- 1School of Architecture and Urban Planning, Chongqing University, Chongqing, China
- 2Key Laboratory of New Technology for Construction of Cities in Mountain Area, Chongqing University, Chongqing, China
Background: Empirical evidence has shown that light therapy (LT) can reduce depression symptoms by stimulating circadian rhythms. However, there is skepticism and inconclusive results, along with confusion regarding dosing. The purpose of this study is to quantify light as a stimulus for the circadian system and create a dose-response relationship that can help reduce maladies among adolescents and young adults (AYAs). This will provide a reference for light exposure and neural response, which are crucial in the neuropsychological mechanism of light intervention. The study also aims to provide guidance for clinical application.
Methods: The latest quantitative model of CLA (circadian light) and CSt,f (circadian stimulus) was adopted to quantify light dose for circadian phototransduction in youth depression-related light therapy. Articles published up to 2023 through Web of Science, Cochrane Library, Medline (OVID), CINAHL, APA PsycINFO, Embase, and Scholars were retrieved. A meta-analysis of 31 articles (1,031 subjects) was performed using Stata17.0, CMA3.0 (comprehensive meta-analysis version 3.0) software, and Python 3.9 platform for light therapy efficacy comparison and dose-response quantification.
Results: Under various circadian stimulus conditions (0.1 < CSt,f < 0.7) of light therapy (LT), malady reductions among AYAs were observed (pooled SMD = −1.59, 95%CI = −1.86 to −1.32; z = −11.654, p = 0.000; I2 = 92.8%), with temporal pattern (p = 0.044) and co-medication (p = 0.000) suggested as main heterogeneity sources. For the efficacy advantage of LT with a higher circadian stimulus that is assumed to be influenced by visualization, co-medication, disease severity, and time pattern, sets of meta-analysis among random-controlled trials (RCTs) found evidence for significant efficacy of circadian-active bright light therapy (BLT) over circadian-inactive dim red light (SMD = −0.65, 95% CI = −0.96 to −0.34; z = −4.101, p = 0.000; I2 = 84.9%) or circadian-active dimmer white light (SMD = −0.37, 95% CI = −0.68 to −0.06; z = −2.318, p = 0.02; I2 = 33.8%), whereas green-blue, circadian-active BLT showed no significant superiority over circadian-inactive red/amber light controls (SMD = −0.21, 95% CI = −0.45 to 0.04; z = −2.318, p = 0.099; I2 = 0%). Overall, circadian-active BLT showed a greater likelihood of clinical response than dim light controls, with increased superiority observed with co-medication. For pre-to-post-treatment amelioration and corresponding dose-response relationship, cumulative duration was found more influential than other categorical (co-medication, severity, study design) or continuous (CSt,f) variables. Dose-response fitting indicated that the therapeutic effect would reach saturation among co-medicated patients at 32–42 days (900–1,000 min) and 58–59 days (1,100–1,500 min) among non-medicated AYAs. When exerting high circadian stimulus of light therapy (0.6 < CSt,f < 0.7), there was a significantly greater effect size in 1,000–1,500 min of accumulative duration than <1,000 or >1,500 min of duration, indicating a threshold for practical guidance.
Limitations: The results have been based on limited samples and influenced by a small sample effect. The placebo effect could not be ignored.
Conclusions: Although the superiority of LT with higher circadian stimulus over dimmer light controls remains unproven, greater response potentials of circadian-active BLT have been noticed among AYAs, taking co-medication, disease severity, time pattern, and visual characteristics into consideration. The dose-response relationship with quantified circadian stimulus and temporal pattern had been elaborated under various conditions to support clinical depression treatment and LT device application in the post-pandemic era.
1 Introduction
The circadian stimulus of light therapy (1), also known as bright light therapy (BLT), has been found to have positive clinical outcomes in reducing symptoms of depression. Since the dysfunction of the circadian system has been strongly linked to psychological disorders, light therapy has been particularly successful in treating such conditions (2). However, skeptics contend that light therapy's efficacy may be little better than a placebo under great varieties of light administration protocols (e.g., white light or monochromatic light, various intensities) (3), as well as malady conditions. The skepticism surrounding the efficacy of light therapy for treating a wide range of maladies is rooted in the uncertainty of light dosing (2), whether in monotherapy studies or combination cases (4). Therefore, quantifying the amount of light can help establish a reliable and predictable relationship between light therapy and reductions in relevant disorders. This study aims to verify the efficacy of light therapy by quantifying the circadian stimulus and duration time as vital parameters of light dose for the circadian system among adolescents and young adults (AYAs).
1.1 Youth depression and circadian dysrhythmias
There is substantial evidence that links circadian misalignment with depression among young people (5, 6), e.g., major depressive disorder (7), bipolar disorder (8), unipolar depressive disorders (9), delayed sleep phase (DSP), attention deficit hyperactive disorder (ADHD) (10), and “circadian” depression clinical phenotype (11). Besides classical diagnostic depressive subtypes, other primary (e.g., post-natal, peri-menopausal, late-onset) and secondary (e.g., post-infective, comorbid pain syndromes) depressive subtypes may also be linked with underlying circadian dysfunction (12, 13). Circadian responses to bright light therapy are mainly based on the circadian phototransduction mechanism, which is considered beneficial for the treatment of SAD (seasonal affective disorder) (14, 15), NSD (non-seasonal affective disorder) (16), BD (bipolar disorder) (17), MDD (major depressive disorder) (18), ADHD (attention deficit hyperactivity disorder) (19), Parkinson (20), Alzheimer's disease (21), antepartum depression (22), and CRSWDs (circadian rhythm sleep-wake disorders) (23)-related circadian rhythm disturbances, depression, and sleeping problems. Furthermore, there is empirical evidence that depressed youth have been prescribed not only light therapy but also chronotherapy (which is a combination of light therapy and sleep deprivation—wake therapy) for greater circadian adjustment and anti-depressive effect stabilization in seasonal affective disorders and non-seasonal unipolar and bipolar depression (24). Though overall treatment effectiveness may be inconclusive (25), the dose effect and dose response of light therapy have been hypothesized and explored in numerous protocols, e.g., dose equivalence assumption on a duration × light intensity basis (4, 26). Additionally, the temporal pattern has also been found to be related to dose-dependent efficacy and has been studied (27); for instance, depression severity has been found (22) or presumed (28) altering along with varied duration or total treatment period (29–31). Overall, the lack of an accepted standard definition of adequate dosing for experimental light treatment, along with controversial presumptions and results, has made it challenging to assess the effectiveness of such treatment.
