- 1Jiangxi Provincial Key Laboratory of Silviculture, College of Forestry, Jiangxi Agricultural University, Nanchang, China
- 2Key Laboratory of National Forestry and Grassland Administration on Forest Ecosystem Protection and Restoration of Poyang Lake Watershed, College of Forestry, Jiangxi Agricultural University, Nanchang, China
Establishing plantation forests significantly increases the carbon (C) storage of terrestrial ecosystems. However, how vegetation types affect the ecosystem C sequestration capacity is not completely clear. Here, a slash pine plantation (SPP), a Schima superba plantation (SSP), and a Masson pine plantation (MPP), which have been planted for 30 years, were selected in subtropical China. The C storage and distribution patterns of plant, litter, and soil were investigated and calculated. The ecosystem C density was 17.7, 21.6, and 15.3 kg m–2 for SPP, SSP, and MPP, respectively. Ecosystem C stocks were mainly contributed by tree aboveground (39.9–46.0%) and soil C stocks (41.6–44.2%). The ecosystem C density of SSP was higher than that of SPP and MPP, and significant differences were found among three plantations for both aboveground and underground C densities. The aboveground and underground ecosystem C storage of SSP was 27.4 and 53.4% higher than that of MPP, respectively. Meanwhile, root C storage of MPP was lower than that of SPP and SSP, while soil C storage of MPP was lower than that of SSP. In the understory layer, SPP had the highest C density, followed by MPP, and there was a significant difference in C density among three plantations. However, no significant difference was found for the ecosystem C distribution among three plantations. Our results show that vegetation types significantly affect C storage but not C distribution in forest ecosystems and establishing the broad-leaved plantation has the highest ecosystem C storage in the subtropics. This study provides a theoretical basis for us to choose appropriate forest management measures.
1. Introduction
Forests provide the largest carbon (C) pool of terrestrial ecosystems and play a key role in mitigating the effects of climate warming (Houghton et al., 2000; Bonan, 2008; Moomaw et al., 2019). Establishing plantations, as one of the widespread forest management practices, can significantly increase C storage in terrestrial ecosystems (Moomaw et al., 2019). However, tree species may significantly affect the C sequestration capacity of plantations. The uneven C uptake capacity of tree species leads to a significant influence on plant C sequestration (Kang et al., 2006; Thomas and Martin, 2012). Moreover, soil C stocks vary in plantations due to different quantities and quality of organic matter contributed to the soil by plant species (Subashree et al., 2019; Zhang et al., 2020). Broad-leaved trees usually have higher organic matter inputs to the soil (Vesterdal et al., 2008), while coniferous trees have lower litter decomposition rates (Yang et al., 2004a,b). It is obvious that vegetation types have a significant impact on C sequestration, so it is necessary for us to accurately evaluate the effect of vegetation types on ecosystem C storage, which limits us to choose the appropriate forest management strategy.
The vegetation type is generally considered to significantly influence aboveground biomass C storage in forests (Yin et al., 2015; Mensah et al., 2016), while the role of vegetation type on the C stock of tree roots, understory vegetation, litter and soil is less well studied. All these components play vital roles in C storage and cycling in forest ecosystems (Kang et al., 2006; Justine et al., 2017). For example, Justine et al. (2017) showed that soil C stocks accounted for up to 76.6%, vegetation for 22.9%, and forest understory and deadfall layers for 0.6% of ecosystem C storage in subtropical pine plantations. Moreover, a C concentration factor of 0.5 is usually used to estimate plant C stocks (Thomas and Martin, 2012). The C concentrations in different tree species or different organs of the same tree species are different and deviate from 0.5, which leads to an uncertainty in the estimation of vegetation C stocks when a factor of 0.5 is used (Thomas and Martin, 2012; Watzlawick et al., 2014). Therefore, there is a need to gain more information from field measurements to accurately assess the C sequestration capacity of forest ecosystems as C sinks and to compare distinctions between the plantations with different vegetation types.
In the 1990’s, large areas of plantations with broad-leaved and coniferous species were afforested to alleviate soil erosion and improve the quality of degraded soils in subtropical China (FAO, 2010). Masson pine (Pinus massoniana), slash pine (Pinus elliottii), and Schima superba are the representative and commonly used tree species for timber production, C storage and ecological restoration in subtropical areas. The forest area of Masson pine is over 17.4 million hectares, accounting for 8.9% of China’s total forest area (Kuang et al., 2008), while the afforestation area of slash pine is 1.9 × 106 ha due to its strong adaptability and fast growth (Zheng et al., 2008). Meanwhile, Schima superba is the constructive species in subtropical evergreen broad-leaved forests and is also widely planted (Li et al., 2021). Although the construction of plantation provided a variety of ecological services, it is not entirely clear how vegetation types affect the C sequestration and allocation at the ecosystem level.
In this study, two coniferous forests (slash pine plantation, SPP and Masson pine plantation, MPP) and a broad-leaved forest (Schima superba plantation, SSP), which have been planted for 30 years in subtropical China were selected and investigated. The C densities of plants, litter, understory and soil in three plantations were calculated. The purpose of this study was to assess the effects of vegetation type on ecosystem C storage and its distribution pattern. We hypothesized that establishing broad-leaved trees was beneficial to ecosystem C sequestration compared with the coniferous plantations, because the broad-leaved forest is the zone vegetation in the subtropics. The results are expected to establish the best silvicultural practices based on C sequestration.
2. Materials and methods
2.1. Study area
The study area is located at the Vegetation Restoration Ecological Station of Degraded Ecosystem in Taihe County, Jiangxi Province (26°44′ N and 115°04′ E). The study area has a subtropical humid monsoon climate, and is hot and rainy in summer and warm and humid in winter. The average annual precipitation is about 1,700 mm, and the average annual temperature is 18.6°C. The annual frost-free period is 281 days. The soil is a typical red soil classified as Ferralsol with acidity and is moderately well drained and clay textured with a depth of over 100 cm. At present, the vegetation coverage in the study area has reached more than 0.9, and no obvious soil erosion has been found. The elevation is 70–130 m with an average slope of 15° (Fang et al., 2015).
2.2. Experimental design
The zonal vegetation in the study area is the evergreen broad-leaved forest, but the aboveground vegetation is mainly shrubs and herbs before 1990 due to the intensive human disturbance, such as fuel wood and grass cutting, root excavation, and overgrazing. In 1991, coniferous and broad-leaved species, such as Masson pine, slash pine, and Schima superba were selected for vegetation restoration to restore degraded land with a total area of 133 ha. Before the vegetation restoration, the study area had same stand conditions. Additional information on the study sites can be found in Fang et al. (2015).
In July 2021, a Masson pine plantation, a slash pine plantation and a Schima superba plantation established for 30 years were selected. Four plots were randomly chosen in different hills with an area of 20 m × 20 m for each kind of plantation. The plots were away from adjacent plots at least 100 m (Supplementary Figure 1). All plantations were 30-year-old and had reached the mature stage with the relatively stable ecosystem C storage and distribution, which were representative and could reflect the vegetation type on the plant and soil C storage. The sample plots were randomly distributed, which thus could characterize the variation of soil. Basic information such as elevation, slope, litter thickness, canopy density, diameter at breast height (DBH), and stand density of each forest type was shown in Table 1.
