- 1Zhanjiang Planning, Survey and Design Institute Co., Ltd, Zhanjiang, China
- 2Guangzhou Urban Planning and Design Survey Research Institute Co., Ltd, Guangzhou, China
- 3ollaborative Innovation Center for Natural Resources Planning and Marine Technology of Guangzhou, Guangzhou, China
- 4Guangdong Enterprise Key Laboratory for Urban Sensing, Monitoring and Early Warning, Guangzhou, China
- 5School of Geography and Planning, Sun Yat-sen University, Guangzhou, China
As urbanization accelerates, decoupling urban development from environmental consumption, particularly carbon emissions, is crucial for sustainable growth and achieving carbon neutrality goals. This study introduces urban expansion intensity as a new perspective to analyze the decoupling between urban expansion and carbon emissions. Based on the Urban Expansion Disparity Index, 297 Chinese cities are classified. The Tapio model is used to examine the spatiotemporal variations in decoupling from 2007 to 2022, while the LMDI model identifies key factors influencing regional carbon emissions. Findings reveal: (1) a polarized decoupling pattern, dominated by weak and strong negative decoupling; (2) a strong link between urban expansion types and decoupling modes, with fast-expanding cities exhibiting weak/strong decoupling and growth linkage, while slow-expanding or shrinking cities show expansion-negative and strong negative decoupling; (3) expansion and affluence effects drive emissions, while technology mitigates them, with significant regional differences. This study provides theoretical insights into urban expansion and carbon decoupling dynamics, informing region-specific policy interventions.
1 Introduction
Global warming has emerged as a critical environmental challenge, profoundly impacting human production and daily life worldwide. As the primary driver of climate change, carbon emissions have become a central focus in addressing the complex and evolving issues associated with global warming. Reducing carbon emissions is essential for achieving sustainable development at the regional level (Ballantyne et al., 2016). Cities, being the primary hubs of human social activities, are also the main sources of carbon emissions, stemming from industrial activities, energy consumption (Zhao et al., 2025), and residential usage (Fawzy et al., 2020). Research indicates that urban carbon emissions account for more than 85% of China’s total emissions (Han et al., 2018), with key sectors such as industry and transportation being the predominant sources of energy use and carbon output in urban areas (Ma et al., 2017). Moreover, the accelerating process of urbanization has led to changes in land use patterns (Wu et al., 2011), energy demand (Dong et al., 2020), industrial structure, and the mobility of population and technology, making urban carbon reduction increasingly complex. As a result, an increasing number of scholars have focused their research on the nonlinear relationship between urbanization and carbon emissions (Xu et al., 2018), as well as the impact of urbanization on carbon emission performance (Liu et al., 2018). Undoubtedly, promoting low-carbon development in cities has become a pressing and critical challenge that must be urgently addressed.
The concept of decoupling in environmental studies refers to the weakening or separation of the relationship between economic development, resource consumption, and environmental pressures (Chen et al., 2018). It is typically categorized into two types: resource decoupling, which focuses on reducing the link between economic growth and resource/energy use, and impact decoupling, which aims to mitigate the connection between economic development and environmental degradation (Wiedenhofer et al., 2020). Decoupling indicators, which enable the dynamic assessment and quantification of the relationship between economic activities and environmental impacts, have been widely applied in studies exploring the evolving interaction between environmental change and economic development in recent years (Ward et al., 2016; Jiang et al., 2022). In particular, as carbon reduction has become an urgent global climate challenge, decoupling models have been increasingly used to examine the relationship between economic growth and carbon emissions (Li et al., 2022), gradually evolving into a key theoretical framework for promoting green and low-carbon development.
Research on decoupling primarily focuses on several key areas. In terms of methodology, commonly used approaches include: the OECD model, proposed by the Organisation for Economic Co-operation and Development, which compares the growth rates of environmental pressure and economic development; the Tapio decoupling model, which offers a more detailed characterization of the interactions between economic growth and environmental impacts (Wu et al., 2018b; Wu et al., 2024); and integrated methods that combine decoupling analysis with decomposition techniques, such as the Logarithmic Mean Divisia Index (LMDI), to explore the underlying drivers of decoupling (Li et al., 2019). At the research scale, studies on decoupling have primarily focused on the national and provincial levels. At the national level, research has become increasingly comprehensive, including panel studies covering multiple countries (Shuai et al., 2019), comparative analyses of decoupling performance among countries within the same organization (such as the OECD) (Magazzino et al., 2023), comparisons between developed and developing countries regarding the decoupling of economic growth and carbon emissions (Wu et al., 2018), as well as case studies on typical countries such as the United States and China (Wang and Wang, 2019). At the provincial level, existing studies similarly encompass panel analyses across 30 provinces (Cohen et al., 2019), as well as investigations into the relationship between differentiated economic development and resource–environment dynamics in specific regions, such as the Yangtze River Economic Belt and the Central China Urban Agglomeration (Luo et al., 2021; Yuan et al., 2023). Case studies focusing on individual provinces, such as Jiangsu and Guangdong (Xu et al., 2021; Wang et al., 2017), have also been increasing, contributing to the development of a more comprehensive research framework. In terms of research content, current studies primarily focus on two areas: the decoupling between economic development and carbon emissions across different sectors, and the key factors influencing decoupling. For the former, research has increasingly concentrated on energy-intensive industries such as metal products, non-metallic manufacturing, construction, manufacturing, and transportation (Lin and Teng, 2022; Wang and Feng, 2019; Wu et al., 2018a; Ren and Hu, 2012; Li et al., 2023). For the latter, existing studies have demonstrated that both macro- and micro-level factors—including energy structure, industrial structure, technological progress, urbanization rate, and household income levels—play significant roles in shaping the decoupling process (Zhao et al., 2022; Hao et al., 2022).
However, we also identify certain limitations in existing studies on the decoupling of economic development and carbon emissions. First, in terms of spatial scale, most research has focused on large-scale analyses at the national, regional, or provincial levels or on decoupling studies of individual cities (Zheng et al., 2019; Li et al., 2019), while multi-city analyses at smaller scales remain insufficient. However, increasing evidence suggests that cities within the same province exhibit significant differences in development characteristics, economic growth responses, policy implementation, and environmental changes (Chen et al., 2019; Xie et al., 2025; Feng et al., 2025). These disparities underscore the necessity of conducting decoupling analyses at the urban scale, as city-level studies can more accurately capture the diversity of development trajectories and provide a stronger basis for targeted policy interventions. Second, regarding the selection of development indicators, existing decoupling studies predominantly define economic development in terms of GDP growth. While GDP serves as a quantitative measure of economic activity, it fails to directly reflect the associated environmental resource consumption. Moreover, economic growth is subject to an “optimal scale,” implying that GDP alone cannot comprehensively capture the societal and environmental implications of decoupling, whether positive or negative (Herman, 2014; Vadén et al., 2020). To better elucidate the relationship between economic development and carbon emissions decoupling, researchers have increasingly incorporated additional variables, such as human wellbeing, into their analyses (Kallis et al., 2018). Thus, it is evident that beyond conventional indicators, incorporating additional economic development indicators closely linked to both the economy and the environment is crucial for accurately capturing the real societal and ecological impacts of decoupling. To address these gaps, this study takes cities as the fundamental research unit and employs urban construction land expansion intensity as a key variable to examine its decoupling relationship with carbon emissions. Urban construction land, defined as land within urban areas designated to meet the economic, social, and daily needs of residents, encompasses residential, industrial, commercial, public service facilities, transportation, municipal infrastructure, and green spaces (Lai et al., 2020). As a critical resource for urban development, the expansion of urban construction land significantly drives economic growth (Xie et al., 2018), making it a key explicit indicator of urbanization. However, it also represents a scarce resource and a substantial “carbon source” (Peng et al., 2022). On one hand, changes in urban land expansion and utilization patterns directly influence carbon emissions (Sun et al., 2022); on the other hand, human activities on construction land generate indirect carbon emissions (Anser, 2019). From this analysis, it is evident that the expansion of urban construction land and sustainable development presents a paradoxical relationship. Achieving effective decoupling between urban expansion and carbon emissions is essential for reconciling carbon reduction goals with the pursuit of high-quality urban development. This underscores the need for innovative approaches to urban planning and resource management that balance growth with environmental sustainability.