1.2 Circadian phototransduction and current quantifying model
Human circadian phototransduction, which is closely related to the non-visual effects of light, should be distinguished from visual effects. Multiple neural channels emanate from the retina, each with different spectral sensitivities that convert optical radiation into neural signals. Yet, the quantitative photopic luminous efficiency function V(λ) defined by CIE (Commission Internationale de l'Eclairage) and the photometric illuminance (lux) (for quantifying light therapy devices) is not relevant to all of these neural channels (32). Circadian phototransduction is a non-visual effect of light primarily based on photoreceptor-like intrinsically photosensitive retinal ganglion cells (ipRGCs) that send light information to the biological clock in the hypothalamic suprachiasmatic nucleus (SCN), which then synchronizes biological rhythms and projects signals to (including but not limited to) the ventrolateral preoptic (VLPO) nucleus, extended amygdala (33), pineal body, etc. The synthesis of melatonin in the pineal gland and core body temperature rhythmically rise and fall over a 24-h period and are used experimentally as markers of the endogenous rhythm (34); mood oscillates in parallel to core body temperature and is strongly influenced by the circadian process (35). Besides, other chronobiological effects of light are based on SCN-related physiological mechanisms (36). The human retina has five photoreceptors that contribute to the circadian system phototransduction (37). These include rods, cones, and ipRGCs, along with their respective photopigments, retinal opsin proteins such as melanopsin, rhodopsin, S-, M-, and L-cone opsin. Among these photoreceptors, ipRGCs, and melanopsin are mostly involved in the process, and they are more sensitive to “blue” wavelength at around 480 nm (38). S-cone-opic also plays a role in the circadian system phototransduction (39, 40). Therefore, the relative potency of light sources for light therapy would be more influenced by the amount of short-wavelength light mediated by the melanopsin system than the illuminance (lux) at eye level (41). Short-wavelength enriched light with lower intensity may have a stronger effect on the circadian system than visually stronger light.
The circadian light (CLA) model, which has been undergoing retinal neurophysiology and psychophysics experiments (42, 43), can be explained as a spectral weighting function suitable for SCN-oriented circadian responses. The CLA model can quantify a functional relationship between optical radiation incident on the retina and the spectral, temporal, and absolute responses of the SCN. The circadian stimulus (CS) was developed as the operating range of the circadian system from threshold to saturation, taking nocturnal melatonin suppression as the outcome measure (42). These models have photoreceptors (cones, rods, ipRGCs) and retina amacrines and their neuroanatomical and neurophysiological interactions (43) and have shown a response magnitude characteristic of different amounts of spectrally weighted optical radiation of the single circadian phototransduction circuit (42). Moreover, the latest models of circadian stimulus (CS) and circadian light (CLA) have reduced discrepancy in response to “warm” and “cold” light sources, optimized duration, and light exposure distribution; therefore, in this study, they were adopted to quantify circadian phototransduction.
It has also been proven that besides the SCN-dependent circadian phototransduction circuit, separate non-circadian (34) or SCN-independent pathways exist with light effects on mood that may be direct, immediate, and sustained. For instance, the simultaneous inhibition of the sleep-inducing ventrolateral preoptic (VLPO) and the activation of the monoaminergic (44), thalamic, and hypothalamic regions (45) are involved in the control of mood and alertness. Likewise, the orexinergic and monoamine-dependent pathways impact mood. Moreover, there are also emotional processing pathways (light-sensitive circuits) in the cortical system, frontal cortex, and limbic system that ameliorate depressive symptoms (46). For example, the ipRGCs-vLGN/IGL (thalamic region)-LHb (lateral habenula) pathway may be the crucial sluice through which light ameliorates depression-like behaviors (47). However, for this study, we did not adopt indicators besides CS because light dose-related experiments are limited or have merely been verified in animal models (48), and there are huge differences in the efficacious light dose between humans and other species (49).
1.3 Scoping “circadian” treatment for young people
Depressive disorders are among the most prominent health problems among young people. Extensive research efforts have reported unsatisfactory outcomes among AYAs who have undergone prescribed medication side-effects, treatment resistance, breakthrough depressive symptoms, much lower response rates under combined medication and psychotherapy compared with adults (50), or deficient evidence for pharmaxgical treatments on comorbidity of depression (51), or potential recurrence risk (52). However, light therapy has shown potential for AYAs suffering from these issues. Additionally, depression and sleep disorders exhibit high comorbidity among youth (53); all these have urged light as a zeitgeber for synchronization, as well as a non-invasive treatment for depression. Current evidence has shown BLT is likely well-tolerated in adolescents but pointed out that the highly variable selection of light dosing presents a challenge in comparing treatment response and tolerability (54).
It is noteworthy that age exerts influence on light therapy in both visual and non-visual ways. Older people normally receive decreased retinal illumination due to reduced pupil size, increased ocular lens absorption (55), and other substantial changes in visual organs. Correspondingly, young people obtain higher lens transmittance, especially for short-wavelength light that peaks non-visual sensitivity, which is shifted to longer wavelengths in older people (56). CIE has also outlined the sensitivity variation of light-sensitive photoreceptors (38); compared to 32-year-old reference observers, populations aged 22 and 42 expressed weighted, fluctuated sensitivity, which may influence synchronizing input and melatonin suppression (57). Phase and amplitude of circadian functions are also related to age, such as alterations in SCN-related molecular and neuronal factors (58) and output levels [e.g., VLPO and pineal gland (59)]. Therefore, this study focuses on the current circadian aspect of light therapy in the depression treatment of the young population.
In this study, the therapeutic effect of circadian light therapy among AYAs will be quantitatively explored between circadian stimulus (defined by certain spectrum, illuminance, exposure duration time, and lighting distribution factor) and reductions in relevant maladies, using mainly the SMD values of clinical depression measurement scales as outcomes.
2 Materials and methods
2.1 Literature research
This systematic review study was registered with PROSPERO under code number CRD42022375211 and was conducted following Preferred Reporting Items for Systematic Reviews and Meta-Analysis (PRISMA) guidelines (60). Articles published up to 2023 were searched through Web of Science, Embase, CINAHL, Cochrane Library, Medline (OVID), APA PsycINFO databases, as well as websites of the National Library of Medicine, ClinicalTrials.gov and Scholars. Multiple databases were also searched simultaneously using PubMed. Advanced searches with MeSH (medical subject heading) terms were included when available. The search strategy contained the following terms: (light therapy OR light treatment OR bright light therapy OR BLT OR chronotherapy OR phototherapy OR wake and light therapy) AND (youth OR adolescent OR students OR young adults OR teens OR teenagers) AND (depression OR depressive OR mood disorder). The detailed strategies can be checked in Supplementary Table S1. Full text was required but not restricted to English. The preliminary screening results were 90 in CINAHL, 52 in Cochrane Library, 237 in Embase, 125 in Medline (OVID), 127 in APA PsycINFO, and 56 in Web of Science. Additional studies that had not been captured by the original database search were retrieved mainly through Google Scholar. After removing duplication, 599 articles were left (Figure 1).
