- 1College of Agriculture and Veterinary Medicine, Jimma University, Jimma, Ethiopia
- 2College of Agriculture and Forestry, Mettu University, Mettu, Ethiopia
Woody plant species in homegarden agroforestry store a large proportion of carbon stocks. However, there is limited information on the carbon stock potential of homegarden agroforestry along altitudinal gradients in southwest Ethiopia. Therefore, this study aims to estimate aboveground and belowground carbon stocks in homegardens using a non-destructive allometric model. Data were collected from 72 homegardens selected using a random sampling method. Woody plants were measured for diameter at breast height (DBH) of ≥ 5 cm and height of ≥ 1.5 m. The study revealed that the mean aboveground carbon stock (14.39 ± 2.95 Mg C ha−1) was significantly (p < 0.05) higher in the middle altitude than (6.12 ± 0.72 Mg C ha−1) in the low altitude. Carbon stocks were significantly different between middle and low altitudes. The overall mean carbon stock was 11.25 ± 1.60, with mean aboveground and belowground carbon stocks of 9.39 ± 1.34 and 1.88 ± 0.27 Mg C ha−1, respectively. The top 10 woody species contributed to 78.50% of the total carbon stock, of which 56.73% were Persea americana and Cordia africana. Wealth status and size of homegardens were significantly correlated (r = 0.298 and r = 0.307, respectively) with the carbon stock. The overall woody carbon stock distributions varied primarily due to altitudinal gradients, woody species, and socioeconomic factors. As a result, this study will assist researchers and policymakers in selecting optimal ecological areas and addressing socioeconomic gaps for agroforestry practices that produce biomass and store carbon for long-term climate change mitigation.
1 Background
The practice of agroforestry is acknowledged for its role in climate change mitigation and adaptation because of its positive social and environmental impacts (Mbow et al., 2014; Meragiaw, 2017; Amadu et al., 2020; Jahan et al., 2022). It helps overcome environmental constraints in response to climate change shocks by absorbing atmospheric greenhouse gases and generating a hospitable environment (Verchot et al., 2007; Schoeneberger et al., 2012). In Ethiopia, agroforestry systems provide sustainable ecosystem services, such as climate change mitigation, carbon sequestration, and soil fertility improvement, in addition to livelihood provisions (Negash et al., 2005; Negash, 2013; Meragiaw, 2017). The year-round woody components of the agroforestry systems most frequently contribute to high aboveground biomass production, which is positively correlated with the sequestration and accumulation of biomass carbon (Henry et al., 2009; Alves et al., 2010; Mayele et al., 2020; Yasin et al., 2021). The dense and diverse woody biomass production in agroforestry systems further encourages carbon sequestration, carbon storage potential, and the substitution of fossil fuel products in the climate change mitigation process (Jose and Bardhan, 2012; Liu and Li, 2012).
Trees in agroforestry play a crucial role in mitigating atmospheric greenhouse gas through photosynthesis and accumulation of carbon stocks in aboveground and belowground biomass (Unwin and Kriedemann, 2000; Terakunpisut et al., 2007; Komal et al., 2022). Trees are a dominant carbon pool landscape resource, as 50% of live tree biomass is built from carbon (Tadesse, 2015; Shiferaw et al., 2022). The study in Sri Lanka revealed that trees in homegardens significantly contribute to carbon sequestration and carbon storage processes, while reducing atmospheric greenhouse gas (CO₂) accumulation (Lowe et al., 2022). These findings highlight the potential of agroforestry practices in the global carbon cycle and climate change mitigation based on their extensive biomass production and carbon storage (Nair et al., 2021).
However, woody plant biomass and carbon pool capacity are determined by factors such as tree size, diversity, management, habitat suitability, and altitudinal gradient (Borah et al., 2013; Hagos et al., 2021; Siarudin et al., 2021). Sharma et al. (2023) revealed that biomass production and carbon storage potential vary across different agroforestry systems based on altitudinal gradients. According to Kumar (2023), carbon stocks are negatively correlated with altitudinal gradients and the size of holdings in tropical homegardens of central Kerala, India. Tree diversity, genotype, plantation site, and stand management also influence agroforestry tree biomass and carbon content (Unwin and Kriedemann, 2000; Komal et al., 2022). Previous studies have shown that management systems that are directly or indirectly associated with basal area, abundance, richness, and density also affect the biomass and carbon stock ability of trees (Atsbha et al., 2019; Pokhrel and Sherpa, 2020; Meragiaw et al., 2021; Soazafy et al., 2021). Verma et al. (2024) reported different biomass and carbon distributions for different tree components and agroforestry systems due to varying tree management options, site quality, growth rates, and tree age.
In Ethiopia, studies have investigated the diversification of woody species and their importance for biomass and carbon accumulation in agroforestry practices (Kassa et al., 2022; Tesfay et al., 2022). Gebrewahid and Meressa (2020) revealed tree diversity and carbon stocks of plant communities in parkland agroforestry in Northern Ethiopia. Manaye et al. (2021) repeated a similar assessment to compare different agroforestry systems in Northern Ethiopia. Gebrewahid et al. (2018) and Birhane et al. (2020) reported woody diversity and carbon stocks in scattered trees in farmland and homegarden agroforestry following altitudinal changes in northern and southern Ethiopia, respectively. Other studies have evaluated the same variables in agroforestry and adjacent forests in northern and western Ethiopia (Eyasu et al., 2020; Enkossa et al., 2023).
However, none of these studies have considered biomass carbon stocks from an altitudinal gradient point of view. Therefore, this study aimed to identify the aboveground and belowground carbon stock potential in homegarden agroforestry along altitudinal gradients in southwest Ethiopia. In this study, we hypothesized that aboveground and belowground carbon stocks would vary among altitudinal gradients and woody species in homegarden agroforestry. Furthermore, we hypothesized that aboveground and belowground carbon stocks would positively correlate with household socioeconomic status in southwest Ethiopia.