2.3. Plot survey and sampling
The DBH and height of all trees ≥2 cm in the sample plots were measured, and the species names were recorded. Five representative trees were selected according to the average DBH and tree height as standard trees to collect leaves, branches, trunks, and roots. Branches and leaves were collected from the middle and upper of the tree canopy. Trunks were collected with growth cones, and roots were collected by digging. Samples of the same organ from the same tree species in each plot were mixed to obtain respective composite samples. All samples were brought back to the laboratory and dried at 105°C for 30 min and at 65°C to a constant weight to determine the organic C concentration.
Five subplots of 2 m × 2 m were set up at the four corners and in the middle of the plots, and all shrubs with roots were collected in the subplots. In each subplot, 1 m × 1 m and 0.5 m × 0.5 m sub-sub plots were established for herb and litter collection, respectively. All samples of shrubs and herds were brought back to the laboratory, dried to constant weight at 65°C to calculate total biomass and ground to determine the organic C concentration (Ma et al., 2015).
Two soil profiles were randomly dug from each plot, and soil samples were collected at 0–15 cm, 15–30 cm, 30–45 cm, 45–60 cm, and 60–100 cm. After removing roots and organic debris, soil samples were air-dried and passed through a 2 mm sieve for determination of organic C concentration. Soil bulk density was determined by the coring method (Justine et al., 2015). The samples of two soil profiles were determined independently, but the average value was taken in the analysis of variance.
2.4. Organic C measurement
The dry samples of plant and soil were ground through 100 mesh, and the organic C concentration was determined by the Walkley-Black wet oxidation method (Allen, 1989; Nelson and Sommers, 1996).
2.5. Plant C stocks calculation
Tree organ biomass was estimated using specific biomass regression equations (Supplementary Table 1) with a diameter as the variable. The biomass of shrubs, herbs, and aboveground litter was measured by the dry weight per unit area. Plant C stock was calculated by the following equation (Zhang et al., 2020):
Where TPC, is the plant organic C stock (kg m–2); CP is the organic C concentration of each component (leaf, branch, trunk, root, shrub, herb, and aboveground litter) (g kg–1); MP, is the biomass of the corresponding component (kg m–2).
2.6. Soil C stock estimation
Soil organic C (SOC) stock was calculated by the following formula (Zhang et al., 2020):
Where TSC, is the SOC stock at 0–100 cm depth (kg m–2); i, is the depth of soil layer; Csi, is the SOC concentration in layer i (g kg–1); ρsi, is the bulk density of layer i (g cm–3); dsi, is the thickness of layer i (cm).
2.7. Statistical analysis
All data were tested for homogeneity of variances (Levene’s test) and used for subsequent calculations. One-way ANOVA and Duncan’s multiple comparisons were used to analyze the differences in the biomass, organic C concentration, and stocks among three plantations, tree organs and soil layers (α = 0.05). SPSS 22.0 software was used for all statistical analyzes.
3. Results
3.1. C storage of trees
The C density of trees under SPP, SSP, and MPP was 9.3, 11.8, and 8.5 kg m–2, respectively, where the trunk contributed 59.8% to the tree C density, followed by the roots with a proportion of 20.8% (Figures 1A, B). The tree C density was significantly lower in SPP and MPP than in SSP. The C density of branch and root in SSP was 55.3 and 77.7% higher than that of MPP, respectively (Figure 1B). The root C density in MPP was lower than that in SPP, while the opposite trend was found for the leaf C density. Meanwhile, organic C concentrations in all organs were higher in Masson pine and slash pine than that in Schima superba (Table 2).
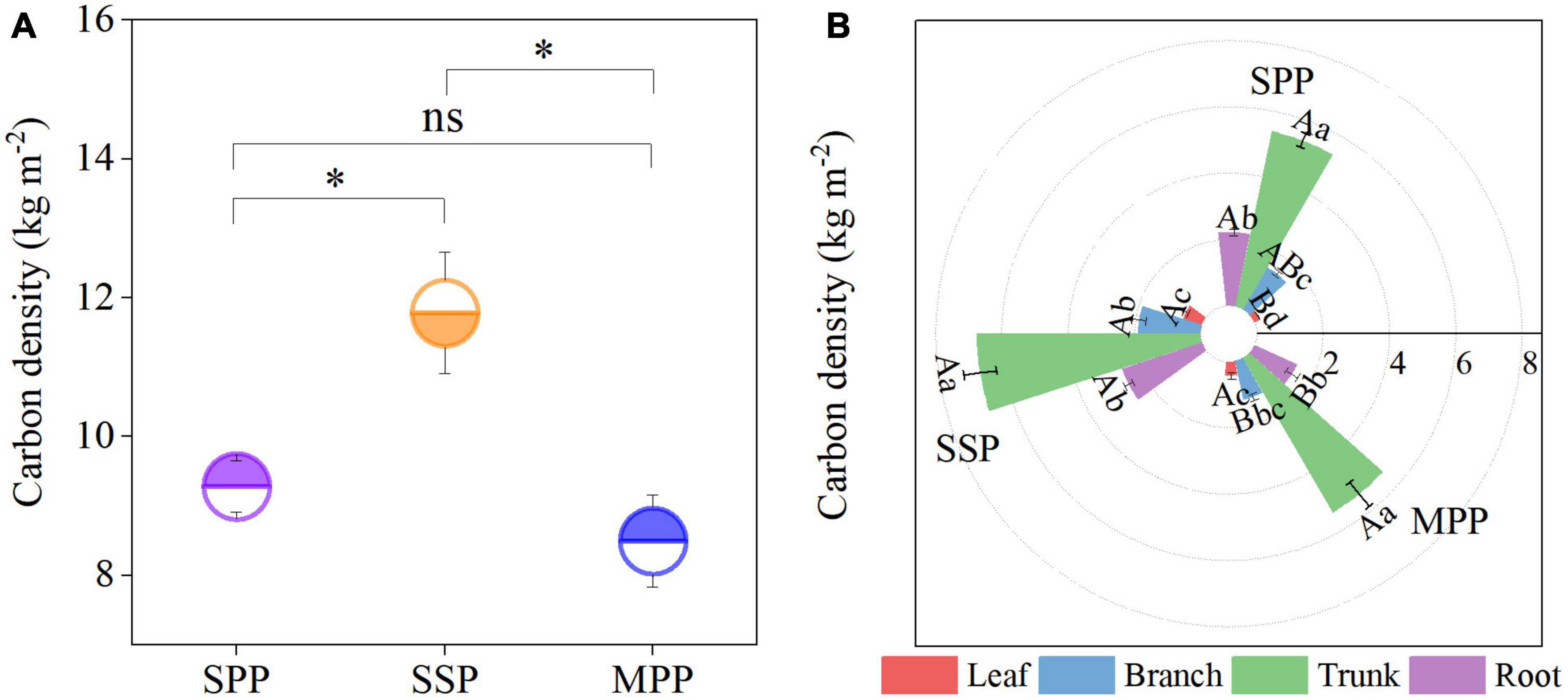
Figure 1. Tree carbon density (A) and its distribution in different organs (B) under three plantations in subtropical China. SPP, slash pine plantation; SSP, Schima superba plantation; MPP, Masson pine plantation. The asterisks (*) indicate significant differences between the two plantations (p < 0.05); ns: not significant. Different capital letters indicate significant differences among plantations of the same organ and different lowercase letters indicate significant differences among tree organs under the same plantation (p < 0.05).