2.2 Inclusion criteria
Studies that met the following criteria were included: (A) The subjects are youth or young adults who averagely aged < 32 years—the age considered responding consistently to light stimulus in circadian phototransduction progress as addressed; (B) Must have bright light therapy as primary independent intervention, no sleep deprivation combined, since potential therapeutic effect may be disturbed by sleep deprivation; (C) Only difference between experiment and control groups should be BLT treatment, that when combined with antidepressants, it must be equally administered in both intervention and control to rule out the effect of the adjunct treatment; (D) Details of BLT included (e.g., specific device, light sources, illumination, correlated color temperature, continuous duration) for CSt,f calculation; (E) Outcomes reported in standardized depression scales, e.g., Hamilton Depression Scale HAMD, HIGH-SAD, Hospital Anxiety and Depression Scale (HADS), Beck Depression Inventory BDI-II, Montgomery Asberg Depression Rating Scale (MADRS), Center for Epidemiologic Studies Depression Scale (CES-D) or other scales with similar structures proved reliable and validate among worldwide AYA (61).
Studies that met the following criteria were excluded: (1) Broader age groups (average age >32); (2) LLLT (low-level laser therapy) treatment studies; (3) Abstracts, case reports, case series, or literature reviews excluded.
2.3 Data extraction
Among the 599 articles excluding duplication (Figure 1), the research team reviewed the titles and abstracts of all downloaded literature for initial screening. The articles were further filtered based on the relevancy of contents, and 279 were left. After thorough screening for relevancy and eligibility through a full-text review, we finalized 31 pieces of literature that met the criteria. Then, two dependent reviewers, R.P. and X.C., extracted the main information shown in Table 1: (A) Subject information, including age and gender; (B) Experimental design. BLT period, duration (weeks, times, exposure minutes); (C) Sample size of experiment group, control group; (D) Light therapy devices, spectra, illumination at eye level, lighting details, and distribution, which are parameters needed for CSt,f calculation; (E) Pre-to-post-treatment quantitative outcomes of measurement scales (detailed information can be checked in Supplementary Table S2).
2.4 Data analysis
Although the literature included patients with various pathologic features and was not limited to randomized controlled trials (RCT), the continuous outcomes variables of clinical depression measurement were recorded and analyzed. The mean (standard deviation) was derived as the main outcome at the starting point before intervention and the endpoint after intervention. The changes in scores were converted into the starting point and endpoint values. Heterogeneity among included studies was analyzed using χ2 tests (α = 0.05), and the magnitude was quantified in conjunction with Cochran's Q statistics and I2. The primary outcome was an improvement in depressive symptoms on a clinician-rated depression rating scale, including differences in endpoint scores on the scale between active and control conditions with intent-to-treat samples, analyzed using standardized mean differences (SMD). If the secondary outcomes were available (clinical response defined a priori as a 50% reduction on a clinician-rated depression rating scale, assumed more reported by RCTs), we calculated risk ratios and odds ratios for the categorical data. Correspondingly, the included studies were preliminarily categorized with additional consideration of co-medication × study design (Table 1). Besides, as light dose quantification had barely been reported in previous BLT-oriented reviews, the dose-response analysis was drawn from neighboring methodologies.
3 Results
3.1 Study characteristics
The selected 31 articles were mainly small-sample clinical trials (<30 subjects). Only four studies involved larger samples (31, 62–64). Twenty-two studies were randomized controlled trials (RCTs), three of which adopted intervention beyond light therapy: e,g., CBT (cognitive–behavioral psychotherapy) (29), LT + CBT (65), and wake therapy + LT (66) as control to testify whether BLT acted as an effective adjunctive intervention. Nine were non-randomized controlled experiments (quasi-experimental studies) that may have been launched because lighting properties (colors, levels) are intuitively recognized by the human eye (1); therefore, true blindness for light treatment studies is difficult to achieve.
Depression was mainly screened by the Diagnostic and Statistical Manual of Mental Disorders (DSM) criteria; similarly maladies, where depression was a frequent co-morbid condition. One article studied depressed adolescents with anorexia nervosa (29), one study focused on depression in co-morbidity with cystic fibrosis (67), one for cancer-related fatigue (CRF)/depression (68), one for Tourette's disorder/depression (69), two for bulimia nervosa/winter binge/depression (70, 71), one for borderline personality disorder (BPD) (72), four for perinatal/postpartum depression (22, 30, 73, 74), one for non-seasonal subthreshold depression (31). Besides, non-specific depressed non-depressed were also included, especially those accompanied by sleep disturbances. Three studies targeted people with Delayed Sleep-Wake Phase Disorder (DSWPD) (65, 75, 76). One article studied burnout (77), one was on insomnia/shift work disorder (63), and one focused on mild traumatic brain injury (mTBIs) that excluded Axis I mental disorders (78). One study was on healthy first-time mothers with low-birth-weight (LBW) infants (79). One study aimed to investigate the alerting effect of BLT; thus, only healthy people were included (80). The majority of participants in two studies were mainly healthy college students (81, 82), but depressed individuals constituted a certain proportion and were balanced among groups. In a few co-medication studies, BLT was accompanied by antidepressants or other treatments, but it could be guaranteed that BLT was the only variable.
In terms of intervention time, one study (83) carried out both morning and night BLT, two studies carried out BLT in the early evening (71, 81) and two experimented in late night (63, 80), and in the rest, BLT were all morning interventions. In terms of a consecutive duration time, 16 studies were carried out daily for consecutive 30–45 min of exposure, seven studies for 45–60 min, four studies for 50–60 min, one study for 90 min, one for daily 120 min, and one for 150 min. In terms of BLT devices, all but seven studies used light boxes or lamps, where light visors (six studies) and light masks (one study) were employed. As for active bright white light, treatment illuminance varied between 3,000 and 10,000 lux, and only a few adopted 2,500 lux (71). The glasses mainly emitted blue or green light at a much lower intensity, and the intervention CSt,f (circadian stimulus) ranged from <0.1–0.7 (Supplementary Table S2). A CS = 0.3 in the original metric of CS for at least 1 h in the morning has been shown to improve sleep and reduce depression empirically (84, 85), while CS < 0.3 was not expected to considerably suppress nocturnal melatonin. However, these conclusions may be less convincing for depression amelioration since this value was merely hypothesized for group comparisons. The analogic CSt metric also indicated no strict definition for high or low CSt threshold (32). As the CSt,f metric represents the instantaneous luminous stimulus for the circadian system (42), the vast majority of included studies considered responding to applied light intervention from circadian ways.
