- 1Grupo de Pesquisa Ecologia, Monitoramento e uso Sustentável de Areas Úmidas (MAUA), Instituto Nacional de Pesquisas da Amazônia (INPA), Manaus, Brazil
- 2Department of Wetland Ecology, Institute of Geography and Geoecology, Karlsruhe Institute of Technology (KIT), Karlsruhe, Germany
- 3Department of Biodiversity (CBIO), National Institute for Amazonian Research (INPA), Manaus, Brazil
- 4Museu Paraense Emílio Goeldi, Belém, Brazil
- 5National Institute of Amazonian Research (INPA), Manaus, Brazil
- 6Corporación para Investigaciones Biológicas (CIB), Medellín, Colombia
- 7Jardim Botânico do Rio de Janeiro, Rio de Janeiro, Brazil
- 8Laboratório de Ecologia, Departamento de Meio Ambiente e Desenvolvimento, Universidade Federal do Amapá, Macapá, Brazil
- 9New York Botanical Garden, New York, NY, United States
- 10Departamento de Ciencias Forestales, Universidad Nacional de Colombia Sede Medellín, Medellín, Colombia
- 11Programa de Pós-Graduação em Biodiversidade Tropical, Universidade Federal do Amapá, Macapá, Brazil
- 12Quantitative Biodiversity Dynamics, Utrecht University Botanic Gardens, Utrecht University, Utrecht, Netherlands
- 13Fort Worth Botanical Garden and Botanical Research Institute of Texas, Fort Worth, TX, United States
- 14Naturalis Biodiversity Center, Leiden, Netherlands
- 15Independent Researcher, Amsterdam, Netherlands
- 16Institute for Biodiversity and Ecosystem Dynamics, University of Amsterdam, Amsterdam, Netherlands
- 17Naturalis Biodiversity Center, Institute of Environmental Sciences, Leiden University, Leiden, Netherlands
- 18Instituto de Ecología Regional, Universidad Nacional de Tucumán – Consejo Nacional de Investigaciones Científicas y Técnicas (CONICET), Yerba Buena, Argentina
Epiphytes are still an understudied plant group in Amazonia. The aim of this study was to identify distributional patterns and conservation priorities for vascular epiphyte assemblages (VEA) across Amazonia. We compiled the largest Amazonian epiphyte plot database to date, through a multinational collaborative effort of 22 researchers and 32 field sites located across four Amazonian countries – the Amazonian Epiphyte Network (AEN). We addressed the following continental-scale questions by utilizing the AEN database comprising 96,448 epiphyte individuals, belonging to 518 vascular taxa, and growing on 10,907 tree individuals (phorophytes). Our objectives here are, first, to present a qualitative evaluation of the geographic distribution of the study sites and highlight regional lacunae as priorities for future quantitative inventories. Second, to present the floristic patterns for Amazonia-wide VEA and third, to combine multivariate analyses and rank abundance curves, controlled by major Amazonian habitat types, to determine how VEA vary geographically and ecologically based on major Amazonian habitat types. Three of the most striking patterns found are that: (1) VEA are spatially structured as floristic similarity decays with geographic distance; (2) a core group of 22 oligarchic taxa account for more than a half of all individuals; and (3) extensive floristic sampling gaps still exist, mainly across the highly threatened southern Amazonian deforestation belt. This work represents a first step toward unveiling distributional pattern of Amazonian VEA, which is important to guide future questions on ecology and species distribution ranges of VEA once the collaborative database grows allowing a clearer view of patterns.
Introduction
Amazonia, here defined as the tropical moist forest of the Amazon basin and the Guiana Shield, occupies approximately 40% of South America, and is home to the largest expanse of the world’s remaining contiguous tropical rain forest harboring an estimated 50,000 seed plant species, of which 14–16,000 are estimated to be trees (ter Steege et al., 2013, 2020). The Amazon basin supports approximately 40% of the world’s remaining rainforest, accounts for some 10% of global terrestrial primary productivity, provides 16–18% of the world’s freshwater and harbors 10% of the world’s plant species, of which over half are considered endemic to the region (Latrubesse et al., 2017; Science Panel for the Amazon, 2021). Such astounding numbers are attributed to the regions’ continental size, high levels of primary productivity, spatial variability in edaphic and climatic conditions, and long-term geological stability (Malhado et al., 2013).
Due to a combination of logistical and financial impediments, large expanses of the Amazonian flora and fauna remain woefully understudied (Hopkins, 2007). This is even more evident for some specialized groups with difficult access, such as vascular epiphyte assemblages: plants which depend on the structural support of trees during some stages, if not all, of their life cycle. They contribute substantially to global species richness, accounting for approximately 10% of all vascular plant species (Zotz et al., 2021), and in some tropical forests up to 50% of the local plant species richness (Kelly et al., 2004). This pattern is not different in Amazonia, and the Andean-Amazon interfaces one of the most important speciation centers for this particular group (Kreft et al., 2004). Likewise, lowland Amazonia harbors a relatively rich vascular epiphyte flora (Ribeiro et al., 1999). However, most knowledge about this hyper-diverse functional group in Amazonia originates from single, small-scale inventories (ter Steege and Cornellisen, 1989; Nieder et al., 2000; Benavides et al., 2011; Obermüller et al., 2012; Irume et al., 2013; Boelter et al., 2014; Quaresma and Jardim, 2014; Mari et al., 2016; Quaresma et al., 2017, 2018), which complicates the interpretation of biodiversity patterns at larger geographic scales. To date, no compilation of studies addressing the Amazonian continental scale was carried out.
Because epiphytes by definition are exposed in tree canopies and lack underground root systems, they are thought to be particularly vulnerable to local climate conditions (Nadkarni and Solano, 2002; Zotz and Bader, 2009; Zotz, 2016) and potentially respond more strongly to environmental gradients than most terrestrial plants (Taylor et al., 2021). Epiphytes, in addition, provide important ecosystem services as they play key roles in water retention, nutrient cycling, and provision of habitat shelter and food for several animals (Lowman and Schowalter, 2012; Gotsch et al., 2016; Nakamura et al., 2017). Therefore, we consider it fundamental to characterize baseline biogeographic patterns underlying vascular epiphyte assemblages (hereafter referred to as VEA) for future reference in light of climate change and to provide a first handle toward conservation planning, as changes in their communities can potentially negatively affect the ecological role they play and the ecosystem services provided.
Amazonia is characterized by a South to North-West gradient of increasing moisture, and an East to West gradient in soil fertility (ter Steege et al., 2006). This huge forest territory is far from uniform, containing different forest types like upland forests (terra firme), seasonally inundated floodplains (várzeas and igapós), coastal forest (restingas) and savanas (white-sand forests, campinaranas), among others (Junk et al., 2011). Earlier studies have indicated that these forest types differ in their structure and species composition (Oliveira-Filho et al., 2021). The Andes-Amazonia interface is one of the most important speciation centers for epiphytes (Kreft et al., 2004), and Western Amazonian forests have been assumed to host a substantially richer epiphyte flora compared to other Amazonian regions (Gentry and Dodson, 1987; Kreft et al., 2004; Küper et al., 2004). This prediction, however, has not yet been adequately tested due to lack of data.
Here, using information of 32 inventories across the region yielding a total of 518 vascular epiphytic species, 96,448 epiphyte individuals on 10,907 phorophytes, we highlight emerging patterns and provide: (1) the geographic distribution of the study sites and highlight regions deemed of high priority for future quantitative inventories; (2) the geographic structure of Vascular Epiphyte Assemblages (VEAs) at the region scale and (3) a quantification of community attributes across major Amazonian habitat types.