Table 2. Organic carbon (OC) concentration in different organs of main tree species under three plantations in subtropical China.
3.2. C storage in the understory layer
The C density of SPP, SSP, and MPP in the understory layer (the sum of shrub, herb, and litter) was 0.60, 0.38, and 0.48 kg m–2, respectively. The litter C density was the highest, accounting for 92.1% of the C stock in the understory layer, while percentages of C stock in shrub and herb were 2.4 and 5.5%, respectively. The shrub C density in MPP were significantly higher than that in SSP. In contrast, the C density of herb and litter in SSP was lower than that in SPP. The C density in the understory layer was highest in SPP and was differ significantly among three plantations (Table 3).
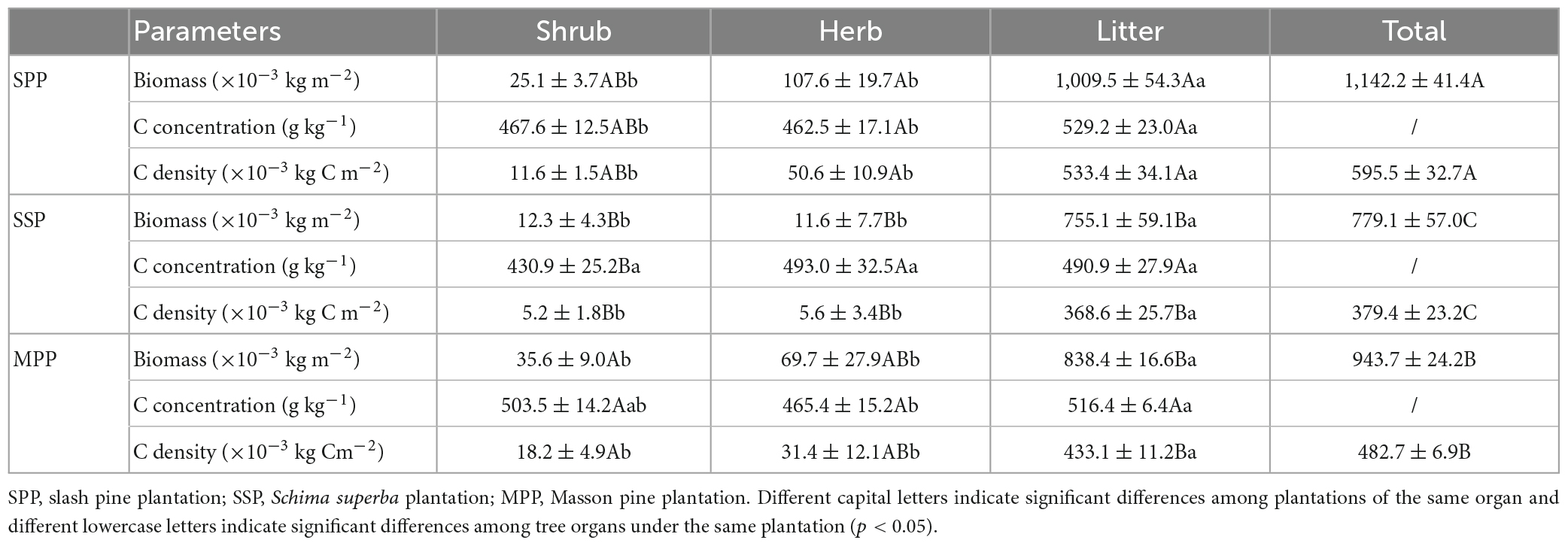
Table 3. Biomass, carbon concentration and carbon density of shrub, herb, and litter under three plantations in subtropical China.
3.3. Soil C storage
No significant difference was found for the SOC concentration at 0–30 cm among three plantations, and the SOC concentration at 30–60 cm in MPP was lower than that in SSP and SPP. SOC concentration decreased along the soil depth and was significantly higher in the 0–10 cm soil layer than that in other soil layers (Figure 2).
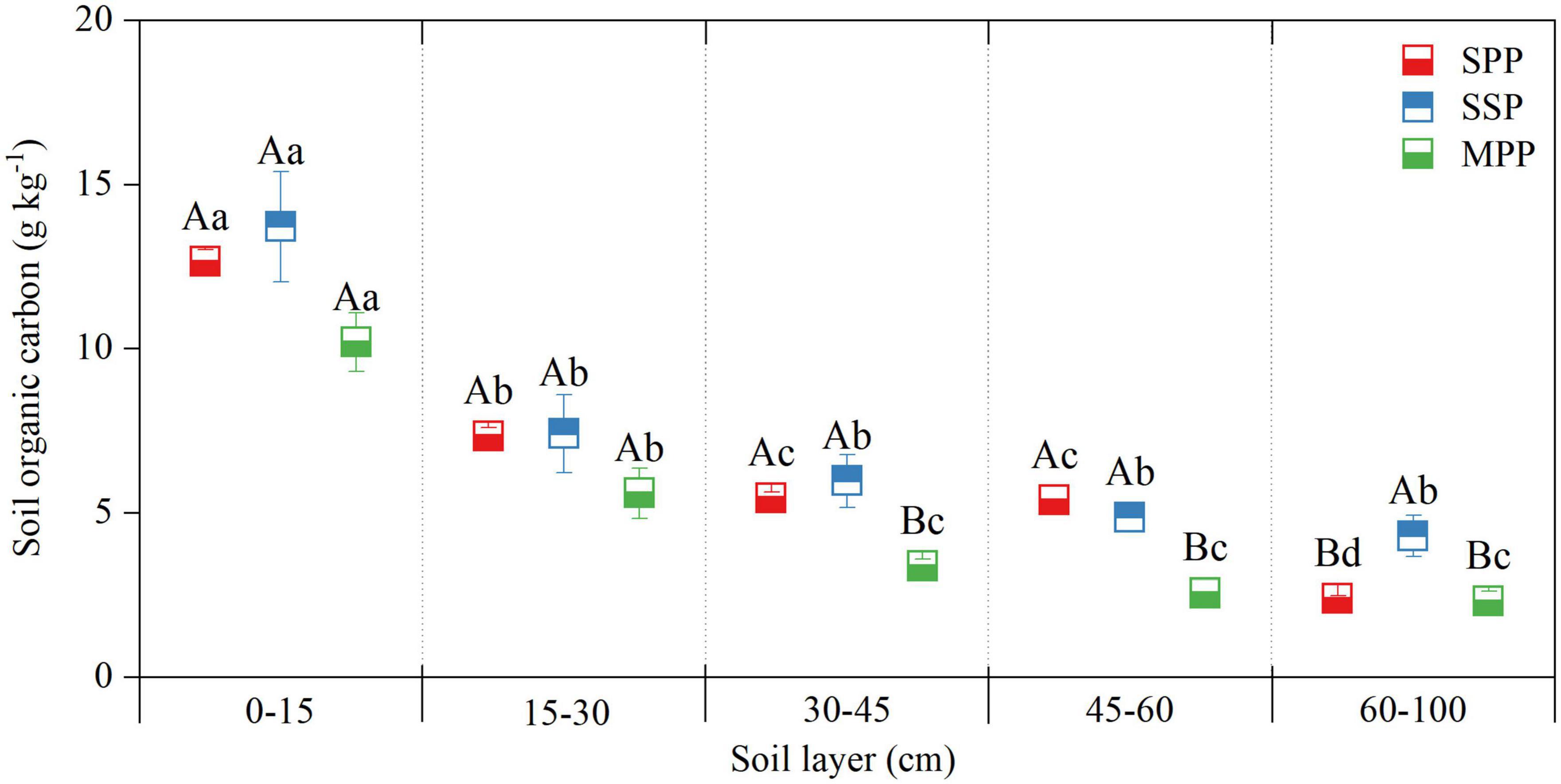
Figure 2. Soil organic carbon concentration in different soil layers at 0–1 m depth under three plantations in subtropical China. SPP, slash pine plantation; SSP, Schima superba plantation; MPP, Masson pine plantation. Different capital letters indicate significant differences among plantations of the same soil depth and different lowercase letters indicate significant differences among soil depths under the same plantation (p < 0.05).