3.2 Risk-of-bias assessment
For randomized controlled trials (RCTs), the quality of evidence was evaluated with the revised Cochrane Risk of Bias Tool (86) (Supplementary Figure 1). One study adopted a randomizer box (87), six studies adopted computer software (31, 65, 68, 73, 76, 88), two studies adopted a random-number table (30, 81), one study adopted a random digit table (63), and one study adopted numbered, sequential, sealed envelopes (82). The remaining RCTs that indicated randomization sequence generation were also considered to be low risk in selection bias. Most studies owned moderate risk in allocation concealment since it was not reported (89). As for performance bias, seven studies showed moderate risk when participants were possibly non-blinded while specific examiners were blinded (29, 31, 65, 87, 90) or vice versa (22, 82). For attrition bias assessment, statistical approaches like LOCF (last observation carried forward) (87), BOCF (baseline observation carried forward) (78), and multiple imputations (66) were adopted, whereas bias still existed among dropouts. Linear Mixed Modeling (LLM) (69, 73, 76), General Linear Model (GLM) (66), and Hierarchical Linear Modeling (HLM) (77) were optimal, accounting for missing data, small sample size, and non-parametric distributions (91); thus, six studies were considered as at low risk. As for detection bias, seven studies where participants were informed of comparing different types of light therapy (30, 31, 66, 77, 78, 82, 87) were regarded as moderate risk since the blinding of outcome assessment remained unclear. For nine quasi-experimental/non-randomized controlled studies, the quality of evidence was evaluated using the Joanna Briggs Institute (JBI) Critical Appraisal Checklist for Quasi-Experimental studies (Supplementary Table S3). In two studies (83, 92), other measures remained unclear, and in two studies (69, 75), the baseline conditions could not be totally balanced, but the overall quality was eligible.
3.3 Circadian stimulus models and deduction
The evolving CLA and CS model and calculation formula had been optimized by Rea et al. (42) as follows (Eqs. 1, 2):
Where, b − y = ∫ScλEλdλ − k ∫ VcλEλdλ
k = 0.2616;
ab − y = 0.21;
arod1 = 2.30;
arod2 = 1.60;
g1 = 1.00;
g2= 0.16;
RodSat = 6.5 W/m2, representing the half-saturation constant of bleached rod cells;
,
Eλ: light source spectral irradiance
Mcλ: melanopsin sensitivity (corrected for crystalline lens transmittance) (93)
Sλ: S-cone fundamental (94)
mpλ: macular pigment transmittance (95)
Vλ: photopic luminous efficiency function (96)
: scotopic luminous efficiency function (96)
And,
where t represents the exposure duration time and has been fitted by a duration of consecutive 0.5–3 h in previous studies (56). f represents the spatial distribution of circadian light exposure. As most of the related studies used light boxes, lamps, or light visors/glasses with less visual field covering than Ganzfeld, f was all valued as 1.0. CLA, representing circadian light illumination (circadian lux), was determined by the physiological properties of human eyes and light sources. As some spectral information of the devices was unavailable, similar CIE standard light sources or devices have been substituted for CSt,f calculation (Table 1). Besides, we deduced that the accumulative light dose was also important and would contribute significantly to therapeutic efficacy. So that relationship was presumed as (Eq. 3):
where P(u) means the function of effect size, T(u) represents the function of accumulative exposure time, and CSt,f represents a continuous circadian stimulus. However, whether T stands for min or days was not yet known and was verified through meta-analysis and dose-response fitting.
4 Clinical efficacy of circadian lighting
The clinical efficacy of circadian lighting was elaborated through between-group efficacy comparison, pre-to-post-treatment evaluation, dose-response, and saturation deduction. We anticipated heterogeneity of study methodologies with variability in diagnosis (depression or not), co-medication, lighting administration, duration, and so on and hence planned several exploratory subgroup analyses (protocol/flow can be checked in Supplementary Figure 2).
4.1 Main heterogeneity sources
Pre- to-post-treatment outcomes were sub grouped based on study characteristics, based on which preliminary meta-regression was stratified separately by categorical covariates: (a) co-medication, (b) disease severity, (c) light intensity, (d) light color, (e) accumulative duration of exposure to light during intervention, (f) circadian stimulus of light, (g) intervention period and follow-up, and (h) whether study designed as RCT (Supplementary Table S4). The p-values of meta-regression had implied co-medication (p = 0.01) and temporal pattern (p = 0.000) as main sources of heterogeneity. Subgroup meta-analysis was further adopted to quantify the between-study differences, with I2 values of 25, 50, and 75% reflecting a small, medium, and large degree of heterogeneity, and H values of 1, <1.2, 1.2–1.5, >1.5 indicating non, small, substantial, and considerable heterogeneity.
Subgroup meta-analysis subdivided by co-medication × depression (shown in Tables 1, 2 Subgroup A) yielded a significant effect of this covariate on the outcome (p = 0.000), suggesting significant difference existed between studies with co-medication, without co-medication, and non-depressed (non-medicated) groups. Significant differences also existed between temporal pattern subgroups during the intervention (p = 0.044). None of the other tests showed a statistically significant influence of the moderator variable: light intensity (p = 0.208), light color (p = 0.241), CSt,f division (p = 0.543), intervention period/follow-up (p = 0.361) or study design (p = 0.105), whereas disease severity (p = 0.085) may be slightly more influencing (Supplementary Table S5). Notably, whether these factors yield a significant effect on the primary and secondary outcomes or not (see discussion in Section 4.2) could be dose-response confounding variables (see discussion in Section 4.3).
