- 1Metabolism, Diabetes and Obesity Programme, Monash Biomedicine Discovery Institute, Monash University, Clayton, VIC, Australia
- 2Woodrudge LTD, London, United Kingdom
Nine out of 10 people breathe air that does not meet World Health Organization pollution limits. Air pollutants include gasses and particulate matter and collectively are responsible for ~8 million annual deaths. Particulate matter is the most dangerous form of air pollution, causing inflammatory and oxidative tissue damage. A deeper understanding of the physiological effects of particulate matter is needed for effective disease prevention and treatment. This review will summarize the impact of particulate matter on physiological systems, and where possible will refer to apposite epidemiological and toxicological studies. By discussing a broad cross-section of available data, we hope this review appeals to a wide readership and provides some insight on the impacts of particulate matter on human health.
Introduction
In preindustrial civilizations, domestic combustion of biomass was the primary source of hazardous anthropogenic air pollution; the lungs of exhumed Ancient Egyptian mummies have been found blackened by it (1, 2). In Airs, waters and places written around the 4th century BCE, Hippocrates considered the relationship between air quality and health, whilst in the 1st century AD, Seneca The Younger wrote about the health benefits of escaping Rome's “ruinous mess of steam and soot” (3, 4). In response to Queen Eleanor's objections to the “unendurable smoke” emitted from bituminous sea coal fires, English Parliament passed the Smoke Abatement Law in 1273 (5). Nonetheless, London's air quality remained poor for centuries and was made significantly worse by the 12-fold increase in coal consumption during the Industrial Revolution (6). In Bleak House, Charles Dickens described the environment as “…Smoke lowering down from chimney-pots, making a soft black drizzle, with flakes of soot in it as big as full-grown snow-flakes gone into mourning, one might imagine, for the death of the sun…” (7). As well as increased respiratory disease risk, air pollution in Victorian Britain served as a selection pressure for peppered moth pigmentation. Here, predators could easily distinguish light-colored wings set against surfaces blackened by soot, and so over time darker wings were selected for and became the most common phenotype (8). After World War II, mass-export of high-quality coal left Londoners with little choice but to burn low-quality, high-sulfur lignite. In December 1952, cold weather increased coal use and a patch of high air pressure prevented dissipation of the soot and sulfur dioxide filled smoke (9). Within 5 days, The Great Smog of London killed around 12,000 people and precipitated the implementation of the UK Clean Air Act 1956, which was shortly followed by similar legislation in Europe and North America. In 1950, Europe and the United States contributed 85% of global CO2 emissions and by 2000 this figure was closer to 50% (10). Throughout the second half of the 20th century and beyond, industrialization of the Global South resulted in East Asia and South-East Asia becoming major contributors to global emissions (10). Today, of the 10 countries with the highest pollution-associated death rates, two are in South-East Asia (Bangladesh and India), two are in Europe (Georgia and Bosnia and Herzegovina) and six are in Africa (Chad, Nigeria, Somalia, Sierra Leone, Cote d Ivoire and Central African Republic) (11). In these countries (and many others) morbidity and mortality are driven by high population densities, traffic emissions, coal-fueled power stations and domestic use of biomass (12). Global socioeconomic inequity is a critical driver of air pollution-associated deaths; low- and middle-income countries account for 91% of the 8 million annual air pollution-associated deaths (12). This review provides an overview of the multiple physiological effects of particulate matter (PM) air pollution, including those on the respiratory cardiovascular, metabolic, endocrine, neurological and reproductive systems. The authors' intent has been to summarize a combination of recent and significant work in this growing research field.
Particulate Matter
Particulate matter (PM) are solid compounds suspended in air that are sufficiently small to be inhaled (Figure 1). PM is categorized by particle diameter (measured in μm); PM0.1, PM2.5 and PM10 whilst ambient concentration is usually quantified as μg/m3. Some PM are of natural origin (bushfires, dust, sea spray, aerosols, etc.) but anthropogenic PM (diesel, coal and biomass combustion and emissions from metal refineries etc.) are the most dangerous to health (13). High atmospheric concentrations of human-made PM, and toxic and oxidative chemical characteristics render them disproportionately hazardous (13). Elemental and complex chemical species of PM are diverse, with surface shape, chemistry and charge impacted by emission source and environmental conditions. PM chemistry can change through reactions with other airborne PM and be affected by the oxidative effects of ozone and low ambient pH (14, 15).
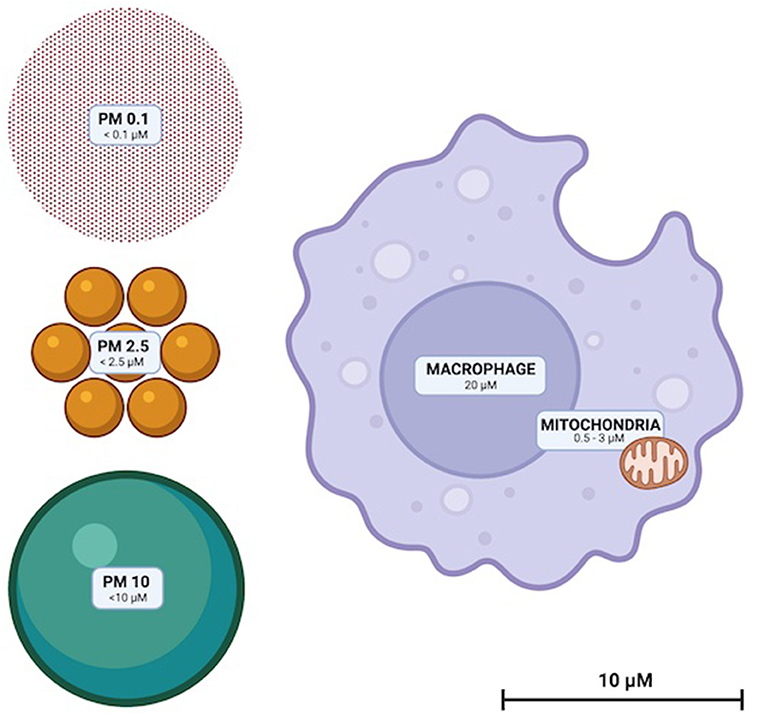
Figure 1. To scale illustration of the relative sizes of PM10, PM2.5, and PM0.1. Representative macrophage and mitochondria are included to scale for reference.
In air sampled from 187 counties in the USA between 2000 and 2005, 52 PM2.5 species were identified (16). Of this number, only seven species made up 83% of total PM mass (Figure 2). PM composition was found to be 28% organic carbon, 26%, sulfate, 12% nitrate, 11% ammonia, 5% elemental carbon, 1% silicon and 1% sodium (16). Components of the remaining 17% included alkali metals (K, Cs & Rb), alkaline earth metals (Ca, B, Mg & Sr), transition metals (Fe, Zn, Hf, Ta, V, Cd, Ti, Ag, Cu, Ir, M, Mn, Au, Mg, Hg, W, Sc, Cr, Nb, Zr, Ni, Y, and Co) basic metals, (Al, Sn, In, Pb, and Ga) semimetals (Sb), non-metals (P and Se), halogens (Cl and Br) and lanthanides (Ce, La, Eu, Tb, Sm and As) (16).
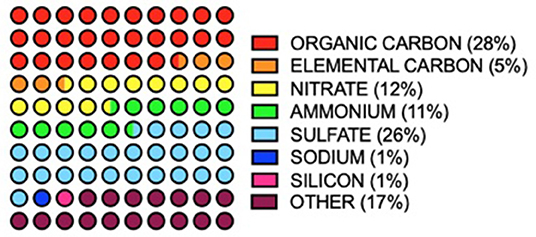
Figure 2. Annual averages of major PM2.5 components (>1 total mass%) in 187 USA counties between 2000 and 2005. Values from (16).
PM Exposure
When inhaled, larger diameter PM (>PM10) is limited to the upper airway whilst smaller particulates (< PM2.5) can access alveoli (Figure 3). PM2.5 can also cross respiratory endothelium, enter capillaries and systemic circulation thereafter (17). From the blood, PM2.5 can once again translocate endothelia once more and get into multiple extrapulmonary organs. In addition to inhalation exposure, particulates can enter circulation by crossing olfactory epithelia and via the gastrointestinal tract when swallowed after mucociliary removal from the lungs (18, 19). PM can damage endothelial cell layers as well as cross them; PM2.5 has been shown to compromise nasal epithelia intercellular tight junctions and reduce trans-epithelial resistance (20). A study in humans using gold nanoparticles as an inert proxy for PM demonstrated particles in the circulation as early as 6 h post inhalation (21). Nanoparticles were also detectable in the liver and in aortic atherosclerotic plaques and were detectable in participants' blood and urine for 3 months (21). In humans and animals PM has been found in multiple organs including the liver, kidneys and brain (17, 22–24).