The SOC density under SPP, SSP, and MPP was 7.8, 9.4, and 6.4 kg m–2, respectively (Figure 3). The SOC density of 0–15 cm soil layer accounted for 32.1–34.1% of the total SOC density and was significantly higher than that of other soil layers (Figure 3). The SOC density of SSP was higher than that of MPP. The SOC density of 30–45 cm in SSP and 45–60 cm in SPP was higher than that in MPP.
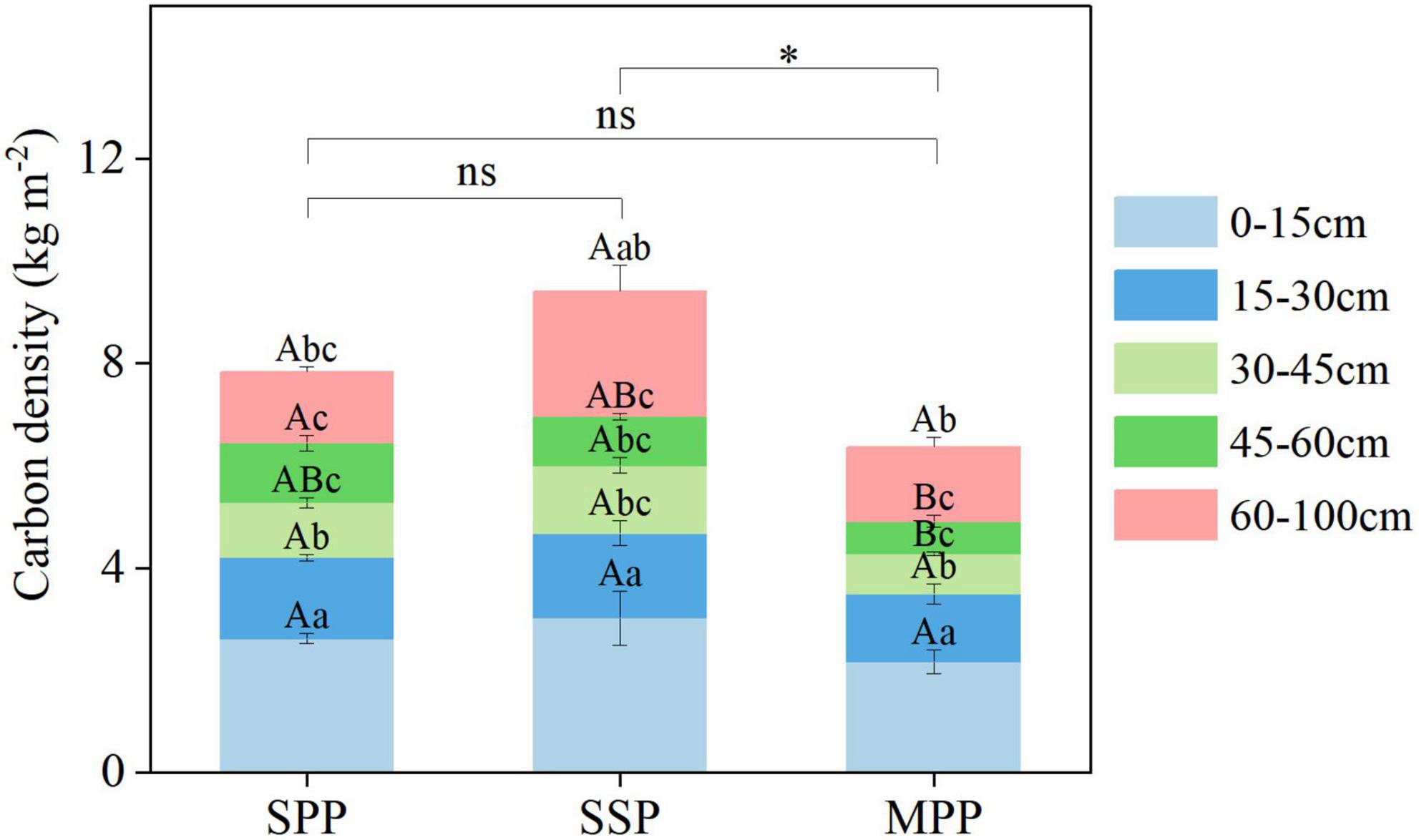
Figure 3. Soil carbon density and its distribution in different soil layers under three plantations in subtropical China. SPP, slash pine plantation; SSP, Schima superba plantation; MPP, Masson pine plantation. The asterisks (*) indicate significant differences between the two plantations (p < 0.05); ns: not significant. Different capital letters indicate significant differences among plantations of the same soil depth and different lowercase letters indicate significant differences among soil depths under the same plantation (p < 0.05).
3.4. Ecosystem C storage and their allocation
The ecosystem C density was 17.7, 21.6, and 15.3 kg m–2 for SPP, SSP, and MPP, respectively (Figure 4A). Ecosystem C stocks were mainly contributed by tree aboveground (39.9–46.0%) and SOC stocks (41.6–44.2%). The contribution of C stocks in the understory layer was less than 4% (Figure 4B).
