- 1Department of Respiratory, Children's Hospital of Chongqing Medical University, National Clinical Research Center for Child Health and Disorders, Ministry of Education Key Laboratory of Child Development and Disorders, Chongqing, China
- 2Department of Respiratory, Chongqing Key Laboratory of Child Rare Diseases in Infection and Immunity, Chongqing, China
Human adenovirus is a significant viral pathogen causing lower respiratory tract infections in children, prone to developing into severe pneumonia and systemic inflammation with a high mortality rate, especially in immunocompromised children, drawing widespread attention worldwide. Sepsis, a life-threatening organ dysfunction caused by a dysregulated inflammatory response to infection, has historically been focused on bacterial origins. However, nearly all viruses can cause sepsis, which is often underestimated in clinical settings. In recent years, severe infections and even sepsis caused by adenovirus have shown a trend of periodic outbreaks. Early diagnosis of adenovirus-induced sepsis can not only prevent the overuse of broad-spectrum antibiotics but also ensure that patients receive timely and appropriate antiviral treatment. This article aims to provide a comprehensive review of the epidemiology, pathogenesis, diagnostic methods, and recent advances in treatment strategies for viral sepsis caused by adenovirus.
1 Introduction
Sepsis is a syndrome characterized by abnormal physiological and pathological reactions caused by infection (bacteria, viruses or other pathogens) and is one of the major causes of death in children (1, 2). There are many cases of viral sepsis in patients with negative blood cultures (3–6), However, in actual clinical diagnosis and treatment, these viral causes are often underestimated, resulting in an unnecessary increase in the use of broad-spectrum antibiotics and missed opportunities to use antiviral treatment plans, which increases the disease burden (7). Human adenovirus (HAdV) is an important viral pathogen that causes lower respiratory tract infections and severe pneumonia in children under 5 years old, accounting for 3.5%–11% of community-acquired pneumonia in children (8, 9), The severe infection rate is as high as 37% (10, 11), and the mortality rate can be as high as 50% (12), There are a large number of cases of viral sepsis caused by adenovirus in severe infections. Some studies have shown that early use of antiviral strategies and neutralizing antibodies can improve the prognosis of severe adenovirus infection (13–15), therefore, this article reviews the latest research progress in the definition, epidemiology, pathogenic mechanism, diagnosis and treatment of adenovirus sepsis, in order to provide a certain reference for early clinical decision-making for adenovirus sepsis.
1.1 Definition
In 2015, the third edition of the international consensus on sepsis defined sepsis as a life-threatening organ dysfunction caused by a dysregulated host response to infection. Unlike the first and second editions of the sepsis definition (16, 17), the third edition no longer uses the systemic inflammatory response syndrome (SIRS) to define sepsis, but it still plays an important role in the early identification of severe infection (18). Today, domestic and international research on the etiology of sepsis mainly focuses on bacterial infection. Studies have shown that about 1/3–2/3 of sepsis patients have negative blood cultures, among which viral sepsis cannot be excluded (3–6). However, there is currently no clear definition of viral sepsis. Since the latest definition of sepsis does not specifically limit the pathogen, viral sepsis is also applicable to this definition (1, 7). The most common site of infection in sepsis patients is the respiratory tract (64%–68%) (19). Severe infections caused by HAdV are often caused by lower respiratory tract infections (12), and there are manifestations of persistent disseminated viremia. The increase in serum viral load and persistent viremia are risk factors for severe prognosis and death in children (13, 20). Therefore, combined with the diagnostic basis of adenovirus infection, adenovirus sepsis is considered to be defined as cases in which adenovirus is confirmed as a clear pathogen through pathogen detection [antigen detection, adenovirus polymerase chain reaction (PCR) detection], adenoviremia exists, and the international consensus definition of sepsis is met. However, it is still very challenging to clarify the causal relationship between HAdV infection and sepsis, because a positive result of HAdV alone is not sufficient to diagnose adenovirus sepsis. It is also necessary to consider whether there is a concurrent infection and whether a secondary infection has occurred. Clinicians need to make a comprehensive judgment based on their clinical characteristics. The causal relationship between HAdV infection and sepsis needs to be further and better determined in other prospective cohort studies.
1.2 Epidemiology
Currently, the diagnosis of viral sepsis is often neglected, and the incidence of viral sepsis is often underestimated. However, a recent study included patients diagnosed with viral pneumonia and found through retrospective analysis that 61% of patients had viral sepsis, and HAdV is one of the main pathogens causing viral sepsis (21). According to different biological characteristics, HAdV is divided into seven subgenera from A to G. A total of 116 genotypes have been found, and they are still being updated (http://hadvwg.gmu.edu/). Different types of HAdV have different tissue tropisms. Their pathogenicity, epidemic trends and other characteristics are also different. HAdV infection in patients with normal immune function is usually self-limiting, such as infections of the eyes, upper respiratory tract, and gastrointestinal tract (12). However, HAdV is prone to cause lower respiratory tract infections in children (the proportion is 3.5%–11.0%) (8, 9), and is prone to develop into severe cases (the severe cases rate is about 37%) (10, 11). Severe patients may have respiratory failure, intracranial infection, and even death, including children with adenovirus-induced sepsis. Adenovirus types 3, 7, 14, 21, and 55 in the B subgenus, types 1, 2, 5, and 6 in the C subgenus, and type 4 in the E subgenus are commonly detected in respiratory infections in children (12, 22). In China, HAdV-3 (32.73%) and HAdV-7 (27.48%) are the main detected types (23). In Asia, HAdV-1–3 and HAdV-7 are the main detected types (24, 25). In Europe, HAdV-1–3 (45.6%) are the main detected types (26). In North America, represented by the United States, adenovirus type 3 (39.7%) is the most commonly detected type among civilians (including children and adults), and adenovirus types 1–5 and 7 are the main detected types (89.7%) (27, 28). In many countries in South America, HAdV-7 is the predominant strain associated with respiratory infections requiring hospitalization (29). In African countries, HAdV1- is the predominant adenovirus detection type (30, 31). Worldwide, HAdV-1–HAdV-7 are the most common types, while HAdV-4 and HAdV-5 have the highest serum positivity rates (32) (Figure 1). However, HAdV-7, HAdV-55, HAdV-4, and HAdV-14 often cause outbreaks in relatively closed environments such as military camps and schools (33). Types 7, 55, and 4 are more likely to be combined with severe pneumonia, leading to viral sepsis and endangering the lives of children (34–37). This is especially true for children under 2 years old, those after organ transplantation, and those with congenital immunodeficiency (38). In adults and children with normal immune function, there have also been reports of severe infections and even death caused by adenovirus (13, 39).
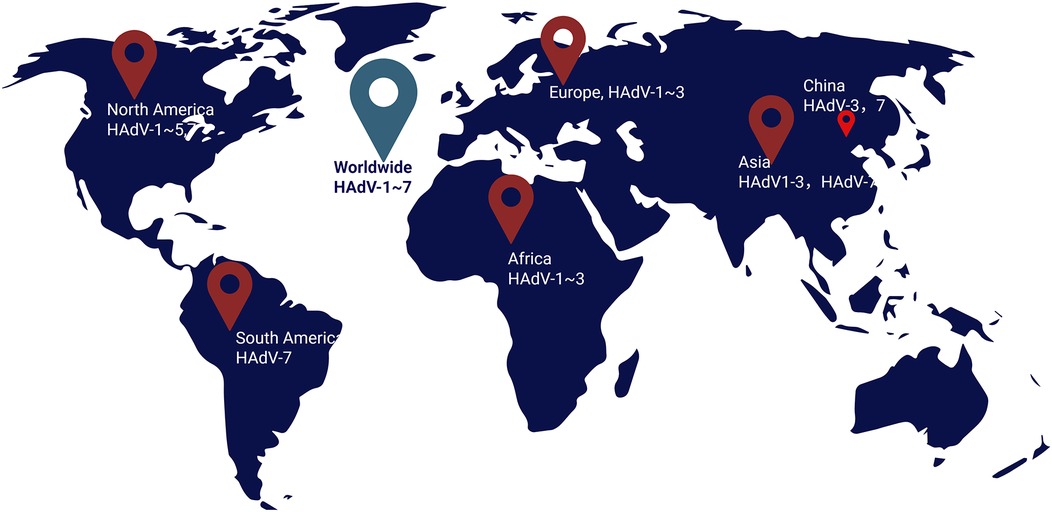
Figure 1. The worldwide map of molecular epidemiology trends of adenovirus (the predominant adenovirues genotype worldwide were marked).