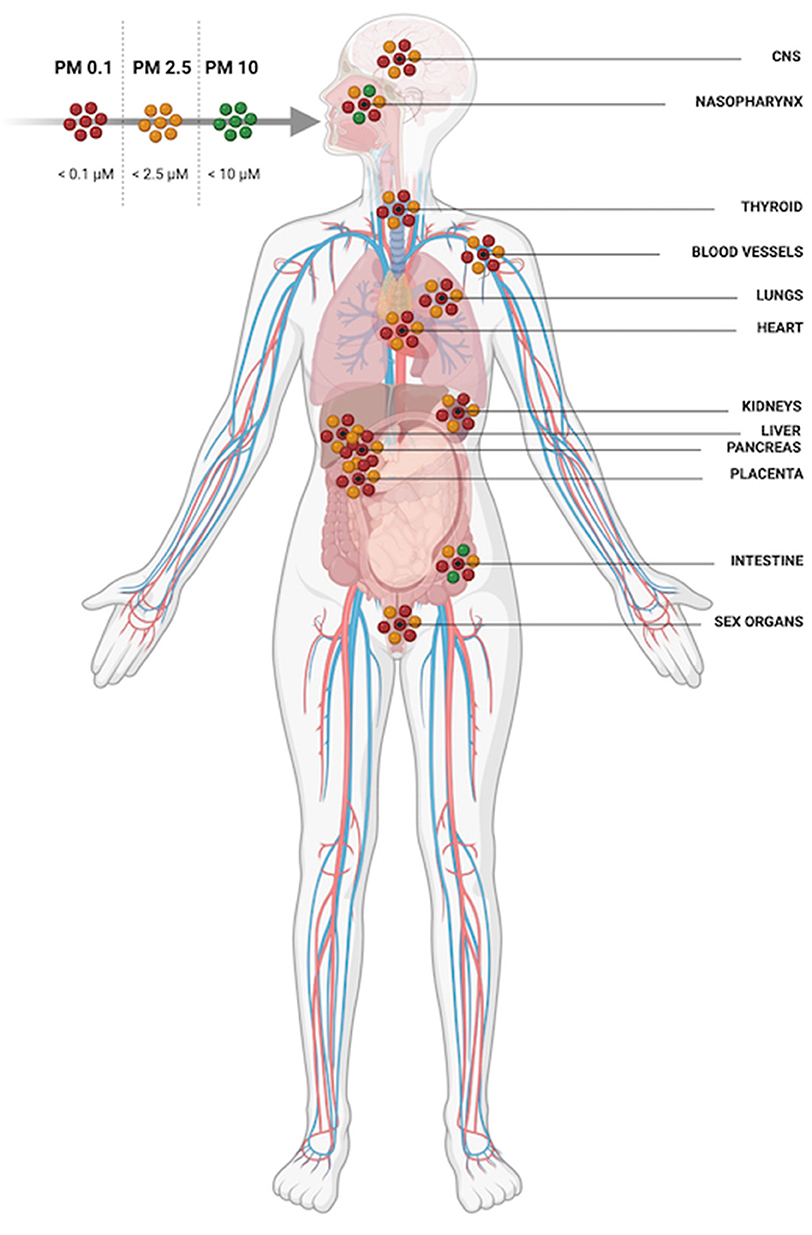
Figure 3. PM 10 is restricted to the upper airway and digestive system whilst PM2.5 and PM0.1 can be inhaled deeply into the lungs, translocate epithelial barriers and gain access to multiple organ systems.
Disease Mechanisms
According to the World Health Organization, air pollution and climate change are the collective No. 1 threat to human health (25). Air pollution contributes to 9% of all global human deaths, and of these, 58% are from ischemic heart disease and cerebrovascular disease, 18% are from chronic obstructive pulmonary disease and acute lower respiratory tract infections, 6% are from lung cancer. Causes of death in the remaining 18% are mixed and many (12). Not all PM equally toxic, with the pathophysiological mechanisms varying between PM species (26). PM are mutagenic, can cause oxidative damage, activate inflammatory signal cascades and induce cell death (27–30). Toxicological research has investigated the differential oxidative and inflammatory effects of PM species (including black carbon, ammonia, nitrate and sulfate) and PM of varying origin (13). A common anthropogenic source of PM is incomplete combustion of diesel, gasoline, coal, and biomass (13). Trace metal content significantly contributes to the oxidative potential of PM (13, 26). The oxidative effects of PM can damage mitochondria, endoplasmic reticulum and DNA, can be carcinogenic and activate cell death signaling pathways (26). Inside cells, iron-based PM can overwhelm superoxide dismutase and glutathione peroxidase activity, inducing ferroptosis (29). PM2.5 can activate cytokine-dependent autophagy pathways, signaling through toll-like receptors, the Janus kinase-signal transducer and activator of transcription (JAK-STAT) pathway, and via cyclooxygenase 2-mitochondrial and prostaglandin E synthase. Here, increased tissue levels of C-reactive protein, tumor necrosis factor-α, interleukins 1, 6 and 8 (31–34). Inflammatory, oxidative, and toxic mechanisms are the primary effectors of PM-induced cell damage. Tissue-specific pathophysiology is an important determining factor in health outcomes, is discussed below and outlined in Figure 4.
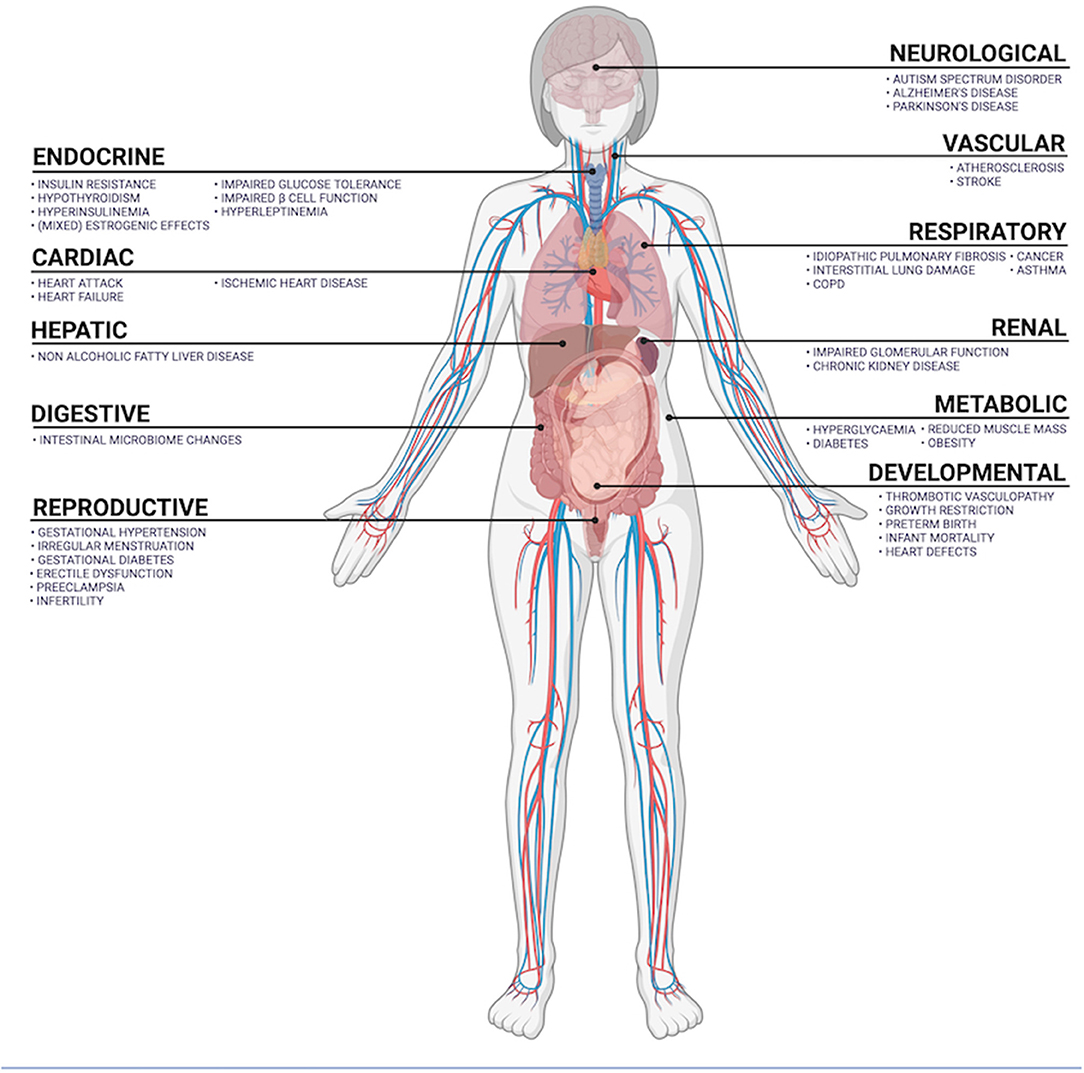
Figure 4. Summary of organ systems exposed to airborne PM and diseases positively correlated with PM exposure.
Respiratory Disease
The lungs are the primary site of PM-induced pathophysiology and best characterized in terms of the effects of PM exposure. Each 10 μg/m3 increase in ambient PM10 has been linked to a 0.58% increase in respiratory mortality, whist the same increase of PM2.5 has been associated with a 2.07% increase in respiratory disease hospitalization (35, 36). Research has shown human exposure to PM to be associated with multiple respiratory diseases including chronic obstructive pulmonary disease, asthma, interstitial lung damage and lung cancers (37–39). For patients with idiopathic pulmonary fibrosis, PM exposure has been shown to correlate with reduced lung forced vital capacity (39).
Ex vivo analysis of mouse lungs exposed to PM2.5 for three months exhibited significantly elevated levels of PM2.5, carbon monoxide, nitrogen oxides, interleukin-4, tumor necrosis factor-α and transforming growth factor-β1 when compared to controls (40). Of these circulating factors, interleukin-4 is known to promote B lymphocyte production of immunoglobulin E; a driver of allergic diseases including asthma and chronic obstructive pulmonary disease (41). PM2.5 exposure has been shown to induce pulmonary fibrosis both in vivo and in vitro experiments (42). PM2.5 increased tissue concentrations of transforming growth factor-β1; a fibroblast chemokine that can decrease protease secretion and increase extracellular expression of collagen and fibronectin (43). In a mouse model of idiopathic pulmonary fibrosis, ex vivo histological analysis revealed that exposure to black carbon PM2.5 aggravated lung inflammation and exacerbated histopathological changes to lung tissue including increased inflammatory cell infiltration and epithelial cell hyperplasia (44). This study also found that exposure to black carbon PM2.5 exacerbated already elevated interleukin-6 mRNA and reduced interferon-γ mRNA expression (44). Together, these preclinical studies not only highlight the potential danger of PM to health but also suggest that PM can increase the severity of existing health conditions like idiopathic pulmonary fibrosis. Increased counts of neutrophils, lymphocytes, eosinophils, M1 and M2 macrophages have been found in PM-exposed lung tissue (40). Whilst M1 macrophages can induce oxidative damage to lung epithelia, chronic elevation of M2 macrophages can cause pulmonary fibrosis and lung cancer (45–47). Mechanisms by which PM may induce fibrosis include increased intracellular edema, microvilli density, lamellar bodies and the density of macrophages containing endocytosed PM (40). In mice, PM2.5 exposure reduced mitochondrial density, increased NADPH oxidase 2 expression, significantly reduced total lung capacity, inspiratory capacity, and lung compliance (48). This same study found that PM2.5 exposure increased lung epithelia expression of N-Cadherin and reduced that of E-Cadherin; markers of epithelial-mesenchymal transition, a process common to cancer metastasis (48).
