- 1Department of Biotechnology and Life Science, University of Insubria, Varese, Italy
- 2Department of Economics, University of Insubria, Varese, Italy
Quercus rubra L. is a moderately shade-tolerant tree species native to eastern North America, readily regenerating since its introduction in the Central and Southern European forests to displace the native pedunculate oak (Quercus robur). Under a scenario of increasing drought, understanding the seedling responses of these two species to water limitation is critical for forest conservation and management. To this aim, morphological, physiological and non-structural carbohydrates analyzes were performed on very-fine and fine roots of Q. robur and Q. rubra seedlings grown under controlled conditions and exposed to two levels of drought before allowing them to recover. Results show significant differences between species for all the investigated traits. The alien Q. rubra showed lower shoot and root biomasses than the native Q. robur, particularly for the thicker fine root 1–2 mm diameter class. However, both species equally invested more biomass in the shoot than the root system (R:S ratio <1). A significant response to drought was observed for the 0.5–1 mm fine root class, with moderate and severe droughted seedlings showing slightly higher biomass than control, particularly in Q. rubra. The overall growth reduction of Q. rubra suggests that the costs associated with the construction and maintenance outweigh the inputs from aboveground, as supported by the lower values of photochemical efficiency (Fv/Fm), quantum yield (ΦPSII) of PSII and stomatal conductance. In particular, the reduced stomatal conductance assured high midday leaf water potential (i.e., tissue hydration levels) at the expense of growth. The low starch concentration in Q. rubra’s very-fine roots correlated positively with the low photochemical efficiency under drought conditions, probably due to the reduction of photosynthate inputs from aboveground. In contrast to the anisohydric behavior reported, these outcomes highlight a rather isohydric behavior for Q. rubra, at least at the seedling stage and in the adopted experimental conditions, making this species highly competitive under the drier condition in the canopy openings during the summer period.
1 Introduction
Quercus rubra L. (red oak) is an economically important tree species native to eastern North America, well-known for its moderate shade-tolerance behavior (Sander, 1990). In addition, red oak is the dominant canopy species in many northern hardwood forests, being relevant in terms of hydrologic budgets and carbon sequestration (Cavender-Bares and Bazzaz, 2000).
Although this species is facing difficulties in regenerating in several areas within its native range (Crow, 1992; Abrams, 1996), red oak has been successfully regenerating since its introduction in Central European forests estimated at the turn of the 17th and 18th centuries (Vansteenkiste et al., 2005; Major et al., 2013), and in Italian forests since the early-19th century (Maniero, 2015). Concerns are growing over whether its rapid growth in European forests means this non-native species is becoming an aggressive invader (Chmura and Sierka, 2005; Vor, 2005; Kuehne et al., 2014), as different studies reported adverse effects on native biodiversity (Reinhardt et al., 2003; Chmura, 2020; Dimitrova et al., 2022). Related, an average annual growth of 12 m3 ha−1 year−1 was estimated, outperforming all native oak species, including the indigenous Quercus robur (English oak, pedunculate oak) (Kiedrzyński et al., 2011) that reaches only about half of this productivity (Nicolescu et al., 2020; Kormann et al., 2023).
Several authors highlighted potential recruitment differences of red oak when analyzing the native and introduced ranges in favor of the latter (Steiner et al., 1993; Major et al., 2013). In the years following the establishment of red oak seedlings, soil moisture content appears to play a crucial role in their survival and growth (Jacobs et al., 2009), but several studies and regional reports suggest that Q. rubra has better resistance to drought than the native Q. robur and Quercus petraea (sessile oak) (Nicolescu et al., 2020 and references therein). In the native range, red oak drought adaptation is associated with the rapid production of large seedlings from large seeds, allowing seedlings to avoid drought by quickly accessing water in deeper soil layers (Walters et al., 2023). Similar behavior has also been reported for Q. robur (Zadworny et al., 2021), whose valid seedling response to water limitation appears to similarly depend on the root-to-shoot allometry, the taproot development, and carbon reserve mobilization for plant functioning under water shortage. In contrast, other evidence highlights, particularly for red oak seedlings, that large root systems do not show enhanced drought avoidance during their first season after field transplant (Jacobs et al., 2009; Walters et al., 2023).
At a finer scale, drought is known to stimulate fine roots (diameter <2 mm), especially very-fine root (diameter <0.5 mm) growth (Montagnoli et al., 2012, 2019) and starch accumulation (Domingo et al., 2023) up to a threshold beyond which growth stops, starch consumption and even mortality increase (for a review see Brunner et al., 2015; Di Iorio et al., 2016; Domingo et al., 2023). Sustained dry soil highlights this pattern also for the fine roots of both Q. robur (Gieger and Thomas, 2002; Zadworny et al., 2021) and Q. rubra (Jacobs et al., 2009; Zadworny et al., 2018). Stimulation of very fine root growth is often accompanied by increased specific root length (SRL, m g−1), i.e., the ratio of the length to root mass, which is proportional to resource acquisition (benefit) and construction and maintenance (cost), respectively (Eissenstat and Yanai, 1997; Montagnoli et al., 2019). A high SRL value indicates an optimal benefit/cost ratio under drought conditions (Ostonen et al., 2007).
Water shortage and carbon starvation are the leading causes of fine-roots and other tree compartments’ mortality (Hartmann et al., 2013). Indeed, even very-fine roots are crucial concerning starch dynamics since previous studies highlighted that starch and soluble sugars (SS) were significantly replenished at the end of the growing season (Nguyen et al., 1990; Kosola et al., 2002). However, unlike the well-documented physiological and morphological plasticity, existing studies for these two species are few and/or of limited scope in terms of temporal variation of the starch concentration and growth in response to drought.
Drought resistance and mortality of tree species growing under water-shortage conditions have been widely assessed through the iso/anisohydric classification (Martínez-Vilalta et al., 2014). In particular, isohydric species are more prone to carbon starvation, and anisohydric ones are more vulnerable to hydraulic failure (Garcia-Forner et al., 2016). Red oak (Yi et al., 2017), pedunculate oak (Gieger and Thomas, 2002) and sessile oak (Aranda et al., 2000; Klein, 2014; Martínez-Sancho et al., 2017) are usually considered anisohydric species, i.e., able to maintain high transpiration and stomatal conductance and to track environmental fluctuations in water potentials under moderate drought conditions (Martínez-Vilalta et al., 2014). In their native range, seedlings of red oak are considered less drought-resistant than white (Quercus alba) or black oaks (Quercus velutina) (Sander, 1990). In contrast, in the introduced ranges, red oak seedlings appear more drought resistant than the native pedunculate and sessile oaks (Nicolescu et al., 2020, and references therein). Therefore, red oak appears to efficiently regenerate under the drier conditions of the exposed soil surface generated by the regular thinning of managed European forests. Under a scenario of increasing drought, understanding the seedling responses of these two species to water limitation becomes critical, as drought represents an abiotic stress that in the next future may select the forest tree species composition in favor of more drought-resistant alien species. Management activities should promote both the native Q. robur for forest biodiversity conservation and the alien Q. rubra for its valuable wood properties and high economic value (Nicolescu et al., 2020).
Based on this evidence, it was hypothesized that Q. rubra seedlings modulate morpho-physiological traits for better coping with severe drought stress compared to Q. robur. In particular, for Q. rubra, it was expected (i) greater biomass production, (ii) higher specific root length (SRL) and starch accumulation, and (iii) higher PSII yield and stomatal conductance.
To test this hypothesis, Q. robur and Q. rubra seedlings were grown under controlled conditions and exposed to progressive soil drying from moderate to severe before being brought to recover. The growth and related functional characteristics were evaluated, including total biomass, leaf chlorophyll fluorescence, stomatal conductance, and water potential for the aboveground portion, fine root length and biomass by diameter classes and non-structural carbohydrates (starch and soluble sugars) for the very-fine roots only for the belowground portion.
2 Materials and methods
2.1 Plant material and growing conditions
Acorns of Q. rubra were collected from a 69 years-old plantation located within the Parco Regionale delle Groane (Lentate sul Seveso, Lombardy region, Italy; 45° 41′ 19.748″ N, 9° 8′ 23.308″ E). A similar amount of Q. robur acorns were provided by the ‘Centro Nazionale per lo studio e la conservazione della Biodiversità Forestale di Peri’ (VR, Italy). The season preceding the acorn harvest was not affected by extreme climatic events such as severe droughts, heat waves or floods. After hydration by immersion for 24 h, 12 acorns were sown at an 8 cm distance from each other in a 3.5 L tray (30 × 23 cm height 10 cm), for a total of five trays and 60 acorns per species, filled with a 1:1:2 mixture of peat, compost, and sand (pH 6.0–6.8). These trays were positioned in a growth chamber under 25/18°C day/night temperature, 100 μmol m−2 s−1 PPFD, 16 h photoperiod, and 45/70% relative humidity (RH). Thirty days after the germination, 96 uniformly sized seedlings (48 per species) were transplanted in larger pots (24 h × 24 ⌀ cm; volume 9.5 L; one seedling per pot) filled with the same medium with the addition of 20 g of slow-release fertilizer (NPK 14-7-14). The plants were grown under 25/18°C day/night temperature, 350 μmol m−2 s−1 PPFD, 16 h photoperiod, and 45/70% relative humidity (RH) throughout the experiment. All plants, 96 in total, were watered to 70%–80% field capacity for additional 60 days until shoot expansion ceased and then were set up for the experimental design (3 months-old seedlings).
2.2 Drought application and experimental design
The experiment lasted 44 days, and a sketch of the design is illustrated in Figure 1. A cohort equal to half of the seedlings (24 for each species) was maintained at field capacity throughout the experiment time course (control). Two drought intensities, moderate-drought stress (MD) and severe-drought stress (SD), were applied sequentially to the exact second-half cohort of seedlings (24 for each species). To this aim, volumetric soil water content (SWC, % vol) at field capacity was determined by the gravimetric method (Louki and Al-Omran, 2022). In detail, (i) potting soil was drenched and left to drain overnight, (ii) the following morning, a wet 200 mL soil sample was weighed and oven-dried at 105°C to a constant weight, and finally, (iii) the dried soil sample was weighed to measure the water mass lost. SWC was calculated by multiplying the gravimetric water value by the bulk density (0.487 g cm−3) and associated with Time Domain Reflectometry (TDR; model ML2x, ΔT Devices, Cambridge, UK) measurements with 1% error. Successively, SWC was monitored every 3–4 days only by TDR.