With the easy availability of rapid viral diagnostic testing, HAdV infections could be identified more often. After comprehensively analyzing relevant literature, Lin, G.L. et al. believed that disseminated disease and adenovirus sepsis in children account for 2% of all adenovirus infections, with a mortality rate of up to 55%. The main risk factors for the development of sepsis are immunosuppression (especially allogeneic hematopoietic stem cell transplantation), young children (less than 18 months old), and infection with highly virulent adenovirus serotypes (such as HAdV-7) (7). A recent retrospective cohort study found that among all patients hospitalized for viral pneumonia with sepsis, the detection rate of adenovirus was 8% of all viral sepsis caused by non-influenza viruses (21). A multicenter study on the risk factors for death from pediatric sepsis found that serum HAdV-DNA-positive adenovirus sepsis accounted for approximately 10% of viral sepsis, and after adjusting for factors such as age, child mortality risk score, previous health status, and immunocompromised status at the onset of sepsis, the adjusted odds ratio (AOR) of HAdV was as high as 3.50 (P = 0.006), indicating that adenovirus viremia is closely associated with increased mortality (40). In a retrospective study of 415 immunocompetent children hospitalized for adenovirus infection, a mortality rate of 15% was observed (41). In a recent cohort study of patients hospitalized for severe adenovirus pneumonia and transferred to the ICU, a mortality rate of 31.1% was observed (13). Among these deaths, a large number of cases met the diagnosis of adenovirus sepsis as described above. However, more detailed epidemiological data on adenovirus sepsis urgently need to be supported by more systematic multicenter epidemiological studies.
1.3 Pathogenic mechanism
The pathogenesis of HAdV viral sepsis is limited, but similar to bacterial sepsis, its core is life-threatening organ dysfunction caused by the dysregulated host response to infection, which mainly involves systemic immune dysfunction, combined destruction of the epithelial-endothelial barrier, and a series of multi-organ functional damage caused by infection, including the respiratory system, cardiovascular system, nervous system, blood system, endocrine system, etc. (19) (Figure 2).
1.3.1 Systemic immune dysfunction
HAdV is a non-enveloped icosahedral double-stranded DNA virus with a viral capsid protein surrounding the viral core. The virus core is composed of genomic DNA and core proteins pVII, pV, Mu (pX), pIVa2, terminal protein (TP) and protease encoded by AdV. The adenovirus core protein plays an important role in degranulation, genome nuclear transfer, progeny virus encapsulation and release (42). Capsid protein is divided into major capsid protein and minor capsid protein. The major capsid protein is composed of hexon, penton base and fiber, and the minor capsid protein is composed of pIX, pIIIa, pVI and pVIII. These proteins play a very important role in stabilizing the protein-protein interaction structure of virus particles (42, 43).
The immune dysfunction of HAdV viral sepsis is mainly manifested in the inability of the host's innate and adaptive immune response to HAdV to restore homeostasis, ultimately leading to a pathological syndrome characterized by persistent excessive inflammation and immunosuppression (44).
1.3.1.1 Innate immunity
Like influenza virus and new coronavirus, HAdV first activates innate immunity after infecting the host. This initial host perception of pathogens is mediated by pattern recognition receptors (PRRs), including toll-like receptors (TLRs), retinoic acid-induced gene-1-like receptors (RLRs), nod-like receptors (NLRs) and c-type lectin receptors (45, 46). After HAdV binds to adhesion receptors through the fiber knob, it mediates endocytosis and signaling cascades through the binding of Penton to integrin receptors (such as αvβ5), and then the virus degranulates to the cytoplasm and transports to the vicinity of the nuclear pore. After further degranulation, the genetic material is transported to the nucleus (47), and pathogen-associated molecular patterns (PAMPs) are expressed. PRRs activate innate immunity by recognizing PAMPs. Normally, the innate immune system clears viruses by promoting the release of cytokines and chemokines (tumor necrosis factor, TNF, interleukin, IL-1β, IL-12 and IL-18), the recruitment of phagocytes, and the local activation of the complement and coagulation systems (44, 46). For example, Penton binding to integrins can activate phosphatidylinositol-3-hydroxykinase (PI3K) signaling, which can increase viral intracellular transport and trigger the production of the proinflammatory cytokine TNFα (48, 49). TLR9 can detect adenovirus in the cytoplasm, conduct signal transduction through the MYD88-dependent pathway, activate transcription factor NF-κB and other pathways, leading to the production of proinflammatory cytokines IL-6 and IL-8, and the release of IFNα/β (47, 50, 51). Activation of the NF-κB pathway can also promote the maturation of IL-1β and IL-18 and the release of necrosis factor and proinflammatory factor HMGB1 by inducing NOD-like receptor thermoprotein domain protein 3 (NLRP3) inflammasome activation (47, 52). In addition, adenovirus binding to membrane auxiliary factor CD46 can also induce complement activation, thereby inhibiting the release of adenovirus fiber and the exposure of protein VI (53).
1.3.1.2 Adaptive immunity
The major capsid proteins of adenovirus (hexon, penton, and fiber) are highly immunogenic and can stimulate B cells to produce specific IgG antibodies, with the hexon protein being the primary target of neutralizing antibodies (54, 55). HAdV infection can also activate a CD4+ T cell immune response. T cell activation depends on MHC molecules presenting viral peptides: the MHC class I pathway degrades antigens via the proteasome to activate CD8+ T cells, while the MHC class II pathway degrades antigens via lysosomes to activate CD4+ T cells and promote B cell activation (56, 57). This T cell response exhibits cross-protective effects (56).
Autophagy degrades intracellular components (including pathogens) by forming autophagosomes and facilitates antigen presentation through the MHC class II pathway (58, 59). Additionally, the TRIM21 protein can bind to viral DNA-antibody complexes and degrade capsid proteins via the proteasome, thereby inhibiting viral replication (50, 60). Antibody-mediated endocytosis can also lead to viral uncoating, promoting viral clearance (60).
1.3.1.3 Immune evasion
HAdV employs multiple immune evasion strategies to protect infected cells. The E3-encoded proteins disrupt MHC presentation, preventing CTL recognition (61). Specifically, E3-gp19K binds MHC I in the ER domain, blocking its transport to the cell surface and reducing CD8+ T cell and NK cell-mediated killing (62). Other E3 proteins (E3–14.7K, E3–10.4K, E3–14.5K, E3–6.7K) inhibit apoptosis by downregulating death receptors and suppressing NF-κB. E1B-19K and E1B-55K counteract p53-mediated apoptosis (63), while E1B-55K also suppresses IFN-induced gene expression and, with E4, inactivates the MRN complex to enhance viral replication (50). Early-expressed E1A blocks IFN-stimulated gene (ISG) transcription, further promoting viral persistence (64, 65). These mechanisms allow HAdV to evade immune clearance, sustain low-level viral release, and trigger chronic inflammation, contributing to long-term pulmonary complications.
1.3.1.4 Excessive inflammation and immunosuppression
When the virus cannot be cleared by the host immune system, the homeostasis of the immune system will be broken, leading to excessive inflammation and immunosuppression, resulting in sepsis (66).
In the stage of excessive inflammation, HAdV viral sepsis is similar to sepsis caused by other reasons. It activates many PRRs through the release of injury-associated molecular patterns (IAMPs) by inflammatory cells such as leukocytes, macrophages, endothelial cells, and platelets. These PRRs in turn recognize PAMPs, further mediating tissue damage and leading to a vicious cycle (67, 68). At the same time, the excessive inflammation stage will also activate the coagulation system, complement system, neutrophils and vascular endothelium, causing organ damage and dysfunction (19, 69, 70).
