- 1Department of Forestry, Faculty of Agriculture, Universitas Syiah Kuala, Banda Aceh, Indonesia
- 2Research Center for Forestry and Ecosystem, Universitas Syiah Kuala, Banda Aceh, Indonesia
- 3Department of Agribusiness, Faculty of Agriculture, Universitas Syiah Kuala, Banda Aceh, Indonesia
- 4Center for Sustainable Agricultural and Rural Development, Universitas Syiah Kuala, Banda Aceh, Indonesia
- 5Department of Post Harvest Technology, Faculty of Agriculture, Universitas Syiah Kuala, Banda Aceh, Indonesia
- 6Aceh Environment and Forestry Agency, Banda Aceh, Indonesia
Tropical agroforestry systems can mitigate climate change through carbon storage while supporting biodiversity and improving livelihoods. This study evaluates the potential of coffee agroforestry systems in the Gayo Highlands, Indonesia, to achieve carbon storage levels comparable to secondary and primary forests. Vegetation data are collected across three locations, covering coffee agroforestry, secondary forest, and primary forest. We found that primary forests had the highest carbon stocks, with 223.53 tC ha−1 in Location 1, 194.68 tC ha−1 in Location 2, and 542.55 tC ha−1 in Location 3. Coffee agroforestry in Location 1 had carbon stocks comparable to secondary forest, while in Location 3, it showed the lowest carbon stock (6.26 tC ha−1). Higher carbon stocks were linked to greater tree species richness, larger DBH, and higher basal areas. Additionally, we observed a positive relationship between tree basal area and aboveground carbon stock, confirming that forests with higher basal area values exhibit greater carbon storage capacity. The findings underscore the critical role of mature trees in carbon storage and highlight the value of preserving mature forest species in coffee agroforestry systems.
1 Introduction
Arabica coffee agriculture is one of the crop plantations dominating highlands in Indonesia, which is found on almost every island with highlands of at least 1,000 meters above sea level (m asl) (Anhar et al., 2021a,b). This crop plantations are spread across small and large Indonesian islands, mainly Sumatra, Sulawesi, Bali and Papua (Azis and Irjayanti, 2023). In recent years, climate change has presented a significant threat to Arabica coffee production, particularly in Indonesia. The impact of climate change on this crop is due to the rise in temperatures and shifts in rainfall patterns that reduces climatically suitable areas for coffee cultivation by up to 84%, leading to lower yields and declines in coffee quality (Koutouleas et al., 2022). It was projected that by 2050, more than half of current production zones may become unsuitable, which results in productivity losses and increasing the prevalence of pests and diseases (Schroth et al., 2015; Ramadhillah and Masjud, 2024).
In response to the impact of climate change, many farmers, especially those living in the forest frontiers, have been compelled to expand cultivation into higher elevations, which often include protected forests (Ramadhillah and Masjud, 2024). This practice not only raises concerns about deforestation but also jeopardizes the sustainability of the coffee sector itself. Farmers may face difficulties in selling their coffee at premium prices or to reputable markets, as the lack of legal compliance prevents them from obtaining necessary certifications (Wahyudi et al., 2020). Arabica coffee grown in forest areas can be an option to increase production to meet market demand while also improving farmers’ livelihoods (Jezeer et al., 2019). Indonesian government has offered the resolution of legalizing agroforestry plantations within forest areas through the social forestry program (Djamali et al., 2022; Sanudin et al., 2024). This initiative enables farmers who have already cultivated coffee in forested areas to receive guidance on managing their land through organic agroforestry practices (Sanudin et al., 2024). These practices can improve community livelihoods while maintaining the forest’s woody plant cover, thereby helping to preserve the forest’s ecological functions (Kebebew and Ozanne, 2024).
Tropical agroforestry systems provide a range of ecological and socioeconomic benefits, so that they become a sustainable alternative to conventional agricultural methods. The systems incorporate trees with crops, which results in enhanced biodiversity and ecosystem services, including soil fertility, water regulation, and natural pest control (Jezeer et al., 2019). For smallholder farmers, agroforestry offers diversified income opportunities through products like fruits, timber, and medicinal resources, thus improving food security and resilience against market variability (Jezeer et al., 2019). Agroforestry systems also contribute to nature conservation by creating layered habitats that support diverse species, helping to mitigate the adverse effects of deforestation and complementing the protection of primary forests (Justine et al., 2019). A significant benefit of tropical agroforestry is its carbon storage potential, which makes it an effective climate change mitigation strategy. The integration of trees into agricultural landscapes stores carbon both in biomass and soil, offering higher sequestration potential than other terrestrial and oceanic options (Justine et al., 2019). Coffee agroforestry systems with shade trees exhibit greater aboveground carbon storage compared to coffee monocultures, while still maintaining relatively similar amount of crop yields (Ortiz-Ceballos et al., 2020).
Despite the increasing adoption of agroforestry within Indonesia’s SF program, empirical data on its effectiveness in enhancing carbon storage within protected forest landscapes remains limited. Most studies on agroforestry under SF have focused on its socio-economic benefits, such as improving farmers’ income, securing land tenure, and promoting sustainable farming practices. However, its ecological contributions—particularly in terms of carbon sequestration and forest restoration—are underexplored. Additionally, there is a lack of well-defined thresholds for optimizing carbon storage in coffee agroforestry systems. While agroforestry is widely recognized as a climate mitigation strategy, existing research rarely quantifies the ideal composition of tree species and stand structures needed to maximize carbon sequestration.
Given that SF regulations mandate a combination of native and economic tree species within agroforestry plantations, there remains a critical need for scientific assessment to determine whether these systems contribute meaningfully to climate mitigation. This knowledge gap is particularly pressing in Indonesia, where illegal encroachment and deforestation pressures persist despite the implementation of SF policies. Addressing this research deficit is essential for refining policies that ensure SF programs achieve both conservation and carbon sequestration goals. By providing empirical evidence on the carbon storage potential of coffee agroforestry relative to secondary and primary forests, this study offers crucial insights into the environmental effectiveness of Indonesia’s SF initiatives.
The Gayo Highlands is Indonesia’s leading region for Arabica coffee production, with an annual yield reaching up to 40,800 tons from a cultivation area spanning 103,000 hectares (Anhar et al., 2021a). Coffee plantations here are managed through different systems, including monoculture and shade-based agroforestry with tree species such as avocado (Persea americana), river tamarind (Leucaena leucocephala), and durian (Durio zibethinus). However, as climate pressures intensify, farmers in this region are increasingly expanding cultivation toward forest borders, often encroaching into protected forest areas (Anhar et al., 2021b; Fardinatri et al., 2024). Farmers operating within these protected zones under the SF scheme require targeted assistance to implement sustainable coffee cultivation practices that preserve forest ecosystem services, particularly carbon storage. This study aims to identify the most adaptive agroforestry patterns for enhancing carbon storage, specifically assessing which tree compositions within agroforestry systems can achieve carbon stocks comparable to those found in Indonesia’s secondary and primary forests.
2 Theoretical grounding
2.1 Impact of climate change on coffee production
Increasing atmospheric carbon dioxide (CO2) concentrations have led to systematic changes in average climate conditions, including greater temperature and precipitation variability and more frequent extreme weather events (Ahmed et al., 2021). These changes are affecting coffee production in multiple ways, including shifts in suitable geographic ranges, altered trophic interactions in agroecosystems, impacts on productivity, and changes in crop quality (Ahmed et al., 2021). Coffee, predominantly sourced from Coffea arabica (Arabica coffee) and Coffea canephora (Robusta coffee), is highly sensitive to changes in temperature and precipitation. The optimal growing conditions for Arabica coffee are temperatures between 14 and 26°C, annual rainfall between 1,000 and 2,700 mm, and a dry period of 1–3 months annually (Ahmed et al., 2021). Increasing occurrences of heat waves, droughts, and other climate extremes are pushing many coffee-growing regions outside these optimal ranges, threatening productivity and quality. A temperature increase of just 1°C above the mean minimum of 16.2°C during the growing season can reduce Robusta coffee production by 350–460 kg per hectare, equivalent to a 14% yield decline (Bilen et al., 2022). Moreover, temperature level that exceeds the optimal range for coffee plants and decreased water availability negatively impact its yield and quality, with the potential for further adverse interactions between these environmental variables under field conditions. This suggests that high temperatures and limited water availability may not only individually affect coffee production but could also interact with each other in complex ways under real-world growing conditions, leading to even greater negative impacts. For example, prolonged heat can increase the plant’s water demand, worsening the effects of drought. At the same time, water shortages can reduce the plant’s ability to cool itself through transpiration, making it more vulnerable to heat stress (Cassamo et al., 2023).
