- Department of Entomology, Center for Pollinator Research, The Pennsylvania State University, University Park, PA, USA
Agrochemical risk assessment that takes into account only pesticide active ingredients without the spray adjuvants commonly used in their application will miss important toxicity outcomes detrimental to non-target species, including humans. Lack of disclosure of adjuvant and formulation ingredients coupled with a lack of adequate analytical methods constrains the assessment of total chemical load on beneficial organisms and the environment. Adjuvants generally enhance the pesticidal efficacy and inadvertently the non-target effects of the active ingredient. Spray adjuvants are largely assumed to be biologically inert and are not registered by the USA EPA, leaving their regulation and monitoring to individual states. Organosilicone surfactants are the most potent adjuvants and super-penetrants available to growers. Based on the data for agrochemical applications to almonds from California Department of Pesticide Regulation, there has been increasing use of adjuvants, particularly organosilicone surfactants, during bloom when two-thirds of USA honey bee colonies are present. Increased tank mixing of these with ergosterol biosynthesis inhibitors and other fungicides and with insect growth regulator insecticides may be associated with recent USA honey bee declines. This database archives every application of a spray tank adjuvant with detail that is unprecedented globally. Organosilicone surfactants are good stand alone pesticides, toxic to bees, and are also present in drug and personal care products, particularly shampoos, and thus represent an important component of the chemical landscape to which pollinators and humans are exposed. This mini review is the first to possibly link spray adjuvant use with declining health of honey bee populations.
Introduction
Applications of modern pesticide formulations, particularly in combinations, are often accomplished using proprietary spray adjuvants to achieve high efficacy for targeted pests and diseases (1). An adjuvant is an additive or supplement used to enhance the performance or aid in the stability of formulations of active ingredients (2). Adjuvant products are formulated combinations of surfactants, penetrant enhancers, activators, spreaders, stickers, cosolvents, wetting agents, pH modifiers, defoaming agents, drift retardants, nutrients, etc., depending on their proposed utility. Usually, adjuvants are much less expensive than formulated active ingredients and can reduce the active ingredient dose needed by an order or more of magnitude (3, 4). Similarly, contemporary drug delivery to humans and animals transdermally (5) and orally (6) is often mediated via adjuvant technologies that enhance penetration. Newer agrochemical technologies include co-formulants such as polyethoxylated tallow amines, cosolvents such as N-methyl-2-pyrrolidone (NMP), and spray adjuvants such as organosilicone polyethoxylates (7).
Numerous studies have found that pesticide active ingredients elicit very different physiological effects on non-target organisms when combined with their co-formulants and tank adjuvants (7–9). Despite the widespread assumption that formulation ingredients and spray adjuvants are biologically inert, substantial evidence suggests that this is often not the case. Indeed, glyphosate has weak ecotoxicity and systemic movement without tallow amines and other adjuvants (10–12), including its toxicity to mammals (13) and human cells (14). Noteworthy is the fact that spray tank adjuvants by themselves harm non-target organisms from all taxa studied. Adjuvant-dependent toxicities, often more than the associated formulations of herbicides and fungicides, have been reported for bacteria (15), cyanobacteria (16), algae (17), and snails (18). The non-ionic spray adjuvant R-11 synergized the acute toxicity of the insecticides spinosad (19) and imidacloprid (20) on aquatic crustaceans and, in the absence of an insecticide, reduced the growth rate of Daphnia pulex at relevant field concentrations found after application near aquatic systems (21). Aquatic organisms are particularly vulnerable to the general ecotoxicity of adjuvant surfactants ranging from invertebrates (22, 23) to fish (19, 24, 25) and amphibians (26). Terrestrial insects, in turn, have long been shown susceptible to insecticide synergisms associated with spray adjuvants (27, 28). Many of the classical cases of ecotoxicities found with spray adjuvants and used with pesticides other than glyphosate are due to older surfactant classes, such as nonylphenol polyethoxylates, which environmentally degrade to the endocrine disrupting nonylphenols (29). It is clear that agrochemical risk assessment that takes into account only pesticide active ingredients and their formulations in absence of the spray adjuvants commonly used in their application (30, 31) will miss important toxicity outcomes that may prove detrimental, even to humans. Here, we attempt to characterize the scope of spray adjuvant use, especially organosilicone surfactants, and explore a possible link between their increasing presence in California almonds and the declining health of honey bee populations.
Spray Adjuvants Contribute to the Toxic Load
Supplemental adjuvants used in tank mixes generally enhance the pesticidal efficacy as well as inadvertently the non-target effects of the active ingredient after application (7, 14). Dramatic impacts of agrochemical formulants on the bee toxicity of pesticide active ingredients have been documented (32). Formulations are generally more toxic than active ingredients, particularly fungicides, by up to 26,000-fold based on published literature. The highest oral toxicity of three insecticide formulations tested was for Vertimec® 18 EC that was 8,970 times more toxic to the stingless bee Melipona quadrifasciata and 709 times more toxic to the honey bee than the topically applied active ingredient abamectin in acetone (33). However, the largest documented formulation compared to active ingredient differences in bee toxicity have been with the least toxic pesticides, particularly fungicides. Among the 300 pesticide formulations tested for oral toxicity to adult honey bee in China, a 25% EC formulation of the fungicide tebuconazole was equally toxic to the most bee-toxic insecticide known, emamectin benzoate (LD50 = 0.0035 μg/bee), whereas a 5% suspension concentrate of tebuconazole was > 25,000 times less toxic (34). This product-dependent range in toxicity is presumably determined by the undisclosed fungicide co-formulants. While technical glyphosate has virtually no toxicity for honey bees, common formulations such as WeatherMAX® do (35). Commercial formulations of fumagillin acid used to control Nosema and other microsporidian fungal diseases in honey bees and mammals, respectively, are actually salts of the base dicyclohexylamine. This co-formulant is five times more toxic and persistent than the active ingredient to rodents and other organisms, serving as a sensitive bioindicator of fumagillin pollution (36). Most studies documenting pesticide effects on honey bees are performed without the formulation or other relevant spray adjuvant components used when applying the active ingredient, most often due to lack of such required tests for product registration (7).