2 Materials and methods
2.1 Description of the study area
The study was conducted along altitudinal gradients in three districts: Mana and Gomma districts of the Jimma zone and Dhidhessa district of the Buno Bedele zone, Oromia Regional State, Ethiopia. The study areas are located at 7°40ˈN–8°20ˈN and 36°20ˈE–36°40ˈE, with altitudinal ranges between 1,459 and 2,542 m above sea level (Figure 1). The region received a mean annual rainfall of 1,853 mm, with minimum and maximum temperatures averaging 11.5°C and 24.2°C, respectively, over the past 32 years (WMO, 2022). The midland had the highest area coverage among the three agro-ecological zones (lowland, midland, and highland). The dominant land use systems include cultivated lands, wetlands, settlements, commercial farms, shrublands, grasslands, and forestland, where natural forests serve as shade for coffee cultivation and as a source of various forest products (Tolessa et al., 2019). The farming system in the area is characterized by mixed forest coffee cultivation, cereal production, and livestock rearing. Homegarden agroforestry is one of the dominant farming systems, practiced by more than 85% of local farmers. Homegarden agroforestry in the study area was composed of different indigenous and exotic woody species, including Cordia africana, Persea americana, Catha edulis, Coffea arabica, Mangifera indica, Psidium guajava, Albizia gummifera, Carica papaya, and Grevillea robusta.
2.2 Sampling design
A stratified sampling technique was used to collect woody plant data following altitudinal gradients in southwest Ethiopia. The altitude of the study area was classified as low (1459–1750 m), middle (1750–2050 m), and high (2050–2542 m), considering the elevation range and homegarden agroforestry distributions. Elevation data were obtained from the digital elevation model available in Google Earth and accessed for free via EarthExplorer.1 In addition to altitudinal gradients, household wealth status was stratified into three classes to examine the correlation between socioeconomic variables and woody biomass carbon. Wealth status was classified as rich, medium, and poor with the assistance of developmental agents and local elders. Local criteria, including the number of livestock, size of land, annual crop production, and housing standards, were used for this classification. Then, 72 homegarden agroforestry sites (24 from the mentioned categories) were randomly selected for a complete enumeration of woody plants following Motuma et al. (2008).
2.3 Data collection methods
Data were collected from all live woody plants with a DBH of ≥5 cm and a height of ≥1.5 m, except for coffee, which was measured at 40 cm above the ground. Woody plants below 5 cm in diameter and 1.5 m in height were excluded from data collection because most biomass equations are not valid for smaller stem diameters and heights (MacDicken, 1997). Woody plant diameter and height were measured using a caliper and a Suunto Clinometer, respectively. The elevation of each sampled homegarden was measured using a Global Positioning System (GPS Garmin 72). Woody species were identified in the field using voucher specimens. For species not identified in the field, plant samples were collected, coded, pressed, dried, and identified using the Flora of Ethiopia and Eritrea (Edwards et al., 1995; Hedberg and Edwards, 1995; Hedberg et al., 2006; Azene et al., 1993).
2.4 Data analysis
The present study used previously published non-destructive allometric models, as farm owners did not permit the destruction of multipurpose woody species in their homegardens. Therefore, biomass and carbon stocks for aboveground and belowground woody plants were estimated using a non-destructive allometric model (Kuyah et al., 2012). Estimation of biomass and carbon stocks in dead wood and litter was not included due to their negligible quantities and their potential for soil surface disturbance in homegarden agroforestry. Woody biomass, except for coffee, was calculated using the allometric equation developed by Kuyah et al. (2012). Coffee biomass was calculated using the allometric model developed by Negash (2013).
Therefore:
Aboveground woody biomass was calculated following the method of Kuyah et al. (2012) as follows:
where AGB is the aboveground biomass of live trees in kg and dbh is the stem diameter at breast height in cm.
The aboveground biomass of coffee plants was calculated according to Negash (2013) as follows:
where AGBcoffee is the aboveground biomass of coffee and d40 is the stem diameter of coffee at 40 cm.
Aboveground carbon content was calculated as a conversion factor of 0.48 multiplied by AGB, following Kuyah et al. (2012).
Aboveground coffee shrub carbon was calculated using the 43% carbon content of the aboveground biomass, following Negash (2013).
Carbon sequestration from woody species was calculated to estimate the contribution of woody plants to climate change mitigation in homegardens. Aboveground biomass carbon dioxide emissions were estimated using a conversion factor of 3.67, based on the molecular weight ratio of carbon dioxide to carbon ratio, in accordance with the IPCC guidelines (Intergovernmental Panel on Climate Change (IPCC), 2006). Belowground carbon dioxide emissions were calculated following trends similar to those of aboveground carbon dioxide emissions.
Belowground biomass was calculated as a conversion factor of 20% of the AGB following the methods of MacDicken (1997) and Nair (2012). The belowground carbon content of woody species was calculated to be similar to the aboveground biomass, following the method of Kuyah et al. (2012). Total carbon stock was calculated by summing the carbon content of aboveground and belowground carbon (Pearson et al., 2007).
Prior to analysis, the data were checked for normality and analyzed using the Statistical Package for Social Science (SPSS) version 26. Differences between woody carbon stocks and altitudinal gradients were analyzed using a one-way analysis of variance (ANOVA). The least significant difference (LSD) test was used to compare the level of significance (p < 0.05) between the variables.