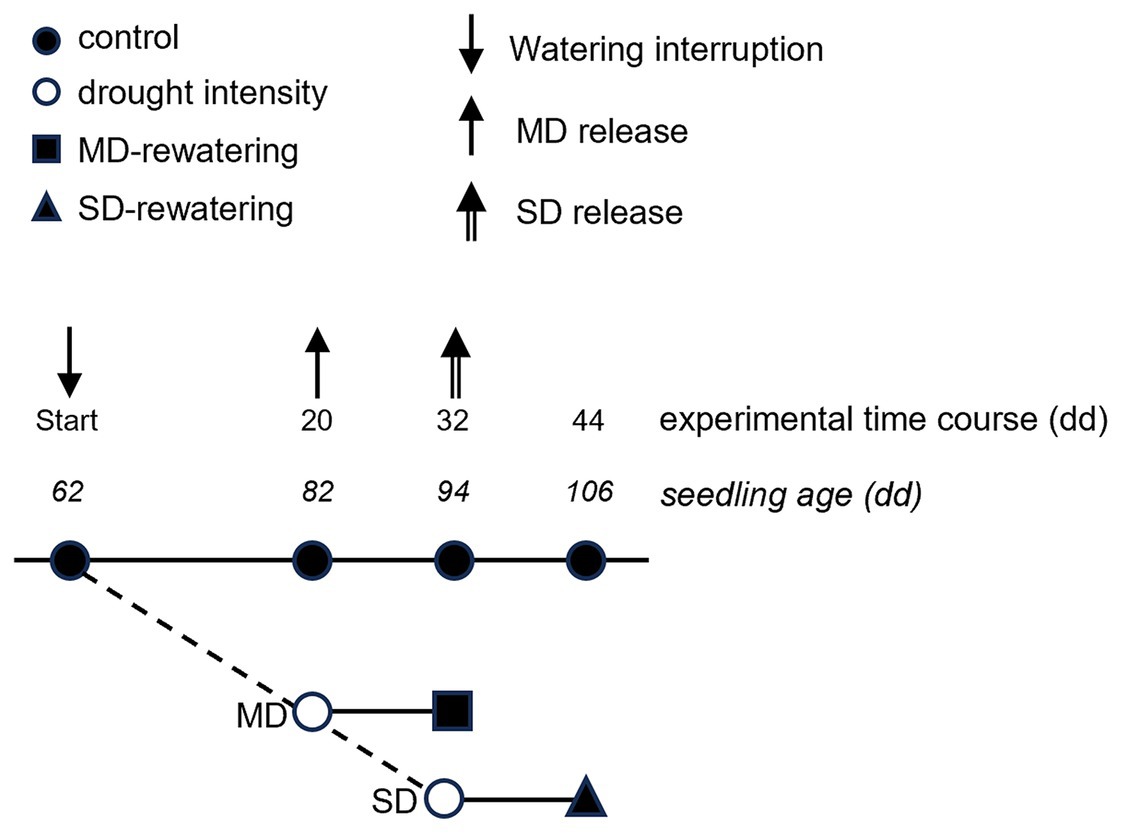
Figure 1. Sketch of schedule and sequential connection between the control, the two drought treatments and the two rewatering treatments. The numbers indicate the day of the experiment (top) and the age of the seedling (bottom). Arrows indicate the experimental days of the beginning of the rewatering period (mild and severe drought release).
Volumetric soil water content decreased over time in drought-treated plants (Figure 2). The MD treatment was fixed at ≈35% of the SWC measured at field capacity, reached after 20 days of water withheld when SWC dropped from 42.93 ± 1.19 and 45.47 ± 0.49% to 13.36 ± 0.89% and 16.84 ± 0.38% for Q. robur and Q. rubra, respectively (Figure 2). The SD treatment, fixed at ≈17% of field capacity, was reached after a further 12 days of progressive water withheld (32 days in total), when water content dropped to 5.54 ± 0.65% and 9.94 ± 0.92% for Q. robur and Q. rubra, respectively (Figure 2). Two events of stress interruption (i.e., rewatering) were applied on six seedlings per species on days 20 and 32 by restoring the regular watering regime for 12 days. Six seedlings per species per treatment were collected at each of the four sampling points corresponding to day 0 (≈ 62 days from seed sowing), day 20 (MD and control), day 32 (MD-rewatering, SD, and control), and day 44 (SD-rewatering and control).
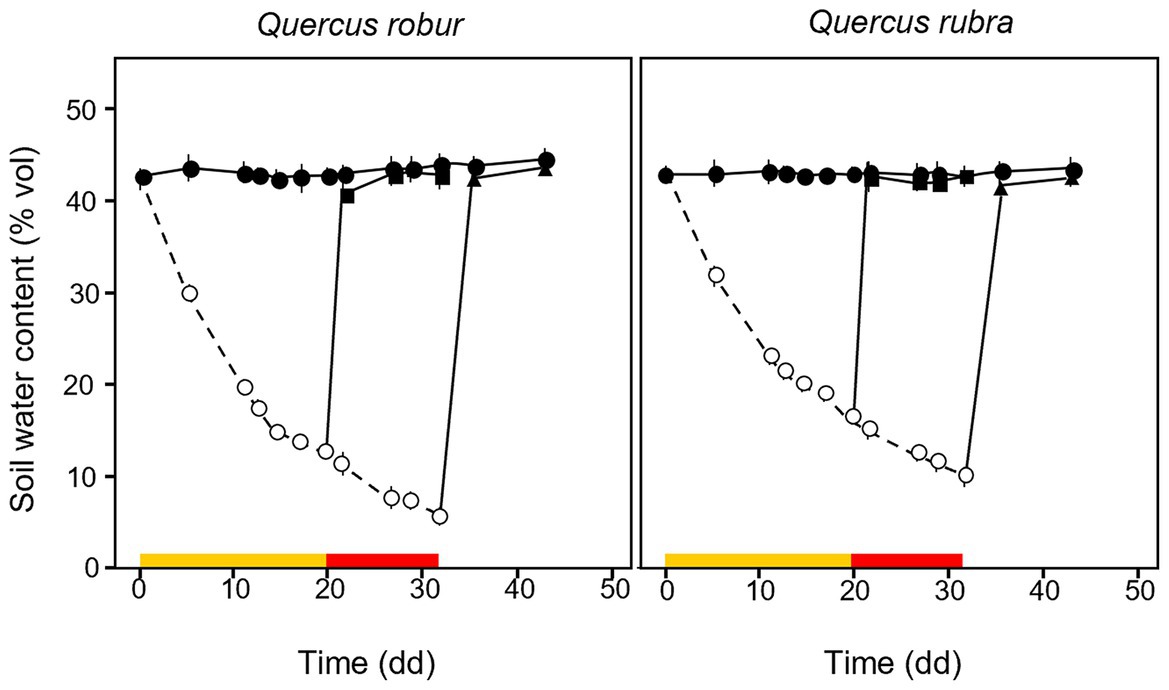
Figure 2. Temporal pattern of soil volumetric water content (SWC) over the experimental period for 4 months-old Quercus robur (left column) and Quercus rubra (right column) seedlings under two drought intensity levels with the respective rewatering events. Continuous (–) and dashed (– – –) lines indicate watered and drought conditions, respectively; filled and white circles indicate control and droughted plants, respectively; filled square and triangle indicate the release from drought 12 days from MD and SD stress, respectively. Values are the means of 6 replicates ±1SE. Colored strips indicate the duration and intensity of the drought treatments: dark-yellow for moderate-drought, red for severe-drought.
2.3 Morphological measurements
At each sampling point, seedlings were retrieved from the growth chamber. The root system was carefully washed by hand using a paintbrush after being freed from the soil to minimize the loss of fine roots. All lateral roots were separated with scissors from the main axis and scanned immersed in water at a resolution of 400 dpi with a calibrated flatbed scanner coupled to a lighting system for image acquisition (Epson Expression 10000 XL). The obtained images were analyzed by WinRhizo Pro V. 2007d (Regent Instruments Inc. Quebec). A color-coded diameter classes method was utilized to accurately measure the length, surface area, and volume of fine roots, according to the protocol described in Amolikondori et al. (2021). Specifically, three sub-classes with diameters less than 2 mm, d < 0.5 mm, 0.5 < d < 1.0, and 1.0 < d < 2.0 mm were set up, and any roots greater than 2 mm were excluded. Successively, to evaluate biomass allocation, plants were divided into shoots, leaves, taproots, and fine roots. Each component was oven-dried to constant weight at 70°C and then weighed. Finally, specific root length (SRL, m g−1), defined as the fine root length to root dry mass ratio, root tissue density (RTD, g cm−3), defined as the fine root dry mass to root volume ratio, and specific leaf area (SLA, m2 kg−1), defined as the one-sided leaf surface to dry mass ratio, were calculated.
2.4 Physiological measurements
The day before each morphology sampling time, leaf chlorophyll fluorescence, leaf stomatal conductance, and leaf water potentials were measured sequentially on all treated seedlings, according to the protocol described in Chiatante et al. (2015) for different species of oak seedlings. Chlorophyll fluorescence was measured on two fully expanded leaves per seedling per treatment with a portable pulse-modulated fluorometer (OS1-FL, Opti-Sciences, Inc. United States). Minimal fluorescence (F0) was measured on dark-adapted leaves during the dark period. The maximal fluorescence yield (Fm) was recorded after exposing the same dark-adapted leaves to a saturating pulse of white light (800 ms at about 15,000 μmol photons m−2 s−1). The resulting F0 and Fm values were used to calculate the maximal photochemical efficiency of PSII (Fv/Fm), where Fv is variable fluorescence (Fv = Fm – F0).
The Quantum yield of photosystem II (ΦPSII) was measured on the same leaves during the light period, i.e., 4 h after the lights were switched on. Steady-state fluorescence (Fs) was measured on light-adapted leaves; the maximal fluorescence yield (Fm′) after exposing the same light-adapted leaves to a saturating pulse of white light (800 ms at about 15,000 μmol photons m−2 s−1). Values of Fs and Fms were used to calculate ΦPSII according to the equation ΦPSII = (Fms − Fs)/Fms.
Leaf water potential was determined on two different fully expanded leaves per seedling per treatment, 1 h before lights were switched on (Ψpd) and approximately 4 h later when the temperature had reached its daily maximum (Ψmd), using a pressure chamber (SKPM 1400, Skye Instruments Ltd., United Kingdom).
Stomatal conductance (gs) was measured on the same two leaves used for midday water potentials and at the same temperature using a steady-state porometer (PMR 3, PPSystem, MA, United States).
2.5 Non-structural carbohydrates measurements
Soluble sugar and starch content of the only very-fine root portion (d < 0.5 mm) were determined following the method of Landhäusser et al. (2018). Dried samples were ground in a Wiley Mill to pass through a 40-mesh screen. A solution of 80% ethanol was used for extracting soluble sugars (SS), and a spectrophotometric method via phenol–sulfuric acid assay was applied for their content determination. Enzymes α-amylase and amyloglucosidase (Sigma-Aldrich, St. Louis, MO) were used for digesting the starch remaining in the pellet after extraction. The enzyme digestion-obtained glucose was determined spectrophotometrically using glucose oxidase/peroxidase-o-dianisidine solution and converted to starch equivalent. Sugar and starch concentrations were calculated as a percent of the sample dry weight. Non-structural-carbohydrates (NSC) concentrations are the sum of starch and soluble sugars.
2.6 Statistical analysis
The experiment was a completely randomized design with factorial treatments (2 species × 2 watering regimes × 2 drought duration). The main and interaction effects for the investigated traits were tested with a three-way ANOVA using the General Linear Model (SPSS Inc., Chicago, IL). This analysis considered only the control and drought (MD and SD) treatments of the second and third sampling points, as the first sampling was the same for both. Recovery of rewatered plants was tested against MD and SD treatments only. All interactions were initially included in the model, the nonsignificant ones being stepwise excluded. Before the analysis, all above-and below-ground morpho-physiological and chemical data were tested with the Kolmogorov–Smirnov test for normality and the Levene test for the homoscedasticity assumption. In particular, the stomatal conductance and shoot dry mass met these two requirements after being square-root and log transformed, respectively, whereas the predawn and midday Leaf water potential (Leaf wp), Fv/Fm, and ΦPSII did not. For these latter, the non-parametric two-groups Mann–Whitney test was carried out on the medians of the main factors. For each variable, the independent samples student t-test was performed among treatments within each sampling point and among droughted and rewatered plants for both MD and SD treatments. All differences were considered significant at p < 0.05.
Principal component analysis (PCA) was performed on all standardized data to detect the pattern of association between the root morphological and chemical (Starch and SS) variables and the shoot physiological variables (software SYN-TAX 2000, Podani, Budapest, Hungary).