Against the backdrop of accelerating urban expansion in China, the contradiction between rising carbon emissions and national emission reduction targets has created a “dual pressure,” posing significant challenges for urban carbon control (Wang et al., 2024b). To address the research gap in decoupling studies from the perspective of “expansion intensity–carbon emissions” at the city level and to enrich the theoretical framework of low-carbon urban development, this study selects 297 cities in China as the sample area and classifies them based on their urban expansion speed using the Urban Expansion Disparity Index. Departing from conventional approaches, the study innovatively replaces the traditional GDP indicator, commonly employed in decoupling research, with the urban land expansion intensity indicator. By applying the Tapio decoupling model, the study investigates the spatiotemporal dynamics of the decoupling relationship between urban expansion intensity and carbon emissions across different city types from 2007 to 2022. Furthermore, an LMDI (Logarithmic Mean Divisia Index) model is developed to analyze the influence of urban construction land expansion, affluence, and technological effects on carbon emissions in various city types. This approach aims to identify key leverage points for implementing effective carbon reduction strategies, providing a nuanced understanding of the interplay between urban growth and environmental sustainability.
2 Materials and methods
2.1 Urban expansion indicator construction
Urban expansion typically refers to the outward growth and development of cities, most visibly manifested by the increase in the area of urban built-up land (Zhang and Han, 2024). To facilitate a more detailed analysis of the temporal patterns and spatial disparities in urban expansion, this study employs two key indicators: urban expansion intensity and the urban disparity change index. The specific formulas for these indicators are as follows.
2.1.1 Urban expansion intensity
Urban expansion intensity (UI) refers to the rate of increase in urban built-up land area over a specific period. It is commonly used to compare the expansion levels of different cities or the same city across different periods (Yang et al., 2023). In this study, it is defined as the ratio of the average annual growth in urban built-up land area to the initial land area, as shown in Equations 1:
In the equation, UI represents the urban expansion intensity across different cities, reflecting the level of urban built-up land expansion during the urbanization process. Area refers to the urban built-up land area during the given period, t1 and t2 represent different years, with the years 2007, 2010, 2013, 2016, 2019, and 2022 being considered in this study. T denotes the time gap between the panel years.
2.1.2 Urban expansion disparity index
The Urban Expansion Disparity Index (UEDI) is defined as the ratio between the urban expansion rate of a specific sample area and the overall urban expansion rate of the study region. This index quantitatively characterizes the spatial heterogeneity in urban expansion intensity among different cities during the same temporal period. The mathematical formulation of UEDI is expressed in Equations 2:
According to relevant literature (Liu et al., 2016), urban expansion levels can be classified into six categories: High-speed expansion (HE), Rapid expansion (RE), Moderate expansion (ME), Slow expansion (SE), Ultra-slow expansion (USE), and Negative expansion (NE). The specific classification criteria are provided in Table 1.
2.2 Decoupling analysis method
Decoupling refers to the process of reducing or eliminating the link between economic growth and resource consumption or environmental degradation. In other words, it signifies a situation where economic growth is no longer constrained by environmental and resource limitations, making decoupling a key indicator for assessing sustainable development. This concept is widely employed in studies examining the relationship between economic benefits and environmental costs (Xin et al., 2021). Common methods for decoupling analysis include the OECD approach and the Tapio decoupling model. Among these, the Tapio model offers an advantage by capturing the dynamic characteristics of variable changes, rather than providing mere static descriptions. Moreover, it is particularly effective in capturing complex nonlinear relationships, making it applicable in a broader range of scenarios (Hou et al., 2023). Given that urban construction land serves as an intuitive reflection of functional area changes—such as residential, industrial, and commercial zones, which are primary sites of economic activity and carbon emissions—this study substitutes the conventional economic change indicator in the Tapio model with urban expansion intensity. This approach aims to explore the decoupling relationship between urban expansion intensity and carbon emissions. The formula is as follows in Equations 3 (Tapio, 2005):
Here,
2.3 LMDI decomposition analysis
The Kaya identity is a structural decomposition framework for analyzing changes in carbon emissions, derived from the IPAT model (Wang et al., 2005). Building on this analysis, this study uses the Kaya identity to decompose the factors influencing carbon emissions into scale effect (P), affluence effect (A), and technology effect (T). The specific decomposition formula is as follows in Equations 4:
Here,
Here,
2.4 Data resources
The data utilized in this study can be categorized into two primary types. The first type comprises city-level carbon emission data, sourced from the Emissions Database for Global Atmospheric Research (EDGAR v8.0) (Crippa et al., 2023). The second type includes data on urban built-up areas and industrial GDP, obtained from various statistical resources, including the China Statistical Yearbook, China City Statistical Yearbook, and China Urban Construction Statistical Yearbook. Based on data availability and completeness, a total of 297 cities were selected for the analysis. The study focuses on the years 2007, 2010, 2013, 2016, 2019, and 2022 to investigate the decoupling relationship between urban expansion and carbon emissions. Missing data points were addressed using interpolation methods to ensure the robustness of the dataset.
3 Results
3.1 Urban expansion and carbon emission changes
Table 3 and Figure 1 illustrate the changes in urban expansion intensity and the distribution of expansion disparity indices among the sample cities over the study period. Overall, urban land use in China has continuously expanded, though at a relatively low intensity, predominantly within the range of 0–5. Over time, urban expansion intensity has shown a steady decline. For example, cities with an intensity range of 20–30 decreased from 10 during 2007–2010 to just two during 2019–2022. Notably, the number of cities with an expansion intensity below five has increased since 2013, a trend that can be attributed to policies promoting efficient and intensive land use, ecological civilization initiatives, and the implementation of ecological redlines (Nuissl and Siedentop, 2021; Yang and Xie, 2021). Concurrently, there has been a rise in the number of cities experiencing negative expansion. From the perspective of expansion disparity indices, urban expansion has exhibited an overall increasing trend, accompanied by growing polarization. High-speed expansion and slow or negative expansion have emerged as the dominant patterns. During 2019–2022, the number of cities with high-speed expansion reached 98, accounting for 33.0% of all expansion types. In contrast, the number of cities with ultra-low-speed or declining expansion ranged between 50 and 60. This polarization is likely the result of the uneven pace of urban development during periods of rapid urbanization and industrialization. While some cities have faced constraints due to policies or resource limitations, others have experienced significantly higher expansion intensity than most regions. Spatially, areas of high-speed urban expansion have gradually shifted from central regions to southeastern coastal areas, while low-speed expansion zones are predominantly located in northeastern regions. This spatial pattern is consistent with China’s development strategies and the current state of economic and urbanization progress.