In the immunosuppressive stage, innate immunity and adaptive immunity play a role simultaneously. With the increase of apoptosis of T cells, B cells and dendritic cells. T cells are exhausted, regulatory T cells and myeloid-derived suppressor cells (MDSC) are expanded (71, 72). In addition, in pathological conditions such as tumors, infectious diseases and autoimmune diseases, MDSCs can be rapidly accumulated and activated by IAMPs or PAMPs, and MDSCs are immature bone marrow cells that can hinder T cell function, thereby inhibiting immune response, inhibiting viral clearance, promoting viral persistence, and aggravating tissue damage (72).
1.3.2 Combined destruction of the epithelial-endothelial barrier and multi-organ functional damage
The combined destruction of the epithelial-endothelial barrier caused by HAdV infection is also an important pathophysiological process for the occurrence of multi-organ function damage in HAdV sepsis (66). The inflammatory cytokine storm caused by persistent excessive inflammation in the early stage of infection is the main cause of endothelial dysfunction, loss of endothelial integrity, and multi-organ edema, leakage, and failure (19).
Besides, HAdVs infection can also directly damage host cells through various mechanisms. Different adenovirus serotypes enter cells by binding their fiber proteins to specific host cell surface receptors (e.g., CAR, CD46, DSG-2, etc.), triggering signal transduction and endocytosis. Following internalization, the virus undergoes uncoating - a process facilitated by adenovirus protease (AVP) that dissolves the viral protein capsid. The viral particles are then transported along microtubules to nuclear pores where the genome is released to initiate transcription. Subsequently, the virus penetrates the endosomal membrane and escapes into the cytosol, ultimately inducing cell death (73). When HAdV infects alveolar epithelial cells, inducing ferroptosis of alveolar epithelial cells, HAdVs directly damages the alveolar epithelial barrier, releases inflammatory mediators such as IL-6, IL-8, IL-18, and TNF-α, and mediates the increase of reactive oxygen species (ROS) and lipid peroxides (74). These inflammatory mediators and ROS can damage the endothelial cell. The endothelial cell damage can also be led by direct infection of HAdV through autophagy (75). Endothelial cell damage lead to the damage of epithelial-endothelial barrier and the polysaccharide coating on the surface of endothelial cells, leading to the degradation of glycocalyx, cell rearrangement, destruction of tight junctions between cells, and increased endothelial cell apoptosis, thereby damaging endothelial cells and increasing endothelial permeability (19, 75, 76). The endothelial cell damage lead to increased secretion of angiopoietin 2, which competes with anti-angiopoietin 1 for binding to Tie2 receptors, thereby activating RhoA enzymes, causing skeleton rearrangement and increased capillary permeability, leading to a vicious cycle (77), further aggravating the destruction of the endothelial barrier and damage to multiple organ functions.
1.4 Clinical manifestations
Similar to bacterial sepsis, adenovirus sepsis lacks specific clinical manifestaions (1). HAdV exhibit distinct tissue tropisms depending on their serotypes. Different adenovirus types could cause diseases in various tissues and organs, including pharynx, digestive system, liver, heart, urinary system, central nervous system, the respiratory tract and hematopoietic system (78). HAdV frequently causes ocular infections, resulting in self-limiting conditions such as simple follicular conjunctivitis and pharyngoconjunctival fever (79). HAdV is an important pathogen causing gastroenteritis and diarrhea in infants and young children. According to China's diarrheal syndrome pathogen surveillance data from 2009 to 2018, the detection rate of HAdV reached 9.27% (80). Recent evidence highlights HAdV's emerging role in severe pediatric hepatitis of unknown origin. While traditionally linked to immunocompromised hosts (e.g., transplant recipients), HAdV-41—typically causing gastroenteritis—has been implicated in unexplained hepatitis outbreaks, including cases requiring liver transplantation in immunocompetent children (UK, US) (81, 82). These findings challenge conventional paradigms and urge inclusion of HAdV in differential diagnoses for unexplained hepatitis, particularly in children. Besides, HAdV can induce myocarditis and dilated cardiomyopathy (DCM). In pediatric patients, these conditions may manifest with fulminant progression, severe cases can be life-threatening, necessitating early respiratory and circulatory support (83, 84). HAdV infections of the urinary system frequently cause hemorrhagic cystitis, predominantly occurring in school-aged children with immunocompromised status, particularly following renal transplantation or hematopoietic stem cell transplantation (85). In pediatric patients, HAdV-induced central nervous system disorders encompass febrile seizures, epileptic episodes, encephalitis, and meningoencephalitis. While mild cases typically demonstrate favorable prognoses, severe infections may progress to acute necrotizing encephalopathy, potentially resulting in permanent neurological sequelae (86).
HAdV is a significant viral pathogen of respiratory infections, predominantly affecting children aged 0–5 years and immunocompromised individuals. Notably, up to 20% of HAdV respiratory infections progress to HAdV pneumonia in neonates and infants (87). Adenovirus pneumonia often presents with persistent high fever, cough, and wheezing. Children with severe infection may experience persistent or difficult-to-correct hypoxemia and may also have extrapulmonary symptoms, such as poor mental and appetite, alarm, shock, and decreased blood pressure (13). There are a large number of cases of viral sepsis caused by adenovirus among patients with severe adenovirus pneumonia, which are manifested as acute respiratory distress syndrome (ARDS), persistent serum adenovirus dissemination, increased adenovirus load in serum and nasopharyngeal aspirate (NPA), systemic inflammatory cytokine storm, systemic immune cell exhaustion, and multiple organ dysfunction (13, 34, 35, 38, 88).
Notably, severe adenovirus (HAdV) spesis often leads to secondary hematological disorders, with hemophagocytic lymphohistiocytosis (HLH) being the most predominant (89). HLH is a rare, life-threatening immune dysregulation syndrome. While HAdV-associated HLH typically occurs post-hematopoietic stem cell transplantation due to immune dysfunction (90).
The condition manifests with persistent high fever, hepatosplenomegaly, cytopenia, coagulopathy, and hemophagocytosis on bone marrow smears. Due to insufficient understanding of its pathogenesis, mortality remains high [138–140]. Cases are distributed across all age groups—infants, older children, and adults—without significant predilection (89–91).
Early identification of adenovirus sepsis is of great significance for improving the prognosis of children. In recent years, there have been many reports on risk factors for severe illness or death caused by adenovirus infection. Among them, high serum viral load, highly pathogenic adenovirus serotypes (such as HAdV-7), combined immune deficiency, and young children are more recognized risk factors (13, 23, 34, 35, 38, 88, 92).
1.5 Treatment
1.5.1 Pathogen-directed treatment of adenovirus sepsis
Sepsis guidelines emphasize the important role of early anti-infection treatment in improving the prognosis of sepsis patients and reducing mortality, and antiviral treatment should be initiated as early as possible for sepsis or septic shock caused by virus (1, 2, 92). At present, there are no prospective randomized clinical trials supporting the use of antiviral drugs for severe infections caused by HAdV, and no antiviral drugs have been approved by the FDA (93). Cidofovir is a viral DNA polymerase inhibitor and is the drug of choice for the treatment of adenovirus infection in immunocompromised populations. However, due to limited antiviral activity and nephrotoxicity, it is generally not recommended for use in immunocompetent patients (87, 94). Its potential therapeutic efficacy and safety in immunocompetent patients has only been confirmed in case reports and small sample group (95), more evidence is needed (15, 96). Compared with cidofovir, the lipid conjugate of cidofovir (Brincidofovir) has better bioavailability, no nephrotoxicity, and higher antiviral activity (97, 98). In an animal experiment, the anti-adenovirus efficacy of Brincidofovir was much higher than that of cidofovir (99). Another retrospective study on severe adenovirus infection found that Brincidofovir as a compensatory treatment could continuously reduce the viral load of 67% of patients and improve survival (100). However, the phase Ⅱ clinical trials revealed no statistically significant difference from the placebo treatment, and gastrointestinal toxicity has also been reported (101–103). The broad-spectrum antiviral drug ribavirin is a guanosine analogue with broad antiviral activity against RNA and DNA viruses. Studies have reported that ribavirin has a certain effect on severe adenovirus infection in immunodeficient children, newborns, and solid organ transplant patients (2/5), but its broad effectiveness and safety still need to be verified by prospective randomized controlled trials (104). Besides, Li et al. reviewed many other Nucleoside/nucleotide analogues (such as zalcitabine and alovudine), along with some natural compounds (such as cardamomin and phenolic compounds extracted from Camellia sinensis Kuntze), Epigenetic regulators inhibitors (such as valproic acid, vorinostat and trichostatin),and steroid-based compounds (such as digoxin, digitoxigenin and lanatoside C) to be potential candidates for HAdV therapy in vitro or in vivo (105) (Table 1).