Materials and Methods
Description of the Epiphyte Inventories in the Amazon Basin
The Amazon Epiphyte Network (AEN) consists of 32 inventories of vascular epiphytes conducted in the Amazon basin (Figure 1; dd. February 2022). The network was formed with the aim of specialists and knowledge about epiphytes in the Amazon that allow the integrated analysis of this plant assembly on a basin scale. The work combines results from different independent initiatives in four countries (Brazil, Colombia, Guiana, and French Guiana). To our knowledge, based on the number of published manuscripts on epiphytes in Amazonia, the AEN represents 94.1% of the total inventories with vascular epiphytes in the Amazon basin. We divided the biome in six biogeographic regions: CA, central Amazonia; EA, eastern Amazonia; GS, Guiana Shield; SA, southern Amazonia, WAN, northern part of western Amazonia; WAS, southern part of western Amazonia (sensu ter Steege et al., 2013). Study sites were plotted geographically to identify those areas well represented but also those still remaining underrepresented and where future endeavors for inventories should be focused (Figure 1).
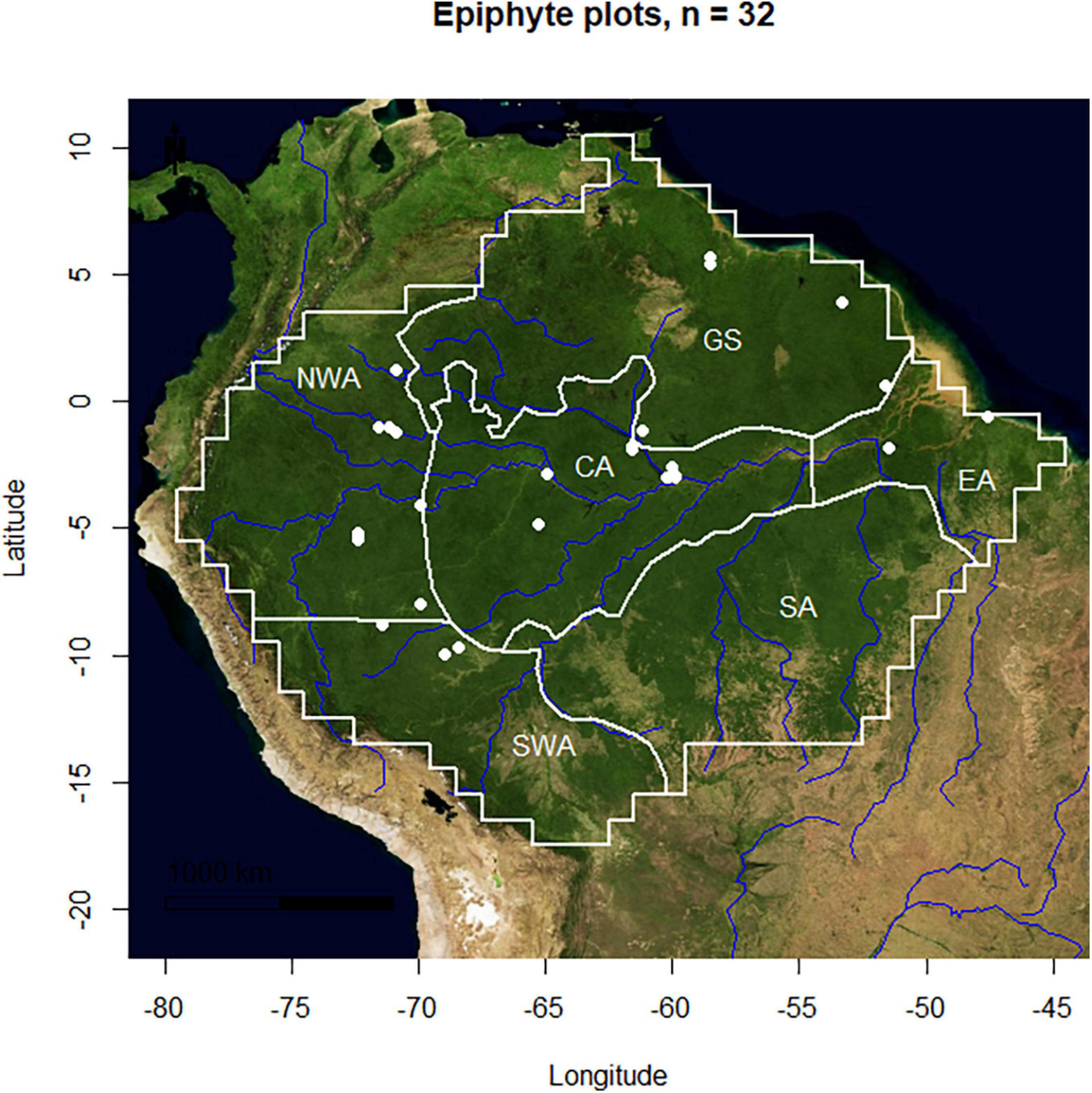
Figure 1. Map showing epiphytes floristic inventories in Amazon biome and its six biogeographic regions. CA, central Amazonia; EA, eastern Amazonia; GS, Guiana Shield; SA, southern Amazonia, NWA, northern part of western Amazonia; SWA, southern part of western Amazonia.
Data Gathering
The 32 studies used several different sampling methods working with epiphytes in Amazonia, particularly relating to the selection of minimum tree sizes and the definition of the sampling unit itself. The sampling method in 78% of the datasets included a combination of crown access and ground observations utilizing binoculars, while 22% were entirely restricted to single-rope climbing techniques or other methods for actual crown access. In 51% of the cases epiphytes were inventoried in trees with DBH (diameter at breast height) ≥ 10 cm, and in 24.2% all trees inside the plots were inventoried (but without access to all tree canopies); in another 18% only big trees (DBH ≥ 20 and 30 cm) were inventoried, and in 6% sampled trees were ≥ 5 cm. The definition of sampling unit varied among studies: it can correspond to the entire phorophyte – i.e., a single list of species per phorophyte, or to the different height zones in one phorophyte – i.e., a number of species lists per phorophyte, according to a pre-determined subdivision of the sampling. Either way, compositional data can be easily lumped and brought down to phorophyte level to ensure comparability among datasets. The details of the methods are presented in Appendix 1.
Taxonomic Vetting
Spelling and synonymy of all names gathered in this preliminary epiphyte species list was checked using with the Taxonomic Name Resolution Service,1 the Plant List,2 and the Brazilian flora checklist.3 When inconsistencies were encountered, we chose to use the most current database as our reference. For example, all Codonanthes, present in TROPICOS and the Plant List, were changed to Codonanthopsis present in Brazilian Flora List, as recent morphological analysis has revealed that all Amazonian species can be considered Codonanthopsis (Chautems and Perret, 2013).
All lianas (no loss of soil connection), parasites (fully dependent on the phorophyte), and tree species (accidental germination in the epiphytic environment) were excluded from the database. Varietal and subspecies status were ignored (i.e., all individuals were assigned to the species level). All epiphyte species, as defined by Benzing (1990), were included, i.e., holoepiphytes (always germinating and growing on other plants), facultative holoepiphytes (growing either as epiphyte or terrestrial); accidental holoepiphytes (terrestrial with occasional presence as an epiphyte); primary hemiepiphytes (germinating on plants with subsequent soil connection); secondary hemiepiphytes (soil germination with subsequent establishment on the phorophyte, and possible loss of the basal portion of the stem/root system). Here we chose to follow Benzing’s (1990) classification for a better comparison of our dataset with previous studies, which mostly used Benzing’s classification or adaptation of it.
Compatibility Among Datasets to Avoid Taxonomic and Ontogenetic Entanglements
For our analysis, we only used species observations that were validated for their taxonomic identification as described above. All morphospecies were removed from the database so that comparisons between environments and biogeographic regions were possible. To minimize the discrepancy between different sampling efforts we performed a rarefaction analysis for each inventory and reduced the sample units to a minimum number that still represented the species richness present in the data. In order to evaluate differences in epiphytic composition between environments and regions, we used non-metric multidimensional scaling (NMDS) with the Bray-Curtis distance metric to represent compositional differences. Relative abundance distributions were used to investigate the species abundances in each Amazonian environment, defined by ter Steege et al. (2013). We conducted all analyses in R (R Development Core Team, 2011). NMDS was conducted using the vegan package (Oksanen et al., 2016), using the metaMDS function. Rarefaction and extrapolation curves were performed using the iNEX library (Hsieh et al., 2020).