Less potent bee toxicities are usually found when spray adjuvants are tested alone or relative to the pesticide formulations used in tank combinations. About one-third of non-ionic, organosilicone and other surfactant spray adjuvants at up to a 0.2% aqueous solution have been shown to deter or kill honey bees (37–39). Exposure to the nonylphenol polyethoxylate adjuvant N-90 by itself at field rates impaired nest recognition behavior of two managed solitary bees, Osmia lignaria and Megachile rotundata (40). While the organosilicone adjuvant Break-Thru® fed to nurse bees at 200 ppm with or without 400 ppm of the fungicide Pristine® did not impact honey bee queen development or survival (41), toxic interaction of the co-occurring insect growth regulator (IGR) dimilin with this adjuvant is likely [cf., Ref. (42)]. Higher toxicities were found when honey bees are fed related commercial organosilicone surfactants in 50% sucrose with oral LC50s around 10 ppm (7). A discontinued agrochemical surfactant perfluorooctylsulfonic acid is highly and orally toxic to Bombus terrestris (43). The penetration enhancing solvent NMP commonly present in agrochemical formulations is a dietary toxicant for honey bee larvae at 100 ppm (44).
Organosilicone surfactants are particularly potent as super-penetrants, super-spreaders, and probable ecotoxicants (7). They are used worldwide at up to 1% (10,000 ppm) of the spray tank mix, while other adjuvant classes require higher amounts up to 5% of the spray tank mix (3, 32). All organosilicone surfactant adjuvants (OSSA) tested (Dyne-Amic®, Syl-Tac®, Sylgard 309®, and Silwet L-77®) impaired honey bee olfactory learning much more than other non-ionic adjuvants (Activator 90®, R-11®, and Induce®), while the crop oil concentrates (Penetrator®, Agri-Dex®, and Crop Oil Concentrate®) were inactive at 20 μg per bee (45). The greater surfactancy of organosilicones over other non-ionic adjuvants and crop oil concentrates can drive the stomatal uptake of large bacterial-sized mineral particles (46) and Agrobacterium transformation of grape plantlets (47), and thus may aid movement of pathogens into bee tissues.
Spray Adjuvant Use during Pollination of California Almonds
Pollination of California almonds during February and March is the single largest pollination event in the world. Over 60% (1.5 million) of USA honey bee colonies are transported to California each year to pollinate the crop. A workshop convened to address reduced overwinter survivorship of commercial honey bee colonies used in almond pollination since the 2006 onset of colony collapse disorder (CCD) judged neonicotinoids unlikely to be a sole factor and Varroa mites plus viruses to be a probable cause (48). However, fungicides, herbicides, and spray adjuvants were not evaluated. Recent surveys of migratory beekeepers who pollinate almonds do not self-report overwintering losses greater than the majority of non-migratory beekeepers, although their summer colony losses tend to be higher (49). Better management practices employed by migratory beekeepers who pollinate almonds may explain their lower winter losses in comparison with sideline or backyard beekeepers (50). Nevertheless, it has been surmised by beekeepers and documented by researchers that decreasing honey bee health issues are initiated in almonds, a winter/early spring pollinated crop, and then progressed over the course of the year as colonies are employed to pollinate other crops including apples, blueberries, alfalfa, cotton, pumpkin, cantaloupe, etc. Although the rates of foraging honey bees were not reduced over time during almond pollination in contrast to those pollinating cotton and alfalfa, there was no corresponding increase in foraging population though a significant increase in colony size occurred (51). Some of the highest pesticide residues, especially fungicides, were found on almonds, which represents a notable pesticide exposure risk and ranked fifth in hazard among the eight crops assessed (51). Ironically, increasing fungicide load in pollen has been associated with increased probability of fungal Nosema infection in exposed bees (52).
California law defines adjuvants packaged and sold separately as pesticide products that require registration (53). Every application of a spray tank adjuvant is reported with detail that is unprecedented globally. California almond exposes most USA honey bees to highly documented pesticide and adjuvant applications and is an unique crop to assess all other agrochemical inputs in the absence of neonicotinoids, presently considered to be the primary pesticide factor associated with pollinator decline (54). There are no substantial applications of neonicotinoids to this monoculture (55), particularly when honey bees are present, and almond pollen and nectar tend to be the sole food source unless supplemental sugar feeding is employed (52). Pesticide usage information for California has been archived since 1990 in the pesticide use reporting (PUR) database maintained by the California Department of Pesticide Regulations (55). The great utility of this data for assessing environmental risks of spatial and temporal pesticide use in California almonds to aquatic organisms and earthworms has been demonstrated (56). However, our study is the first to include spray adjuvants as potentially toxic agrochemical inputs in risk evaluation.
We analyzed annual trends in applications of tank adjuvants and associated formulated products of active ingredients during almond pollination (February and March). January applications were also included since their foliar residues may pose toxicity risks for newly arriving bee colonies. Over 3.3 million records for almond applications were downloaded from PUR (55) and sorted for January to March of 2001–2013 using Microsoft Excel (Mac 2011). Only synthetic pesticides were analyzed for trends, thereby excluding bulky applications of older natural products and biologicals, such as sulfur, petroleum and mineral oils, copper salts, and microbials, since CCD was first noted in 2006, decades after major regular inputs of these natural pesticides were initiated. While overall statewide synthetic fungicide and insecticide use on almonds has not increased over this evaluation period, applications of herbicides and spray adjuvants, the latter including nutrient and buffer supplements, have doubled (Figure 1). Yearly application rates were normalized to total almond bearing acres, which increased from 530,000 in 2001 to 850,000 acres in 2013 (57), indicating that the total synthetic pesticide load has increased on almonds since the onset of CCD (Figure 1). Because herbicide applications are generally made to the understory and not to the flowering canopy where pollinator exposure is likely, we focused on actual tank adjuvant and pesticide mixes that may provide direct exposure risks for bees. Among adjuvant classes, the organosilicone surfactants pose the greatest toxicity risks for honey bees (7).