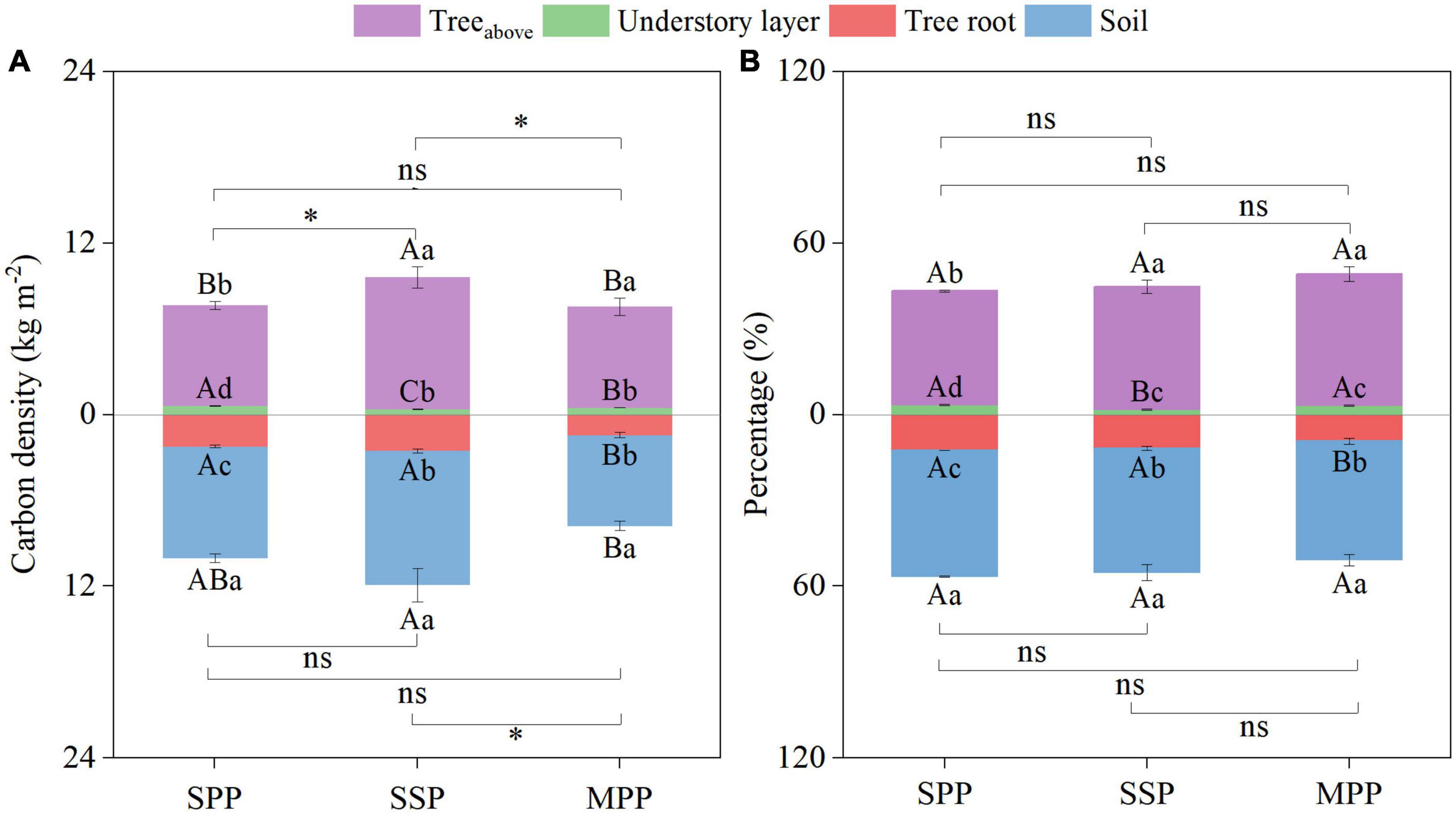
Figure 4. Absolute (A) and relative distribution (B) of ecosystem carbon density under three plantations in subtropical China. SPP, slash pine plantation; SSP, Schima superba plantation; MPP, Masson pine plantation. Treeabove: the sum of leaf, branch and trunk. Understory layer: the sum of shrub, herb and litter. The asterisks (*) indicate significant differences between the two plantations (p < 0.05) under aboveground (the sum of treeabove and understory layer) or underground (the sum of tree root and soil); ns: not significant. Different capital letters indicate significant differences among plantations of the same component and different lowercase letters indicate significant differences among components under the same plantation (p < 0.05).
The ecosystem C density of SSP was higher than that of MPP and SPP. Meanwhile, the aboveground C density (the sum of treeabove and understory layer) of SSP was 25.7 and 27.4% higher than that of SPP and MPP, respectively. And the underground C density (the sum of tree roots and soil) of MPP was 53.4% lower than that of SSP (Figure 4A). However, there was no significant differences in the proportion of C density of each component among three plantations (Figure 4B).
4. Discussion
4.1. Tree C stocks and their allocation in response to vegetation types
The average tree C density of three plantations was 9.9 kg m–2. This value is higher than the tree C density of Asian forests of 3.4–8.6 kg m–2 (FAO, 2010), but lower than the average tree C density of 12.6 kg m–2 for typical evergreen broad-leaved forests (Zhang et al., 2020) and of 12.4 kg m–2 for tropical forests (Chaudhury et al., 2022). In addition, the average C density of the tree root was 2.1 kg m–2, accounting for 11.2% of the total tree C density, which was in sharp contrast with the results of natural spruce forests in northwest China, where the root C density accounted for 4.9% of the total biomass C (Yue et al., 2018). This difference may be due to specific forest conditions (e.g., water availability and soil fertility), tree species or community characteristics.
Vegetation types significantly affected the tree C density, which was greater in the broad-leaved plantation than in coniferous plantations (Zhang et al., 2020). It is generally accepted that broad-leaved trees have relatively large biomass C (Zhang et al., 2020; Osei et al., 2022). In this study, the C density of tree root in SSP was higher than that in MPP, which was related to the physiological habit of tree species (Hu et al., 2021). Broad-leaved trees usually had relatively large root biomass (Kang et al., 2006; Hu et al., 2021), which ensured that broad-leaved trees were more competitive in the subtropics. Planting broad-leaved trees in subtropical regions facilitates the accumulation of C in biomass relative to coniferous trees, and this accumulation is mainly concentrated in the tree root layer. Therefore, the difference in the C sequestration capacity of tree root needs to be paid attention to in C assessment.
This research emphasized the importance of measured data. The organ C concentration of Masson pine and slash pine ranged from 49.3 to 58.9% and 45.5 to 57.9%, with an average of 53.7 and 52.3%, respectively, which was in the range of 46.8–58.6% reported by the previous study (Kang et al., 2006). However, the average C concentration of Schima superba in this study was 45.3%, and the mean C concentration of Masson pine and slash pine in four organs was 18.7 and 15.5% higher than that of Schima superba, respectively. It can be seen that using a C concentration factor of 0.5 will underestimate the C density of conifers but overestimate the C density of broad-leaved trees, thus reducing the difference between them (Thomas and Martin, 2012; Watzlawick et al., 2014). In a natural forest, it isn’t easy to measure the C content of each plant organ because of the high diversity of tree species. Still, the method of actual measurement should be encouraged to accurately estimate the C sequestration in plantation ecosystems.
Walkley-Black method is a common method for the determination of soil and plant organic C (He et al., 2021; Yang et al., 2023; Zhang et al., 2023). The concentration of organic C varies with soil layers, as well as between soil and plants. In the determination, the accuracy of the data determination can be ensured by adjusting the sample weighing. Moreover, there is a good correlation between the results of Walkley-Black method and dry combustion method (Wang et al., 2013). After multiplying the correction coefficient, the results are more accurate. In addition, the recalcitrance organic matter in the plant samples grounded through 100 meshes can be fully oxidized under the action of excessive H2SO4 and K2Cr2O7, so the determination results are comparable with other results. Therefore, Walkley-Black method was widely used in previous studies related to the determination of organic C concentration (He et al., 2021; Yang et al., 2023; Zhang et al., 2023).