Cardiovascular Disease
Air pollution is associated with elevated cardiovascular disease risk and cardiovascular disease-related mortality (49). PM2.5 exposure is linked to higher risk of heart attack, heart failure, ischemic heart disease, stroke, atherosclerosis, arrhythmia, hypertension, preeclampsia and neonatal hypertension (50–52). Air pollution exacerbates cardiovascular mortality risk for people with pre-existing cardiopulmonary disease (49). In adults, exposure to PM exposure has been linked to elevated systolic blood pressure and elevated pulse pressure, whilst in children, it has been found to associate with increased mean pulmonary arterial pressure and increased plasma endothelin-1 concentration (53, 54). Endothelin-1 is an endogenous atherogenic vasoconstrictor and may contribute to PM induced atherosclerotic plaque accumulation (55). The Multi Ethnic Study of Atherosclerosis (MESA) found short-term PM2.5 exposure to associate with to decreased flow-mediated vasodilation and vasoconstriction, indicating that particulates may impair endothelial function (56). Analysis of the same MESA cohort revealed a correlation between exposure to black carbon PM and pulmonary vascular remodeling (57). Here, changes in vascular volume – indicative of elevated blood pressure – were comparable to the effect of >15 pack years of cigarette smoking (57). PM exposure has been found to exacerbate high-risk atherosclerotic plaque progression, plaque destabilization and coronary calcification (58). PM exposure has also been linked to atrial fibrillation and reduced heart rate variability, with the later exacerbated by pre-existing diabetes (59). Preclinical models have demonstrated that PM can induce hypertension in healthy animals, secondary disease in animal models of heart failure and hypertension, and induce symptoms of cardiovascular dysfunction via central and renal cardiovascular regulation disruption (60–63). Exposure of rats to black carbon PM for 4 weeks dose and time dependently increased blood pressure (60). Four-day PM2.5 exposure to spontaneously hypertensive rats significantly increased heart rate and blood pressure and reduced heart rate variability (61). In a mouse model of chronic left ventricular heart failure, PM2.5 exposure significantly exacerbated lung oxidative stress, lung fibrosis, inflammation, vascular remodeling, and right ventricle hypertrophy (62, 63). Hypertensive, angiotensin II-infused apoe−/− mice, exposed to PM2.5 for 4 weeks had a significantly increased incidence of abdominal aortic aneurysm compared to controls (64). Here, aortic aneurysm was associated with significant vascular elastin degradation, increased maximal abdominal aortic diameter and elevated expression of senescence proteins P16 and P21 (64). Increased vascular P21 is implicated in the development of atherosclerosis, causes of which include inflammation, hemodynamic damage and aberrant lipid metabolism (65, 66). Multiple models have shown PM2.5 exposure to stimulate endothelial release of inflammatory cytokines and adhesion molecules, promote macrophage infiltration, vascular smooth muscle cell dysfunction and plaque formation (67). Hypertensive, apolipoprotein-deficient mice exposed to PM2.5 for 3 months exhibited increased atherosclerotic lesion area, hepcidin and iron plaque depositions, increased plasma iron, ferritin, total cholesterol, low density cholesterol, vascular endothelial derived growth factor, monocyte chemoattractant protein-1 and pro-atherosclerotic cytokines interleukin 6 and tumor necrosis factor-α (64). Both blood pressure and heart rate are partially regulated by the central nervous system, with sympathetic output from the hypothalamus significantly impacting cardiovascular tone (68). In wild-type mice, long-term PM2.5 exposure has been found to increase basal blood pressure; an effect that was reversed with central alpha-2 adrenergic receptor antagonism. Concurrent inflammation of the hypothalamic arcuate nucleus was observed in hypertensive PM2.5-exposed mice (69). Increased noradrenergic signaling in the hypothalamic periventricular nucleus is known to increase sympathetic output and cardiovascular tone (70). Exposure of lean Brown Norway rats to PM for 1 day increased noradrenaline concentrations in the paraventricular nucleus and corticotropin releasing hormone concentration in the median eminence (71).
Renal Disease
Human kidneys filter ~180 L of blood each day and are therefore vulnerable to PM exposure (21, 72). In people, PM2.5 exposure has been linked to an accelerated decline in glomerular filtration rate, diminished glomerular function during pregnancy, increased risks of chronic kidney disease, end stage renal disease, renal failure and chronic kidney disease mortality (72–74). Human studies have also revealed that PM2.5 exposure to positively correlate with risk of albuminuria; a marker of glomerular disfunction (72). A comparison of renal biomarkers in welders and office workers revealed that welders - exposed to much higher levels of PM2.5 that office worker controls – had elevated plasma markers of renal tubule damage; urinary kidney injury molecule-1 and neutrophil gelatinase-associated lipocalin (75). Another study investigating the potential for tubule damage in humans revealed a 10 μg/m3 increase in PM2.5 exposure to be associated with increased nephritis hospital admissions (76). PM2.5 exposure is associated with an elevated risk of adverse post kidney transplant outcomes, including acute rejection, graft failure and death (73, 74). A study specifically investigating the impact of PM on post-transplant outcomes found a 10 μg/m3 increase of PM2.5 to correlate with a 1.31-fold increase in the odds of transplant failure, a 1.59-fold increase in odds of delayed graft function and a 1.15-fold increase in all-cause mortality within 1 year of surgery (77). A similar study revealed an increase of 1 μg/m3 in PM10 exposure to be associated with increased risk of biopsy proven rejection, graft failure and mortality (78). Intratracheal exposure of PM2.5 to immunodeficient mice revealed no obvious renal histopathology. However, PM exposure was associated with elevated serum markers of renal damage including kidney injury molecule-1, cystatin C and uric acid. Moreover, 14-day PM exposure progressively increased renal concentrations of malondialdehyde, hydrogen peroxide, glutathione peroxidase, nuclear factor kappa-β, tumor necrosis factor-α, transcription factor protein-65, NADPH oxidase 4 and heme oxygenase-1 (79). In rats, sub-chronic exposure of PM2.5 resulted in elevated plasma β-2-microglobulin and cystatin-C; serum markers of early-stage kidney damage (80–82). PM exposure has also been found to induce histopathological lung damage, increase median blood pressure, increase urine volume and water consumption (80–82). Exposure of rats to diesel emission PM significantly reduced renal blood flow in controls and to a greater extent in rats with adenine-induced chronic kidney disease (81). Similar work in a mouse model of adenine-induced CKD revealed that PM exposure elevated renal tumor necrosis factor-α, lipid peroxidation, reactive oxygen species, collagen deposition, necrotic cell counts, dilated tubules cast formation and collapsing glomeruli (49).
Endocrine Disease
The known effects of cigarette smoke on reproductive and thyroid hormones provide indications of the risks associated with PM exposure. Cigarette smoke is a risk factor in Graves hypothyroidism and is associated with elevated plasma cortisol, aldosterone, adrenal androgens and impacts female fertility by increasing steroid hormone binding globulin and decreasing circulating free estrogens (83–86). Several PM species have been identified as endocrine disrupting chemicals (87). In humans PM exposure is linked to insulin resistance, elevated circulating adipokines, hypothyroidism and (mixed) estrogenic effects (88). Thyroid hormones triiodothyronine (T3) and thyroxine (T4) regulate metabolic rate, cardiovascular tone and promote growth rate during fetal development and early life (89). In humans, PM exposure is associated with decreased plasma T4 both in pregnant women and new-borns, as well as congenital hypothyroidism and reduced infant birth weight (90). Black carbon, ammonia, organic matter and nitrate PM species appear to have the strongest links to thyroid dysfunction (91–94). Effective insulin signaling is required for glucose homeostasis, and insulin resistance is closely associated with obesity and is a risk factor for the onset of type-2 diabetes (95). PM exposure is associated with insulin resistance and non-alcoholic fatty liver disease, driven by oxidative stress and dyslipidaemia (96, 97). Together these studies highlight the link between air pollution and metabolic diseases including diabetes. Of >106 chemicals to which gas and oil extraction workers are exposed, 21 have been shown to exert estrogenic, androgenic and/or steroidogenic effects (98). Some chemicals identified as impacting endocrine function include benzene, toluene, ethylbenzene xylene, mercury, polychlorinated dibenzodioxins (PBDDs) and several polycyclic aromatic hydrocarbons (PAH) (88, 98, 99). Atmospheric sources of PAHs are vehicle emissions and biomass and coal combustion. Low molecular weight PAHs are in gas phase whereas high molecular weight PAHs are bound to the surface of PM (100). PAHs are classed as endocrine disrupting compounds and have been found to both increase and decrease estrogen receptor mRNA expression and function (REF). Estrogenic dysfunction has been shown to be both direct at estrogen receptors and indirect via aryl hydrocarbon receptor (AhR) signaling (101, 102). PBDDs also exert endocrine effects via AhRs, and preclinical experiments have shown AhR-mediated effects of dioxin exposure to include weight loss, reproductive and developmental toxicity, tumorigenesis and immune system dysfunction (103). PM contains many metal elements, some of which interfere with estrogenic signaling by mimicking endogenous estrogens (104). Metalloestrogens include aluminum, selenium, antimony, arsenic (arsenite; NaAsO2), barium, cadmium, chromium, cobalt, copper, lead, mercury, nickel, tin and vanadium (vanadate; V2O5) (16, 104).
Obesity and Diabetes
In humans, the association between PM2.5 exposure and obesity is dependent on age, gender and socioeconomic demographic (105, 106). A growing body of evidence indicates that PM2.5 exposure is a risk factor for reduced skeletal muscle mass, obesity, diabetes and hypertension (107–109). Long-term PM exposure is associated with a high risk for type 2 diabetes, and road traffic-specific PM is correlated with an elevated risk (110). Increased incidence of type-2 diabetes remains when adjusted for age, body mass index (BMI), and socioeconomic status (111, 112). PM exposure is associated with higher levels of circulating complement factor 3 (C3c), and women with elevated plasma C3c are more susceptible to diabetes than those with low C3c (112). PM2.5 exposure is associated with a faster decline in insulin sensitivity during childhood and higher BMI by age 18 (113–115). The associated between PM exposure and hypertension is greater in overweight and obese children (116). In animal studies, exposure of rats to PM increased chocolate consumption whereas in chow-fed wild-type mice, 10-week PM2.5 exposure increased visceral fat mass, insulin resistance and adipose tissue inflammation (117, 118). In mice, short-term PM exposure increased food intake, fat mass and UCP-1 expression in brown adipose tissue (119). PM exposure also induced hypothalamic inflammation indicated by increased microglia density, increased toll-like receptor-4 and elevated inhibitory nuclear factor-kappa-B-kinase-epsilon expression (119). After 12 weeks of PM exposure, mice exhibited increased food intake and elevated fat mass and had lower energy expenditure. Mice had elevated levels of plasma leptin and insulin and increased Homeostatic Model Assessment for Insulin Resistance (HOMA-IR) indicators of insulin resistance (119). This same study also revealed that PM exposure decreased hypothalamic satiety markers, including reduced levels of phosphorylated STAT 3, and diminished proopiomelanocortin expression (119).