Traditional coffee-growing regions are experiencing reduced suitability due to increased temperatures and irregular precipitation patterns. For example, extended periods of temperatures above 30°C can significantly decrease Arabica coffee yields (Cassamo et al., 2023). Shifts in climate conditions are also contributing to the increased incidence and distribution of insect pests and diseases, further threatening coffee productivity and farmer livelihoods (Chemura et al., 2021; Bilen et al., 2022). Coffee quality is highly sensitive to environmental and management conditions throughout the production process. Key environmental factors influencing coffee quality include altitude, light exposure, and rainfall patterns (Ahmed et al., 2021). For example, increased altitude and decreased light exposure (up to certain thresholds) enhance sensory attributes such as acidity, body, and flavor. Climate change is also expected to significantly impact the production of specialty coffee due to its strong dependence on specific environmental conditions that contribute to its unique flavor profiles. According to Chemura et al. (2021), specialty coffee quality is influenced by a combination of local climatic factors (such as rainfall, temperature, humidity, and radiation), topographical features (including elevation, slope angle, and aspect), and soil characteristics (like depth, acidity, and fertility). These conditions are unique to each coffee-growing region, giving specialty coffee its distinctive taste and aroma. However, even slight modifications in these environmental variables—often driven by climate change—can disrupt this delicate balance, leading to changes in the sensory attributes of the coffee. Therefore, climate change is redrawing the map of suitable coffee cultivation zones. While some regions, particularly higher elevations in East Africa and Asia, may become more conducive to coffee production, these shifts often come at a steep environmental cost. Exploiting new coffee-growing areas frequently necessitates land-use changes, including deforestation, which contributes to biodiversity loss and increased greenhouse gas emissions.
2.2 Land use and land cover change and carbon dynamic
Land use and land cover changes (LULCC) are recognized as critical drivers of global carbon dynamics, particularly in tropical zones. These alterations significantly contribute to greenhouse gas (GHG) emissions, primarily through deforestation, forest degradation, and the transformation of natural landscapes into agricultural areas (Berhanu et al., 2023). Notably, tropical forests are experiencing substantial annual losses, exceeding 9.3 million hectares, and are estimated to contribute approximately 10–20% of anthropogenic carbon dioxide emissions (Berhanu et al., 2023). Tropical forests serve as major global carbon reservoirs, storing approximately 40% of terrestrial carbon, distributed as 58% in vegetation, 41% in soil, and 1% in litter (Bera et al., 2024). The conversion of these carbon-rich ecosystems to agricultural land, including coffee cultivation, has considerable ramifications for global carbon stocks and sequestration capabilities. Deforestation and forest degradation driven by agricultural expansion, logging, and shifting cultivation are primary causes of forest loss in tropical countries, leading to marked reductions in carbon storage capacity (Bera et al., 2024). Although certain land use changes, such as the expansion of coffee plantations and plantation forests, have demonstrated some potential for GHG mitigation, their carbon sequestration capacity remains inadequate to compensate for the carbon losses resulting from deforestation (Berhanu et al., 2023).
Coffee production exerts a substantial influence on LULCC, particularly in tropical regions where it is predominantly cultivated. The conversion of natural forests and secondary vegetation into coffee plantations has led to significant impacts on carbon stocks and ecosystem services. Shade-grown coffee agroecosystems are traditionally incorporated within forest, rainforest, and secondary vegetation covers, contributing to both biodiversity conservation and carbon sequestration. However, these land cover types have undergone considerable declines due to the expansion of coffee plantations, which reflects a transition from conventional shade coffee systems toward more intensive agricultural practices (Navidad Murrieta et al., 2023). The reduction in vegetation cover leads to a decrease in carbon stocks as the biomass and soil organic matter that sequester carbon are lost during land conversion. This process contributes to elevated greenhouse gas emissions, thus impacting the regional carbon balance and accelerating climate change (Ango et al., 2020; Navidad Murrieta et al., 2023).
2.3 Agroforestry for climate and economic solution
Agroforestry, by definition, integrates tree-based forestry practices with agricultural commodities, promoting sustainable land management. Several regulations govern agroforestry practices in Indonesia, including Government Regulation (PP) Number 26/2020 on forest rehabilitation and reclamation. This regulation defines rehabilitation as reforestation through agroforestry on degraded open land, shrublands, gardens, mixed-crop gardens, dryland farms, and areas where agricultural activities are already taking place. Furthermore, the Minister of Environment and Forestry Regulation Number P.105/2018 outlines the procedures for implementing, supporting, incentivizing, and overseeing forest and land rehabilitation efforts. Under this regulation, agroforestry within protected or production forests must include: (a) staple crops consisting of woody plant species and/or non-timber forest products, with a minimum of 400 plants per hectare, and (b) intercropping species, hedgerows, and firebreaks, including Leucaena leucocephala (lamtoro), Gliricidia sepium (gamal), Caesalpinia sappan (secang), coffee (Coffea spp.), and Calliandra calothyrsus (kaliandra). The regulation mandates that intercropping plants, hedgerows, and firebreak species must constitute at least 25% of the total vegetation in an agroforestry system, while the primary tree species make up the remaining 75%.
Agroforestry systems (AFS) have been widely recognized as a nature-based strategy to enhance the resilience of coffee production to climate change while simultaneously providing ecological and economic benefits. AFS integrates forest trees or shrubs with agricultural crops, creating an ecologically and economically interactive system that can mitigate the adverse effects of climate change (Moreira et al., 2018; Kath et al., 2021; Koutouleas et al., 2022). In coffee production, shading strategies have been shown to reduce the negative impact of rising temperatures, contributing to climate adaptation and mitigation (Cassamo et al., 2023). The microclimatic benefits of AFS include lower air and leaf temperatures, higher humidity, and improved water use efficiency, which help regulate vapor pressure deficit and reduce evapotranspiration, enhancing coffee plants’ resilience to heat and drought (Koutouleas et al., 2022). AFS also improves soil quality by enhancing chemical and physical properties, increasing organic matter, and sequestering carbon both above and below ground. Diversifying tree species further boosts carbon storage while maintaining biodiversity and ecosystem services (Bera et al., 2024).
Despite its advantages, AFS also presents challenges, as shade trees can influence coffee growth, yield, and bean quality in complex ways. The impact of shade varies depending on local environmental conditions, shade density, crop management practices, and the specific Coffea arabica cultivars being grown. In some cases, excessive shading may reduce net photosynthesis and exacerbate pest and disease pressures, leading to uncertainty regarding the optimal application of agroforestry practices (Koutouleas et al., 2022). However, when properly managed, AFS can create a more favorable microenvironment for coffee plants, mitigating climate-related stressors without significantly reducing yield while enhancing coffee quality (Gomes et al., 2020; De Leijster et al., 2021; Haggar et al., 2021). Globally, AFS has been successfully implemented in major coffee-producing regions, including Latin America (e.g., Costa Rica, El Salvador, Guatemala, Mexico, and Colombia) and Africa, which shows its potential as a sustainable crop management strategy (Gomes et al., 2020; De Leijster et al., 2021; Haggar et al., 2021). Beyond its role in climate adaptation, AFS also provides economic benefits by supporting diversified income streams for farmers through additional tree-based products such as timber, fruits, and fuelwood (Moreira et al., 2018; Kath et al., 2021; Koutouleas et al., 2022).
