- 1State Key Laboratory of Hydraulics and Mountain River Engineering, Sichuan Universiry, Chengdu, China
- 2The National Key Laboratory of Water Disaster Prevention, Nanjing Hydraulic Research Institute, Nanjing, China
To evaluate the water replenishment effect of ecological sluices in the Tarim River’s mainstream and the water demand for ecological restoration, this study focused on the area controlled by a typical sluice group in the middle reaches. Using Landsat imagery (2000–2020) and the Penman-Monteith method, we quantified the spatiotemporal evolution of vegetation water consumption and ecological water demand, proposing corresponding sluice operation strategies. The results show: (1) From 2000 to 2020, natural vegetation consumed 1.62 × 108 m3 of water on average, increasing by 0.1 × 108 m3 per 5 years. Woodland and grassland expanded by 67.18 km2 per 5 years, demonstrating effective ecological restoration. (2) Vegetation water consumption varied spatiotemporally, peaking near sluice-fed branch canals and declining with distance from the river. The highest consumption occurred in 2015, concentrated in mid-growth periods. (3) Ecological water demands were: 1.76 × 108 m3 (maintaining 2020 conditions), 1.62 × 108 m3 (2000–2020 average), and 2.01 × 108 m3 (optimal 2015 level). By linking sluice impacts to vegetation water use and quantifying restoration needs, this study provides a scientific basis for water management and ecological regulation.
1 Introduction
Inland rivers are generally regarded as the main water resources in arid areas, and the desert riverbanks along them play a key role in resisting wind and sand, curbing desertification, and maintaining regional ecological balance (Keyimu et al., 2018; Sun et al., 2022). However, with the interferences such as global warming and human activities, the water resources of inland rivers in arid areas are facing increasingly severe pressure. At the same time, excessive land development and water resource exploitation have also hurt desert riparian ecosystems (Feng et al., 2001; Xu et al., 2008; Ye et al., 2014). At the end of the 20th century, to achieve the goals of ecological protection in arid areas and restoration of riparian vegetation, many regions worldwide carried out ecological water transfer work (Chen et al., 2010; Zeng et al., 2016; Zhao et al., 2020).
The Tarim River is located in the arid region of northwest China and is the longest inland river in China. It has the dual characteristics of rich natural resources and a fragile ecological environment. It is typical and representative in the study of inland river ecohydrology in arid areas. The Tarim River’s main stream surrounds the Taklimakan Desert’s northern part. The vegetation community, composed of trees, shrubs, and herbaceous plants along the river, is also a unique “green corridor” in Xinjiang. In the 1970s, with the decrease in water inflow of the Tarim River and the continuous increase in water consumption in the middle reaches, the lower reaches of the river and the Tetma Lake dried up, the groundwater level continued to drop, and the desert riverside forest dominated by Populus euphratica and Tamarix chinensis completely declined. Since 2000, the Chinese government has implemented large-scale ecological restoration, efficient water resource utilization, and water conservancy infrastructure projects in the Tarim River Basin (Zhang, 2022). In May of that year, to improve the deteriorating ecological conditions in the downstream area, the first ecological water conveyance project was launched in the lower reaches of the Tarim River (Jiao et al., 2022). Water dikes with a length of nearly 500 km were built next year on both sides of the middle reaches of the mainstream river to restrain the flooding during the flood season and improve the efficiency of water transfer to the downstream (Chen et al., 2013). The water dikes changed the water supply mode on both sides of the river and a series of ecological sluices were built on both sides of the dikes to maintain the meet ecological water supply for vegetation on both sides (Du et al., 2005). Finally, an ecological water transfer pattern has been basically formed in which water resources are transported by ecological sluices in the middle reaches of the Tarim River and water volume is dispatched by the Daxihaizi Reservoir in the lower reaches.
At present, the research on ecological water replenishment in the Tarim River Basin mainly focuses on two aspects: one is about the hydrological control mechanism of desert riparian forests, which exploring the impact of groundwater level changes caused by water transfer on vegetation individuals, populations, and communities (Chen et al., 2003; Deng et al., 2016; Keram et al., 2021; Li et al., 2013; Wang et al., 2023); Another one is to estimate the water demand of vegetation in desert riparian ecosystems, thereby providing suggestions and guidelines for ecological water transfer (Chen et al., 2008a; Ye et al., 2022; Ye et al., 2007). The joint dispatch of ecological sluices to replenish water is a unique means of water resource regulation in the Tarim River Basin. Water is mainly transported through water diversion channels. The farther away from the river, the smaller the vegetation density and the lower the vegetation coverage. Therefore, the variation of vegetation coverage can effectively evaluate the water replenishment effect of ecological sluices (Guo et al., 2017; Huang et al., 2020). In recent years, the relationship between land cover type change and ecological effects has received widespread attention, and vegetation restoration area has become an important indicator for evaluating ecological water transfer effects (Hu et al., 2019; Tian et al., 2020; Wang et al., 2021). The Tarim River Basin has scarce precipitation and its water cycle can be summarized as the process of “river water → groundwater → surface evapotranspiration” (Yuan et al., 2015). Evapotranspiration is the main form of water resource consumption here, which can reflect the ecological water demand of natural vegetation and provide a scientific basis for reasonable water resource allocation. Therefore, revealing the ecological water transfer effect and understanding the evapotranspiration and water consumption patterns of desert riparian forests are of great scientific significance for the current ecological management and restoration of the Tarim River Basin. We selected the water transfer range of a typical ecological sluice group in the middle reaches as the study area. Based on Landsat series remote sensing images, meteorological data, soil moisture content and other data, the land cover type and vegetation coverage in typical years from 2000 to 2020 were counted, and the spatiotemporal distribution and change trends were analyzed to explore the water transfer effect of the ecological sluices. The improved P-M method was used in combination with GIS technology to calculate the water consumption of natural vegetation in the control range of a typical ecological sluice group, and the ecological water demand was estimated, while corresponding water transfer strategies were proposed to provide scientific ideas for water resources management and desertification control in inland rivers in arid areas around the world.