PM exposure to mice was found to induce hepatic oxidative stress, inflammation, negatively affect glucose tolerance and induce insulin resistance (96, 120). Interestingly PM exposure has been found to increase hepatic triacylglycerols, free fatty acids and cholesterol levels in female but not in male mice (96). In addition to insulin resistance, PM exposure has been shown to exert toxic effects directly on the pancreas (121). In a streptozotocin-induced mouse model of type-1 diabetes, PM from diesel exhaust fumes exacerbated pancreatic cell vacuolation and islet cell apoptosis, increased pancreatic amylase activity, increased expression of oxidative stress markers 8-isoprostane and superoxide dismutase and reduced levels of the antioxidant glutathione peroxidase (121). In a rat model of gestational diabetes PM exposure induced maternal pancreatic inflammation indicated by diminished pancreatic glucose transporter-2 expression (122).
Gastrointestinal Disease
Mucociliary clearance of PM from the lungs followed by its ingestion within saliva leads to gastrointestinal PM exposure. A growing body of preclinical data has revealed PM-induced gastrointestinal inflammation and gut microbiome changes (123–125). In mice, PM exposure altered the relative proportions of microbiota component species, impaired gut permeability through oxidative stress and increased proinflammatory cytokine expression in an interleukin-10 knock out model of inflammatory bowel disease (123–125). However, epidemiological studies have not yet identified a clear link between PM exposure and inflammatory bowel disease (126).
Neurological Disease
Increased ambient PM concentration positively correlates with the incidence of Alzheimer's disease, Parkinson's disease, Multiple Sclerosis, dementia and autism spectrum disorder (Figure 2) (127). Long-term PM2.5 exposure significantly increased age adjusted risk of mortality and hospital admission for Alzheimer's disease, Parkinson's disease and non-Alzheimer's disease dementia (128). This study found the strongest correlation to exist between PM2.5 and Alzheimer's disease (128). One longitudinal study found that people living within 50 meters of a main road had a 12% greater chance of dementia diagnosis (129). PM2.5 exposure is linked to faster decline in new learning and immediate recall, as well as MRI-detected gray matter atrophy in brain areas vulnerable to Alzheimer's disease pathology (130). PM2.5 exposure has been linked to Alzheimer's specific cognitive impairments (CERAD score but not ABC score) however post-mortem analysis of neuropathology in the brains of Alzheimer's disease patients failed to reveal any link between PM2.5 exposure 10 years before death, and disease progression indicated by Braak stage (131). The impact of specific PM (including black carbon, organic matter, nitrate, sulfate, sea salt and soil) exposure on the rate of initial Parkinson's disease hospitalization in New York State was investigated. This study revealed that with each standard deviation increase in either nitrate or organic matter PM, the risk of hospitalizations increased 1.06-fold (132).
PM 0.1 can cross the blood brain barrier and cause inflammatory and oxidative tissue damage as well as microglial activation (133). Glutamatergic excitotoxicity is a common reported endpoint for acute PM induced pathophysiology in the central nervous system. PM has been found in neurons, glia, endothelium, choroid plexus ependymal cells, cerebrospinal fluid, nasal epithelium, and olfactory epithelium of individuals subjected to PM exposure (134). PM2.5 has been found to reduce nervous system expression of the tight junction proteins, zonula occludens 1 and 2 (135). This study found a compromised blood brain barrier permeable to macrophage infiltration, and nervous system tissue subject to glutamatergic excitotoxicity, triggered by macrophage-derived glutamate (135). In mice, PM2.5 has been shown to reach the olfactory bulb and induce microglial activation and glutamatergic excitotoxicity that could be blocked with the antioxidant N-acetylcysteine (136).
Alzheimer's disease is characterized by cortical and hippocampal amyloid-β plaque and tau tangle deposition. Amyloid-β plaque formation and gliosis underlie at least some of the cognitive deficits associated with AD progression (137). In a transgenic mouse model of Alzheimer's disease, exposure to diesel emission PM2.5 exacerbated amyloid-β plaque deposition, and increased astrocytosis and microgliosis. Additionally, elevated inflammatory cytokines including tumor necrosis factor, nuclear factor-α, interleukins 1β and 6, interferon-γ and macrophage inflammatory protein-3α were identified in the cortices of double transgenic mice (138). In a similar study, 13-week exposure to diesel exhaust PM also accelerated cortical amyloid-β plaque deposition, an effect associated with significant impairments to motor coordination (139). Parkinson's disease is caused by loss of dopaminergic neurons in the substantia nigra of the basal ganglia. Neuron loss results in diminished cortical input and associated behavioral and cognitive deficits. In a rotenone-induced mouse model of Parkinson's disease, PM2.5 exposure induced mitochondrial dysfunction, oxidative stress and apoptosis in the substantia nigra. In the same study, PM exposure also exacerbated motor and somatosensory deficits (140). Multiple Sclerosis (MS) is a progressive, demyelinating and neurodegenerative disease of the CNS. Short-term PM exposure is associated with increased MS hospital admissions and relapse (127). In a mouse model of lipophosphatidylcholine-induced demyelination, PM exposure impairs myelin repair and sustains astroglia and microglia dependent neuroinflammation. PM2.5 exposure to rats impaired spatial learning and memory, inquiring ability and sensory function, these changes were related to ultrastructural changes to mitochondria and myelin (141). Mice exposed to PM2.5 for 10 months developed structural hippocampal alterations including diminished apical spine density and dendritic branching of hippocampal neurons and behavioral studies revealed reduced spatial learning and memory impairments (142).
Developmental and Gestational Disease
PM2.5 has been found on the fetal side of the placenta. Given the ability of PM to cross endothelial barriers, it is possible that during pregnancy, PM impacts gestation at the level of the mother, the fetus and the placenta. The placenta is critical to fetal development and dysfunction can lead to preeclampsia, gestational diabetes, fetal growth restriction fetal thrombotic vasculopathy, congenital heart defects, reduced birth size, birth weight, preterm birth, and infant mortality (143–151). Pathophysiological mechanisms of PM-induced placental damage may include oxidative stress, inflammation, coagulation and endothelial dysfunction (152).
In rodents, PM exposure at later gestational stages has been shown to decrease gestational duration and birth weight (153). A similar study also found that this lower body weight was exacerbated during lactation. Here, pups exposed to PM in utero were even lighter relative to controls by time of weaning (154). In rats, PM exposure increased blastocyst absorption, reduced maternal weight gain and fetal weight (155). PM2.5 exposure to pregnant Sprague Dawley rats caused increased blood pressure of pups as well as reduced sodium excretion, reduced renal dopamine 1 receptor expression and dopamine 1 receptor-mediated natriuresis and diuresis (156). The placentas of mice exposed to PM during gestation, exhibited increased inflammation and embolism, furthermore maternal blood contained elevated circulatory mononuclear cells, platelets and levels of interleukin-6 (155). At the embryonic level, trophoblasts have been shown to endocytose PM and when exposed to PM, human trophoblasts have been found to exhibit reduced cell growth, endoplasmic reticulum stress and decreased beta-human chorionic gonadotropin secretion (157).
Perspective and Conclusions
It is well documented that PM is associated with harmful outcomes to animal and human health. In addition to direct exposure of the respiratory system, airborne particulates can cross endothelial barriers, enter circulation, and accumulate in multiple organ systems. As we integrate current environmental changes in our research a rigorous multidisciplinary approach is necessary to ascertain the extent to which individual and relevant combinations of PM impact human health. Understanding the exact inflammatory, toxic and oxidative mechanisms of pathophysiology in all exposed physiological systems is needed in order to improve global health outcomes. More preclinical research into the respiratory, cardiovascular, endocrine, metabolic, digestive, reproductive and neurological effects of PM exposure is required to inform prevention, treatment, and policy change. Future work in the field of pollution and physiology should determine the extent of damage, mechanisms of pathophysiology, time course and reversibility of PM induced health outcomes. Due to the ubiquity of PM in the organs of exposed subjects, the extent of required research is vast, but will undoubtedly expedite improved methodologies of prevention and treatment for PM associated diseases.
Author Contributions
Manuscript written by JP and SS. Manuscript edited by JP, LC, and SS. All authors contributed to the article and approved the submitted version.
Funding
SS has been supported by both NHMRC and NHF, Australia.
Conflict of Interest
JP was employed by Woodrudge LTD.
The remaining authors declare that the research was conducted in the absence of any commercial or financial relationships that could be construed as a potential conflict of interest.
Publisher's Note
All claims expressed in this article are solely those of the authors and do not necessarily represent those of their affiliated organizations, or those of the publisher, the editors and the reviewers. Any product that may be evaluated in this article, or claim that may be made by its manufacturer, is not guaranteed or endorsed by the publisher.
References
1. Walker R, Parsche F, Bierbrier M, McKerrow JH. Tissue identification and histologic study of six lung specimens from Egyptian mummies. Am J Phys Anthropol. (1987) 72:43–8. doi: 10.1002/ajpa.1330720106
2. Isidro A, Malgosa A, Prats-Munoz G. Anthracosis in a Coptic mummy. Arch Bronconeumol. (2014) 50:368–9. doi: 10.1016/j.arbr.2014.06.006
3. Hippocrates. Hippocrates on Airs, Waters, and Places, 6th ed. London: Wyman and Sons (1881). doi: 10.1093/nq/s6-III.63.209d
4. Fowler D, Brimblecombe P, Burrows J, Heal MR, Grennfelt P, Stevenson DS, et al. A chronology of global air quality. Philos Trans A Math Phys Eng Sci. (2020) 378:20190314. doi: 10.1098/rsta.2019.0314
5. Christy WG. History of the air pollution control association. J Air Pollut Control Assoc. (1960) 10:126–74. doi: 10.1080/00022470.1960.10467911
6. Hanlon WW. Coal smoke, city growth, and the costs of the industrial revolution. Econ J. (2019) 130:462–88. doi: 10.1093/ej/uez055
8. Cook LM. The rise and fall of the Carbonaria form of the peppered moth. Q Rev Biol. (2003) 78:399–417. doi: 10.1086/378925
9. Stone R. Air pollution. Counting the cost of London's killer smog. Science. (2002) 298:2106–7. doi: 10.1126/science.298.5601.2106b
10. Hickel J. Quantifying national responsibility for climate breakdown: an equality-based attribution approach for carbon dioxide emissions in excess of the planetary boundary. Lancet Planet Health. (2020) 4:e399–404. doi: 10.1016/S2542-5196(20)30196-0
11. World Health Organization. Global Health Observatory: Mortality Attributed to Household and Ambient Air Pollution. Geneva: World Health Organizaiton (2022). Available online at: https://www.who.int/data/gho/data/themes/topics/indicator-groups/indicator-group-details/GHO/mortality-attributed-to-household-and-ambient-air-pollution (accessed March 20, 2022).