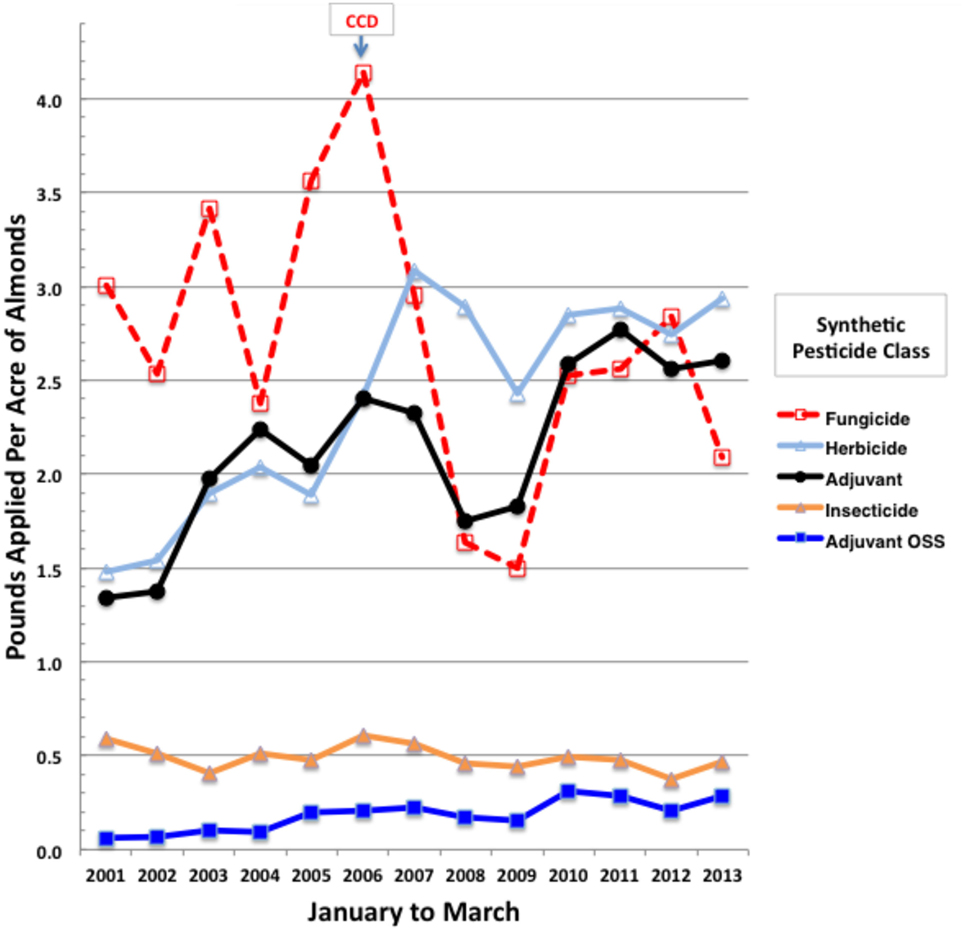
Figure 1. Total pounds of synthetic pesticides by class applied per acre of California almonds during January to March of 2001 through 2013. Yearly total almond bearing acres were from the CA Department of Food and Agriculture (57).
We then conducted a detailed analysis of temporal trends in organosilicone applications for Stanislaus Co., a major almond producing county in California (57), which had the largest number of pesticide applications over our evaluation period. PUR records (55) were sorted by date, county/meridian/township/range/section (COMTRS) location, and amount of treated almond acres. Co-occurring and synonymous records were assumed to represent combined pesticide and adjuvant products within the same tank application mix. Based on this premise, most of the spray combinations comprised, in addition to one or more pesticide formulations, at least one tank adjuvant. Focused assessment was then made out of the total number and percentages of applications containing an OSSA, which included 45 products (Table S1 in Supplementary Material) dominated by Dyne-Amic®, Syl-Tac®, Sylgard 309®, RNA Si 100®, First Choice Break-Thru®, Freeway®, Kinetic®, Multi-Spred®, Widespread Max®, and Silwet L-77®. Similar combinations of products were assigned unique tank mix codes and resorted. Almost 10,000 pesticide applications on almonds in Stanislaus Co. contained an OSSA over the years evaluated, each on average to 40 acres. The greatest increase in major agrochemical inputs observed before and after onset of CCD in 2006 was the tripling of total pesticide applications containing an OSSA from 587 in January–March 2001 to 1,781 in January–March 2006 (Figure 2A). Greater than 80% of these applications contained fungicides, followed by 10% insecticides, and 5% herbicides. Ergosterol biosynthesis inhibitor (EBI) fungicides and IGR insecticides were greatly increased, whereas herbicide and other insecticide applications were fairly static across this period (Figures 2A,B). Pristine® (a combination of boscalid and pyraclostrobin), chlorothalonil, and EBIs (propiconazole > myclobutanil > fenbuconazole > metconazole > difenoconazole) dominated the increasing trends in fungicide use at the onset of CCD (Figure 2B). The IGRs (diflubenzuron > methoxyfenozide > pyriproxyfen > tebufenozide) displayed the greatest increases among insecticides in spray tank mixes containing OSSA during the onset and continuation of CCD (Figure 2B). Concomitantly, greatest decreasing tendencies in almond pesticide applications were for other fungicides (cyprodinil, iprodione, and azoxystrobin) and the older EBI myclobutanil, while inputs of herbicides (primarily glyphosate, oxyfluorfen, and paraquat) with OSSA did not change markedly. Based on the CDPR data for agrochemical applications to California almonds during pollination, increasing adjuvant use, particularly the OSSAs, in tank mixes with fungicides, including EBIs, Pristine®, and chlorothalonil, and with IGR insecticides may be associated with recent USA honey bee declines.
