- 1Institute of Clinical and Biomedical Sciences, University of Exeter Medical School, Exeter, United Kingdom
- 2National Obesity Centre and Endocrinology and Metabolic Diseases Unit, Yaounde Central Hospital, Yaoundé, Cameroon
- 3Academic Department of Clinical Biochemistry, Royal Devon and Exeter NHS Foundation Trust, Exeter, United Kingdom
- 4Department of Internal Medicine and Specialities, Faculty of Medicine and Biomedical Sciences, University of Yaoundé 1, Yaoundé, Cameroon
- 5Macleod Diabetes and Endocrine Centre, Royal Devon and Exeter NHS Foundation Trust, Exeter, United Kingdom
The phenotype of type 1 diabetes in Africa, especially sub-Saharan Africa, is poorly understood. Most previously conducted studies have suggested that type 1 diabetes may have a different phenotype from the classical form of the disease described in western literature. Making an accurate diagnosis of type 1 diabetes in Africa is challenging, given the predominance of atypical diabetes forms and limited resources. The peak age of onset of type 1 diabetes in sub-Saharan Africa seems to occur after 18–20 years. Multiple studies have reported lower rates of islet autoantibodies ranging from 20 to 60% amongst people with type 1 diabetes in African populations, lower than that reported in other populations. Some studies have reported much higher levels of retained endogenous insulin secretion than in type 1 diabetes elsewhere, with lower rates of type 1 diabetes genetic susceptibility and HLA haplotypes. The HLA DR3 appears to be the most predominant HLA haplotype amongst people with type 1 diabetes in sub-Saharan Africa than the HLA DR4 haplotype. Some type 1 diabetes studies in sub-Saharan Africa have been limited by small sample sizes and diverse methods employed. Robust studies close to diabetes onset are sparse. Large prospective studies with well-standardized methodologies in people at or close to diabetes diagnosis in different population groups will be paramount to provide further insight into the phenotype of type 1 diabetes in sub-Saharan Africa.
Introduction
Type 1 diabetes (T1D), formally referred to as “juvenile diabetes” or “insulin-dependent diabetes mellitus (IDDM)”, is one of the most commonest diseases of childhood and adolescence, although it can occur at any age, and it is likely that the majority of cases occur in adults (1, 2). The incidence of type 1 diabetes varies considerably from one region of the world to another (3, 4). The classical form of the disease is generally often thought to be less frequent in sub-Saharan Africa (SSA) compared to Europe and North America (5). However, robust epidemiological data on the incidence and prevalence of type 1 diabetes in the African continent are scarce. Estimates from some sub-Saharan African countries have found that the incidence of type 1 diabetes varies from 1.5 to 10.1 per 100 000 depending on the country and age group studied (6). In 2019, the International Diabetes Federation (IDF) estimated that about 25 000 children and adolescents aged <20 years have diabetes in Africa (7). There are suggestions that this number is an underestimation of the true burden of type 1 diabetes in sub-Saharan Africa, given the lack of data from many countries and high disease mortality. It is thought likely that many people with type 1 diabetes may die before a diagnosis is made (8). Therefore, the true economic burden of the condition in Africa is largely unknown.
Atypical young-onset diabetes, including patients with the type 1 diabetes phenotype but who do not appear to have the absolute insulin requirement seen in classical type 1 diabetes, has been reported in African populations (9, 10). Some authors have argued that there may be differences in genetic and autoimmune underpinnings between type 1 diabetes in patients of African and European descent (6, 9, 10), Whether these differences are true remains debatable as most of the studies that have investigated the condition in SSA are limited by small sample size numbers, the use of non-robust type 1 diabetes case definition the use of non-standardized biological assays and the possibility that many reported differences in cross sectional studies (such as high levels of retained insulin secretion) are a result of survival bias (9, 11–13).
Recently, there has been a rising interest in phenotyping type 1 diabetes in African populations. While type 1 diabetes in sub-Saharan Africa is slowly gaining prominence in international research collaborations (11, 14), one key question remains; what is the true phenotype and etiology of type 1 diabetes in sub-Saharan Africa? This has implications for understanding the etio-pathogeny of the condition and providing adequate clinical management strategies. This review aims to provide an up-to-date insight from previously published literature into the characteristics of type 1 diabetes in sub-Saharan Africa, focusing on the clinical description, endogenous insulin secretion, and the contribution of autoimmunity and genetic markers. We also review the context and challenges of type 1 diabetes research and care in sub-Saharan Africa. This understanding is crucial in setting up future research priority areas for type 1 diabetes in sub-Saharan Africa.
The context and challenges of type 1 diabetes research and care in sub-Saharan Africa
There are several critical challenges to understanding the phenotype and etiology of T1D in SSA. These challenges can be summarized as the absence of robust epidemiological and clinical research on T1D, difficulties in making a correct diagnosis, and suspected mortality attributable to poor insulin access and therapeutic management (9, 13, 15, 16).
The majority of epidemiological and mechanistic research focusing on T1D in SSA dates back to the early 80s and 90s (13). While results were often intriguing, these studies were frequently limited by small sample sizes, limited geographical representation and/or the methodologies employed. Most of the early research interest was fueled by the suggestion that T1D in African populations may differ from the classical form described in western countries, with many studies suggesting links to malnutrition in a subset of those with apparent type 1 diabetes, and ketosis prone type 2 diabetes also described (9, 17). However, this enthusiasm for T1D research diminished in the late 90s and 2000s. This was probably due to the global drive to tackle infectious diseases such as HIV/AIDS, Tuberculosis and Malaria, which were associated with excess mortality in developing countries (18). This targeted focus on infectious diseases meant that funding and research-driven initiatives for non-infectious conditions such as T1D were relegated to the rear of the global health priorities for the region. However, there are a few recent studies from an increasing number of research collaborations between countries in SSA and high-income countries. High-quality longitudinal studies in T1D in SSA are, however, still lacking.
The problem of case definition and diagnosis of type 1 diabetes in sub-Saharan Africa
The diagnosis of T1D is usually made in symptomatic individuals who present with clinical features suggestive of type 1 diabetes which include younger age, low BMI, ketonuria and presence of autoimmune disease. However, T1D can be difficult to identify clinically, with features overlapping between T1D and other forms of diabetes. As a result, misclassification of diabetes at diagnosis can occur even in countries with high resources and access to classification tests such as islet autoantibodies and C-peptide (19–21). In SSA, the diagnosis of T1D is mainly based on clinical features: classification biomarker tests are rarely available (5). The implications of this are that many people clinically diagnosed with T1D may have other types of diabetes, given the predominance of atypical diabetes presentation in Africa and people of African ancestry.
Atypical presentations of type 2 diabetes (T2D) are known to be predominant in Africa and may further increase the challenge of differentiating between type 1 and 2 diabetes using clinical features alone (9). For example, frequent atypical diabetes forms in SSA include adults with non-obese T2D or those with ketosis-prone diabetes (KPD) (11). Non-obese or lean T2D (BMI <25 kg/m2) is common and has been extensively studied in South Asian populations. Studies from Asian populations have demonstrated that non-obese or lean non-autoimmune (‘type 2' diabetes is characterized by younger age and indices of insulin resistance (lower glucose disposal, high insulin levels, higher total abdominal and visceral fat), though beta cell failure remains a critical contributor to the development of diabetes (22–25). While lean T2D has been less comprehensively studied in African populations, a recent study from Uganda found that about one-third of people with adult-onset diabetes had lean non-autoimmune (apparent type 2) diabetes (26). In this study, lean T2D was associated with male sex and beta-cell deficiency without evidence of increased insulin resistance. This means that a significant number of people with low BMI and young age (factors that would support a type 1 diagnosis) in African populations may have non-autoimmune (type 2 diabetes) diabetes.
The paradox of malnutrition-related diabetes mellitus (MDRD)
Non-autoimmune young-onset diabetes has frequently been described as related to malnutrition in some SSA's impoverished communities. This clinical entity was initially called Malnutrition-Related Diabetes Mellitus (MRDM) (27) and figured in the 1985 World Health Organization (WHO) diabetes classification (28). This was initially described as a diabetes phenotype common in the young (age <30 years), with clinical features of malnutrition, not prone to ketosis, receiving high insulin doses, and living in deprived socioeconomic settings. MRDM is no longer considered a specific diabetes type by the WHO for lack of substantial and consistent data (29). MDRD was further separated into fibro calculous pancreatic diabetes mellitus (FCPD) and protein-deficient or malnutrition-modulated diabetes mellitus. These are atypical forms of diabetes common in lean young males with evidence of chronic clinical malnutrition and could easily be mistaken for type 1 diabetes. These two diseases were described to have similar clinical features with people with FCPD having radiological evidence of pancreatic calcification. There have been numerous reports of FCPD or Tropical Calcific Pancreatitis (TCP) in settings of Africa and Asia (India) that are severely deprived (30, 31). A recent study from a carefully selected group of male adults with low BMI diabetes and a history of malnutrition from India showed they had significantly lower measures of insulin resistance and beta cell function than those with overt type 2 diabetes (32). However, it remains a controversial topic (32, 33).
Ketosis-prone diabetes is common in African populations and can be mistaken for type 1 diabetes at diagnosis
Ketosis-prone diabetes (KPD) is highly prevalent in Africa and populations of African descent (African-Americans, Afro-Carribeans) but also in some Hispanic and Asian populations (34, 35). People with KPD are often young, middle-aged men who usually present with severe ketosis and high insulin requirements (17). Hence, this group of people with diabetes can easily be mistaken for having T1D. However, they do not have evidence of autoimmunity or type 1 diabetes-related HLA and frequently do not require long-term insulin treatment (17, 36). KPD is also associated with a strong family history of type 2 diabetes with markers of insulin resistance (37–39). Studies have shown that lipid parameters in KPD seem similar to those with T2D in African and Asian populations, although hypertriglyceridemia has been strongly linked to KPD in some Asian populations (40, 41).
In summary, the predominance of atypical presentations of diabetes amongst African populations and limited access to classification tests (islet autoantibodies, C-peptide) in many areas of sub-Saharan Africa mean that robustly diagnosing T1D is challenging, and clinically diagnosed T1D is therefore likely to encompass a range of underlying diabetes pathophysiology. The implications of this are that many phenotype studies may be examining mixed etiology populations, those with and without true autoimmune “type 1” diabetes. This may explain some differences between studies in SSA and T1D cohorts in high-income countries, such as later diabetes onset and lower islet antibody prevalence (5, 6, 42–45).
High mortality associated with type 1 diabetes in sub-Saharan Africa
T1D in SSA has been associated with very high mortality, especially around the first years of diagnosis (16). This high mortality is often attributed to misdiagnosis and poor insulin access (5, 16, 46). Misdiagnosis of acute diabetes onset, especially in younger children, is common in SSA. It is thought that in many African countries, a substantial proportion of children and young adults with diabetes die before diagnosis or during their first admission from diabetic ketoacidosis (47). Historical evidence suggests that the mortality rate of T1D in Africa after diagnosis may be as high as 80%, similar to the pre-insulin era (16). The reasons for inadequate or poor insulin access in Africa are numerous, ranging from elevated market prices due to the monopoly of few insulin manufacturing industries, challenging regulatory ecosystem for biosimilar “generic” insulins, inadequate procurement and distribution systems to the irrational allocation of health resources within poorly-constructed health systems in Africa (15, 48). Even when insulin treatment is available, its accessibility and proper use is limited due to its high costs and the absence of blood glucose testing devices and accessories needed for adequate control (11, 49).