12. World Health Organization. Global Health Observatory: Ambient Air Pollution. Geneva: World Health Organization (2021). Available online at: https://www.who.int/data/gho/data/themes/topics/indicator-groups/indicator-group-details/GHO/ambient-air-pollution (accessed January 30, 2022).
13. Park M, Joo HS, Lee K, Jang M, Kim SD, Kim I, et al. Differential toxicities of fine particulate matters from various sources. Sci Rep. (2018) 8:17007. doi: 10.1038/s41598-018-35398-0
14. Masiol M, Squizzato S, Formenton G, Khan MB, Hopke PK, Nenes A, et al. Hybrid multiple-site mass closure and source apportionment of PM25 and aerosol acidity at major cities in the Po Valley. Sci Total Environ. (2020) 704:135287. doi: 10.1016/j.scitotenv.2019.135287
15. Kleinman MT, Bufalino C, Rasmussen R, Hyde D, Bhalla DK. Mautz WJ. Toxicity of chemical components of ambient fine particulate matter (PM 25) inhaled by aged rats. J Appl Toxicol. (2000) 20:357–64. doi: 10.1002/1099-1263(200009/10)20:5<357::AID-JAT699>3.0.CO;2-6
16. Bell ML, Dominici F, Ebisu K, Zeger SL, Samet JM. Spatial and temporal variation in PM(25) chemical composition in the United States for health effects studies. Environ Health Perspect. (2007) 115:989–95. doi: 10.1289/ehp.9621
17. Li D, Li Y, Li G, Zhang Y, Li J, Chen H. Fluorescent reconstitution on deposition of PM25 in lung and extrapulmonary organs. Proc Natl Acad Sci USA. (2019) 116:2488–93. doi: 10.1073/pnas.1818134116
18. Garcia GJ, Schroeter JD, Kimbell JS. Olfactory deposition of inhaled nanoparticles in humans. Inhal Toxicol. (2015) 27:394–403. doi: 10.3109/08958378.2015.1066904
19. Mutlu EA, Comba IY, Cho T, Engen PA, Yazici C, Soberanes S, et al. Inhalational exposure to particulate matter air pollution alters the composition of the gut microbiome. Environ Pollut. (2018) 240:817–30. doi: 10.1016/j.envpol.2018.04.130
20. Xian M, Ma S, Wang K, Lou H, Wang Y, Zhang L, et al. Particulate matter 25 causes deficiency in barrier integrity in human nasal epithelial cells. Allergy Asthma Immunol Res. (2020) 12:56–71. doi: 10.4168/aair.2020.12.1.56
21. Miller MR, Raftis JB, Langrish JP, McLean SG, Samutrtai P, Connell SP, et al. Inhaled nanoparticles accumulate at sites of vascular disease. ACS Nano. (2017) 11:4542–52. doi: 10.1021/acsnano.6b08551
22. Calderon-Garciduenas L, Maronpot RR, Torres-Jardon R, Henriquez-Roldan C, Schoonhoven R, Acuna-Ayala H, et al. DNA damage in nasal and brain tissues of canines exposed to air pollutants is associated with evidence of chronic brain inflammation and neurodegeneration. Toxicol Pathol. (2003) 31:524–38. doi: 10.1080/01926230390226645
23. Maher BA, Ahmed IA, Karloukovski V, MacLaren DA, Foulds PG, Allsop D, et al. Magnetite pollution nanoparticles in the human brain. Proc Natl Acad Sci USA. (2016) 113:10797–801. doi: 10.1073/pnas.1605941113
24. Bove H, Bongaerts E, Slenders E, Bijnens EM, Saenen ND, Gyselaers W, et al. Ambient black carbon particles reach the fetal side of human placenta. Nat Commun. (2019) 10:3866. doi: 10.1038/s41467-019-11654-3
25. World Health Organization. Climate Change and Health. World Health Organization (2021). Available online at: https://www.who.int/news-room/fact-sheets/detail/climate-change-and-health (accessed December 20, 2022).
26. Valavanidis A, Vlachogianni T, Fiotakis K, Loridas S. Pulmonary oxidative stress, inflammation and cancer: respirable particulate matter, fibrous dusts and ozone as major causes of lung carcinogenesis through reactive oxygen species mechanisms. Int J Environ Res Public Health. (2013) 10:3886–907. doi: 10.3390/ijerph10093886
27. Wu W, Jin Y, Carlsten C. Inflammatory health effects of indoor and outdoor particulate matter. J Allergy Clin Immunol. (2018) 141:833–44. doi: 10.1016/j.jaci.2017.12.981
28. Rao X, Zhong J, Brook RD, Rajagopalan S. Effect of particulate matter air pollution on cardiovascular oxidative stress pathways. Antioxid Redox Signal. (2018) 28:797–818. doi: 10.1089/ars.2017.7394
29. Wang Y, Tang M. PM2.5 induces ferroptosis in human endothelial cells through iron overload and redox imbalance. Environ Pollut. (2019) 254(Pt A):112937. doi: 10.1016/j.envpol.2019.07.105
30. Xie W, You J, Zhi C, Li L. The toxicity of ambient fine particulate matter (PM25) to vascular endothelial cells. J Appl Toxicol. (2021) 41:713–23. doi: 10.1002/jat.4138
31. Wang J, Zhang WJ, Xiong W, Lu WH, Zheng HY, Zhou X, et al. PM25 stimulated the release of cytokines from BEAS-2B cells through activation of IKK/NF-kappaB pathway. Hum Exp Toxicol. (2019) 38:311–20. doi: 10.1177/0960327118802628
32. Fu H, Liu X, Li W, Zu Y, Zhou F, Shou Q, et al. PM25 exposure induces inflammatory response in macrophages via the TLR4/COX-2/NF-kappaB pathway. Inflammation. (2020) 43:1948–58. doi: 10.1007/s10753-020-01269-y
33. Hu H, Wu J, Li Q, Asweto C, Feng L, Yang X, et al. Fine particulate matter induces vascular endothelial activation via IL-6 dependent JAK1/STAT3 signaling pathway. Toxicol Res. (2016) 5:946–53. doi: 10.1039/C5TX00351B
34. Park EJ, Chae JB Lyu J, Yoon C, Kim S, Yeom C, et al. Ambient fine particulate matters induce cell death and inflammatory response by influencing mitochondria function in human corneal epithelial cells. Environ Res. (2017) 159:595–605. doi: 10.1016/j.envres.2017.08.044
35. Analitis A, Katsouyanni K, Dimakopoulou K, Samoli E, Nikoloulopoulos AK, Petasakis Y, et al. Short-term effects of ambient particles on cardiovascular and respiratory mortality. Epidemiology. (2006) 17:230–3. doi: 10.1097/01.ede.0000199439.57655.6b
36. Zanobetti A, Franklin M, Koutrakis P, Schwartz J. Fine particulate air pollution and its components in association with cause-specific emergency admissions. Environ Health. (2009) 8:58. doi: 10.1186/1476-069X-8-58
37. Kyung SY, Jeong SH. Particulate-matter related respiratory diseases. Tuberc Respir Dis. (2020) 83:116–21. doi: 10.4046/trd.2019.0025
38. Guarnieri M, Balmes JR. Outdoor air pollution and asthma. Lancet. (2014) 383:1581–92. doi: 10.1016/S0140-6736(14)60617-6
39. Winterbottom CJ, Shah RJ, Patterson KC, Kreider ME, Panettieri RA Jr, Rivera-Lebron B, et al. Exposure to ambient particulate matter is associated with accelerated functional decline in idiopathic pulmonary fibrosis. Chest. (2018) 153:1221–8. doi: 10.1016/j.chest.2017.07.034
40. Yang J, Chen Y, Yu Z, Ding H. Ma Z. The influence of PM25 on lung injury and cytokines in mice. Exp Ther Med. (2019) 18:2503–11. doi: 10.3892/etm.2019.7839
41. Lommatzsch M, Speer T, Herr C, Jorres RA, Watz H, Muller A, et al. IgE is associated with exacerbations and lung function decline in COPD. Respir Res. (2022) 23:1. doi: 10.1186/s12931-021-01847-0
42. Xu Z, Li Z, Liao Z, Gao S, Hua L, Ye X, et al. PM25 induced pulmonary fibrosis in vivo and in vitro. Ecotoxicol Environ Saf. (2019) 171:112–21. doi: 10.1016/j.ecoenv.2018.12.061
43. Kim KK, Sheppard D, Chapman HA. TGF-beta1 signaling and tissue fibrosis. Cold Spring Harb Perspect Biol. (2018) 10:a022293. doi: 10.1101/cshperspect.a022293
44. Saputra D, Yoon JH, Park H, Heo Y, Yang H, Lee EJ, et al. Inhalation of carbon black nanoparticles aggravates pulmonary inflammation in mice. Toxicol Res. (2014) 30:83–90. doi: 10.5487/TR.2014.30.2.083
45. Su R, Jin X, Zhang W, Li Z, Liu X, Ren J. Particulate matter exposure induces the autophagy of macrophages via oxidative stress-mediated PI3K/AKT/mTOR pathway. Chemosphere. (2017) 167:444–53. doi: 10.1016/j.chemosphere.2016.10.024
46. Sun L, Chen B, Jiang R, Li J, Wang B. Resveratrol inhibits lung cancer growth by suppressing M2-like polarization of tumor associated macrophages. Cell Immunol. (2017) 311:86–93. doi: 10.1016/j.cellimm.2016.11.002
47. Kimura Y, Sumiyoshi M. Resveratrol prevents tumor growth and metastasis by inhibiting lymphangiogenesis and M2 macrophage activation and differentiation in tumor-associated macrophages. Nutr Cancer. (2016) 68:667–78. doi: 10.1080/01635581.2016.1158295
48. Xu M, Wang X, Xu L, Zhang H, Li C, Liu Q, et al. Chronic lung inflammation and pulmonary fibrosis after multiple intranasal instillation of PM2 5 in mice. Environ Toxicol. (2021) 36:1434–46. doi: 10.1002/tox.23140
49. Aryal A, Harmon AC, Dugas TR. Particulate matter air pollutants and cardiovascular disease: strategies for intervention. Pharmacol Ther. (2021) 223:107890. doi: 10.1016/j.pharmthera.2021.107890
50. Zhang M, Mueller NT, Wang H, Hong X, Appel LJ. Wang X. Maternal exposure to ambient particulate matter ≤ 25 μm during pregnancy and the risk for high blood pressure in childhood. Hypertension. (2018) 72:194–201. doi: 10.1161/HYPERTENSIONAHA.117.10944
51. van Rossem L, Rifas-Shiman SL, Melly SJ, Kloog I, Luttmann-Gibson H, Zanobetti A, et al. Prenatal air pollution exposure and newborn blood pressure. Environ Health Perspect. (2015) 123:353–9. doi: 10.1289/ehp.1307419
52. Liang R, Zhang B, Zhao X, Ruan Y, Lian H, Fan Z. Effect of exposure to PM2.5 on blood pressure: a systematic review and meta-analysis. J Hypertens. (2014) 32:2130–40. discussion 41. doi: 10.1097/HJH.0000000000000342
53. Auchincloss AH, Diez Roux AV, Dvonch JT, Brown PL, Barr RG, Daviglus ML, et al. Associations between recent exposure to ambient fine particulate matter and blood pressure in the Multi-ethnic Study of Atherosclerosis (MESA). Environ Health Perspect. (2008) 116:486–91. doi: 10.1289/ehp.10899
54. Calderon-Garciduenas L, Vincent R, Mora-Tiscareno A, Franco-Lira M, Henriquez-Roldan C, Barragan-Mejia G, et al. Elevated plasma endothelin-1 and pulmonary arterial pressure in children exposed to air pollution. Environ Health Perspect. (2007) 115:1248–53. doi: 10.1289/ehp.9641
55. Fan J, Unoki H, Iwasa S, Watanabe T. Role of endothelin-1 in atherosclerosis. Ann N Y Acad Sci. (2000) 902:84–93. discussion−4. doi: 10.1111/j.1749-6632.2000.tb06303.x
56. Krishnan RM, Adar SD, Szpiro AA, Jorgensen NW, Van Hee VC, Barr RG, et al. Vascular responses to long- and short-term exposure to fine particulate matter: MESA Air (Multi-Ethnic Study of Atherosclerosis and Air Pollution). J Am Coll Cardiol. (2012) 60:2158–66.