2.4 Coffee production under social forestry scheme in Indonesia
Social Forestry (SF) is a sustainable forest management approach that integrates local and indigenous communities as key actors in managing state or customary forests. This scheme aims to enhance livelihoods, maintain environmental balance, and support sociocultural dynamics through various mechanisms, including village forests, community forestry, and forestry partnerships (Octavia et al., 2022). Policy reforms in forest governance under the SF framework have contributed to a significant decline in deforestation rates. Between 2017 and 2018, deforestation decreased by 0.49 million hectares, and in 2019–2020, it further declined by 75.03%, reaching a historic low of 115.46 thousand hectares (Octavia et al., 2022). These policy measures have also expanded opportunities for local communities to gain legal access to forest resources, including the development of agroforestry systems for coffee cultivation.
Smallholder plantations dominate coffee production in Indonesia, covering 98.3% of the total coffee-growing area and contributing nearly all (99.9%) of the national coffee output (Ulya et al., 2023). A large portion of these smallholder plantations operate within agroforestry systems, which are increasingly incorporated into Social Forestry (SF) programs, particularly in protection and production forests. In Central Aceh, approximately 3,406 hectares of coffee plantations are situated within protected forests. Additionally, coffee cultivation has been recorded in strictly protected areas, including national parks and nature reserves, highlighting the complexities of land use in conservation zones (Fardinatri et al., 2024). The integration of coffee agroforestry within SF schemes presents both opportunities and challenges. One of the key management strategies is balancing shade levels to optimize both forest conservation and coffee productivity. While SF regulations encourage farmers to maintain native forest trees within coffee plantations, excessive shading can negatively impact coffee growth and yield (Haggar et al., 2021). Thus, successful implementation of coffee agroforestry within SF programs requires coordinated efforts between farmers and forest managers to develop sustainable shade management strategies that support both ecological integrity and economic viability.
3 Hypothesis development
The development of our hypothesis is grounded in the interplay between climate change, land use dynamics, and the role of agroforestry in carbon sequestration. Climate change has significantly affected coffee production, leading to shifts in land use as farmers expand plantations into previously forested areas (Section 2.1). These land use and land cover changes alter carbon storage potential, particularly when mature trees are removed in favor of monocultures or less diverse agroecosystems (Section 2.2). However, agroforestry presents a potential solution, offering both climate resilience and economic benefits while maintaining ecological functions (Section 2.3).
Agroforestry has been widely recognized as a sustainable land-use strategy that enhances carbon storage while supporting agricultural productivity. Within Indonesia’s social forestry framework, agroforestry serves as a means to resolve land tenure conflicts in state forest areas while promoting forest rehabilitation and sustainable livelihoods. Government regulations mandate that agroforestry systems in protected and production forests maintain a structured composition, with at least 400 woody plants per hectare and a 75:25 ratio between primary tree species and intercropped plants, hedgerows, or firebreaks. This structured approach ensures that agroforestry contributes to both ecological and economic objectives. One of the key ecological benefits of agroforestry is its potential to enhance carbon sequestration, particularly when incorporating mature forest tree species. The presence of large-diameter trees significantly influences carbon dynamics, as trees with a diameter at breast height (DBH) ≥ 20 cm contribute disproportionately to total carbon storage.
Based on this premise, we hypothesize that coffee agroforestry systems that maintain a 25% composition of mature forest tree species can achieve carbon storage levels similar to those of secondary forests. This hypothesis aligns with existing policy frameworks that regulate agroforestry composition while also considering the role of mature trees in carbon sequestration.
4 Materials and methods
4.1 Study area
The Gayo Highlands span three adjacent districts, namely Aceh Tengah, Bener Meriah, and Gayo Lues. This area is the largest Arabica coffee producer in Indonesia (Cassamo et al., 2023). Our study location falls within the jurisdiction of Aceh Tengah, which is the main coffee producer district with the most area of coffee plantation among the three. The geographical coordinates of Aceh Tengah range from 4°22′14.42″–4°42′40.80″N and 96°15′23.60″–97°22′10.76″E. Approximately 37% of the land lies below 1,000 meters above sea level (asl), while 20.7 and 42.3% are situated at elevations between 1,000 and 1,600 meters asl, and above 1,600 meters asl, respectively (Anhar et al., 2021a). The mean annual temperature is 19°C, with humidity varying as low as 57% during dry seasons and up to 98% during rainy seasons. The mean annual rainfall in the area is 1,575 mm, with one peak in February–March and another one in October–November. Most of the district’s higher elevation zones directly bordering protected forests of primary tropical rain forest (Figure 1).
4.2 Sampling procedure
Vegetation data are collected using the Vegetation Analysis method with Line Plot Sampling across four growth phases: seedlings, saplings, poles, and trees. Sampling is conducted at three research locations: Location 1 in Jagong Jeget and Pegasing District, Location 2 in Ketol District, and Location 3 in Permata District. These districts were selected because they encompass all major land use types and serve as a representative model of the Gayo Highland’s land use conditions, particularly in terms of coffee agroforestry, secondary forest, and primary forest. Moreover, these 3 districts are actively implementing coffee agroforestry inside protected forests under the social forestry scheme. In each of these locations, we conducted the data collection in three land cover types—coffee agroforestry, secondary forest, and primary forest (Table 1). The selection of agroforestry and secondary forest sites was based on areas that had been accessed and managed by local communities, whereas primary forest sites were chosen from areas that remained unaccessed by local communities. In these locations, we established five transects, each was 1,000 meters long and 20 meters wide, with a 100-meter distance between transects. A total of 113 sampling plots, each measuring 20 m2, are established across three locations: Location 1 (39 plots), Location 2 (37 plots), and Location 3 (37 plots). we collected data on tree species and measure the tree height and diameter at breast height (DBH). The tree identification was done by a tree expert and local guide who helps identify the local name of the species, and the confirmation was also done using various guidebooks for tree species identification in Indonesia’s forests.
4.3 Biomass and carbon estimation
Biomass and carbon estimation were conducted using non-destructive methods based on field measurements and allometric equations. Tree height and diameter at breast height (DBH) were recorded in the field and used to calculate tree volume. The volume estimation applied a form factor to account for variations in tree shape and structure. Wood density values, obtained from regional forestry databases, were used to convert tree volume into biomass. These values were species-specific to improve accuracy in biomass estimation. Aboveground biomass (AGB) was then calculated using allometric equations developed for tropical forests, which integrate species growth characteristics and structural attributes. These equations have been validated for use in Southeast Asian forest ecosystems. Carbon storage was estimated by applying a standard carbon fraction of 47% to the total aboveground biomass, following the guidelines set by the Badan Standardisasi Nasional (BSN) (2011).
The parameters used were tree heights and DBH, which then analyzed using the following model (Equations 1, 2):
where: V [Tree Volume (m3)]; Π (3,14); t [Tree Height (m)]; d (DBH); f [Form Factor (0,6)]
The density of vegetation types affects the total biomass within a given area and helps in determining the density of each group of plant species present. Vegetation density was calculated by dividing the number of individuals by the area of the sampling plot, allowing for an assessment of both density and average distance between plants. After obtaining the volume calculations, biomass analysis was conducted. Aboveground biomass for the seedling phase was estimated at 0.17% of the total stand biomass (Irvianty and Defira, 2023). Biomass for the sapling, pole, and tree phases was then calculated using the allometric equation for secondary forests (Manuri et al., 2016) (Equation 3).