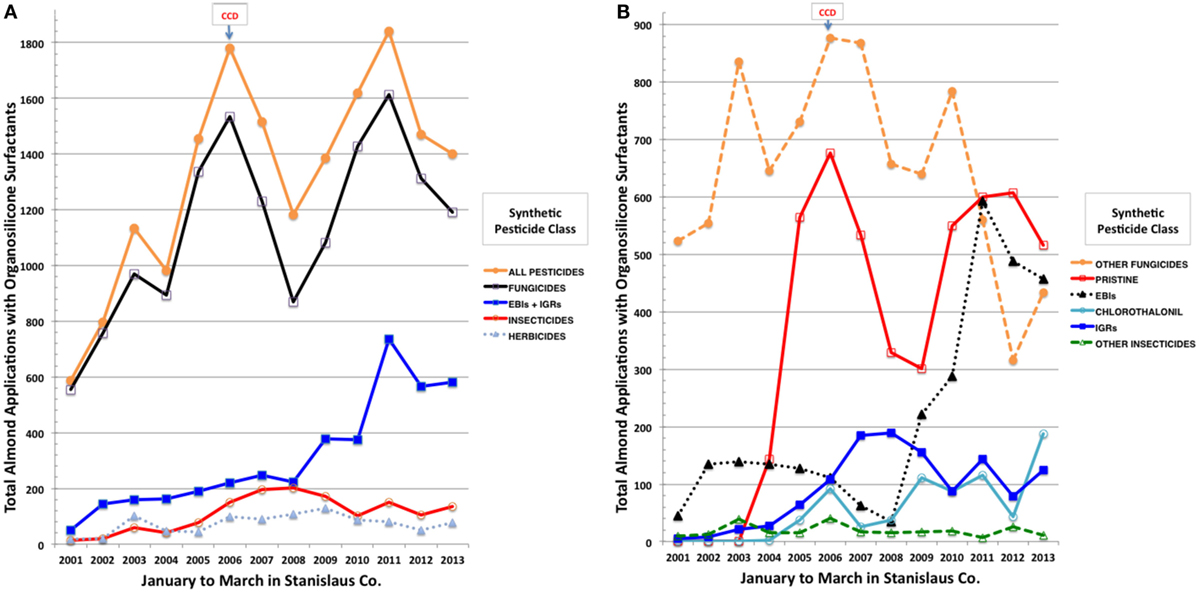
Figure 2. Total applications to almonds in Stanislaus County, CA, USA of synthetic pesticides by class (A) and by more selective class and individual pesticides (B) during January to March of 2001 through 2013 using an organsilicone surfactant.
Organosilicones: The Most Powerful Surfactants
Organosilicone surfactants are the most potent adjuvants and super-penetrants available to growers (58, 59). These polyethoxylates and those containing the nonyl- and octylphenols are widely used as non-ionic surfactants in spray adjuvants or additives in agrochemical formulations applied during bloom when bees are foraging. Organosiloxane surfactants were detected in all wax samples and 60% of pollen samples, although absent from honey (60). Their general wide occurrence as residues in beehive samples is noteworthy since spray adjuvants are not presently regulated by the EPA (61). Nonylphenol more than organosiloxane and octylphenol polyethoxylates were found in wax samples, while pollen and particularly honey residues were lower (62). Major commercial spray tank adjuvants are blends of organosilicone, nonylphenol, and octylphenol polyethoxylates, making it more difficult to associate environment residues with any specific product (63). Nevertheless, sample levels of the more abundant nonylphenol polyethoxylate residues may be used as a risk predictor for pesticide exposure because of their frequent coincidence in tank mixes of formulations and adjuvants (62). Spray tank adjuvants containing these polyethoxylates greatly influence pesticide fate (64) in pollinator or other environments, generally increasing the residue levels, of particularly fungicides (65) and herbicides (66), available to expose pollinators and other non-target species. The impact of OSSA sprays on the frequent incidence of neonicotinoid residues in bee environments (67) and their often associated roles in pollinator decline (68) may be great since the highest imidacloprid residue ever reported in pollen (7.4 ppm) was after use of Dyne-Amic® on citrus [(69), Appendix E].
Even at 10 ppm, OSSAs are good, stand-alone insecticides and miticides (7, 70), and can be more toxic to beneficial insects than the active ingredient used to control the associated pest (71). Silwet L-77® and Kinetic® are known to synergize the neonicotinoid imidacloprid used to control the psyllid vector of citrus greening disease (72). Yearly use of these potent adjuvants continues to increase, with an estimated annual global production of 1.3 billion pounds of OSSAs in 2008 among 10 billion pounds of all organosilicones (73). This is 30 times greater than the highest estimated global annual imidacloprid use of 44 million pounds (74). Silwet L-77® was the most potent endocrine disruptor among surfactants tested in a screen of 1,814 chemicals, with composite scores that placed it in the top 38 of the 465 endocrine disruptors found [(75), supplemental data], much more active than polyoxyethylene(10)nonylphenyl ether. All six neonicotinoids, including imidacloprid, were inactive in the entire battery of endocrine tests used. Organosilicone surfactants are also present in drug and personal care products, particularly shampoos (76), and thus represent an important component of the chemical landscape to which bees (32) and humans (77) are exposed. These widely used super surfactants readily move across membranes, become systemic in plants and animals, and can ultimately degrade to silica (78) causing silicosis in sensitive tissues of exposed organisms.
Are Organosilicone Surfactants Causing Harm and Underregulated?
Organosilicone surfactants are the “gold” standard for effecting solution of complex mixtures of agrochemical components of wide-ranging polarites in the spray tank. Hundreds of thousands of pounds of organosilicone adjuvants are applied every year on almonds in California alone (7, 45), both during and subsequent to bloom when bee pollinators are present. The high incidence of OSSAs in USA beehives and their ability to impair adult learning and be toxic to honey bees at all stages of development points to their great potential to harm bees and other non-target species, and yet, they are typically not even considered in the risk assessment process. It is clear that relevant pesticide risk assessment for pollinators and other non-target species cannot be addressed solely by evaluating the active ingredients without the concomitant formulation ingredients and spray tank adjuvants. Lack of risk mitigation on spray tank adjuvants presently allows major OSSA products such as Break-Thru®, Kinetic®, RNA Si 100®, Silwet Eco Spreader®, Syl-Coat®, and Widespread® to be used on any “organic” crop under a certified Organic Materials Review Institute (OMRI) label (79).