57. Aaron CP, Hoffman EA, Kawut SM, Austin JHM, Budoff M, Michos ED, et al. Ambient air pollution and pulmonary vascular volume on computed tomography: the MESA Air Pollution and Lung cohort studies. Eur Respir J. (2019) 53:1802116. doi: 10.1183/13993003.02116-2018
58. Kaufman JD, Adar SD, Barr RG, Budoff M, Burke GL, Curl CL, et al. Association between air pollution and coronary artery calcification within six metropolitan areas in the USA (the Multi-Ethnic Study of Atherosclerosis and Air Pollution): a longitudinal cohort study. Lancet. (2016) 388:696–704. doi: 10.1016/S0140-6736(16)00378-0
59. Park SK, O'Neill MS, Vokonas PS, Sparrow D, Schwartz J. Effects of air pollution on heart rate variability: the VA normative aging study. Environ Health Perspect. (2005) 113:304–9. doi: 10.1289/ehp.7447
60. Niwa Y, Hiura Y, Sawamura H, Iwai N. Inhalation exposure to carbon black induces inflammatory response in rats. Circ J. (2008) 72:144–9. doi: 10.1253/circj.72.144
61. Wagner JG, Kamal AS, Morishita M, Dvonch JT, Harkema JR. Rohr AC. PM25-induced cardiovascular dysregulation in rats is associated with elemental carbon and temperature-resolved carbon subfractions. Part Fibre Toxicol. (2014) 11:25. doi: 10.1186/1743-8977-11-25
62. Wan Q, Yang M, Liu Z, Wu J. Ambient fine particulate matter aggravates atherosclerosis in apolipoprotein E knockout mice by iron overload via the hepcidin-ferroportin axis. Life Sci. (2021) 264:118715. doi: 10.1016/j.lfs.2020.118715
63. Yue W, Tong L, Liu X, Weng X, Chen X, Wang D, et al. Short term Pm25 exposure caused a robust lung inflammation, vascular remodeling, and exacerbated transition from left ventricular failure to right ventricular hypertrophy. Redox Biol. (2019) 22:101161. doi: 10.1016/j.redox.2019.101161
64. Jun X, Jin G, Fu C, Jinxuan Z, Xueling L, Jiaxin H, et al. PM25 promotes abdominal aortic aneurysm formation in angiotensin -infused apoe-/- mice. Biomed Pharmacother. (2018) 104:550–7. doi: 10.1016/j.biopha.2018.04.107
65. Kunieda T, Minamino T, Nishi J, Tateno K, Oyama T, Katsuno T, et al. Angiotensin II induces premature senescence of vascular smooth muscle cells and accelerates the development of atherosclerosis via a p21-dependent pathway. Circulation. (2006) 114:953–60. doi: 10.1161/CIRCULATIONAHA.106.626606
66. Yang X, Li Y, Li Y, Ren X, Zhang X, Hu D, et al. Oxidative stress-mediated atherosclerosis: mechanisms and therapies. Front Physiol. (2017) 8:600. doi: 10.3389/fphys.2017.00600
67. Araujo JA, Nel AE. Particulate matter and atherosclerosis: role of particle size, composition and oxidative stress. Part Fibre Toxicol. (2009) 6:24. doi: 10.1186/1743-8977-6-24
68. Simonds SE, Cowley MA. Hypertension in obesity: is leptin the culprit? Trends Neurosci. (2013) 36:121–32. doi: 10.1016/j.tins.2013.01.004
69. Ying Z, Xu X, Bai Y, Zhong J, Chen M, Liang Y, et al. Long-term exposure to concentrated ambient PM25 increases mouse blood pressure through abnormal activation of the sympathetic nervous system: a role for hypothalamic inflammation. Environ Health Perspect. (2014) 122:79–86. doi: 10.1289/ehp.1307151
70. Harland D, Gardiner SM, Bennett T. Paraventricular nucleus injections of noradrenaline: cardiovascular effects in conscious Long-Evans and Brattleboro rats. Brain Res. (1989) 496:14–24. doi: 10.1016/0006-8993(89)91047-0
71. Balasubramanian P, Sirivelu MP, Weiss KA, Wagner JG, Harkema JR, Morishita M, et al. Differential effects of inhalation exposure to PM25 on hypothalamic monoamines and corticotrophin releasing hormone in lean and obese rats. Neurotoxicology. (2013) 36:106–11. doi: 10.1016/j.neuro.2012.02.016
72. Rasking L, Vanbrabant K, Bove H, Plusquin M, De Vusser K, Roels HA, et al. Adverse effects of fine particulate matter on human kidney functioning: a systematic review. Environ Health. (2022) 21:24. doi: 10.1186/s12940-021-00827-7
73. An Y, Liu ZH. Air pollution and kidney diseases: PM25 as an emerging culprit. Contrib Nephrol. (2021) 199:274–84. doi: 10.1159/000517710
74. Chang SH, Merzkani M, Murad H, Wang M, Bowe B, Lentine KL, et al. Association of ambient fine particulate matter air pollution with kidney transplant outcomes. JAMA Netw Open. (2021) 4:e2128190. doi: 10.1001/jamanetworkopen.2021.28190
75. Chuang KJ, Pan CH, Su CL, Lai CH, Lin WY, Ma CM, et al. Urinary neutrophil gelatinase-associated lipocalin is associated with heavy metal exposure in welding workers. Sci Rep. (2015) 5:18048. doi: 10.1038/srep18048
76. Gu J, Shi Y, Zhu Y, Chen N, Wang H, Zhang Z, et al. Ambient air pollution and cause-specific risk of hospital admission in China: a nationwide time-series study. PLoS Med. (2020) 17:e1003188. doi: 10.1371/journal.pmed.1003188
77. Feng Y, Jones MR, Ahn JB, Garonzik-Wang JM, Segev DL, McAdams-DeMarco M. Ambient air pollution and posttransplant outcomes among kidney transplant recipients. Am J Transplant. (2021) 21:3333–45. doi: 10.1111/ajt.16605
78. Kim YC, Kim E, Jung J, Park JY, Lee H, Kim DK, et al. Clinical outcomes associated with long-term exposure to airborne particulate pollution in kidney transplant recipients. Environ Health. (2021) 20:61. doi: 10.1186/s12940-021-00741-y
79. Zhang Y, Li Q, Fang M, Ma Y, Liu N, Yan X, et al. The kidney injury induced by short-term pm25 exposure and the prophylactic treatment of essential oils in BALB/c mice. Oxid Med Cell Longev. (2018) 2018:9098627. doi: 10.1155/2018/9098627
80. Nemmar A, Karaca T, Beegam S, Yuvaraju P, Yasin J, Hamadi NK, et al. Prolonged pulmonary exposure to diesel exhaust particles exacerbates renal oxidative stress, inflammation and DNA damage in mice with adenine-induced chronic renal failure. Cell Physiol Biochem. (2016) 38:1703–13. doi: 10.1159/000443109
81. Al Suleimani YM, Al Mahruqi AS, Al Za'abi M, Shalaby A, Ashique M, Nemmar A, et al. Effect of diesel exhaust particles on renal vascular responses in rats with chronic kidney disease. Environ Toxicol. (2017) 32:541–9. doi: 10.1002/tox.22258
82. Aztatzi-Aguilar OG, Uribe-Ramirez M, Narvaez-Morales J, De Vizcaya-Ruiz A. Barbier O. Early kidney damage induced by subchronic exposure to PM25 in rats. Part Fibre Toxicol. (2016) 13:68. doi: 10.1186/s12989-016-0179-8
83. Kapoor D, Jones TH. Smoking and hormones in health and endocrine disorders. Eur J Endocrinol. (2005) 152:491–9. doi: 10.1530/eje.1.01867
84. Tweed JO, Hsia SH, Lutfy K, Friedman TC. The endocrine effects of nicotine and cigarette smoke. Trends Endocrinol Metab. (2012) 23:334–42. doi: 10.1016/j.tem.2012.03.006
85. Kato I, Toniolo P, Koenig KL, Shore RE, Zeleniuch-Jacquotte A, Akhmedkhanov A, et al. Epidemiologic correlates with menstrual cycle length in middle aged women. Eur J Epidemiol. (1999) 15:809–14. doi: 10.1023/A:1007669430686
86. Kirschbaum C, Wust S, Strasburger CJ. “Normal” cigarette smoking increases free cortisol in habitual smokers. Life Sci. (1992) 50:435–42. doi: 10.1016/0024-3205(92)90378-3
87. Darbre PD. Endocrine disruptors and obesity. Curr Obes Rep. (2017) 6:18–27. doi: 10.1007/s13679-017-0240-4
88. Darbre PD. Overview of air pollution and endocrine disorders. Int J Gen Med. (2018) 11:191–207. doi: 10.2147/IJGM.S102230