3 Results
3.1 Aboveground and belowground woody carbon
A one-way ANOVA showed that the aboveground and belowground carbon stocks of woody species in homegarden agroforestry varied significantly (p < 0.05) among altitudinal gradients. The highest carbon stocks of 14.39 ± 2.95 Mg C ha−1 and 2.80 ± 0.60 Mg C ha−1 were recorded at the middle altitude in aboveground and belowground stock, respectively. The highest mean carbon stock (16.79 Mg C ha−1) was stored in the middle altitude, followed by 9.62 Mg C ha−1 in the high altitude and 8.78 Mg C ha−1 in the low altitude. These findings indicate that mean carbon stocks of 63.07 ± 13.01, 33.98 ± 10.54, and 26.95 ± 3.19 Mg C ha−1 were sequestered by woody plants at middle, high, and low altitudes, respectively (Table 1). There was a significant difference between the mean carbon stocks at the middle and low altitudes (Table 1). The aboveground and belowground carbon stocks of trees were significantly different (p < 0.05) among altitudinal gradients, while coffee did not show such variation. The belowground carbon stock of trees decreased from the middle altitude (2.77 Mg C ha−1) to high (1.59 Mg C ha−1) and low (1.19 Mg C ha−1) altitudes. Hence, trees dominated the total landscape carbon stock distribution in homegarden agroforestry; there was a corresponding change in carbon stock between altitude for trees and total carbon (Supplementary Table 1).

Table 1. Mean (±SE) aboveground and belowground carbon stocks (Mg C ha−1) in woody plants along altitudinal gradients.
3.2 Total woody carbon stocks
The overall live woody carbon stock potential of woody plants in the homegarden agroforestry is presented in Table 2. The mean carbon stock was 9.39 ± 1.34, 1.88 ± 0.27, and 11.25 ± 1.60 Mg C ha−1 in aboveground, belowground, and total carbon stock, respectively. The overall carbon stock within the entire homegarden agroforestry was 810.11 Mg C ha−1 (Table 2). Furthermore, the carbon stock and carbon sequestration were calculated for the top 10 woody species and showed a total of 635.95 and 2,333.98 Mg ha−1 to carbon stock and carbon sequestration, respectively, per sampled homegarden agroforestry. Among these species, Persea americana and Cordia africana contributed to 360.79 Mg ha−1 carbon stock (Table 3). Persea americana and Cordia africana alone contributed to 44.54% of the total carbon stock of woody species in homegarden agroforestry in the present study area. The result indicates that agroforestry practices had a significant position in climate change mitigation strategies adopted through farm woody plant diversification for effective biomass production and carbon accumulation.

Table 2. Summary of total carbon stock of homegarden agroforestry woody species in southwest Ethiopia.
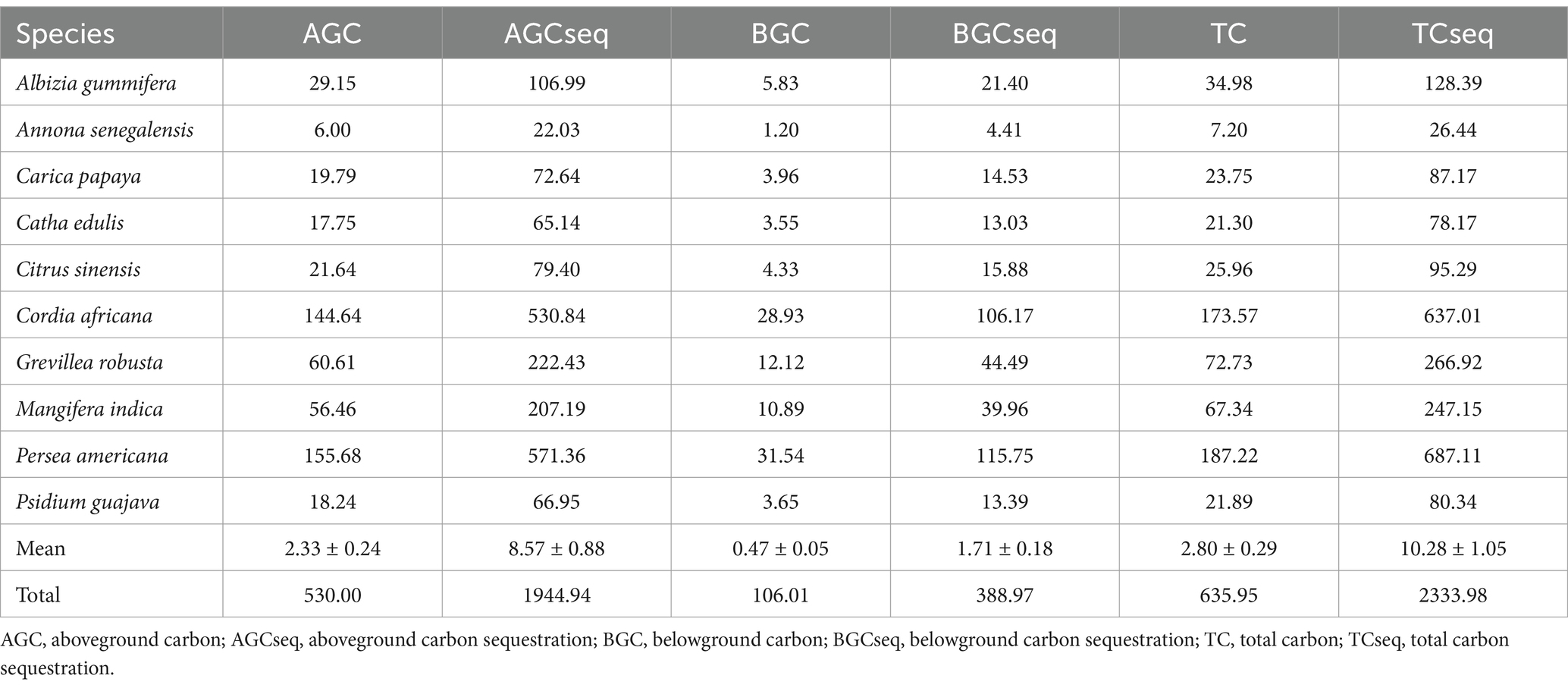
Table 3. Biomass carbon stock and carbon sequestration potential of the top 10 woody species in homegarden agroforestry in southwest Ethiopia.