4.4 Carbon storage potential
Carbon storage measurement was conducted using the model from the Badan Standardisasi Nasional (BSN) (2011), which determined that 47% of the forest’s vegetation biomass consists of carbon. Thus, the formula is as follows (Equation 4):
The data are analyzed using a descriptive quantitative approach, followed by further analysis of observational and measurement results with the APN Forest Carbon Calc Tool 2021 formula (Samek et al., 2022). The APN Forest Carbon Calc Tool is an innovative platform developed based on multiple allometric equations for carbon calculation across various forest types, including tropical dipterocarp forests, secondary tropical dipterocarp forests, peat swamp forests, secondary peat swamp forests, and mangrove forests. This tool automatically differentiates between plot types, categorizing them into measurement plots for different growth phases—seedlings, saplings, poles, and trees—based on field observation plot sizes. During field measurements, only tree circumference and angle degrees are required to determine tree height, after which the tool automatically calculates key parameters, including volume, density, aboveground biomass, carbon stock potential, and carbon sequestration potential for the observed area.
5 Results
5.1 Tree composition
5.1.1 Tree species composition in across land cover type
Using the Line Plot Sampling for vegetation analysis, we collected data on the vegetation composition. We categorized the study sites into three land cover types to compare tree species in coffee agroforests, secondary forests, and primary forests. The results revealed a total of 22 tree species in coffee agroforestry areas, 53 species in secondary forests, and 63 species in primary forests (Table 2). Tree species native to local forests are found predominantly in the primary and secondary forest sites. In contrast, coffee agroforestry areas included several introduced species used as shade trees for coffee plantations, such as Persea americana and Manilkara zapota.
According to the analysis of tree density, we found that coffee agroforestry has the highest density (637.5 trees ha−1), followed by the primary forest (472.9 trees ha−1). Secondary forests had the lowest tree density among the three land cover types, at 437.5 trees ha−1. The high density observed in coffee agroforestry is attributed to the inclusion of coffee plants in the analysis. The composition of vegetation species in the three forest types represents a model of forest typology found in the Gayo Highlands. In general, the study sites in these three areas are representative of other regions within the Gayo Highlands. Primary and secondary forests predominantly consist of dipterocarp and pine tree species, while agroforestry lands are managed by local communities, with coffee serving as the primary cultivated commodity (see Table 3).
5.1.2 Tree species composition across agroforestry systems
We also analyzed the differences of tree species composition in coffee agroforestry among the three study locations. This analysis aimed at understanding how the species composition differs in the agroforestry systems between these three locations. Our results (Table 4) suggest that the differences in species richness across the locations are not significant (n = 9–10), with Location 3 having the most species (n = 10), while Location 1 and 2 each had 9 species. However, despite hosting the most tree species, Location 3 seems to contain smaller trees, with an average DBH of 0.06 meters. Adversely, the trees found in Location 1 and 2 had average DBH of approximately 0.13 and 0.11 meters each. These differences in tree size align with the average basal area values across the locations. The average basal areas of all species were 0.018 m2, 0.017 m2, and 0.005 m2 in Locations 1, 2, and 3, respectively. For the total basal area of all species, Location 1 exhibited the highest value at 5.161 m2, followed by Location 2 at 1.428 m2. Location 3 had the smallest total basal area, measuring only 0.671 m2. The analysis of average basal area per species also accounted for saplings and poles, which explains the smaller average values observed despite many individuals are found for each species.
5.1.3 Composition of mature trees across locations
There is no universally established standard for categorizing mature tree individuals. Consequently, in this study, we define mature trees based on the widely accepted framework of four growth stages (seedlings, saplings, poles, stands), considering trees with a DBH of at least 20 cm (0.20 m) as mature, in accordance with the criteria for a stand. The analysis of tree populations across three locations reveals notable differences in the maturity and structural composition of the stands. In Location 1, a significant proportion of the basal area (78%) is comprised of trees with a DBH ≥ 20 cm, with 31% of the individual trees meeting this size threshold. Location 2 also exhibits a high basal area proportion (77%) of mature trees, although the percentage of individual trees with DBH ≥ 20 cm drops to 20%, suggesting that while mature trees dominate in terms of size, the tree population includes a larger proportion of smaller individuals. In Location 3, the basal area of mature trees is substantially lower at 61%, and only 16% of individual trees meet the 20 cm DBH threshold, reflecting a younger or less mature forest where smaller trees predominate. These findings suggest that Location 1 hosts the most mature and structurally developed stand, while Location 3 may be characterized by a younger tree population, with Location 2 falling between the two in terms of maturity (see Table 5).
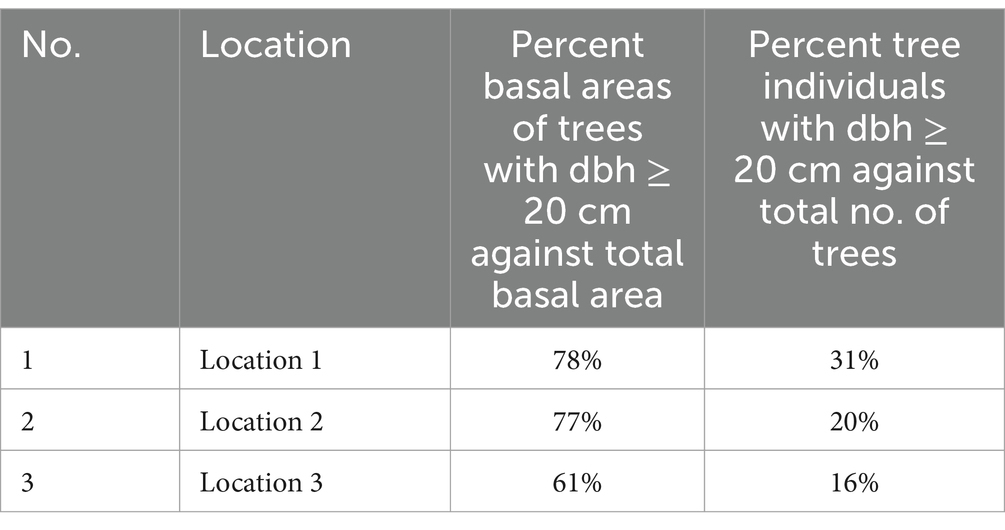
Table 5. Percentage of tree basal area and individual trees with minimum DBH of 20 cm in agroforestry plots in three locations.
5.2 Carbon storage
From the vegetation composition data, we calculated the carbon stocks and carbon sequestration potential based on land use type. Expectedly, our results indicate that the highest carbon stock potential is found in the primary forest across all study locations, each containing 223.53 tC ha−1 in Location 1, 194.68 tC ha−1 in Location 2, and 542.55 tC ha−1 in Location 3. Interestingly, the carbon stock in the coffee agroforest in Location 1 is relatively comparable to that in the secondary forest. Additionally, in Location 3, the coffee agroforestry area has the lowest carbon stock (6.26 tC ha−1), while the primary forest in the same location exhibits significantly higher carbon stocks (542.55 tC ha−1) than those in Locations 1 and 2 (Figure 2).
The carbon stock findings are consistent with the vegetation composition results, where areas with greater tree species richness, larger average tree DBH, and higher total basal areas also exhibit higher carbon stocks. Notably, primary forests, which contain the highest species richness, have the largest carbon stocks. Among the coffee agroforestry systems, Location 1 contains higher carbon stocks than those in Locations 2 and 3. This outcome aligns with the vegetation characteristics of the area, as Location 1’s coffee agroforestry has trees with larger average DBH, greater average basal areas, and higher total basal areas compared to the other locations (see Table 6).