89. Shahid MA, Ashraf MA, Sharma S. Physiology, Thyroid Hormone. Island, FL: StatPearls Treasure (2022).
90. Harari-Kremer R, Calderon-Margalit R, Korevaar TIM, Nevo D, Broday D, Kloog I, et al. Associations between prenatal exposure to air pollution and congenital hypothyroidism. Am J Epidemiol. (2021) 190:2630–8. doi: 10.1093/aje/kwab187
91. Pan S, Ni W, Li W, Li G, Xing Q. Effects of PM2.5 and PM10 on congenital hypothyroidism in Qingdao, China, 2014-2017: a quantitative analysis. Ther Adv Endocrinol Metab. (2019) 10:2042018819892151. doi: 10.1177/2042018819892151
92. Wang X, Liu C, Zhang M, Han Y, Aase H, Villanger GD, et al. Evaluation of maternal exposure to PM25 and its components on maternal and neonatal thyroid function and birth weight: a cohort study. Thyroid. (2019) 29:1147–57. doi: 10.1089/thy.2018.0780
93. Ghassabian A, Pierotti L, Basterrechea M, Chatzi L, Estarlich M, Fernandez-Somoano A, et al. Association of exposure to ambient air pollution with thyroid function during pregnancy. JAMA Netw Open. (2019) 2:e1912902. doi: 10.1001/jamanetworkopen.2019.12902
94. Kuehn BM. Air pollution's effects on newborns' thyroid hormones. JAMA. (2021) 326:592. doi: 10.1001/jama.2021.13123
95. Taylor R. Insulin resistance and type 2 diabetes. Diabetes. (2012) 61:778–9. doi: 10.2337/db12-0073
96. Li R, Sun Q, Lam SM, Chen R, Zhu J, Gu W, et al. Sex-dependent effects of ambient PM25 pollution on insulin sensitivity and hepatic lipid metabolism in mice. Part Fibre Toxicol. (2020) 17:14. doi: 10.1186/s12989-020-00343-5
97. Xu MX, Ge CX, Qin YT, Gu TT, Lou DS, Li Q, et al. Prolonged PM25 exposure elevates risk of oxidative stress-driven nonalcoholic fatty liver disease by triggering increase of dyslipidemia. Free Radic Biol Med. (2019) 130:542–56. doi: 10.1016/j.freeradbiomed.2018.11.016
98. Bolden AL, Schultz K, Pelch KE, Kwiatkowski CF. Exploring the endocrine activity of air pollutants associated with unconventional oil and gas extraction. Environ Health. (2018) 17:26. doi: 10.1186/s12940-018-0368-z
99. Shibamoto T, Yasuhara A, Katami T. Dioxin formation from waste incineration. Rev Environ Contam Toxicol. (2007) 190:1–41. doi: 10.1007/978-0-387-36903-7_1
100. Straif K, Baan R, Grosse Y, Secretan B, El Ghissassi F, Cogliano V, et al. Carcinogenicity of polycyclic aromatic hydrocarbons. Lancet Oncol. (2005) 6:931–2. doi: 10.1016/S1470-2045(05)70458-7
101. Sahay D, Lloyd SE, Rivera JA, Jezioro J, McDonald JD, Pitiranggon M, et al. Prenatal polycyclic aromatic hydrocarbons, altered ERalpha pathway-related methylation and expression, and mammary epithelial cell proliferation in offspring and grandoffspring adult mice. Environ Res. (2021) 196:110961. doi: 10.1016/j.envres.2021.110961
102. Zhang Y, Dong S, Wang H, Tao S, Kiyama R. Biological impact of environmental polycyclic aromatic hydrocarbons (ePAHs) as endocrine disruptors. Environ Pollut. (2016) 213:809–24. doi: 10.1016/j.envpol.2016.03.050
103. Otarola G, Castillo H, Marcellini S. Aryl hydrocarbon receptor-based bioassays for dioxin detection: thinking outside the box. J Appl Toxicol. (2018) 38:437–49. doi: 10.1002/jat.3575
104. Darbre PD. Metalloestrogens: an emerging class of inorganic xenoestrogens with potential to add to the oestrogenic burden of the human breast. J Appl Toxicol. (2006) 26:191–7. doi: 10.1002/jat.1135
105. Fioravanti S, Cesaroni G, Badaloni C, Michelozzi P, Forastiere F, Porta D. Traffic-related air pollution and childhood obesity in an Italian birth cohort. Environ Res. (2018) 160:479–86. doi: 10.1016/j.envres.2017.10.003
106. An R, Ji M, Yan H, Guan C. Impact of ambient air pollution on obesity: a systematic review. Int J Obes. (2018) 42:1112–26. doi: 10.1038/s41366-018-0089-y
107. Miller KA, Siscovick DS, Sheppard L, Shepherd K, Sullivan JH, Anderson GL, et al. Long-term exposure to air pollution and incidence of cardiovascular events in women. N Engl J Med. (2007) 356:447–58. doi: 10.1056/NEJMoa054409
108. Pearson JF, Bachireddy C, Shyamprasad S, Goldfine AB, Brownstein JS. Association between fine particulate matter and diabetes prevalence in the US. Diabetes Care. (2010) 33:2196–201. doi: 10.2337/dc10-0698
109. Chen CH, Huang LY, Lee KY, Wu CD, Chiang HC, Chen BY, et al. Effects of PM25 on skeletal muscle mass and body fat mass of the elderly in Taipei, Taiwan. Sci Rep. (2019) 9:11176. doi: 10.1038/s41598-019-47576-9
110. Weinmayr G, Hennig F, Fuks K, Nonnemacher M, Jakobs H, Mohlenkamp S, et al. Long-term exposure to fine particulate matter and incidence of type 2 diabetes mellitus in a cohort study: effects of total and traffic-specific air pollution. Environ Health. (2015) 14:53. doi: 10.1186/s12940-015-0031-x
111. Wolf K, Popp A, Schneider A, Breitner S, Hampel R, Rathmann W, et al. Association between long-term exposure to air pollution and biomarkers related to insulin resistance, subclinical inflammation, and adipokines. Diabetes. (2016) 65:3314–26. doi: 10.2337/db15-1567
112. Kramer U, Herder C, Sugiri D, Strassburger K, Schikowski T, Ranft U, et al. Traffic-related air pollution and incident type 2 diabetes: results from the SALIA cohort study. Environ Health Perspect. (2010) 118:1273–9. doi: 10.1289/ehp.0901689
113. Alderete TL, Habre R, Toledo-Corral CM, Berhane K, Chen Z, Lurmann FW, et al. Longitudinal associations between ambient air pollution with insulin sensitivity, beta-cell function, and adiposity in Los Angeles Latino children. Diabetes. (2017) 66:1789–96. doi: 10.2337/db16-1416
114. Thiering E, Cyrys J, Kratzsch J, Meisinger C, Hoffmann B, Berdel D, et al. Long-term exposure to traffic-related air pollution and insulin resistance in children: results from the GINIplus and LISAplus birth cohorts. Diabetologia. (2013) 56:1696–704. doi: 10.1007/s00125-013-2925-x
115. Lavigne E, Ashley-Martin J, Dodds L, Arbuckle TE, Hystad P, Johnson M, et al. Air pollution exposure during pregnancy and fetal markers of metabolic function: the MIREC study. Am J Epidemiol. (2016) 183:842–51. doi: 10.1093/aje/kwv256
116. Dong GH, Wang J, Zeng XW, Chen L, Qin XD, Zhou Y, et al. Interactions between air pollution and obesity on blood pressure and hypertension in Chinese children. Epidemiology. (2015) 26:740–7. doi: 10.1097/EDE.0000000000000336
117. Sun Q, Yue P, Deiuliis JA, Lumeng CN, Kampfrath T, Mikolaj MB, et al. Ambient air pollution exaggerates adipose inflammation and insulin resistance in a mouse model of diet-induced obesity. Circulation. (2009) 119:538–46. doi: 10.1161/CIRCULATIONAHA.108.799015
118. da Silveira CG, Di Domenico M, Hilario Nascimento Saldiva P, Ramos Rhoden C. Subchronic air pollution exposure increases highly palatable food intake, modulates caloric efficiency and induces lipoperoxidation. Inhal Toxicol. (2018) 30:370–80. doi: 10.1080/08958378.2018.1530317
119. Campolim CM, Weissmann L, Ferreira CKO, Zordao OP, Dornellas APS, de Castro G, et al. Short-term exposure to air pollution (PM25) induces hypothalamic inflammation, and long-term leads to leptin resistance and obesity via Tlr4/Ikbke in mice. Sci Rep. (2020) 10:10160. doi: 10.1038/s41598-020-67040-3
120. Hill BG, Rood B, Ribble A, Haberzettl P. Fine particulate matter (PM25) inhalation-induced alterations in the plasma lipidome as promoters of vascular inflammation and insulin resistance. Am J Physiol Heart Circ Physiol. (2021) 320:H1836–50. doi: 10.1152/ajpheart.00881.2020
121. Nemmar A, Al-Salam S, Beegam S, Yuvaraju P, Yasin J, Ali BH. Pancreatic effects of diesel exhaust particles in mice with type 1 diabetes mellitus. Cell Physiol Biochem. (2014) 33:413–22. doi: 10.1159/000356680
122. Yi L, Wei C, Fan W. Fine particulate matter (PM2.5), a risk factor of rat gestational diabetes with altered blood glucose and pancreatic GLUT2 expression. Gynecol Endocrinol. (2017) 33:611–6. doi: 10.1080/09513590.2017.1318368
123. Kish L, Hotte N, Kaplan GG, Vincent R, Tso R, Ganzle M, et al. Environmental particulate matter induces murine intestinal inflammatory responses and alters the gut microbiome. PLoS ONE. (2013) 8:e62220. doi: 10.1371/journal.pone.0062220
124. Mutlu EA, Engen PA, Soberanes S, Urich D, Forsyth CB, Nigdelioglu R, et al. Particulate matter air pollution causes oxidant-mediated increase in gut permeability in mice. Part Fibre Toxicol. (2011) 8:19. doi: 10.1186/1743-8977-8-19
125. Pambianchi E, Pecorelli A, Valacchi G. Gastrointestinal tissue as a “new” target of pollution exposure. IUBMB Life. (2022) 74:62–73. doi: 10.1002/iub.2530