Results
All Amazonian biogeographic regions showed large gaps of completed inventories of vascular epiphytes (Figure 1). The northern part of western Amazonia and central Amazonia had the largest number of inventories, but still extensive unsampled areas. The largest sampling gap is shown to be in the southern Amazon region, which does not have any epiphyte inventory. Most of the inventories are located in the Brazilian (60.6%) and Colombian Amazon (27.2%), which together comprise 87.8% of all data. The inventories were established in six different forest-ecosystem types (white-water floodplain várzea (VA), black-water floodplain igapó (IG); upland terra firme of the Guiana Shield (TFGS) and upland terra firme of the Pebas Formation (TFPF); white or brown sand forests campinaranas on podzols (PZ) and coastal sand forests (CF) (Appendix 1). Terra firme forests (TFGS) had the greatest species richness. This pattern is consistent even when using rarefaction as explained above (Figure 2).
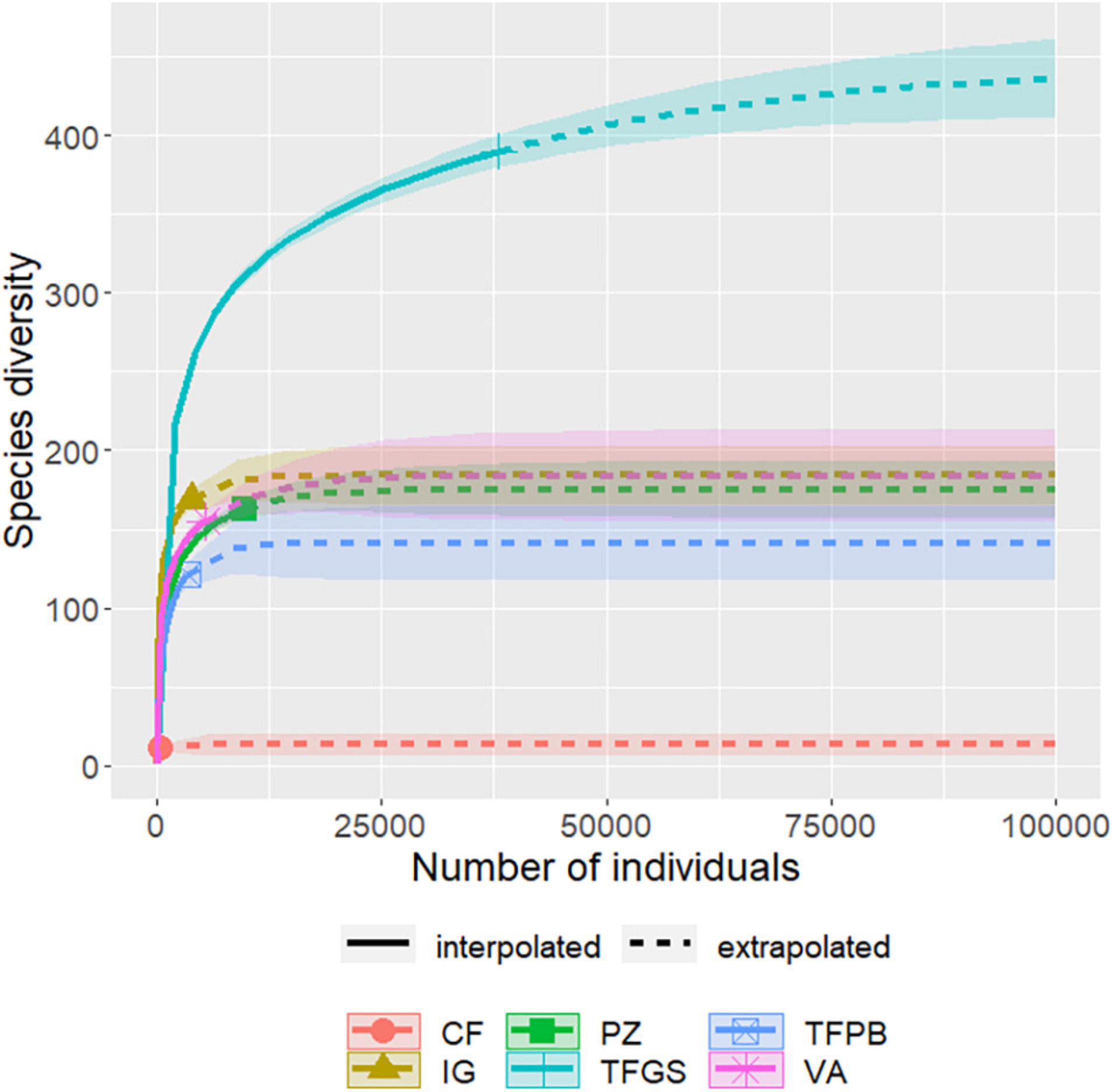
Figure 2. Rarefaction and extrapolation curves for the six environments sampled in the Amazon. Colors represent environments. Dotted lines represent the richness found and shaded lines represent the extrapolation of species richness.
Composition
Our raw data consisted in a total of 833 epiphytic taxa (including taxa identified only at the family, genus or morphospecies level), 70% of these were indentified to species, 26% to genus and 3.2% to the family level, while 1.2% remained unidentified. After filtering and taxonomic cleaning, approximately 9% of the identified species names were considered synonyms and corrected. The final number of 518 valid epiphyte species belonged to 36 families, 154 genera and 60,467 individuals. Most species were registered in Brazilian inventories (204) followed by Colombia (132; Figure 3A), and the most species rich environment was TFGS (387), followed by PZ (177), IG (170), and VA (156; Figure 3B). The family with the highest species richness was Orchidaceae (168 species, 32.3%), followed by Araceae (105 species, 20.1%), Bromeliaceae (42 species, 8%), Polypodiaceae (27 species, 5.2%), Clusiaceae (21 species, 4%), and Dryopteridaceae (20 species, 4%). Together, these families represented nearly three-quarters of all species in the dataset. But the representation of families changed according to the environment (Supplementary Material, 3). The dominant ecological category was holoepiphytes with 342 species (65.9%), followed by primary hemiepiphytes with 128 species (24.8%) and secondary hemiepiphytes with 48 species (9.1%), (Supplementary Material, 1).
