Any product that may be evaluated in this article, or claim that may be made by its manufacturer, is not guaranteed or endorsed by the publisher.
References
AbdelAleem, B. H., and Hassan, A. A. (2022). Use of rubberized engineered cementitious composite in strengthening flexural concrete beams. Eng. Struct. 262, 114304. doi:10.1016/j.engstruct.2022.114304
Abu-Hamdeh, N. H., and Reeder, R. C. (2000). Soil thermal conductivity effects of density, moisture, salt concentration, and organic matter. Soil Sci. Soc. Am. J. 64 (4), 1285–1290. doi:10.2136/sssaj2000.6441285x
Ahmed, K. Z., and Faizan, M. (2024). Comprehensive characterization of waste tire rubber powder. J. Inst. Eng. India Ser. E105, 11–20. doi:10.1007/s40034-023-00282-8
Al-Ajlan, S. A. (2006). Measurements of thermal properties of insulation materials by using transient plane source technique. Appl. Therm. Eng. 26 (17-18), 2184–2191. doi:10.1016/j.applthermaleng.2006.04.006
Alaloul, W. S., Musarat, M. A., Tayeh, B. A., Sivalingam, S., Rosli, M. F. B., Haruna, S., et al. (2020). Mechanical and deformation properties of rubberized engineered cementitious composite (ECC). Case Stud. Constr. Mater. 13, e00385. doi:10.1016/j.cscm.2020.e00385
Alsheyab, M. A., Khedaywi, T., and Ogiliat, O. (2023). Effect of waste tire rubber on properties of asphalt cement and asphalt concrete mixtures: state of the art. Int. J. Pavement Res. Technol. 18, 516–527. doi:10.1007/s42947-023-00361-4
Bery, A. A., and Ismail, N. E. H. (2018). Empirical correlation between electrical resistivity and engineering properties of soils. Soil Mech. Found. Eng. 54, 425–429. doi:10.1007/s11204-018-9491-7
Brandon, T. L., and Mitchell, J. K. (1989). Factors influencing thermal resistivity of sands. J. Geotech. Eng. 115 (12), 1683–1698. doi:10.1061/(asce)0733-9410(1989)115:12(1683)
Côté, J., and Konrad, J. M. (2005). A generalized thermal conductivity model for soils and construction materials. Can. Geotech. J. 42 (2), 443–458. doi:10.1139/t04-106
Datsios, Z. G., Mikropoulos, P. N., and Karakousis, I. (2017). Laboratory characterization and modeling of DC electrical resistivity of sandy soil with variable water resistivity and content. IET Electr. Insul. 24 (5), 3063–3072. doi:10.1109/TDEI.2017.006583
Dong, Y., McCartney, J. S., and Lu, N. (2015). Critical review of thermal conductivity models for unsaturated soils. Geotech. Geol. Eng. 33, 207–221. doi:10.1007/s10706-015-9843-2
Fagbemigun, S. T., Oyebamiji, R. A., Faloyo, I. J., Arowoogun, I. K., Amosun, O. J., and Sanuade, A. O. (2021). Integration of electrical resistivity and soil analysis for Agricultural soil characterization—a case study. Arab. J. Geosci. 14, 377. doi:10.1007/s12517-021-06772-6
Gangadhara Rao, M. V. B. B., and Singh, D. N. (1999). A generalized relationship to estimate thermal resistivity of soils. Can. Geotech. J. 36 (4), 767–773. doi:10.1139/t99-037
Gunnink, B. W., and El-Jayyousi, J. (1993). Soil-fabric measurement using phase transition porosimetry. J. Geotech. Eng. 119 (6), 1019–1036. doi:10.1061/(ASCE)0733-9410(1993)119:6(1019)
Hanif, M. N., Aijaz, N., Azam, K., Akhtar, M., Laftah, W. A., Babur, M., et al. (2024). Impact of microplastics on soil (physical and chemical) properties, soil biological properties/soil biota, and response of plants to it: a review. Int. J. Environ. Sci. Technol. 21, 10277–10318. doi:10.1007/s13762-024-05656-y
Hasan, M. F., Abuel-Naga, H., and Leong, E. C. (2021). A modified series-parallel electrical resistivity model of saturated sand/clay mixture. Eng. Geol. 290, 106193. doi:10.1016/j.enggeo.2021.106193