6 Discussion
A comparative analysis of tree composition and carbon stock across three locations provided critical insights into the capacity of coffee agroforestry systems to emulate the carbon storage capabilities of secondary and primary forests. While tree species diversity plays a role in carbon sequestration, the structural characteristics of trees, particularly their DBH and basal area, have a more direct impact on carbon storage. Our hypothesis, positing that agroforestry systems incorporating 25% mature forest trees could attain comparable carbon storage levels, was partially validated. Location 1, characterized by a mature tree composition of 31%, exhibited the closest approximation to secondary forest levels. Conversely, Location 2, despite the presence of 20% mature trees, fell short of the carbon sequestration potential observed in secondary forests. To enhance carbon sequestration, strategic interventions aimed at improving tree growth, density, and diversity would be necessary. Location 3, distinguished by the lowest proportion of trees exceeding a 20 cm diameter at breast height (DBH) and the lowest carbon stock, underscored the important role of mature trees in carbon storage. These findings align with the previous research demonstrating that tree DBH and basal area are strong predictors of aboveground biomass and carbon sequestration (Ma et al., 2020; Mildrexler et al., 2020). The introduction of fast-growing tree species could potentially increase carbon storage capacity and facilitate a closer approximation to the carbon storage levels characteristic of secondary forests. This suggests that while mature tree composition is an essential factor, other ecological and management-related variables may also influence carbon sequestration efficiency.
Mature trees play a critical role in carbon sequestration, which significantly influence forest carbon dynamics and ecosystem health. Large-diameter trees store substantial amounts of carbon, acting as major drivers of the global carbon cycle (Mildrexler et al., 2020). These trees continue to accumulate carbon at significant rates throughout their lifespan. This is due to their extensive root systems and dense wood structure, which contribute to both aboveground and belowground carbon pools. The presence of mature trees is essential for forests to reach their full carbon storage potential. The relationship between large-diameter trees and overall forest biomass suggests that forests cannot reach their ecological carbon storage potential without these mature trees (Mildrexler et al., 2020). Mature forests with large trees provide long-term, stable carbon storage. However, it takes centuries for forests to recover their carbon stocks after disturbances like logging. This emphasizes the value of preserving existing mature trees for immediate and long-term climate mitigation (Mildrexler et al., 2020). The composition of large trees within a forest also influences aboveground biomass (AGB) and carbon stocks. Research has demonstrated a strong correlation between basal area and AGB in various forest ecosystems (Ma et al., 2023). Studies in mature tropical forests have shown that a significant portion of their AGB is stored within large trees, which emphasize their critical role in maintaining forest biomass (Borah et al., 2015). While AGB is influenced by various factors, basal area has been identified as a more significant determinant of AGB compared to tree density across different ecosystems, including agroforestry (Bisht et al., 2022; Ma et al., 2023). In addition to basal area, higher diameters were also found to have a positive relationship with aboveground carbon stock in tropical forests (Khan et al., 2021; Lee et al., 2022), which also support our results where the carbon stock were found to be higher in the location with more individual trees with the diameter of more than 20 cm.
This study underscores the critical need for preserving mature, old-growth trees, particularly within coffee agroforestry systems situated in forested regions, to maximize carbon storage potential. Our findings, coupled with existing literature emphasizing the carbon sequestration capacity of mature trees, suggest that maintaining these trees is crucial for coffee agroforestry to approach the carbon storage levels of secondary tropical forests. Furthermore, diversifying tree species within these agroforestry systems is equally vital. Research demonstrates a strong correlation between species diversity, functional diversity (encompassing wood density, maximum diameter, and height), and aboveground carbon storage in both tropical and temperate forests (Sintayehu et al., 2020a; Teshome et al., 2020a). This enhanced aboveground carbon storage is often attributed to complementarity effects, where the more diverse species will utilize resources more efficiently. While research suggests species richness alone may not correlate with carbon storage in natural ecosystems (Sintayehu et al., 2020a), prioritizing functional diversity, specifically trees with high wood density, large diameters, and significant height, can maximize carbon storage in coffee agroforestry. This approach contributes to increased basal area and aboveground biomass, directly impacting carbon sequestration capacity. Conserving existing old-growth trees, especially native species, is highly recommended, as certain species inherently contribute disproportionately to carbon stocks due to their size and wood density (Ribeiro et al., 2018; Sugiyama et al., 2024). However, agroforestry systems that rely solely on a single shade tree species should be avoided. Research by Obonyo et al. (2023) have shown that diverse, interspecific stands exhibit significantly higher live and dead tree aboveground carbon compared to single-species stands, further emphasizing the importance of species diversity for maximizing carbon storage in coffee agroforestry.
Agroforestry systems have demonstrated significant potential for carbon storage, with several studies indicating that their carbon stocks can rival or even surpass those of secondary forests (Tschora and Cherubini, 2020; Siarudin et al., 2021). Our results are consistent with these findings, particularly in Location 1, where coffee agroforestry maintained relatively high carbon stocks. However, in Locations 2 and 3, where fewer mature trees were present, carbon storage was significantly lower, reinforcing the idea that tree age and structural diversity are critical determinants of carbon sequestration. The extent of the carbon storage potential, however, is contingent upon the vegetation composition within the agroforestry system (Tschora and Cherubini, 2020; Siarudin et al., 2021), as well as regional ecological conditions and site-specific factors such as biological diversity, climate, soil properties, and management practices (Ma et al., 2020; Raju Singh et al., 2020). Despite their merits, agroforestry systems cannot match the carbon sequestration capacity or broader ecosystem services provided by primary forests. Old-growth forests store significantly higher amounts of carbon estimated at 1.6 to 2.3 times more than secondary or managed forests (Keith et al., 2024). Globally, primary tropical forests account for 49–53% of the carbon stored in tropical forests, representing 91–103% of the remaining carbon budget necessary to limit global warming to 1.5°C above pre-industrial levels (Mackey et al., 2020). Hernández Núñez et al. (2021) also confirmed through his studies in Colombia’s Eastern Plains that agroforestry systems in store much less carbon than natural forests. Nonetheless, in regions where primary forests have already experienced encroachment, agroforestry presents a practical approach to optimizing ecosystem services in degraded landscapes. While agroforestry offers a feasible solution in such contexts, the preservation of remaining primary forests is essential to safeguard their unparalleled carbon storage capacity and prevent further encroachment.
The integration of coffee cultivation within protected forests through agroforestry systems represents a pragmatic approach to balancing conservation objectives with the livelihoods of local and indigenous communities. This compromise helps mitigate land-use conflicts by providing economic incentives for these communities to actively engage in sustainable land management practices. A key challenge, however, is ensuring that such interventions do not compromise biodiversity conservation or lead to unintended ecological degradation. Agroforestry systems allow for the coexistence of coffee plants with native tree species, maintaining forest canopy cover and biodiversity while supporting income generation through coffee production. The inclusion of native forest tree species in agroforestry systems not only aligns with conservation goals but also enhances ecosystem services such as carbon sequestration, soil fertility, and water regulation (Salve et al., 2022; Getachew and Mulatu, 2024). By involving local and indigenous people in forest management, this approach leverages traditional ecological knowledge and fosters a sense of stewardship (Akalibey et al., 2024). Moreover, it reduces the likelihood of illegal deforestation and unsustainable agricultural expansion by formalizing the integration of agroforestry practices within protected areas (Nurrochmat et al., 2019; Ordóñez et al., 2023). In Indonesia, the Hutan Desa scheme led to decreased deforestation and poverty alleviation, with outcomes varying across different land-use zones, such as improved community well-being due to improved land tenure security and environmental awareness (Santika et al., 2019). Similarly, community forestry initiatives in Central Lombok have helped reduce illegal logging and encroachment while promoting sustainable forest management practices through ecotourism and agroforestry practices (Nurrochmat et al., 2019). The practice of integrating coffee agroforestry with the social forestry strategy aligns with participatory forest management principles, where communities play a central role in maintaining forest health and biodiversity. It underscores the importance of creating policies that recognize the socio-economic needs of forest-dependent communities while advancing conservation objectives.