2 Materials and methods
2.1 Study region
The study area is located in Yuli County, Bayingolin Mongolian Autonomous Prefecture, Xinjiang Uygur Autonomous Region, in the Yingbaza-Usman section of the middle reaches of the Tarim River (Figure 1). From west to east, it is controlled by an ecological sluice group consisting of the Yasenkadersluice, Patamusluice, Wushouhansluice, Xiadaisluice, and Yilanliksluice. The total area is about 1,084.79 km2. It belongs to the Tarim Populus euphratica National Nature Reserve in Xinjiang. The reserve has no cropland or construction land, so it is less affected by human factors and has no agricultural water use. The study area has a temperate continental warm temperate desert arid climate with abundant light and heat resources, an average annual sunshine of 2,574 h, an average annual temperature of 10.6°C, an extreme maximum temperature of 40.1°C, an extreme minimum temperature of −25.5°C, an average multi-year precipitation of 52.5 mm, and an average annual evaporation of 1,085 mm. Precipitation is scarce and evaporation is intense in this area, and atmospheric precipitation is negligible.
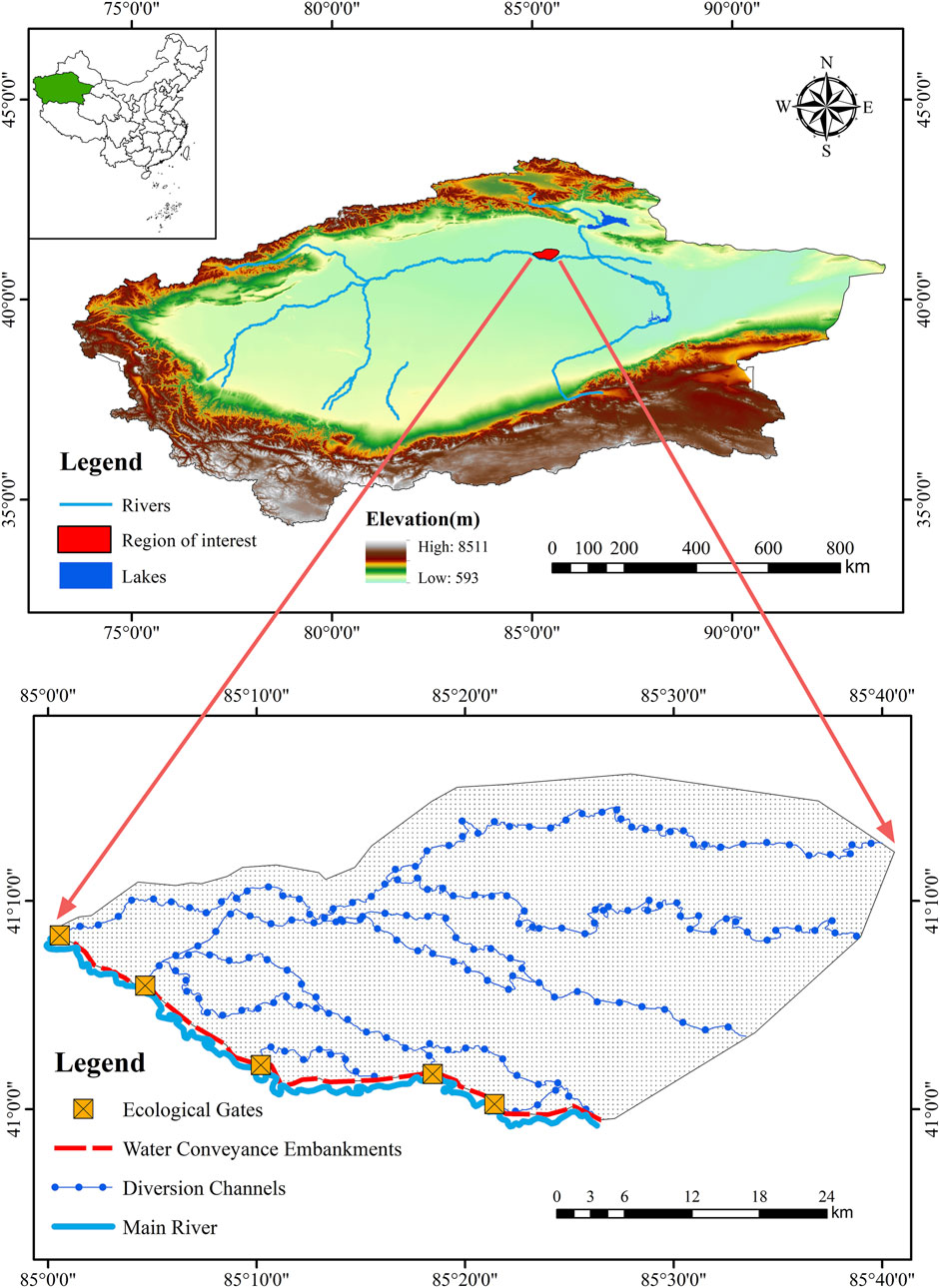
Figure 1. Map of study area The map is from the Chinese Standard Map (http://bzdt.ch.mnr.gov.cn/GS (accessed on 1 April 2023) (2019) 1822).
Due to the obstruction of water dikes on both sides of the main stream, the main way to replenish water in the area is through ecological sluices during the flood season. The shallow soil moisture content is greatly affected by the depth of groundwater. The growth of natural vegetation is seriously affected by water stress and is mainly composed of non-zonal vegetation that relies on groundwater for growth. Its species are single and distributed in a corridor-like manner along the water diversion channel. The main ones are Populus euphratica, Tamarix chinensis, Lycium barbarum, Bellthorn, Phragmites australis, Glycyrrhiza uralensis, etc., which constitute the communities of trees, shrubs and herbs.
2.2 Data sources and preprocessing
The data used in this study mainly include LANDSAT remote sensing satellite data, DEM data, meteorological data and ecological sluice water diversion data (Table 1). Among them, the meteorological data use the daily scale data of Tieganlik Meteorological Station which is closest to the study area, including daily rainfall, average temperature, average wind speed, sunshine hours and average relative humidity. For some unmonitored data, interpolation of data from adjacent periods is used to complete the data list. The remote sensing data screened the Landsat series remote sensing images with low cloud coverage from July to November 2000, 2005, 2010, 2015, and 2020, and performed preprocessing such as image cropping, radiation calibration, and atmospheric correction on the acquired remote sensing images using software such as ENVI5.3.