Spray adjuvants are largely assumed to be biologically inert and are not registered by EPA at the federal level in the USA (7, 55). Registration and monitoring of adjuvant use patterns are regulated at the state level in the USA, and most states do not participate in this process. To the best of our knowledge, only California, Washington, and perhaps Oregon make substantial effort to monitor use patterns or regulate these major chemical inputs into the environmental landscape. This lack of federal oversight is surprising since Department of Transportation employees of Pennsylvania and Iowa claim that herbicide applications to right-of-ways and roadways always contain a separate spray tank adjuvant (personal communications, 2015). Leaving regulation to the mandate of individual states results in a “wild west” approach that, in most cases, leaves these chemicals unaccounted for and allows for their increasing presence in our environment. Requiring regulation of spray tank adjuvants at the federal level in the USA would be a reasonable step toward addressing this problem.
While we recognize that chemical stressors alone are likely not responsible for the decline of pollinator or other non-target organisms, the true impact of chemical exposure is impossible to determine given our lack of understanding of the total chemical burden, a burden that clearly includes unknown and unevaluated materials. Coincidence of virus and pesticide exposures in declining honey bee colonies (80) is most noteworthy among other factors, which also includes malnutrition and elevated Varroa mites. More industry and regulatory agency disclosure of the identity of agrochemical adjuvant and formulation components would aid in evaluating risk and hazard assessment. Most adjuvants and inert ingredients are presently exempted from human safety tolerances, generally recognized as safe, and thus no environmental monitoring is required (7). A needed improvement is to include all formulation (81) and adjuvant (82) ingredients at relevant environmental input and exposure levels, and not just active ingredients, in studies to document the safety and risk for pollinators and other non-target species prior to product registration and commercialization.
Author Contributions
CM and MF were the primary authors and contributed substantially to the concept, design, final drafting, and primary accountability of the content of this mini review. JF and RR were key to the acquisition, analysis, and interpretation of cited data and were involved in drafting and final approval for work cited here.
Conflict of Interest Statement
The authors declare that the research was conducted in the absence of any commercial or financial relationships that could be construed as a potential conflict of interest.
Acknowledgments
The authors thank our previous graduate students Timothy J. Ciarlo and Wanyi Zhu, and postdoctoral Jing Chen for their research efforts included here. Funding for this work was provided by the USDA-NIFA-AFRI Coordinated Agricultural Project and Foundational Award Programs, particularly nos. 2011-67013-30137 and 2014-67013-21591.
Supplementary Material
The Supplementary Material for this article can be found online at http://journal.frontiersin.org/article/10.3389/fpubh.2016.00092
Abbreviations
AI, active ingredient; CCD, colony collapse disorder; EBI, ergosterol biosynthesis inhibitor; EC, emulsifiable concentrate; IGR, insect growth regulator; NMP, N-methyl-2-pyrrolidone; OSSA, organosilicone surfactant adjuvant; ppm, part per million.
References
1. Green JM, Beestman GB. Recently patented and commercialized formulation and adjuvant technology. Crop Prot (2007) 26(3):320–7. doi: 10.1016/j.cropro.2005.04.018
2. Hazen JL. Adjuvants – terminology, classification, and chemistry. Weed Technol (2000) 14(4):773–84. doi:10.1614/0890-037X(2000)014[0773:ATCAC]2.0.CO;2
3. Green JM. Adjuvant outlook for pesticides. Pestic Outlook (2000) 11(5):196–9. doi:10.1039/b008021g
4. Ryckaert B, Spanoghe P, Heremans B, Haesaert G, Steurbaut W. Possibilities to use tank-mix adjuvants for better fungicide spreading on triticale ears. J Agric Food Chem (2008) 56(17):8041–4. doi:10.1021/jf8005257
5. Karande P, Jain A, Ergun K, Kispersky V, Mitragotri S. Design principles of chemical penetration enhancers for transdermal drug delivery. Proc Natl Acad Sci U S A (2005) 102(13):4688–93. doi:10.1073/pnas.0501176102
6. Whitehead K, Karr N, Mitragotri S. Safe and effective permeation enhancers for oral drug delivery. Pharm Res (2008) 25(8):1782–8. doi:10.1007/s11095-007-9488-9
7. Mullin CA, Chen J, Fine JD, Frazier MT, Frazier JL. The formulation makes the honey bee poison. Pestic Biochem Physiol (2015) 120:27–35. doi:10.1016/j.pestbp.2014.12.026
8. Holloway PJ, Rees RT, Stock D. Interactions Between Adjuvants, Agrochemicals and Target Organisms. Berlin: Springer-Verlag (1994). 195 p.