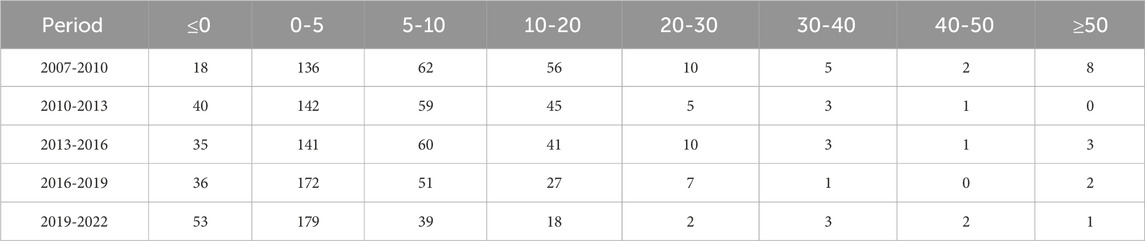
Table 3. The number of cities corresponding to changes in urban expansion intensity during the study period.
Figure 2 illustrates the changes in carbon emissions across cities over the study period. From a temporal perspective, between 2007 and 2013, carbon emissions in most cities exhibited a rapid growth trend, with increases ranging from 10 to 25 t. During the 2013–2016 period, carbon emissions decreased in the majority of cities, with a year-on-year reduction of approximately 0–5 t. From 2016 to 2022, carbon emissions rebounded, although the increase was modest, with a growth range of 0–5 t. Spatially, inland and northeastern regions experienced relatively higher carbon emission increases, with growth concentrated in resource-intensive or industrial cities such as Huanggang, Xianning, and Yanan. Furthermore, this analysis reveals a general trend: the pattern of changes in urban expansion intensity closely mirrors the trends in carbon emission fluctuations across cities.
3.2 Urban expansion and decoupling of carbon emissions
3.2.1 Types of urban decoupling
Figure 3 illustrates the distribution of decoupling types among the sample cities during the study period. In summary, weak decoupling (WD), strong negative decoupling (SND), and expanding negative decoupling are the most prevalent decoupling relationships between urban expansion and carbon emissions in China. Over the study period, the number of cities exhibiting WD remained around 100, while those with SND fluctuated around 74. Strong decoupling (SD) and growth linkage (GL) were also relatively common decoupling types, with the number of cities categorized under SD peaking at 149 during the 2013–2016 period but remaining below 50 in other years. The number of cities with SND remained relatively stable, hovering around 40 throughout the study. On the other hand, declining decoupling (DD), declining linkage (DL), and weak negative decoupling (WND) were less frequent, with some years showing no cities in these categories. Overall, the structure of decoupling types in Chinese cities has remained relatively stable over the past 15 years, though a clear polarization is evident. On one hand, while urban expansion intensity and carbon emissions continue to rise, most regions are gradually reducing the negative by-products of carbon emissions, with initial successes in low-carbon urban development. On the other hand, a significant number of cities still face rising carbon emissions, despite a decrease in urban construction land, which highlights persistent issues related to inefficient land use and energy-intensive industrial structures.
Figure 4 illustrates the spatial distribution of the decoupling dynamics between urban expansion intensity and carbon emissions. It is evident that cities characterized by expanding negative decoupling (END) and strong negative decoupling (SND) are primarily concentrated in the northeastern region and certain economically fast-growing cities in central China. In contrast, weak decoupling (WD) and strong decoupling (SD) cities are predominantly located in the southeastern coastal areas, with this spatial pattern becoming increasingly pronounced over time. Overall, the spatial distribution of decoupling characteristics is closely related to factors such as the level of economic development, industrial structure, and technological progress. Different urban development patterns lead to varying intensities and functional demands for construction land, resulting in distinct decoupling states. Generally, regions with advanced economies, significant industrial upgrading, and strong innovation capabilities exhibit better decoupling performance. In contrast, areas with a single economic structure, reliance on high-carbon industries, and a lack of green technology adoption tend to experience weaker decoupling. Moreover, policy orientation also plays a crucial role in the decoupling between construction land use and carbon emissions. For instance, early policies like the Western Development Strategy and the Northeast Industrial Revitalization Plan increased construction land use intensity and carbon emissions. On the other hand, the implementation of the “Zero-Waste City” policy has promoted resource-efficient development (Qian et al., 2025), accelerated the green transition of enterprises and localities, and directly reduced urban carbon emissions.
At the city level, specific patterns further emerge, SND cities are concentrated in areas where traditional industries dominate and economic transformation is still in its early stages. Examples include Taiyuan and Jincheng in Shanxi, and Daqing and Jiamusi in Heilongjiang. Despite the slowdown in urban expansion due to factors such as land use policies, fiscal constraints, or population loss (Huo and Huang, 2023), these cities’ economic structures remain heavily dependent on high-carbon industries, such as coal and steel. The persistence of inefficient energy systems and outdated industrial practices places these cities in the SND stage. On the other hand, END cities are typically found in rapidly developing regions, such as Shenzhen and Chongqing. While urbanization continues to generate high demand for construction land, these cities, despite implementing some low-carbon policies and efforts to transition toward service-oriented and high-tech industries, still heavily rely on manufacturing and construction sectors. As a result, the growth rate of carbon emissions exceeds that of urban expansion, with limited benefits from energy structure optimization. In contrast, WD and SD cities are predominantly concentrated in Beijing and the Yangtze River Delta region, including cities like Shanghai, Suzhou, and Hangzhou. In these areas, heavy industries have been optimized or relocated to surrounding regions, while the development of e-commerce, internet-based technologies, and other high-tech service industries has matured (Wang et al., 2022). The application of low-carbon technologies further supports this transition, resulting in slower urban expansion and a clear decoupling from the negative impacts of carbon emissions.
3.2.2 Decoupling analysis by urban expansion types
Figure 5 illustrates the decoupling status of carbon emissions across different urban expansion types, revealing a significant correspondence between the two, with only minor changes over time. Overall, cities with rapid expansion predominantly exhibit weak decoupling (WD) and growth linkage (GL), where the growth rate of carbon emissions is either comparable to or slightly lower than the rate of urban expansion. Over time, these cities tend to transition toward strong decoupling (SD), an increasingly desirable state. In contrast, cities with slower or stagnant expansion mainly experience expanding negative decoupling (END), characterized by a highly unbalanced development pattern where carbon emissions rise significantly faster than urban land expansion. Examining the cities corresponding to these expansion types, it becomes evident that the rate of urban land expansion reflects, to some extent, the vitality of urban development, particularly in terms of industrial and economic growth. Cities with faster expansion generally exhibit greater economic diversification, continuous industrial upgrading, and improved energy efficiency, facilitating a transition from high-carbon, resource-intensive growth to a more low-carbon and efficient development model. Consequently, land-use efficiency and environmental benefits steadily improve. Conversely, cities with slower expansion—or even contraction—often face geographical constraints, resource limitations, or funding shortages that hinder their development. These cities tend to have a single economic structure and remain heavily reliant on traditional high-carbon industries, lacking new growth drivers. As a result, urban land use remains inefficient, and progress toward green development is significantly lagging.