However, while our findings highlight the potential of agroforestry for carbon sequestration, it is important to acknowledge the limitations of this study. First, the study focused on aboveground carbon stocks without incorporating belowground carbon storage, which may have led to an underestimation of total ecosystem carbon sequestration. Future research should include soil carbon assessments to provide a more comprehensive understanding of carbon storage in agroforestry systems. Second, our analysis relied on a single measurement period, which does not capture seasonal variations in biomass accumulation and carbon fluxes. Longitudinal studies tracking carbon storage over multiple years would offer deeper insights into the temporal dynamics of carbon sequestration.
6.1 Theoretical and policy implication
This study advances the theoretical understanding of carbon sequestration in coffee agroforestry by demonstrating that tree composition and maturity significantly influence aboveground carbon stocks. While previous research has broadly recognized agroforestry as a climate mitigation strategy, empirical evidence quantifying its effectiveness relative to secondary and primary forests remains limited. This study fills that gap by showing that coffee agroforestry systems with at least 30% mature forest trees (DBH ≥ 20 cm) can achieve carbon storage levels comparable to secondary forests. These findings refine existing agroforestry models by providing a measurable threshold for optimizing carbon sequestration in coffee-based systems. Furthermore, the results highlight the critical role of tree basal area and species composition, supporting the hypothesis that maintaining mature, native forest trees within agroforestry landscapes enhances their ecological function.
From a policy perspective, these findings offer valuable insights for improving Indonesia’s Social Forestry (SF) program and similar community-based forest management initiatives. Current agroforestry guidelines often lack specific criteria for tree retention, which can lead to suboptimal carbon sequestration outcomes. This study suggests that policymakers should integrate minimum thresholds for mature tree composition into SF regulations to ensure that coffee agroforestry systems contribute meaningfully to climate mitigation goals. Additionally, the research underscores the need to incorporate carbon stock assessments into agroforestry-based payment for ecosystem services (PES) programs, allowing smallholder farmers to benefit from carbon financing mechanisms such as REDD+. Besides, this study also strengthens the case for land-sharing approaches by demonstrating that well-managed coffee agroforestry systems enhance carbon storage while sustaining farmer livelihoods. These insights are particularly relevant for integrating forest conservation policies with rural development objectives in tropical landscapes.
7 Conclusion
This study highlights the critical importance of integrating sustainable agroforestry practices into Arabica coffee cultivation to enhance carbon sequestration and maintain ecological functions in forested landscapes. The findings confirm that agroforestry systems incorporating a significant proportion of mature, large-diameter trees, particularly native species, exhibit higher carbon storage potential. While such systems may approach the carbon storage levels of secondary forests, they fall short compared to primary forests, which remain irreplaceable reservoirs of carbon and biodiversity. Therefore, preserving mature forest trees and promoting functional diversity within coffee agroforestry systems are essential strategies for achieving sustainability. In regions like the Gayo Highlands, where forest encroachment for coffee cultivation is prevalent, social forestry programs provide a promising framework for balancing production with conservation. Encouraging the use of diverse tree species with high wood density, basal area, and substantial growth potential can enhance carbon storage while sustaining coffee yields. However, reliance on monoculture systems or a single shade tree species should be avoided, as diverse tree compositions significantly improve ecosystem resilience and carbon sequestration. Ultimately, while coffee agroforestry systems offer a practical solution for mitigating the adverse effects of deforestation and climate change, their implementation should complement efforts to preserve intact primary forests. Protecting these irreplaceable ecosystems is paramount, not only for their unparalleled carbon storage capacity, but also for maintaining critical ecosystem services essential for combating global warming and ensuring long-term agricultural sustainability.
Data availability statement
The datasets presented in this article are not readily available because the sharing of the raw datasets has to be consulted with the Indonesian Government due to some data are generated inside the protected forests. It is possible to share the raw data under if it is urgent, by following the permit procedure. Requests to access the datasets should be directed to ZHVycmFoQHVzay5hYy5pZA==.
Author contributions
AA: Conceptualization, Funding acquisition, Methodology, Writing – original draft. DH: Conceptualization, Writing – original draft. AM: Conceptualization, Methodology, Writing – review & editing. AS: Writing – review & editing. MJ: Writing – review & editing. AB: Writing – review & editing. MM: Writing – review & editing. YA: Writing – review & editing. AH: Writing – review & editing.
Funding
The author(s) declare that financial support was received for the research and/or publication of this article. This research was funded by Indonesian Ministry of Education, Culture, Research, and Technology through Program Dana Pananan Kedaireka Batch 3 under 763/UN11.2.1/PG.01.03/SPK/DRTPM/2024–July 2024.
Acknowledgments
The author would like to thank the reviewers for their insightful comments and constructive suggestions.
Conflict of interest
The authors declare that the research was conducted in the absence of any commercial or financial relationships that could be construed as a potential conflict of interest.
Generative AI statement
The authors declare that Gen AI was used in the creation of this manuscript. Generative AI was used to edit the text to fix grammar and suggest better terms to fit the narrative context.
Publisher’s note
All claims expressed in this article are solely those of the authors and do not necessarily represent those of their affiliated organizations, or those of the publisher, the editors and the reviewers. Any product that may be evaluated in this article, or claim that may be made by its manufacturer, is not guaranteed or endorsed by the publisher.
References
Ahmed, S., Brinkley, S., Smith, E., Sela, A., Theisen, M., Thibodeau, C., et al. (2021). Climate change and coffee quality: systematic review on the effects of environmental and management Variation on secondary metabolites and sensory attributes of Coffea arabica and Coffea canephora. Front. Plant Sci. 12:708013. doi: 10.3389/fpls.2021.708013
Akalibey, S., Hlaváčková, P., Schneider, J., Fialová, J., Darkwah, S., and Ahenkan, A. (2024). Integrating indigenous knowledge and culture in sustainable forest management via global environmental policies. J. For. Sci. 70, 265–280. doi: 10.17221/20/2024-JFS
Ango, T. G., Hylander, K., and Börjeson, L. (2020). Processes of Forest cover change since 1958 in the coffee-producing areas of Southwest Ethiopia. Land 9:278. doi: 10.3390/land9080278
Anhar, A., Abubakar, Y., Widayat, H. P., Muslih, A. M., and Baihaqi, A. (2021a). Altitude, shading, and management intensity effect on Arabica coffee yields in Aceh, Indonesia. Open Agric. 6, 254–262. doi: 10.1515/opag-2021-0220
Anhar, A., Rasyid, U. H. A., Muslih, A. M., Baihaqi, A., and Abubakar, Y. (2021b). Sustainable Arabica coffee development strategies in Aceh, Indonesia. IOP Conf. Series Earth Environ. Sci. 667:012106. doi: 10.1088/1755-1315/667/1/012106
Azis, A., and Irjayanti, M. (2023). How does the coffee supply chain work in Indonesia. J. Optimiz. Ind. Eng. 16, 87–97. doi: 10.22094/joie.2023.1986337.2068
Badan Standardisasi Nasional (BSN). (2011). Measurement and calculation of carbon stock: field measurement for forest carbon stock estimation (ground based forest carbon accounting). Jakarta: National Standardization Agency of Indonesia.