3.3 Relationship between socioeconomic variables and woody carbon stocks
The Pearson correlation coefficient indicated a positive relationship between the total carbon stocks and socioeconomic factors (wealth status, size, and age of homegardens). The wealth status and size of homegarden holdings by households were significantly correlated with the total carbon stock. The size of the homegardens correlated with the total carbon stock (r = 0.307, p = 0.009). Wealth status correlated with total carbon stocks (r = 0.298, p = 0.011). However, there was no significant relationship (r = 0.187, p = 0.116) between the age of homegarden agroforestry and total carbon stock (Table 4).

Table 4. Relationship between wealth status, size of homegarden, and age of homegarden with total carbon stock in homegarden agroforestry.
4 Discussion
4.1 Woody carbon stocks
Homegarden agroforestry enhances the environmental sustainability of agricultural landscapes by increasing carbon stocks and reducing carbon dioxide (Sharma et al., 2022). It is an effective land-use system for sequestering carbon, as it is composed of structurally complex and biologically diverse woody species (Maryo et al., 2023). The mean aboveground carbon stock recorded (9.39 Mg C ha−1) is lower than the mean aboveground carbon stock (16.74 Mg C ha−1) reported in coffee-based agroforestry in southern Ethiopia (Tesfay et al., 2022). This result is also lower than the mean aboveground biomass carbon of 36.5 Mg C/ha reported in Matale district, Sri Lanka (Lowe et al., 2022), and 23.80 Mg C/ha reported in the Jaffna peninsula, Sri Lanka (Lowe et al., 2024). The stock of belowground biomass carbon (1.91 Mg C ha−1) was lower than that reported by Gebrewahid and Meressa (2020), who reported a mean belowground biomass carbon stock of 3.03 Mg C ha−1 in northern Ethiopia.
Biomass carbon stocks varied significantly at different altitudes in the present study area. Total biomass carbon stocks (aboveground and belowground) decreased from middle to high altitudes and then to low altitudes. In contrast, previous studies have revealed a linear relationship between total biomass carbon stocks and altitudinal gradients. For instance, Chemeda et al. (2022) reported that decreased biomass carbon stocks with increasing altitudinal gradients in coffee-based agroforestry in Western Ethiopia. Birhane et al. (2020) reported a significant increase in total biomass carbon with increasing altitude in homegarden agroforestry in southern Ethiopia. Asanok et al. (2024) reported that total carbon stock increased with increasing elevation gradients in northern Thailand.
The change in woody carbon stocks along altitudinal gradients is attributed to the decreased number of woody species with large diameter, but not to the increased number of trees per hectare with elevation change (Tsedeke et al., 2021). According to Gebre et al. (2018), species composition and management systems can also cause variations in land use. The mean total carbon of 11.25 Mg C ha−1 recorded was comparable with 10.93 Mg C ha−1 reported by Gebrewahid et al. (2018) and higher than 7.90 Mg C ha−1 reported by Gebrewahid and Meressa (2020). However, it is lower than the carbon stock (13.82 Mg C ha−1) reported by Gebremeskel et al. (2021) for Rhamnus prinoides-based agroforestry practices in northern Ethiopia and far lower than the 36.35 Mg C ha−1 reported for homestead forests in Bangladesh (Baul et al., 2021).
Tree carbon stock changed along altitudinal gradients, similar to the total carbon stock observed. In contrast, coffee shrub values decreased with increasing altitudinal gradients (Supplementary Table 1). The species-specific contributions of woody carbon stocks and carbon sequestration were calculated for 10 frequently observed species and showed differences among species. The result showed that carbon stock contributions varied between 7.20 and 187.22 Mg C ha−1 for Annona senegalensis and Persea americana, respectively. Similarly, carbon stock variation among tree species (16.20 Mg C ha−1 to 57.35 Mg C ha−1) was reported for Tecomella undulata and Hardwickia binata, respectively, in the agroforestry systems of the arid zones of India (Verma et al., 2024). On the other hand, Persea americana and Cordia africana contributed to 44.54% of the carbon stock, indicating their great contribution to climate change mitigation among the observed woody species. The result is in line with the study by Lowe et al. (2022), who reported the contribution of coconut and jackfruit to climate change mitigation by providing 55% of carbon accumulation in Sri Lanka.
Previous studies have reported that woody carbon stocks are influenced by species abundance (Manaye et al., 2021). Meragiaw et al. (2021) reported the highest carbon content in areas with a high abundance of large-diameter and tall tree species. Similarly, Persea americana, a dominant large-diameter tree, provided the highest carbon stock compared to the less abundant species and trees with small diameters in this study area. Aboveground woody biomass in homegardens plays a vital role in carbon sequestration, thereby helping mitigate climate change conditions (Siyum and Tassew, 2019; Kassa et al., 2022). Similarly, a higher proportion of carbon dioxide was sequestered by aboveground biomass (1944.94 Mg ha−1) than belowground biomass (388.97 Mg ha−1) in climate change mitigation efforts.