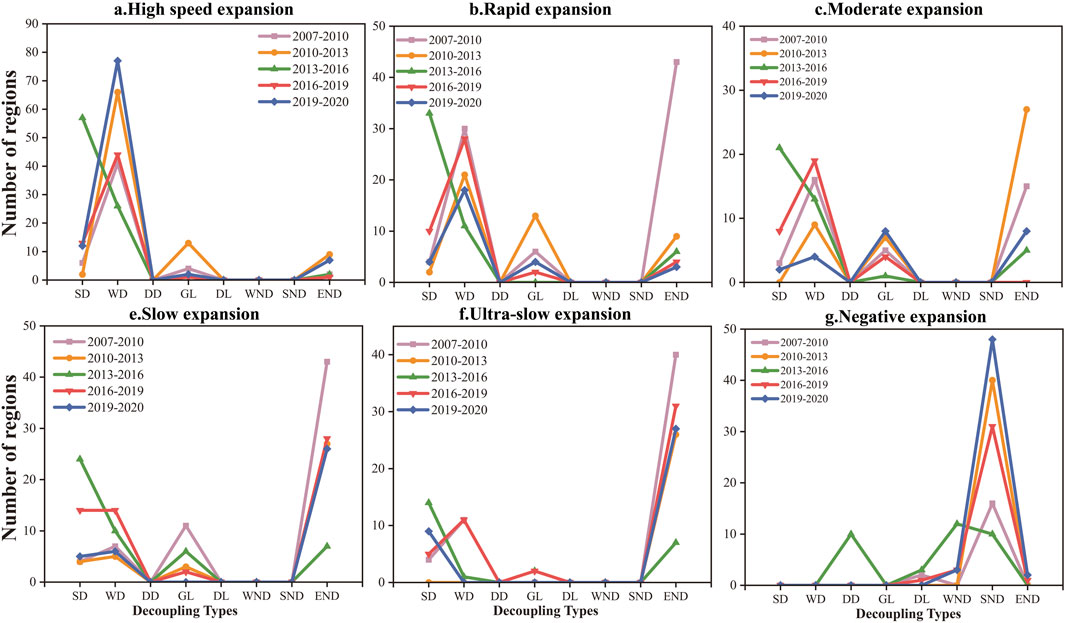
Figure 5. Sub-expansion type decoupling condition of China’s cities, 2007–2022. (a) High speed expansion. (b) Rapid expansion. (c) Moderate expansion. (d) Slow expansion. (e) Ultra-slow expansion. (f) Negative expansion.
A detailed analysis of specific cities reveals: WD cities are predominantly located in moderately developed cities in central and southern China, including Zhengzhou, Chengdu, Wuxi, and Ningbo. Despite their strong demand for construction land, these cities have increasingly adopted efficient and intensive land-use practices, transitioned to cleaner energy sources, and shifted their industrial structures toward low-carbon sectors, such as services. Taking Chengdu as an example, the city experienced rapid urban expansion from 2007 to 2022, with construction land increasing by 34.31 square kilometers. However, carbon emission growth gradually slowed, rising by 3.14 tCO2 from 2007 to 2010 but only by 1.45 tCO2 between 2019 and 2022. Notably, from 2013 to 2019, carbon emissions even showed a negative growth trend. This deceleration is closely linked to the upgrading of Chengdu’s automotive and equipment manufacturing industries, as well as the gradual refinement of green spatial optimization strategies, such as the “Eastward Expansion, Central Optimization” initiative. Compared to cities with other expansion types, these WD cities represent an ideal model of economic and environmental synergy. However, they still have significant room for improvement before achieving SD scenarios. For cities with slow or ultra-slow expansion, expanding negative decoupling (END) is the dominant decoupling type. These cities, often located in central and northeastern regions such as Tangshan, Lanzhou, and Jilin, are typically industrial hubs facing a conflict between high land demand and resource constraints. The failure of these cities’ industrial structures to transition to low-carbon models, combined with persistent energy demand, has resulted in unchecked growth of carbon emissions, leading to land-use overload (Bao et al., 2022). Taking Jilin City as an example, from 2007 to 2022, the expansion of urban construction land was minimal, increasing by only 13.38 square kilometers, with just 1.20 square kilometers added between 2016 and 2022. However, carbon emissions surged significantly, rising by 12.60 tCO2. Throughout the study period, Jilin City’s economic development remained heavily dominated by industrial sectors. Despite the gradual implementation of farmland balance and land reclamation policies, the rapid growth of traditional industries such as chemicals, automotive manufacturing, and metallurgy continued to generate substantial carbon emissions, resulting in a poor decoupling performance. In cities exhibiting negative expansion, strong negative decoupling (SND) emerges as the primary decoupling type. Such cities are predominantly located in northeastern provinces like Yichun and Harbin. In these regions, land contraction is accompanied by rising carbon emissions, driven by inefficient, energy-intensive development models dominated by heavy industries. This high-energy, low-efficiency development warrants urgent attention (Wang et al., 2020; Zeng et al., 2022). Taking Yichun City as an example, from 2007 to 2022, urban construction land contracted by 13.03 square kilometers. However, carbon emissions continued to rise, increasing by 26.37 tCO2. This trend is likely driven by the city’s status as a resource-declining economy, where the forestry and mining industries—once the backbone of local development—have steadily declined. As a result, land demand has decreased, and some industrial areas, due to inefficient utilization and business closures, have been left idle, prompting the government to reclaim and repurpose them. It is important to note that these classifications represent the predominant decoupling types for each urban expansion category, though exceptions do exist. For example, cities like Beijing and Shanghai, despite ongoing industrial transformation and widespread adoption of low-carbon policies, face challenges such as population influx and saturated land use. These factors have led to decoupling statuses like END. To address these challenges, strategies such as enclave development and cross-regional collaboration policies have gained increasing prominence and are being actively implemented in recent years.
3.3 Decomposition effect analysis
Based on the LMDI decomposition results, the effects of land expansion, affluence, and technological progress on changes in carbon emission intensity are analyzed across different decoupling and urban land expansion types. Table 4 presents the specific effects of these three factors on cities with varying decoupling types. Overall, both the land expansion effect and the affluence effect contribute positively to carbon emissions, thereby driving increases in emission levels. However, the influence of these effects weakens over time. Specifically, the total land expansion effect decreased from 175.85 in the 2007–2010 period to 57.91 in 2019–2022, while the affluence effect declined from 331.38 to 30.38 over the same period. This trend can be attributed to the fact that urban land expansion often entails the proliferation of energy-intensive and high-carbon activities, such as infrastructure development and industrial transportation, while economic growth leads to higher income levels and increased consumption, both of which contribute to greater carbon emissions. However, as industrial structures evolve and low-carbon awareness increases, the magnitude of these promoting effects gradually diminishes (Sun and Huang, 2020; Zhang et al., 2017). In contrast, the technological effect consistently exerts a negative inhibitory impact on carbon emissions, contributing to a reduction in emissions throughout the study period. Technological advancements, particularly those related to efficiency improvements and the adoption of clean energy technologies, have proven to be effective mechanisms for mitigating carbon emissions (Xie et al., 2021).
The effects of the three factors—land expansion, affluence, and technological progress—differ across specific decoupling types. For instance, in the 2019–2022 analysis, regions characterized by SD, WD, and GL decoupling types are typically in a transitional phase, shifting from traditional low-value-added industries to emerging high-value-added sectors. This structural transformation is often accompanied by substantial economic growth, which in turn diminishes or even reverses the positive impact of the affluence effect on carbon emissions. Conversely, regions with SND, WND, and DD decoupling types tend to experience a suppressive effect on carbon emissions due to urban decline. In these areas, urban land use is generally contracting, and any expansion typically focuses on redeveloping existing urban areas, such as creative industry parks, which are less associated with high-carbon activities. As a result, the influence of land expansion on carbon emissions is reduced (Liu et al., 2023). Finally, in regions exhibiting SND and END decoupling types, technological advancements contribute to increased carbon emissions. These regions continue to heavily rely on high-carbon industries for economic growth, and while technological improvements may enhance the production efficiency of these industries, they do not substantially alter the underlying energy structure or industry characteristics. Consequently, the application of such technologies still results in significant carbon emissions.