2.3 Research method
2.3.1 Trend analysis
The linear trend method is combined with GIS analysis to estimate the linear trend (K) of fractional vegetation cover (FVC) and water consumption changes per pixel, and quantitatively describe its spatiotemporal evolution characteristics on the grid scale (Zhang Q. et al., 2008). The calculation formula is as follows (Equations 1):
where K is the linear trend (when K > 0, it means that the variable value increases with the increase of year i; when K < 0, it means that the variable value decreases with the increase of year i); n is the length of the year sequence, n = 5 here; i is the specific calculation year sequence; xi is the variable value in the i-th year.
2.3.2 Estimation of FVC
Fractional vegetation cover is an important parameter to describe the surface vegetation coverage. The fractional vegetation cover estimated based on the Normalized Difference Vegetation Index (NDVI) is currently widely used (Zhang et al., 2013). The pixel binary model is a simple and practical model to estimate fractional vegetation cover. Since the vegetation species in the Tarim River Basin are relatively simple, the pixel binary model has its unique advantages in improving the accuracy of fractional vegetation cover. The calculation formula is as follows (Equations 2):
where F is the Fractional Vegetation Cover, INDV, soil is the pixel NDVI value in the area without vegetation coverage, and INDV,veg is the pixel NDVI value in the area completely covered by vegetation. INDV, soil and INDV,veg are theoretically 0 and 1, but considering the differences in surface environment, they cannot be directly assigned. In this paper, the minimum and maximum NDVI values within a certain confidence interval are intercepted by the raster data statistics tool in ENVI as INDV, soil and INDV,veg.
2.3.3 Vegetation water consumption calculation model
Evapotranspiration is the main water loss in the region. Therefore, the Penman-Monteith formula recommended by FAO 56 was used to calculate evapotranspiration to obtain vegetation water consumption (Allen et al., 2005). Due to drought and water shortage in the study area, the water in dry soils has a low potential energy and is strongly bound by capillary and absorptive forces to the soil matrix, and is less easily extracted by the crop. So the soil water stress conditions should be considered. The calculation method is as follows (Equations 3):
where ETC is the evapotranspiration, KS is the water stress coefficient (the specific calculation in section 2.3.5); Kc is the crop coefficient (the specific calculation in section 2.3.4); ET0 is the reference evapotranspiration (mm/d), which can be derived (Equations 4):
where ∆ is the slope vapour pressure curve (kPa·°C−1); Rn is the net radiation at the crop surface (MJ·m−2·d−1); G is the soil heat flux density (MJ·m−2·d−1); γ is the psychrometric constant (kPa·°C−1); T is the mean daily air temperature at 2 m height (°C); u2 is the wind speed at 2 m height (m·s−1); es is the saturation vapour pressure (kPa); ea is the actual vapour pressure (kPa); es - ea means the saturation vapour pressure deficit (kPa).
2.3.4 Crop coefficient (Kc)
Based on remote sensing images and previous research data (Bai et al., 2014), the supervised classification method was used to determine the land cover type in the lower reaches of the Tarim River. Typical vegetation of different land covers was selected, and according to the method recommended by FAO-56 (Allen et al., 1998), the vegetation growth cycle was divided into four periods: early growth period, developmental period, mid-growth period and late growth period (Table 2).
The vegetation coefficient (Kc), is a crucial parameter reflecting the water consumption capacity of vegetation, and its curve illustrates the water consumption process throughout the entire vegetative cycle. The initial growth stage’s vegetation coefficient (
2.3.5 Water stress coefficient (Ks)
The soil moisture stress coefficient (Ks), is a crucial indicator reflecting the water surplus or deficit during vegetation growth, determined by factors such as soil moisture content at the root zone. The determination of Ks references the method outlined in FAO-56 for natural vegetation water consumption under conditions of partial irrigation and water stress (Allen et al., 1998). The necessary depth of vegetation roots is established according to research by Zhang et al. (2003), while field capacity (θFC) follows the methodology proposed by Saxton et al. (1986), and the wilting point (θWP) is derived from the approach suggested by Qiao (2008). Parameters such as soil clay content are determined based on soil particle analysis results (refer to Table 3). The soil moisture content at the root depth for each land cover type is determined through on-site drying experiments, and the distribution of soil moisture content with depth is outlined in Table 4. Based on this data, the soil moisture stress coefficient (Ks), for various land cover types in the midstream of the Tarim River is determined (refer to Table 5).
3 Results and analysis
3.1 Spatial and temporal evolution of land cover patterns
3.1.1 Spatial and temporal changes in woodland, grassland and desert areas
The distribution of land cover types in the study area is shown in Figure 2. The average area of desert from 2000 to 2020 was 491.55 km2, accounting for 45.31% of the control area behind the ecological sluices. Woodland and grassland are mainly distributed along rivers and overflow areas, where vegetation expansion was directly driven by water release from ecological sluices. The average area of woodland is 109.59 km2 with a proportion of 10.1% and the grassland area is 398.37 km2 with a proportion of 36.72%, a trend attributed to sustained ecological water supply promoting natural revegetation. The water area is affected by the ecological sluices and the average area is 85.28 km2 with a proportion of 7.86%. Over the past 21 years, the areas of desert and natural vegetation have changed significantly (Figure 3). Among them, the desert area decreased at a rate of 67.32 km2/5 years and dropped to 32.18% of the study area by 2020. The woodland area increased at a rate of 15.93 km2/5 years and rose to 12.09% of the study area by 2020. The grassland area increased at a rate of 51.25 km2/5 years and as of 2020, the proportion has increased to 49.22% of the study area. This transformation was primarily driven by long-term water release from the ecological sluices, which improved hydrological conditions and promoted natural vegetation regeneration.