9. Surgan M, Condon M, Cox C. Pesticide risk indicators: unidentified inert ingredients compromise their integrity and utility. Environ Manag (2010) 45(4):834–41. doi:10.1007/s00267-009-9382-9
10. Krogh KA, Halling-Sorensen B, Mogensen BB, Vejrup KV. Environmental properties and effects of nonionic surfactant adjuvants in pesticides: a review. Chemosphere (2003) 50(7):871–901. doi:10.1016/S0045-6535(02)00648-3
11. Howe CM, Berrill M, Pauli BD, Helbing CC, Werry K, Veldhoen N. Toxicity of glyphosate-based pesticides to four North American frog species. Environ Toxicol Chem (2004) 23(8):1928–38. doi:10.1897/03-71
12. Mesnage R, Defarge N, de Vendomois JS, Seralini GE. Potential toxic effects of glyphosate and its commercial formulations below regulatory limits. Food Chem Toxicol (2015) 84:133–53. doi:10.1016/j.fct.2015.08.012
13. Williams GM, Kroes R, Munro IC. Safety evaluation and risk assessment of the herbicide roundup and its active ingredient, glyphosate, for humans. Regul Toxicol Pharmacol (2000) 31(2):117–65. doi:10.1006/rtph.1999.1371
14. Mesnage R, Bernay B, Seralini GE. Ethoxylated adjuvants of glyphosate-based herbicides are active principles of human cell toxicity. Toxicology (2013) 313(2–3):122–8. doi:10.1016/j.tox.2012.09.006
15. Nobels I, Spanoghe P, Haesaert G, Robbens J, Blust R. Toxicity ranking and toxic mode of action evaluation of commonly used agricultural adjuvants on the basis of bacterial gene expression profiles. PLoS One (2011) 6(11):e24139. doi:10.1371/journal.pone.0024139
16. Ma J, Qin W, Lu N, Wang P, Huang C, Xu R. Differential sensitivity of three cyanobacteria (Anabaena flos-aquae, Microcystis flos-aquae and Mirocystis aeruginosa) to 10 pesticide adjuvants. Bull Environ Contam Toxicol (2005) 75(5):873–81. doi:10.1007/s00128-005-0831-8
17. Ma JY, Lin FC, Zhang RZ, Yu WW, Lu NH. Differential sensitivity of two green algae, Scenedesmus quadricauda and Chlorella vulgaris, to 14 pesticide adjuvants. Ecotox Environ Saf (2004) 58:61–7. doi:10.1016/j.ecoenv.2003.08.023
18. Coutellec MA, Delous G, Cravedi JP, Lagadic L. Effects of the mixture of diquat and a nonylphenol polyethoxylate adjuvant on fecundity and progeny early performances of the pond snail Lymnaea stagnalis in laboratory bioassays and microcosms. Chemosphere (2008) 73(3):326–36. doi:10.1016/j.chemosphere.2008.05.068
19. Deardorff AD, Stark JD. Acute toxicity and hazard assessment of spinosad and R-11 to three Cladoceran species and Coho salmon. Bull Environ Contam Toxicol (2009) 82(5):549–53. doi:10.1007/s00128-009-9643-6
20. Chen XD, Culbert E, Hebert V, Stark JD. Mixture effects of the nonylphenyl polyethoxylate, R-11 and the insecticide, imidacloprid on population growth rate and other parameters of the crustacean, Ceriodaphnia dubia. Ecotox Environ Saf (2010) 73(2):132–7. doi:10.1016/j.ecoenv.2009.09.016
21. Stark JD, Walthall WK. Agricultural adjuvants: acute mortality and effects on population growth rate of Daphnia pulex after chronic exposure. Environ Toxicol Chem (2003) 22(12):3056–61. doi:10.1897/02-504
22. Henry CJ, Higgins KF, Buhl KJ. Acute toxicity and hazard assessment of Rodeo®, X-77 spreader®, and Chem-Trol® to aquatic invertebrates. Arch Environ Contam Toxicol (1994) 27(3):392–9. doi:10.1007/BF00213176
23. Tatum VL, Borton DL, Streblow WL, Louch J, Shepard JP. Acute toxicity of commonly used forestry herbicide mixtures to Ceriodaphnia dubia and Pimephales promelas. Environ Toxicol (2011) 27(12):671–84. doi:10.1002/tox.20686
24. Haller WT, Stocker RK. Toxicity of 19 adjuvants to juvenile Lepomis macrochirus (bluegill sunfish). Environ Toxicol Chem (2003) 22(3):615–9. doi:10.1002/etc.5620220321
25. Sanchez W, Palluel O, Lagadic L, Ait-Aissa S, Porcher JM. Biochemical effects of nonylphenol polyethoxylate adjuvant, Diquat herbicide and their mixture on the three-spined stickleback (Gasterosteus aculeatus L.). Mar Environ Res (2006) 62:S29–33. doi:10.1016/j.marenvres.2006.04.028
26. Mann RM, Bidwell JR. The acute toxicity of agricultural surfactants to the tadpoles of four Australian and, two exotic frogs. Environ Pollut (2001) 114(2):195–205. doi:10.1016/S0269-7491(00)00216-5
27. Matteson JW, Taft HM. Effect of various adjuvants on systemic insecticidal activity of Phorate and Zectran. J Econ Entomol (1964) 57(3):325–6. doi:10.1093/jee/57.3.325
28. Sims SR, Appel AG. Linear alcohol ethoxylates: insecticidal and synergistic effects on German cockroaches (Blattodea: Blattellidae) and other insects. J Econ Entomol (2007) 100(3):871–9. doi:10.1093/jee/100.3.871
29. Schlenk D, Lavado R, Loyo-Rosales JE, Jones W, Maryoung L, Riar N, et al. Reconstitution studies of pesticides and surfactants exploring the cause of estrogenic activity observed in surface waters of the San Francisco bay delta. Environ Sci Technol (2012) 46(16):9106–11. doi:10.1021/es3016759
30. Lin N, Garry VF. In vitro studies of cellular and molecular developmental toxicity of adjuvants, herbicides, and fungicides commonly used in Red River Valley, Minnesota. J Toxicol Environ Hlth A (2000) 60(6):423–39. doi:10.1080/00984100050033494
31. Bakke D. Human and ecological risk assessment of nonylphenol polyethoxylate-based (NPE) surfactants in Forest Service herbicide applications. USDA Forest Service Report. Vallejo, CA: Pacific Southwest Region, USDA Forest Service (2003). 110 p.
32. Mullin CA. Effects of “inactive” ingredients on bees. Curr Opin Insect Sci (2015) 10:194–200. doi:10.1016/j.cois.2015.05.006
33. Del Sarto MCL, Oliveira EE, Guedes RNC, Campos LAO. Differential insecticide susceptibility of the Neotropical stingless bee Melipona quadrifasciata and the honey bee Apis mellifera. Apidologie (2014) 45(5):626–36. doi:10.1007/s13592-014-0281-6
34. Zhao S, Yuan S-K, Cai B, Jiang H, Wang X-J, Lin R-H, et al. The acute oral toxicity of 300 formulated pesticides to Apis mellifera. Agrochemicals (2011) 5:278–80.
35. Boily M, Sarrasin B, DeBlois C, Aras P, Chagnon M. Acetylcholinesterase in honey bees (Apis mellifera) exposed to neonicotinoids, atrazine and glyphosate: laboratory and field experiments. Environ Sci Pollut Res (2013) 20(8):5603–14. doi:10.1007/s11356-013-1568-2
36. van den Heever JP, Thompson TS, Curtis JM, Pernal SF. Determination of dicyclohexylamine and fumagillin in honey by LC-MS/MS. Food Anal Methods (2015) 8(3):767–77. doi:10.1007/s12161-014-9956-x
37. Moffett JO, Morton HL. Repellency of surfactants to honey bees. Environ Entomol (1975) 4(5):780–2. doi:10.1093/ee/4.5.780
38. Goodwin RM, McBrydie HM. Effect of surfactants on honey bee survival. N Z Pl Prot (2000) 53:230–4.
39. Donovan BJ, Elliott GS. Honey bee response to high concentrations of some new spray adjuvants. N Z Pl Prot (2001) 54:51–5.