Bera, D., Chatterjee, N. D., Dinda, S., Ghosh, S., Dhiman, V., Bashir, B., et al. (2024). Assessment of carbon stock and sequestration dynamics in response to land use and land cover changes in a tropical landscape. Land 13:1689. doi: 10.3390/land13101689
Berhanu, Y., Dalle, G., Sintayehu, D. W., Kelboro, G., and Nigussie, A. (2023). Land use/land cover change-induced greenhouse gas emissions in the global biosphere reserve of tropical Afromontane forest. Sci. Afr. 21:e01811. doi: 10.1016/j.sciaf.2023.e01811
Bilen, C., el Chami, D., Mereu, V., Trabucco, A., Marras, S., and Spano, D. (2022). A systematic review on the impacts of climate change on coffee Agrosystems. Plan. Theory 12:102. doi: 10.3390/plants12010102
Bisht, S., Bargali, S. S., Bargali, K., Rawat, G. S., Rawat, Y. S., and Fartyal, A. (2022). Influence of anthropogenic activities on Forest carbon stocks—A case study from Gori Valley, Western Himalaya. Sustain. For. 14:16918. doi: 10.3390/su142416918
Borah, M., das, D., Kalita, J., Deka Boruah, H. P., Phukan, B., and Neog, B. (2015). Tree species composition, biomass and carbon stocks in two tropical forest of Assam. Biomass Bioenergy 78, 25–35. doi: 10.1016/j.biombioe.2015.04.007
Cassamo, C. T., Draper, D., Romeiras, M. M., Marques, I., Chiulele, R., Rodrigues, M., et al. (2023). Impact of climate changes in the suitable areas for Coffea arabica L. production in Mozambique: agroforestry as an alternative management system to strengthen crop sustainability. Agric. Ecosyst. Environ. 346:108341. doi: 10.1016/j.agee.2022.108341
Chemura, A., Mudereri, B. T., Yalew, A. W., and Gornott, C. (2021). Climate change and specialty coffee potential in Ethiopia. Sci. Rep. 11:8097. doi: 10.1038/s41598-021-87647-4
De Leijster, V., Santos, M. J., Wassen, M. W., Camargo-Garcia, J. C., Fernández, I. L., Verkuil, L., et al. (2021). Ecosystem services trajectories in coffee agroforestry in Colombia over 40 years. Ecosyst. Serv. 48:101246. doi: 10.1016/j.ecoser.2021.101246
Djamali, R. A., Cahyaningrum, D. T., and Putra, D. E. (2022). Determinants and strategies of social forestry development for integrated coffee agribusiness in the foot of mount Argopuro area, Jember regency. IOP Conf. Series 980:012053. doi: 10.1088/1755-1315/980/1/012053
Fardinatri, I. D., Condro, A., Hayati, D., and Anhar, A. (2024). Environmental dynamics in the Sumatran coffee landscapes: opportunities and challenges through spatial perspectives. J. Pengelolaan Sumberdaya Alam Lingkungan 14:438. doi: 10.29244/jpsl.14.3.438
Getachew, G., and Mulatu, A. (2024). Agroforestry contribution to native Woody species conservation, carbon sequestration, and livelihood benefits in Ethiopia: A systematic review. J. Landsc. Ecol. 17, 101–129. doi: 10.2478/jlecol-2024-0012
Gomes, L. C., Bianchi, F. J. J. A., Cardoso, I. M., Fernandes, R. B. A., Filho, E. I. F., and Schulte, R. P. O. (2020). Agroforestry systems can mitigate the impacts of climate change on coffee production: A spatially explicit assessment in Brazil. Agric. Ecosyst. Environ. 294:106858. doi: 10.1016/j.agee.2020.106858
Haggar, J., Casanoves, F., Cerda, R., Cerretelli, S., Gonzalez-Mollinedo, S., Lanza, G., et al. (2021). Shade and agronomic intensification in coffee agroforestry systems: trade-off or synergy? Front. Sustain. Food Syst. 5:645958. doi: 10.3389/fsufs.2021.645958
Hernández Núñez, H. E., Andrade, H. J., Suárez Salazar, J. C., Sánchez, J. R., Gutiérrez, D. R., Gutiérrez García, G. A., et al. (2021). Almacenamiento de carbono en sistemas agroforestales en los Llanos Orientales de Colombia. Rev. Biol. Trop. 69:959. doi: 10.15517/rbt.v69i1.42959
Irvianty, S. Z., and Defira, C. N. (2023). Biomassa, Potensi Cadangan Karbon dan Serapan Karbon Pada Hutan Kota. JST 12:626. doi: 10.23887/jstundiksha.v12i2.52626
Jezeer, R. E., Santos, M. J., Verweij, P. A., Boot, R. G. A., and Clough, Y. (2019). Benefits for multiple ecosystem services in Peruvian coffee agroforestry systems without reducing yield. Ecosyst. Serv. 40:101033. doi: 10.1016/j.ecoser.2019.101033
Justine, N., Tumwebaze, B. S., Ritah, K., and Nabanoga, G. (2019). “Aboveground species diversity and carbon stocks in smallholder coffee agroforestry in the highlands of Uganda” in Agriculture and Ecosystem Resilience in Sub Saharan Africa. Climate Change Management. eds. Y. Bamutaze, S. Kyamanywa, B. Singh, G. Nabanoga, and R. Lal (Cham: Springer), 403–415.
Kath, J., Mittahalli Byrareddy, V., Mushtaq, S., Craparo, A., and Porcel, M. (2021). Temperature and rainfall impacts on robusta coffee bean characteristics. Clim. Risk Manag. 32:100281. doi: 10.1016/j.crm.2021.100281
Kebebew, Z., and Ozanne, C. (2024). Diversity, preference, and conservation priority of woody plant species in coffee agroforestry system in Southwest Ethiopia. Front. For. Global Change 7:1269141. doi: 10.3389/ffgc.2024.1269141
Keith, H., Kun, Z., Hugh, S., Svoboda, M., Mikoláš, M., Adam, D., et al. (2024). Carbon carrying capacity in primary forests shows potential for mitigation achieving the European green Deal 2030 target. Commun. Earth Environ. 5:256. doi: 10.1038/s43247-024-01416-5
Khan, I., Hayat, U., Mujahid, A., Majid, A., Badshah, T., Chaudhary, A., et al. (2021). Evaluation of growing stock, biomass, and soil carbons and their association with A diameter: A case study from A planted Chir pine (Pinus roxburghii) Forest. Appl. Ecol. Environ. Res. 19, 1457–1472. doi: 10.15666/aeer/1902_14571472
Koutouleas, A., Sarzynski, T., Bertrand, B., Bordeaux, M., Bosselmann, A. S., Campa, C., et al. (2022). Shade effects on yield across different Coffea arabica cultivars — how much is too much? A meta-analysis. Agron. Sustain. Dev. 42:55. doi: 10.1007/s13593-022-00788-2
Koutouleas, A., Sarzynski, T., Bordeaux, M., Bosselmann, A. S., Campa, C., Etienne, H., et al. (2022). Shaded-coffee: A nature-based strategy for coffee production under climate change? A Review. Front. Sustain. Food Syst. 6:877476. doi: 10.3389/fsufs.2022.877476
Lee, C. B., Ali, A., Yuan, Z., and Lutz, J. A. (2022) Large-diameter trees control forest structure and function in successional temperate forests. Biogeosciences Discussions.
Ma, Z., Chen, H. Y. H., Bork, E. W., Carlyle, C. N., and Chang, S. X. (2020). Carbon accumulation in agroforestry systems is affected by tree species diversity, age and regional climate: A global meta-analysis. Global Ecol. Biogeogr. 29, 1817–1828. doi: 10.1111/geb.13145
Ma, Y., Eziz, A., Halik, Ü., Abliz, A., and Kurban, A. (2023). Precipitation and temperature influence the relationship between stand structural characteristics and aboveground biomass of forests—A Meta-analysis. Forests 14:896. doi: 10.3390/f14050896
Mackey, B., Kormos, C. F., Keith, H., Moomaw, W. R., Houghton, R. A., Mittermeier, R. A., et al. (2020). Understanding the importance of primary tropical forest protection as a mitigation strategy. Mitig. Adapt. Strateg. Glob. Chang. 25, 763–787. doi: 10.1007/s11027-019-09891-4
Manuri, S., Brack, C., Fatmi, N., Teddy, R., Shema, M. A., Helmut, D., et al. (2016). Improve allometric equations for tree aboveground biomass estimation in tropical dioterocarp forest of Kalimantan, Indonesia. For. Ecosyst. 3:28.