Early and high type 1 diabetes may significantly affect the phenotype landscape of those with young-onset diabetes. High mortality may alter the phenotype of people with diabetes recruited into cross-sectional studies. Therefore, individuals seen in the multiple cross-sectional studies conducted across SSA may not represent the true population of incident T1D. Hence the phenotype of those who survive (survival effect) may be enriched for those who do not develop absolute insulin deficiency or misdiagnosed individuals with other forms of diabetes.
The introduction of free insulin donation programmes in sub-Saharan Africa
For the past two decades, sub-Saharan Africa has seen the birth of foreign-funded insulin donation programmes. Examples of such programmes are Life For A Child (LFAC) and Changing Diabetes in Children (CDiC) programmes (50). These programmes tend to enroll and cater for children and young adults <25 years old while providing training and capacity building to health workers toward diagnosing and managing T1D. A crucial observation from these programmes is that a targeted intervention may improve the capacity of health systems in SSA to recognize and diagnose younger-aged children with diabetes even in precarious conditions such as DKA, which were often missed in the past leading to early mortality (51, 52). While the longterm impact of these programmes on the health systems is yet to be completely understood, recent evidence suggests that mortality has improved in countries where these programmes have been introduced (53, 54).
The clinical characteristics of patients with type 1 diabetes in sub-Saharan Africa
The diagnosis of type 1 diabetes in SSA is primarily or solely based on clinical features. These clinical features include the age of diabetes onset, low or normal BMI, and acute presentation, often with ketoacidosis (55, 56). Table 1 shows summary findings of the clinical characteristics of patients with T1D at or close to diagnosis in SSA. Importantly, in the absence of classification biomarker testing, the clinical features of a population with clinically diagnosed T1D will reflect those features that the clinician considers relevant rather than necessarily the true phenotype of autoimmune etiology diabetes in these populations.
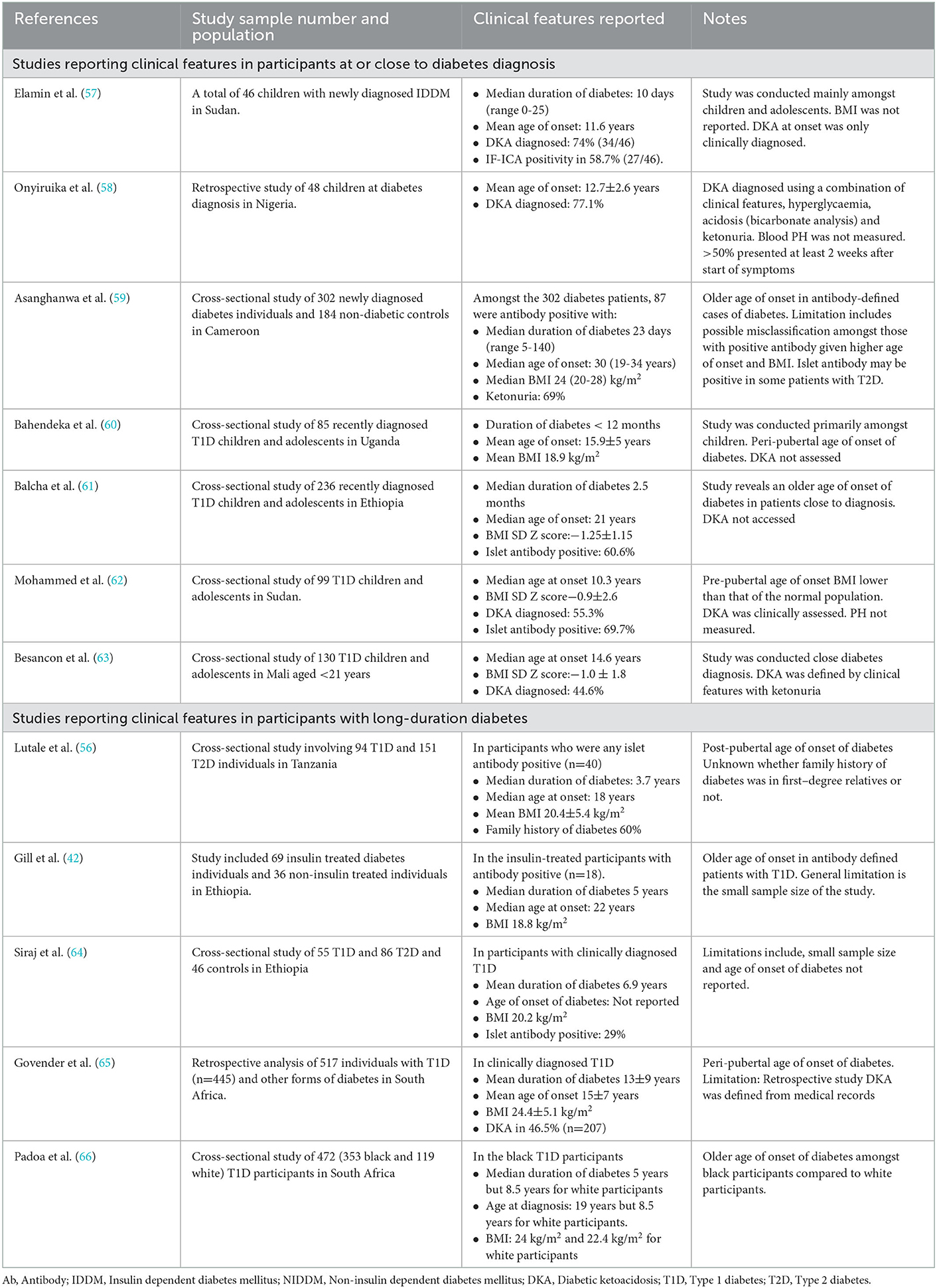
Table 1. Summary of studies reporting the clinical features of people with type 1 diabetes in sub-Saharan Africa.
Previous studies show the peak age of onset of T1D is in the second decade of life in sub-Saharan Africa
Traditionally, the peak age of onset of type 1 diabetes has mostly been studied amongst children and adolescents. However, recent evidence suggests that the incidence of T1D in adults may be almost as high as that seen in children and adolescents in developed countries (data from low-and-middle income countries is lacking) (67). Interestingly, a later peak age of onset seems disproportionately reported in the African literature (9). Most studies from SSA have consistently reported that the peak age of onset of T1D occurs about a decade later than the age of diabetes onset observed in people with T1D in Caucasian populations (43, 68). The reason for this observed difference in the peak age of onset of diabetes between patients of African and European ancestry is unknown. High and early mortality among younger patients with T1D in most African countries due to poor medical infrastructure may be a plausible explanation (46, 69). Whether differences in peak age of onset point to heterogeneity in phenotype is unclear. Recent evidence from pancreatic immunohistological studies from developed countries shows that T1D may exist in two distinct endotypes depending on the age of onset of diabetes (70). Findings from these studies have reported that children diagnosed with diabetes <7 years had a hyperimmune insulitis pattern characterized by a lower proportion of residual insulin-containing beta cells, markers of anomalous proinsulin processing, lower peripheral C-peptide levels with higher proinsulin/C-peptide ratios. Contrariwise, children diagnosed with diabetes ≥13 years had a pauci-immune insulitis pattern, with many more beta cells with residual insulin, less signs of anomalous proinsulin processing, higher levels of peripheral C-peptide, and lower proinsulin-to–C-peptide ratios (71–73). The differences observed in the age of diabetes onset in black vs. white South Africans may suggest that a hyperimmune endotype may be common in white vs. pauci-immune endotype being common in black South Africans. Unfortunately, there are no pancreatic immunohistological studies in African populations to assess this hypothesis.
In a recent study in South Africa, Padoa et al. described a bimodal distribution of the age of onset of the disease among the black South African population at 11–15 years and 26–30 years, whereas the peak age of onset was between 0 and 10 years in white South Africans. Also, more than 60% of white South African people with diabetes were diagnosed before 10 years of age (66). A similar pattern has previously been described in black South Africans by Kalk and colleagues (43). Major limitations to these studies are the limited sample sizes, T1D was clinically defined at study onset, only a small proportion of participants were close to diagnosis, and C-peptide was performed only in a small group of participants in the study conducted by Kalk and colleagues. In the study conducted by Padoa and colleagues, the seroprevalence of the GAD65 autoantibody significantly reduced from 73% in those diagnosed at an age < 21 years to 42% in participants diagnosed ≥ 21 years in the black population. This significant reduction was not observed in the white population. Given that atypical diabetes presentations are common in black Africans, the older peak age of onset of diabetes and the reduction of the positivity rate of GAD65 autoantibody may suggest non-autoimmune diabetes in these younger black adults – a finding that has been recently demonstrated in older adults diagnosed with T1D in Europe, where a reduction in islet antibody prevalence with older age in clinically diagnosed T1D appears to reflect misclassification (74, 75). Another recent study in Ethiopia amongst a group of carefully selected patients with young-onset diabetes requiring insulin treatment at diagnosis showed that the median age was 21 years. Only 15.3% were diagnosed at <15 years (61). Though islet autoantibodies were not used to define autoimmune diabetes from onset, the proportion of GAD autoantibody reduced significantly from 80.6% in those <15 years to 43.5% in those >26 years.
In contrast, only a handful of studies in SSA have reported a younger age of onset of T1D, between 10 and 14 years (55, 60, 76), closer to what is described in developed countries. These studies have mainly been conducted in cohorts of children and adolescents with diabetes in insulin donation programs which usually focus on providing care to children under 18 years. Islet autoantibody and C-peptide studies have not been routinely performed in these cohorts. Therefore the proportion of those with true autoimmune T1D is unknown.
Anthropometric characteristics and family history of diabetes should be considered with caution
Low BMI in young-onset diabetes is usually used as a clinical criterion to identify T1D in SSA. While people with T1D are typically lean, low-to-normal BMI should be considered cautiously since non-obese T2D is common amongst populations of African descent (77). Also, the global increase in the rates of childhood obesity, including in some parts of Africa, may further render the use of BMI challenging in differentiating T1D from T2D. Furthermore, there is increasing evidence that T1D may occur in the presence of obesity (78).
Also, the absence of a family history of diabetes should be avoided as a clinical criterion for T1D. Generally, about 10–20% of individuals with T1D may have a family history of diabetes in a first-degree relative. The risk in first-degree relatives is about 15 times higher than in the general population (79). The heritability risk profile for T1D has been extensively studied in other populations but is utterly unknown in SSA. Few studies from SSA have generally pointed out that about 20–40% of people with T1D have a first-degree relative with diabetes (42, 55, 80). However, the specific type of diabetes was not mentioned.