3 Results
3.1 Morphological traits
In general, both shoot and root biomasses of control plants were more developed in Q. robur than in Q. rubra throughout the experiment (time and species main effect p < 0.001, Table 1 and Figures 3A–D). However, the shoot biomass was almost double the root biomass for both species (Figures 3A–D). For Q. robur, shoot and root biomasses of both MD- and SD-treated plants did not differ from control (Figures 3A,C). Differently, for Q. rubra, the shoot and root biomasses were similar to and significantly higher than those of the control for MD- and SD-treated plants, respectively (p < 0.05; Figures 3B,D) (S × D interaction p < 0.05, Table 1). For both species, rewatering events (MD-rewatering and SD-rewatering) did not significantly change the growth parameters from the previous drought condition except for root dry mass in Q. robur, which was significantly higher (Figure 3C).
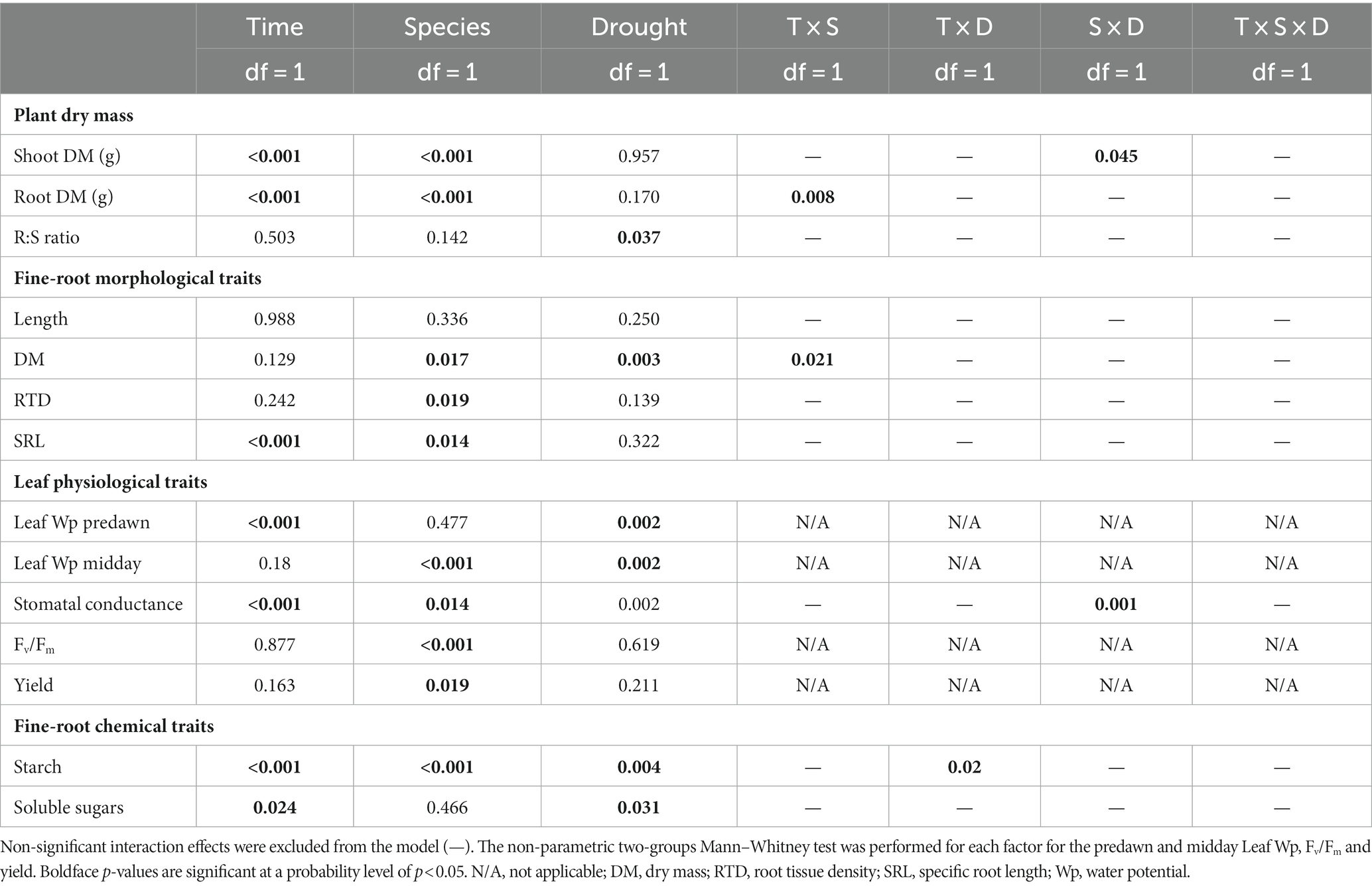
Table 1. p-values of ANOVA (general linear model) for the effects of time (at 20 and 32 days only), species and drought intensity on morphological and chemical traits for the fine and very-fine roots, respectively, and physiological traits for the leaves of Quercus robur and Quercus rubra.
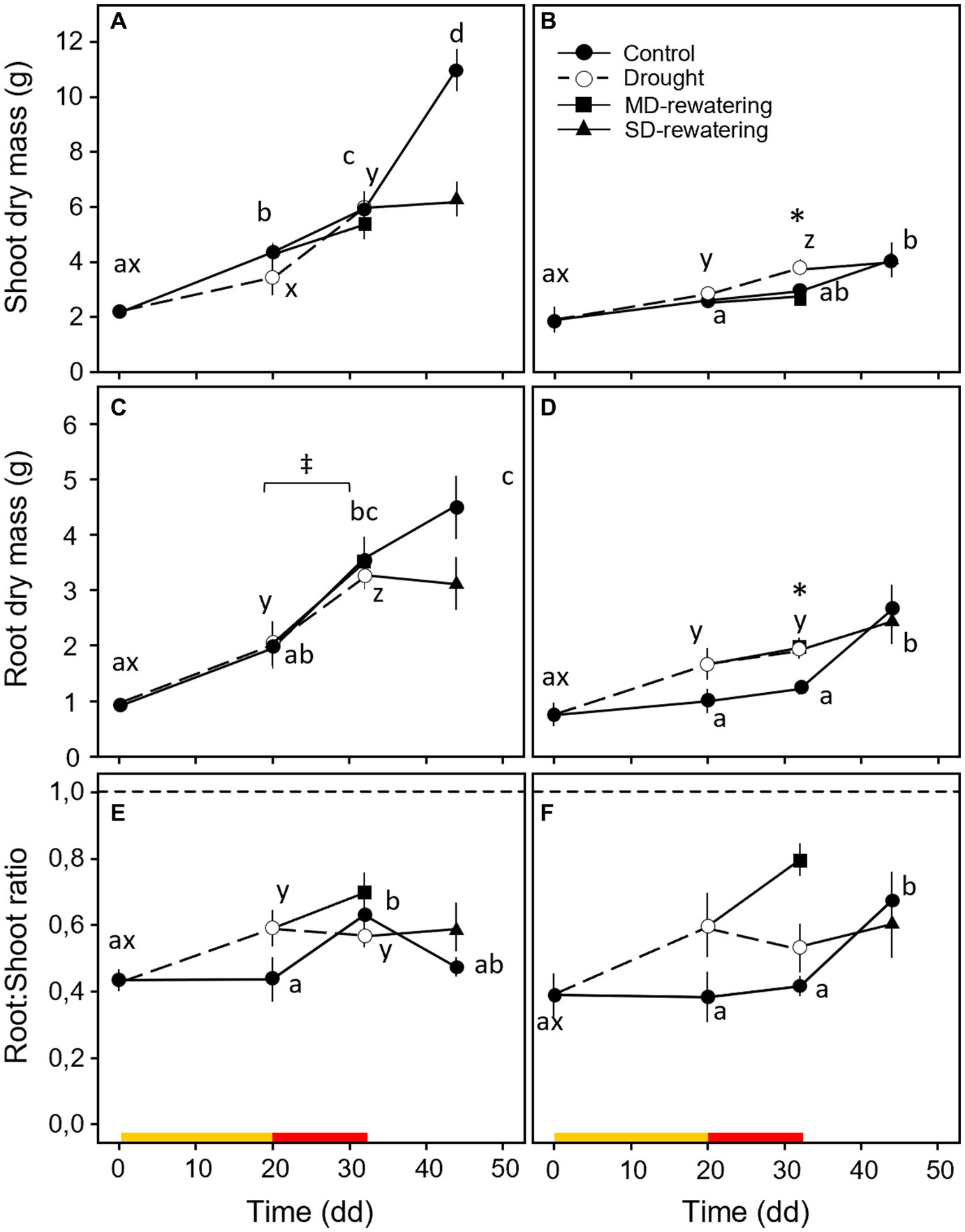
Figure 3. Temporal pattern of shoot (A,B) and root (C,D) dry mass and root-to-shoot ratio (E,F) over the experimental period for 4 months-old Quercus robur (left column) and Quercus rubra (right column) seedlings under two drought intensity levels with the respective rewatering events. Continuous (–) and dashed (– – –) lines indicate watered and drought conditions, respectively; filled and white circles indicate control and droughted plants, respectively; filled square and triangle indicate the recovery from drought 12 days from MD and SD stress, respectively. Values are the means of 6 replicates ±1SE. Colored strips indicate the duration and intensity of the drought treatments: dark-yellow for moderate-drought, red for severe-drought. If written, a, b, c and x, y, z indicate significant differences between different sampling points within control and drought treatments, respectively (LSD, p < 0.05); * indicate significant differences between control and drought within each sampling point, and ‡ between droughted and rewatered plants for both MD and SD treatments (student’s t-test, p < 0.05).
In terms of the R:S ratio, Q. robur control plants increased momentarily after 32 days, with a similar ratio to Q. rubra occurring 12 days later (Figures 3E,F). For both species, droughted plants showed greater values than the control, highlighting an increase in the root portion (drought effect p = 0.037, Table 1); this ratio decreases slightly between the second and third (32 days) sampling points. Both species responded to MD interruption by increasing the R:S ratio, although not significantly (Figures 3E,F), whereas no differences were observed when plants were rewatered after SD stress.
These two Quercus species differed significantly (p < 0.05) for most of the traits when fine roots were analyzed as one pool <2 mm in diameter, except for the length (p = 0.336; Table 1). If fine roots were analyzed at the subclass level, significant differences between the two species emerged for the length, with lower and higher values for 0.5–1 mm and 1–2 mm classes in Q. robur than Q. rubra, respectively (Figures 4A,B). All classes slightly increased their values under drought conditions.