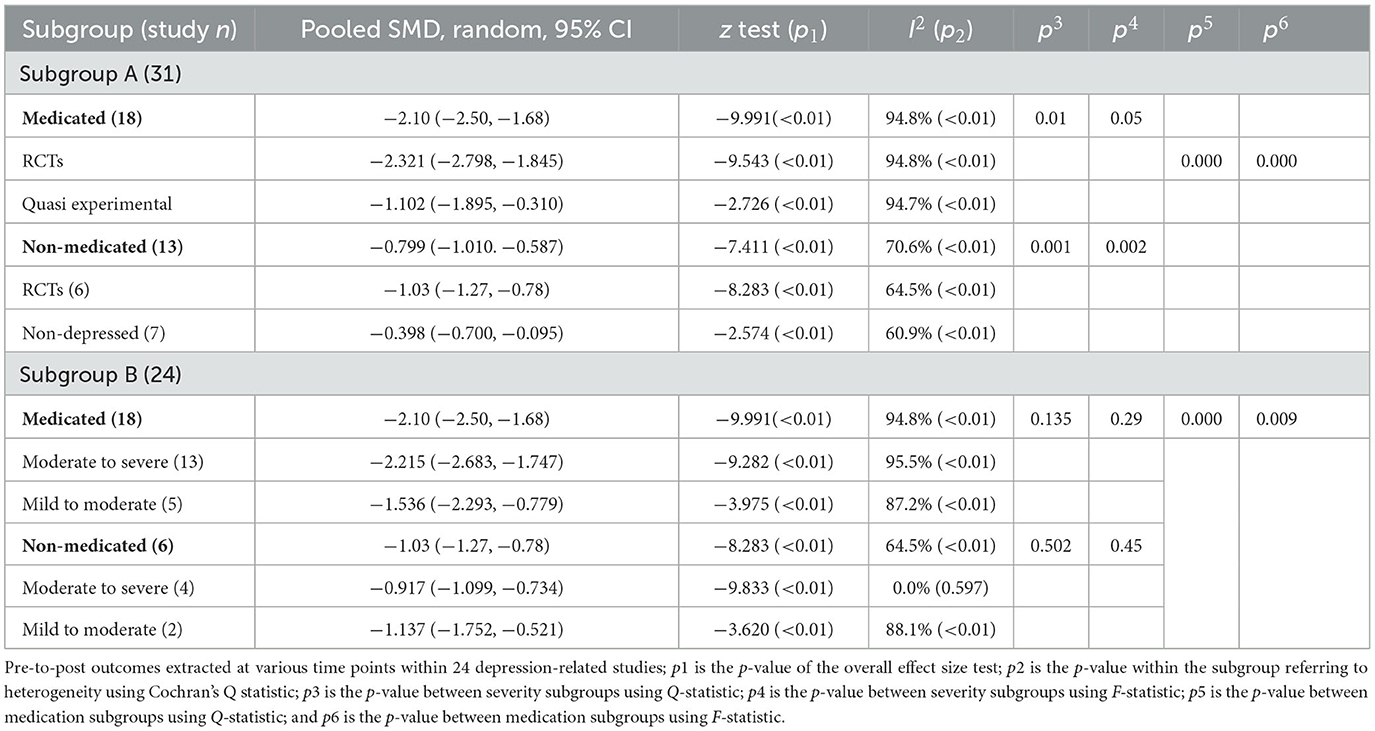
Table 2. Pre- to-post-treatment outcome sub grouped by study design, co-medication, and disease severity.
With co-medication suggested as a confounding factor, outcome data were further extracted within both co-medicated and non-medicated studies with only depressed participants. The meta-analysis (Table 2, Subgroup B) showed that 18 studies with co-medication (575 participants) obtained higher pre-to-post effect size (SMD = −2.1, 95% CI = −2.5 to −1.68; z = −9.991, p = 0.000; I2 = 94.8%) than those non-medicated (231 participants) (SMD = −1.03, 95% CI = −1.27 to −0.78; z = −8.283, p = 0.000, I2 = 64.5%), and the effect of this variable showed significant between-group heterogeneity [ = 19.14, p = 0.000]. The outcome was simultaneously confirmed in conjunction with the F statistic [F(1,83) = 7.08, p = 0.009].
To confirm the disease severity factor, a further subgroup meta-analysis of severity was undertaken. There was no significant between-group heterogeneity both with [ = 2.24, p = 0.135] and without [ = 0.45, p = 0.502] co-medication. Some inconsistency was observed in the subgroup with more severity—it yielded a numerically higher effect size (SMD = −2.215, 95% CI = −2.68 to −1.75; z = −9.282, p = 0.000; I2 = 95.5%) than a presumed milder subgroup (SMD = −1.53, 95% CI = −2.29 to −0.77; z = −3.975, p = 0.000; I2 = 87.2%) under co-medication conditions, whereas the reverse was observed in non-medicated studies.
4.2 Between-group efficacy comparison
Between-group efficacy comparison of circadian-active BLT vs. dim light control among RCTs was also studied with possible confounding factors such as visualization, co-medication, disease severity, as well as time pattern, which were all reported in the primary and secondary results.
4.2.1 Visualization factors
Assuming light intensity and light color across RCTs may exert visualization influence, they were examined as weighted random-effects meta-analyses (Figure 2), undertaking for each of the comparisons that were eligible for analysis, i.e., higher CSt,f of BLT vs. lower CSt,f of DLT- dim light therapy (four studies); higher CSt,f of BLT vs. non-circadian dim red light-DRL (seven studies); and higher CSt,f of GLT (green light therapy) vs. non-circadian DLT/amber light therapy-ALT (two studies). The intervention/control conditions were compared after parameter conversion (CSt,f in the intervention group > CSt,f in the control group).