Diabetic ketoacidosis (DKA) is a common clinical feature in the presentation of type 1 diabetes
Studies from SSA show that the reported DKA at diabetes diagnosis is highly variable, from 20 and 80% depending on the population studied (55, 58, 80–82). For most of these studies, DKA has not been rigorously defined, with the diagnosis of DKA based on clinical presentation and urine ketones (83). This may explain the high rates in some studies (false positives) when DKA may be absent. Conversely, if DKA is not robustly defined, the false negative rate would be very high. Hence, children with DKA may be misdiagnosed and treated for other conditions, such as severe malaria or meningitis (82, 83). In the few studies where blood ketones confirmed DKA at diagnosis, its prevalence was <40%. This estimate remains substantially high compared to the rate of DKA at diabetes onset in high-income countries (84). In SSA, the time from onset of symptoms to hospital admission can be very long, allowing acidosis to develop in the absence of proper insulin treatment. This may explain why DKA rates are very high in patients with T1D at diagnosis in SSA.
In summary, one may argue that based on current evidence and limitations surrounding case definition in previously conducted studies, and excessively high early type 1 diabetes-associated mortality in African children, it is unclear whether the age of onset of T1D is different in SSA compared to that reported elsewhere. Also, low-to-normal BMI and the absence of a family history of diabetes should not be considered in isolation to make a clinical diagnosis of diabetes in people with young-onset diabetes in SSA. While they are important clinical parameters, they must be considered with other biochemical features to strengthen the robustness of a diagnosis of T1D.
Type 1 diabetes and autoimmunity in sub-Saharan Africa
Classical type 1 diabetes is a metabolic disease marked by absolute or near-absolute insulin deficiency, usually resulting from autoimmune destruction of the beta cells of the islet of Langerhans (3). The commonest autoantibody markers present in T1D are insulin autoantibodies (IAA), glutamic acid decarboxylase (GAD) autoantibodies, islet antigen 2 (IA-2) autoantibodies and variants of the zinc transporter 8 (ZnT8) autoantibodies (85, 86). Usually, at least one autoantibody is present in over 90% of cases with T1D at the time of diagnosis (75, 86, 87). In clinically suspected T1D, a positive islet antibody will confirm the diagnosis, although the absence of the autoimmune markers does not rule out the presence of the condition (88).
Studies in sub-Saharan Africa have suggested a lower seroprevalence rate of islet autoantibody in people with type 1 diabetes than elsewhere
Table 2 shows a summary of the seroprevalence of different immunological studies that have been carried out in SSA. These studies have consistently reported lower rates of islet autoantibodies in clinically diagnosed T1D populations than in developed countries (56, 59, 60, 64, 91). This suggests a possible difference in the etiological underpinnings between populations of African and European descent. Racial differences in the prevalence of islet autoantibodies have also been confirmed in immunological studies between black and white individuals living in the African continent. In a more recent analysis of patients with T1D in South Africa, the prevalence of islet antigen 2 (IA2) autoantibodies was 19% (66/352) in black and 41% in white participants (48/118; P < 0.001) (66). However, this study did not show any significant difference in GAD (65 kDa) autoantibody positivity between the two ethnicities. The overall median duration of diabetes was 6 years in the study. Furthermore, most immunological studies among people with T1D in SSA have been cross-sectional analyses in people with long-standing diabetes (usually over 3 years).
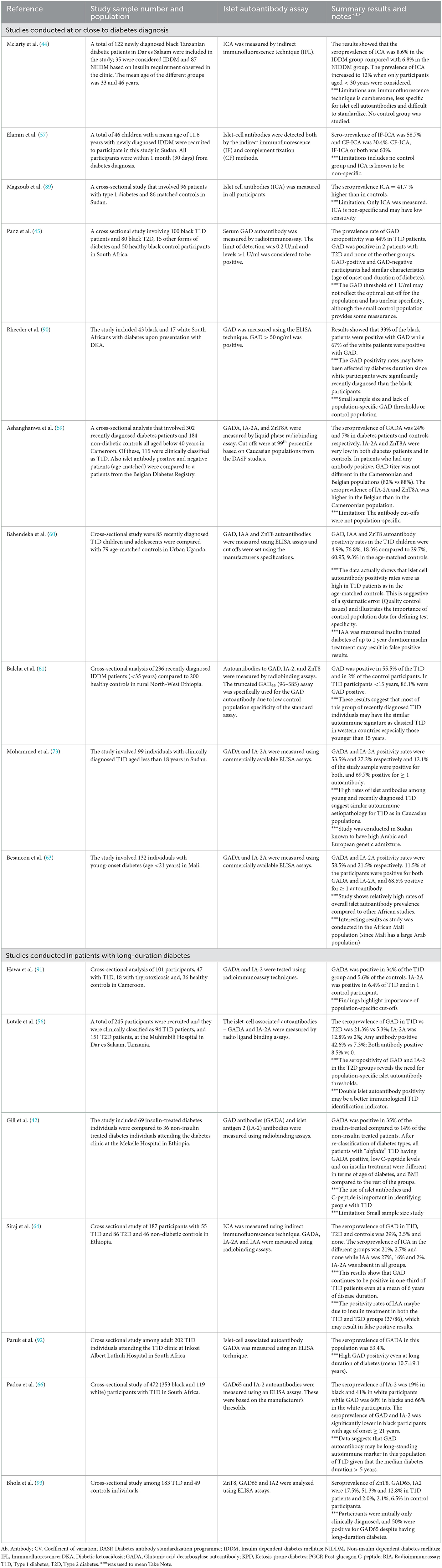
Table 2. Summary of studies reporting the seroprevalence of islet autoantibodies in type 1 diabetes in sub-Saharan Africa.
Some recent immunological studies in insulin-requiring young-onset diabetes individuals have been conducted at or close to diagnosis. For example, in Sudan, a recent study of clinically diagnosed T1D patients <18 years old who were close to diagnosis (<18 months of diabetes duration) showed that GAD and IA-2 autoantibody positivity rates were 53.5 and 27.2%, respectively, with an overall 69.7% positive for at least one autoantibody (62). Also, Balcha and colleagues in Ethiopia showed that the overall seroprevalence of GAD65 autoantibody among newly-diagnosed T1D patients aged <35 years was 55.5% (61). The age-specific seroprevalence of GAD autoantibody was 80.6% (29/36) in those <years, 55.6% (69/124) in the age group 16–25 years, and 43.4% (33/76) in those 26–25 years. IA-2 and ZnT8 positivity rates were generally extremely low irrespective of the age of onset of diabetes. This study enrolled 236 newly-diagnosed patients with insulin-dependent diabetes mellitus (median duration of diabetes was 2.5 months) from a rural setting (61). For the first time, an immunological study done in SSA reported rates of GAD autoantibody as high as that seen in Caucasian populations in those <15 years. It is also worth noting that the GAD assay used in this study was the highly specific truncated GAD65 (96–585) (chosen because of the low specificity of the standard assay in this population described above). These findings underscore that many more studies conducted close to diabetes onset employing robust immunological methods are needed in other parts of the continent to verify these findings in carefully selected cohorts of patients.
These findings are a stark contrast to most of the previous immunological studies conducted in SSA, which have reported low islet autoantibody titers. The differences may come from the timing of the different studies, which were often conducted in groups with varying diabetes duration. Immunological studies among people with T1D close to diagnosis should be encouraged in other African settings. It is important to note that differences may also result from the populations studied. Sudan and Ethiopia are countries located around the horn of Africa with solid historical exchanges with populations in the Middle East, where T1D is very prevalent (94, 95). The Amhara ethnic group from North Ethiopia seem to be a genetically very distinct population group from other black African groups. The relative genetic contribution to T1D susceptibility of this population compared to other black African Bantu and Semi-Bantu populations of SSA to autoimmunity may be different.
In studies where multiple islet autoantibodies have been measured, GAD autoantibody is often strikingly the most commonly detectable autoantibody. The seroprevalence of GAD autoantibody in people with long-standing (>3 years) T1D is estimated at 20–60% (56, 93). Very few studies in SSA have measured IA-2 and ZnT8 autoimmune markers and have reported absent or low rates close to or away from diabetes diagnosis (59, 61, 64, 66). Whether low rates of IA-2 and ZnT8 autoantibodies dispute the relative contribution of these markers to the autoimmune pathogenesis of T1D in SSA is a subject for future research. However, there are suggestions that the differences in the seroprevalence of GADA, IA-2A and ZnT8A observed between Africans, Asians, and Caucasians may arise from differences in background frequency of type 1 diabetes HLA risk alleles (96). For example, HLA DR4 haplotypes are more predominant in Caucasian populations than Africans and Asians. Furthermore, IA-2A and ZnT8A have been shown to be strongly associated with HLA DR4 which may explain their lower prevalence in African populations (96–98). Conversely, HLA DR3 seems to be highly predominant in populations of African ancestries, which may explain the higher rates of GADA in African populations compared to the other islet autoantibodies (59, 66, 96). Therefore, based on current evidence, GAD autoantibody appears to be the single best islet autoantibody to test for in African populations.
In summary, the vast majority of immunological studies conducted among people with T1D or young-onset diabetes have shown very low rates of islet autoimmune markers compared to elsewhere. Two recent studies have demonstrated high islet autoantibody detection rates. Possible reasons for the lower rates of islet autoimmune markers previously reported in multiple studies and their associated limitations are discussed in the subsequent sections.
Reasons for lower rates of islet autoantibody in people clinically diagnosed with T1D in sub-Saharan Africa
These lower rates of islet autoantibody titers in people with T1D in SSA suggest that non-autoimmune diabetes etiologies may be common in those diagnosed with T1D (9). This may result from the high prevalence of atypical forms of diabetes in African populations (discussed above) that will be extremely challenging to distinguish from autoimmune type 1 diabetes without classification biomarker testing. This may be exacerbated by the low prevalence of ‘true' autoimmune etiology type 1 diabetes in these populations, with studies of confirmed autoimmune type 1 diabetes suggesting autoimmune diabetes is much less frequent than seen in many other regions. Several hypotheses have been put forward to explain lower rates of auto-immune type 1 diabetes in African populations. This includes the widespread practice of infant breastfeeding and “immune priming” from recurrent childhood infections. While prolonged maternal breastfeeding may be protective, there is substantial evidence to show that formula feeding may increase the risk of developing islet-cell autoimmunity (99, 100). Also, recurrent childhood infection is commonplace in SSA and may contribute to immune modulation leading to generally lower rates of autoimmune diseases, including T1D (13, 101). These postulated hypotheses have never been verified in prospective studies in African populations. Other possible mechanisms that could result in lowered islet-cell autoimmunity in those with clinically diagnosed T1D in SSA may be the high prevalence of background intestinal helminth infestation in infants and children (102, 103). Intestinal helminths are well known for modulating their host's immune response. They release immunomodulatory molecules, which are now recognized to interfere with the host's innate and adaptive immune response (104). The impact of such immune molecules on the development of T1D has been extensively studied in animal models but not humans.
Another reason for the lower rates of islet autoantibody in people with a clinician diagnosis of type 1 diabetes in SSA might result from background genetic differences between Africans and other population groups. As discussed in the previous section, there is a great body of evidence suggesting that type 1 diabetes-associated HLA haplotypes DR3 and DR4 are comparatively less predominant in African populations than in Asian, Arabs and European populations (96, 105). A low population background distribution of these HLA haplotypes may influence the islet autoantibody titer levels given the strong association between HLA haplotype and islet autoantibody appearance and titers (86, 87).