4 Discussion
In the context of rapid urbanization, urban expansion stands as one of the most significant socio-economic phenomena. Urban land functions as the primary spatial platform for infrastructure, industries, and human activities. The process of urban expansion reflects the dynamic interaction between economic development and resource-environmental factors. Investigating the relationship between urban expansion and carbon emissions offers valuable insights into land-use efficiency, clarifies the link between urbanization and green, low-carbon development, and provides a crucial theoretical foundation for achieving carbon neutrality within the context of urban development (Wang et al., 2024a). Therefore, this study classifies 297 sample cities into distinct urban expansion types using the Urban Expansion Disparity Index. It then examines the spatiotemporal evolution of the decoupling relationship between urban expansion intensity and carbon emissions from 2007 to 2022 using the Tapio decoupling index. Finally, the study explores the differentiated contributions of urban expansion, affluence, and technological effects to carbon emissions through LMDI structural decomposition.
The study demonstrates that the decoupling between urban expansion and carbon emissions in China has remained relatively stable over time, gradually progressing towards the ideal scenario of “urban expansion increasingly decoupling from the negative externalities of carbon emissions.” However, significant room for improvement remains. Spatially, there is a pronounced polarization in the decoupling outcomes, with weak decoupling (WD) and strong negative decoupling (SND) emerging as the two dominant and contrasting decoupling types across Chinese cities. A closer analysis by city type reveals that cities exhibiting improved decoupling trends tend to be characterized by dynamic urban vitality, successful industrial restructuring, and robust economic transformation. The Yangtze River Delta region stands out as a key cluster of such cities. In contrast, cities with deteriorating decoupling trends, often experience what is referred to as “Hegangzation” (a term describing the economic stagnation, population outflow, and urban decline faced by resource-dependent cities like Hegang in Heilongjiang Province) (Huang et al., 2024), face persistent challenges such as entrenched development patterns and stalled industrial transitions. These cities are predominantly located in the northeastern provinces and certain parts of central and western China. The emergence of different decoupling types is closely linked to regional development policies, economic structures, and local vitality. The unequal decoupling patterns highlight the role of rapid urbanization in exacerbating regional disparities and polarization in urban development (Hu et al., 2024). To achieve comprehensive decoupling between urban expansion and carbon emissions, it is crucial to prioritize coordinated development across urban areas.
Analyzing the relationship between urban expansion types and carbon emission decoupling types reveals a significant and relatively stable correspondence. In general, cities experiencing rapid expansion are predominantly associated with weak decoupling (WD) and growth linkage (GL) decoupling types. These cities, typically at an upper-middle stage of development, continue to exhibit strong demand for land. However, the direction of their development—whether toward WD or GL—largely depends on factors such as the intensity of land use (extensive vs intensive) and the nature of their industrial structure (high-carbon vs energy-efficient). Cities characterized by slow expansion are mainly linked to expansion-negative decoupling (END). These cities often grapple with the contradiction of high land demand and limited available resources. Their development remains heavily reliant on energy-intensive industries, positioning them as both major sources of environmental pollution and key targets for low-carbon governance efforts. Conversely, cities in decline predominantly exhibit stagnation-negative decoupling (SND). Under the combined pressures of population loss and resource depletion, these cities are marked by inefficient and energy-intensive development patterns. To address these challenges, it is crucial for these cities to cultivate new growth drivers and undergo structural transformation.
The effect analysis results indicate that, overall, both urban land expansion and affluence contribute to increased carbon emissions, while technological effects play a suppressive role. This suggests that China’s current economic development remains heavily dependent on resource consumption, although technological innovation can help mitigate carbon emissions to some extent. However, the impact of these effects varies across different decoupling types, reflecting regional disparities in development stages. In regions undergoing industrial restructuring towards low-carbon, high-value-added sectors, the contribution of the affluence effect to carbon emissions significantly weakens, and may even become suppressive. Conversely, in regions where industrial structures continue to rely heavily on energy-intensive industries, both affluence and technological effects contribute substantially to carbon emissions. In shrinking cities, which suffer from a lack of economic vitality, the relationship between urban land expansion and carbon emissions is weaker, and the expansion effect has a minimal impact on driving emissions.
5 Conclusions and policy implications
This study analyzes 297 Chinese cities, classifying them by urban expansion intensity using the Urban Expansion Disparity Index. The Tapio decoupling model is employed to investigate the spatiotemporal evolution of decoupling relationships between urban expansion and carbon emissions from 2007 to 2022. Additionally, the LMDI model is applied to assess the impacts of land expansion, affluence, and technological factors on the carbon intensity of cities across different decoupling types. The results reveal a pronounced polarization in the decoupling states of urban expansion intensity and carbon emissions in China, with Weak Decoupling (WD) and Strong Negative Decoupling (SND) being the dominant types. From an urban typology perspective, there is a clear correspondence between urban expansion types and decoupling outcomes: rapidly expanding cities are predominantly categorized as WD and Growth Linkage (GL) types, slowly expanding cities are mainly classified as Expansion-Negative Decoupling (END) types, while declining cities are primarily characterized by SND. Furthermore, in the context of China’s current development stage, urban expansion and affluence significantly contribute to increased carbon emissions, while technological advancements effectively mitigate them. Compared to previous studies, this research innovatively adopts urban construction land expansion intensity as an indicator of economic development, addressing the limitations of GDP, which fails to capture the environmental resource consumption associated with economic growth and, consequently, cannot fully reflect the social and environmental impacts of the decoupling process between urban development and carbon emissions. By systematically analyzing the decoupling relationship between urban expansion and carbon emissions across different city types, this study provides a comprehensive examination of the underlying mechanisms linking urban development and environmental sustainability. From a land-use perspective, it establishes a solid theoretical foundation for formulating differentiated carbon reduction policies tailored to cities with varying urban expansion patterns, thereby contributing to a synergistic balance between new urbanization and low-carbon sustainable development across diverse urban contexts.
Based on these findings, several policy recommendations are proposed to address the identified challenges and leverage the potential of low-carbon urban development.
Firstly, from a regional development perspective, addressing the unequal patterns of urban expansion and carbon emission decoupling necessitates the establishment of a collaboration-oriented regional strategy to reduce interregional disparities. On the one hand, the government should prioritize policy support for the northeastern provinces and certain underdeveloped central regions. This can be achieved through specific measures such as encouraging the development of the non-public economy, implementing policies to promote border-area openness in the northeast, and enhancing infrastructure and transportation networks in key development zones, remote and underprivileged areas, and other less-developed regions. These efforts aim to accelerate industrial restructuring, expand external openness, and foster new growth drivers, ultimately reversing urban decline and revitalizing regional development. On the other hand, further advancing urban cluster integration should be accompanied by targeted support for less-developed areas through policies such as paired assistance and cooperative partnerships. These initiatives should facilitate resource and technology sharing while ensuring that potential risks, such as carbon leakage during industrial relocation, are effectively managed. This strategy aims to harness urban growth dynamics to drive high-quality regional development, achieving both sustainable progress and balanced regional equity.
Secondly, improving the current decoupling status through industrial restructuring and land-use optimization remains a crucial pathway for achieving low-carbon development. To this end, tailored carbon reduction policies should be implemented based on the specific developmental characteristics of different urban expansion types. For rapidly expanding cities, efforts should focus on promoting the rapid development of low-carbon, high-value-added industries, advancing smart city initiatives, and expanding renewable energy adoption to reduce carbon emissions. Additionally, optimizing land-use efficiency is essential—implementing a compact urban development model, restructuring land-use patterns, curbing inefficient urban sprawl, and encouraging high-density, low-carbon communities can significantly mitigate the high carbon footprint associated with extensive urban expansion. For slowly expanding cities, priority should be given to accelerating the low-carbon transition of energy-intensive industries. This can be achieved by encouraging enterprises to adopt cleaner production technologies, improving energy efficiency (Sun et al., 2024), and exploring digitalization and smart manufacturing pathways to reduce their carbon footprint. Simultaneously, in terms of land use, optimizing land-use planning, revitalizing underutilized land, and promoting the renewal and redevelopment of old industrial areas can enhance both economic and ecological benefits. For declining cities, the key lies in fostering emerging industries and stimulating new growth drivers. This can be achieved by attracting external investment and introducing advanced technology industries to transform the urban development model, addressing both economic stagnation and environmental degradation. Additionally, land reclamation and ecological restoration measures should be implemented, alongside strategic urban zoning to prevent unregulated expansion that could harm the environment. Promoting green agriculture and eco-tourism can further enhance the comprehensive value of land, supporting sustainable development.