1.5.2 Immunomodulatory therapy
In the hyperinflammatory response stage of sepsis, glucocorticoids play a very important role in reducing the inflammatory response. However, there is controversy about the efficacy and timing of glucocorticoid use in severe infection. Some studies have pointed out that the use of glucocorticoids may be a risk factor for poor prognosis of severe HAdV infection (92), and some studies have pointed out that the timing and dose of glucocorticoid use have no effect on the mortality outcome of severe HAdV infection (13). Some studies have reported that intravenous immunoglobulin may help improve the prognosis of severe HAdV infection, especially in patients with hypogammaglobulinemia (93). For children with normal immune function, the early use of immunoglobulin may reduce the mortality outcome of children (13). The therapeutic effect of neutralizing monoclonal antibodies against HAdV fiber and penton on HAdV infection has been confirmed in animal models (106). In addition, a study involving 92 children with severe adenovirus pneumonia showed that highly effective antibody plasma screened from fresh frozen plasma of healthy blood donors can shorten the fever time of children with severe adenovirus pneumonia and improve the survival rate of patients, and no serious adverse reactions were reported (107). Such results were further confirmed in a prospective cohort study involving 59 children with fatal HAdV pneumonia (14). However, the therapeutic effect of monoclonal antibodies in humans still needs to be confirmed by more large-sample prospective cohort studies.
In the immunosuppressive stage of sepsis, immunostimulatory therapy can help restore immune function and promote rapid clearance of pathogens, thereby reducing the incidence of secondary infections and the mortality rate of late sepsis (108). Many immune stimulators have entered the animal experiment or clinical trial stage, such as programmed cell death 1 (PD 1)/programmed death protein ligand 1 inhibitor, IFN γ, IL 15, IL 7, etc. The clinical application prospects of these immune stimulators in adenovirus sepsis are worth looking forward to (109).
Adaptive immunotherapy such as adenovirus-specific T cell therapy may become a promising immunotherapy for severe HAdV infection. It has been reported that adaptive immunotherapy can be used to treat fatal adenovirus infection in hematopoietic stem cell transplantation (HSCT) patients with T cell exhaustion (110). A clinical trial of HSCT patients found that patients receiving Hexon adaptive T cell transplantation (ACT) had antiviral immunity for up to 6 months, viremia clearance rate of 86%, antigen-specific T cell response, and mortality rate decreased by about 90% (111) (Table 1).
1.5.3 Supportive treatment and complication management
Supportive treatment for adenovirus sepsis is the same as for other pathogens, emphasizing early fluid resuscitation and continuous assessment of hemodynamic stability and fluid responsiveness. Notably, since adenoviral sepsis frequently develops from severe pneumonia caused by adenovirus infection, early respiratory support is critical for managing adenovirus sepsis. A 22-year U.S. study of hospitalized children with adenovirus infections revealed that 11.9% required non-invasive or invasive mechanical ventilation during hospitalization, while 0.4% underwent t extracorporeal membrane oxygenation (ECMO) (28). Recent researches indicates that the mechanical ventilation and ECMO might be beneficial for the patients with severe adenovirus infection (13, 28, 112–114). Besides, multiorgan failure is the one of the main causes of death in sepsis, so treatment to enhance endothelial and epithelial barrier function also plays a pivotal role in the treatment of adenovirus sepsis (19). In addition, vasopressor therapy for septic shock, blood transfusion therapy for anemia, sedation and blood sugar control, nutritional support, etc., all play a very important role in the treatment of adenovirus sepsis (115) (Table 1).
1.6 Discussion
Adenovirus is the main viral pathogen causing severe pneumonia in children, with a high mortality rate. It can cause adenovirus viremia. However, the incidence of viral viremia is often underestimated. Understanding the definition, epidemiology, pathogenic mechanism, diagnosis and treatment of adenovirus sepsis can provide a reference for early clinical decision-making of adenovirus sepsis, in order to improve prognosis and reduce disease mortality.
Brincidofovir is expected to become an effective anti-adenovirus treatment drug, and the early use of intravenous immunoglobulin may help improve the prognosis of severe HAdV infection. The application of many immune stimulatory factors and adaptive T cell transplantation therapy provide new therapeutic prospects for immunotherapy of severe HAdV infection.
Author contributions
WJ: Investigation, Validation, Writing – original draft, Conceptualization, Data curation, Methodology, Supervision, Writing – review & editing. ZL: Investigation, Supervision, Validation, Writing – review & editing. HY: Data curation, Validation, Writing – review & editing. LJ: Data curation, Investigation, Validation, Writing – review & editing. LE: Conceptualization, Supervision, Validation, Writing – review & editing. ZN: Conceptualization, Investigation, Resources, Supervision, Validation, Writing – review & editing.
Funding
The author(s) declare that financial support was received for the research and/or publication of this article. This work was funded by General Project of National Natural Science Foundation of China (32071123), Chongqing High-End Medicine for Young and Middle-Aged People Talent Program (CQYC202203091217), Youth Basic Research Project from the Ministry of Education Key Laboratory of Child Development and Disorders (No. YBRP-202121).
Conflict of interest
The authors declare that the research was conducted in the absence of any commercial or financial relationships that could be construed as a potential conflict of interest.
Generative AI statement
The author(s) declare that no Generative AI was used in the creation of this manuscript.
Publisher's note
All claims expressed in this article are solely those of the authors and do not necessarily represent those of their affiliated organizations, or those of the publisher, the editors and the reviewers. Any product that may be evaluated in this article, or claim that may be made by its manufacturer, is not guaranteed or endorsed by the publisher.