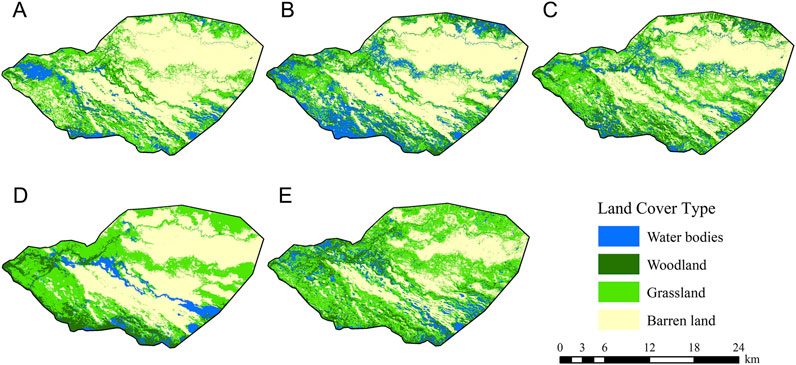
Figure 2. Land cover types in the Tarim River Basin from 2000 to 2020: (A) 2000; (B) 2005; (C) 2010; (D) 2015 and (E) 2020.
3.1.2 Analysis of land-use structural changes
GIS technology was used to intersect the land cover type data in 2000 and 2020 to obtain the area transfer map (Figure 4). It can be seen that in the past 21 years (Table 6), 261.02 km2 of desert area was converted into woodland or grassland (accounting for 95.08% of the proportion of desert area converted into other land cover types). There is also a mutual conversion relationship between grassland and woodland, of which 70.22 km2 of grassland was converted into woodland (accounting for 69.14% of the proportion of grassland converted into other land cover types), and 21.73 km2 of woodland was converted into grassland (accounting for 70.28% of the proportion of grassland converted into other land cover types). Overall, through years of water transfer by ecological sluices, a large amount of desert area far away from the main stream was converted into woodland and grassland, and part of grassland was converted into woodland. The area of natural vegetation in the study area showed a significant increase.
3.1.3 Accuracy evaluation of remote sensing interpretation
Accuracy evaluation involves comparing field survey data with remote sensing classification results to assess the precision and reliability of the classification. Based on the ENVI platform, the confusion matrix tool was employed to evaluate classification accuracy. The confusion matrix is an array that contrasts the number of actual pixels with the number of classified pixels, presented as an n × n matrix. It is one of the most commonly used computational tools for assessing image classification results.
The accuracy validation results obtained from the confusion matrix tool in the ENVI platform are shown in Table 7. The overall accuracy of image extraction reached 99.69%, with a Kappa coefficient of 0.994, indicating that the remote sensing interpretation classification has high accuracy.
3.2 Spatial and temporal changes in FVC
Affected by water stress, the overall fractional vegetation cover in the middle reaches of the Tarim River is relatively low, and the vegetation grows poorly under natural growth conditions. Therefore, the changes in fractional vegetation cover can clearly reflect the effect of water transfer from the ecological sluices. Based on the spatial distribution of fractional vegetation cover in the Tarim River (Figure 5), the fractional vegetation cover of the corresponding year was extracted using the land cover type maps interpreted by remote sensing in different years. Then, the average fractional vegetation cover of each land cover type was determined through spatial analysis and geographic statistical methods (Figure 6). The average coverage over the years was 85.51% for woodland, 60.11% for grassland, and 15.3% for desert. Over the past 21 years, the fractional vegetation cover of different land cover types has shown a slow increase.
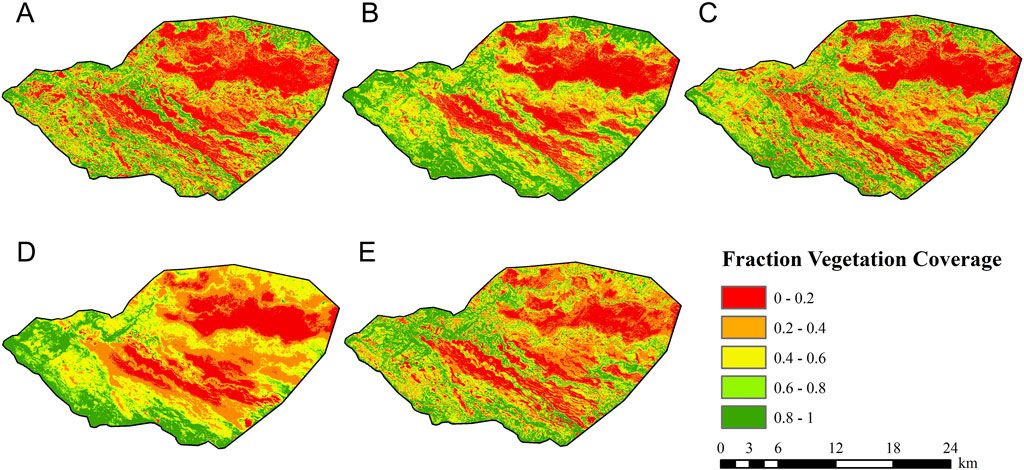
Figure 5. The spatial distribution of vegetation coverage in the study area from 2000 to 2020: (A) 2000; (B) 2005; (C) 2010; (D) 2015 and (E) 2020.
3.3 Spatial and temporal changes in vegetation water consumption
3.3.1 Changing trends of daily water consumption of different vegetation types
According to Table 8, the vegetation water consumption in the study area varies significantly among land cover types. The average water consumption in the mid-growth period is 3.95 mm/d for woodland, 1.44 mm/d for grassland, and 0.48 mm/d for desert area, showing a pattern of woodland > grassland > desert area, and it remains consistent in many years. This indicates that the natural vegetation in the lower reaches of the Tarim River is subject to long-term water stress under natural growth. As for the average annual water consumption of the desert riverbank vegetation in the Tarim River (Figure 7), it remains stable in the early growth period, and the water consumption fluctuates greatly in the development period, the mid-growth period and the late growth period, showing an overall upward trend. The main reason is that in the development and growth stage of the desert riverbank vegetation, the timely release of water from the ecological sluices promotes the vegetation growth and increases the vegetation coverage area. The effective water replenishment of the ecological sluices is the main driving factor leading to the changes in water consumption.
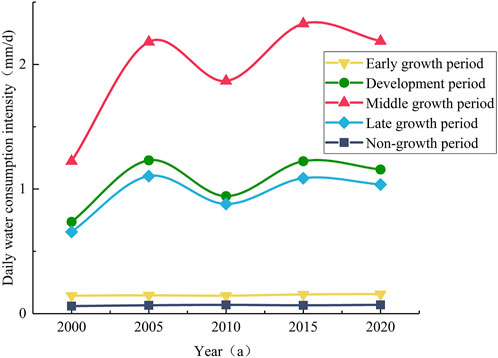
Figure 7. Interannual variation trend of daily water consumption of natural vegetation in different growth stages.