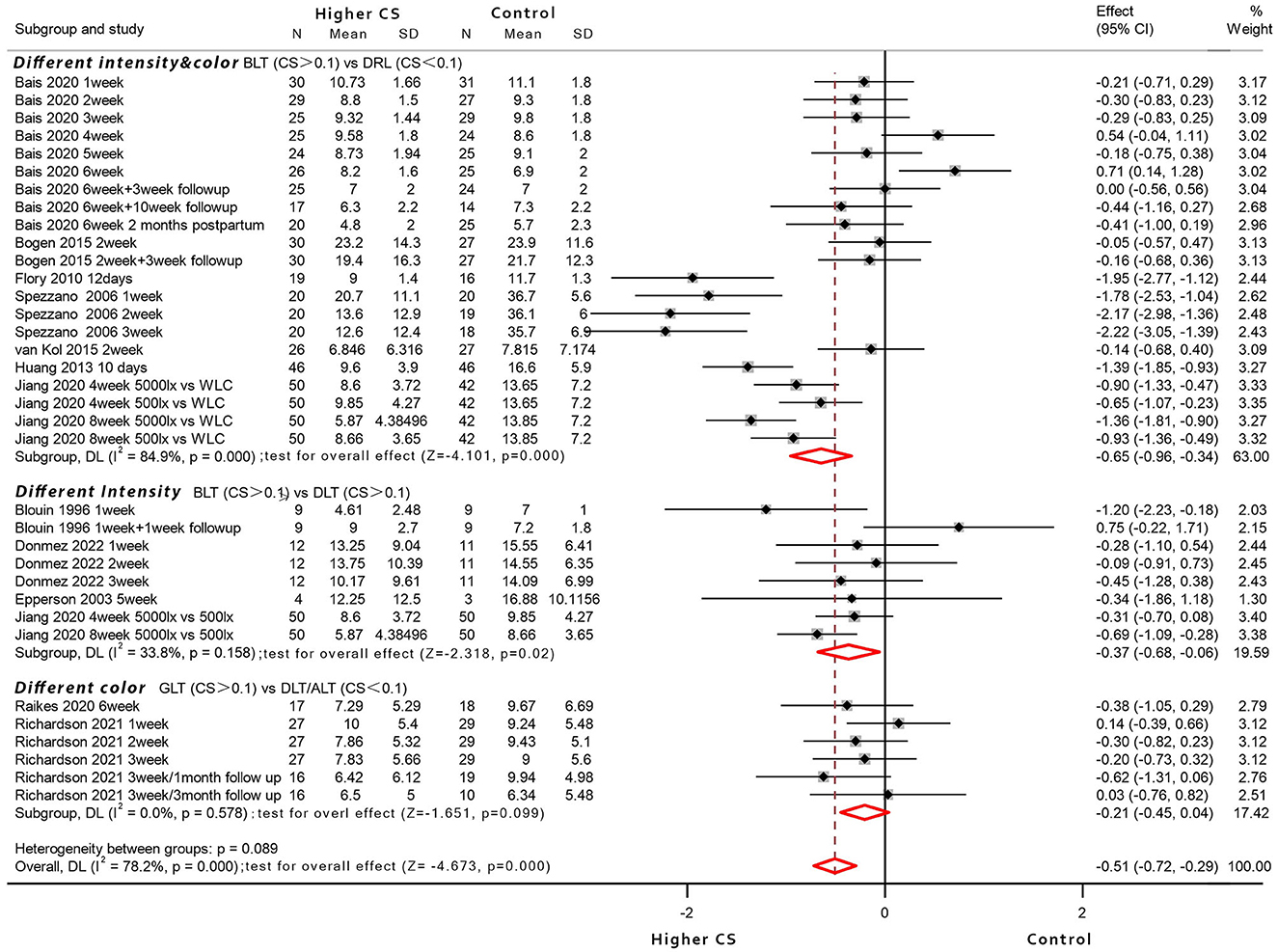
Figure 2. Forest plot displaying between-group meta-analysis of bright light therapy (higher circadian stimulus) vs. the control group (n = 12 articles; RCTs without identical baseline were excluded).
Eleven studies had <100 lux dim-light control (light box or light visor), four studies utilized a sham negative ion generator with working lights and sound (81, 88, 90, 97), four studies adopted 500 lux light to eliminate placebo effect as much as possible (22, 30, 31, 71), and one study employed an untreated control arm (31). To conclude, ten studies where intervention could be visually distinguished among different arms were included as a subgroup, and so were the two RCT studies that adopted similar devices with identical photopic lux (76, 78). The participants were reported keeping as naive as possible regarding the existence and effects of LT (70, 71, 77, 81), or they held identical expectations for different interventions (70, 78, 88, 90, 97), or the light devices were identical in shape and appearance among double-blind studies (30, 78). However, the placebo effect could hardly be ruled out—it was proposed that the circadian stimulus from a non-visual perspective would exert additional influence; thus, the between-group comparison was based on higher/lower CSt,f variation. Apart from exclusionary RCTs with unequal baseline [or not reported identical (68, 70, 79, 80, 82)], all others reported no statistically significant baseline difference and identical expectation of treatment response.
For the higher CSt,f of GLT vs. non-circadian DLT/ALT (different color, two studies) subgroup, where LT devices shared similar appearance with equal photon densities of blue-green light vs. amber/red light, the results for a total of 91 participants were evaluated, of which 44 patients received blue-green light (CSt,f > 0.1) and 47 patients received a placebo control light therapy (CSt,f < 0.1). The meta-analysis with random-effects models found no evidence for the significant efficacy of GLT compared to amber/red light conditions (SMD = −0.21, 95% CI = −0.45 to 0.04; z = −1.651, p = 0.099; I2 = 0%).
For the higher CSt,f of BLT vs. lower CSt,f of DLT (different intensity of white light, four studies) subgroup where bright white light (N = 75 participants) compared with mainly 500 lux white light (N = 73 participants), the former yielded significant efficacy with pooled effect size of −0.37 (95% CI = −0.68 to −0.06; z = −2.318, p = 0.02; I2 = 33.8%) compared to controls (CSt,f > 0.1 in both groups).
For higher CSt,f of BLT vs. circadian-inactive DRL (different intensity and color, seven studies), the results for a total of 430 participants were evaluated, of which 221 patients received BLT (CSt,f > 0.1), and 209 patients received a placebo control, presumably circadian-inactive (CSt,f < 0.1). The results showed an overall high heterogeneity with a significant heterogeneity index when included trials were pooled (I2 = 84.9%) and revealed the bright light superiority in depressive symptoms reduction compared to the control group (pooled SMD = −0.65, 95% CI = −0.96 to −0.34; z = −4.101, p = 0.000).
The secondary outcome was also pooled on the random-effects inverse-variance model. Relative risk and 95% CIs were calculated for the subset of nine RCT studies of which the number of subjects who experienced response was known (Supplementary Figures 3A, B). The pooled estimate RR of BLT over no light placebo was 2.39 (95% CI = 1.54–3.73; z = 3.856, p = 0.000; I2 = 28.4%; three studies). For comparison over DLT and DRL controls, a revealed RR of 1.33 (95% CI = 0.98–1.80; z = 1.823, p = 0.068; I2 = 0.0%; four studies) and 1.53 (95% CI = 0.71–3.29; z = 1.085, p = 0.278; I2 = 0.0%; three studies) showed no significant superiority. Similarly, calculated odds ratios (95% CI) showed a significant advantage over no light control (OR = 9.59, 95% CI = 3.7–24.88; z = 4.648, p = 0.000; I2 = 70.3%) and DLT control (OR = 9.59, 95% CI = 3.7–24.88; z = 3.370, p = 0.001; I2 = 0.0%), but no significant difference of response rates between BLT and DRL control was found (pooled OR = 2.13, 95% CI = 0.76–5.99; Z = 1.434, p = 0.152; I2 = 12.3%). Overall, there was no significant difference between the three subgroups subdivided by visual characteristics [SMD: = 4.83, p = 0.09; RR: = 2.78, p = 0.25; OR: = 0.50, p = 0.78].
4.2.2 Co-medication and disease severity factors
Since co-medication was largely a main heterogeneity source, in addition to the fact that response outcomes were barely reported by quasi-experimental trials, a subgroup meta-analysis was further carried out but focused on depression-oriented RCTs accompanied with or without co-medication (Supplementary Figures 3C, E). Where primary results indicated circadian-active BLT, the analysis showed significant efficacy compared to dimmer light controls under both co-medicated (pooled SMD = −0.47, 95% CI = −0.80 to −0.13; z = −2.749, p = 0.006; I2 = 82.0%; five studies) and non-medicated conditions (pooled SMD = −0.57, 95% CI = −0.81 to −0.33; z = −4.67, p = 0.000; I2 = 58.0%; four studies). There was no significant between-group heterogeneity caused by co-medication [ = 0.24, p = 0.62].