4.2 Relationship between socioeconomic variables and woody carbon stocks
Analyzing the effects of socioeconomic factors on woody carbon stocks in homegarden agroforestry is essential for the development of environmental sustainability and community welfare (Maryo et al., 2023). The present study revealed a positive and significant relationship between socioeconomic variables, including wealth status, size of homegardens, and age of homegardens, and total carbon stock. Previous studies have reported the effect of socioeconomic variables on biomass carbon stocks from different land uses (Subba et al., 2017; Contosta et al., 2020). Carbon stored in agroforestry can vary widely, depending on the type, age, and size of agroforestry, the species of trees used, and the management practices used (Nair, 2012; Feliciano et al., 2018). For example, Kumar (2023) reported a weak negative relationship between the size of homegarden and carbon stocks in India. Similarly, Deneke and Negash (2023) reported the effect of wealth status on carbon storage in homegardens, where a significant decrease in carbon stock was observed with decreasing household wealth status in Ethiopia. Kassa et al. (2022) reported differences in biomass carbon between the ages of homegarden agroforests. A review of the literature by Sharma et al. (2022) showed that carbon sequestration by homegarden agroforestry is significantly influenced by age distribution.
5 Conclusion
The aboveground and belowground carbon stock potential of woody species in homegarden agroforestry was assessed along altitudinal gradients. The results indicate that altitudinal change had a significant impact on carbon stocks in homegarden agroforestry. Carbon stocks did not show a parallel change with altitudinal gradients. Woody carbon density was highest at middle, low, and high altitudes, respectively. Stocks also varied for each woody species in homegarden agroforestry. Persea americana, Cordia africana, Grevillea robusta, and Mangifera indica were the most prominent tree species in the carbon repositories. Socioeconomic variables, including wealth status, size of homegarden holdings, and age of homegardens, are positively and significantly correlated with biomass and carbon stocks. Therefore, altitudinal gradients and household socioeconomic variations had a substantial effect on the development of agroforestry woody carbon stocks for climate change mitigation. However, studies including other ecological factors such as temperature, precipitation, and slope should provide all-inclusive evidence on carbon accumulation and potential climate change mitigation strategies adopted in agroforestry practices of southwest Ethiopia. Furthermore, studies considering litter, dead woody biomass, and soil carbon could be useful in estimating land-use woody carbon stocks.
Data availability statement
The original contributions presented in the study are included in the article/Supplementary material, further inquiries can be directed to the corresponding author.
Author contributions
TJ: Writing – original draft, Investigation, Conceptualization, Formal analysis, Methodology. KH: Conceptualization, Supervision, Writing – review & editing. ZK: Conceptualization, Writing – review & editing, Supervision. AB: Conceptualization, Supervision, Writing – review & editing.
Funding
The author(s) declare that no financial support was received for the research and/or publication of this article.
Acknowledgments
The authors would like to acknowledge the study area farmers, who willingly gave us permission to undertake vegetation surveys in their homegarden agroforestry. We would also like to thank IDEA WILD, a nonprofit donating agency, for their material support.
Conflict of interest
The authors declare that the research was conducted in the absence of any commercial or financial relationships that could be construed as a potential conflict of interest.
Generative AI statement
The authors declare that no Gen AI was used in the creation of this manuscript.
Publisher’s note
All claims expressed in this article are solely those of the authors and do not necessarily represent those of their affiliated organizations, or those of the publisher, the editors and the reviewers. Any product that may be evaluated in this article, or claim that may be made by its manufacturer, is not guaranteed or endorsed by the publisher.
Supplementary material
The Supplementary material for this article can be found online at: https://www.frontiersin.org/articles/10.3389/ffgc.2025.1512150/full#supplementary-material
Footnotes
References
Alves, L. F., Vieira, S. A., Scaranello, M. A., Camargo, P. B., Santos, F. A., Joly, C. A., et al. (2010). Forest structure and live aboveground biomass variation along an elevational gradient of tropical Atlantic moist forest (Brazil). For. Ecol. Manag. 260, 679–691. doi: 10.1016/j.foreco.2010.05.023
Amadu, F. O., Miller, D. C., and McNamara, P. E. (2020). Agroforestry as a pathway to agricultural yield impacts in climate-smart agriculture investments: evidence from southern Malawi. Ecol. Econ. 167:106443. doi: 10.1016/j.ecolecon.2019.106443
Asanok, L., Krueama, K., Pakketanang, J., and Chiangrang, P. (2024). Variation of shade tree composition and carbon stock of smallholder coffee agroforestry systems along an elevation gradient in Khun Mae Kuang Forest area, northern Thailand. Agrofor. Syst. 98, 3045–3060. doi: 10.1007/s10457-024-01073-9
Atsbha, T., Desta, A. B., and Zewdu, T. (2019). Carbon sequestration potential of natural vegetation under grazing influence in southern Tigray, Ethiopia: implications for climate change mitigation. Heliyon 5:e02329. doi: 10.1016/j.heliyon.2019.e02329
Azene, B., Birnie, A., and Tengnäs, B. (1993). Useful trees and shrubs for Ethiopia: identification, propagation, and management of agricultural and pastoral communities. Ethiopia: SIDA Regional Soil Conservation Unit, 474.
Baul, T. K., Peuly, T. A., Nandi, R., Schmidt, L. H., and Karmakar, S. (2021). Carbon stocks of homestead forests have a mitigation potential for climate change in Bangladesh. Sci. Rep. 11:9254. doi: 10.1038/s41598-021-88775-7
Birhane, E., Ahmed, S., Hailemariam, M., Negash, M., Rannestad, M. M., and Norgrove, L. (2020). Carbon stock and woody species diversity in homegarden agroforestry along an elevation gradient in southern Ethiopia. Agrofor. Syst. 94, 1099–1110. doi: 10.1007/s10457-019-00475-4
Borah, N., Nath, A. J., and Das, A. K. (2013). Aboveground biomass and carbon stocks of tree species in tropical forests of Cachar District, Assam, Northeast India. Int. J. Ecol. Environ. Sci. 39, 97–106.