4.2. C storage in the understory layer and soil in response to vegetation types
The C storage of understory plants and litter was closely related to vegetation types (Ma et al., 2015; Mensah et al., 2016). The C storage of understory layer in the coniferous plantation was higher than that in the broad-leaved plantation, which may be the reason for light and structure. Coniferous plantation usually has low canopy density, which is beneficial to the growth of understory plants and then increases the C storage of understory shrub and herb. Moreover, C storage of understory litter is directly related to that of the upper vegetation. The organic matter decomposition rate of coniferous trees was relatively low (Yang et al., 2004a,b), which will lead to a higher C density of litter in coniferous plantations, as observed in this study. However, the average C density of litter in this study was 0.44 kg m–2, much lower than evergreen broad-leaved forests in the same area (Zhang et al., 2020). This was mainly because none of plantations in this study had reached the over-mature stage, and there were few fallen trees with lower litter C density.
Vegetation types significantly affected the formation of soil C pools (Vesterdal et al., 2008; Subashree et al., 2019). We found significant differences in soil C concentration and density among three plantations. The SOC concentration of 30–60 cm in MPP was significantly lower than in SSP and SPP. Masson pine had a shallow root system, while the roots of Schima superba and slash pine can reach deep into the soil (Yan et al., 2013), which benefited the accumulation of SOC in subsoil. Moreover, the slow root litter decomposition rate in the coniferous plantation compared with broad-leaved trees led to a relatively low SOC concentration. e.g., many studies shown that Schima superba root litter was easier to decompose than Masson pine (Yang et al., 2004b). Thus, the SOC concentration and storage at 30–45 cm in SSP was significantly higher than that in MPP. In addition, root litter and exudates strongly regulated the biomass and biological activity of soil microorganisms by changing the C input (Shen et al., 2020), and increasing rhizosphere C input, which will lead to higher organic C decomposition (Finzi et al., 2015; Shen et al., 2020). Therefore, the higher C input and the acceleration of decomposition may counteract each other, resulting in no significant difference in the soil C storage at 0–30 cm among three plantations.
4.3. Ecosystem C storage and distribution in response to vegetation types
The average C density of three plantations was 18.2 kg m–2, which was lower than the range of 28.3–46.0 kg m–2 reported in the subtropical Mytilaria laosensis plantation (Ming et al., 2014) and was in the range of 12.6–21.7 kg m–2 observed in global plantations (FAO, 2010). The C density of the coniferous plantation was 16.5 kg m–2, which was roughly similar to the national average of 16.4 kg m–2 in China (Yu et al., 2000). However, the average C density of all three plantations was much lower than the average of evergreen broad-leaved forests in China (30.9 kg m–2) (Yu et al., 2000) and in globe (24.4 kg m–2) (Helmer et al., 2008). This suggests that the size of ecosystem C pools depends on many factors, such as climate and species composition, etc., (Toriyama et al., 2015; Sheikh et al., 2020).
Among the biomass C, tree account for the largest proportion of ecosystem C stock, followed by litter, then shrub and herb, which was consistent with previous studies (Kang et al., 2006; He et al., 2021). However, Zhu et al. (2010) showed that the aboveground tree biomass consistently had a larger C pool than soil, which was inconsistent with our results. In this study, the average aboveground tree and soil accounted for 42.9 and 43.1% of ecosystem C stocks, respectively. There was a larger soil C pool than aboveground biomass C stock in SSP and SPP, which may be because the ratio of aboveground tree C storage to soil increased with the increase of tree ages (He et al., 2021) and indicates the importance of soil in forest C sequestration.
The ecosystem C density of the broad-leaved plantations was higher than that of coniferous plantations, suggesting that planting broad-leaved trees is effective forest management measures to increase forest C storage in subtropical areas. The differences in ecosystem C stocks under three plantations were found both in the aboveground and belowground parts. Usually, in plantation ecosystems, much attention has been focused on changes in the aboveground biomass C storage (Mukaro et al., 2017), ignoring the effects of soil and tree root on C sequestration. As we highlighted earlier, broad-leaved trees had a relatively large litterfall, root biomass, and faster root and litter turnover rates, which increased soil and root C sequestration (Vesterdal et al., 2008; Zhang et al., 2009). Moreover, the broad-leaved tree is the constructive species of the climax community in this area, which has good adaptability to the regional climate (Sun et al., 2014; Li et al., 2021). Thus, planting broad-leaved plantations could improve soil microbial characteristics and fertility, stimulate soil nutrient cycling, which in turn enhanced aboveground plant nutrient uptake and C sequestration and ultimately increased ecosystem density (Rehschuh et al., 2021).
Altitude is one of the factors affecting the carbon density of forest ecosystem and forest vegetation C density exhibited decreasing trends along the altitudinal gradient (Zhu et al., 2010). In this study, the altitude of SSP was lower than that of MPP and SPP, and tree C density had an opposite trend. Although the result was like this, the average altitude difference was about 8 m, which was generally believed to cause a temperature difference of only 0.048°C (Danielson et al., 2003). Such a small temperature variation may be masked by microclimate differences caused by plantation communities (Seidelmann et al., 2016; Joly et al., 2017). Therefore, we reasonably speculated that the difference in tree C storage between the three plantations was caused by the growth characteristics of broad-leaved trees and coniferous trees as mentioned earlier.
5. Conclusion
This study emphasized the impact of vegetation type on ecosystem C storage in subtropical areas and found that planting the broad-leaved plantation were beneficial to more C storage. This result provided a theoretical basis for us to choose appropriate forest management measures. Moreover, the soil and root C density of broad-leaved was higher than that of the coniferous plantation, which highlighted the importance of forest underground C storage in evaluating ecosystem C storage. This study suggests that establishing the broad-leaved plantation benefits more C conservation in subtropical areas.
Data availability statement
The original contributions presented in this study are included in the article/Supplementary material, further inquiries can be directed to the corresponding author.
Author contributions
WQH: investigation, data analysis, and writing—original draft. BX and YYZ: perform the experiments. FSC: conceptualization and methodology. XQD, GXZ, and ZJW: investigation. XMF: conceptualization, methodology, and writing—review and editing. All authors contributed to the article and approved the submitted version.
Funding
This work was supported by the National Natural Science Foundation of China (32260379), the Jiangxi Provincial Science Fund for Distinguished Young Scholars (20224ACB215005), the Jiangxi “Double Thousand Plan” (jxsq2019201080), and the Jiangxi Provincial Key R&D Program (20214BBG74008).
Acknowledgments
We thank Tinglong Qu, Jin Ma, Tongtao Hu, and Xueli Jiang for their help in sampling and analyzes.
Conflict of interest
The authors declare that the research was conducted in the absence of any commercial or financial relationships that could be construed as a potential conflict of interest.