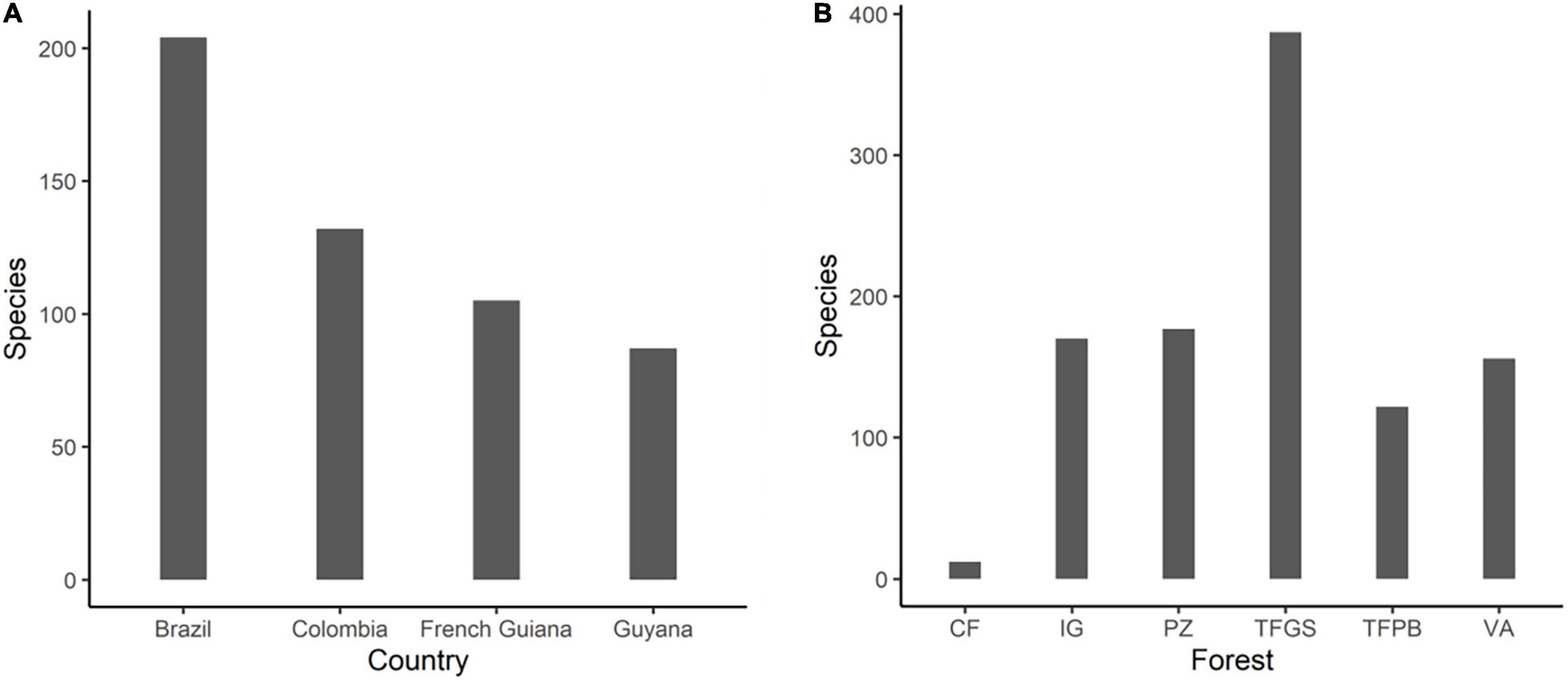
Figure 3. Species richness by country (A) and by environment (B) in Amazonia. There are clear differences in the number of species by environment and by country, however, this may be a reflection of the sampling effort for each location VA, Várzea; IG, Igapó; TFGS, Terra Firme Guiana Shield and TFPB, Terra Firme from the Pebas region; PZ, White Sand Forests on Podzols and CF, Coastal Sand Forests.
Philodendron was the most species-rich genus with 54 species recorded, followed by Anthurium (23 spp.), Clusia (21 spp.), Maxillaria and Trichomanes (19 spp., each). The most abundant species were Maxillaria camaridii (7,392 individuals), Prosthechea fragrans (2,026), Codonanthopsis crassifolia (1,879), Philodendron linnaei (1,694) and Ornithidium rigidum (1,651) with a combined total representing nearly one fourth of all individuals sampled.
Rank Abundance Distribution of Epiphyte Species
The twenty-two most abundant species represented half of all sampled individuals. Likewise, 103 species were either represented as singletons or doubletons (i.e., occurring only once or twice), and 198 species were represented by less than 10 individuals. Consequently, whereas less than three percent of the species represented half of all individuals sampled, half of the species sampled were represented fewer than 10 times in the data (Figure 4).
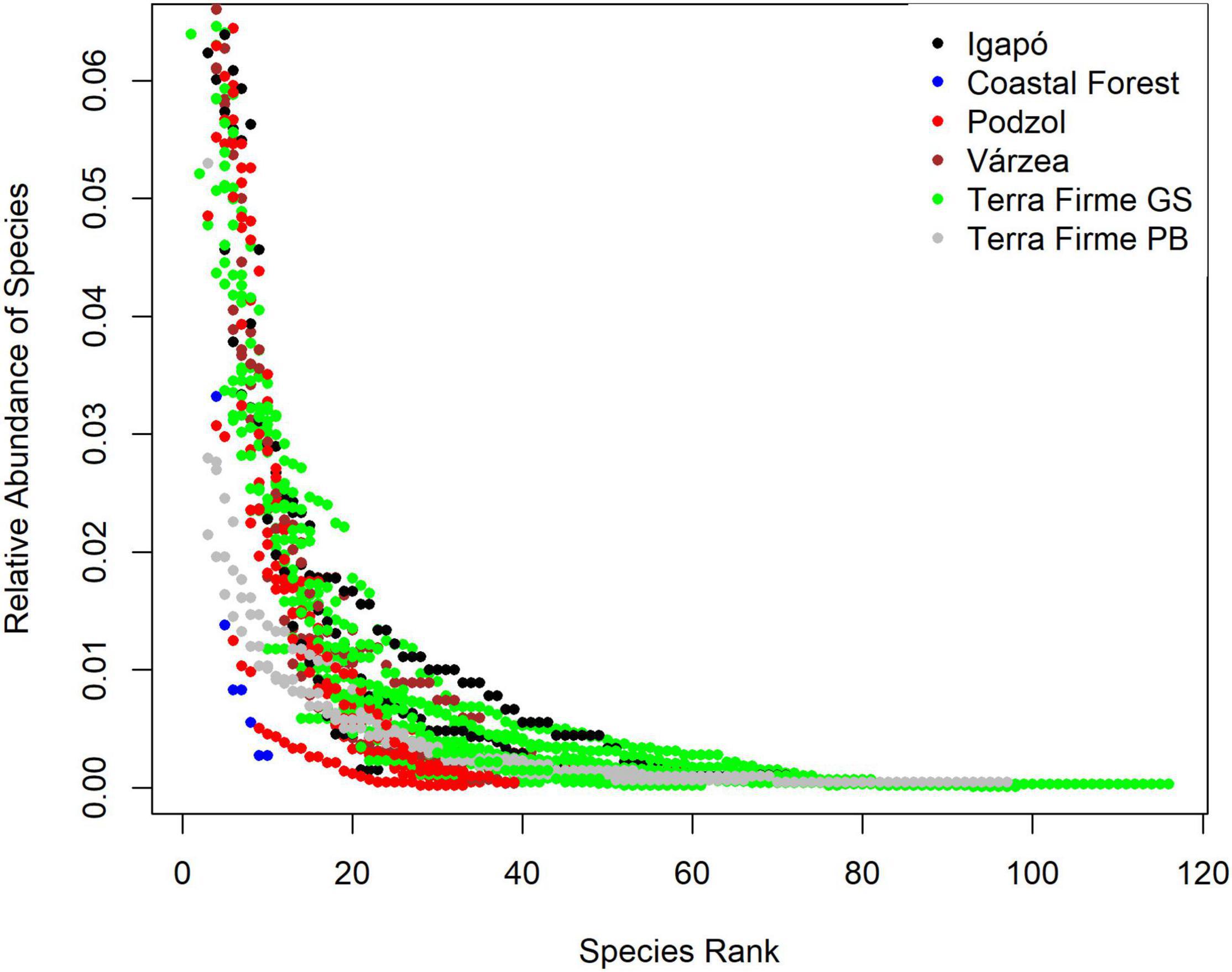
Figure 4. Abundance ranking of vascular epiphyte species for the different environments in the Amazon basin.
Compositional Variation
Variation in composition of VEAs was shown to be mostly spatially and not environmentally structured with floristic similarity generally decaying with geographic distance (i.e., plots in the same biogeographic region had higher similarity than plots in different regions independent of the habitat type). One of the most visible result was that different habitat types from the same region shared higher floristic similarities than did similar habitat types among regions. For example, seasonally inundated and podzol-based forests from Central Amazonia share a much greater composition of VEA than each of these habitat types share with their counterparts in other regions, as can be seen in the NMDS analysis, which shows a sharp distinction between regions (Figure 5).