Chemeda, B. A., Wakjira, F. S., and Hizikias, E. B. (2022). Tree diversity and biomass carbon stock analysis along altitudinal gradients in coffee-based agroforestry system of Western Ethiopia. Cogent Food Agric. 8:2123767. doi: 10.1080/23311932.2022.2123767
Contosta, A. R., Lerman, S. B., Xiao, J., and Varner, R. K. (2020). Biogeochemical and socioeconomic drivers of above and below-ground carbon stocks in urban residential yards of a small city. Landsc. Urban Plan. 196:103724. doi: 10.1016/j.landurbplan.2019.103724
Deneke, D., and Negash, M. (2023). Effects of wealth status on home-garden biomass and soil carbon stocks: the case of midland kebeles of Ofa District, Wolaita zone, southern Ethiopia. J. For. Nat. Resour. 2, 1–15.
Edwards, S., Tadesse, M., and Hedberg, I. (1995). Flora of Ethiopia and Eritrea, vol. 2, Part 2. Addis Ababa, Ethiopia & Uppsala, Sweden: The National Herbarium, Addis AbabaUniversity, Addis Ababa, Ethiopia and Department of SystematicBotany, Uppsala University, Uppsala, Sweden.
Enkossa, T., Nemomissa, N., and Lemessa, D. (2023). Woody species diversity and the carbon stock potentials of different land use types in agroecosystem of Jimma Ganati District, Western Ethiopia. Environ. Chall. 13:100761. doi: 10.1016/j.envc.2023.100761
Eyasu, G., Tolera, M., and Negash, M. (2020). Woody species composition, structure, and diversity of homegarden agroforestry systems in southern Tigray, Northern Ethiopia. Heliyon 6:e05500. doi: 10.1016/j.heliyon.2020.e05500
Feliciano, D., Ledo, A., Hillier, J., and Nayak, D. (2018). Which agroforestry options give the greatest soil and above ground carbon benefits in different world regions? Agric. Ecosyst. Environ. 254, 117–129. doi: 10.1016/j.agee.2017.11.032
Gebre, A. B., Birhane, E., Gebresamuel, G., Hadgu, K. M., and Norgrove, L. (2018). Woody species diversity and carbon stock under different land use types at Gergera watershed in eastern Tigray, Ethiopia. Agrofor. Syst. doi: 10.1007/s10457-018-0226-6
Gebremeskel, D., Birhane, E., Rannestad, M. M., Gebre, S., and Tesfay, G. (2021). Biomass and soil carbon stocks of Rhamnus prinoides based agroforestry practice with varied density in the drylands of Northern Ethiopia. Agrofor. Syst. doi: 10.1007/s10457-021-00608-8
Gebrewahid, Y., Gebre-Egziabhier, T., Teka, K., and Birhane, E. (2018). Carbon stock potential of scattered trees on farmland along an altitudinal gradient in Tigray, Northern Ethiopia. Ecol. Process. 7:40. doi: 10.1186/s13717-018-0152-6
Gebrewahid, Y., and Meressa, E. (2020). Tree species diversity and its relationship with carbon stock in the parkland agroforestry of Northern Ethiopia. Cogent Biol. 6:1728945. doi: 10.1080/23312025.2020.1728945
Hagos, H., Tesfay, G., Brhane, E., Abrha, H., Bezabh, T., Tesfay, B., et al. (2021). Comparison of carbon stock potential of farmland trees in the midlands of Hawzen, Northern Ethiopia. Sustain. Environ. 7, 1–6. doi: 10.1080/27658511.2021.1973696
Hedberg, I., and Edwards, S. (1995). “Flora of Ethiopia and Eritrea” in Poaceae, vol. 7 (Uppsala, Sweden: TheNational Herbarium, Addis Ababa, Ethiopia, and Department of Systematic Botany).
Hedberg, I., Kelbessa, E., Edwards, S., Demissew, S., and Persson, E. (2006). “Flora of Ethiopia and Eritrea” in Gentianaceae to Cyclocheilaceae, vol. 5 (Addis Ababa, Ethiopia & Uppsala, Sweden: The National Herbarium, Addis Ababa University, Addis Ababa, Ethiopia and Department of SystematicBotany, Uppsala University, Uppsala, Sweden).