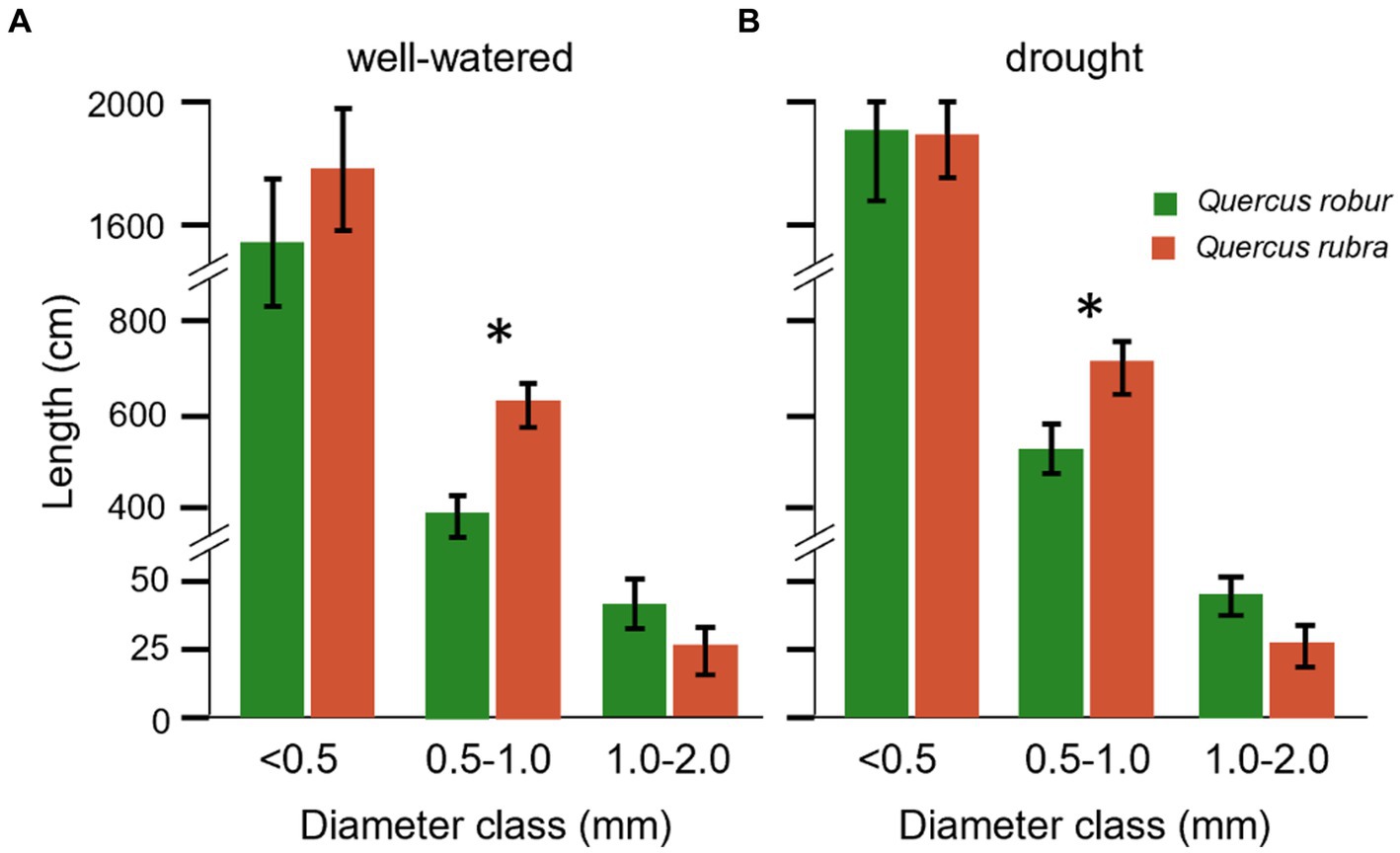
Figure 4. Root length for different diameter classes of Quercus robur (green) and Quercus rubra (dark red) under well-watered (A) and drought treatments (B). Values refer to and pool the 20–32 days temporal range; each bar represents the mean of 24 replicates ±1SE. An asterisk (*) indicates a significant difference between species within each diameter class (student’s t-test, p < 0.05).
Fine root (<2 mm) biomass (p = 0.017) and RTD (p = 0.019) were significantly higher in Q. robur than in Q. rubra (Table 1 and Figures 5A,B), the opposite for SRL (p = 0.014, Table 1 and Figure 5C). In particular, SRL showed a significant decrease over time in both species (p < 0.001, Table 1 and Figures 6A,B), more marked in Q. robur.
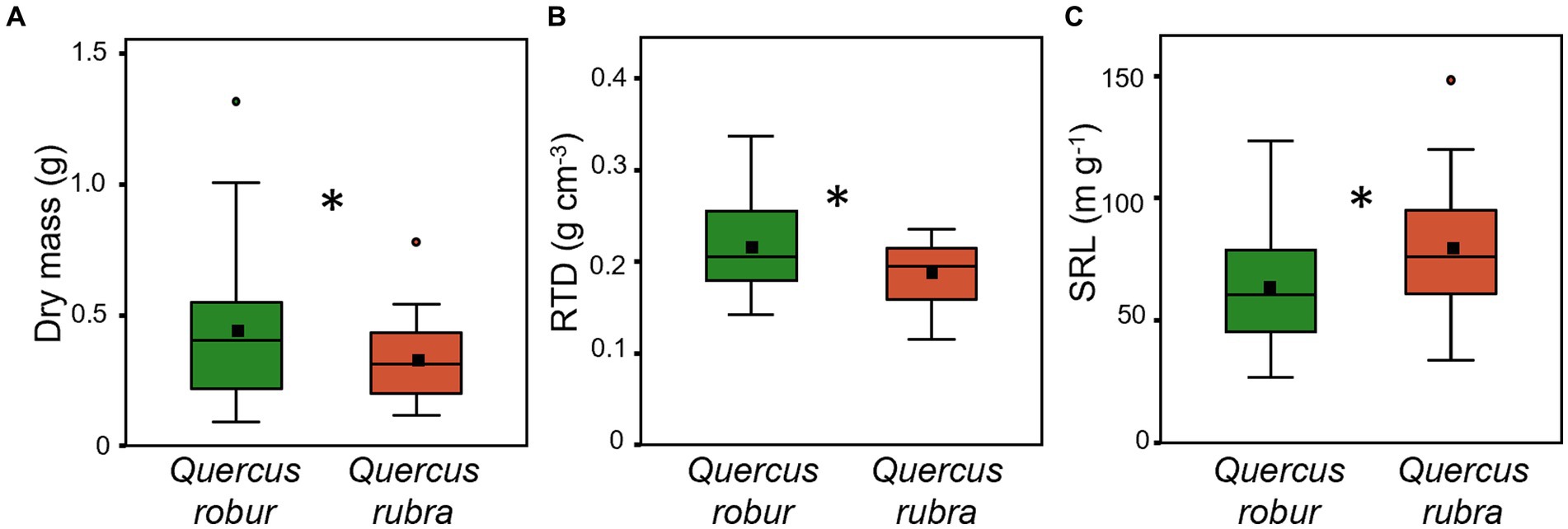
Figure 5. Fine root (<2 mm) dry mass (A), RTD (B) and SRL (C) for 4 months-old Quercus robur (green) and Quercus rubra (dark red) seedlings. Values refer to the 20–32 days temporal range and pooled well-watered and drought treatments (n = 24). An asterisk (*) indicates a significant difference between species (three-way ANOVA main factor, p < 0.05). Vertical boxes represent approximately 50% of the observations (25th to 75th percentiles), and lines extending from each box are the upper and lower 25% of the distribution. Within each box, the solid horizontal line indicates the median, while the squared filled dot represents the mean.
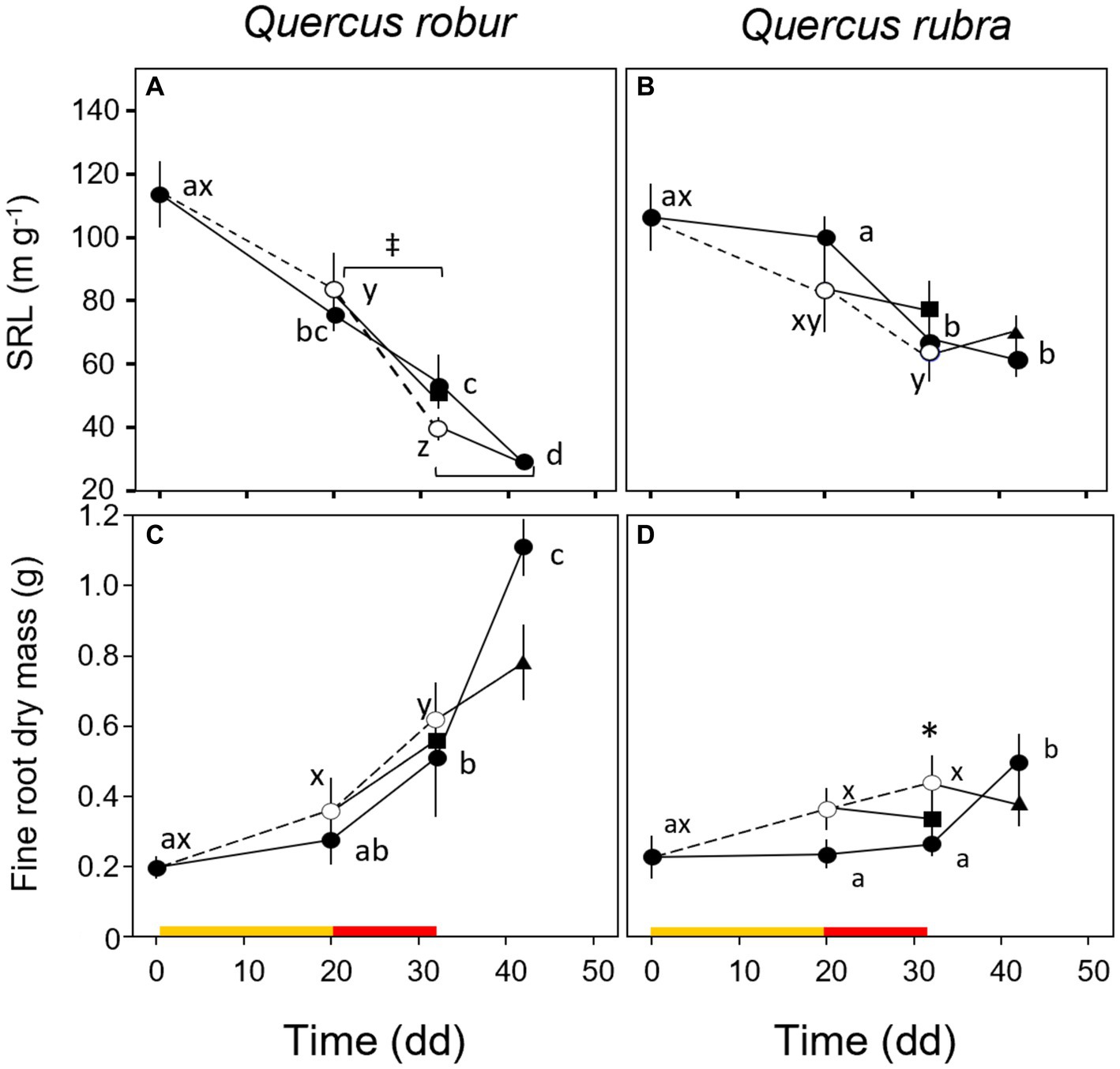
Figure 6. Temporal pattern of specific root length (A,B) and dry mass (C,D) of very-fine roots over the experimental period for 4 months-old Quercus robur (left column) and Quercus rubra (right column) seedlings under two drought intensity levels with the respective rewatering events. Continuous (–) and dashed (– – –) lines indicate watered and drought conditions, respectively; filled and white circles indicate control and droughted plants, respectively; filled square and triangle indicate the recovery from drought 12 days from MD and SD stress, respectively. Values are the means of 6 replicates ±1SE. Colored strips indicate the duration and intensity of the drought treatments: dark-yellow for moderate-drought, red for severe-drought. If written, a, b, c and x, y, z indicate significant differences between different sampling points within control and drought treatments, respectively (LSD, p < 0.05); * indicate significant differences between control and drought within each sampling point, and ‡ between droughted and rewatered plants for both MD and SD treatments (student’s t-test, p < 0.05).
A significant response to drought alone was observed only for the fine root (<2 mm) biomass trait (p = 0.003, Table 1), with MD and SD slightly higher than control in both species, in particular in Q. rubra (Figures 6C,D). For both control and drought-treated plants, the biomass significantly increased over time (from MD to SD) only in Q. robur (T × S interaction p = 0.021, Table 1 and Figures 6C,D).
For both species, rewatering events (MD-rewatering and SD-rewatering) did not significantly change the growth parameters from the previous drought condition except for SRL in Q. robur, which was significantly lower (Figure 6A).