Publisher’s note
All claims expressed in this article are solely those of the authors and do not necessarily represent those of their affiliated organizations, or those of the publisher, the editors and the reviewers. Any product that may be evaluated in this article, or claim that may be made by its manufacturer, is not guaranteed or endorsed by the publisher.
Supplementary material
The Supplementary Material for this article can be found online at: https://www.frontiersin.org/articles/10.3389/ffgc.2023.1149799/full#supplementary-material
References
Bonan, G. B. (2008). Forests and climate change: Forcings, feedbacks, and the climate benefits of forests. Science 320, 1444–1449. doi: 10.1126/science.1155121
Chaudhury, G., Darji, C. B., Basumatari, M., Dutta, G., Devi, A., and Bharadwaj, N. (2022). Stand structure, biomass and carbon stock along disturbance gradients in differently managed tropical forests of Assam, northeast India. Trees For. People 9:100296. doi: 10.1016/j.tfp.2022.100296
Fang, X. M., Chen, F. S., Wan, S. Z., Yang, Q. P., and Shi, J. M. (2015). Topsoil and deep soil organic carbon concentration and stability vary with aggregate size and vegetation type in subtropical China. PLoS One 10:e0139380. doi: 10.1371/journal.pone.0139380
FAO. (2010). Global forest resources assessment, Main Report; FAO Forestry Paper 163. Rome Italy: FAO.
Finzi, A. C., Abramoff, R. Z., Spiller, K. S., Brzostek, E. R., Darby, B. A., Kramer, M. A., et al. (2015). Rhizosphere processes are quantitatively important components of terrestrial carbon and nutrient cycles. Glob. Change Biol. 21, 2082–2094. doi: 10.1111/gcb.12816
He, J., Dai, Q. H., Xu, F. W., Peng, X. D., and Yan, Y. J. (2021). Variability in carbon stocks across a chronosequence of Masson pine plantations and the trade-off between plant and soil systems. Forests 12:1342. doi: 10.3390/f12101342
Helmer, E. H., Brandeis, T. J., Lugo, A. E., and Kennaway, T. (2008). Factors influencing spatial pattern in tropical forest clearance and stand age: Implications for carbon storage and species diversity. J. Geophys. Res. Biogeosci. 113:G02S04. doi: 10.1029/2007jg000568
Houghton, R. A., Skole, D., Nobre, C. A., Hackler, J., Lawrence, K., and Chomentowski, W. H. (2000). Annual fluxes of carbon from deforestation and regrowth in the Brazilian Amazon. Nature 403, 301–304. doi: 10.1038/35002062
Hu, M. Y., Zou, B. Z., Huang, Z. Q., Wang, S. R., Su, X. P., Ding, X. X., et al. (2021). Fine root biomass and necromass dynamics of chinese fir plantations and natural secondary forests in subtropical China. For. Ecol. Manag. 496:119413. doi: 10.1016/j.foreco.2021.119413
Joly, F. X., Milcu, A., Scherer-Lorenzen, M., Jean, L. K., Bussotti, F., Dawud, S. M., et al. (2017). Tree species diversity affects decomposition through modified micro-environmental conditions across European forests. New Phytol. 214, 1281–1293. doi: 10.1111/nph.14452
Justine, M. F., Yang, W., Wu, F., and Khan, M. N. (2017). Dynamics of biomass and carbon sequestration across a chronosequence of Masson pine plantations. J. Geophys. Res. Biogeosci. 122, 578–591. doi: 10.1002/2016jg003619
Justine, M., Yang, W. Q., Wu, F. Z., Tan, B., Khan, M., and Zhao, Y. Y. (2015). Biomass stock and carbon sequestration in a chronosequence of Pinus massoniana plantations in the upper reaches of the Yangtze River. Forests 6, 3665–3682. doi: 10.3390/f6103665
Kang, B., Liu, S. R., Zhang, G. J., Chang, J. G., Wen, Y. G., Ma, J. M., et al. (2006). Carbon accumulation and distribution in Pinus massoniana and Cunninghamia lanceolata mixed forest ecosystem in Daqingshan, Guangxi, China. Acta Ecol. Sin. 26, 1320–1327. doi: 10.1016/s1872-2032(06)60024-3
Kuang, Y. W., Sun, F. F., Wen, D. Z., Zhou, G. Y., and Zhao, P. (2008). Tree-ring growth patterns of Masson pine (Pinus massoniana L.) during the recent decades in the acidification Pearl River Delta of China. For. Ecol. Manag. 255, 3534–3540. doi: 10.1016/j.foreco.2008.02.036
Li, W. Q., Huang, Y. X., Chen, F. S., Liu, Y. Q., Lin, X. F., Zong, Y. Y., et al. (2021). Mixing with broad-leaved trees shapes the rhizosphere soil fungal communities of coniferous tree species in subtropical forests. For. Ecol. Manag. 480:118664. doi: 10.1016/j.foreco.2020.118664
Ma, J., Bu, R. C., Liu, M., Chang, Y., Qin, Q., and Hu, Y. M. (2015). Ecosystem carbon storage distribution between plant and soil in different forest types in northeastern China. Ecol. Eng. 81, 353–362. doi: 10.1016/j.ecoleng.2015.04.080
Mensah, S., Veldtman, R., du Toit, B., Glèlè Kakaï, R., and Seifert, T. (2016). Aboveground biomass and carbon in a South African mistbelt forest and the relationships with tree species diversity and forest structures. Forests 7:79. doi: 10.3390/f7040079
Ming, A., Jia, H., Zhao, J., Tao, Y., and Li, Y. (2014). Above-and below-ground carbon stocks in an indigenous tree (Mytilaria laosensis) plantation chronosequence in subtropical China. PLoS One 9:e109730. doi: 10.1371/journal.pone.0109730
Moomaw, W. R., Masino, S. A., and Faison, E. K. (2019). Intact forests in the united states: Proforestation mitigates climate change and serves the greatest good. Front. For. Glob. Change 2:00027. doi: 10.3389/ffgc.2019.00027
Mukaro, E., Nyakudya, I. W., and Jimu, L. (2017). Edaphic conditions, aboveground carbon stocks and plant diversity on nickel mine tailings dump vegetated with Senegalia polyacantha (Willd.) Seigler & Ebinger. Land Degrad. Dev. 28, 1641–1651. doi: 10.1002/ldr.2696
Nelson, D. W., and Sommers, L. E. (1996). “Total carbon, organic carbon, and organic matter,” in Methods of soil analysis: Part 3 Chemical methods, ed. D. L. Sparks (Madison, WI: Soil Science Society of America), 961–1010.