126. Beamish LA, Osornio-Vargas AR, Wine E. Air pollution: an environmental factor contributing to intestinal disease. J Crohns Colitis. (2011) 5:279–86. doi: 10.1016/j.crohns.2011.02.017
127. Parolisi R, Montarolo F, Pini A, Rovelli S, Cattaneo A, Bertolotto A, et al. Exposure to fine particulate matter (PM25) hampers myelin repair in a mouse model of white matter demyelination. Neurochem Int. (2021) 145:104991. doi: 10.1016/j.neuint.2021.104991
128. Rhew SH, Kravchenko J. Lyerly HK. Exposure to low-dose ambient fine particulate matter PM25 and Alzheimer's disease, non-Alzheimer's dementia, and Parkinson's disease in North Carolina. PLoS ONE. (2021) 16:e0253253. doi: 10.1371/journal.pone.0253253
129. Shi L, Wu X, Danesh Yazdi M, Braun D, Abu Awad Y, Wei Y, et al. Long-term effects of PM25 on neurological disorders in the American Medicare population: a longitudinal cohort study. Lancet Planet Health. (2020) 4:e557–e65. doi: 10.1016/S2542-5196(20)30227-8
130. Younan D, Petkus AJ, Widaman KF, Wang X, Casanova R, Espeland MA, et al. Particulate matter and episodic memory decline mediated by early neuroanatomic biomarkers of Alzheimer's disease. Brain. (2020) 143:289–302. doi: 10.1093/brain/awz348
131. Shaffer RM Li G, Adar SD, Dirk Keene C, Latimer CS, Crane PK, et al. Fine particulate matter and markers of Alzheimer's disease neuropathology at autopsy in a community-based cohort. J Alzheimers Dis. (2021) 79:1761–73. doi: 10.3233/JAD-201005
132. Nunez Y, Boehme AK Li M, Goldsmith J, Weisskopf MG, Re DB, et al. Parkinson's disease aggravation in association with fine particle components in New York State. Environ Res. (2021) 201:111554. doi: 10.1016/j.envres.2021.111554
133. Block ML, Calderon-Garciduenas L. Air pollution: mechanisms of neuroinflammation and CNS disease. Trends Neurosci. (2009) 32:506–16. doi: 10.1016/j.tins.2009.05.009
134. Gonzalez-Maciel A, Reynoso-Robles R, Torres-Jardon R, Mukherjee PS, Calderon-Garciduenas L. Combustion-derived nanoparticles in key brain target cells and organelles in young urbanites: culprit hidden in plain sight in Alzheimer's disease development. J Alzheimers Dis. (2017) 59:189–208. doi: 10.3233/JAD-170012
135. Liu F, Huang Y, Zhang F, Chen Q, Wu B, Rui W, et al. Macrophages treated with particulate matter PM25 induce selective neurotoxicity through glutaminase-mediated glutamate generation. J Neurochem. (2015) 134:315–26. doi: 10.1111/jnc.13135
136. Chen X, Guo J, Huang Y, Liu S, Huang Y, Zhang Z, et al. Urban airborne PM25-activated microglia mediate neurotoxicity through glutaminase-containing extracellular vesicles in olfactory bulb. Environ Pollut. (2020) 264:114716. doi: 10.1016/j.envpol.2020.114716
137. Sakakibara Y, Sekiya M, Saito T, Saido TC, Iijima KM. Amyloid-beta plaque formation and reactive gliosis are required for induction of cognitive deficits in App knock-in mouse models of Alzheimer's disease. BMC Neurosci. (2019) 20:13. doi: 10.1186/s12868-019-0496-6
138. Sahu B, Mackos AR, Floden AM, Wold LE, Combs CK. Particulate matter exposure exacerbates amyloid-beta plaque deposition and gliosis in APP/PS1 mice. J Alzheimers Dis. (2021) 80:761–74. doi: 10.3233/JAD-200919
139. Hullmann M, Albrecht C, van Berlo D, Gerlofs-Nijland ME, Wahle T, Boots AW, et al. Diesel engine exhaust accelerates plaque formation in a mouse model of Alzheimer's disease. Part Fibre Toxicol. (2017) 14:35. doi: 10.1186/s12989-017-0213-5
140. Wang Y, Li C, Zhang X, Kang X, Li Y, Zhang W, et al. Exposure to PM25 aggravates Parkinson's disease via inhibition of autophagy and mitophagy pathway. Toxicology. (2021) 456:152770. doi: 10.1016/j.tox.2021.152770
141. Zhang Q, Li Q, Ma J, Zhao Y. PM2.5 impairs neurobehavior by oxidative stress and myelin sheaths injury of brain in the rat. Environ Pollut. (2018) 242(Pt A):994–1001. doi: 10.1016/j.envpol.2018.07.031
142. Fonken LK, Xu X, Weil ZM, Chen G, Sun Q, Rajagopalan S, et al. Air pollution impairs cognition, provokes depressive-like behaviors and alters hippocampal cytokine expression and morphology. Mol Psychiatry. (2011) 16:987–95, 73. doi: 10.1038/mp.2011.76
143. Wang YY Li Q, Guo Y, Zhou H, Wang X, Wang Q, et al. Association of long-term exposure to airborne particulate matter of 1 μm or less with preterm birth in China. JAMA Pediatr. (2018) 172:e174872. doi: 10.1001/jamapediatrics.2017.4872
144. Lee PC, Roberts JM, Catov JM, Talbott EO, Ritz B. First trimester exposure to ambient air pollution, pregnancy complications and adverse birth outcomes in Allegheny County, PA. Matern Child Health J. (2013) 17:545–55. doi: 10.1007/s10995-012-1028-5
145. Malmqvist E, Liew Z, Kallen K, Rignell-Hydbom A, Rittner R, Rylander L, et al. Fetal growth and air pollution - a study on ultrasound and birth measures. Environ Res. (2017) 152:73–80. doi: 10.1016/j.envres.2016.09.017
146. Dadvand P, Ostro B, Amato F, Figueras F, Minguillon MC, Martinez D, et al. Particulate air pollution and preeclampsia: a source-based analysis. Occup Environ Med. (2014) 71:570–7. doi: 10.1136/oemed-2013-101693
147. Wylie BJ, Matechi E, Kishashu Y, Fawzi W, Premji Z, Coull BA, et al. Placental pathology associated with household air pollution in a cohort of pregnant women from Dar es Salaam, Tanzania. Environ Health Perspect. (2017) 125:134–40. doi: 10.1289/EHP256
148. DeFranco E, Hall E, Hossain M, Chen A, Haynes EN, Jones D, et al. Air pollution and stillbirth risk: exposure to airborne particulate matter during pregnancy is associated with fetal death. PLoS ONE. (2015) 10:e0120594. doi: 10.1371/journal.pone.0120594
149. Bobak M, Leon DA. The effect of air pollution on infant mortality appears specific for respiratory causes in the postneonatal period. Epidemiology. (1999) 10:666–70. doi: 10.1097/00001648-199911000-00004
150. Zhang B, Liang S, Zhao J, Qian Z, Bassig BA, Yang R, et al. Maternal exposure to air pollutant PM25 and PM10 during pregnancy and risk of congenital heart defects. J Expo Sci Environ Epidemiol. (2016) 26:422–7. doi: 10.1038/jes.2016.1
151. Roberts JM, Escudero C. The placenta in preeclampsia. Pregnancy Hypertens. (2012) 2:72–83. doi: 10.1016/j.preghy.2012.01.001
152. Kannan S, Misra DP, Dvonch JT, Krishnakumar A. Exposures to airborne particulate matter and adverse perinatal outcomes: a biologically plausible mechanistic framework for exploring potential effect modification by nutrition. Environ Health Perspect. (2006) 114:1636–42. doi: 10.1289/ehp.9081
153. Blum JL, Chen LC, Zelikoff JT. Exposure to ambient particulate matter during specific gestational periods produces adverse obstetric consequences in mice. Environ Health Perspect. (2017) 125:077020. doi: 10.1289/EHP1029
154. Hougaard KS, Jensen KA, Nordly P, Taxvig C, Vogel U, Saber AT, et al. Effects of prenatal exposure to diesel exhaust particles on postnatal development, behavior, genotoxicity and inflammation in mice. Part Fibre Toxicol. (2008) 5:3. doi: 10.1186/1743-8977-5-3
155. Liu Y, Wang L, Wang F. Li C. Effect of fine particulate matter (PM25) on rat placenta pathology and perinatal outcomes. Med Sci Monit. (2016) 22:3274–80. doi: 10.12659/MSM.897808
156. Ye Z, Lu X, Deng Y, Wang X, Zheng S, Ren H, et al. In utero exposure to fine particulate matter causes hypertension due to impaired renal dopamine D1 receptor in offspring. Cell Physiol Biochem. (2018) 46:148–59. doi: 10.1159/000488418
Keywords: PM2.5, disease, physiology, particulate matter, air pollution
Citation: Pryor JT, Cowley LO and Simonds SE (2022) The Physiological Effects of Air Pollution: Particulate Matter, Physiology and Disease. Front. Public Health 10:882569. doi: 10.3389/fpubh.2022.882569
Received: 01 March 2022; Accepted: 15 June 2022;
Published: 14 July 2022.
Edited by:
Yang Liu, Emory University, United StatesReviewed by:
Lei Huang, Nanjing University, ChinaHasan Mahmud Reza, North South University, Bangladesh
Copyright © 2022 Pryor, Cowley and Simonds. This is an open-access article distributed under the terms of the Creative Commons Attribution License (CC BY). The use, distribution or reproduction in other forums is permitted, provided the original author(s) and the copyright owner(s) are credited and that the original publication in this journal is cited, in accordance with accepted academic practice. No use, distribution or reproduction is permitted which does not comply with these terms.
*Correspondence: Stephanie E. Simonds, c3RlcGhhbmllLnNpbW9uZHNAbW9uYXNoLmVkdQ==