References
1. Singer M, Deutschman CS, Seymour CW, Shankar-Hari M, Annane D, Bauer M, et al. The third international consensus definitions for sepsis and septic shock (sepsis-3). JAMA. (2016) 315(8):801–10. doi: 10.1001/jama.2016.0287
2. Nunes-Silva C, Vilares AT, Schweitzer V, Castanhinha S, Martins A, Lopes MJ, et al. Non-COVID-19 respiratory viral infection. Breathe (Sheff). (2022) 18(1):210151. doi: 10.1183/20734735.0151-2021
3. Sigakis MJG, Jewell E, Maile MD, Cinti SK, Bateman BT, Engoren M. Culture-negative and culture-positive sepsis: a comparison of characteristics and outcomes. Anesth Analg. (2019) 129(5):1300–9. doi: 10.1213/ane.0000000000004072
4. Yang L, Lin Y, Wang J, Song J, Wei B, Zhang X, et al. Comparison of clinical characteristics and outcomes between positive and negative blood culture septic patients: a retrospective cohort study. Infect Drug Resist. (2021) 14:4191–205. doi: 10.2147/idr.S334161
5. Gupta S, Sakhuja A, Kumar G, McGrath E, Nanchal RS, Kashani KB. Culture-negative severe sepsis: nationwide trends and outcomes. Chest. (2016) 150(6):1251–9. doi: 10.1016/j.chest.2016.08.1460
6. Phua J, Ngerng W, See K, Tay C, Kiong T, Lim H, et al. Characteristics and outcomes of culture-negative versus culture-positive severe sepsis. Crit Care. (2013) 17(5):R202. doi: 10.1186/cc12896
7. Lin GL, McGinley JP, Drysdale SB, Pollard AJ. Epidemiology and immune pathogenesis of viral sepsis. Front Immunol. (2018) 9:2147. doi: 10.3389/fimmu.2018.02147
8. Duan Y, Li C, Deng L, An S, Zhu Y, Wang W, et al. Genetic analysis of human adenovirus type 7 strains circulating in different parts of China. Virol Sin. (2021) 36(3):382–92. doi: 10.1007/s12250-020-00334-y
9. Wen S, Lin Z, Zhang Y, Lv F, Li H, Zhang X, et al. The epidemiology, molecular, and clinical of human adenoviruses in children hospitalized with acute respiratory infections. Front Microbiol. (2021) 12:629971. doi: 10.3389/fmicb.2021.629971
10. Akello JO, Kamgang R, Barbani MT, Suter-Riniker F, Leib SL, Ramette A. Epidemiology of human adenoviruses: a 20-year retrospective observational study in hospitalized patients in Bern, Switzerland. Clin Epidemiol. (2020) 12:353–66. doi: 10.2147/clep.S246352
11. Zeng L, Wei J, Tang Y, Liu E, Li Q, Zang N. Clinical characteristics of human adenovirus plastic bronchitis in 10 pediatric cases: a retrospective study of seven years. Virol Sin. (2021) 36(3):550–4. doi: 10.1007/s12250-021-00394-8
12. Shieh WJ. Human adenovirus infections in pediatric population—an update on clinico-pathologic correlation. Biomed J. (2022) 45(1):38–49. doi: 10.1016/j.bj.2021.08.009
13. Wei J, Zang N, Zhang J, He Y, Huang H, Liu X, et al. Genome and proteomic analysis of risk factors for fatal outcome in children with severe community-acquired pneumonia caused by human adenovirus 7. J Med Virol. (2023) 95(11):e29182. doi: 10.1002/jmv.29182
14. Peng H, Chen F, Zuo Y, Huang B, Yang Y, Dang R. Mortality reduction in pediatric patients with severe fatal human adenoviral pneumonia treated with high titer neutralizing antibodies (NAbs) plasma: a retrospective cohort study. BMC Pediatr. (2022) 22(1):151. doi: 10.1186/s12887-022-03225-1
15. Alcamo AM, Wolf MS, Alessi LJ, Chong HJ, Green M, Williams JV, et al. Successful use of cidofovir in an immunocompetent child with severe adenoviral sepsis. Pediatrics. (2020) 145(1):e20191632. doi: 10.1542/peds.2019-1632
16. Bone RC, Balk RA, Cerra FB, Dellinger RP, Fein AM, Knaus WA, et al. Definitions for sepsis and organ failure and guidelines for the use of innovative therapies in sepsis. The ACCP/SCCM consensus conference committee. American college of chest physicians/society of critical care medicine. Chest. (1992) 101(6):1644–55. doi: 10.1378/chest.101.6.1644
17. Levy MM, Fink MP, Marshall JC, Abraham E, Angus D, Cook D, et al. 2001 SCCM/ESICM/ACCP/ATS/SIS international sepsis definitions conference. Intensive Care Med. (2003) 29(4):530–8. doi: 10.1007/s00134-003-1662-x
18. Simpson SQ. Sirs in the time of sepsis-3. Chest. (2018) 153(1):34–8. doi: 10.1016/j.chest.2017.10.006
19. Cross D, Drury R, Hill J, Pollard AJ. Epigenetics in sepsis: understanding its role in endothelial dysfunction, immunosuppression, and potential therapeutics. Front Immunol. (2019) 10:1363. doi: 10.3389/fimmu.2019.01363
20. Zhang R, Wang H, Tian S, Deng J. Adenovirus viremia may predict adenovirus pneumonia severity in immunocompetent children. BMC Infect Dis. (2021) 21(1):213. doi: 10.1186/s12879-021-05903-4
21. Cillóniz C, Dominedò C, Magdaleno D, Ferrer M, Gabarrús A, Torres A. Pure viral sepsis secondary to community-acquired pneumonia in adults: risk and prognostic factors. J Infect Dis. (2019) 220(7):1166–71. doi: 10.1093/infdis/jiz257
22. Lenaerts L, De Clercq E, Naesens L. Clinical features and treatment of adenovirus infections. Rev Med Virol. (2008) 18(6):357–74. doi: 10.1002/rmv.589
23. Liu MC, Xu Q, Li TT, Wang T, Jiang BG, Lv CL, et al. Prevalence of human infection with respiratory adenovirus in China: a systematic review and meta-analysis. PLoS Negl Trop Dis. (2023) 17(2):e0011151. doi: 10.1371/journal.pntd.0011151
24. Lin KH, Lin YC, Chen HL, Ke GM, Chiang CJ, Hwang KP, et al. A two decade survey of respiratory adenovirus in Taiwan: the reemergence of adenovirus types 7 and 4. J Med Virol. (2004) 73(2):274–9. doi: 10.1002/jmv.20087
25. Kim YJ, Hong JY, Lee HJ, Shin SH, Kim YK, Inada T, et al. Genome type analysis of adenovirus types 3 and 7 isolated during successive outbreaks of lower respiratory tract infections in children. J Clin Microbiol. (2003) 41(10):4594–9. doi: 10.1128/jcm.41.10.4594-4599.2003
26. Cooper RJ, Hallett R, Tullo AB, Klapper PE. The epidemiology of adenovirus infections in greater Manchester, UK 1982–96. Epidemiol Infect. (2000) 125(2):333–45. doi: 10.1017/s0950268899004550
27. Gray GC, McCarthy T, Lebeck MG, Schnurr DP, Russell KL, Kajon AE, et al. Genotype prevalence and risk factors for severe clinical adenovirus infection, United States 2004–2006. Clin Infect Dis. (2007) 45(9):1120–31. doi: 10.1086/522188
28. Totapally BR, Totapalli S, Sendi P, Martinez PA. Epidemiology of adenovirus infection in hospitalized children in the United States from 1997 to 2019. Pediatr Infect Dis J. (2024) 43(8):748–55. doi: 10.1097/inf.0000000000004365
29. Kajon AE, Mistchenko AS, Videla C, Hortal M, Wadell G, Avendaño LF. Molecular epidemiology of adenovirus acute lower respiratory infections of children in the south cone of South America (1991–1994). J Med Virol. (1996) 48(2):151–6. doi: 10.1002/(SICI)1096-9071(199602)48:2%3C151::AID-JMV6%3E3.0.CO;2-8
30. Kenmoe S, Vernet MA, Le Goff J, Penlap VB, Vabret A, Njouom R. Molecular characterization of human adenovirus associated with acute respiratory infections in Cameroon from 2011 to 2014. Virol J. (2018) 15(1):153. doi: 10.1186/s12985-018-1064-x