3.3.2 Analysis of spatial and temporal evolution trend of vegetation water consumption
The total vegetation water consumption in the study area showed an increasing trend from 2000 to 2020 (Table 9), among which the water consumption of woodland and grassland showed an increasing trend, while the desert vegetation showed a decreasing trend. In 2020, the vegetation overall water consumption increased by 0.37 × 108 m3 compared with the multi-year average vegetation water consumption which was 1.43 × 108 m3. The woodland and grassland increased by 0.43 × 108 m3, while the desert vegetation decreased by 0.06 × 108 m3. The increase in water consumption of woodland land and grassland was the main reason for the increase in total water consumption.
The spatial distribution of vegetation water consumption is obviously different (Figure 8). The high values are mainly concentrated in the main stream, along the water diversion channel and in the natural overflow area, while the low values are mainly distributed in the desert area. As the desert area decreases, the area of low values also decreases. This shows that the main factor leading to the reduction of desert vegetation water consumption is the change in land cover types.
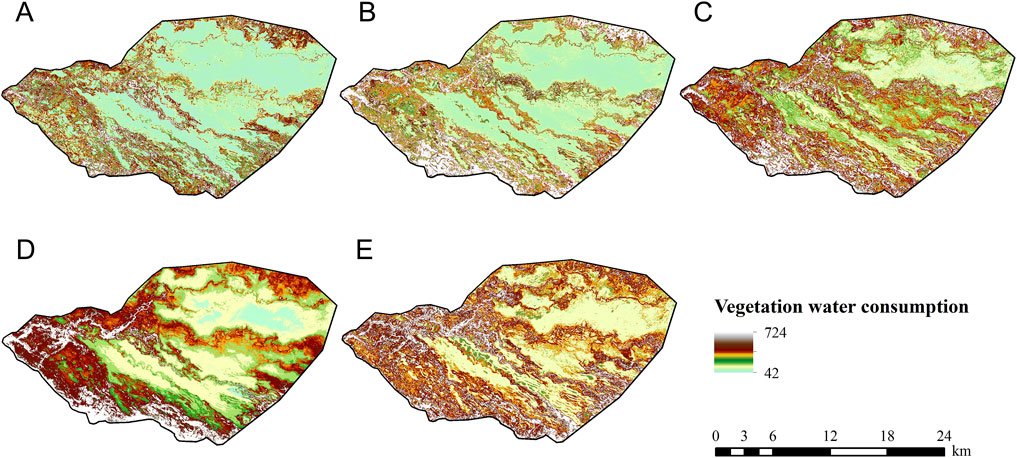
Figure 8. Spatial distribution of vegetation water consumption from 2000 to 2020: (A) 2000; (B) 2005; (C) 2010; (D) 2015 and (E) 2020.
According to the trend in Slope changes (Figure 9), it is evident that over a span of 21 years, there has been a significant increase in vegetation water consumption near the canal. Within a 1 km range on either side of the canal, there’s a slight increase in vegetation water consumption, while the areas farther away from the canal show minimal change in vegetation water consumption. This indicates that over many years, the ecological sluice, by releasing water during the flood season and diverting water through the downstream canal, has effectively reduced the desert area. It has expanded the extent of forest and grassland, effectively increasing the vegetation water consumption along the desert riverbanks adjacent to the canal.
4 Discussion
After nearly two decades of ecological restoration, significant changes have occurred in the land cover types of the Tarim River Basin, primarily characterized by an increase in forest and grassland areas, while desert areas have been substantially reduced. This change aligns with the findings of Hou et al. (2022), who used CLCD remote sensing data products to analyze the spatial-temporal patterns of land cover changes over the past 30 years in the basin. Meanwhile, the water consumption of riparian forest vegetation in desert areas has continuously increased, a trend that is consistent with the results of Thevs et al. (2015), who used MODIS remote sensing products to analyze the water consumption of natural vegetation in the Aksu-Tarim River Basin from 2009 to 2011. However, this study has certain limitations. For instance, the impact of climate variability on vegetation water consumption was not explicitly considered, which may introduce uncertainties in long-term trend analysis. Additionally, the remote sensing-based PM (Penman-Monteith) method for estimating vegetation water consumption lacks validation against ground-measured data, potentially affecting the accuracy of the results.
At present, most studies, both domestic and international, focus on the ecological hydrology of the downstream or the entire basin. However, research specifically addressing the role of ecological sluice irrigation, a unique water allocation method in the Tarim River Basin, remains limited. Wu et al. (2024) utilized the Google Earth Engine (GEE) platform to investigate the response of surface water area (SWA) to ecological sluice water delivery and its relationship with vegetation restoration. This study, using remote sensing imagery, focuses on analyzing the spatiotemporal evolution of natural vegetation water consumption within the control range of the middle reach ecological sluice group, providing a detailed examination of the irrigation effect of ecological sluices and filling a research gap in this area. Future studies can build on the analysis of ecological water demand and irrigation modes to provide scientific support for ecological scheduling decisions and further optimize water resource management strategies.
4.1 Determination of water requirements for ecological restoration
At present, the concept of ecological water demand has not been unified, and there are different understandings in practical applications (Zhang L. et al., 2008). The general understanding is that the water consumption of natural vegetation under standard conditions where growth or evaporation is not restricted. In actual situations, due to insufficient water supply, soil restrictions and other reasons, the normal growth of natural vegetation may be subject to certain restrictions. The actual evaporation of natural vegetation is the ecological vegetation water consumption (Zhang et al., 2021). Considering that the traditional ecological vegetation water demand is the growth pattern of vegetation under ideal conditions, it is difficult to achieve it in the entire region in the actual situation of arid areas due to restrictions such as water resources and soil moisture content. Therefore, based on the study of the evolution characteristics of regional long-series vegetation water consumption, this paper follows the technical route of historical scenario analysis and natural landscape reproduction, and through a combined analysis of typical annual vegetation water consumption, the water demand for ecological restoration of desert riverbank vegetation under different scenario objectives is obtained.