3.2 Physiological traits
Species significantly differed for all the investigated physiological traits except for predawn LWP (Table 1). Q. rubra generally showed mean values lower than Q. robur for the stomatal conductance (Figure 7A), the maximal photochemical efficiency (Fv/Fm; Figure 7B), and the quantum Yield (Φ; Figure 7C) of PSII, the opposite for the midday LWP (Figure 7D).
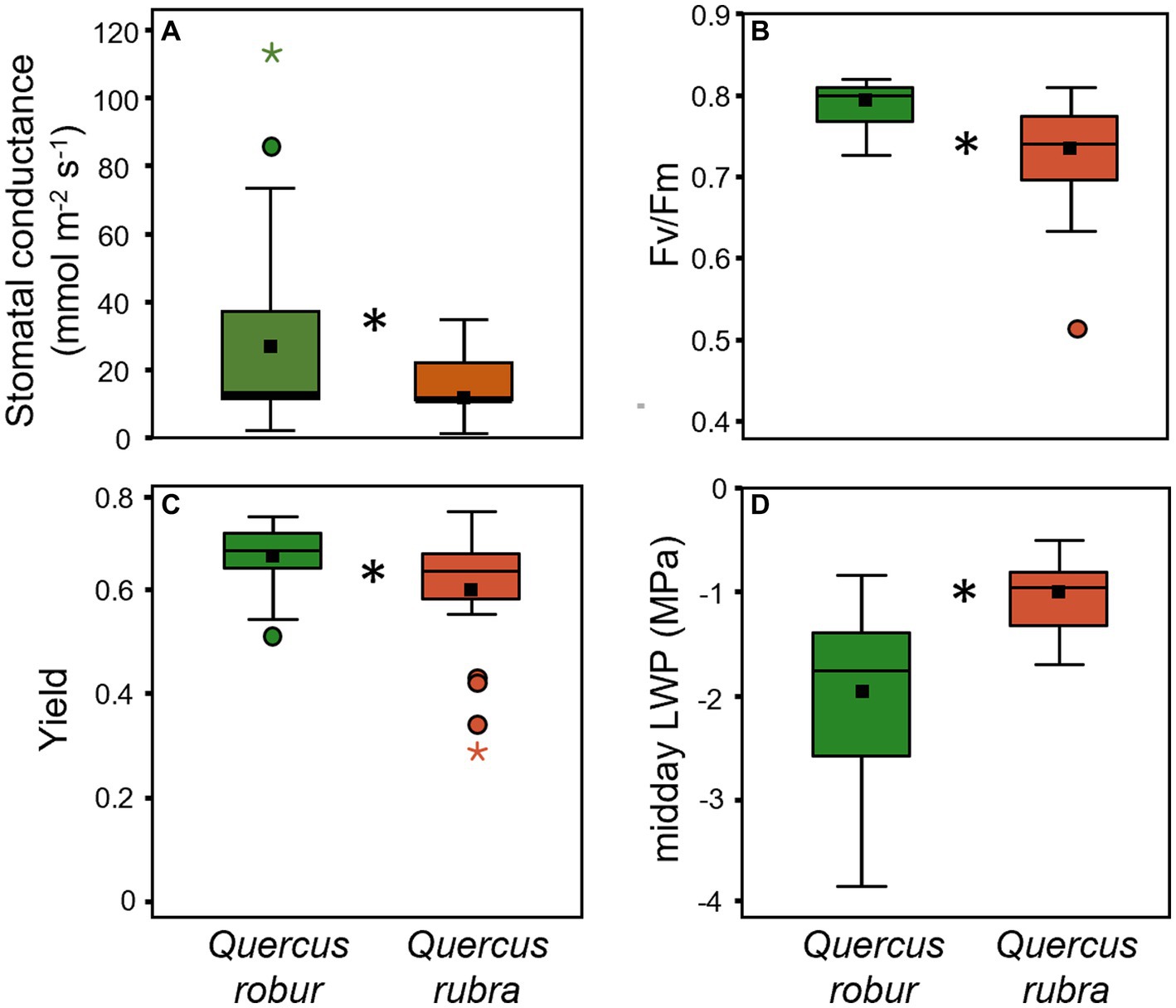
Figure 7. Stomatal conductance (A), Fv/Fm (B), yield (C), and midday LWP (D) for 4 months-old Quercus robur (green) and Quercus rubra (dark red) seedlings. Values refer to the 20–32 days temporal range and pooled well-watered and drought treatments (n = 24). An asterisk (*) indicates a significant difference between species (three-way ANOVA main factor for stomatal conductance, non-parametric Mann–Whitney test for Fv/Fm, yield, and midday LWP, p < 0.05). Vertical boxes represent approximately 50% of the observations, and lines extending from each box are the upper and lower 25% of the distribution. Within each box, the solid horizontal line indicates the median, while the squared filled dot represents the mean.
During the experiment, predawn LWP did not differ between species (Table 1) and remained higher than midday-LWP (Figures 8A–D), although drought significantly decreased both potentials in both species (Table 1). A significant drop of LWP to −2.74 ± 0.22 MPa at predawn (Figure 8A) and to −3.40 ± 0.18 MPa at midday (Figure 8C) occurred only in Q. robur after 32 days of progressively soil drying. Leaf water potential was the trait that better indicated the response to the rewatering, partially increasing after MD and significantly recovering to control values 12 days after SD (Figures 8A–D). The stomatal conductance was significantly higher for well-watered than drought-treated plants in Q. robur (Figure 8E), whereas no differences emerged in Q. rubra (S × D interaction p = 0.001, Table 1; Figure 8F).
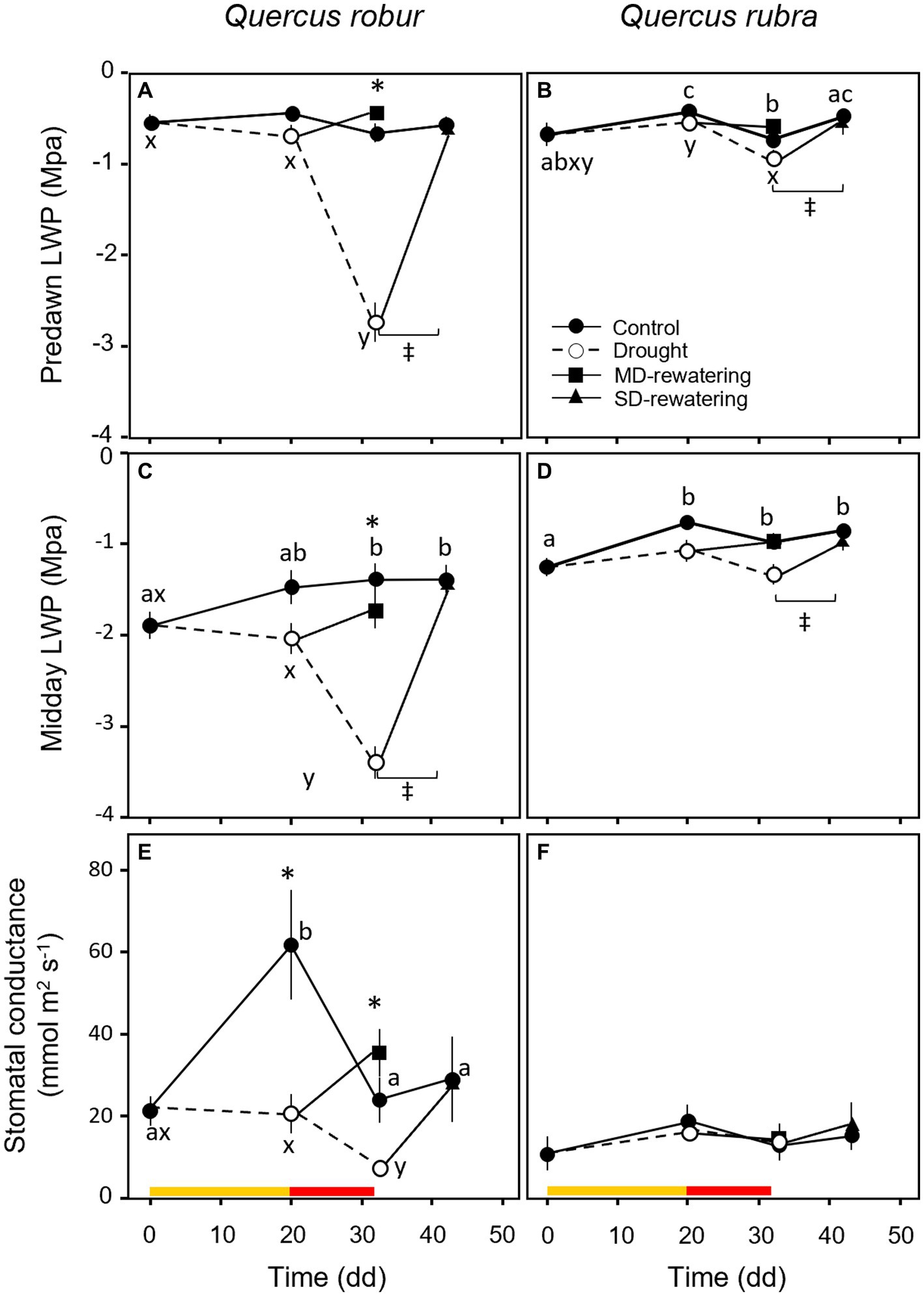
Figure 8. Temporal pattern of predawn (A,B) and midday (C,D) LWP and stomatal conductance (E,F) over the experimental period for 4 months-old Quercus robur (left column) and Quercus rubra (right column) seedlings under two drought intensity levels with the respective rewatering events. Continuous (–) and dashed (– – –) lines indicate watered and drought conditions, respectively; filled and white circles indicate control and droughted plants, respectively; filled square and triangle indicate the recovery from drought 12 days from MD and SD stress, respectively. Values are the means of 6 replicates ±1SE. Colored strips indicate the duration and intensity of the drought treatments: dark-yellow for moderate-drought, red for severe-drought. If written, a, b, c and x, y, z indicate significant differences between different sampling points within control and drought treatments, respectively (LSD, p < 0.05); * indicate significant differences between control and drought within each sampling point, and ‡ between droughted and rewatered plants for both MD and SD treatments (student’s t-test, p < 0.05). Differences were considered significant at p < 0.05.
3.3 Non-structural carbohydrates
Species significantly differed for the starch rather than the SS concentrations (Table 1), with the overall mean of starch concentration significantly higher (p < 0.001) in Q. robur than in Q. rubra (Figures 9A,D). In both species, drought significantly reduced both starch (p = 0.004) and SS (p = 0.031) concentrations (Table 1). These differences were of higher magnitude after 32 days of progressive soil drying (T × D interaction p = 0.02, Table 1; Figures 9B,C,E,F).