Hidalgo, J., Artetxe, U., Becerril, J. M., Gómez-Sagasti, M. T., Epelde, L., Vilela, J., et al. (2024). Biological remediation treatments improve the health of a mixed contaminated soil before significantly reducing contaminant levels. Environ. Sci. Pollut. Res. 31 (4), 6010–6024. doi:10.1007/s11356-023-31550-0
Huang, X., Li, D. Q., Ming, F., Bing, H., and Peng, W. W. (2015). Experimental study on acoustic characteristics and physico-mechanical properties of frozen silty clay. Chin. J. Rock Mech. Eng. 34 (7), 1489–1496. (in Chinese with English abstract). doi:10.13722/j.cnki.jrme.2014.1248
Jia, G. S., Tao, Z. Y., Meng, X. Z., Ma, C. F., Chai, J. C., and Jin, L. W. (2019). Review of effective thermal conductivity models of rock-soil for geothermal energy applications. Geothermics 77, 1–11. doi:10.1016/j.geothermics.2018.08.001
Jiang, Y., Chen, W., Wang, G., Sun, G., and Zhang, F. (2017). Influence of initial dry density and water content on the soil–water characteristic curve and suction stress of a reconstituted loess soil. Bull. Eng. Geol. Environ. 76, 1085–1095. doi:10.1007/s10064-016-0899-x
Kalinski, R. J., and Kelly, W. E. (1993). Estimating water content of soils from electrical resistivity. Geotech. Test. J. 16 (3), 323–329. doi:10.1520/GTJ10053J
Khan, Z. A., and Faizan, M. (2023). Mixing strategy and tensile strength characterization of WTR-PP composite. Int. J. Polym. Anal. Charact. 28 (5), 407–418. doi:10.1080/1023666X.2023.2238439
Kwon, J. H., Ji, M. K., Kumar, R., Islam, M. M., Khan, M. A., Park, Y. K., et al. (2023). Recent advancement in enhanced soil flushing for remediation of petroleum hydrocarbon-contaminated soil: a state-of-the-art review. Rev. Environ. Sci. Bio/Technol. 22 (3), 679–714. doi:10.1007/s11157-023-09657-0
Li, J., Xu, Y., Jiang, R., Yang, Z., and Lu, H. (2017). Establishment and verification of model for ultrasonic soil water content detector. Trans. Chin. Soc. Agric. Eng. 33 (13), 127–133. (in Chinese with English abstract). doi:10.11975/j.issn.1002-6819.2017.13.017
Li, P., Sun, Q., Geng, J., Yan, X., and Tang, L. (2022). Radon exhalation from temperature treated loess. Sci. Total Environ. 832, 154925. doi:10.1016/j.scitotenv.2022.154925
Lv, R., Sun, Q., Li, P., Geng, J., Xin, Y., and Wang, S. (2024). Effect of different water and organic matter content on the resistivity of loess. Sci. Total Environ. 906, 167624. doi:10.1016/j.scitotenv.2023.167624
Lyu, C., Sun, Q., Zhang, W., and Hao, S. (2019). Effects of NaCl concentration on electrical resistivity of clay with cooling. J. Appl. Geophys. 170, 103843. doi:10.1016/j.jappgeo.2019.103843
McCarter, W. J. (1984). The electrical resistivity characteristics of compacted clays. Geotechnique 34 (2), 263–267. doi:10.1680/geot.1984.34.2.263
Mendes, G. P., Soares, L. C. D. R., Viegas, R. M. A., Chiavone-Filho, O., and do Nascimento, C. A. O. (2024). Lead (Pb) in shooting range soil: a systematic literature review of contaminant behavior, risk assessment, and remediation options. Water Air Soil Pollut. 235 (1), 1. doi:10.1007/s11270-023-06783-x
Mohajerani, A., Burnett, L., Smith, J. V., Markovski, S., Rodwell, G., Rahman, M. T., et al. (2020). Recycling waste rubber tyres in construction materials and associated environmental considerations: a review. Resour. Conserv. Recycl. 155, 104679. doi:10.1016/j.resconrec.2020.104679
Mojid, M. A., Rose, D. A., and Wyseure, G. C. L. (2007). A model incorporating the diffuse double layer to predict the electrical conductivity of bulk soil. Eur. J. Soil Sci. 58 (3), 560–572. doi:10.1111/j.1365-2389.2006.00831.x
Münzel, T., Hahad, O., Lelieveld, J., Aschner, M., Nieuwenhuijsen, M. J., Landrigan, P. J., et al. (2024). Soil and water pollution and cardiovascular disease. Nat. Rev. Cardiol. 22, 71–89. doi:10.1038/s41569-024-01068-0