Taking the vegetation water consumption of five typical years as reference, it is divided into three restoration goals: maintaining the current ecological status (2020), reaching the 20-year ecological average level from 2000 to 2020, and reaching the 20-year ecological optimal level (2015). The water demand under different ecological restoration goals (Table 10) shows that the water demand for maintaining the current ecological status is 1.76 × 108 m3, the water demand for reaching the 20-year ecological average level is 1.62 × 108 m3, and the water demand for reaching the 20-year ecological optimal level is 2.01 × 108 m3.
4.2 Water replenishment strategy of ecological sluices
As an important project to regulate the main stream of the Tarim River, the ecological sluices and the water dikes on both sides have changed the previous water supply method. On the basis of meeting the needs of farmland irrigation water, the ecological sluices are used for ecological water replenishment, and the ecological water supply is improved. Ecological areas outside the mainstream rivers can be provided with water supply on a “point-by-point basis”. On the premise of ensuring water transfer to the downstream, the ecological vegetation outside the scope on both sides of the main stream has been restored to the maximum extent.
4.2.1 Water replenishment range
In order to increase the guaranteed of water transfer from the main stream to the downstream, the total amount of water resources in the upper and middle reaches of the ecological sluice is limited. In the process of ecological restoration, it is necessary to balance the constraints and competition between agricultural and ecological water use. Instead of blindly pursuing the expansion of the ecological area, the ineffective dissipation of water should be avoided as much as possible, and the protection of vegetation diversity and habitats of unique species should be achieved by maintaining an appropriate oasis scale.
4.2.2 Water delivery time
By processing the water diversion data of the ecological sluices in the study area in the past 10 years (Figure 10), it can be seen that the planting time in the Tarim River Basin lasts from April to December, and shows a decreasing trend on both sides with August as the central axis. The water delivery time and volume of the ecological sluices are concentrated in August and September. During other periods, the amount of water flowing into the ecological area behind the sluices is less or even almost non-existent.
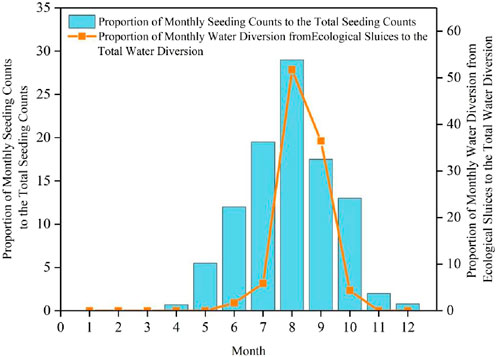
Figure 10. The annual distribution ratio of the number of vegetation species planting and the monthly water delivery of the ecological sluices.
In recent years, as the flood season of the Tarim River has been coming earlier and earlier, the multi-period combined ecological sluices dispatching mode can be adopted for better results. The first stage is April. During this period, due to the spring flood caused by the melting of ice and snow in winter, the use of ecological sluices can use part of the ecological water to meet the needs of the initial growth of vegetation. The second stage of water transfer time is from July to September. Summer and autumn are the main flood seasons of the Tarim River. In addition to the factors of melting ice and snow, there is also the increase in summer precipitation. On the premise of meeting agricultural water use and downstream water transfer rates, a large amount of ecological water can be used to meet the needs of the mid-term growth of vegetation, achieving the ecological fit between the water transfer time and the time of vegetation planting.
5 Conclusion
This paper selected a typical ecological sluices group in the middle reaches of the Tarim River as the research object, and took the range of ecological sluices recharge as the research area. From the perspective of land cover type transfer, fractional vegetation cover, and vegetation water consumption change, the functions and effects of ecological sluices were explained, and a reasonable water requirement for ecological restoration was proposed. The main research conclusions are as follows:
(1) The P-M method was systematically applied to the middle reaches of the Tarim River. The water consumption of natural vegetation was calculated on a 30 m spatial grid. The crop coefficient Kc of various land uses was determined to be 0.85 for woodland, 0.44 for grassland, and 0.21 for desert vegetation. The water stress coefficient was 0.78 for woodland, 0.54 for grassland, and 0.36 for desert vegetation. This is a basic achievement in the study of vegetation restoration and the evaluation of ecological water transfer effects in the middle reaches of the Tarim River.
(2) After years of water transfer through the ecological sluices, the woodland and grassland behind the sluices increased from 396.32 km2 in 2000 (36.53% of the total area) to 507.96 km2 (47.02%) in 2020; the woodland and grassland coverage increased from 82.4% to 11.74% to 86.2% to 16.6% respectively. The water consumption of natural vegetation increased from 1.35 × 108 m3/a to 1.76 × 108m3/a. The changes in land cover types and the improvement of natural vegetation show good feedback on the water delivery effect of the ecological sluices, indicating that the water replenishment effect of the ecological sluices is significant.
(3) From 2000 to 2020, the average annual natural vegetation water consumption in the area behind the sluices was 1.62 × 108m3/a, of which woodland water consumption was 0.61 × 108m3/a, grassland water consumption was 0.62 × 108m3/a, and desert water consumption was 0.39 × 108m3/a. The spatial water consumption was limited by the constraints of the existing water transfer method. The vegetation water consumption was mainly concentrated along the branch canal and the overflow area. The overflow range can last until winter, which aggravates the ineffective evaporation of the water surface and causes a large number of original vegetation to freeze to death. It is necessary to make corresponding improvements to the ecological water transfer strategy according to the changes in water consumption methods.
Data availability statement
The original contributions presented in the study are included in the article/supplementary material, further inquiries can be directed to the corresponding author.
Author contributions
SF: Conceptualization, Data curation, Formal Analysis, Writing – original draft. YX: Funding acquisition, Writing – review and editing. RS: Methodology, Resources, Writing – review and editing. XH: Supervision, Writing – review and editing. YW: Project administration, Writing – review and editing. GW: Data curation, Software, Writing – original draft, Funding acquisition.
Funding
The author(s) declare that financial support was received for the research and/or publication of this article. This study was financially supported by the National Key Research and Development Program of China (grant no.2021YFB3900602), the National Natural Science Foundation of China (grant no.42075191, grant no.52009080).
Conflict of interest
The authors declare that the research was conducted in the absence of any commercial or financial relationships that could be construed as a potential conflict of interest.
Generative AI statement
The author(s) declare that no Generative AI was used in the creation of this manuscript.