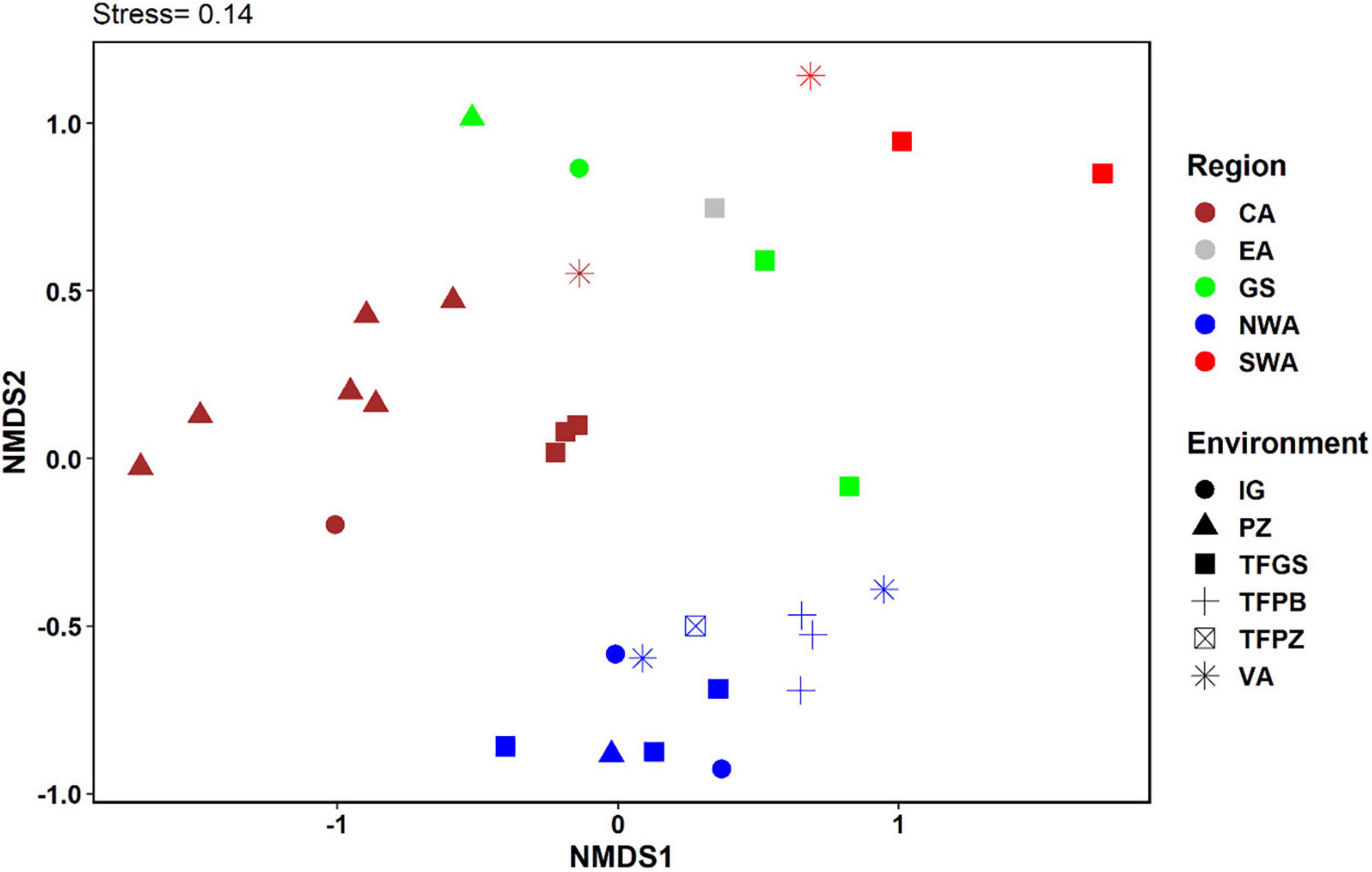
Figure 5. The first two axes of a Non-metric Multidimensional Scaling (NMDS) illustrating VEA compositional structure across the Amazon Basin. Colors represent biogeographical regions and symbols represent environments.
Discussion
Epiphytes grow detached from the ground (at least at some stage of their life cycle), and are dependent on the structural support of trees for survival (Zotz, 2016). These unique characters make them exceptionally sensitive to climate change, habitat alterations via land-use change and any other related anthropogenic activities (Zotz and Bader, 2009; Zotz, 2016). Despite continued threats to their survival (i.e., Barthlott et al., 2001; Krömer et al., 2014, Leao et al., 2014), our limited knowledge regarding Amazonian epiphyte assemblages has not allowed us to establish fundamental baselines such as estimates for basin-wide species number, general compositional patterns and patterns of rarity and commonality. Here, we explore the general patterns in VEAs across Amazonia. To our knowledge, this is the first study which presents data at the Amazonian scale. The vascular epiphyte distribution reported here is a first step to help us unveiling priority regions for the future sampling of epiphyte inventories.
Our results reinforce the richness pattern frequently found in tree species diversity wherein uplands forest has higher species richness than other environments (Oliveira-Filho et al., 2021). However, our result can be biased in our knowledge between environments because most epiphytes inventories were in terra firme forests with other environments being less represented. The greater arboreal richness in terra firme is explained mainly by edaphic and hydrological differences, but the reasons for these differences in epiphyte assemblages are still not clear. In contrast, it could have been expected that seasonally flooded ecosystems (várzeas and igapós) potentially might present higher species richness, due to their proximity to water-bodies and consequent increases in air humidity (i.e., Flores-Palacios and García-Franco, 2006), but this was not found. This might be because long and high flooding (manly in central Amazonia) results in the scarce or even absent colonization of epiphytes on flooded tree stems and twigs (Quaresma et al., 2017). In turn, trees grow taller (vertically) and slower (laterally), but more evenly, throughout the year in terra firme forests (Bredin et al., 2020). Slow lateral growth results in more structural matter per unit volume wood, and thus greater stability, supportive of higher stems (Chave et al., 2009; Bredin et al., 2020). Generally, terra firme forests present taller canopies with higher levels of stratification, which is associated to a higher diversity of phorophytes, which in turn contributes to a higher diversity of epiphytes. The greater height of the trees provides a greater amount of substrate (which is also more stable) and vertical niches to be colonized, fundamental characteristics for the occurrence of epiphytes (Ruiz-Cordova et al., 2014; Zotz, 2016).
The number of species recorded was below our expectations, even considering the low number of inventories relative to the extent of the study area, because some local studies in Amazonia registered a high number of species. For reference, nearly half the total number of species tallied for this study (256) was recorded only at the Tiputini Biodiversity Station, Ecuador (Kreft et al., 2004), in an area of 650 ha. The species number calculated from this entire study is also an order of magnitude lower than that recorded for other Neotropical forests phytogeographic domains such as the Atlantic Forest (2,095 species; Ramos et al., 2019). The large number of unidentified taxa or identified to the genus or family level may have contributed substantially to the low species numbers, considering that across all databases, between 10 and 20% of the samples were not identified at the specific level. On the other hand, considering that the most tropical plant species have an aggregated distribution, another factor for the low number of species, recorded in this study, was probably the large inventory gaps in our sampling, for example, an entire biogeographic region (southeast Amazonia) did not have any registered inventory. Therefore, despite recent advances in the studies of epiphytes in Amazonia, improving our understanding of biogeographic questions, it is urgent that more inventories are carried out; because the lack of information is clearly problematic as it obscures representative characterizations of species diversity and distribution patterns at broad spatial scales (Küper et al., 2004; Engemann et al., 2015; Mendieta-Leiva et al., 2020).
In general, the systematic composition of Amazonian epiphyte communities is highly predictable at the family level with Orchidaceae, Araceae and Bromeliaceae being the most species-rich families (Kreft et al., 2004). This pattern was reported earlier by Taylor et al. (2021), using inventories and distribution information for 27,850 epiphyte species derived from literature sources, where Orchidaceae comprises 67% of the epiphytic flora, Bromeliaceae (11%) and Araceae (4%) being the dominant plant families. Marcusso et al. (2022) it also reported this same pattern for the Neotropics and our results confirm this for Amazonia. Noteworthy, most of Araceae species (60%) recorded in our inventories are excluded in the new classification of vascular epiphytes and are now considered vines by Zotz (2013) and Zotz et al. (2021). Future efforts should focus on in-depth knowledge of species ecology to confirm this new classification, mainly for Amazonia, where in some places aroids are more important than orchids (Leimbeck and Balslev, 2001; Benavides et al., 2005). Another example derived of the personal observation on monitoring permanent plots (Quaresma pers. obs.) in black-water floodplain ecosystems (Igapó), where some secondary hemiepiphytes (i.e., Philodendron solimoesense A.C.Sm. and Philodendron billietiae Croat) never were observed growing on the forest floor during 6 years of monitoring, likely due to annual floods which in some areas may persevere for up to 300 days per year (Junk et al., 2011).