40. Artz DR, Pitts-Singer TL. Effects of fungicide and adjuvant sprays on nesting behavior in two managed solitary bees, Osmia lignaria and Megachile rotundata. PLoS One (2015) 10(8):e0135688. doi:10.1371/journal.pone.0135688
41. Johnson RM, Percel EG. Effect of a fungicide and spray adjuvant on queen-rearing success in honey bees (Hymenoptera: Apidae). J Econ Entomol (2013) 106(5):1952–7. doi:10.1603/ec13199
42. Oliver R. The case of Pristine® and the dying queen cells – pesticide mystery solved? Amer Bee J (2013) 153(3):287–93.
43. Mommaerts V, Hagenaars A, Meyer J, De Coen W, Swevers L, Mosallanejad H, et al. Impact of a perfluorinated organic compound PFOS on the terrestrial pollinator Bombus terrestris (Insecta, Hymenoptera). Ecotoxicology (2011) 20(2):447–56. doi:10.1007/s10646-011-0596-2
44. Zhu WY, Schmehl DR, Mullin CA, Frazier JL. Four common pesticides, their mixtures and a formulation solvent in the hive environment have high oral toxicity to honey bee larvae. PLoS One (2014) 9(1):e77547. doi:10.1371/journal.pone.0077547
45. Ciarlo TJ, Mullin CA, Frazier JL, Schmehl DR. Learning impairment in honey bees caused by agricultural spray adjuvants. PLoS One (2012) 7(7):e40848. doi:10.1371/journal.pone.0040848
46. Kaiser H. Stomatal uptake of mineral particles from a sprayed suspension containing an organosilicone surfactant. J Pl Nutr Soil Sci (2014) 177(6):869–74. doi:10.1002/jpln.201300607
47. Lizamore D, Winefield C. The addition of an organosilicone surfactant to Agrobacterium suspensions enables efficient transient transformation of in vitro grapevine leaf tissue at ambient pressure. Plant Cell Tiss Organ Cult (2015) 120(2):607–15. doi:10.1007/s11240-014-0627-9
48. Staveley JP, Law SA, Fairbrother A, Menzie CA. A causal analysis of observed declines in managed honey bees (Apis mellifera). Hum Ecol Risk Assess (2014) 20(2):566–91. doi:10.1080/10807039.2013.831263
49. Steinhauer NA, Rennich K, Wilson ME, Caron DM, Lengerich EJ, Pettis JS, et al. A national survey of managed honey bee 2012-2013 annual colony losses in the USA: results from the bee informed partnership. J Apic Res (2014) 53(1):1–18. doi:10.3896/ibra.1.53.1.01
50. Lee KV, Steinhauer N, Rennich K, Wilson ME, Tarpy DR, Caron DM, et al. A national survey of managed honey bee 2013-2014 annual colony losses in the USA. Apidologie (2015) 46(3):292–305. doi:10.1007/s13592-015-0356-z
51. Frazier M, Mullin C, Frazier J, Ashcraft S, Leslie E, Mussen E, et al. Assessing honey bee (Hymenoptera: Apidae) foraging populations and the potential impact of pesticides on eight U. S. crops. J Econ Entomol (2015) 108(4):2141–52. doi:10.1093/jee/tov195
52. Pettis JS, Lichtenberg EM, Andree M, Stitzinger J, Rose R, VanEngelsdorp D. Crop pollination exposes honey bees to pesticides which alters their susceptibility to the gut pathogen Nosema ceranae. PLoS One (2013) 8(7):e70182. doi:10.1371/journal.pone.0070182
53. California Department of Pesticide Regulation, California Pesticide Information Portal (CALPIP). (2015). Available from: http://calpip.cdpr.ca.gov/infodocs.cfm?page=knownissues
54. Lundin O, Rundlof M, Smith HG, Fries I, Bommarco R. Neonicotinoid insecticides and their impacts on bees: a systematic review of research approaches and identification of knowledge gaps. PLoS One (2015) 10(8):e0136928. doi:10.1371/journal.pone.0136928
55. California Department of Pesticide Regulation, California Pesticide Information Portal (CALPIP). (2015). Available from: http://calpip.cdpr.ca.gov/main.cfm
56. Zhan Y, Zhang MH. Spatial and temporal patterns of pesticide use on California almonds and associated risks to the surrounding environment. Sci Total Environ (2014) 472:517–29. doi:10.1016/j.scitotenv.2013.11.022
57. California Department of Food and Agriculture. 2014 California Almond Acreage Report. (2015). 8 p. Available from: http://www.nass.usda.gov/Statistics_by_State/California/Publications/Fruits_and_Nuts/index.php
58. Stevens PJG. Organosilicone surfactants as adjuvants for agrochemicals. Pestic Sci (1993) 38(2–3):103–22. doi:10.1002/ps.2780380206
59. Penner D. Activator adjuvants. Weed Technol (2000) 14(4):785–91. doi:10.1614/0890-037X(2000)014[0785:AA]2.0.CO;2
60. Chen J, Mullin CA. Quantitative determination of trisiloxane surfactants in beehive environments based on liquid chromatography coupled to mass spectrometry. Environ Sci Technol (2013) 47(16):9317–23. doi:10.1021/es4010619
61. US EPA. Pesticide Registration: Inert Ingredients Overview and Guidance. (2015). Available from: http://www2.epa.gov/pesticide-registration/inert-ingredients-overview-and-guidance
62. Chen J, Mullin CA. Determination of nonylphenol ethoxylate and octylphenol ethoxylate surfactants in beehive samples by high performance liquid chromatography coupled to mass spectrometry. Food Chem (2014) 158:473–9. doi:10.1016/j.foodchem.2014.03.004
63. Chen J, Mullin CA. Characterization of trisiloxane surfactants from agrochemical adjuvants and pollinator-related matrices using liquid chromatography coupled to mass spectrometry. J Agric Food Chem (2015) 63(12):5120–5. doi:10.1021/jf505634x
64. Katagi T. Surfactant effects on environmental behavior of pesticides. In: Whitacre DM, editor. Reviews of Environmental Contamination and Toxicology. (Vol. 194), New York: Springer (2008). p. 71–177.