Publisher’s note
All claims expressed in this article are solely those of the authors and do not necessarily represent those of their affiliated organizations, or those of the publisher, the editors and the reviewers. Any product that may be evaluated in this article, or claim that may be made by its manufacturer, is not guaranteed or endorsed by the publisher.
References
Allen, R. G., Pereira, L. S., Raes, D., and Smith, M. (1998). Crop evapotranspiration. Guidelines for computing crop water requirements. J. Hydrology 285, 19–40.
Allen, R. G., Pereira, L. S., Smith, M., Raes, D., and Wright, J. L. (2005). FAO-56 dual crop coefficient method for estimating evaporation from soil and application extensions. J. Irrig. Drain. Eng-ASCE 131, 2–13. doi:10.1061/(asce)0733-9437(2005)131:1(2)
Bai, Y., Xu, H. L., Ling, H. B., and Wang, X. Y. (2014). Spatial distribution characteristics and ecological water requirementof natural vegetation along the mainstream of the Tarim River. J. Desert Res. 34, 1410–1416. (In Chinese). doi:10.7522/j.issn.1000-694X.2013.00436
Chen, Y. J., Li, W. H., Liu, J. Z., and Yang, Y. H. (2013). Effects of water conveyance embankments on riparian forest communities at the middle reaches of the Tarim River, Northwest China. Ecohydrology 6, 937–948. doi:10.1002/eco.1418
Chen, Y. N., Chen, Y. P., Xu, C. C., Ye, Z. X., Li, Z. Q., Zhu, C. G., et al. (2010). Effects of ecological water conveyance on groundwater dynamics and riparian vegetation in the lower reaches of Tarim River, China. Hydrol. Process. 24, 170–177. doi:10.1002/hyp.7429
Chen, Y. N., Hao, X. M., Li, W. H., chen, y.p., Ye, Z. X., and Zhao, R. F. (2008a). An Analysis of the ecological security and ecological water requirements in the inland river of arid region. Adv. Earth Sci. 23, 732–738. (In Chinese). doi:10.11867/J.ISSN.1001-8166.2008.07.0732
Chen, Y. N., Li, W. H., Xu, H. L., Liu, J. Z., Zhang, H. F., and Chen, Y. P. (2003). The influence of groundwater on vegetation in the lower reaches of Tarim River, China. Acta Geogr. Sin. 58, 542–549. doi:10.3321/j.issn:0375-5444.2003.04.008
Deng, m.J., Zhou, H. Y., Xu, H. L., Ling, H. B., and Zhang, P. (2016). Research on the ecological operation in the lower reaches of Tarim River based on water conveyance. Sci. Sin. Technol. 46, 864–876. (In Chinese). doi:10.1360/N092015-00263
Du, H., He, Y. H., and Qiao, J. P. (2005). Brief talk about the design features of Tarim River ecological gates project. Yellow River 27, 54–55. (In Chinese). doi:10.3969/j.issn.1000-1379.2005.02.025
Feng, Q., Endo, K. N., and Cheng, G. D. (2001). Towards sustainable development of the environmentally degraded arid rivers of China - a case study from Tarim River. Environ. Geol. 41, 229–238. doi:10.1007/s002540100387
Guo, H., Jiapaer, G., Bao, A. M., Li, X. Y., Huang, Y., Ndayisaba, F., et al. (2017). Effects of the Tarim River's middle stream water transport dike on the fractional cover of desert riparian vegetation. Ecol. Eng. 99, 333–342. doi:10.1016/j.ecoleng.2016.10.074
Hou, Y., Chen, Y., Ding, J., Li, Z., Li, Y., and Sun, F. (2022). Ecological impacts of land use change in the arid Tarim River Basin of China. Remote Sens. 14, 1894. doi:10.3390/rs14081894
Hu, M. M., Li, Z. T., Wang, Y. F., Jiao, M. Y., Li, M., and Xia, B. C. (2019). Spatio-temporal changes in ecosystem service value in response to land-use/cover changes in the Pearl River Delta. Resour. Conservation Recycl. 149, 106–114. doi:10.1016/j.resconrec.2019.05.032
Huang, F., Ochoa, C. G., Chen, X., Cheng, Q. B., and Zhang, D. R. (2020). An entropy-based investigation into the impact of ecological water diversion on land cover complexity of restored oasis in arid inland river basins. Ecol. Eng. 151, 105865. doi:10.1016/j.ecoleng.2020.105865
Jiao, A. Y., Wang, W. Q., Ling, H. B., Deng, X. Y., Yan, J. J., and Chen, F. L. (2022). Effect evaluation of ecological water conveyance in Tarim River Basin, China. Front. Environ. Sci. 10, 16. doi:10.3389/fenvs.2022.1019695
Keram, A., Halik, U., Aishan, T., Keyimu, M., Jiapaer, K., and Li, G. L. (2021). Tree mortality and regeneration of Euphrates poplar riparian forests along the Tarim River, Northwest China. For. Ecosyst. 8, 49. doi:10.1186/s40663-021-00323-x
Keyimu, M., Halik, U., Betz, F., and Dulamsuren, C. (2018). Vitality variation and population structure of a riparian forest in the lower reaches of the Tarim River, NW China. J. For. Res. 29, 749–760. doi:10.1007/s11676-017-0478-4
Li, B. F., Chen, Y. N., Li, W. H., Chen, Z. S., Zhang, B. H., and Guo, B. (2013). Spatial and temporal variations of temperature and precipitation in the arid region of northwest China from 1960-2010. Fresenius Environ. Bull. 22, 362–371. doi:10.1007/s00704-012-0753-4
Qiao, Z. H. (2008). Study on the influence factors of wilting point. J. Water Resour. and Water Eng. 19, 82–84. (In Chinese). doi:10.1163/156939308783122788
Saxton, K. E., Rawls, W. J., Romberger, J. S., and Papendick, R. I. (1986). Estimating generalized soil-water characteristics from texture. Soil Sci. Soc. Am. J. 50, 1031–1036. doi:10.2136/sssaj1986.03615995005000040054x
Sun, H. T., Wang, P., Chen, Q., Zhang, D. P., and Xing, Y. H. (2022). Coupling the water use of Populus euphratica and Tamarix ramosissima and evapotranspiration partitioning in a desert riparian forest ecosystem. Agric. For. Meteorol. 323, 109064. doi:10.1016/j.agrformet.2022.109064