Results furthermore showed that a core group of 22 oligarchic taxa accounted for more than a half of all epiphyte individuals. In Amazonian tree communities it is well established that a small proportion of species form so called oligarchies and are hyperdominant across huge geographical areas, but these results were shown only for tree species (Pitman et al., 2001; ter Steege et al., 2013). For epiphytes, in contrast, this oligarchy is geographically structured as the species dominance changes with habitat type. For instance, M. camaridii has 97.1% of individuals occurring in the TFGS and PZ; and P. fragrans has 98.3% of individuals occurring only in PZ, suggesting that the dominance of species may be related to the type of environment (or region).
Hyperdominance, however, can also be explained without invoking the role of the habitat type. Using a density-dependent speciation model and the framework of neutral theory, Janzen et al. (2020) hypothesized that very abundant epiphytic species are either less prone to speciation or have a competitive advantage over rare species. Fitting the models on field data, the authors showed that, in the case of lower speciation rates, species would reach higher number of individuals in the assemblage and become more plastic, which leads to hyperdominance and in turn to lower speciation rates. This is corroborated by epiphyte population structure data that show that more abundant species tend to have a higher number of juveniles (Zotz, 2007) and can help to explain our results for few species with many individuals (hyperdominant) and many species with low number of individuals. Future collection efforts are needed to have a clearer view of this pattern.
Amazonia VEA characterized from inventories of a similar region, even when sampled from different environments, are more floristically similar when compared among regions. A similar pattern was reported by Marcusso et al. (2022) where some distant areas in Amazonia Basin were grouped in the same cluster. Such as pattern would suggest a “spillover-effect” where habitat types in proximity literally “share” species through constant propagule rain. Specifically, higher levels of extinction of epiphytes recruited into suboptimal habitat type are buffered by the elevated rates of nearby dispersal events from their nearby highly abundant sources. In summary, this broad-scale partitioning of VEA floristic similarity which comparatively washes out local compositional distinctions among habitat types could suggest that either region wide historical and/or dispersal related factors influence the distributional factors of the more than half of the epiphyte species which were deemed rare (< 10 individuals).
Interestingly, we found clear evidence for floristic discontinuity and heterogeneity in species composition among biogeographic regions of Amazonia. Janzen et al. (2020) hypothesized (but their results were inconclusive) that VEA in the Amazonian basin are not limited by dispersal, as the region does not present many dispersal barriers (such as the mountainous regions of the Andes and Central America) and have a higher prevalence of wind-dispersed species (e.g., orchids and ferns), which may attain long-tail dispersal kernels. Others also suggested that epiphytes have a wider distribution range than terrestrial plants (Ibisch et al., 1996; Nieder et al., 1999; Kessler, 2000), our results, however, do not support these hypotheses as we observe a clear geographic structure of epiphyte composition.
We hypothesize that the revealed large-scale patterns for vascular epiphytes in the Amazon are similar to those found for trees because epiphyte composition follows the unique phorophyte composition in different biogeographic regions. It is already well known that vascular epiphytes do not occur randomly on their phorophytes and show preferences for specific tree species (ter Steege and Cornellisen, 1989; Burns and Zotz, 2010; Zotz, 2016), also shown in Amazonian igapó forests (Quaresma et al., 2018), supporting this view.
In addition, Amazonia presents a wide variation of local environmental conditions resulting in an arboreal flora of specific hosts for epiphytes, causing compositional differences across the basin. Even in relatively close areas, where soil gradients cause changes in tree composition, marked differences are found in the composition of vascular epiphytes (Boelter et al., 2014; Quaresma et al., 2017). On the other hand, recent studies with vascular epiphytes on a global scale strongly suggest that epiphytes vary relative to trees in their responses to environmental gradients, with epiphytes responding more quickly to the atmospheric gradient due to their air and water-limited growth habit (Taylor et al., 2021).
We hope that future efforts make these aspects clearer and help us to understand the diversity and compositional patterns for Amazon vascular epiphytes. For that, it is necessary increase of inventories of VEA in biogeographic regions (i.e., Southern Amazonia) and ecosystems (i.e., buritizais) improving the species identification. Further it is necessary to improve techniques for more efficient inventories of VEA, for instances, using drones with high-resolution cameras, as conventional sampling techniques climbing trees are time-consuming and limited. Concurrent to this, we need to model species occurrence and phenology of VEA (vs. tree community), verify relationships between local and regional diversity, start studies analyzing functional traits of epiphytes (i.e., hyperdominant vs. rare species) in the background of climate change (increasing temperature) and land-use change affecting tree species communities, verify relationships between VEA and phorophytes on species level (tree age, physiochemical characteristics of bark) and community level (succession, disturbances).
Data Availability Statement
The raw data supporting the conclusions of this article will be made available by the authors, without undue reservation.
Author Contributions
AQ and CZ had the original idea and under the guidance of HTS started the formation and organization of Amazonia Epiphyte Network. AQ, CZ, and MP wrote the first version of the manuscript. HTS, FW, MJ, and JT contributed with many good comments and analyses. VK, MI, AB, LF, CB, FO, AD, MM, JS, EA, EP, RE, JD, KB, and IB improved the manuscript. FP improved the manuscript in the final revision. All authors contributed data and approved the submitted version.
Funding
We are grateful for the constructive comments of Sylvia Mota de Oliveira. We would like to thank CNPq for financing the research projects: the Long-term Ecological Research Network–PELD (CNPq/CAPES/FAPS/BC, NEWTON PROGRAM FUND, grant number 441590/2016–0; MCTI/CNPq/FAPs, grant number 403792/2012-6). This study was financed by the Fundação de Amparo á Pesquisa do Estado do Amazonas (FAPEAM) (FIXAM/FAPEAM, grant number 017/2914 and PELD/FAPEAM, grant number 062.01357/2017), by the Institute for Geography and Geoecology, Karlsruhe Institute for Technology - KIT, Dep. of Wetland Ecology, Germany, and by Technical/Scientific Cooperation Agreement between INPA and the Max-Planck-Society. We would also like to thank the CLAMBIO consortium and the BiodivERsA 2019–2020 Joint COFUND Call on “Biodiversity and Climate Change” (German Federal Ministry of Education and Research - BMBF: 16LC2025A). We acknowledge support by the KIT-Publication Fund of the Karlsruhe Institute for Technology.
Conflict of Interest
The authors declare that the research was conducted in the absence of any commercial or financial relationships that could be construed as a potential conflict of interest.
Publisher’s Note
All claims expressed in this article are solely those of the authors and do not necessarily represent those of their affiliated organizations, or those of the publisher, the editors and the reviewers. Any product that may be evaluated in this article, or claim that may be made by its manufacturer, is not guaranteed or endorsed by the publisher.
Supplementary Material
The Supplementary Material for this article can be found online at: https://www.frontiersin.org/articles/10.3389/ffgc.2022.828759/full#supplementary-material
Footnotes
References
Barthlott, W., Schmit-Neuerburg, V., Nieder, J., and Engwald, S. (2001). Diversity and abundance ofvascular epiphytes: a comparison of secondary vegetation and primary montane rain forest inthe Venezuelan Andes. Plant Ecol. 152, 145–156. doi: 10.1023/A:1011483901452
Benavides, A. M., Vasco, A., Duque, A. J., and Duivenvoorden, J. F. (2011). Association of vascular epiphytes with landscapeunits and phorophytes in humid lowland forests of Colombian Amazonia. J. Trop. Ecol. 27, 223–237.
Benavides, D. A. M., Duque, A. J., Duivenvoorden, M. J. F., Vasco, A., and Callejas, R. (2005). A first quantitative census of vascular epiphytes in rain forests of Colombian Amazonia. Biodivers. Conserv 14, 739–758.