Limitations in many immunological studies conducted amongst people with type 1 diabetes in sub-Saharan Africa
Most immunological studies in sub-Saharan Africa showing lower rates of islet autoimmune markers than those in developed countries have been conducted in small sample numbers. They would usually employ non-standardized methods, reporting positivity thresholds based on cut-offs set by the manufacturer's instructions. Population-specific reference ranges for positive islet autoantibody testing are essential (85, 106). To our knowledge, no study from sub-Saharan Africa has reported population-specific thresholds for islet autoantibodies. Notably, testing 152 controls without diabetes in a recent Ethiopian cohort, using a well-established GAD assay with high specificity in European populations, suggested low specificity with 8.5% of those without diabetes testing positive. This indicates that population-specific thresholds may be particularly important for the robust definition of autoimmune diabetes in African populations.
There is also limited available data from prospective studies on the impact of duration of diabetes on the kinetics of these islet-cell autoimmune markers.
Characteristics of people with islet autoimmune positive type 1 diabetes in SSA
Numerous studies from SSA show that C-peptide levels are lower in people with T1D who are positive for islet autoantibodies compared to autoantibody negative (42, 59, 61, 90). Other characteristics of islet autoantibody-negative T1D show they are older-aged and have higher BMI (59, 93). This strongly suggests the presence of alternative etiologies of diabetes amongst those with islet autoantibody-negative diabetes.
Beta-cell function and endogenous insulin secretion in type 1 diabetes patients in sub-Saharan Africa
Type 1 diabetes usually results in near absolute loss of insulin secretion due to the autoimmune destruction of the beta-cells of the islet of Langerhans of the pancreas. Idiopathic loss of endogenous insulin secretion is also defined as type 1 diabetes in some international definitions (3, 107). The loss of endogenous insulin secretion drives the treatment requirements of T1D. Endogenous insulin secretion in those treated with insulin can be assessed using C-peptide, a peptide secreted in equimolar concentrations with insulin (108). Low levels (<200 pmol/L in blood or urine after stimulation) confirm the treatment requirement of type 1 diabetes and are seen in the vast majority of those with young onset type 1 diabetes with a duration of diabetes over 3 years in other populations (108).
C-peptide levels are low in Type 1 diabetes in sub-Saharan Africa
Table 2 shows the list of studies that have reported on assessing endogenous insulin secretion in SSA. A general limitation applicable to the vast majority of studies is the use of clinically diagnosed T1D, without islet autoantibody confirmation. This means that retained insulin secretion may reflect the classification challenges discussed above rather than a specific phenotype of African autoimmune type 1 diabetes. Studies conducted at or close to diagnosis have generally shown low but detectable C-peptide levels, substantially lower than comparable populations of other diabetes types were available (42, 59, 60, 90, 109). Long-duration studies suggest much higher levels of retained endogenous insulin secretion than in other type 1 diabetes populations. Of note, studies comparing islet antibody-positive and negative participants have consistently shown higher C-peptide levels in antibody-negative individuals. This may indicate the prevalence of other diabetes subtypes in those without islet antibodies, which is also supported by lower type 1 diabetes HLA susceptibility in this group in the recent study by Balcha and colleagues (61).
Clinical reports suggesting retained endogenous insulin secretion in SSA
Some clinical observations and studies have reported the existence of groups of people with “type 1 diabetes” like phenotype who are insulin-requiring but can interrupt their routine insulin treatment without developing severe metabolic complications (9, 65, 110). This has suggested that some patients with T1D in SSA may have substantial levels of preserved endogenous insulin secretion. However, studies of C-peptide in long-duration clinically diagnosed T1D (Summarized in Table 3) suggest that this will be a modest proportion of patients. In other populations, routine C-peptide testing has led to treatment change and successful insulin withdrawal in a subset of those with clinically diagnosed T1D (20) and is likely to be cost-effective. A similar strategy may have utility in African populations where clinical classification is the mainstay of diagnosis, with the cost of C-peptide testing similar to or less than the cost of a single insulin vial.
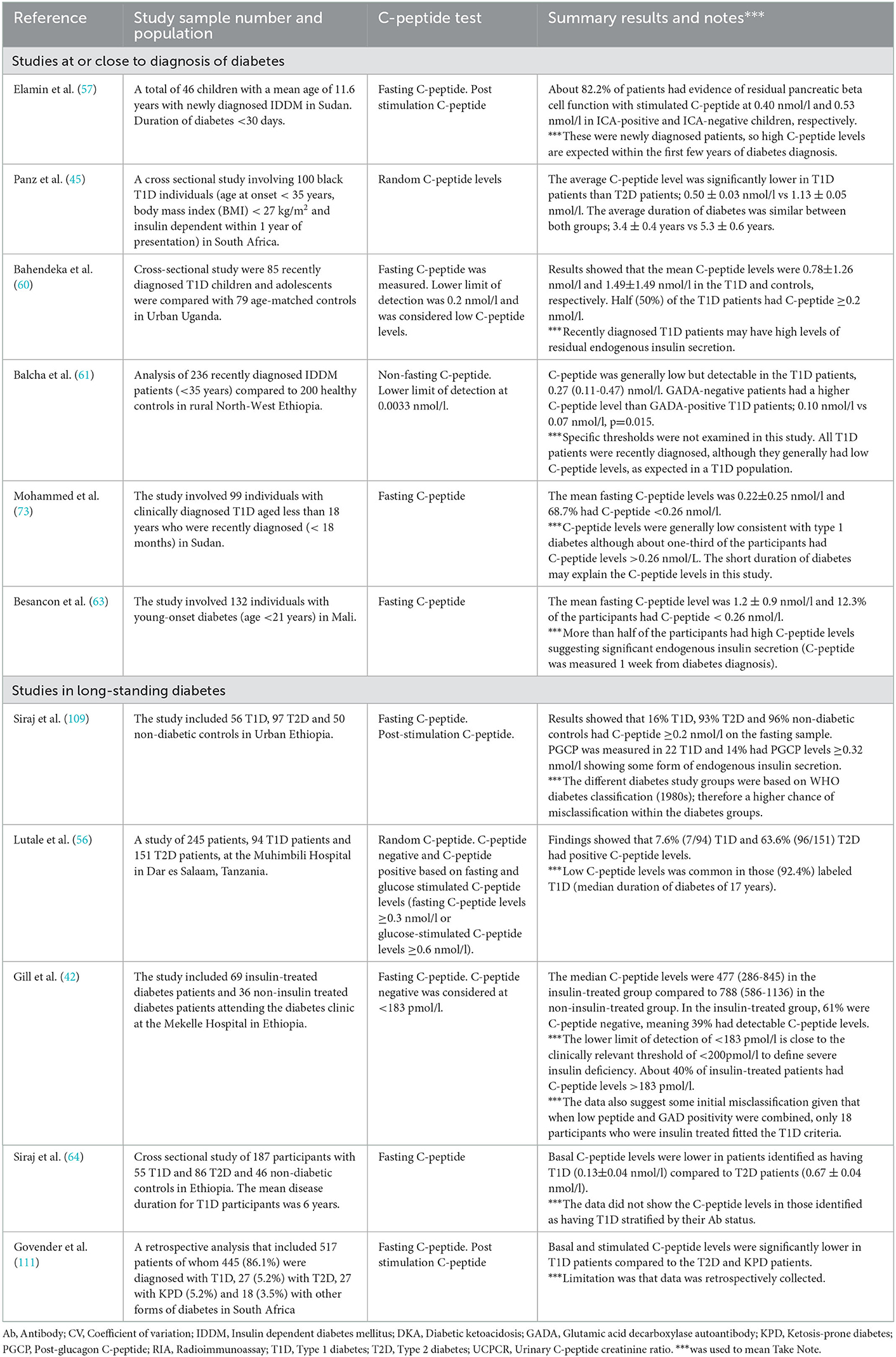
Table 3. Summary of studies reporting the C-peptide levels and the assessment of endogenous insulin secretion in type 1 diabetes in sub-Saharan Africa.
Genetics of type 1 diabetes in sub-Saharan Africa
Genetic factors are known to contribute to the pathogenesis of T1D, and approximately 95% of people with the disease have T1D associated polymorphisms of class II Human Leucocyte Antigen (HLA) genes (88, 112). These HLA genes provide the greatest contribution to overall genetic susceptibility. Type 1 diabetes is known to be strongly associated with HLA-DR3-DQ2 and HLA-DR4-DQ8 haplotypes (87). Apart from these HLA genes, about 60 loci have been associated with susceptibility to T1D through genome-wide association studies (113).
HLA DR3 has the highest genetic susceptibility for type 1 diabetes patients in sub-Saharan Africa
Table 4 shows a summary of the studies that have been conducted in SSA examining the association between HLA genes and T1D. These studies generally report that the commonly reported HLA genes associated with T1D elsewhere are also found in people with T1D in SSA. In some early studies, Magzoub and colleagues in 1991 showed that the HLA DQB1*0201 ad DQA1*0301 were strongly associated with IDDM patients in Sudan compared to ethnically-matched non-diabetic controls (115). Several small-scale studies have further described the association between HLA DR3 genes and type 1 diabetes in West and East African populations (115–118). When compared to DR3 genes, DR4 genes seem to be less frequent. Also, HLA genes known to confer protection from T1D have been reported in SSA. In a recent study in North West Ethiopia, GAD-negative diabetes patients had the HLA-DRB1*04, including the HLA-DRB1*15 alleles (61), and the HLA-DRB1*15 allele, which has also been shown in other populations to confer one of the strongest protection for type 1 diabetes (121).
The HLA loci are highly polymorphic and vary significantly from one population to another (122). A combination of HLA DR3 and DR4 is known to confer the greatest genetic susceptibility to type 1 diabetes in Caucasian populations, while HLA-DR15 haplotypes are protective (87). The mechanisms underpinning the interaction between different HLA haplotypes to engender susceptibility or protection are poorly understood. Furthermore, very little is known about these interactions in African populations. The HLA DR4 haplotype has been demonstrated to be strongly associated with type 1 diabetes in Caucasian populations. Contrariwise, small-scale studies from African populations have shown a lower relative contribution of the HLA DR4 haplotype to type 1 diabetes susceptibility. The HLA DR3 type 1 diabetes susceptibility risk alleles are consistent with what has been observed in African Americans (11). Previous small-scale HLA genotyping studies in Cameroon and other African populations have pointed to the strong association between HLA DR3 compared to DR4 risk alleles and type 1 diabetes genetic susceptibility (119, 120). A recent study amongst newly diagnosed children and adolescents (age <21 years) and healthy controls from Mali showed that HLA DR4 risk alleles [OR 14.76 (95%CI 4.99–58.83)] were more strongly associated with T1D than HLA DR3 risk alleles [OR 2.82 (955CI 1.59–5.03)] (63). These findings generally confirm the association of HLA DR3 and DR4 haplotypes to T1D in this African population, though however limited by the small sample sizes of the groups analyzed. HLA DR3 allele-associated type 1 diabetes risk has also been described in Asian populations (123). Whether the differences in the HLA risk profiles between African and Caucasian populations contribute to the pattern and combinations of islet autoantibodies is unknown. Some studies have suggested that other HLA risk profiles, such as DR7 and DR9 genes, confer extremely high type 1 diabetes susceptibility in African-ancestry populations, although this has not been well elucidated (124). Nevertheless, the differences in HLA haplotypes observed in Africans and Caucasians with type 1 diabetes may further point to the distinct T1D endotypes, given the association between HLA haplotypes, islet autoantibody type, and immunohistological patterns that have recently been described. The hyperimmune T1D endotype (common in children diagnosed <7 years) is also referred to as T1DE1 or “proinsulin autoimmune-DR4” (PADR4) given that it is strongly associated with the HLA DR4 haplotype. Conversely, the pauci-immune T1D endotype (common in children diagnosed >13 years) is also called T1DE2 or the “GAD autoimmune-DR3” (GADR3) endotypes since it is strongly associated with HLA DR3 haplotype (70).