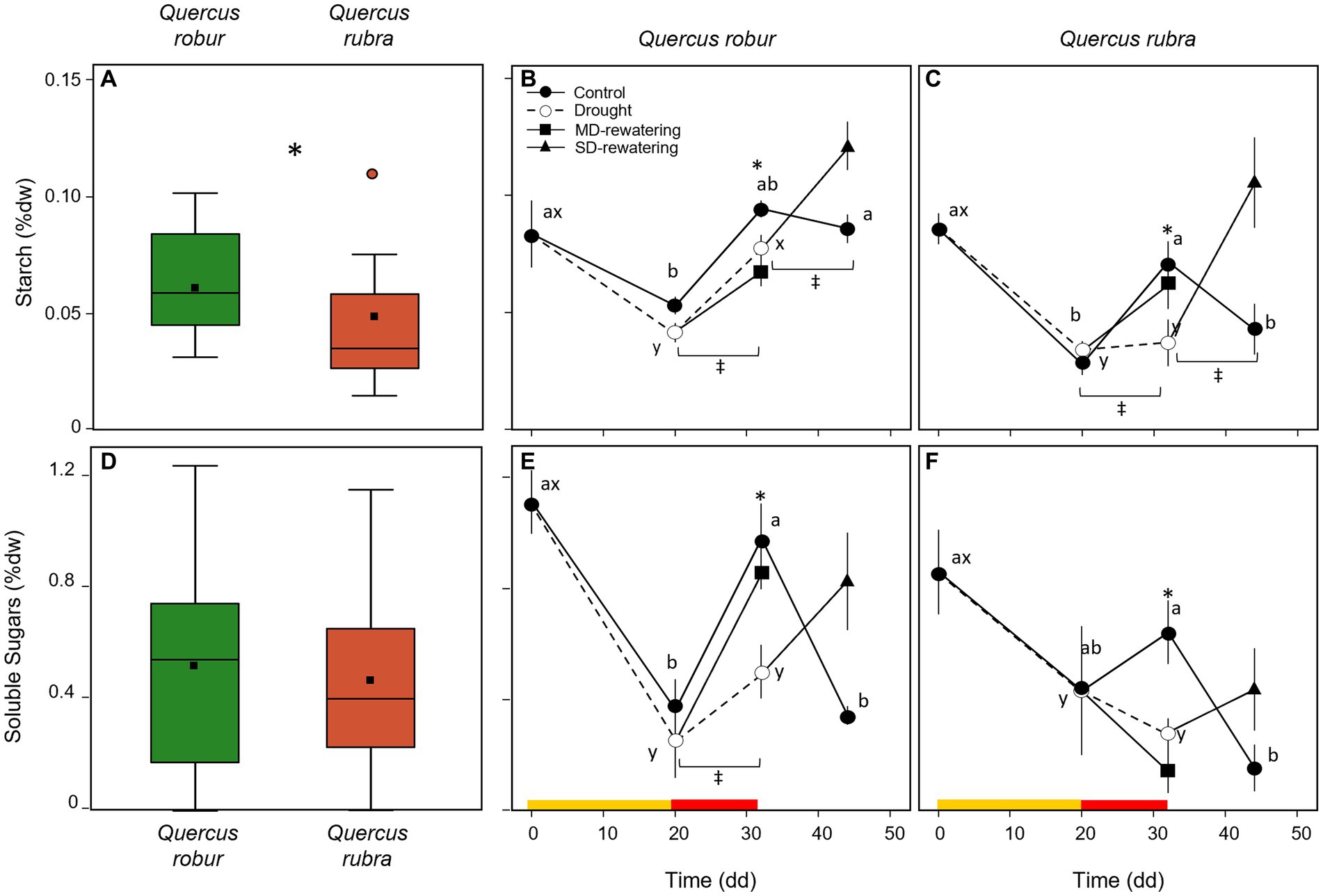
Figure 9. Temporal pattern of starch (B,C) and soluble sugars (E,F) concentrations (% dw) over the experimental period for 4 months-old Quercus robur (left column) and Quercus rubra (right column) seedlings under two drought intensity levels with the respective rewatering events. Continuous (–) and dashed (– – –) lines indicate watered and drought conditions, respectively; filled and white circles indicate control and droughted plants, respectively; filled square and triangle indicate the recovery from drought 12 days from MD and SD stress, respectively. Values are the means of 6 replicates ±1SE. Colored strips indicate the duration and intensity of the drought treatments: dark-yellow for moderate-drought, red for severe-drought. If written, a, b, c and x, y, z indicate significant differences between different sampling points within control and drought treatments, respectively (LSD, p < 0.05); * indicate significant differences between control and drought within each sampling point, and ‡ between droughted and rewatered plants for both MD and SD treatments (student’s t-test, p < 0.05). Differences were considered significant at p < 0.05. On the far-left side, box-plot distribution for the starch (A) and soluble sugars (B) concentrations; values refer to the 20–32 days temporal range and pooled well-watered and drought treatments (n = 24) (three-way ANOVA main factor, p < 0.05).
In both species, 12 days of rewatering after 20 (MD-rewatering) and 32 (SD-rewatering) days from water withholding significantly increased the starch and the SS concentrations from the previous drought condition (Figures 9B,C,E,F), except for the SS in MD-rewatering Q. robur plants (Figure 9E).
3.4 Multivariate analysis
The most striking difference among all types of investigated traits reflected by the first component of PCA (24.37% of total variance) could be attributed to the different responses to SWC changes of the two species, being all Q. rubra treatments grouped toward negative loadings in the coincidence of SWC score, the opposite for most of Q. robur treatments (Figure 10). Q. rubra is associated with high LWP and SRL values, particularly for control and SD-interrupted plants. On the other hand, Q. robur is associated with growth traits (shoot and root dry mass, RTD), photochemical efficiency, and starch concentration.
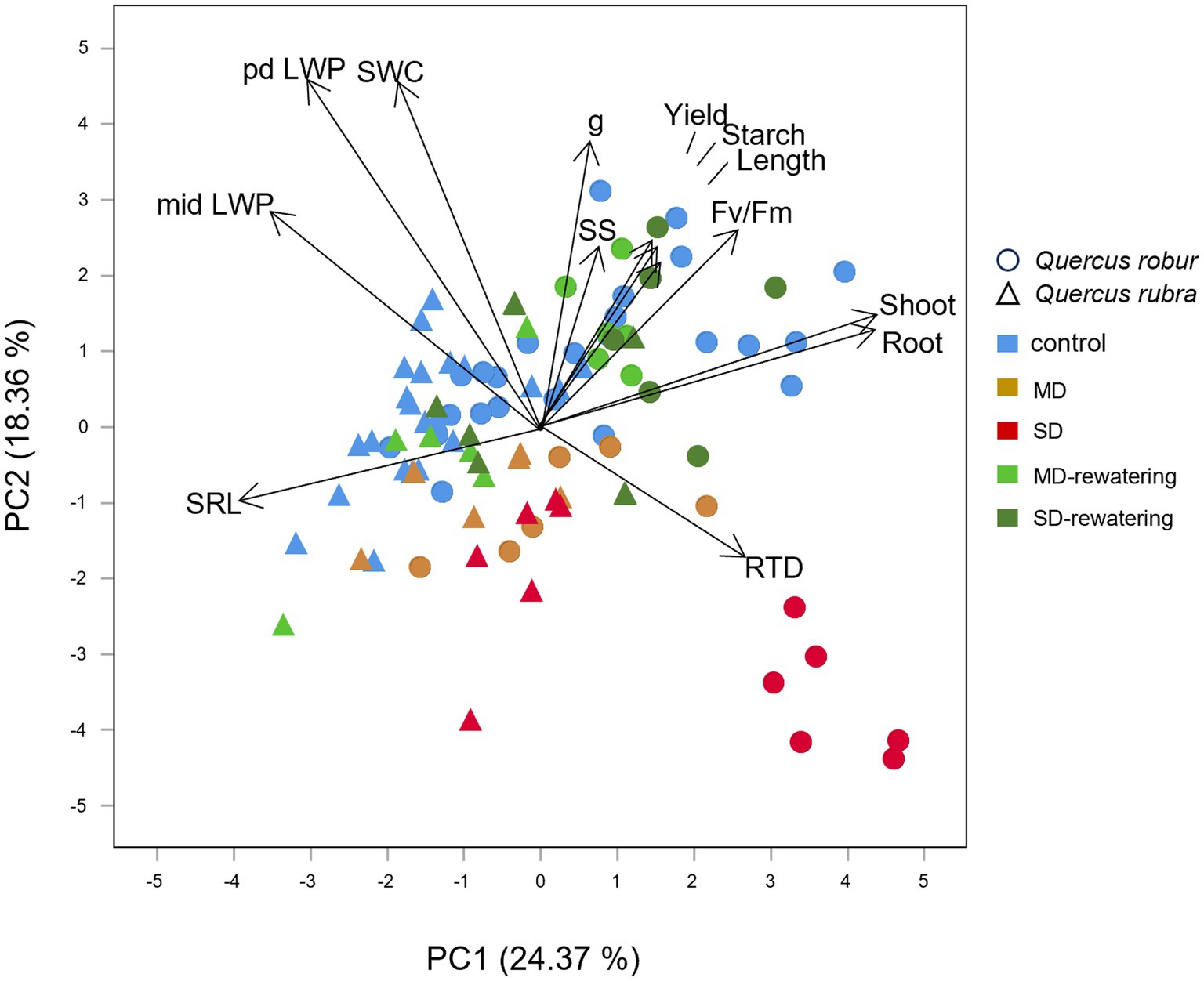
Figure 10. Principal component analysis (PCA) ordination of the first and second axes showing the relationships between physiological for the leaves, chemical and morphological traits for very-fine and fine roots, respectively, shoot and root biomass (arrows) in relation to drought stress condition in 4 months-old Quercus robur and Quercus rubra seedlings. SRL, specific fine root length; length, fine root length; RTD, root tissue density; SS, soluble sugars; pd LWP, predawn leaf water potential; mid LWP, midday leaf water potential; g, stomatal conductance; yield, quantum yield (Φ); Fv/Fm, maximal photochemical efficiency; and the shoot and root, total shoot and root biomass.
The second component explained 18.36% of the total variance. It divided most of the well-watered (controls and MD-and SD-rewatering) from the drought-treated plants, particularly for Q. robur. Moreover, PC2 highlighted the different responses to drought between the morphological structure of fine roots and the physiology of the shoot, with LWP, stomatal conductance, photochemical efficiency and starch positively correlated with SWC than RTD and SRL, which are positioned on the other side (Figure 10). Starch and SS concentrations also decreased with the increasing drought intensity (Figure 10).
4 Discussion
Outcomes from this study highlighted that Q. rubra had significantly lower biomass than Q. robur independently from the treatments rejecting the first (i) hypothesis, although both species equally invested much more biomass in the shoot than the root. Moreover, although not measured, based on the literature, the seed size’s effect on plant growth may be excluded, as there is no significant difference in average dry weight between the two species (Devetaković et al., 2019; Woziwoda et al., 2023). The alien Q. rubra responds to drought differently from the native Q. robur. In particular, the alien Q. rubra responded to drought, increasing both shoot and root biomasses, while the biomass of the native Q. robur remained unchanged when it underwent drought stress. Moreover, the alien Q. rubra seemed to respond better to severe drought since this cohort of plants had similar biomass values to those of the non-stressed ones. On the other hand, the native Q. robur suffered the severe drought, as demonstrated by the unchanged values of SD-rewatered plants after 12 days of water supply.
Consistent with this study, an R:S ratio lower than one was observed in both species at the sapling stage under different canopy openings (Kuehne et al., 2014). This behavior is the opposite of the Mediterranean oak species Quercus pubescens (Di Iorio et al., 2011), Quercus ilex and Quercus suber (Ramírez-Valiente et al., 2018), which invest much more in the belowground compartment (R:S ratio >1) at the initial growth stages, even under well-watered conditions. Plants would allocate more biomass to the roots in their early seedling stages and direct it to the shoots soon after. For example, the R:S ratio significantly decreases with stem biomass and tree height for forests, with the accumulation of standing aboveground biomass for shrubs and grasses (Mokany et al., 2006; Hui et al., 2014). Conversely, the R:S ratio significantly increases with elevation and latitude, i.e., under colder and drier conditions (Qi et al., 2019; Leuschner, 2020). The optimal partitioning hypothesis (Bloom et al., 1985; Chapin et al., 1987) suggests that under more stressful conditions, such as those characterized by low-nutrient or dry soils, greater biomass is allocated to roots to optimize resource use. Moreover, in both temperate (Thomas and Gausling, 2000) and mediterranean (Chiatante et al., 2006, Chiatante et al., 2015; Di Iorio et al., 2011) oak species, the predominant process in the short-term response to drought has been found to involve redistribution of biomass toward the fine roots to increase the soil’s exploitable volume. In this study, the short-term response of both species to dry conditions mirrors the acclimation and adaptation pattern of the oak species, with more biomass allocation to the very-fine and fine roots (Figures 6C,D). SRL resulted higher in Q. rubra than Q. robur independently from the drought treatments, supporting the first part of the second hypothesis. However, over time, the increased fine root biomass was not linked to a proportional increase in length, resulting in a general decrease of the specific root length in both species, that is a reduction in resource acquisition (Eissenstat and Yanai, 1997; Ostonen et al., 2007; Montagnoli et al., 2019). In particular, in Q. rubra, the magnitude of the SRL reduction was lower than in Q. robur, regardless of the drought intensity experienced; this difference might be related to the lower contribution of the heavier 1–2 mm diameter class (Figures 4A,B) in Q. rubra than in Q. robur. Similar behavior was mirrored at the leaf level, with a higher SLA for Q. rubra (Supplementary Figure S1).