Secondary outcomes indicated that circadian-active bright light showed significantly greater response likelihood than controls among both medicated (pooled RR = 1.56, 95% CI = 1.24–1.95; z = 3.869, p = 0.000; three studies) and non-medicated individuals (pooled RR = 6.31, 95% CI = 2.34–16.99; z = 3.645, p = 0.000; five studies). Both subgroups showed non-significant heterogeneity (I2 = 0.0%), indicating significant between-group heterogeneity caused by co-medication [ = 8.45, p = 0.007]. Similar results of superiority could be drawn on a pooled estimate OR of 5.01 (95% CI = 2.79–8.99; z = 5.934, p = 0.000; eight studies) over controls. The noticeable superiority of response possibility may be due to co-medication that caused significant between-group heterogeneity [ = 5.23, p = 0.004]. Both outcomes were further confirmed [co-medication RR: F(1,13) = 8.24, p = 0.01; OR: F(1,13) = 7.75, p = 0.01].
Response data was further extracted and subdivided by disease severity as a secondary subgroup. On the whole, consistent conclusions could be drawn from secondary outcomes among non-medicated studies (Supplementary Figure 3F), with a pooled RR value indicating circadian-active bright light showing overall superior response rate compared to controls, whether not significant under more severe conditions (pooled RR = 2.20, 95% CI = 0.86–5.63; z = 1.648, p = 0.099; I2 = 0.0%; three studies) or significant under milder conditions (pooled RR = 1.53, 95% CI = 1.21–1.92; z = 3.578, p = 0.000; I2 = 0.0%; two studies). Similar results were shown among co-medication studies (Supplementary Figure 3G), which showed significant superiority under more severe conditions (pooled RR = 6.29, 95% CI = 2.18–18.14; z = 3.405, p = 0.001; I2 = 0.0%; two studies) or non-significant under milder conditions (pooled RR = 6.43, 95% CI = 0.39–106.44; z = 1.299, p = 0.194; I2 = 0.0%; one study). There was no significant between-group heterogeneity among severity subgroups regarding response rates both with [ = 0.01, p = 0.457] and without [ = 0.42, p = 0.989] co-medication, confirming “co-medication” rather than “disease severity” was an efficacy comparison influential factor.
4.2.3 Time pattern factors
The time factor (cumulative duration) that was implied as a heterogeneity source was further elaborated among depression-related RCTs. Primary results indicated circadian-active BLT showing significant or non-significant efficacy compared to dimmer light controls under various duration conditions (Supplementary Figure 3H), with combined results significantly indicating superior efficacy than the control group (pooled SMD = −0.49, 95% CI = −0.71 to −0.27; z = −4.34, p = 0.000; I2 = 77.7%; nine studies). There was no significant between-group heterogeneity caused by time pattern factor [ = 9.03, p = 0.06].
Similar secondary pooled RR and OR estimates of eight RCTs indicated that the circadian-active bright light showed overall greater response likelihood than controls. There was no significant between-group heterogeneity caused by time [RR: = 6.72, p = 0.235; OR: = 7.88, p = 0.146], whether significant or not (Supplementary Figures 3I, J).
In conclusion, due to the non-ignoring visual distinction and the placebo effect, the efficacy of the bright light intervention compared to both circadian-inactive and active control conditions cannot be simply elucidated from the “circadian” perspective. Nevertheless, it seemed not all observed treatment responses were related to the non-specific effects since some cases had reported no relationship between expectation and improvement (88). On the whole, circadian-active BLT, whether significant or not, showed a greater possibility of response than active/inactive placebo, regardless of visualization, co-medication, disease severity, or cumulative duration that were assumed as confounding factors; co-medication alone showed more likelihood.
4.3 Dose-response relationship and influencing factors
A dose-response relationship was quantified with only vital continuous parameters such as CSt,f, and accumulative exposure time and was explored among the circadian studies (CSt,f > 0.1; 31 studies, N = 813 participants). The single covariate was carried out in meta-regression to show the statistically significant influence of the moderator variable (Figure 3), indicating accumulative exposure min/h as irrelevant explanatory covariate (no explanation, R2 = 1.01%, p = 0.582) and relevant covariate as accumulative exposure days (some explanation, R2 = 12.43%, p = 0.003 < 0.05) and CSt,f value (weak explanation, R2 = 5.71%, p = 0.041 < 0.05).
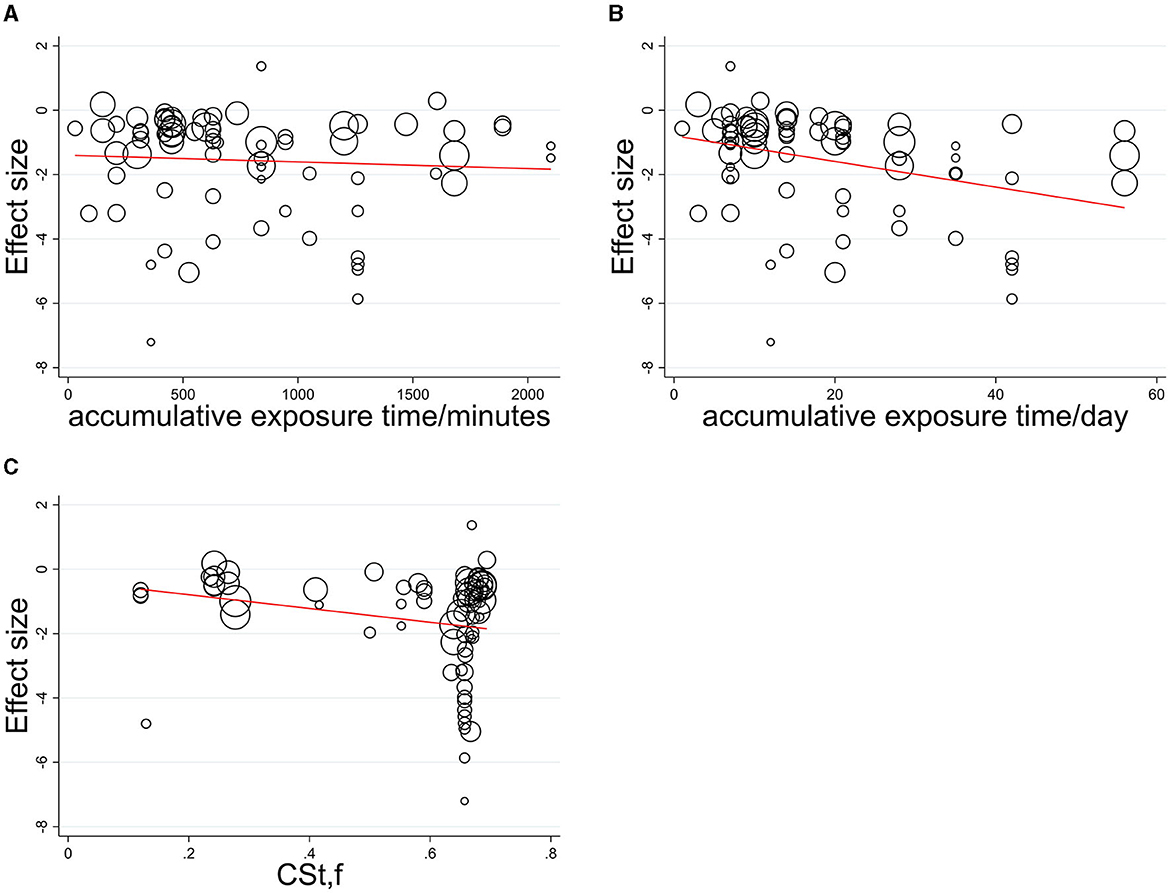
Figure 3. Meta-regression relationship between effect size and its standard error with (A) accumulative exposure min/h, (B) accumulative exposure days, (C) CSt,f as a covariate.