Naseem, A., Mumtaz, W., and De Backer, H. (2019). Stabilization of expansive soil using tire rubber powder and cement kiln dust. Soil Mech. Found. Eng. 56, 54–58. doi:10.1007/s11204-019-09569-8
Olofinnade, O., and Adeyinka, O. (2024). The utilization of pulverized waste tire rubber in a soil–cement composite for sustainable compressed earth brick production. Discov. Civ. Eng. 1 (1), 69. doi:10.1007/s44290-024-00075-x
Pozdnyakov, A. I., Pozdnyakova, L. A., and Karpachevskii, L. O. (2006). Relationship between water tension and electrical resistivity in soils. Eur. Soil Sci. 39, S78–S83. doi:10.1134/S1064229306130138
Saad, A. G., Sakr, M. A., Khalifa, T. M., and Darwish, E. A. (2024). Structural performance of concrete reinforced with crumb rubber: a review of current research. Iran. J. Sci. Technol. Trans. Civ. Eng., 1–44. doi:10.1007/s40996-024-01629-w
Shan, W., Liu, Y., Hu, Z., and Xiao, J. (2015). A model for the electrical resistivity of frozen soils and an experimental verification of the model. Cold Reg. Sci. Technol. 119, 75–83. doi:10.1016/j.coldregions.2015.07.010
Sharma, S., Pathania, S., Bhagta, S., Kaushal, N., Bhardwaj, S., Bhatia, R. K., et al. (2024). Microbial remediation of polluted environment by using recombinant E. coli: a review. Biotechnol. Environ. 1 (1), 8. doi:10.1186/s44314-024-00008-z
Shea, P. F., and Luthin, J. N. (1961). An investigation of the use of the four-electrode probe for measuring soil salinity in situ. Soil Sci. 92 (5), 331–339. doi:10.1097/00010694-196111000-00006
Shiozawa, S., and Campbell, G. S. (1990). Soil thermal conductivity. Remote Sens. Rev. 5 (1), 301–310. doi:10.1080/02757259009532137
Simpson, M. J. (2023). Application of metabolomic methods to evaluate the impact of pollutants on soil organisms: recent progress and future perspectives. Curr. Opin. Environ. Sci. Health. 31, 100431. doi:10.1016/j.coesh.2022.100431
Solís-Ramos, L. Y., Coto-López, C., and Andrade-Torres, A. (2021). Role of arbuscular mycorrhizal symbiosis in remediation of anthropogenic soil pollution. Symbiosis 84 (3), 321–336. doi:10.1007/S13199-021-00774-4
Song, K., Zhao, Z., Ma, P., Feng, R., Meng, S., and Yang, Y. (2019). Experiment of ultrasonic propagation characteristics based on farm soil. J. Agric. Mech. Res. 41 (08), 175–179+185. (in Chinese with English abstract). doi:10.13427/j.cnki.njyi.2019.08.030
Sun, Q., and Lü, C. (2019). Semiempirical correlation between thermal conductivity and electrical resistivity for silt and silty clay soils. Geophysics 84 (3), MR99–MR105. doi:10.1190/geo2018-0549.1
Tarnawski, V. R., Leong, W. H., Gori, F., Buchan, G. D., and Sundberg, J. (2002). Inter-particle contact heat transfer in soil systems at moderate temperatures. Int. J. Energy Res. 26 (15), 1345–1358. doi:10.1002/er.853
Vincent, N. A., Shivashankar, R., Lokesh, K. N., and Nath, D. (2021). Shrinkage limit studies from moisture content: electrical resistivity relationships of soils. Arab. J. Sci. Eng. 46, 4923–4940. doi:10.1007/s13369-020-05325-5
Wan, Y., Wu, C., Xue, Q., and Hui, X. (2019). Effects of plastic contamination on water evaporation and desiccation cracking in soil. Sci. Total Environ. 654, 576–582. doi:10.1016/j.scitotenv.2018.11.123
Wang, L., Yu, L., and Cai, B. (2024). Characteristics of tetracycline antibiotic resistance gene enrichment and migration in soil–plant system. Environ. Geochem. Health. 46 (11), 427. doi:10.1007/s10653-024-02239-1
Wang, S., Ai, Q., Zou, T. Q., Sun, C., and Xie, M. (2020). Analysis of radiation effect on thermal conductivity measurement of semi-transparent materials based on transient plane source method. Appl. Therm. Eng. 177, 115457. doi:10.1016/j.applthermaleng.2020.115457