Therefore, it is possible to speculate that the lower biomass for the 1–2 mm fine root portion might be related to the lower inputs from aboveground, which did not balance construction and maintenance costs. Indeed, in contrast with the (iii) hypothesis, photochemical efficiency (Fv/Fm), the quantum yield (ΦPSII) of PSII, and stomatal conductance were consistently lower in Q. rubra during the experimental period, probably impacting photosynthesis by reducing the maximum activity of PSII and limiting the CO2 availability at the chloroplast level (Cornic, 1994). Moreover, the low root starch concentration, although limited only to the very-fine class, found in Q. rubra after 32 days of progressive soil drying does not support the second part of the (ii) hypothesis and strongly correlates with the low photochemical efficiency (Figure 10), probably being due to the reduction of photosynthate inputs from aboveground. This hypothesis is further supported by the significantly low SS concentration in droughted plants of both species, like the beech seedlings under similar drought stress conditions (Domingo et al., 2023).
The higher leaf water potential and lower stomatal conductance of Q. rubra, even under drier conditions, suggests an isohydric behavior for this species, at least in the seedling stage and in the adopted experimental conditions. The slightly higher soil water content measured in Q. rubra pots at the end of the drying period further supports the reduced stomatal conductance observed. Q. rubra (Yi et al., 2017) and Q. petraea (Aranda et al., 2000; Klein, 2014; Martínez-Sancho et al., 2017) are usually considered anisohydric species, i.e., able to keep their stomata open during drought, allowing their water potential to decrease while still maintaining C assimilation rates (Martínez-Vilalta et al., 2014), but with a higher risk of hydraulic failure. However, the isohydric-anisohydric spectrum should not be considered a fixed binary category, as both species have certain degrees of isohydricity (Martínez-Vilalta et al., 2014; Yi et al., 2017), and drought response strategies may change with ontogeny (Cavender-Bares and Bazzaz, 2000). In fact, red oak seedlings coping with drought conditions have been observed to reduce the stomata opening with a consecutive lowering in carbon uptake. Differently, at the mature tree level, red oak addresses drought conditions by reaching deeper soil water layers thanks to a deeper root system development, sustaining a higher stomatal conductance (Cavender-Bares and Bazzaz, 2000). The drawback of the isohydric behavior observed here in Q. rubra was the overall reduced growth. However, isohydricity may not always be considered a carbon limiting factor, for example, for the evergreen Q. ilex, whose growth performance was similar to the anisohydric Phyllirea latifolia under extreme drought (Garcia-Forner et al., 2016).
Both drought releases from MD and SD conditions showed a marked recovery of the photochemical efficiency (Fv/Fm) and the quantum yield (ΦPSII) of PSII to pre-drought values, indicating a resilient photosynthetic apparatus in these species. Instead, this trend was not mirrored by the fine roots, whose growth did not recover to control values, except for a slight increase for Q. robur. Several studies reported a remarkable resistance of PSII photochemistry to dehydration, high-temperature and photoinhibition, whether alone or in combination, in the similar sessile oak (Epron et al., 1992) or cedar (Ladjal et al., 2000) and the sclerophyll Heteromeles arbutifolia (Valladares and Pearcy, 1997).
The adopted PPFD was relatively lower than that of direct summer sunlight as the one experienced in a large forest gap (18% of assumed ≈ 2000 μmol m−2 s−1); this light condition could further explain the reduced growth observed for Q. rubra, supporting its profile as intermediate shade tolerant and gap specialist.
In conclusion, outcomes from the present study revealed isohydric behavior rather than specific functional traits as the character that best explains the competitive performance of Q. rubra at the seedling stage. The higher resistance to drought under the low light intensity adopted in this study makes this species highly competitive under the direr conditions in the canopy openings during the summer (Amolikondori et al., 2021). However, the response to the interactive effects of combined stressors may differ from that elicited when the stresses are imposed singly (Mittler, 2006; Suseela et al., 2015), so further experiments combining different light and drought intensities are necessary to elucidate better the growth performance of this alien species in the European habitat.
Data availability statement
The raw data supporting the conclusions of this article will be made available by the authors, without undue reservation.
Author contributions
ADI: Conceptualization, Data curation, Formal analysis, Methodology, Project administration, Supervision, Validation, Visualization, Writing – original draft, Writing – review & editing. ACC: Data curation, Formal analysis, Investigation, Visualization. PB: Formal analysis, Investigation, Supervision. AM: Conceptualization, Funding acquisition, Methodology, Project administration, Supervision, Validation, Visualization, Writing – review & editing, Formal analysis.
Funding
The author(s) declare financial support was received for the research, authorship, and/or publication of this article. This work was supported by the University of Insubria (FAR) and the EC FP7 Project ZEPHYR-308313.
Acknowledgments
The authors are deeply grateful to Giada Falsetti for her help in the non-structural carbohydrate analyzes.
Conflict of interest
The authors declare that the research was conducted in the absence of any commercial or financial relationships that could be construed as a potential conflict of interest.
The author(s) declared that they were an editorial board member of Frontiers, at the time of submission. This had no impact on the peer review process and the final decision.
Publisher’s note
All claims expressed in this article are solely those of the authors and do not necessarily represent those of their affiliated organizations, or those of the publisher, the editors and the reviewers. Any product that may be evaluated in this article, or claim that may be made by its manufacturer, is not guaranteed or endorsed by the publisher.
Supplementary material
The Supplementary material for this article can be found online at: https://www.frontiersin.org/articles/10.3389/ffgc.2024.1307340/full#supplementary-material
References
Abrams, M. D. (1996). Distribution, historical development and ecophysiological attributes of oak species in the eastern United States. Ann. For. Sci. 53, 487–512. doi: 10.1051/FOREST:19960230
Amolikondori, A., Vajari, K. A., Feizian, M., Montagnoli, A., and Di Iorio, A. (2021). Gap size in Hyrcanian Forest affects the lignin and N concentrations of the oriental beech (Fagus orientalis Lipsky) fine roots but does not change their morphological traits in the medium term. Forests 12:137. doi: 10.3390/f12020137
Aranda, I., Gil, L., and Pardos, J. A. (2000). Water relations and gas exchange in Fagus sylvatica L. and Quercus petraea (Mattuschka) Liebl. in a mixed stand at their southern limit of distribution in Europe. Trees 146, 344–352. doi: 10.1007/S004680050229
Bloom, A. J., Chapin, F. S., and Mooney, H. A. (1985). Resource limitation in plants-an economic analogy. Annu. Rev. Ecol. Syst. 16, 363–392. doi: 10.1146/annurev.es.16.110185.002051
Brunner, I., Herzog, C., Dawes, M. A., Arend, M., and Sperisen, C. (2015). How tree roots respond to drought. Front. Plant Sci. 6:547. doi: 10.3389/fpls.2015.00547
Cavender-Bares, J., and Bazzaz, F. A. (2000). Changes in drought response strategies with ontogeny in Quercus rubra: implications for scaling from seedlings to mature trees. Oecologia 124, 8–18. doi: 10.1007/PL00008865
Chapin, F. S., Bloom, A. J., Field, C. B., and Waring, R. H. (1987). Plant responses to multiple environmental factors physiological ecology provides tools for studying how interacting environmental resources control plant growth. Bioscience 37, 49–57. doi: 10.2307/1310177
Chiatante, D., Di Iorio, A., Sciandra, S., Scippa, G. S., and Mazzoleni, S. (2006). Effect of drought and fire on root development in Quercus pubescens Willd. and Fraxinus ornus L. seedlings. Environ. Exp. Bot. 56, 190–197. doi: 10.1016/j.envexpbot.2005.01.014
Chiatante, D., Tognetti, R., Scippa, G. S., Congiu, T., Baesso, B., Terzaghi, M., et al. (2015). Interspecific variation in functional traits of oak seedlings (Quercus ilex, Quercus trojana, Quercus virgiliana) grown under artificial drought and fire conditions. J. Plant Res. 128, 595–611. doi: 10.1007/s10265-015-0729-4
Chmura, D. (2020). The spread and role of the invasive alien tree Quercus rubra (L.) in novel forest ecosystems in central Europe. Forests 11:586. doi: 10.3390/F11050586
Chmura, D., and Sierka, E. (2005). The occurrence of invasive alien plant species in selected forest nature reserves in southern Poland as a conservation problem. Nat. Conserv. 62, 3–11.
Cornic, G. (1994). “Drought stress and high effects on leaf photosynthesis” in Photoinhibition of photosynthesis, from molecular mechanisms to the field. eds. N. R. Baker and J. R. Bowyer (Oxford: BIOS Scientific Publishers), 297–313.