Mildrexler, D. J., Berner, L. T., Law, B. E., Birdsey, R. A., and Moomaw, W. R. (2020). Large trees dominate carbon storage in forests east of the Cascade crest in the United States Pacific northwest. Front. For. Glob. Change 3:594274. doi: 10.3389/ffgc.2020.594274
Moreira, S. L. S., Pires, C. V., Marcatti, G. E., Santos, R. H. S., Imbuzeiro, H. M. A., and Fernandes, R. B. A. (2018). Intercropping of coffee with the palm tree, macauba, can mitigate climate change effects. Agric. For. Meteorol. 256-257, 379–390. doi: 10.1016/j.agrformet.2018.03.026
Navidad Murrieta, D. L., Marceleño Flores, S. M. L., Nájera González, A., Nájera González, O., and Ramírez Silva, J. P. (2023). Effects of land cover and land use change on Nature’s contributions to people of the shade-grown coffee agroecosystem: an analysis of Cumbres de Huicicila, Nayarit, Mexico. Conservation 3, 426–443. doi: 10.3390/conservation3030029
Nurrochmat, D. R., Massijaya, M. Y., Jaya, I. N. S., Abdulah, L., Ekayani, M., Astuti, E. W., et al. (2019). Promoting community forestry to reduce deforestation surrounding Gunung Rinjani National Park in Central Lombok, Indonesia. IOP Conf. Series 285:012014. doi: 10.1088/1755-1315/285/1/012014
Obonyo, O. A., Agevi, H., and Tsingalia, M. H. (2023). Above-ground carbon stocks and its functional relationship with tree species diversity: the case of Kakamega and north Nandi forests, Kenya. Sci. Rep. 13:20921. doi: 10.1038/s41598-023-47871-6
Octavia, D., Suharti, S., Dharmawan, I. W. S., Nugroho, H. Y. S. H., Supriyanto, B., Rohadi, D., et al. (2022). Mainstreaming smart agroforestry for social forestry implementation to support sustainable development goals in Indonesia: a review. Sustain. For. 14:9313. doi: 10.3390/su14159313
Ordóñez, P. J., Baylis, K., and Ramírez, M. I. (2023). Land cover change effects from community forest management in Michoacán, Mexico. Environ. Res. Lett. 18:065008. doi: 10.1088/1748-9326/accdef
Ortiz-Ceballos, G. C., Vargas-Mendoza, M., Ortiz-Ceballos, A. I., Mendoza Briseño, M., and Ortiz-Hernández, G. (2020). Aboveground carbon storage in coffee agroecosystems: the case of the central region of the state of Veracruz in Mexico. Agronomy 10:382. doi: 10.3390/agronomy10030382
Raju Singh, N., Kumar, D., Rao, K. K., and Bhatt, B. P. (2020). “Agroforestry: soil organic carbon and its carbon sequestration potential” in Climate change and agroforestry systems. ed. A. Raj (Florida: Apple Academic Press), 119–142.
Ramadhillah, B., and Masjud, Y. I. (2024). Climate change impacts on coffee production in Indonesia: A review. J. Crit. Ecol. 1, 1–7. doi: 10.61511/jcreco.v1i1.645
Ribeiro, S. C., Jacovine, L. A. G., Torres, C. M. M. E., and Souza, A. L. (2018). Influence of interspecific VARIATION on tree carbon stock of A BRAZILIAN CERRADO. Revista Árvore 41:6. doi: 10.1590/1806-90882017000500006
Salve, A., Tiwari, C., and Baghele, L. (2022). Impact of agroforestry systems: a review. Asian J. Microbiol. Biotechnol. Environ. Sci. 24, 214–223. doi: 10.53550/AJMBES.2022.v24i02.002
Santika, T., Wilson, K. A., Budiharta, S., Kusworo, A., Meijaard, E., and Law, E. A. (2019). Heterogeneous impacts of community forestry on forest conservation and poverty alleviation: Evidence from Indonesia. People Nat. 1, 204–219.
Samek, J. H., Anhar, A., Maimunah, S., and Skole, D. (2022). Measuring forest ecosystem services in Aceh Province for inclusion to local forest resource management plans. APN Sci. Bull. 12, 90–101. doi: 10.30852/sb.2022.1910
Sanudin, F., Fauziyah, E., and Sundawati, L. (2024). Management of coffee agroforestry systems: lessons learned from a social forestry program in West Java, Indonesia. IOP Conf. Series 1315:012002. doi: 10.1088/1755-1315/1315/1/012002
Schroth, G., Läderach, P., Blackburn Cuero, D. S., Neilson, J., and Bunn, C. (2015). Winner or loser of climate change? A modeling study of current and future climatic suitability of Arabica coffee in Indonesia. Reg. Environ. Chang. 15, 1473–1482. doi: 10.1007/s10113-014-0713-x
Siarudin, M., Rahman, S. A., Artati, Y., Indrajaya, Y., Narulita, S., Ardha, M. J., et al. (2021). Carbon sequestration potential of agroforestry Systems in Degraded Landscapes in West Java, Indonesia. Forests 12:714. doi: 10.3390/f12060714
Sintayehu, D. W., Belayneh, A., and Dechassa, N. (2020). Aboveground carbon stock is related to land cover and woody species diversity in tropical ecosystems of eastern Ethiopia. Ecol. Process. 9:37. doi: 10.1186/s13717-020-00237-6
Sugiyama, A., Game, E. T., and Wright, S. J. (2024). Planting exceptional tropical tree species to increase long-term carbon storage in assisted secondary succession. J. Appl. Ecol. 61, 7–12. doi: 10.1111/1365-2664.14533
Teshome, M., Asfaw, Z., and Mohammed, M. (2020). Diversity influences tree aboveground carbon stocks through functional diversity and functional dominance in the dry Evergreen Afromontane Forest of Hararghe Highland, Southeast Ethiopia. Res. Squ. Preprint doi: 10.21203/rs.3.rs-53977/v1
Tschora, H., and Cherubini, F. (2020). Co-benefits and trade-offs of agroforestry for climate change mitigation and other sustainability goals in West Africa. Global Ecol. Conserv. 22:e00919. doi: 10.1016/j.gecco.2020.e00919
Ulya, N. A., Harianja, A. H., Sayekti, A. L., Yulianti, A., Djaenudin, D., Martin, E., et al. (2023). Coffee agroforestry as an alternative to the implementation of green economy practices in Indonesia: A systematic review. AIMS Agric. Food 8, 762–788. doi: 10.3934/agrfood.2023041
Keywords: carbon dynamic, carbon storage, tropical agroforestry, tree species richness, forest-based coffee cultivation
Citation: Anhar A, Hayati D, Muslih AM, Siregar AW, Jamilah M, Baihaqi A, Muzaifa M, Abubakar Y and Hanan A (2025) Comparing aboveground carbon stocks in coffee agroforestry and secondary and primary forests in Gayo Highlands, Indonesia. Front. For. Glob. Change. 8:1541302. doi: 10.3389/ffgc.2025.1541302
Edited by:
Rahim Maleknia, Lorestan University, IranReviewed by:
Donya Rahmani Hesar, Tarbiat Modares University, IranJules Christian Zekeng, University of Douala, Cameroon
Copyright © 2025 Anhar, Hayati, Muslih, Siregar, Jamilah, Baihaqi, Muzaifa, Abubakar and Hanan. This is an open-access article distributed under the terms of the Creative Commons Attribution License (CC BY). The use, distribution or reproduction in other forums is permitted, provided the original author(s) and the copyright owner(s) are credited and that the original publication in this journal is cited, in accordance with accepted academic practice. No use, distribution or reproduction is permitted which does not comply with these terms.
*Correspondence: Ashabul Anhar, YXNoYWJ1bC5hbmhhckB1c2suYWMuaWQ=