31. Wu X, Lu X, Schneider E, Ahmed JA, Njenga MK, Breiman RF, et al. Reassessment of high prevalence human adenovirus detections among residents of two refugee centers in Kenya under surveillance for acute respiratory infections. J Med Virol. (2019) 91(3):385–91. doi: 10.1002/jmv.25320
32. Mennechet FJD, Paris O, Ouoba AR, Salazar Arenas S, Sirima SB, Takoudjou Dzomo GR, et al. A review of 65 years of human adenovirus seroprevalence. Expert Rev Vaccines. (2019) 18(6):597–613. doi: 10.1080/14760584.2019.1588113
33. Heo JY, Noh JY, Jeong HW, Choe KW, Song JY, Kim WJ, et al. Molecular epidemiology of human adenovirus-associated febrile respiratory illness in soldiers, South Korea (1). Emerg Infect Dis. (2018) 24(7):1221–7. doi: 10.3201/eid2407.171222
34. Yu Z, Zeng Z, Zhang J, Pan Y, Chen M, Guo Y, et al. Fatal community-acquired pneumonia in children caused by re-emergent human adenovirus 7d associated with higher severity of illness and fatality rate. Sci Rep. (2016) 6:37216. doi: 10.1038/srep37216
35. Fu Y, Tang Z, Ye Z, Mo S, Tian X, Ni K, et al. Human adenovirus type 7 infection causes a more severe disease than type 3. BMC Infect Dis. (2019) 19(1):36. doi: 10.1186/s12879-018-3651-2
36. Zhang Q, Zhou Z, Fan Y, Liu T, Guo Y, Li X, et al. Higher affinities of fibers with cell receptors increase the infection capacity and virulence of human adenovirus type 7 and type 55 compared to type 3. Microbiol Spectr. (2024) 12(1):e0109023. doi: 10.1128/spectrum.01090-23
37. Zhang J, Kang J, Dehghan S, Sridhar S, Lau SKP, Ou J, et al. A survey of recent adenoviral respiratory pathogens in Hong Kong reveals emergent and recombinant human adenovirus type 4 (HAdV-e4) circulating in civilian populations. Viruses. (2019) 11(2):129. doi: 10.3390/v11020129
38. Schilham M, Claas E, van Zaane W, Heemskerk B, Vossen J, Lankester A, et al. High levels of adenovirus DNA in serum correlate with fatal outcome of adenovirus infection in children after allogeneic stem-cell transplantation. Clin Infect Dis. (2002) 35(5):526–32. doi: 10.1086/341770
39. Zhang R, Wang H, Tian S, Deng J. Adenovirus viremia predicts adenovirus pneumonia severity in immunocompetent children. BMC Infect Dis. (2020) 21(1):213. doi: 10.1186/s12879-021-05903-4
40. Cabler SS, Storch GA, Weinberg JB, et al. Viral dnaemia and DNA virus seropositivity and mortality in pediatric sepsis. JAMA Netw Open. (2024) 7(2):e240383. doi: 10.1001/jamanetworkopen.2024.0383
41. Siminovich M, Murtagh P. Acute lower respiratory tract infections by adenovirus in children: histopathologic findings in 18 fatal cases. Pediatr Dev Pathol. (2011) 14(3):214–7. doi: 10.2350/10-05-0838-oa.1
42. Kulanayake S, Tikoo SK. Adenovirus core proteins: structure and function. Viruses. (2021) 13(3):388. doi: 10.3390/v13030388
43. Russell WC. Adenoviruses: update on structure and function. J Gen Virol. (2009) 90(Pt 1):1–20. doi: 10.1099/vir.0.003087-0
44. van der Poll T, van de Veerdonk FL, Scicluna BP, Netea MG The immunopathology of sepsis and potential therapeutic targets. Nat Rev Immunol. (2017) 17(7):407–20. doi: 10.1038/nri.2017.36
45. Boyd JH, Russell JA, Fjell CD. The meta-genome of sepsis: host genetics, pathogens and the acute immune response. J Innate Immun. (2014) 6(3):272–83. doi: 10.1159/000358835
46. Takeuchi O, Akira S. Pattern recognition receptors and inflammation. Cell. (2010) 140(6):805–20. doi: 10.1016/j.cell.2010.01.022
47. Daussy CF, Pied N, Wodrich H. Understanding post entry sorting of adenovirus capsids; a chance to change vaccine vector properties. Viruses. (2021) 13(7):1221. doi: 10.3390/v13071221
48. Li E, Stupack D, Klemke R, Cheresh DA, Nemerow GR. Adenovirus endocytosis via alpha(v) integrins requires phosphoinositide-3-oh kinase. J Virol. (1998) 72(3):2055–61. doi: 10.1128/jvi.72.3.2055-2061.1998
49. Philpott NJ, Nociari M, Elkon KB, Falck-Pedersen E. Adenovirus-induced maturation of dendritic cells through a pi3 kinase-mediated tnf-alpha induction pathway. Proc Natl Acad Sci U S A. (2004) 101(16):6200–5. doi: 10.1073/pnas.0308368101
50. Hendrickx R, Stichling N, Koelen J, Kuryk L, Lipiec A, Greber UF. Innate immunity to adenovirus. Hum Gene Ther. (2014) 25(4):265–84. doi: 10.1089/hum.2014.001
51. Fejer G, Drechsel L, Liese J, Schleicher U, Ruzsics Z, Imelli N, et al. Key role of splenic myeloid DCS in the ifn-alphabeta response to adenoviruses in vivo. PLoS Pathog. (2008) 4(11):e1000208. doi: 10.1371/journal.ppat.1000208
52. Barlan AU, Griffin TM, McGuire KA, Wiethoff CM. Adenovirus membrane penetration activates the nlrp3 inflammasome. J Virol. (2011) 85(1):146–55. doi: 10.1128/jvi.01265-10
53. Bottermann M, Foss S, Caddy SL, Clift D, van Tienen LM, Vaysburd M, et al. Complement c4 prevents viral infection through capsid inactivation. Cell Host Microbe. (2019) 25(4):617–29.e617. doi: 10.1016/j.chom.2019.02.016
54. Chen H, Xiang ZQ, Li Y, Kurupati RK, Jia B, Bian A, et al. Adenovirus-based vaccines: comparison of vectors from three species of adenoviridae. J Virol. (2010) 84(20):10522–32. doi: 10.1128/jvi.00450-10
55. Bradley RR, Lynch DM, Iampietro MJ, Borducchi EN, Barouch DH. Adenovirus serotype 5 neutralizing antibodies target both hexon and fiber following vaccination and natural infection. J Virol. (2012) 86(1):625–9. doi: 10.1128/jvi.06254-11
56. Veltrop-Duits L.A, Heemskerk B, Sombroek CC, van Vreeswijk T, Gubbels S, Toes RE, et al. Human cd4+t cells stimulated by conserved adenovirus 5 hexon peptides recognize cells infected with different species of human adenovirus. Eur J Immunol. (2006) 36(9):2410–23. doi: 10.1002/eji.200535786
57. Rock KL, Reits E, Neefjes J. Present yourself! by MHC class I and MHC class ii molecules. Trends Immunol. (2016) 37(11):724–37. doi: 10.1016/j.it.2016.08.010
58. Münz C. Autophagy beyond intracellular MHC class ii antigen presentation. Trends Immunol. (2016) 37(11):755–63. doi: 10.1016/j.it.2016.08.017
59. Nimmerjahn F, Milosevic S, Behrends U, Jaffee EM, Pardoll DM, Bornkamm GW, et al. Major histocompatibility complex class II-restricted presentation of a cytosolic antigen by autophagy. Eur J Immunol. (2003) 33(5):1250–9. doi: 10.1002/eji.200323730
60. Vaysburd M, Watkinson RE, Cooper H, Reed M, O’Connell K, Smith J, et al. Intracellular antibody receptor trim21 prevents fatal viral infection. Proc Natl Acad Sci U S A. (2013) 110(30):12397–401. doi: 10.1073/pnas.1301918110
61. Oliveira ERA, Bouvier M. Immune evasion by adenoviruses: a window into host-virus adaptation. FEBS Lett. (2019) 593(24):3496–503. doi: 10.1002/1873-3468.13682