Boelter, C. R., Dambros, C. S., Nascimento, H. E. M., and Zartman, C. E. (2014). A tangled web in tropical tree-tops:effects of edaphic variation, neighbourhood phorophyte composition and bark characteristics on epiphytesin a central Amazonian forest. J. Veg. Sci. 25, 1090–1099. doi: 10.1111/jvs.12154
Bredin, Y. K., Hawes, J. E., Peres, C. A., and Haugaasen, T. (2020). Structure and composition of terra firmeand seasonally flooded várzea forests in theWestern Brazilian Amazon. Forests 11, 13–61. doi: 10.3390/f11121361
Burns, K. C., and Zotz, G. (2010). A hierarchical framework for investigating epiphyte assemblages: networks, metacommunities, and scale. Ecology 91, 377–385. doi: 10.1890/08-2004.1
Chautems, A., and Perret, M. (2013). Redefinition of the Neotropical genera Codonanthe (Mart.) Hanst. and Codonanthopsis Mansf. (Gesneriaceae). Selbyana 31, 143–156.
Chave, J., Coomes, D., Jansen, S., Lewis, S. L., Swenson, N. G., and Zanne, A. E. (2009). Towards a worldwide woodeconomics spectrum. Ecol. Lett. 12, 351–366. doi: 10.1111/j.1461-0248.2009.01285.x
Engemann, K., Enquist, B. J., Sandel, B., Boyle, B., Jørgensen, P. M., Morueta-Holme, N., et al. (2015). Limited sampling hampers ‘big data’ estimation of species richness in a tropical biodiversity hotspot. Ecol. Evol. 5, 807–820. doi: 10.1002/ece3.1405
Flores-Palacios, A., and García-Franco, G. (2006). The relationship between tree size and epiphyte species richness: testing four different hypotheses. J. Biogeogr. 33, 323–330. doi: 10.1111/j.1365-2699.2005.01382.x
Gentry, H. A., and Dodson, C. H. (1987). Diversity and biogeography of neotropical vascular epiphytes. Ann. Missouri Bot. Gard. 74, 205–233. doi: 10.3897/BDJ.9.e71974
Gotsch, S. G., Nadkarni, N., and Amici, A. (2016). The functional roles of epiphytes and arboreal soils in tropical montane cloud forests. J. Trop. Ecol. 32, 455–468. doi: 10.1017/s026646741600033x
Hopkins, M. J. G. (2007). Modelling the known and unknown plant biodiversity of the Amazon Basin. J. Biogeogr. 34, 1400–1411. doi: 10.1111/j.1365-2699.2007.01737.x
Hsieh, T. C., Ma, K. H., and Chao, A. (2020). INEXT: Interpolation and Extrapolation for Species Diversity. R Package Version 2.0.20. Available online at: http://chao.stat.nthu.edu.tw/wordpress/software_download/ (accessed November 25, 2021).
Ibisch, P. L., Boegner, A., Nieder, J., and Barthlott, W. (1996). How diverse are neotropical epiphytes? An analysis based on the ‘Catalogue of the flowering plants and gymnosperms of Peru. Ecotropica 2, 13–28.
Irume, M. V., Moraes, M. C. L. S., Zartman, C. E., and Amaral, I. L. (2013). Floristic composition and community structureof epiphytic angiosperms in a terra firme forest in central Amazonia. Acta. Bot. Bras. 27, 378–393. doi: 10.1590/S0102-33062013000200012
Janzen, T., Zotz, G., and Etienne, R. S. (2020). Community structure of vascular epiphytes: a neutral perspective. Oikos 129, 853–867. doi: 10.1111/oik.06537
Junk, W. J., Piedade, M. T. F., Schöngart, J., Cohn-Haft, M., Adeney, M. J., and Wittmann, F. (2011). A classificationof major naturally-occurring Amazonian lowland wetlands. Wetlands 31, 623–640.
Kelly, D. L., O’Donovan, G., Feehan, J., Murphy, S., Drangeid, S. O., and Marcano-Berti, L. (2004). The epiphyte communities of a montane rain forest in the Andes of Venezuela: patterns in the distribution of the flora. J. Trop. Ecol. 20, 643–666. doi: 10.1017/S0266467404001671
Kessler, M. (2000). Elevational gradients in species richness and endemism of selected plant groups in the central Bolivian Andes. Plant Ecol. 149, 181–193. doi: 10.1023/A:1026500710274
Kreft, H., Köster, N., Küper, W., Nieder, J., and Barthlott, W. (2004). Diversity and biogeography of vascular epiphytes in westernAmazonia, Yasunı, Ecuador. J. Biogeogr. 31, 1463–1476. doi: 10.1111/j.1365-2699.2004.01083.x
Krömer, T., García-Franco, J. G., and Toledo-Aceves, T. (2014). “Epífitas vasculares como bioindicadores dela calidad forestal: impacto antrópico sobre su diversidad y composición,” in Bioindicadores: Guardianes Denuestro Futuro Ambiental, eds C. A. González-Zuarth, A. Vallarino, J. C. Pérez-Jimenez, and A. M. Low-Pfeng (México: Instituto Nacional de Ecologia y Cambio Climático (INECC)—ElColegio de la Frontera Sur (ECOSUR)), 606–623.
Küper, W., Kreft, H., Nieder, J., Köster, N., and Barthlott, W. (2004). Large-scale diversity patterns of vascular epiphytes in Neo-tropical montane rain forests. J. Biogeogr. 31, 1477–1487. doi: 10.1111/j.1365-2699.2004.01093.x
Latrubesse, E. M., Arima, E. Y., Dunne, T., Park, E., Baker, V., d’Horta, F., et al. (2017). Damming the rivers of the Amazon Basin. Nature 546:369. doi: 10.1038/nature22333
Leao, T. C. C., Fonseca, C. R., Peres, C. A., and Tabarelli, M. (2014). Predicting extinction risk of brazilianatlantic forest angiosperms. Conserv. Biol. 28, 1349–1359. doi: 10.1111/cobi.12286
Leimbeck, R. M., and Balslev, H. (2001). Species richness and abundance of epiphytic Araceae on adjacentfloodplain and upland forest in Amazonian Ecuador. Biodivers. Conserv. 10, 1579–1593.
Lowman, M. D., and Schowalter, T. D. (2012). Plant science in forest canopies - the first 30 years of advances and challenges (1980-2010). New Phytologist 194, 12–27. doi: 10.1111/j.1469-8137.2012.04076.x
Malhado, A. C. M., Ladle, R., Whittaker, R., Neto, A., Malhi, Y., and ter Steege, H. (2013). The ecological biogeography of Amazonia. Front. Biogeogr. 5, 102–112. doi: 10.21425/F5FBG13314
Marcusso, G. M., Kamimura, V. A., Borgiani, R., Menini-Neto, L., and Lombardi, J. A. (2022). Phytogeographic meta-analysis of the vascular epiphytes in the neotropical region. Bot. Rev. doi: 10.1007/s12229-021-09270-2
Mari, M. L. G., Toledo, J. J., Nascimento, H. M. E., and Zartman, C. E. (2016). Regional and fine scale variation of holoepiphytecommunity structure in Central Amazonian white-sand forests. Biotropica 48, 70–80. doi: 10.1111/btp
Mendieta-Leiva, G., Ramos, F. N., Elias, J. P. C., Zotz, G., Alvim, F. S., Barbosa, E. F., et al. (2020). EpIG-DB: a database of vascular epiphyte assemblages in the Neotropics. J. Veg. Sci. 31, 518–528. doi: 10.1111/jvs.12867
Nadkarni, N. M., and Solano, R. (2002). Potential effects of climate change on canopy communities in a tropical cloudforest: an experimental approach. Oecologia 131, 580–586. doi: 10.1007/s00442-002-0899-3
Nakamura, A., Kitching, R. L., Min, C., Creedy, T. J., Fayle, T. M., Freiberg, M., et al. (2017). Forests and their canopies: achievements and horizons in canopy science. Trends Ecol. Evol. 32, 438–451. doi: 10.1016/j.tree.2017.02.020
Nieder, J., Engwald, S., and Barthlott, W. (1999). Patterns of neotropical epiphyte diversity. Selbyana 20, 66–75.