Henry, M., Tittonell, P., Manlay, R. J., Bernoux, M., Albrecht, A., and Vanlauwe, B. (2009). Biodiversity, carbon stocks, and sequestration potential in aboveground biomass in smallholder farming systems of western Kenya. Agric. Ecosyst. Environ. 129, 238–252. doi: 10.1016/j.agee.2008.09.006
Intergovernmental Panel on Climate Change (IPCC) (2006). 2006 IPCC guidelines for National Greenhouse gas Inventories. In: H. S. Eggleston, L. Buendia, K. Miwa, T. Ngara, and K. Tanabe (Eds.) Geneva, Switzerland: Agriculture, Forestry and Other Land Use (AFOLU). vol. 4. Available at: https://www.ipcc-nggip.iges.or.jp/public/2006gl/vol4.html
Jahan, H., Rahman, M. W., Islam, M. S., Rezwan-Al-Ramim, A., Tuhin, M. M., and Hossain, M. E. (2022). Adoption of agroforestry practices in Bangladesh as a climate change mitigation option: investment, drivers, and SWOT analysis perspectives. Environ. Chall. 7:100509. doi: 10.1016/j.envc.2022.100509
Jose, S., and Bardhan, S. (2012). Agroforestry for biomass production and carbon sequestration: an overview. Agrofor. Syst. 86, 105–111. doi: 10.1007/s10457-012-9573-x
Kassa, G., Bekele, T., Demissew, S., and Abebe, T. (2022). Above- and belowground biomass and biomass carbon stocks in homegarden agroforestry systems of different age groups at three sites of southern and southwestern Ethiopia. Carbon Manag. 13, 531–549. doi: 10.1080/17583004.2022.2133743
Komal, N., Zaman, Q., Yasin, G., Nazir, S., Ashraf, K., Waqas, M., et al. (2022). Carbon storage potential of agroforestry systems near brick kilns in irrigated agroecosystems. Agriculture 12, 1–13. doi: 10.3390/agriculture12020295
Kumar, B. M. (2023). Do carbon stocks and floristic diversity of tropical homegardens vary along an elevational gradient and based on holding size in Central Kerala, India? Agrofor. Syst. 97, 751–783. doi: 10.1007/s10457-023-00821-7
Kuyah, S., Dietz, J., Catherine, M., Jamnadassa, R., Mwangi, P., Coe, R., et al. (2012). Allometric equations for estimating biomass in agricultural landscapes: I. Aboveground biomass. Agric. Ecosyst. Environ. 158, 216–224. doi: 10.1016/j.agee.2012.05.011
Liu, C., and Li, X. (2012). Carbon storage and sequestration by urban forests in Shenyang, China. Urban For. Urban Green. 11, 121–128. doi: 10.1016/j.ufug.2011.03.002
Lowe, A., Kuruppuarachchi, N., Silva, P., Pushpakumara, G., Suriyagoda, L., and Weerahewa, J. (2024). Does human intervention modulate the above-ground carbon biomass of agroforestry systems? A study of homegardens in Jaffna peninsula, Sri Lanka. Agric. Syst. 215:103842. doi: 10.1016/j.agsy.2023.103842
Lowe, W. A. M., Silva, G. L. L. P., and Pushpakumara, D. K. N. G. (2022). Homegardens as a modern carbon storage: assessment of tree diversity and above-ground biomass of homegardens in Matale district, Sri Lanka. Urban For. Urban Green. 74:127671. doi: 10.1016/j.ufug.2022.127671
MacDicken, K. G. (1997). A guide to monitoring carbon storage in forestry and agroforestry projects. Little Rock, Arkansas, USA: Winrock Internationl Institute for Agricultural Development.
Manaye, A., Tesfamariam, B., Tesfaye, M., Worku, A., and Guf, Y. (2021). Tree diversity and carbon stocks in agroforestry systems in northern Ethiopia. Carbon Balance Manag. 16:14. doi: 10.1186/s13021-021-00174-7
Maryo, M., Wolde, A., and Negash, M. (2023). Woody species diversity and carbon stock potentials in homegarden agroforestry and other land-use systems in northern Ethiopia. Heliyon 9:e19243. doi: 10.1016/j.heliyon.2023.e19243
Mayele, J. M., Okullo, J. B. L., Tumwebaze, S. B., and Bongo, A. L. (2020). Diversity and aboveground carbon sequestration potential of on-farm agroforestry trees/shrub species in Rajaf County, South Sudan. Int. J. Innov. Sci. Res. Technol. 5, 987–1000. doi: 10.38124/IJISRT20JUN634
Mbow, C., Smith, P., Skole, D., Duguma, L., and Bustamante, M. (2014). Achieving mitigation and adaptation to climate change through sustainable agroforestry practices in Africa. Curr. Opin. Environ. Sustain. 6, 8–14. doi: 10.1016/j.cosust.2013.09.002
Meragiaw, M. (2017). Role of agroforestry and plantation on climate change mitigation and carbon sequestration in Ethiopia. J. Tree Sci. 36, 1–15. doi: 10.5958/2455-7129.2017.00001.2
Meragiaw, M., Woldu, Z., Martinsen, V., and Singh, B. R. (2021). Carbon stocks of above- and belowground tree biomass in Kibate Forest around Wonchi Crater Lake, Central Highland of Ethiopia. PLoS One 16:e0254231. doi: 10.1371/journal.pone.0254231
Motuma, T., Zebene, A., Mulugeta, L., and Karltun, E. (2008). Woody species diversity in a changing land scape in the south-central highlands of Ethiopia. Agric. Ecosyst. Environ. 128:58. doi: 10.1016/j.agee.2008.05.001
Nair, P. K. R. (2012). Carbon sequestration studies in agroforestry systems: a reality check. Agrofor. Syst. 86, 243–253. doi: 10.1007/s10457-011-9434-z
Nair, P. K. R., Kumar, B. M., and Nair, V. D. (2021). An introduction to agroforestry: four decades of scientific developments. 2nd Edn. The Netherlands: Springer Science.
Negash, M. (2013). The indigenous agroforestry systems of the south-eastern Rift Valley escarpment, Ethiopia: Their biodiversity, carbon stocks, and litterfall. PhD Dissertation. Finland: University of Helsinki.
Negash, M., Abdulkadir, A., and Hagberg, S. (2005). Farmers’ planting practices of Eucalyptus in Enset-Coffee-based agroforestry system of Sidama, Ethiopia. Ethiopian J. Nat. Resour. 7, 239–251.
Pearson, T. R., Brown, S. L., and Birdsey, R. A. (2007). Measurement guidelines for the sequestration of forest carbon : General Technical Report, NRS-18. US Department of Agriculture, Forest Service, Northern Research Station.