62. McSharry BP, Burgert HG, Owen DP, Stanton RJ, Prod’homme V, Sester M, et al. Adenovirus e3/19k promotes evasion of NK cell recognition by intracellular sequestration of the nkg2d ligands major histocompatibility complex class I chain-related proteins a and b. J Virol. (2008) 82(9):4585–94. doi: 10.1128/jvi.02251-07
63. Teodoro JG, Branton PE. Regulation of p53-dependent apoptosis, transcriptional repression, and cell transformation by phosphorylation of the 55-kilodalton e1b protein of human adenovirus type 5. J Virol. (1997) 71(5):3620–7. doi: 10.1128/jvi.71.5.3620-3627.1997
64. Fonseca GJ, Thillainadesan G, Yousef AF, Ablack JN, Mossman KL, Torchia J, et al. Adenovirus evasion of interferon-mediated innate immunity by direct antagonism of a cellular histone posttranslational modification. Cell Host Microbe. (2012) 11(6):597–606. doi: 10.1016/j.chom.2012.05.005
65. Ackrill AM, Foster GR, Laxton C.D, Flavell DM, Stark GR, Kerr I.M. Inhibition of the cellular response to interferons by products of the adenovirus type 5 e1a oncogene. Nucleic Acids Res. (1991) 19(16):4387–93. doi: 10.1093/nar/19.16.4387
66. Gu X, Zhou F, Wang Y, Fan G, Cao B. Respiratory viral sepsis: epidemiology, pathophysiology, diagnosis and treatment. Eur Respir Rev. (2020) 29(157):200038. doi: 10.1183/16000617.0038-2020
67. Deutschman CS, Tracey KJ. Sepsis: current dogma and new perspectives. Immunity. (2014) 40(4):463–75. doi: 10.1016/j.immuni.2014.04.001
68. Chan JK, Roth J, Oppenheim JJ, Tracey KJ, Vogl T, Feldmann M, et al. Alarmins: awaiting a clinical response. J Clin Invest. (2012) 122(8):2711–9. doi: 10.1172/jci62423
69. Merle NS, Noe R, Halbwachs-Mecarelli L, Fremeaux-Bacchi V, Roumenina LT. Complement system part II: role in immunity. Front Immunol. (2015) 6:257. doi: 10.3389/fimmu.2015.00257
70. Levi M, van der Poll T. Coagulation and sepsis. Thromb Res. (2017) 149:38–44. doi: 10.1016/j.thromres.2016.11.007
71. Boomer JS, To K, Chang KC, Takasu O, Osborne DF, Walton AH, et al. Immunosuppression in patients who die of sepsis and multiple organ failure. JAMA. (2011) 306(23):2594–605. doi: 10.1001/jama.2011.1829
72. Hotchkiss RS, Monneret G, Payen D. Sepsis-induced immunosuppression: from cellular dysfunctions to immunotherapy. Nat Rev Immunol. (2013) 13(12):862–74. doi: 10.1038/nri3552
73. Mangel WF, San Martín C. Structure, function and dynamics in adenovirus maturation. Viruses. (2014) 6(11):4536–70. doi: 10.3390/v6114536
74. Yang Z, WEI J, REN L, Chen S, Liu E, Zang N. HAdV-7 infection induces ferroptosis in alveolar epithelial cells. Lu Jun Jun Yi Da Xue Xue Bao. (2022) 44(21):2146–56. doi: 10.16016/j.2097-0927.202204131
75. Chen Z, Yang Z, Rao L, Li C, Zang N, Liu E. Human adenovirus type 7 (HAdV-7) infection induces pulmonary vascular endothelial injury through the activation of endothelial autophagy. Respir Res. (2024) 25(1):425. doi: 10.1186/s12931-024-03025-4
76. Steinberg BE, Goldenberg NM, Lee WL. Do viral infections mimic bacterial sepsis? The role of microvascular permeability: a review of mechanisms and methods. Antiviral Res. (2012) 93(1):2–15. doi: 10.1016/j.antiviral.2011.10.019
77. Leligdowicz A, Richard-Greenblatt M, Wright J, Crowley VM, Kain KC. Endothelial activation: the ang/tie axis in sepsis. Front Immunol. (2018) 9:838. doi: 10.3389/fimmu.2018.00838
78. Yanhao H, Jianhua W, Na Z. Advances in epidemiological studies of human adenovirus infection. Adv Clin Med. (2024) 14(10):1337–48. doi: 10.12677/acm.2024.14102805
79. Jonas RA, Ung L, Rajaiya J, Chodosh J. Mystery eye: human adenovirus and the enigma of epidemic keratoconjunctivitis. Prog Retin Eye Res. (2020) 76:100826. doi: 10.1016/j.preteyeres.2019.100826
80. Wang LP, Zhou SX, Wang X, Lu QB, Shi LS, Ren X, et al. Etiological, epidemiological, and clinical features of acute diarrhea in China. Nat Commun. (2021) 12(1):2464. doi: 10.1038/s41467-021-22551-z
81. Mücke MM, Zeuzem S. The recent outbreak of acute severe hepatitis in children of unknown origin—what is known so far. J Hepatol. (2022) 77(1):237–42. doi: 10.1016/j.jhep.2022.05.001
82. Portal TM, Reymão TKA, Quinderé Neto G.A, Fiuza M, Teixeira DM, Lima ICG, et al. Detection and genotyping of enteric viruses in hospitalized children with acute gastroenteritis in Belém, Brazil: occurrence of adenovirus viremia by species f, types 40/41. J Med Virol. (2019) 91(3):378–84. doi: 10.1002/jmv.25321
83. Amabile N, Fraisse A, Bouvenot J, Chetaille P, Ovaert C. Outcome of acute fulminant myocarditis in children. Heart. (2006) 92(9):1269–73. doi: 10.1136/hrt.2005.078402
84. Gupalo EM, Buryachkovskaya LI, Chumachenko PV, Mironova NA, Narusov OY, Tereschenko SN, et al. Implication of inflammation on coxsackie virus and adenovirus receptor expression on cardiomyocytes and the role of platelets in patients with dilated cardiomyopathy. Cardiovasc Pathol. (2022) 60:107452. doi: 10.1016/j.carpath.2022.107452
85. Numazaki Y, Shigeta S, Kumasaka T, Miyazawa T, Yamanaka M, Yano N, et al. Acute hemorrhagic cystitis in children. Isolation of adenovirus type II. N Engl J Med. (1968) 278(13):700–4. doi: 10.1056/nejm196803282781303
86. Zhang XF, Tan CB, Yao ZX, Jiang L, Hong SQ. Adenovirus infection-associated central nervous system disease in children. Pediatr Infect Dis J. (2021) 40(3):205–8. doi: 10.1097/inf.0000000000003000
87. Lynch JP 3rd, Kajon AE. Adenovirus: epidemiology, global spread of novel serotypes, and advances in treatment and prevention. Semin Respir Crit Care Med. (2016) 37(4):586–602. doi: 10.1055/s-0036-1584923
88. Gu L, Qu J, Sun B, Yu X, Li H, Cao B. Sustained viremia and high viral load in respiratory tract secretions are predictors for death in immunocompetent adults with adenovirus pneumonia. PLoS One. (2016) 11(8):e0160777. doi: 10.1371/journal.pone.0160777
89. Hosnut F, Ozçay F, Malbora B, Hizli S, Ozbek N. Severe adenovirus infection associated with hemophagocytic lymphohistiocytosis. Turk J Haematol. (2014) 31(1):103–5. doi: 10.4274/Tjh.2013.0218
90. Mellon G, Henry B, Aoun O, Boutolleau D, Laparra A, Mayaux J, et al. Adenovirus related lymphohistiocytic hemophagocytosis: case report and literature review. J Clin Virol. (2016) 78:53–6. doi: 10.1016/j.jcv.2016.03.011
91. Censoplano N, Gorga S, Waldeck K, Stillwell T, Rabah-Hammad R, Flori H. Neonatal adenovirus infection complicated by hemophagocytic lymphohistiocytosis syndrome. Pediatrics. (2018) 141(Suppl 5):S475–80. doi: 10.1542/peds.2017-2061
92. Hong Y, Wang Q, Song Y, Liu G, Shen J. Factors associated with severe adenovirus pneumonia in children: a retrospective study from Guangzhou, China (2017–2019). Med Sci Monit. (2023) 29:e939578. doi: 10.12659/msm.939578
93. Florescu DF, Schaenman JM. Adenovirus in solid organ transplant recipients: guidelines from the American society of transplantation infectious diseases community of practice. Clin Transplant. (2019) 33(9):e13527. doi: 10.1111/ctr.13527