Finally, harnessing the emission-reduction potential of technological innovation is a vital pathway for achieving synergy between urban development and carbon reduction (Liang et al., 2024). Efforts should focus on advancing the research, development, and deployment of low-carbon technologies through increased financial investment and targeted policy support. Accelerating the adoption of these technologies across diverse cities and industries is essential, particularly in energy-intensive cities and traditional industrial sectors. Such measures will provide the necessary technological foundation to facilitate the decoupling of urban expansion from carbon emissions, driving progress toward sustainable urban development.
However, this study has several limitations. First, the reliance on local statistical yearbook data for urban construction land may introduce discrepancies between reported data and actual land use. These discrepancies arise from variations in statistical standards and data accuracy across different regions and years. Second, the selection of sample areas is constrained by data availability, leading to incomplete coverage of urban areas nationwide. This limitation is particularly evident in the underrepresentation of cities in northwestern provinces, such as Tibet, Xinjiang, and Qinghai, which may result in regional gaps in the analysis. Finally, this study provides a relatively simplified analysis of the mechanisms influencing the decoupling relationship between urban expansion and carbon emissions. It primarily considers internal factors such as affluence effects, technological effects, and emission intensity effects, while overlooking potential external influences, such as policy interventions, on the decoupling process. Future research should employ more sophisticated models, such as the PSM-DID approach (Wang et al., 2024), to further investigate the underlying mechanisms shaping the relationship between urban expansion and carbon emissions, thereby offering a more comprehensive theoretical foundation for carbon reduction policies.
Data availability statement
The original contributions presented in the study are included in the article/supplementary material, further inquiries can be directed to the corresponding author.
Author contributions
XL: Resources, Software, Writing – original draft. YL: Writing – original draft. SW: Conceptualization, Supervision, Writing – original draft.
Funding
The author(s) declare that financial support was received for the research and/or publication of this article. This work was supported by Collaborative Innovation Center for Natural Resources Planning and Marine Technology of Guangzhou (Nos. 2023B04J0301 and 2023B04J0046).
Acknowledgments
Last but not least, many thanks for the support of Academic Specialty Group for Urban Sensing in Chinese Society of Urban Planning.
Conflict of interest
Author XL was employed by Survey and Design Institute Co., Ltd. Author YL was employed by Guangzhou Urban Planning & Design Survey Research Institute Co., Ltd.
The remaining author declares that the research was conducted in the absence of any commercial or financial relationships that could be construed as a potential conflict of interest.
Generative AI statement
The author(s) declare that no Generative AI was used in the creation of this manuscript.
Publisher’s note
All claims expressed in this article are solely those of the authors and do not necessarily represent those of their affiliated organizations, or those of the publisher, the editors and the reviewers. Any product that may be evaluated in this article, or claim that may be made by its manufacturer, is not guaranteed or endorsed by the publisher.
References
Anser, M. K. (2019). Impact of energy consumption and human activities on carbon emissions in Pakistan: application of STIRPAT model. Environ. Sci. Pollut. Res. 26 (13), 13453–13463. doi:10.1007/s11356-019-04859-y
Ballantyne, A. G., Wibeck, V., and Neset, T.-S. (2016). Images of climate change – a pilot study of young people’s perceptions of ICT-based climate visualization. Clim. Change 134, 73–85. doi:10.1007/s10584-015-1533-9
Bao, C., Wang, H., and Sun, S. (2022). Comprehensive simulation of resources and environment carrying capacity for urban agglomeration: a system dynamics approach. Ecol. Indic. 138, 108874. doi:10.1016/j.ecolind.2022.108874
Chen, J., Wang, P., Cui, L., Huang, S., and Song, M. (2018). Decomposition and decoupling analysis of CO2 emissions in OECD. Appl. Energy 231, 937–950. doi:10.1016/j.apenergy.2018.09.179
Chen, L., Xu, L., and Yang, Z. (2019). Inequality of industrial carbon emissions of the urban agglomeration and its peripheral cities: a case in the pearl River Delta, China. Renew. Sustain. Energy Rev. 109, 438–447. doi:10.1016/j.rser.2019.04.010
Crippa, M., Guizzardi, D., Pagani, F., and Pisoni, E. (2023). GHG Emissions at sub-national level. European Commission, Joint Research Centre (JRC). Available online at: http://data.europa.eu/89h/d67eeda8-c03e-4421-95d0-0adc460b9658
Dong, B., Ma, X., Zhang, Z., Zhang, H., Chen, R., Song, Y., et al. (2020). Carbon emissions, the industrial structure and economic growth: evidence from heterogeneous industries in China. Environ. Pollut. 262, 114322. doi:10.1016/j.envpol.2020.114322
Fawzy, S., Osman, A. I., Doran, J., and Rooney, D. W. (2020). Strategies for mitigation of climate change: a review. Environ. Chem. Lett. 18 (6), 2069–2094. doi:10.1007/s10311-020-01059-w
Feng, W., Guo, B., and Yu, Y. (2025). The impact of green finance reform on industrial water pollution: evidence from innovation pilot zones in China. Water Econ. Policy, March, 2440010. doi:10.1142/S2382624X24400101
Han, F., Xie, R., Lu, Y., Fang, J., and Liu, Y (2018). The effects of urban agglomeration economies on carbon emissions: evidence from Chinese cities. J. Clean. Prod. 172, 1096–1110. doi:10.1016/j.jclepro.2017.09.273
Hao, J., Gao, F., Fang, X., Nong, X., Zhang, Y., and Hong, F. (2022). Multi-factor decomposition and multi-scenario prediction decoupling analysis of China’s carbon emission under dual carbon goal. Sci. Total Environ. 841, 156788. doi:10.1016/j.scitotenv.2022.156788
Herman, E. D. (2014). From uneconomic growth to a steady-state economy. Cheltenham; Edward Elgar Publishing.