65. Ryckaert B, Spanoghe P, Haesaert G, Heremans B, Isebaert S, Steurbaut W. Quantitative determination of the influence of adjuvants on foliar fungicide residues. Crop Prot (2007) 26(10):1589–94. doi:10.1016/j.cropro.2007.02.011
66. Holloway PJ, Western NM. Tank-mix adjuvants and pesticide residues: some regulatory and quantitative aspects. Pest Mgt Sci (2003) 59(11):1237–44. doi:10.1002/ps.761
67. Botias C, David A, Horwood J, Abdul-Sada A, Nicholls E, Hill E, et al. Neonicotinoid residues in wildflowers, a potential route of chronic exposure for bees. Environ Sci Technol (2015) 49(21):12731–40. doi:10.1021/acs.est.5b03459
68. Bonmatin JM, Giorio C, Girolami V, Goulson D, Kreutzweiser DP, Krupke C, et al. Environmental fate and exposure; neonicotinoids and fipronil. Environ Sci Pollut Res (2015) 22(1):35–67. doi:10.1007/s11356-014-3332-7
69. US EPA. Imidacloprid Preliminary Pollinator Assessment Table of Appendices. (2016). Available from: http://www.regulations.gov/#!documentDetail;D=EPA-HQ-OPP-2008-0844-0139
70. Cowles RS, Cowles EA, McDermott AM, Ramoutar D. “Inert” formulation ingredients with activity: toxicity of trisiloxane surfactant solutions to twospotted spider mites (Acari: Tetranychidae). J Econ Entomol (2000) 93(2):180–8. doi:10.1603/0022-0493-93.2.180
71. Acheampong S, Stark JD. Effects of the agricultural adjuvant Sylgard 309 and the insecticide pymetrozine on demographic parameters of the aphid parasitoid, Diaeretiella rapae. Biol Control (2004) 31(2):133–7. doi:10.1016/j.biocontrol.2004.03.010
72. Srinivasan R, Hoy MA, Singwand R, Rogers ME. Laboratory and field evaluations of Silwet L-77 and kinetic alone and in combination with imidacloprid and abamectin for the management of the Asian citrus psyllid, Diaphorina citri (Hemiptera: Psyllidae). Fl Entomologist (2008) 91(1):87–100. doi:10.1653/0015-4040(2008)091[0087:LAFEOS]2.0.CO;2
73. Rücker C, Kümmerer K. Environmental chemistry of organosiloxanes. Chem Rev (2015) 115(1):466–524. doi:10.1021/cr500319v
74. Simon-Delso N, Amaral-Rogers V, Belzunces LP, Bonmatin JM, Chagnon M, Downs C, et al. Systemic insecticides (neonicotinoids and fipronil): trends, uses, mode of action and metabolites. Environ Sci Pollut Res (2015) 22(1):5–34. doi:10.1007/s11356-014-3470-y
75. Rotroff DM, Martin MT, Dix DJ, Filer DL, Houck KA, Knudsen TB, et al. Predictive endocrine testing in the 21st century using in vitro assays of estrogen receptor signaling responses. Environ Sci Technol (2014) 48(15):8706–16. doi:10.1021/es502676e
76. Barel AO, Paye M, Maibach HI. Handbook of Cosmetic Science and Technology. 3rd ed. New York: Informa Healthcare (2009). 869 p.
77. Bouslimania A, Porto C, Rath CM, Wang M, Guo Y, Gonzalez A, et al. Molecular cartography of the human skin surface in 3D. Proc Natl Acad Sci U S A (2015) 112:E2120–9. doi:10.1073/pnas.1424409112
78. Laubie B, Bonnafous E, Desjardin V, Germain P, Fleury E. Silicone-based surfactant degradation in aqueous media. Sci Total Environ (2013) 454:199–205. doi:10.1016/j.scitotenv.2013.02.022
79. OMRI. OMRI Product List. (2015). Available from: http://www.omri.org/omri-lists
80. Simon-Delso N, San Martin G, Bruneau E, Minsart LA, Mouret C, Hautier L. Honeybee colony disorder in crop areas: the role of pesticides and viruses. PLoS One (2014) 9(7):e103073. doi:10.1371/journal.pone.0103073
81. Poquet Y, Bodin L, Tchamitchian M, Fusellier M, Giroud B, Lafay F, et al. A pragmatic approach to assess the exposure of the honey bee (Apis mellifera) when subjected to pesticide spray. PLoS One (2014) 9(11):e113728. doi:10.1371/journal.pone.0113728
Keywords: adjuvant, agrochemical formulation, organosilicone surfactant, non-target effects, spray tank mix
Citation: Mullin CA, Fine JD, Reynolds RD and Frazier MT (2016) Toxicological Risks of Agrochemical Spray Adjuvants: Organosilicone Surfactants May Not Be Safe. Front. Public Health 4:92. doi: 10.3389/fpubh.2016.00092
Received: 20 November 2015; Accepted: 25 April 2016;
Published: 11 May 2016
Edited by:
Robin Mesnage, King’s College London, UKReviewed by:
Johann G. Zaller, University of Natural Resources and Life Sciences, AustriaAnton Safer, Heidelberg University, Germany
Copyright: © 2016 Mullin, Fine, Reynolds and Frazier. This is an open-access article distributed under the terms of the Creative Commons Attribution License (CC BY). The use, distribution or reproduction in other forums is permitted, provided the original author(s) or licensor are credited and that the original publication in this journal is cited, in accordance with accepted academic practice. No use, distribution or reproduction is permitted which does not comply with these terms.
*Correspondence: Christopher A. Mullin, Y2FtdWxsaW5AcHN1LmVkdQ==