94. Sulejmani N, Nagai S, Safwan M, Rizzari MD, Raoufi M, Abouljoud M.S, et al. Brincidofovir as salvage therapy for adenovirus disease in intestinal transplant recipients. Pharmacotherapy. (2018) 38(4):470–5. doi: 10.1002/phar.2094
95. Zalcman J, Pasternak Y, Kenan D, Dotan M, Gueta I, Kadmon G, et al. Safety of cidofovir treatment for suspected or confirmed adenovirus infection in immunocompetent pediatric population. Pediatr Infect Dis J. (2024) 43(3):198–202. doi: 10.1097/inf.0000000000004186
96. Le TK, Brown BK, Namtu KC, Berman DM, Kiskaddon AL. Use of cidofovir with extracorporeal membrane oxygenation to treat adenovirus-associated acute respiratory distress syndrome in paediatric patients- a case series. J Clin Pharm Ther. (2020) 45(6):1505–10. doi: 10.1111/jcpt.13244
97. Hiwarkar P, Amrolia P, Sivaprakasam P, Lum SH, Doss H, O’Rafferty C, et al. Brincidofovir is highly efficacious in controlling adenoviremia in pediatric recipients of hematopoietic cell transplant. Blood. (2017) 129(14):2033–7. doi: 10.1182/blood-2016-11-749721
98. Painter W, Robertson A, Trost LC, Godkin S, Lampert B, Painter G. First pharmacokinetic and safety study in humans of the novel lipid antiviral conjugate cmx001, a broad-spectrum oral drug active against double-stranded DNA viruses. Antimicrob Agents Chemother. (2012) 56(5):2726–34. doi: 10.1128/aac.05983-11
99. Toth K, Spencer JF, Dhar D, Sagartz JE, Buller RM, Painter GR, et al. Hexadecyloxypropyl-cidofovir, cmx001, prevents adenovirus-induced mortality in a permissive, immunosuppressed animal model. Proc Natl Acad Sci U S A. (2008) 105(20):7293–7. doi: 10.1073/pnas.0800200105
100. Florescu DF, Pergam SA, Neely MN, Qiu F, Johnston C, Way S, et al. Safety and efficacy of cmx001 as salvage therapy for severe adenovirus infections in immunocompromised patients. Biol Blood Marrow Transplant. (2012) 18(5):731–8. doi: 10.1016/j.bbmt.2011.09.007
101. Prasad V, Papanicolaou G, Marón G, Vainorius E, Brundage T, Chittick G, et al. Treatment of adenovirus (ADV) infection in allogeneic hematopoietic cell transplant (allo hct) patients (pts) with brincidofovir: final 36 week results from the advise trial. Biol Blood Marrow Transplant. (2017) 23(3):S57–8. doi: 10.1016/j.bbmt.2017.01.062
102. Grimley M, Chemaly RF, Englund JA, Kurtzberg J, Chittick G, Brundage TM, et al. Brincidofovir for asymptomatic adenovirus viremia in pediatric and adult allogeneic hematopoietic cell transplant recipients: a randomized placebo-controlled phase II trial. Biol Blood Marrow Transplant. (2017) 23(3):512–21. doi: 10.1016/j.bbmt.2016.12.621
103. Detweiler CJ, Mueller SB, Sung AD, Saullo JL, Prasad VK, Cardona DM. Brincidofovir (cmx001) toxicity associated with epithelial apoptosis and crypt drop out in a hematopoietic cell transplant patient: challenges in distinguishing drug toxicity from GVHD. J Pediatr Hematol Oncol. (2018) 40(6):e364–8. doi: 10.1097/mph.0000000000001227
104. Gavin PJ, Katz BZ. Intravenous ribavirin treatment for severe adenovirus disease in immunocompromised children. Pediatrics. (2002) 110(1 Pt 1):e9. doi: 10.1542/peds.110.1.e9
105. Li L, Xie Z, Xu L. Current antiviral agents against human adenoviruses associated with respiratory infections. Front Pediatr. (2024) 12:1456250. doi: 10.3389/fped.2024.1456250
106. Liu X, Li Z, Li X, Zhang X, Zheng Y, Su W, et al. Neutralizing monoclonal antibodies protect against human adenovirus type 55 infection in transgenic mice and tree shrews. Emerg Microbes Infect. (2024) 13(1):2307513. doi: 10.1080/22221751.2024.2307513
107. Peng HY, Chen FY, Dang R, Zuo YL, Hu PD, Yang YY, et al. Effect of high-titer plasma in pediatric patients with severe adenovirus pneumonia. Zhonghua Er Ke Za Zhi. (2020) 58(5):392–7. doi: 10.3760/cma.j.cn112140-20191111-00713
108. Leentjens J, Kox M, van der Hoeven JG, Netea MG, Pickkers P. Immunotherapy for the adjunctive treatment of sepsis: from immunosuppression to immunostimulation. Time for a paradigm change? Am J Respir Crit Care Med. (2013) 187(12):1287–93. doi: 10.1164/rccm.201301-0036CP
109. Rubio I, Osuchowski MF, Shankar-Hari M, Skirecki T, Winkler MS, Lachmann G, et al. Current gaps in sepsis immunology: new opportunities for translational research. Lancet Infect Dis. (2019) 19(12):e422–36. doi: 10.1016/s1473-3099(19)30567-5
110. Chakrabarti S, Collingham KE, Fegan CD, Pillay D, Milligan DW. Adenovirus infections following haematopoietic cell transplantation: is there a role for adoptive immunotherapy? Bone Marrow Transplant. (2000) 26(3):305–7. doi: 10.1038/sj.bmt.1702508
111. Feucht J, Opherk K, Lang P, Kayser S, Hartl L, Bethge W, et al. Adoptive t-cell therapy with hexon-specific th1 cells as a treatment of refractory adenovirus infection after HSCT. Blood. (2015) 125(12):1986–94. doi: 10.1182/blood-2014-06-573725
112. Shi T, Chen C, Fan H, Yu M, Li M, Yang D, et al. Impact of extracorporeal membrane oxygenation in immunocompetent children with severe adenovirus pneumonia. BMC Pulm Med. (2023) 23(1):41. doi: 10.1186/s12890-022-02284-5
113. Cui Y, Shi J, Zhou Y, Dou J, Xiong X, Sun T, et al. Extracorporeal membrane oxygenation for paediatric refractory hypoxic respiratory failure caused by adenovirus in Shanghai: a case series. BMC Pediatr. (2022) 22(1):138. doi: 10.1186/s12887-022-03197-2
114. Lee M, Kim S, Kwon OJ, Kim JH, Jeong I, Son JW, et al. Treatment of adenoviral acute respiratory distress syndrome using cidofovir with extracorporeal membrane oxygenation. J Intensive Care Med. (2017) 32(3):231–8. doi: 10.1177/0885066616681272
Keywords: human adenovirus (HAdV), viral sepsis, pathogenesis, treatment, epidemiology
Citation: Jianhua W, Lingjian Z, Yanhao H, Jing L, Enmei L and Na Z (2025) Research progress on human adenovirus sepsis. Front. Pediatr. 13:1552958. doi: 10.3389/fped.2025.1552958
Received: 29 December 2024; Accepted: 2 May 2025;
Published: 19 May 2025.
Edited by:
Gen Lu, Guangzhou Medical University, ChinaReviewed by:
Siqi Hu, Seventh Medical Center of PLA General Hospital, ChinaBalagangadhar Totapally, Nicklaus Children's Health System, United States
Copyright: © 2025 Jianhua, Lingjian, Yanhao, Jing, Enmei and Na. This is an open-access article distributed under the terms of the Creative Commons Attribution License (CC BY). The use, distribution or reproduction in other forums is permitted, provided the original author(s) and the copyright owner(s) are credited and that the original publication in this journal is cited, in accordance with accepted academic practice. No use, distribution or reproduction is permitted which does not comply with these terms.
*Correspondence: Zang Na, emFuZ25hMTIxNEAxMjYuY29t