Hou, L., Wang, Y., Hu, L., Wang, Y., Li, Y., and Zheng, Y. (2023). Economic growth and carbon emissions analysis based on tapio-ekc coupled integration and scenario simulation: a case study of China’s transportation industry. Environ. Dev. Sustain. 26 (7), 18855–18881. doi:10.1007/s10668-023-03418-3
Hu, Y., Connor, D. S., Stuhlmacher, M., Peng, J., and Turner Ii, B. L. (2024). More urbanization, more polarization: evidence from two decades of urban expansion in China. Npj Urban Sustain. 4 (1), 33. doi:10.1038/s42949-024-00170-z
Huang, S., Lyu, Y., Shi, H., and Wu, C. (2024). Spatiotemporal analysis of the coupling relationship between urban infrastructure and land utilization in a shrinking city: a case study of Hegang, northeast China. Land 13 (11), 1904. doi:10.3390/land13111904
Huo, J., and Huang, S. (2023). Spatio-temporal characteristics and influencing factors of urban shrinkage in county level of Heilongjiang province, northeast China. Heliyon 9 (11), e21436. doi:10.1016/j.heliyon.2023.e21436
Jiang, Y., Tian, S., Xu, Z., Gao, L., Xiao, L., Chen, S., et al. (2022). Decoupling environmental impact from economic growth to achieve sustainable development goals in China. J. Environ. Manag. 312, 114978. doi:10.1016/j.jenvman.2022.114978
Kallis, G., Kostakis, V., Lange, S., Muraca, B., Paulson, S., and Schmelzer, M. (2018). Research on degrowth. Annu. Rev. Environ. Resour. 43 (1), 291–316. doi:10.1146/annurev-environ-102017-025941
Lai, Y., Chen, K, Zhang, J., and Liu, F. (2020). Transformation of industrial land in urban renewal in shenzhen, China. Land 9 (10), 371. doi:10.3390/land9100371
Li, J., Chen, Y., Li, Z., and Huang, X. (2019). Low-carbon economic development in central asia based on LMDI decomposition and comparative decoupling analyses. J. Arid Land 11 (4), 513–524. doi:10.1007/s40333-019-0063-0
Li, J., Huang, G., Li, Y., Liu, L., and Zheng, B. (2022). Decoupling degrees of China’s economic growth from three-perspective carbon emissions. J. Clean. Prod. 368, 133209. doi:10.1016/j.jclepro.2022.133209
Li, X.-Y., Chen, T., and Chen, B. (2023). Research on the influencing factors and decoupling state of carbon emissions in China’s transportation industry. Sustainability 15 (15), 11871. doi:10.3390/su151511871
Li, Y.-N, Cai, M., Wu, K., and Wei, J. (2019). Decoupling analysis of carbon emission from construction land in Shanghai. J. Clean. Prod. 210, 25–34. doi:10.1016/j.jclepro.2018.10.249
Liang, J., Wang, S., Liao, Y., and Feng, K. (2024). Carbon Emissions embodied in investment: assessing emissions reduction responsibility through multi-regional input-output analysis. Appl. Energy. 358, 122558. doi:10.1016/j.apenergy.2023.122558
Lin, B., and Teng, Y. (2022). Decoupling of economic and carbon emission linkages: evidence from manufacturing industry chains. J. Environ. Manag. 322, 116081. doi:10.1016/j.jenvman.2022.116081
Liu, B., Chen, T., Li, Y., Song, H., and Ma, Z. (2018). Research on the effects of urbanization on carbon emissions efficiency of urban agglomerations in China. J. Clean. Prod. 197, 1374–1381. doi:10.1016/j.jclepro.2018.06.295
Liu, F., Zhang, Z., Shi, L., Zhao, X., Xu, J., Yi, L., et al. (2016). Urban expansion in China and its spatial-temporal differences over the past four decades. J. Geogr. Sci. 26, 1477–1496. doi:10.1007/s11442-016-1339-3
Luo, H., Li, L, Lei, Y., Wu, S., Yan, D., Fu, X., et al. (2021). Decoupling analysis between economic growth and resources environment in central plains urban agglomeration. Sci. Total Environ. 752, 142284. doi:10.1016/j.scitotenv.2020.142284
Ma, M., Yan, R., Du, Y., Ma, X., Cai, W., and Xu, P. (2017). A methodology to assess China’s building energy savings at the national level: an IPAT–LMDI model approach. J. Clean. Prod. 143, 784–793. doi:10.1016/j.jclepro.2016.12.046
Magazzino, C., Pakrooh, P., and Abedin, M. Z. (2023). A decomposition and decoupling analysis for carbon dioxide emissions: evidence from OECD countries. Environ. Dev. Sustain. 26 (11), 28539–28566. doi:10.1007/s10668-023-03824-7
Nuissl, H., and Siedentop, S. (2021). “Urbanisation and land use change,” in Sustainable land management in a European context. Human-environment interactions. Editors T. Weith, T. Barkmann, N. Gaasch, S. Rogga, C. Strauß, and J. Zscheischler (Cham: Springer International Publishing), 8, 75–99. doi:10.1007/978-3-030-50841-8_5
Peng, J., Zheng, Y., and Liu, C. (2022). The impact of urban construction land use change on carbon emissions: evidence from the China land market in 2000–2019. Land 11 (9), 1440. doi:10.3390/land11091440
Qian, Y., Jiang, J., Guo, B., and Hu, F. (2025). The impact of ‘zero-waste city’ pilot policy on corporate green transformation: a causal inference based on double machine learning. Front. Environ. Sci. 13, 1564418. doi:10.3389/fenvs.2025.1564418
Ren, S., and Hu, Z. (2012). Effects of decoupling of carbon dioxide emission by Chinese nonferrous metals industry. Energy Policy 43, 407–414. doi:10.1016/j.enpol.2012.01.021
Shuai, C., Chen, X, Wu, Y., Zhang, Y, and Tan, Y. (2019). A three-step strategy for decoupling economic growth from carbon emission: empirical evidences from 133 countries. Sci. Total Environ. 646, 524–543. doi:10.1016/j.scitotenv.2018.07.045
Sun, C., Zhang, Y., Ma, W., Wu, R., and Wang, S. (2022). The impacts of urban form on carbon emissions: a comprehensive review. Land 11 (9), 1430. doi:10.3390/land11091430
Sun, W., and Huang, C. (2020). How does urbanization affect carbon emission efficiency? Evidence from China. J. Clean. Prod. 272, 122828. doi:10.1016/j.jclepro.2020.122828
Sun, Y., Wen, G., Dai, H., Feng, Y., Azaele, S., Lin, W., and Zho, F. (2024). Quantifying the resilience of coal energy supply in china toward carbon neutrality. Research, 7 0398. doi:10.34133/research.0398
Tapio, P. (2005). Towards a theory of decoupling: degrees of decoupling in the EU and the case of road traffic in Finland between 1970 and 2001. Transp. Policy 12 (2), 137–151. doi:10.1016/j.tranpol.2005.01.001
Vadén, T., Lähde, V., Majava, A., Järvensivu, P., Toivanen, T., Hakala, E., et al. (2020). Decoupling for ecological sustainability: a categorisation and review of research literature. Environ. Sci. and Policy 112, 236–244. doi:10.1016/j.envsci.2020.06.016
Wang, C., Chen, J., and Zou, J (2005). Decomposition of energy-related CO2 emission in China: 1957–2000. Energy 30 (1), 73–83. doi:10.1016/j.energy.2004.04.002
Wang, H., Wang, Y., Fan, C., Wang, X., Yao, W., Zhang, Z., et al. (2020). Material consumption and carbon emissions associated with the infrastructure construction of 34 cities in northeast China. Complexity 2020, 1–20. doi:10.1155/2020/4364912
Wang, J., Dong, X., and Dong, K. (2022). How does ICT agglomeration affect carbon emissions? The case of Yangtze River Delta urban agglomeration in China. Energy Econ. 111, 106107. doi:10.1016/j.eneco.2022.106107
Wang, M., and Feng, C. (2019). Decoupling economic growth from carbon dioxide emissions in China’s metal industrial sectors: a technological and efficiency perspective. Sci. Total Environ. 691, 1173–1181. doi:10.1016/j.scitotenv.2019.07.190