Crow, T. R. (1992). Population dynamics and growth patterns for a cohort of northern red oak (Quercus rubra) seedlings. Oecologia 91, 192–200. doi: 10.1007/BF00317783
Devetaković, J., Nonić, M., Prokić, B., Popović, V., and Šijačić-Nikolić, M. (2019). Acorn size influence on the quality of pedunculate oak (Quercus robur L.) one-year old seedlings. REFORESTA 8, 17–24. doi: 10.21750/REFOR.8.02.72
Di Iorio, A., Giacomuzzi, V., and Chiatante, D. (2016). Acclimation of fine root respiration to soil warming involves starch deposition in very fine and fine roots: a case study in Fagus sylvatica saplings. Physiol. Plant. 156, 294–310. doi: 10.1111/ppl.12363
Di Iorio, A., Montagnoli, A., Scippa, G. S., and Chiatante, D. (2011). Fine root growth of Quercus pubescens seedlings after drought stress and fire disturbance. Environ. Exp. Bot. 74, 272–279. doi: 10.1016/j.envexpbot.2011.06.009
Dimitrova, A., Csilléry, K., Klisz, M., Lévesque, M., Heinrichs, S., Cailleret, M., et al. (2022). Risks, benefits, and knowledge gaps of non-native tree species in Europe. Front. Ecol. Evol. 10:908464. doi: 10.3389/fevo.2022.908464
Domingo, G., Vannini, C., Marsoni, M., Costantini, E., Bracale, M., and Di Iorio, A. (2023). A multifaceted approach to reveal the very-fine root’s response of Fagus sylvatica seedlings to different drought intensities. Physiol. Plant. 175, e13934–e13915. doi: 10.1111/ppl.13934
Eissenstat, D. M., and Yanai, R. D. (1997). The ecology of root lifespan. Adv. Ecol. Res. 27, 1–60. doi: 10.1016/S0065-2504(08)60005-7
Epron, D., Dreyer, E., and Bréda, N. (1992). Photosynthesis of oak trees [Quercus petraea (Matt.) Liebl.] during drought under field conditions: diurnal course of net CO2 assimilation and photochemical efficiency of photosystem II. Plant Cell Environ. 15, 809–820. doi: 10.1111/J.1365-3040.1992.TB02148.X
Garcia-Forner, N., Biel, C., Savé, R., and Martínez-Vilalta, J. (2016). Isohydric species are not necessarily more carbon limited than anisohydric species during drought. Tree Physiol. 37, 441–455. doi: 10.1093/treephys/tpw109
Gieger, T., and Thomas, F. M. (2002). Effects of defoliation and drought stress on biomass partitioning and water relations of Quercus robur and Quercus petraea. Basic Appl. Ecol. 3, 171–181. doi: 10.1078/1439-1791-00091
Hartmann, H., Ziegler, W., and Trumbore, S. (2013). Lethal drought leads to reduction in nonstructural carbohydrates in Norway spruce tree roots but not in the canopy. Funct. Ecol. 27, 413–427. doi: 10.1111/1365-2435.12046
Hui, D., Wang, J., Shen, W., Le, X., Ganter, P., and Ren, H. (2014). Near isometric biomass partitioning in forest ecosystems of China. PLoS One 9:e86550. doi: 10.1371/journal.pone.0086550
Jacobs, D. F., Francis Salifu, K., and Davis, A. S. (2009). Drought susceptibility and recovery of transplanted Quercus rubra seedlings in relation to root system morphology. Ann. For. Sci. 66:504. doi: 10.1051/forest/2009029
Kiedrzyński, M., Zielińska, K., and Grzelak, P. (2011). Transformation of Forest vegetation after 40 years of protection in the Tomczyce nature reserve (central Poland). Acta Univ. Lodz., Folia Biol. Oecol. 7, 207–227. doi: 10.2478/V10107-009-0026-X
Klein, T. (2014). The variability of stomatal sensitivity to leaf water potential across tree species indicates a continuum between isohydric and anisohydric behaviors. Funct. Ecol. 28, 1313–1320. doi: 10.1111/1365-2435.12289
Kormann, J. M., Liesebach, M., and Liepe, K. J. (2023). Provenances from introduced stands of northern red oak (Quercus rubra L.) outperform those from the natural distribution. For. Ecol. Manag. 531:120803. doi: 10.1016/j.foreco.2023.120803
Kosola, K. R., Dickmann, D. I., and Parry, D. (2002). Carbohydrates in individual poplar fine roots: effects of root age and defoliation. Tree Physiol. 22, 741–746. doi: 10.1093/treephys/22.10.741
Kuehne, C., Nosko, P., Horwath, T., Bauhus, J., and Abrams, M. (2014). A comparative study of physiological and morphological seedling traits associated with shade tolerance in introduced red oak (Quercus rubra) and native hardwood tree species in southwestern Germany. Tree Physiol. 34, 184–193. doi: 10.1093/TREEPHYS/TPT124
Ladjal, M., Epron, D., and Ducrey, M. (2000). Effects of drought preconditioning on thermotolerance of photosystem II and susceptibility of photosynthesis to heat stress in cedar seedlings. Tree Physiol. 20, 1235–1241. doi: 10.1093/TREEPHYS/20.18.1235
Landhäusser, S. M., Chow, P. S., Turin Dickman, L., Furze, M. E., Kuhlman, I., Schmid, S., et al. (2018). Standardized protocols and procedures can precisely and accurately quantify non-structural carbohydrates. Tree Physiol. 38, 1764–1778. doi: 10.1093/treephys/tpy118
Leuschner, C. (2020). Drought response of European beech (Fagus sylvatica L.)—a review. Perspect. Plant Ecol. Evol. Syst. 47:125576. doi: 10.1016/j.ppees.2020.125576
Louki, I. I., and Al-Omran, A. M. (2022). Calibration of soil moisture sensors (ECH2O-5TE) in hot and saline soils with new empirical equation. Agronomy 13:51. doi: 10.3390/agronomy13010051
Major, K. C., Nosko, P., Kuehne, C., Campbell, D., and Bauhus, J. (2013). Regeneration dynamics of non-native northern red oak (Quercus rubra L.) populations as influenced by environmental factors: a case study in managed hardwood forests of southwestern Germany. For. Ecol. Manag. 291, 144–153. doi: 10.1016/J.FORECO.2012.12.006
Martínez-Sancho, E., Dorado-Liñán, I., Hacke, U. G., Seidel, H., and Menzel, A. (2017). Contrasting hydraulic architectures of scots pine and sessile oak at their southernmost distribution limits. Front. Plant Sci. 8:598. doi: 10.3389/fpls.2017.00598
Martínez-Vilalta, J., Poyatos, R., Aguadé, D., Retana, J., and Mencuccini, M. (2014). A new look at water transport regulation in plants. New Phytol. 204, 105–115. doi: 10.1111/nph.12912
Mittler, R. (2006). Abiotic stress, the field environment and stress combination. Trends Plant Sci. 11, 15–19. doi: 10.1016/j.tplants.2005.11.002
Mokany, K., Raison, R. J., and Prokushkin, A. S. (2006). Critical analysis of root: shoot ratios in terrestrial biomes. Glob. Chang. Biol. 12, 84–96. doi: 10.1111/j.1365-2486.2005.001043.x
Montagnoli, A., Dumroese, R. K., Terzaghi, M., Onelli, E., Scippa, G. S., and Chiatante, D. (2019). Seasonality of fine root dynamics and activity of root and shoot vascular cambium in a Quercus ilex L. forest (Italy). For. Ecol. Manag. 431, 26–34. doi: 10.1016/j.foreco.2018.06.044
Montagnoli, A., Terzaghi, M., Di Iorio, A., Scippa, G. S., and Chiatante, D. (2012). Fine-root morphological and growth traits in a Turkey-oak stand in relation to seasonal changes in soil moisture in the southern Apennines, Italy. Ecol. Res. 27, 1015–1025. doi: 10.1007/s11284-012-0981-1
Nguyen, P. V., Dickmann, D. I., Pregitzer, K. S., and Hendrick, R. (1990). Late-season changes in allocation of starch and sugar to shoots, coarse roots, and fine roots in two hybrid poplar clones. Tree Physiol. 7, 95–105. doi: 10.1093/treephys/7.1-2-3-4.95
Nicolescu, V.-N., Vor, T., Mason, W. L., Bastien, J.-C., Brus, R., Henin, J.-M., et al. (2020). Ecology and management of northern red oak (Quercus rubra L. syn. Q. borealis F. Michx.) in Europe: a review. Forestry 93, 481–494. doi: 10.1093/forestry/cpy032
Ostonen, I., Püttsepp, Ü., Biel, C., Alberton, O., Bakker, M. R., Lõhmus, K., et al. (2007). Specific root length as an indicator of environmental change. Plant Biosyst. 141, 426–442. doi: 10.1080/11263500701626069
Qi, Y., Wei, W., Chen, C., and Chen, L. (2019). Plant root-shoot biomass allocation over diverse biomes: a global synthesis. Glob. Ecol. Conserv. 18:e00606. doi: 10.1016/j.gecco.2019.e00606
Ramírez-Valiente, J.-A., Aranda, I., Sanchéz-Gómez, D., Rodríguez-Calcerrada, J., Valladares, F., and Robson, T. M. (2018). Increased root investment can explain the higher survival of seedlings of ‘Mesic’ Quercus suber than “xeric” Quercus ilex in sandy soils during a summer drought. Tree Physiol. 39, 64–75. doi: 10.1093/treephys/tpy084
Reinhardt, F., Herle, V. M., Bastiansen, B. F., and Streit, B. (2003). Economic impact of the spread of alien species in Germany. Federal Environmental Agency (Umweltbundesamt) Berlin.
Sander, I. (1990). “Quercus rubra L.” in Silvics of North America: volume 2. Hardwoods (Agricultural Handbook). eds. R. M. Burns and B. H. Honkala (U.S. Department of Agriculture, Forest Service), 727–738. Available at: http://www.na.fs.fed.us/spfo/pubs/silvics_manual/table_of_contents.htm
Steiner, K. C., Abrams, M. D., and Bowersox, T. W. (1993). “Advance reproduction and other stand characteristics in Pennsylvania and French stands of northern red oak” in Proceedings of the 9th Central Hardwood Forest Conference; Gen. Tech. Rep. NC-161. eds. A. R. Gillespie, G. R. Parker, P. E. Pope, and G. Rink (St. Paul, MN: U.S. Department of Agriculture, Forest Service, North Central Forest Experiment Station), 473–483. Available at: https://www.fs.usda.gov/treesearch/pubs/15342
Suseela, V., Tharayil, N., Xing, B., and Dukes, J. S. (2015). Warming and drought differentially influence the production and resorption of elemental and metabolic nitrogen pools in Quercus rubra. Glob. Chang. Biol. 21, 4177–4195. doi: 10.1111/gcb.13033
Thomas, F. M., and Gausling, T. (2000). Morphological and physiological responses of oak seedlings (Quercus petraea and Q. robur) to moderate drought. Ann. For. Sci. 57, 325–333. doi: 10.1051/forest:2000123
Valladares, F., and Pearcy, R. W. (1997). Interactions between water stress, sun-shade acclimation, heat tolerance and photoinhibition in the sclerophyll Heteromeles arbutifolia. Plant Cell Environ. 20, 25–36. doi: 10.1046/j.1365-3040.1997.d01-8.x
Vansteenkiste, D., De Boever, L., and Van Acker, J. (2005). Alternative processing solutions for Q. rubra (Quercus rubra) from converted forests in Flanders, Belgium. Proceedings of the COST Action E44 Conference on Broad Spectrum Utilization of Wood Vienna, Austria).
Walters, M. B., Kunkle, J. M., Kobe, R. K., and Farinosi, E. J. (2023). Seedling drought responses governed by root traits, site-soil moisture regimes and overstory competition-facilitation. For. Ecol. Manag. 544:121159. doi: 10.1016/j.foreco.2023.121159
Woziwoda, B., Gręda, A., and Frelich, L. E. (2023). High acorn diversity of the introduced Quercus rubra indicates its ability to spread efficiently in the new range. Ecol. Indic. 146:109884. doi: 10.1016/j.ecolind.2023.109884
Yi, K., Dragoni, D., Phillips, R. P., Roman, D. T., and Novick, K. A. (2017). Dynamics of stem water uptake among isohydric and anisohydric species experiencing a severe drought. Tree Physiol. 37, 1379–1392. doi: 10.1093/treephys/tpw126
Zadworny, M., Comas, L. H., and Eissenstat, D. M. (2018). Linking fine root morphology, hydraulic functioning and shade tolerance of trees. Ann. Bot. 122, 239–250. doi: 10.1093/aob/mcy054
Keywords: fine roots, drought, alien species, non-structural carbohydrates, isohydric, anisohydric
Citation: Di Iorio A, Caspani AC, Beatrice P and Montagnoli A (2024) Drought-related root morphological traits and non-structural carbohydrates in the seedlings of the alien Quercus rubra and the native Quercus robur: possible implication for invasiveness. Front. For. Glob. Change. 7:1307340. doi: 10.3389/ffgc.2024.1307340
Edited by:
Ivika Ostonen, University of Tartu, EstoniaReviewed by:
Jesús Rodríguez-Calcerrada, Polytechnic University of Madrid, SpainJoanna Mucha, Polish Academy of Sciences, Poland
Copyright © 2024 Di Iorio, Caspani, Beatrice and Montagnoli. This is an open-access article distributed under the terms of the Creative Commons Attribution License (CC BY). The use, distribution or reproduction in other forums is permitted, provided the original author(s) and the copyright owner(s) are credited and that the original publication in this journal is cited, in accordance with accepted academic practice. No use, distribution or reproduction is permitted which does not comply with these terms.
*Correspondence: Antonino Di Iorio, YW50b25pbm8uZGlpb3Jpb0B1bmluc3VicmlhLml0