These endotypic immunogenetic differences may explain, in part at least, the reason why many African studies have reported a late-onset phenotype of T1D. There are suggestions that the rate of immune aggression may differ due to the pace of the breach to tolerance to pro(insulin) on the HLA DR4 haplotype compared to a slow-paced breach of tolerance to GAD peptides on the HLA DR3 haplotype (70, 73). However, observational and immunohistological studies from African populations will be needed to confirm this hypothesis.
Use of genetic risk scores in African populations
No single gene is either necessary or sufficient to predict the development of the condition, and multiple genes contribute to genetic susceptibility. These findings have led to the development of type 1 diabetes genetic risk scores (T1DGRS) (125). These scores provide a measure of genetic susceptibility to T1D based on information from previous studies of genetic change associated with the disease risk. The scoring system is based on combining Single Nucleotide Polymorphisms (SNPs) in the HLA region and non-HLA loci (126). There is no T1DGRS specific to people with type 1 diabetes in sub-Saharan Africa. However, Balcha and colleagues studied a 19 SNP T1DGRS using SNPs initially developed from populations of European ancestry. They found that the GRS was markedly different between those with diabetes vs. control participants in their study and was substantially higher in autoantibody-positive diabetes cases than those with islet autoantibody-negative diabetes. This result suggests that T1DGRS developed using SNPs from European populations may still help discriminate T1D from other diabetes types in other populations. A similar finding has been reported by Harrison and colleagues, who applied a 9 SNP T1DGRS developed from populations of European origins to a group of people with T1D, T2D and controls in India (123). Although there are always concerns regarding the transferability of GRSs, these findings underscore the point that T1DGRSs developed using SNPs from populations of European ancestry may help discriminate between T1D and other diabetes types in other populations while pending population-specific genetic studies and development of population specific genetic risk scores.
Limitations to type 1 diabetes genetic studies in sub-Saharan Africa
Most of these studies reporting the association of HLA genes and T1D in SSA are limited by their small sample size and type 1 diabetes case definition, primarily based on clinical features. Implications are that the frequency of the targeted genes and the effect size are lowered than expected for typical HLA genes known to contribute to T1D in other populations. This could explain why DR4 genes were not commonly reported in most studies. HLA genes are thought to be very stable across different ethnic groups and therefore are the main predicting factors of T1D genetic susceptibility (87). Also, the HLA genotyping methods employed were very dated compared to the powerful and robust contemporary genotyping methods available.
Few studies have examined the proportion of HLA risk alleles between those with islet autoantibody-positive and islet autoantibody-negative “non-autoimmune” diabetes. This is important to confirm whether participant with a clinician diagnosis of T1D and islet autoantibody-negative diabetes actually have antibody negative autoimmune T1D (suggested by genetic susceptibility HLA risk alleles) or suggested non-autoimmune disease in African populations. For example, Balcha and colleagues showed that the proportion of individuals with susceptible HLA DR3 (DRB1*03:01) haplotype was significantly higher in those with islet autoantibody-positive (52.9%) than those with islet autoantibody-negative diabetes [31.4% (61)]. However, the proportion DRB1*03:01 haplotype in those clinically diagnosed with T1D was 43.1% compared to 26.3% in controls. The reduction in the proportion of the individuals with susceptible HLA in the overall diabetes group may point to the presence of non-autoimmune diabetes especially amongst the group of individuals with islet autoantibody-negative diabetes. However, more detailed studies in other African settings are needed to confirm this hypothesis.
In summary, despite numerous methodological limitations, HLA DR3 genes have been reported in the few genetic studies conducted in SSA. Therefore, there is a need for extensive population-based studies (Genome-wise associated studies, GWAS) to be conducted in SSA to examine the relative contribution of the HLA genes, including the existing non-HLA loci known to contribute to T1D genetic susceptibility in other populations. Also, high throughput HLA genotyping studies (coupled with robust immunological methods) are needed to exclude the possible presence of a non-autoimmune form of diabetes common in children and young adults in African populations who present with T1D-like phenotype and who are negative for all commonly tested islet autoantibodies.
Perspectives and research priorities for type 1 diabetes research in sub-Saharan Africa
Many questions remain unanswered concerning the true phenotype of type 1 diabetes in sub-Saharan Africa. Some of these questions are;
• Is type 1 diabetes different in sub-Saharan African populations when misclassification and effects of poor survival have been excluded?
• Is the late-onset insulin-dependent diabetes mellitus previously described in African populations an accurate description of a distinct clinical entity or due to selection bias caused by the survival of older individuals?
• If there is a late-onset phenotype, what might be the reasons for this decade-long age gap compared to the earlier onset of diabetes observed in Caucasian populations?
• Is preserved endogenous insulin secretion a reality in young-onset diabetes or a result of misclassification and/or survival bias, and what are the determining factors?
• What is the etiology of non-autoimmune type 1 diabetes phenotype in sub-Saharan Africa?
These questions are essential to shape type 1 diabetes research and care in the African continent. Therefore, we recommend that these questions and many more can be effectively tackled by creating centers of excellence in diabetes research in sub-Saharan Africa that can leverage the experience of long-standing international diabetes research institutions in a wide-and-fair manner. Transformational training and capacity-building of young and dynamic African Research Champions interested in focusing on type 1 diabetes research and care should be the bedrock of such initiatives to ensure sustainability. We suggest that these research priority areas should focus and not exclusively on:
• The creation of an active up-to-date research culture mixed with training and capacity building especially toward the set-up of local laboratories to carry out robust C-peptide and islet autoantibody testing in the African continent.
• The development of population-specific islet autoantibody positivity thresholds with appropriate standardization within the Diabetes Antibody Standardization Programme (DASP) workshop packages to better interpret the results of islet cell immunological studies.
• Factors that may be determinant in developing non-autoimmune young-onset diabetes in sub-Saharan Africa.
• The leverage of high-resolution HLA typing methods and GWAS in large dataset studies to better understand the genetic susceptibility of T1D in sub-Saharan African populations.
• Prospective cohort studies of well-defined T1D patients across multiple sites in SSA to study disease progression, treatment response and the incidence of long-term complications.
• The impact of childhood diabetes programmes (insulin donation schemes) on mortality associated with T1D in the African continent.
Author contributions
All authors listed have made a substantial, direct, and intellectual contribution to the work and approved it for publication.
Funding
JCK and ES are partly supported by the National Institute for Health and Care Research (NIHR) grant reference 17/63/131. TJM is an NIHR Senior Clinical Senior Lecturer. Manuscript preparation was supported by the NIHR Global Health Group at the University of Exeter: Improving outcomes in sub-Saharan African diabetes through better diagnosis and treatment (Grant reference 17/63/131). The funders had no role in any part in the writing of this review or the preparation for publication.
Conflict of interest
The authors declare that the research was conducted in the absence of any commercial or financial relationships that could be construed as a potential conflict of interest.
Publisher's note
All claims expressed in this article are solely those of the authors and do not necessarily represent those of their affiliated organizations, or those of the publisher, the editors and the reviewers. Any product that may be evaluated in this article, or claim that may be made by its manufacturer, is not guaranteed or endorsed by the publisher.
Author disclaimer
The views expressed are those of the authors and not necessarily those of the National Health Service (NHS), NIHR, or Department of Health.
Abbreviations
DASP, Diabetes antibody standardization programme; GAD, Glutamic acid decarboxylase; GWAS, Genome-wide association studies; HLA, Human leucocyte antigen; IA-2, Islet antigen 2; MDRD, Malnutrition-Related Diabetes Mellitus; PGCP, Post glucagon C-peptide; SSA, sub-Saharan Africa; T1D, Type 1 diabetes; T2D, Type 2 diabetes; WHO, World Health Organization; ZnT8, Zinc Transporter 8.
References
1. Thomas NJ, Jones SE, Weedon MN, Shields BM, Oram RA, Hattersley AT. Frequency and phenotype of type 1 diabetes in the first six decades of life: a cross-sectional, genetically stratified survival analysis from UK Biobank. Lancet Diabetes Endocrinol. (2018) 6:122–9. doi: 10.1016/S2213-8587(17)30362-5
2. Leslie RD, Evans-Molina C, Freund-Brown J, Buzzetti R, Dabelea D, Gillespie KM, et al. Adult-onset type 1 diabetes: current understanding and challenges. Diabetes Care. (2021) 44:2449–56. doi: 10.2337/dc21-0770
3. Atkinson MA, Eisenbarth GS. Type 1 diabetes: new perspectives on disease pathogenesis and treatment. Lancet. (2001) 358:221–9. doi: 10.1016/S0140-6736(01)05415-0
4. Gale EA. Type 1 diabetes in the young: the harvest of sorrow goes on. Diabetologia. (2005) 48:1435–8. doi: 10.1007/s00125-005-1833-0
5. Majaliwa ES, Elusiyan BE, Adesiyun OO, Laigong P, Adeniran AK, Kandi CM, et al. Type 1 diabetes mellitus in the African population: epidemiology and management challenges. Acta Biomed. (2008) 79:255–9.
6. Padoa CJ. The epidemiology and pathogenesis of type 1 diabetes mellitus in Africa. J Clin Endocrinol Metab. (2011) 16:130–6. doi: 10.1080/22201009.2011.10872264
7. International Diabetes Federation. IDF Diabetes Atlas, 10th edn. Brussels, Belgium. (2021). Available online at: https://www.diabetesatlas.org (accessed 20th October, 2022).