Wang, M., Wang, Y., Yang, Z., and Guo, B. (2024). Does energy-consuming rights trading policy achieve urban pollution and carbon reduction? A quasi-natural experiment from China. Front. Environ. Sci. 12, 1430031. doi:10.3389/fenvs.2024.1430031
Wang, S., Chen, X., Xie, R., Liu, K., Wang, J., Liu, X., et al. (2024a). Demand-side insights for steering human appropriation of net primary productivity within planetary boundaries. One Earth 7 650–662. doi:10.1016/j.oneear.2024.02.010
Wang, S., Zhou, S., Wu, R., Feng, K., and Hubacek, K. (2024b). Interregional flows of embodied carbon storage associated with land-use change in China. Ann. Am. Assoc. Geogr. 114 1526–1545. doi:10.1080/24694452.2024.2356849
Wang, Q., and Wang, S. (2019). Decoupling economic growth from carbon emissions growth in the United States: the role of research and development. J. Clean. Prod. 234, 702–713. doi:10.1016/j.jclepro.2019.06.174
Wang, Y., Xie, T., and Yang, S. (2017). Carbon emission and its decoupling research of transportation in Jiangsu province. J. Clean. Prod. 142, 907–914. doi:10.1016/j.jclepro.2016.09.052
Ward, J. D., Sutton, P. C., Werner, A. D., Costanza, R., Mohr, S. H., and Craig, T. S. (2016). Is decoupling GDP growth from environmental impact possible? PLoS One 11 (10), e0164733. doi:10.1371/journal.pone.0164733
Wiedenhofer, D., Virág, D., Kalt, G., Plank, B., Streeck, J., Pichler, M., et al. (2020). A systematic review of the evidence on decoupling of GDP, resource use and GHG emissions, Part I: bibliometric and conceptual mapping. Environ. Res. Lett. 15 (6), 063002. doi:10.1088/1748-9326/ab8429
Wu, Y, Chau, K. W., Lu, W., Shen, L., Shuai, C., and Chen, J. (2018a). Decoupling relationship between economic output and carbon emission in the Chinese construction industry. Environ. Impact Assess. Rev. 71, 60–69. doi:10.1016/j.eiar.2018.04.001
Wu, Y., Luo, Z., and Wu, Z. (2024). Exploring the relationship between urbanization and vegetation ecological quality changes in the Guangdong–Hong Kong–Macao greater bay area. Land 13 (8), 1246. doi:10.3390/land13081246
Wu, Y., Zhang, X., and Shen, L. (2011). The impact of urbanization policy on land use change: a scenario analysis. Cities 28 (2), 147–159. doi:10.1016/j.cities.2010.11.002
Wu, Y, Zhu, Q., and Zhu, B. (2018b). Decoupling analysis of world economic growth and CO2 emissions: a study comparing developed and developing countries. J. Clean. Prod. 190, 94–103. doi:10.1016/j.jclepro.2018.04.139
Xie, H., Zhu, Z., Wang, B., Liu, G., and Zhai, Q. (2018). Does the expansion of urban construction land promote regional economic growth in China? Evidence from 108 cities in the Yangtze River Economic Belt. Sustainability 10 (11), 4073. doi:10.3390/su10114073
Xie, X., Wang, J., and Liu, M. (2025). Regional disparities and driving factors of residential carbon emissions: an empirical analysis based on samples from 270 cities in China. Land 14 (3), 510. doi:10.3390/land14030510
Xie, Z., Wu, R., and Wang, S. (2021). How technological progress affects the carbon emission efficiency? Evidence from national panel quantile regression. J. Clean. Prod. 307, 127133. doi:10.1016/j.jclepro.2021.127133
Xin, L., Jia, J., Hu, W., Zeng, H., Chen, C., and Wu, B (2021). Decomposition and decoupling analysis of CO2 emissions based on LMDI and two-dimensional decoupling model in gansu province, China. Int. J. Environ. Res. Public Health 18 (11), 6013. doi:10.3390/ijerph18116013
Xin, M., Guo, H., Li, S., and Chen, L. (2023). Can China achieve ecological sustainability? An LMDI analysis of ecological footprint and economic development decoupling. Ecol. Indic. 151, 110313. doi:10.1016/j.ecolind.2023.110313
Xu, Q., Dong, Y.-X, and Yang, R. (2018). Urbanization impact on carbon emissions in the pearl River Delta region: kuznets curve relationships. J. Clean. Prod. 180, 514–523. doi:10.1016/j.jclepro.2018.01.194
Xu, W., Xie, Y., Xia, D., Ji, L., and Huang, G. (2021). A multi-sectoral decomposition and decoupling analysis of carbon emissions in Guangdong province, China. J. Environ. Manag. 298, 113485. doi:10.1016/j.jenvman.2021.113485
Yang, H., Ma, J., Jiao, X., Shang, G., and Yan, H. (2023). Characteristics and driving mechanism of urban construction land expansion along with rapid urbanization and carbon neutrality in Beijing, China. Land 12 (7), 1388. doi:10.3390/land12071388
Yang, M., and Xie, Y. (2021). Spatial pattern change and ecosystem service value dynamics of ecological and non-ecological redline areas in nanjing, China. Int. J. Environ. Res. Public Health 18 (8), 4224. doi:10.3390/ijerph18084224
Yuan, L., Li, R., Wu, X., He, W., Kong, Y., Ramsey, T. S., et al. (2023). Decoupling of economic growth and resources-environmental pressure in the Yangtze River Economic Belt, China. Ecol. Indic. 153, 110399. doi:10.1016/j.ecolind.2023.110399
Zeng, T., Jin, H., Geng, Z., Kang, Z., and Zhang, Z. (2022). The effect of urban shrinkage on carbon dioxide emissions efficiency in northeast China. Int. J. Environ. Res. Public Health 19 (9), 5772. doi:10.3390/ijerph19095772
Zhang, N., Yu, K., and Chen, Z. (2017). How does urbanization affect carbon dioxide emissions? A cross-country panel data analysis. Energy Policy 107, 678–687. doi:10.1016/j.enpol.2017.03.072
Zhang, X., and Han, H. (2024). Characteristics and factors influencing the expansion of urban construction land in China. Sci. Rep. 14 (1), 16040. doi:10.1038/s41598-024-67015-8
Zhao, X., Jiang, M., and Zhang, W. (2022). Decoupling between economic development and carbon emissions and its driving factors: evidence from China. Int. J. Environ. Res. Public Health 19 (5), 2893. doi:10.3390/ijerph19052893
Zhao, Y, Ma, J., Li, Y., Cheng, K., Zhang, M., Liu, Z., and Yang, F. (2025). Carbon emission based predictions of anthropogenic impacts on groundwater storage at typical basins in 2050. Sci. Partner J. 2025 0680. doi:10.34133/research.0680
Keywords: carbon emissions, urban expansion, urban expansion disparity index, Tapio model, LMDI model
Citation: Lin X, Liao Y and Wang S (2025) Spatiotemporal analysis of decoupling urban expansion and carbon emissions in China: insights from different expansion and decoupling patterns. Front. Environ. Sci. 13:1579001. doi: 10.3389/fenvs.2025.1579001
Received: 19 February 2025; Accepted: 15 April 2025;
Published: 12 May 2025.
Edited by:
Ji Zheng, The University of Hong Kong, Hong Kong SAR ChinaReviewed by:
Bingnan Guo, Jiangsu University of Science and Technology, ChinaMingshi Wang, Henan Polytechnic University, China
Copyright © 2025 Lin, Liao and Wang. This is an open-access article distributed under the terms of the Creative Commons Attribution License (CC BY). The use, distribution or reproduction in other forums is permitted, provided the original author(s) and the copyright owner(s) are credited and that the original publication in this journal is cited, in accordance with accepted academic practice. No use, distribution or reproduction is permitted which does not comply with these terms.
*Correspondence: Shaojian Wang, d2FuZ3NoajhAbWFpbC5zeXN1LmVkdS5jbg==