Wu, M., Feng, S., Liu, Z., and Tang, S. (2024). Bioremediation of petroleum-contaminated soil based on both toxicity risk control and hydrocarbon removal—progress and prospect. Environ. Sci. Pollut. Res. 31, 59795–59818. doi:10.1007/s11356-024-34614-x
Xia, F., Zhao, Z., Niu, X., and Wang, Z. (2024). Integrated pollution analysis, pollution area identification and source apportionment of heavy metal contamination in agricultural soil. J. Hazard. Mater. 465, 133215. doi:10.1016/j.jhazmat.2023.133215
Xiao, L., Li, X., Zhao, X., Yu, J., Hu, Z., and Ji, C. (2008). Laboratory on influences of moisture content and porosity on thermal conductivity of soils. J. PLA Univ. Sci. Technol. 9 (3), 241–247. (in Chinese with English abstract). doi:10.7666/j.issn.1009-3443.20080308
Xu, C. J., and Shi, Y. Y. (2004). Characteristics of wave propagation in unsaturated soils. Rock Soil Mech. 25 (3), 354–358. (in Chinese with English abstract). doi:10.16285/j.rsm.2004.03.004
Xu, C. J., Xu, L. Y., and Yang, Y. Y. (2015). Effect of parameters of three-phase unsaturated soils on wave propagation. Rock Soil Mech. 36 (S2), 340–344. (in Chinese with English abstract). doi:10.16285/j.rsm.2015.S2.046
Xu, Z., Jia, Y., Huang, B., Zhao, D., Long, X., Hu, S., et al. (2023). Spatial distribution, pollution characteristics, and health risks of antibiotic resistance genes in China: a review. Environ. Chem. Lett. 21 (4), 2285–2309. doi:10.1007/s10311-023-01605-2
Yu, D., Zeng, S., Wu, Y., Niu, J., Chen, K., Tian, H., et al. (2024). The remediation of organic pollution in soil by persulfate. Water Air Soil Pollut. 235 (11), 689. doi:10.1007/s11270-024-07501-x
Yun, T. S., and Santamarina, J. C. (2008). Fundamental study of thermal conduction in dry soils. Granul. Matter. 10, 197–207. doi:10.1007/s10035-007-0051-5
Zeng, S., Ma, J., Yang, Y., Zhang, S., Liu, G. J., and Chen, F. (2019). Spatial assessment of farmland soil pollution and its potential human health risks in China. Sci. Total Environ. 687, 642–653. doi:10.1016/j.scitotenv.2019.05.291
Zhang, D., Cao, Z., Fan, L., Liu, S., and Liu, W. (2014). Evaluation of the influence of salt concentration on cement stabilized clay by electrical resistivity measurement method. Eng. Geol. 170, 80–88. doi:10.1016/j.enggeo.2013.12.010
Zhang, H. L., Zhao, P., Gao, W. Y., Xiao, B. H., Yang, X. F., Song, L., et al. (2024). Contaminant transport modelling of heavy metal pollutants in soil and groundwater: an example at a non-ferrous smelter site. J. Cent. South Univ. 31 (4), 1092–1106. doi:10.1007/s11771-024-5639-y
Keywords: soil pollution, tire rubber powder, electrical resistivity, thermal conductivity, ultrasonic velocity
Citation: Liu S, Sun Q, Ma P, Lv R, Zhang H and Nan J (2025) The impact of tire rubber powder contaminants on the physical properties of loess. Front. Environ. Sci. 13:1578858. doi: 10.3389/fenvs.2025.1578858
Received: 18 February 2025; Accepted: 24 March 2025;
Published: 09 April 2025.
Edited by:
Pavol Midula, Czech University of Life Sciences Prague, CzechiaReviewed by:
Wenle Hu, Luoyang Institute of Science and Technology, ChinaManfred Sager, Bioforschung Austria, Austria
Copyright © 2025 Liu, Sun, Ma, Lv, Zhang and Nan. This is an open-access article distributed under the terms of the Creative Commons Attribution License (CC BY). The use, distribution or reproduction in other forums is permitted, provided the original author(s) and the copyright owner(s) are credited and that the original publication in this journal is cited, in accordance with accepted academic practice. No use, distribution or reproduction is permitted which does not comply with these terms.
*Correspondence: Qiang Sun, c3VucWlhbmcwNEBjdW10LmVkdS5jbg==