8. Mapa-Tassou C, Katte JC, Mba Maadjhou C, Mbanya JC. Economic impact of diabetes in Africa. Curr Diab Rep. (2019) 19:5. doi: 10.1007/s11892-019-1124-7
9. Gill GV, Mbanya JC, Ramaiya KL, Tesfaye S. A sub-saharan African perspective of diabetes. Diabetologia. (2009) 52:8–16. doi: 10.1007/s00125-008-1167-9
10. Osei K, Schuster DP, Amoah AG, Owusu SK. Diabetes in Africa. Pathogenesis of type 1 and type 2 diabetes mellitus in sub-Saharan Africa: implications for transitional populations. J Cardiovasc Risk. (2003) 10:85–96. doi: 10.1177/174182670301000203
11. Atun R, Davies JI, Gale EAM, Barnighausen T, Beran D, Kengne AP, et al. Diabetes in sub-Saharan Africa: from clinical care to health policy. Lancet Diabetes Endocrinol. (2017) 5:622–67. doi: 10.1016/S2213-8587(17)30181-X
12. Pastakia SD, Pekny CR, Manyara SM, Fischer L. Diabetes in sub-Saharan Africa - from policy to practice to progress: targeting the existing gaps for future care for diabetes. Diabetes Metab Syndr Obes. (2017) 10:247–63. doi: 10.2147/DMSO.S126314
13. Gill G. Diabetes in Africa - puzzles and challenges. Indian J Endocrinol Metab. (2014) 18:249–51. doi: 10.4103/2230-8210.131111
14. Bahendeka SK. Diabetes in sub-Saharan Africa: let us not forget type 1. Lancet Diabetes Endocrinol. (2017) 5:575–7. doi: 10.1016/S2213-8587(17)30223-1
15. Beran D, Lazo-Porras M, Mba CM, Mbanya JC. A global perspective on the issue of access to insulin. Diabetologia. (2021) 64:954–62. doi: 10.1007/s00125-020-05375-2
16. Makame MH. Childhood diabetes, insulin, and Africa. DERI (Diabetes Epidemiology Research International) Study Group. Diabet Med. (1992) 9:571–3. doi: 10.1111/j.1464-5491.1992.tb01841.x
17. Sobngwi E, Mauvais-Jarvis F, Vexiau P, Mbanya JC, Gautier JF. Diabetes in Africans. Part 2: Ketosis-prone atypical diabetes mellitus. Diabetes Metab. (2002) 28:5–12.
18. Mbanya JC, Motala AA, Sobngwi E, Assah FK, Enoru ST. Diabetes in sub-Saharan Africa. Lancet. (2010) 375:2254–66. doi: 10.1016/S0140-6736(10)60550-8
19. Shields BM, Peters JL, Cooper C, Lowe J, Knight BA, Powell RJ, et al. Can clinical features be used to differentiate type 1 from type 2 diabetes? A systematic review of the literature. BMJ Open. (2015) 5:e009088. doi: 10.1136/bmjopen-2015-009088
20. Foteinopoulou E, Clarke CAL, Pattenden RJ, Ritchie SA, McMurray EM, Reynolds RM, et al. Impact of routine clinic measurement of serum C-peptide in people with a clinician-diagnosis of type 1 diabetes. Diabet Med. (2021) 38:e14449. doi: 10.1111/dme.14449
21. Hope SV, Wienand-Barnett S, Shepherd M, King SM, Fox C, Khunti K, et al. Practical classification guidelines for diabetes in patients treated with insulin: a cross-sectional study of the accuracy of diabetes diagnosis. Br J Gen Pract. (2016) 66:e315–22. doi: 10.3399/bjgp16X684961
22. Yajnik CS, Yudkin JS. The Y-Y paradox. Lancet. (2004) 363:163. doi: 10.1016/S0140-6736(03)15269-5
23. Staimez LR, Deepa M, Ali MK, Mohan V, Hanson RL, Narayan KMV. Tale of two Indians: heterogeneity in type 2 diabetes pathophysiology. Diabetes Metab Res Rev. (2019) 35:e3192. doi: 10.1002/dmrr.3192
24. Pomeroy E, Mushrif-Tripathy V, Cole TJ, Wells JCK, Stock JT. Ancient origins of low lean mass among South Asians and implications for modern type 2 diabetes susceptibility. Sci Rep. (2019) 9:10515. doi: 10.1038/s41598-019-46960-9
25. Gujral UP, Pradeepa R, Weber MB, Narayan KM, Mohan V. Type 2 diabetes in South Asians: similarities and differences with white Caucasian and other populations. Ann N Y Acad Sci. (2013) 1281:51–63. doi: 10.1111/j.1749-6632.2012.06838.x
26. Kibirige D, Sekitoleko I, Lumu W, Jones AG, Hattersley AT, Smeeth L, et al. Understanding the pathogenesis of lean non-autoimmune diabetes in an African population with newly diagnosed diabetes. Diabetologia. (2022) 65:675–83. doi: 10.1007/s00125-021-05644-8
27. Abdulkadir J, Mengesha B, Welde Gebriel Z, Keen H, Worku Y, Gebre P, et al. The clinical and hormonal (C-peptide and glucagon) profile and liability to ketoacidosis during nutritional rehabilitation in Ethiopian patients with malnutrition-related diabetes mellitus. Diabetologia. (1990) 33:222–7. doi: 10.1007/BF00404800
28. World Health Organization: Diabetes mellitus. Report of a WHO Study Group. Technical Report Series 727. Geneva: WHO. (1985). p. 727.
29. Balcha SA, Phillips DIW, Trimble ER. Type 1 Diabetes in a resource-poor setting: malnutrition related, malnutrition modified, or just diabetes? Curr Diab Rep. (2018) 18:47. doi: 10.1007/s11892-018-1003-7
30. Kibirige D, Kibudde S, Mutebi E. Fibrocalculous pancreatic diabetes in a young Ugandan patient, a rare form of secondary diabetes. BMC Res Notes. (2012) 5:622. doi: 10.1186/1756-0500-5-622
31. Praveen G, Mohan V. Fibrocalculous pancreatic diabetes—current scenario in developing countries. Int J Diabetes Dev Ctries. (2018) 38:131–2. doi: 10.1007/s13410-018-0638-x
32. Lontchi-Yimagou E, Dasgupta R, Anoop S, Kehlenbrink S, Koppaka S, Goyal A, et al. An atypical form of diabetes among individuals with low BMI. Diabetes Care. (2022) 45:1428–37. doi: 10.2337/dc21-1957
33. Unnikrishnan R, Anjana RM, Mukhopadhyay S, Kesavadev J, Mithal A, Joshi S, et al. Comment on Lontchi-Yimagou et al. an atypical form of diabetes among individuals with low BMI. Diabetes Care. (2022) 45:e157–e8. doi: 10.2337/dc22-1257
34. Banerji MA. Diabetes in African Americans: unique pathophysiologic features. Curr Diab Rep. (2004) 4:219–23. doi: 10.1007/s11892-004-0027-3
35. Kitabchi AE. Ketosis-prone diabetes–a new subgroup of patients with atypical type 1 and type 2 diabetes? J Clin Endocrinol Metab. (2003) 88:5087–9. doi: 10.1210/jc.2003-031656
36. Balti EV, Ngo-Nemb MC, Lontchi-Yimagou E, Atogho-Tiedeu B, Effoe VS, Akwo EA, et al. Association of HLA class II markers with autoantibody-negative ketosis-prone atypical diabetes compared to type 2 diabetes in a population of sub-Saharan African patients. Diabetes Res Clin Pract. (2015) 107:31–6. doi: 10.1016/j.diabres.2014.10.002
37. Mauvais-Jarvis F, Sobngwi E, Porcher R, Riveline JP, Kevorkian JP, Vaisse C, et al. Ketosis-prone type 2 diabetes in patients of sub-Saharan African origin: clinical pathophysiology and natural history of beta-cell dysfunction and insulin resistance. Diabetes. (2004) 53:645–53. doi: 10.2337/diabetes.53.3.645
38. Lebovitz HE, Banerji MA. Ketosis-prone diabetes (flatbush diabetes): an emerging worldwide clinically important entity. Curr Diab Rep. (2018) 18:120. doi: 10.1007/s11892-018-1075-4
39. Choukem SP, Sobngwi E, Fetita LS, Boudou P, De Kerviler E, Boirie Y, et al. Multitissue insulin resistance despite near-normoglycemic remission in Africans with ketosis-prone diabetes. Diabetes Care. (2008) 31:2332–7. doi: 10.2337/dc08-0914
40. Lontchi-Yimagou E, Nguewa JL, Assah F, Noubiap JJ, Boudou P, Djahmeni E, et al. Ketosis-prone atypical diabetes in Cameroonian people with hyperglycaemic crisis: frequency, clinical and metabolic phenotypes. Diabet Med. (2017) 34:426–31. doi: 10.1111/dme.13264
41. Ye S, Ran H, Zhang H, Wu H, Li W, Du S, et al. Elevated serum triglycerides are associated with ketosis-prone type 2 diabetes in young individuals. Diabetes Metab Syndr Obes. (2021) 14:497–504. doi: 10.2147/DMSO.S296085
42. Gill GV, Tekle A, Reja A, Wile D, English PJ, Diver M, et al. Immunological and C-peptide studies of patients with diabetes in northern Ethiopia: existence of an unusual subgroup possibly related to malnutrition. Diabetologia. (2011) 54:51–7. doi: 10.1007/s00125-010-1921-7
43. Kalk WJ, Huddle KR, Raal FJ. The age of onset and sex distribution of insulin-dependent diabetes mellitus in Africans in South Africa. Postgrad Med J. (1993) 69:552–6. doi: 10.1136/pgmj.69.813.552
44. McLarty DG, Athaide I, Bottazzo GF, Swai AM, Alberti KG. Islet cell antibodies are not specifically associated with insulin-dependent diabetes in Tanzanian Africans. Diabetes Res Clin Pract. (1990) 9:219–24. doi: 10.1016/0168-8227(90)90048-X
45. Panz VR, Kalk WJ, Zouvanis M, Joffe BI. Distribution of autoantibodies to glutamic acid decarboxylase across the spectrum of diabetes mellitus seen in South Africa. Diabet Med. (2000) 17:524–7. doi: 10.1046/j.1464-5491.2000.00324.x
46. Majaliwa ES, Mohn A, Chiavaroli V, Ramaiya K, Swai AB, Chiarelli F. Management of diabetic ketoacidosis in children and adolescents in sub-Saharan Africa: a review. East Afr Med J. (2010) 87:167–73. doi: 10.4314/eamj.v87i4.62207
47. Ameyaw E, Asafo-Agyei SB, Thavapalan S, Middlehurst AC, Ogle GD. Clinical profile of diabetes at diagnosis among children and adolescents at an endocrine clinic in Ghana. World J Diabetes. (2017) 8:429–35. doi: 10.4239/wjd.v8.i9.429
48. Beran D, Ewen M, Laing R. Constraints and challenges in access to insulin: a global perspective. Lancet Diabetes Endocrinol. (2016) 4:275–85. doi: 10.1016/S2213-8587(15)00521-5
49. Mbanya JC, Mba CM. Centenary of the discovery of insulin: People with diabetes in Africa still have poor access to insulin. EClinicalMedicine. (2021) 34:100809. doi: 10.1016/j.eclinm.2021.100809
50. Atkinson MA, Ogle GD. Improving diabetes care in resource-poor countries: challenges and opportunities. Lancet Diabetes Endocrinol. (2013) 1:268–70. doi: 10.1016/S2213-8587(13)70172-4
51. Bahendeka S, Mutungi G, Tugumisirize F, Kamugisha A, Nyangabyaki C, Wesonga R, et al. Healthcare delivery for paediatric and adolescent diabetes in low resource settings: Type 1 diabetes clinics in Uganda. Glob Public Health. (2019) 14:1869–83. doi: 10.1080/17441692.2019.1611897
52. Katte JC, Djoumessi R, Njindam G, Fetse GT, Dehayem M, Kengne AP. New-onset diabetic ketoacidosis in a 13-months old african toddler: a case report. Pan Afr Med J. (2015) 22:293. doi: 10.11604/pamj.2015.22.293.7581
53. Sandy JL, Besancon S, Sidibe AT, Minkailou M, Togo A, Ogle GD. Rapid increases in observed incidence and prevalence of Type 1 diabetes in children and youth in Mali, 2007–2016. Pediatr Diabetes. (2021) 22:545–51. doi: 10.1111/pedi.13191
54. Majaliwa ES, Minja L, Ndayongeje J, Ramaiya K, Mfinanga SG, Mmbaga BT. Survival of children and youth with type 1 diabetes mellitus in Tanzania. Pediatr Diabetes. (2022) 23:1560–6. doi: 10.1111/pedi.13425