Pokhrel, S., and Sherpa, C. (2020). Analyzing the relationship, distribution of tree species diversity, and aboveground biomass on the chitwan-annapurna landscape in Nepal. Int. J. For. Res. 2020, 1–10. doi: 10.1155/2020/2789753
Schoeneberger, M., Bentrup, G., de Gooijer, H., Soolanayakanahally, R., Sauer, T., Brandle, J., et al. (2012). Branching out: agroforestry as a climate change mitigation and adaptation tool for agriculture. J. Soil Water Conserv. 67, 128A–136A. doi: 10.2489/jswc.67.5.128A
Sharma, R., Mina, U., and Kumar, B. M. (2022). Homegarden agroforestry systems in achievement of sustainable development goals. A review. Agron. Sustain. Dev. 42:44. doi: 10.1007/s13593-022-00781-9
Sharma, H., Pant, K. S., Bishist, R., Gautam, K. L., Dogra, R., Kumar, M., et al. (2023). Estimation of biomass and carbon storage potential in agroforestry systems of north western Himalayas, India. Catena 225:107009. doi: 10.1016/j.catena.2023.107009
Shiferaw, H., Kassawmar, T., and Zeleke, G. (2022). Above and belowground woody-biomass and carbon stock estimations at Kunzila watershed, Northwest Ethiopia. Trees Forests People 7:100204. doi: 10.1016/j.tfp.2022.100204
Siarudin, M., Rahman, S. A., Artati, Y., Indrajaya, Y., Narulita, S., Ardha, M. J., et al. (2021). Carbon sequestration potential of agroforestry Systems in Degraded Landscapes in West Java, Indonesia. Forests 12:714. doi: 10.3390/f12060714
Siyum, G. E., and Tassew, T. (2019). The use of homegarden agroforestry systems for climate change mitigation in lowlands of southern Tigray, northern Ethiopia. Asian Soil Res. J. 2, 1–13.
Soazafy, M. R., Osen, K., Wurz, A., Raveloaritiana, E., Martin, D. A., Ranarijaona, H. L., et al. (2021). Aboveground carbon stocks in Madagascar’s vanilla production landscape – exploring rehabilitation through agroforestry in the light of land-use history. Global Ecol. Conserv. 31:e01853. doi: 10.1016/j.gecco.2021.e01853
Subba, M., Pala, N. A., Shukla, G., Pradhan, K., and Chakravarty, S. (2017). Relationship of socio-economic factors with attributes of Homegarden agroforestry Systems in Northern Part of West Bengal. J. Tree Sci. 36, 76–91. doi: 10.5958/2455-7129.2017.00029.2
Tadesse, W. (2015). GHG emission assessment guideline volume II: aboveground biomass field guide for baseline survey. Ethiopia: Addis Ababa.
Terakunpisut, J., Gajaseni, N., and Ruankawe, N. (2007). Carbon sequestration potential in aboveground biomass of thong Pha Phum National Forest, Thailand. Appl. Ecol. Environ. Res. 5, 93–102.
Tesfay, F., Moges, Y., and Asfaw, Z. (2022). Woody species composition, structure, and carbon stock of coffee-based agroforestry system along an elevation gradient in the moist mid-highlands of southern Ethiopia. Int. J. For. Res. 2022, 1–13. doi: 10.1155/2022/4729336
Tolessa, T., Dechassa, C., Simane, B., Alamerew, B., and Kidane, M. (2019). Land use/land cover dynamics in response to various driving forces in Didessa sub-basin, Ethiopia. GeoJournal. doi: 10.1007/s10708-019-09990-4
Tsedeke, R. E., Dawud, S. M., and Tafere, S. M. (2021). Assessment of carbon stock potential of parkland agroforestry practice: the case of Minjar Shenkora, north Shewa, Ethiopia. Environ. Syst. Res. 10:2. doi: 10.1186/s40068-020-00211-3
Unwin, G. L., and Kriedemann, P. E. (2000). “Principles and processes of carbon sequestration by trees” in Technical paper No 64. Research and Development division (Australia: State Forests of New South Wales).
Verchot, L. V., Van Noordwijk, M., Kandji, S., Tomich, T., Ong, C., Albrecht, A., et al. (2007). Climate change: linking adaptation and mitigation through agroforestry. Mitig Adapt Strat Glob Change. doi: 10.1007/s11027-007-9105-6
Verma, A., Naorem, A., Keerthika, A., and Kumar, S. (2024). Biomass and carbon partitioning across different components of agroforestry systems in the arid zones of India. Agrofor. Syst. 98, 3089–3108. doi: 10.1007/s10457-024-01076-6
WMO (2022). World Meteorological Organization (WMO). Climate Explorer. Geneva, Switzerland: European Climate Assessment and Dataset.
Keywords: altitudinal change, carbon stock, socioeconomic, woody biomass, woody plant
Citation: Jegora T, Hundera K, Kebebew Z and Bekele AE (2025) Woody carbon stock estimation in homegarden agroforestry along altitudinal gradients in southwest Ethiopia. Front. For. Glob. Change. 8:1512150. doi: 10.3389/ffgc.2025.1512150
Edited by:
Francesco Orsi, Wageningen University and Research, NetherlandsReviewed by:
D. S. Chauhan, Hemwati Nandan Bahuguna Garhwal University, IndiaSumit Chakravarty, Uttar Banga Krishi Viswavidyalaya, India
Larba Hubert Balima, Joseph KI-ZERBO University, Burkina Faso
Copyright © 2025 Jegora, Hundera, Kebebew and Bekele. This is an open-access article distributed under the terms of the Creative Commons Attribution License (CC BY). The use, distribution or reproduction in other forums is permitted, provided the original author(s) and the copyright owner(s) are credited and that the original publication in this journal is cited, in accordance with accepted academic practice. No use, distribution or reproduction is permitted which does not comply with these terms.
*Correspondence: Tefera Jegora, dGVmZXJhamVnb3JhQGdtYWlsLmNvbQ==