Nieder, J., Engwald, S., Klawun, M., and Barthlott, W. (2000). Spatial distribution of vascular epiphytes (includinghemiepiphytes) in a Lowland Amazonian Rain Forest (Surumoni Crane Plot) of Southern Venezuela. Biotropica 32, 385–396. 85.x doi: 10.1111/j.1744-7429.2000.tb004
Obermüller, F. A., Silveira, M., Salimon, C. I., and Daly, D. C. (2012). Epiphytic (including hemiepiphytes) diversityin three timber species in the southwestern Amazon, Brazil. Biodivers. Conserv. 21, 565–575.
Oksanen, O., Blanchet, F. G., Kindt, R., Legendre, P., Minchin, P. R., et al. (2016). Vegan: Community Ecology Package. R Package Version 2.3-5. Available online at: http://CRAN.R-project.org/package=vegan
Oliveira-Filho, A. T., Dexter, K. G., Pennington, R. T., Simon, M. F., Bueno, M. L., and Neves, D. M. (2021). On thefloristic identity of Amazonian vegetation types. Biotropica 53, 767–777. doi: 10.1111/btp.12932
Pitman, N. C. A., Terborgh, J., Silman, M. R., Neill, D. A., and Núñez, P. V. (2001). Dominance and distribution of tree species in upper Amazonian terra firme forests. Ecology 82, 2101–2117. doi: 10.1890/0012-9658
Quaresma, A. C., and Jardim, M. A. G. (2014). Floristic composition and spatialdistribution of vascular epiphytes in the restingas of Maracana. Brazil Acta Bot. Bras. 28, 68–75. doi: 10.1590/S0102-33062014000100007
Quaresma, A. C., Piedade, M. T. F., Feitosa, Y. O., Wittmann, F., and ter Steege, H. (2017). Composition, diversity andstructure of vascular epiphytes in two contrasting Central Amazonian floodplain ecosystems. Acta Bot. Bras. 31, 686–697.
Quaresma, A. C., Piedade, M. T. F., Wittmann, F., and ter Steege, H. (2018). Species richness, composition, and spatial distribution of vascular epiphytesin Amazonian blackwater floodplain forests. Biodivers. Conserv. 27, 1981–2002. doi: 10.1007/s10531-018-1520-3
R Development Core Team (2011). R: A Language And Environment For Statistical Computing. Vienna: R Foundationfor Statistical Computing.
Ramos, F. N., Mortara, S. R., Monalisa-Francisco, N., Elias, J. P. C., Neto, L. M., Freitas, L., et al. (2019). Atlantic Epiphytes: a data set of vascular and non-vascular epiphyte plants and lichens from the Atlantic Forest. Ecology 100:e02541. doi: 10.1002/ecy.2541
Ribeiro, J. E. L. S., Hopkins, M. J. G., Vicentini, A., Sothers, C. A., and Costa, M. A. S. (1999). Flora da Reserva Ducke: Guia De Identificação Das Plantas Vasculares De Uma Florestade Terra Firme Na Amazônia Central. Manaus: INPA.
Ruiz-Cordova, J. P., Toledo-Hernández, V. H., and Flores-Palacios, A. (2014). The effect of substrate abundance in the vertical stratification of bromeliad epiphytes in a tropical dry forest (Mexico). Flora 209, 375–384. doi: 10.1016/j.flora.2014.06.003
Science Panel for the Amazon (2021). Executive Summary of the Amazon Assessment Report (2021), eds C. Nobre, A. Encalada, E. Anderson, F. H. Alcazar, M. Bustamante, et al. (New York, NY: United Nations Sustainable Development Solutions Network), 48.
Taylor, A., Zotz, G., Weigelt, P., Cai, L., Karger, D. N., König, C., et al. (2021). Vascular epiphytes contribute disproportionately to global centres of plant diversity. Glob. Ecol. Biogeogr. 31, 62–74. doi: 10.1111/geb.13411
ter Steege, H., and Cornellisen, J. H. C. (1989). Distribution and ecology of vascular epiphytes in lowland rain forestof Guyana. Biotropica 21, 331–339.
ter Steege, H., Pitman, N. C. A., Phillips, O. L., Chave, J., Sabatier, D., Molino, J. F., et al. (2006). Continental-scale patterns of canopy treecomposition and function across Amazonia. Nature 443, 444–447. doi: 10.1038/nature05134
ter Steege, H., Pitman, N. C. A., Sabatier, D., Baraloto, C., Salomão, R. P., Guevara, J. E., et al. (2013). Hyperdominance in the Amazonian tree flora. Science 342, 1243092–1243091. doi: 10.1126/science.1243092
ter Steege, H., Prado, P. I., de Lima, R., Pos, E., Coelho, L. S., de Andrade Lima, D., et al. (2020). Biased-corrected richness estimates for the amazonian tree flora. Sci. Rep. 10:10130. doi: 10.1038/s41598-020-66686-3
Zotz, G. (2007). The population structure of the vascular epiphytes in a lowland forest in Panama correlates with species abundance. J. Trop. Ecol. 23, 337–342. doi: 10.1017/S026646740700404X
Zotz, G. (2013). Hemiepiphyte: a confusing term and its history. Ann. Bot. 111, 1015–1020. doi: 10.1093/aob/mct085
Zotz, G., and Bader, M. Y. (2009). “Epiphytic plants in a changing world-global: change effects on vascular and non- vascular epiphytes,” in Progress in Botany, Vol. 70, eds U. Lüttge, W. Beyschlag, B. Büdel, and D. Francis (Heidelberg: Springer), doi: 10.1007/978-3-540-68421-3_7
Zotz, G., Weigelt, P., Kessler, M., Kreft, H., and Taylor, A. (2021). EpiList 1.0: a global checklist of vascular epiphytes. Ecology 102:e03326. doi: 10.1002/ecy.3326
Appendix
Keywords: Amazon environments, epiphytes habitat, distribution, Neotropics, oligarchic species, rain forest, species richness
Citation: Quaresma A, Zartman CE, Piedade MTF, Wittmann F, Jardim MAG, Irume MV, Benavides AM, Freitas L, Toledo JJ, Boelter CR, Obermüller F, Duque AJ, Klein VP, Mari MLG, Schöngart J, Arenas E, Pos ET, Vasco A, Ek R, Plantenga FDM, Duivenvoorden JF, Biesmeijer K, Bender IMA and ter Steege H (2022) The Amazon Epiphyte Network: A First Glimpse Into Continental-Scale Patterns of Amazonian Vascular Epiphyte Assemblages. Front. For. Glob. Change 5:828759. doi: 10.3389/ffgc.2022.828759
Received: 03 December 2021; Accepted: 19 May 2022;
Published: 05 July 2022.
Edited by:
Paolo Giordani, University of Genoa, ItalyReviewed by:
Alejandro Flores Palacios, Universidad Autónoma del Estado de Morelos, MexicoLuiz Menini Neto, Federal University of Juiz de Fora, Brazil
Copyright © 2022 Quaresma, Zartman, Piedade, Wittmann, Jardim, Irume, Benavides, Freitas, Toledo, Boelter, Obermüller, Duque, Klein, Mari, Schöngart, Arenas, Pos, Vasco, Ek, Plantenga, Duivenvoorden, Biesmeijer, Bender and ter Steege. This is an open-access article distributed under the terms of the Creative Commons Attribution License (CC BY). The use, distribution or reproduction in other forums is permitted, provided the original author(s) and the copyright owner(s) are credited and that the original publication in this journal is cited, in accordance with accepted academic practice. No use, distribution or reproduction is permitted which does not comply with these terms.
*Correspondence: Adriano Quaresma, YWNxLnF1YXJlc21hQGdtYWlsLmNvbQ==