55. Jasem D, Majaliwa ES, Ramaiya K, Najem S, Swai ABM, Ludvigsson J. Incidence, prevalence and clinical manifestations at onset of juvenile diabetes in Tanzania. Diabetes Res Clin Pract. (2019) 156:107817. doi: 10.1016/j.diabres.2019.107817
56. Lutale JJ, Thordarson H, Holm PI Eide GE, Vetvik K. Islet cell autoantibodies in African patients with type 1 and Type 2 diabetes in Dar es Salaam Tanzania: a cross sectional study. J Autoimmune Dis. (2007) 4:4. doi: 10.1186/1740-2557-4-4
57. Elamin A, Omer MI, Tuvemo T. Islet-cell antibodies and endogenous insulin secretion in Sudanese diabetic children. Diabetes Res Clin Pract. (1992) 16:91–6. doi: 10.1016/0168-8227(92)90078-6
58. Onyiriuka AN, Ifebi E. Ketoacidosis at diagnosis of type 1 diabetes in children and adolescents: frequency and clinical characteristics. J Diabetes Metab Disord. (2013) 12:47. doi: 10.1186/2251-6581-12-47
59. Asanghanwa M, Gorus FK, Weets I, der Auwera BV, Aminkeng F, Mbunwe E, et al. Clinical and biological characteristics of diabetic patients under age 40 in Cameroon: relation to autoantibody status and comparison with Belgian patients. Diabetes Res Clin Pract. (2014) 103:97–105. doi: 10.1016/j.diabres.2013.11.013
60. Bahendeka S, Wesonga R, Were TP, Nyangabyaki C. Autoantibodies and HLA class II DR-DQ genotypes in Ugandan children and adolescents with type 1 diabetes mellitus. Int J Diabetes Dev C. (2019) 39:39–46. doi: 10.1007/s13410-018-0622-5
61. Balcha SA, Demisse AG, Mishra R, Vartak T, Cousminer DL, Hodge KM, et al. Type 1 diabetes in Africa: an immunogenetic study in the Amhara of North-West Ethiopia. Diabetologia. (2020) 63:2158–68. doi: 10.1007/s00125-020-05229-x
62. Ibrahim TAM, Govender D, Abdullah MA, Noble JA, Hussien MO, Lane JA, et al. Clinical features, biochemistry, and HLA-DRB1 status in youth-onset type 1 diabetes in Sudan. Pediatr Diabetes. (2021) 22:749–57. doi: 10.1111/pedi.13209
63. Besancon S, Govender D, Sidibe AT, Noble JA, Togo A, Lane JA, et al. Clinical features, biochemistry, and HLA-DRB1 status in youth-onset type 1 diabetes in Mali. Pediatr Diabetes. (2022).
64. Siraj ES, Gupta MK, Yifter H, Ahmed A, Kebede T, Reja A, et al. Islet cell-associated autoantibodies in Ethiopians with diabetes mellitus. J Diabetes Complications. (2016) 30:1039–42. doi: 10.1016/j.jdiacomp.2016.05.005
65. Ducorps M, Ndong W, Jupkwo B, Belmejdoub G, Poirier JM, Mayaudon H, et al. Epidemiological aspects of diabetes in Cameroon: what is the role of tropical diabetes? Diabetes Metab. (1997) 23:61–7.
66. Padoa CJ, Rheeder P, Pirie FJ, Motala AA, van Dyk JC, Crowther NJ. Identification of a subgroup of black South Africans with type 1 diabetes who are older at diagnosis but have lower levels of glutamic acid decarboxylase and islet antigen 2 autoantibodies. Diabet Med. (2020) 37:2067–74. doi: 10.1111/dme.14204
67. Harding JL, Wander PL, Zhang X, Li X, Karuranga S, Chen H, et al. The Incidence of adult-onset type 1 diabetes: a systematic review from 32 countries and regions. Diabetes Care. (2022) 45:994–1006. doi: 10.2337/dc21-1752
68. Alemu S, Dessie A, Seid E, Bard E, Lee PT, Trimble ER, et al. Insulin-requiring diabetes in rural Ethiopia: should we reopen the case for malnutrition-related diabetes? Diabetologia. (2009) 52:1842–5. doi: 10.1007/s00125-009-1433-5
69. Otieno CF, Kayima JK, Omonge EO, Oyoo GO. Diabetic ketoacidosis: risk factors, mechanisms and management strategies in sub-Saharan Africa: a review. East Afr Med J. (2005) 82:S197–203. doi: 10.4314/eamj.v82i12.9382
70. Battaglia M, Ahmed S, Anderson MS, Atkinson MA, Becker D, Bingley PJ, et al. Introducing the endotype concept to address the challenge of disease heterogeneity in type 1 diabetes. Diabetes Care. (2020) 43:5–12. doi: 10.2337/dc19-0880
71. Parviainen A, Härkönen T, Ilonen J, But A, Knip M. Register tFPD. heterogeneity of type 1 diabetes at diagnosis supports existence of age-related endotypes. Diabetes Care. (2022) 45:871–9. doi: 10.2337/dc21-1251
72. Leete P, Willcox A, Krogvold L, Dahl-Jørgensen K, Foulis AK, Richardson SJ, et al. Differential insulitic profiles determine the extent of β-cell destruction and the age at onset of type 1 diabetes. Diabetes. (2016) 65:1362–9. doi: 10.2337/db15-1615
73. Leete P, Oram RA, McDonald TJ, Shields BM, Ziller C, et al. Studies of insulin and proinsulin in pancreas and serum support the existence of aetiopathological endotypes of type 1 diabetes associated with age at diagnosis. Diabetologia. (2020) 63:1258–67. doi: 10.1007/s00125-020-05115-6
74. Eason RJ, Thomas NJ, Hill AV, Knight BA, Carr A, Hattersley AT, et al. Routine islet autoantibody testing in clinically diagnosed adult-onset type 1 diabetes can help identify misclassification and the possibility of successful insulin cessation. Diabetes Care. (2022). doi: 10.2337/figshare.21120535
75. Thomas NJ, Walkey HC, Kaur A, Misra S, Oliver NS, Colclough K, et al. The relationship between islet autoantibody status and the genetic risk of type 1 diabetes in adult-onset type 1 diabetes. Diabetologia. (2023) 66:310–20. doi: 10.1007/s00125-022-05823-1
76. Marshall SL, Edidin D, Sharma V, Ogle G, Arena VC, Orchard T. Current clinical status, glucose control, and complication rates of children and youth with type 1 diabetes in Rwanda. Pediatr Diabetes. (2013) 14:217–26. doi: 10.1111/pedi.12007
77. Chilunga FP, Henneman P, Meeks KA, Beune E, Requena-Mendez A, Smeeth L, et al. Prevalence and determinants of type 2 diabetes among lean African migrants and non-migrants: the RODAM study. J Glob Health. (2019) 9:020426. doi: 10.7189/jogh.09.020426
78. Ziegler AG, Kick K, Bonifacio E, Haupt F, Hippich M, Dunstheimer D, et al. Yield of a public health screening of children for islet autoantibodies in Bavaria, Germany. JAMA. (2020) 323:339–51. doi: 10.1001/jama.2019.21565
79. Todd JA, Farrall M. Panning for gold: genome-wide scanning for linkage in type 1 diabetes. Hum Mol Genet. (1996) 5:1443–8. doi: 10.1093/hmg/5.Supplement_1.1443
80. Atkilt HS, Turago MG, Tegegne BS. Clinical characteristics of diabetic ketoacidosis in children with newly diagnosed type 1 diabetes in addis ababa, ethiopia: a cross-sectional study. PLoS ONE. (2017) 12:e0169666. doi: 10.1371/journal.pone.0169666
81. Ahmed AM, Khabour OF, Ahmed SM, Alebaid IA, Ibrahim AM. Frequency and severity of ketoacidosis at diagnosis among childhood type 1 diabetes in Khartoum state, Sudan. Afr Health Sci. (2020) 20:841–8. doi: 10.4314/ahs.v20i2.38
82. Ndebele NFM, Naidoo M. The management of diabetic ketoacidosis at a rural regional hospital in KwaZulu-Natal. Afr J Prim Health Care Fam Med. (2018) 10:e1–6. doi: 10.4102/phcfm.v10i1.1612
83. Murunga AN, Owira PM. Diabetic ketoacidosis: an overlooked child killer in sub-Saharan Africa? Trop Med Int Health. (2013) 18:1357–64. doi: 10.1111/tmi.12195
84. Grosse J, Hornstein H, Manuwald U, Kugler J, Glauche I, Rothe U. Incidence of diabetic ketoacidosis of new-onset type 1 diabetes in children and adolescents in different countries correlates with human development index (hdi): an updated systematic review, meta-analysis, and meta-regression. Horm Metab Res. (2018) 50:209–22. doi: 10.1055/s-0044-102090
85. Bingley PJ. Clinical applications of diabetes antibody testing. J Clin Endocrinol Metab. (2010) 95:25–33. doi: 10.1210/jc.2009-1365
86. Regnell SE, Lernmark A. Early prediction of autoimmune (type 1) diabetes. Diabetologia. (2017) 60:1370–81. doi: 10.1007/s00125-017-4308-1
87. Pociot F, Lernmark A. Genetic risk factors for type 1 diabetes. Lancet. (2016) 387:2331–9. doi: 10.1016/S0140-6736(16)30582-7
88. DiMeglio LA, Evans-Molina C, Oram RA. Type 1 diabetes. Lancet. (2018) 391:2449–62. doi: 10.1016/S0140-6736(18)31320-5
89. Magzoub MM, Abdel-Hameed AA, Bottazzo GF. Prevalence of islet cell and thyrogastric autoantibodies in Sudanese patients with type 1 diabetes. Diabet Med. (1994) 11:188–92. doi: 10.1111/j.1464-5491.1994.tb02018.x
90. Rheeder P, Stolk RP, Grobbee DE. Ethnic differences in C-peptide levels and anti-GAD antibodies in South African patients with diabetic ketoacidosis. QJM. (2001) 94:39–43. doi: 10.1093/qjmed/94.1.39
91. Hawa MI, Picardi A, Costanza F, D'Avola D, Beretta Anguissola G, Guglielmi C, et al. Frequency of diabetes and thyroid autoantibodies in patients with autoimmune endocrine disease from Cameroon. Clin Immunol. (2006) 118:229–32. doi: 10.1016/j.clim.2005.09.016
92. Paruk IM, Ganie Y, Maharaj S, Pirie FJ, Naidoo VG, Nkwanyana NM, et al. High prevalence of antithyroid peroxidase and antiparietal cell antibodies among patients with type 1 diabetes mellitus attending a tertiary diabetes centre in South Africa. Postgrad Med J. (2017) 93:338–43. doi: 10.1136/postgradmedj-2016-134420
93. Bhola S, Cave EM, Bhana S, Crowther NJ, Padoa CJ. Zinc transporter 8 (ZnT8) autoantibody prevalence in black South African participants with type 1 diabetes. BMC Endocr Disord. (2021) 21:151. doi: 10.1186/s12902-021-00812-8
94. Patterson CC, Karuranga S, Salpea P, Saeedi P, Dahlquist G, Soltesz G, et al. Worldwide estimates of incidence, prevalence and mortality of type 1 diabetes in children and adolescents: Results from the International Diabetes Federation Diabetes Atlas, 9th edition. Diabetes Res Clin Pract. (2019) 157:107842. doi: 10.1016/j.diabres.2019.107842
95. Mebrahtu G, Maniam J, James S, Ogle GD. High incidence of type 1 diabetes in adolescents and young adults in Eritrea. Diabet Med. (2021) 38:e14544. doi: 10.1111/dme.14544
96. Noble JA, Valdes AM. Genetics of the HLA region in the prediction of type 1 diabetes. Curr Diab Rep. (2011) 11:533–42. doi: 10.1007/s11892-011-0223-x
97. Genovese S, Bonfanti R, Bazzigaluppi E, Lampasona V, Benazzi E, Bosi E, et al. Association of IA-2 autoantibodies with HLA DR4 phenotypes in IDDM. Diabetologia. (1996) 39:1223–6. doi: 10.1007/BF02658510
98. Teisserenc H, Briaud I, Busson M, Albert E, Bignon JD. TAP, LMP and HLA-DM polymorphism: 12th international histocompatibility workshop study. In: Genetic Diversity of HLA-Functional and Medical Implications. Paris: Medical and Scientific International Publisher (1997).