Thevs, N., Peng, H. Y., Rozi, A., Zerbe, S., and Abdusalih, N. (2015). Water allocation and water consumption of irrigated agriculture and natural vegetation in the Aksu-Tarim river basin, Xinjiang, China. J. Arid Environ. 112, 87–97. doi:10.1016/j.jaridenv.2014.05.028
Tian, S. Q., Wang, S. J., Bai, X. Y., Zhou, D. Q., Luo, G. J., Yang, Y. J., et al. (2020). Ecological security and health risk assessment of soil heavy metals on a village-level scale, based on different land use types. Environ. Geochem. Health 42, 3393–3413. doi:10.1007/s10653-020-00583-6
Wang, S. S., Zhou, K. F., Zuo, Q. T., Wang, J. L., and Wang, W. (2021). Land use/land cover change responses to ecological water conveyance in the lower reaches of Tarim River, China. J. Arid. Land 13, 1274–1286. doi:10.1007/s40333-021-0089-y
Wang, Y. H., Li, J., Qian, K. X., and Ye, M. (2023). Response of plant species diversity to flood irrigation in the Tarim River Basin, northwest China. Sustainability 15, 1243. doi:10.3390/su15021243
Wu, J., Gao, F., He, B., Sheng, F., Xu, H., Liu, K., et al. (2024). Ecological gate water control and its influence on surface water dynamics and vegetation restoration: a case study from the middle reaches of the Tarim River. Forests (19994907), 15. doi:10.3390/f15112005
Xu, H. L., Ye, M., and Li, J. M. (2008). The water transfer effects on agricultural development in the lower Tarim River, Xinjiang of China. Agric. Water Manage. 95, 59–68. doi:10.1016/j.agwat.2007.09.004
Ye, Z. X., Chen, S. F., Zhang, Q. F., Liu, Y. C., and Zhou, H. H. (2022). Ecological water demand of taitema lake in the lower reaches of the Tarim River and the cherchen river. Remote Sens. 14, 832. doi:10.3390/rs14040832
Ye, Z. X., Chen, Y. N., and li, H. W. (2007). Ecological water demand of vegetation based on eco-hydrological process in the lower reaches of Tarim River. Acta Geogr. Sin. 62, 451–461. (In Chinese). doi:10.11821/xb200705001
Ye, Z. X., Chen, Y. N., and Li, W. H. (2014). Ecological water rights and water-resource exploitation in the three headwaters of the Tarim River. Quat. Int. 336, 20–25. doi:10.1016/j.quaint.2013.09.029
Yuan, G. F., Luo, Y., Shao, M. A., Zhang, P., and Zhu, X. C. (2015). Evapotranspiration and its main controlling mechanism over the desert riparian forests in the lower Tarim River Basin. Sci. China-Earth Sci. 58, 1032–1042. doi:10.1007/s11430-014-5045-7
Zeng, Y. J., Xie, Z. H., Yu, Y., Liu, S., Wang, L. Y., Jia, B. H., et al. (2016). Ecohydrological effects of stream-aquifer water interaction: a case study of the Heihe River basin, northwestern China. Hydrol. Earth Syst. Sci. 20, 2333–2352. doi:10.5194/hess-20-2333-2016
Zhang, A. M., Hao, T. P., Zhou, H. P., Ma, Z. B., and Cui, S. S. (2021). Analysis on characteristics of Baiyang River Basin and water requirement of ecological vegetation in Xinjiang. Acta Ecol. Sin. 41, 1921–1930. (In Chinese). doi:10.5846/stxb201911092362
Zhang, L., Dong, Z. C., and Zhao, B. (2003). Method for estimating ecological water requirement of natural vegetation in arid area. Adv. Water Sci. 14, 745–748. (In Chinese). doi:10.14042/j.cnki.32.1309.2003.06.014
Zhang, L., Li, L. J., Liang, L. Q., Li, J. Y., Jiang, D. J., Xu, M. X., et al. (2008a). Progress on the research of theory and calculation method of ecological water requirement. Trans. CSAE 07, 37–312. (In Chinese). doi:10.3901/JME.2008.09.177
Zhang, Q. (2022). Adhering to system concept and overall coordination, promoting the governance and management capability of the Tarim River Basin to a new level. Water Resour. Dev. Res. 22, 34–38. (In Chinese). doi:10.13928/j.cnki.wrdr.2022.11.009
Zhang, Q., Xu, C. Y., Zhang, Z., Chen, Y. D., Liu, C. L., and Lin, H. (2008b). Spatial and temporal variability of precipitation maxima during 1960-2005 in the Yangtze River basin and possible association with large-scale circulation. J. Hydrology 353, 215–227. doi:10.1016/j.jhydrol.2007.11.023
Zhang, X., Liao, C., Li, J., and Sun, Q. (2013). Fractional vegetation cover estimation in arid and semi-arid environments using HJ-1 satellite hyperspectral data. Int. J. Appl. Earth Observation Geoinformation 21, 506–512. doi:10.1016/j.jag.2012.07.003
Keywords: Tarim River, ecological sluices, fractional vegetation cover, vegetation water consumption, water transfer effect
Citation: Feng S, Xu Y, Shi R, Huang X, Wu Y and Wang G (2025) Analysis on water replenishment effect of ecological sluices in the middle reaches of the Tarim River. Front. Environ. Sci. 13:1586277. doi: 10.3389/fenvs.2025.1586277
Received: 02 March 2025; Accepted: 28 April 2025;
Published: 20 May 2025.
Edited by:
Chong Jiang, Guangdong Academy of Science (CAS), ChinaReviewed by:
Abdulla Abliz, Xinjiang University, ChinaJie Wu, University of Chinese Academy of Sciences, China
Copyright © 2025 Feng, Xu, Shi, Huang, Wu and Wang. This is an open-access article distributed under the terms of the Creative Commons Attribution License (CC BY). The use, distribution or reproduction in other forums is permitted, provided the original author(s) and the copyright owner(s) are credited and that the original publication in this journal is cited, in accordance with accepted academic practice. No use, distribution or reproduction is permitted which does not comply with these terms.
*Correspondence: Gaoxu Wang, Z3h3YW5nQG5ocmkuY24=