Osei, R., Del Rio, M., Ruiz-Peinado, R., Titeux, H., Bielak, K., Bravo, F., et al. (2022). The distribution of carbon stocks between tree woody biomass and soil differs between Scots pine and broadleaved species (beech, oak) in European forests. Eur. J. For. Res. 141, 467–480. doi: 10.1007/s10342-022-01453-9
Rehschuh, S., Jonard, M., Wiesmeier, M., Rennenberg, H., and Dannenmann, M. (2021). Impact of European beech forest diversification on soil organic carbon and total nitrogen stocks–a meta-analysis. Front. For. Glob. Change 4:606669. doi: 10.3389/ffgc.2021.606669
Seidelmann, K. N., Scherer-Lorenzen, M., and Niklaus, P. A. (2016). Direct vs. microclimate-driven effects of tree species diversity on litter decomposition in young subtropical forest stands. PLoS One 11:e0160569. doi: 10.1371/journal.pone.0160569
Sheikh, M. A., Kumar, M., Todaria, N. P., and Pandey, R. (2020). Biomass and soil carbon along altitudinal gradients in temperate Cedrus deodara forests in Central Himalaya, India: Implications for climate change mitigation. Ecol. Indic. 111:106025. doi: 10.1016/j.ecolind.2019.106025
Shen, X., Yang, F., Xiao, C. W., and Zhou, Y. (2020). Increased contribution of root exudates to soil carbon input during grassland degradation. Soil Biol. Biochem. 146:107817. doi: 10.1016/j.soilbio.2020.107817
Subashree, K., Dar, J. A., and Sundarapandian, S. (2019). Variation in soil organic carbon stock with forest type in tropical forests of Kanyakumari Wildlife Sanctuary, Western Ghats, India. Environ. Monit. Assess. 191, 1–16. doi: 10.1007/s10661-019-7881-6
Sun, Y., Hu, H., Huang, H., and Vargas-Mendoza, C. F. (2014). Chloroplast diversity and population differentiation of Castanopsis fargesii (Fagaceae): A dominant tree species in evergreen broad-leaved forest of subtropical China. Tree Genet. Genomes 10, 1531–1539. doi: 10.1007/s11295-014-0776-3
Thomas, S. C., and Martin, A. R. (2012). Carbon content of tree tissues: A synthesis. Forests 3, 332–352. doi: 10.3390/f3020332
Toriyama, J., Hak, M., Imaya, A., Hirai, K., and Kiyono, Y. (2015). Effects of forest type and environmental factors on the soil organic carbon pool and its density fractions in a seasonally dry tropical forest. For. Ecol. Manag. 335, 147–155. doi: 10.1016/j.foreco.2014.09.037
Vesterdal, L., Schmidt, I. K., Callesen, I., Nilsson, L. O., and Gundersen, P. (2008). Carbon and nitrogen in forest floor and mineral soil under six common European tree species. For. Ecol. Manag. 255, 35–48. doi: 10.1016/j.foreco.2007.08.015
Wang, J. P., Wang, X. J., and Zhang, J. (2013). Evaluating loss-on-Ignition method for determinations of soil organic and Inorganic carbon in arid soils of Northwestern China. Pedosphere 23, 593–599. doi: 10.1016/s1002-0160(13)60052-1
Watzlawick, L. F., Martins, P. J., Rodrigues, A. L., Ebling, Â. A., Balbinot, R., and Lustosa, S. B. C. (2014). Carbon concentration in species of the araucaria forest and effect of the ecological group. Cerne 20, 613–620. doi: 10.1590/01047760201420041492
Yan, H., Dong, X., Feng, G., Zhang, S., and Mucciardi, A. (2013). Coarse root spatial distribution determined using a ground-penetrating radar technique in a subtropical evergreen broad-leaved forest, China. Sci. China Life Sci. 56, 1038–1046. doi: 10.1007/s11427-013-4560-7
Yang, L., Lin, Y., Kong, J., Yu, Y., He, Q., Su, Y., et al. (2023). Effects of fertilization and dry-season irrigation on the timber production and carbon storage in subtropical Eucalyptus plantations. Ind. Crops. Prod. 192:116143. doi: 10.1016/j.indcrop.2022.116143
Yang, Y. S., Chen, G. S., Lin, P., Xie, J. S., and Guo, J. F. (2004a). Fine root distribution, seasonal pattern and production in four plantations compared with a natural forest in Subtropical China. Ann. For. Sci. 61, 617–627. doi: 10.1051/forest:2004062
Yang, Y. S., Guo, J. F., Chen, G. S., Xie, J. S., Cai, L. P., and Lin, P. (2004b). Litterfall, nutrient return, and leaf-litter decomposition in four plantations compared with a natural forest in subtropical China. Ann. For. Sci. 61, 465–476. doi: 10.1051/forest:2004040
Yin, G., Zhang, Y., Sun, Y., Wang, T., Zeng, Z., and Piao, S. (2015). Modis based estimation of forest aboveground biomass in China. PLoS One 10:e0130143. doi: 10.1371/journal.pone.0130143
Yu, Z. L., Zhao, S. D., and Zhou, Y. R. (2000). Carbon storage and budget of major chinese forest types. Chin. J. Plant Ecol. 24, 518–522.
Yue, J. W., Guan, J. H., Deng, L., Zhang, J. G., Li, G., and Du, S. (2018). Allocation pattern and accumulation potential of carbon stock in natural spruce forests in Northwest China. PeerJ 6:e4859. doi: 10.7717/peerj.4859
Zhang, G., Bai, J., Wang, W., Jia, J., Huang, L., Kong, F., et al. (2023). Plant invasion reshapes the latitudinal pattern of soil microbial necromass and its contribution to soil organic carbon in coastal wetlands. Catena 222:106859. doi: 10.1016/j.catena.2022.106859
Zhang, H., Chu, L., and Zhuang, X. (2009). Decomposition of leaf litter of four native broad-leaved tree species in South China. Front. For. China 4:450–457. doi: 10.1007/s11461-009-0062-y
Zhang, Z., Zhong, Q., Cheng, D., Huang, Z., Xu, C., Yu, H., et al. (2020). Carbon dynamics in three subtropical forest ecosystems in China. Environ. Sci. Pollut. Res. 27, 15552–15564. doi: 10.1007/s11356-019-06991-1
Zheng, H., Ouyang, Z., Xu, W., Wang, X., Miao, H., Li, X., et al. (2008). Variation of carbon storage by different reforestation types in the hilly red soil region of southern China. For. Ecol. Manag. 255, 1113–1121. doi: 10.1016/j.foreco.2007.10.015
Keywords: forest ecosystem C density, monoculture plantation, vegetation type, underground C storage, soil C stock
Citation: Huang W-Q, Xu B, Chen F-S, Zong Y-Y, Duan X-Q, Zhang G-X, Wu Z-J and Fang X-M (2023) The effects of vegetation type on ecosystem carbon storage and distribution in subtropical plantations. Front. For. Glob. Change 6:1149799. doi: 10.3389/ffgc.2023.1149799
Received: 23 January 2023; Accepted: 07 April 2023;
Published: 24 April 2023.
Edited by:
Jianping Wu, Yunnan University, ChinaReviewed by:
Weifeng Wang, Nanjing Forestry University, ChinaIngeborg Callesen, University of Copenhagen, Denmark
Copyright © 2023 Huang, Xu, Chen, Zong, Duan, Zhang, Wu and Fang. This is an open-access article distributed under the terms of the Creative Commons Attribution License (CC BY). The use, distribution or reproduction in other forums is permitted, provided the original author(s) and the copyright owner(s) are credited and that the original publication in this journal is cited, in accordance with accepted academic practice. No use, distribution or reproduction is permitted which does not comply with these terms.
*Correspondence: Xiang-Min Fang, eG1mYW5nMjAxM0AxMjYuY29t