99. Borch-Johnsen K, Joner G, Mandrup-Poulsen T, Christy M, Zachau-Christiansen B, Kastrup K, et al. Relation between breast-feeding and incidence rates of insulin-dependent diabetes mellitus. A hypothesis Lancet. (1984) 2:1083–6. doi: 10.1016/S0140-6736(84)91517-4
100. Ziegler AG, Schmid S, Huber D, Hummel M, Bonifacio E. Early infant feeding and risk of developing type 1 diabetes-associated autoantibodies. JAMA. (2003) 290:1721–8. doi: 10.1001/jama.290.13.1721
101. Bach JF, Chatenoud L. The hygiene hypothesis: an explanation for the increased frequency of insulin-dependent diabetes. Cold Spring Harb Perspect Med. (2012) 2:a007799. doi: 10.1101/cshperspect.a007799
102. Erismann S, Diagbouga S, Odermatt P, Knoblauch AM, Gerold J, Shrestha A, et al. Prevalence of intestinal parasitic infections and associated risk factors among schoolchildren in the Plateau Central and Centre-Ouest regions of Burkina Faso. Parasit Vectors. (2016) 9:554. doi: 10.1186/s13071-016-1835-4
103. Speich B, Marti H, Ame SM, Ali SM, Bogoch II, Utzinger J, et al. Prevalence of intestinal protozoa infection among school-aged children on Pemba Island, Tanzania, and effect of single-dose albendazole, nitazoxanide and albendazole-nitazoxanide. Parasit Vectors. (2013) 6:3. doi: 10.1186/1756-3305-6-3
104. Maizels RM, Smits HH, McSorley HJ. Modulation of host immunity by helminths: the expanding repertoire of parasite effector molecules. Immunity. (2018) 49:801–18. doi: 10.1016/j.immuni.2018.10.016
105. Jahromi M, Al-Ozairi E. Human leukocyte antigen (HLA) and islet autoantibodies are tools to characterize type 1 diabetes in arab countries: emphasis on kuwait. Dis Markers. (2019) 2019:9786078. doi: 10.1155/2019/9786078
106. Jones AG, McDonald TJ, Shields BM, Hagopian W, Hattersley AT. Latent autoimmune diabetes of adults (LADA) is likely to represent a mixed population of autoimmune (type 1) and nonautoimmune (type 2) diabetes. Diabetes Care. (2021). doi: 10.2337/dc20-2834
107. American Diabetes A. 2. Classification and diagnosis of diabetes: standards of medical care in diabetes-2021. Diabetes Care. (2021) 44:S15–S33. doi: 10.2337/dc21-S002
108. Jones AG, Hattersley AT. The clinical utility of C-peptide measurement in the care of patients with diabetes. Diabet Med. (2013) 30:803–17. doi: 10.1111/dme.12159
109. Siraj ES, Reddy SS, Scherbaum WA, Abdulkadir J, Hammel JP, Faiman C. Basal and postglucagon C-peptide levels in Ethiopians with diabetes. Diabetes Care. (2002) 25:453–7. doi: 10.2337/diacare.25.3.453
110. Habtu E, Gill G, Tesfaye S. Characteristics of insulin requiring diabetes in rural northern Ethiopia–a possible link with malnutrition? Ethiop Med J. (1999) 37:263–7.
111. Govender P, Elmezughi K, Esterhuizen T, Paruk I, Pirie FJ, Motala AA. Characteristics of subjects with diabetes mellitus diagnosed before 35 years of age presenting to a tertiary diabetes clinic in Durban, South Africa, from 2003 to 2016. J Clin Endocrinol Metab. (2018) 23:26–31. doi: 10.1080/16089677.2017.1417779
112. Noble JA, Erlich HA. Genetics of type 1 diabetes. Cold Spring Harb Perspect Med. (2012) 2:a007732. doi: 10.1101/cshperspect.a007732
113. Bakay M, Pandey R, Grant SFA, Hakonarson H. The genetic contribution to type 1 diabetes. Curr Diab Rep. (2019) 19:116. doi: 10.1007/s11892-019-1235-1
114. MacDonald MJ, Famuyiwa OO, Nwabuebo IA, Bella AF, Junaid TA, Marrari M, et al. HLA-DR associations in black type I diabetics in Nigeria. Further support for models of inheritance. Diabetes. (1986) 35:583–9. doi: 10.2337/diab.35.5.583
115. Magzoub MM, Stephens HA, Gale EA, Bottazzo GF. Analysis of HLA-DR and -DQ gene polymorphisms in Sudanese patients with type 1 (insulin-dependent) diabetes. Immunogenetics. (1991) 34:366–71. doi: 10.1007/BF01787486
116. Garcia-Pacheco JM, Herbut B, Cutbush S, Hitman GA, Zhonglin W, Magzoub M, et al. Distribution of HLA-DQA1, -DQB1 and DRB1 alleles in black IDDM patients and controls from Zimbabwe. Tissue Antigens. (1992) 40:145–9. doi: 10.1111/j.1399-0039.1992.tb02107.x
117. Chauffert M, Cisse A, Chevenne D, Parfait B, Michel S, Trivin F, et al. beta 1 typing and non-Asp57 alleles in the aborigine population of Senegal. Diabetes Care. (1995) 18:677–80. doi: 10.2337/diacare.18.5.677
118. Cisse A, Chauffert M, Chevenne D, Parfait B, Julier C, Assouline Z, et al. Distribution of HLA-DQA1 and -DQB1 alleles and DQA1-DQB1 genotypes among Senegalese patients with insulin-dependent diabetes mellitus. Tissue Antigens. (1996) 47:333–7. doi: 10.1111/j.1399-0039.1996.tb02562.x
119. Mbanya JC, Sobngwi E, Mbanya DN. HLA-DRB1, -DQA1, -DQB1 and DPB1 susceptibility alleles in Cameroonian type 1 diabetes patients and controls. Eur J Immunogenet. (2001) 28:459–62. doi: 10.1046/j.0960-7420.2001.00247.x
120. Fagbemi KA, Medehouenou TCM, Azonbakin S, Adjagba M, Osseni R, Ahoueya J, et al. HLA Class II allele, haplotype, and genotype associations with type 1 diabetes in benin: a pilot study. J Diabetes Res. (2017) 2017:6053764. doi: 10.1155/2017/6053764
121. Zhu M, Xu K, Chen Y, Gu Y, Zhang M, Luo F, et al. Identification of novel T1D risk loci and their association with age and islet function at diagnosis in autoantibody-positive t1d individuals: based on a two-stage genome-wide association study. Diabetes Care. (2019) 42:1414–21. doi: 10.2337/dc18-2023
122. Yan C, Wang R, Li J, Deng Y, Wu D, Zhang H, et al. HLA-A gene polymorphism defined by high-resolution sequence-based typing in 161 Northern Chinese Han people. Genomics Prot Bioinformat. (2003) 1:304–9. doi: 10.1016/S1672-0229(03)01036-2
123. Harrison JW, Tallapragada DSP, Baptist A, Sharp SA, Bhaskar S, Jog KS, et al. Type 1 diabetes genetic risk score is discriminative of diabetes in non-Europeans: evidence from a study in India. Sci Rep. (2020) 10:9450. doi: 10.1038/s41598-020-65317-1
124. Noble JA, Johnson J, Lane JA, Valdes AM, HLA. class II genotyping of African American type 1 diabetic patients reveals associations unique to African haplotypes. Diabetes. (2013) 62:3292–9. doi: 10.2337/db13-0094
125. Oram RA, Patel K, Hill A, Shields B, McDonald TJ, Jones A, et al. A type 1 diabetes genetic risk score can aid discrimination between type 1 and type 2 diabetes in young adults. Diabetes Care. (2016) 39:337–44. doi: 10.2337/dc15-1111
Keywords: phenotype [MeSH], characteristics, type 1 diabetes, islet autoimmunity, beta-cell, C-peptide, genetics, sub-Saharan Africa
Citation: Katte JC, McDonald TJ, Sobngwi E and Jones AG (2023) The phenotype of type 1 diabetes in sub-Saharan Africa. Front. Public Health 11:1014626. doi: 10.3389/fpubh.2023.1014626
Received: 08 August 2022; Accepted: 10 January 2023;
Published: 27 January 2023.
Edited by:
Vikash Chandra, University of Helsinki, FinlandReviewed by:
Zekai Wu, McGill University, CanadaRichard David Leslie, Queen Mary University of London, United Kingdom
Copyright © 2023 Katte, McDonald, Sobngwi and Jones. This is an open-access article distributed under the terms of the Creative Commons Attribution License (CC BY). The use, distribution or reproduction in other forums is permitted, provided the original author(s) and the copyright owner(s) are credited and that the original publication in this journal is cited, in accordance with accepted academic practice. No use, distribution or reproduction is permitted which does not comply with these terms.
*Correspondence: Jean Claude Katte, amNrYXR0ZUBnbWFpbC5jb20=