Fitting was further carried out by two independent variables (CSt,f, time) among the sub grouped medicated and non-medicated studies to show their contribution to therapeutic effect size, utilizing the Levenberg Marquardt algorithm. The 3D fitting outcomes among medicated patients (Figures 4–6) offer a glimpse of the relationship between accumulative circadian stimulus and malady reduction. Based on current data, the fitting adaptability of accumulative exposure days (R2 = 17.0–33.7%) was overall better than minutes (R2 = 4.59–12.62%). Meanwhile, accumulative circadian stimulus, i.e., P(u) illustrated by T(u) and CSt,f may largely be explained by polynomial models with better goodness of fit that shown in Equations 4 and 5:
or
where a, b, c, d, e, f are all parameters working on the slope and direction of the curve. T represents accumulative exposure time. And the models implied that the therapeutic effect may reach saturation.
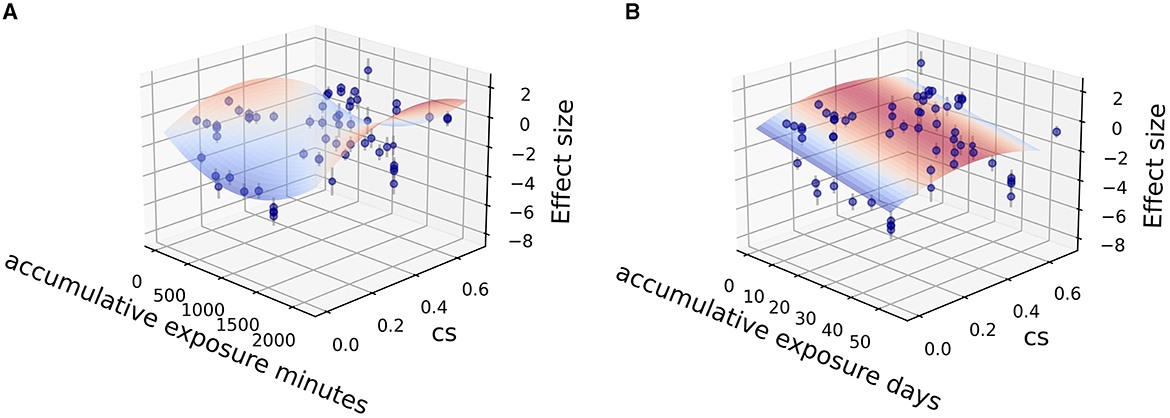
Figure 4. Fitting for data points clusters of 18 co-medication studies on poly 2D fitting models. (A) Poly 2D model fitted with accumulative exposure minutes, z = 1.0178 – 0.004x – 10.544y + 0.000002x2 – 15.33y2 + 0.00156xy (R2 = 6.07%). (B) Poly 2D model fitted with accumulative exposure days, z = −0.488 – 0.153x + 6.999y + 0.002x2 – 9.358y2 + 0.004xy (R2 = 17.0%).
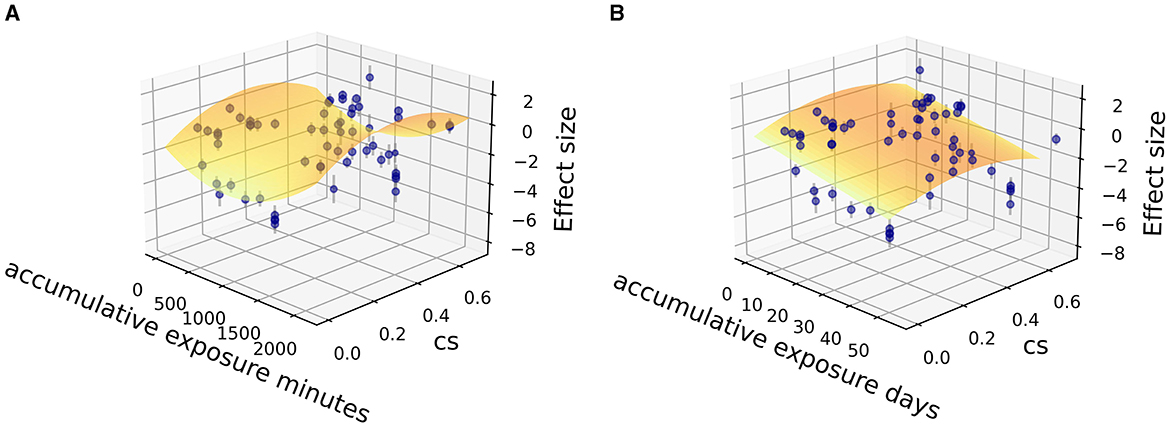
Figure 5. Fitting for data points clusters of 18 co-medication studies on parabola 2D fitting models. (A) Parabola 2D model fitted with accumulative exposure minutes, z = −1.50 – 0.00368x + 11.78y + 0.00000195 x2 – 15.31y2 (R2 = 4.59%). (B) Parabola 2D model fitted with accumulative exposure days, z = – 0.52 – 0.15x + 7.127y + 0.0021 x2 – 9.421 y2 (R2 = 17.0%).
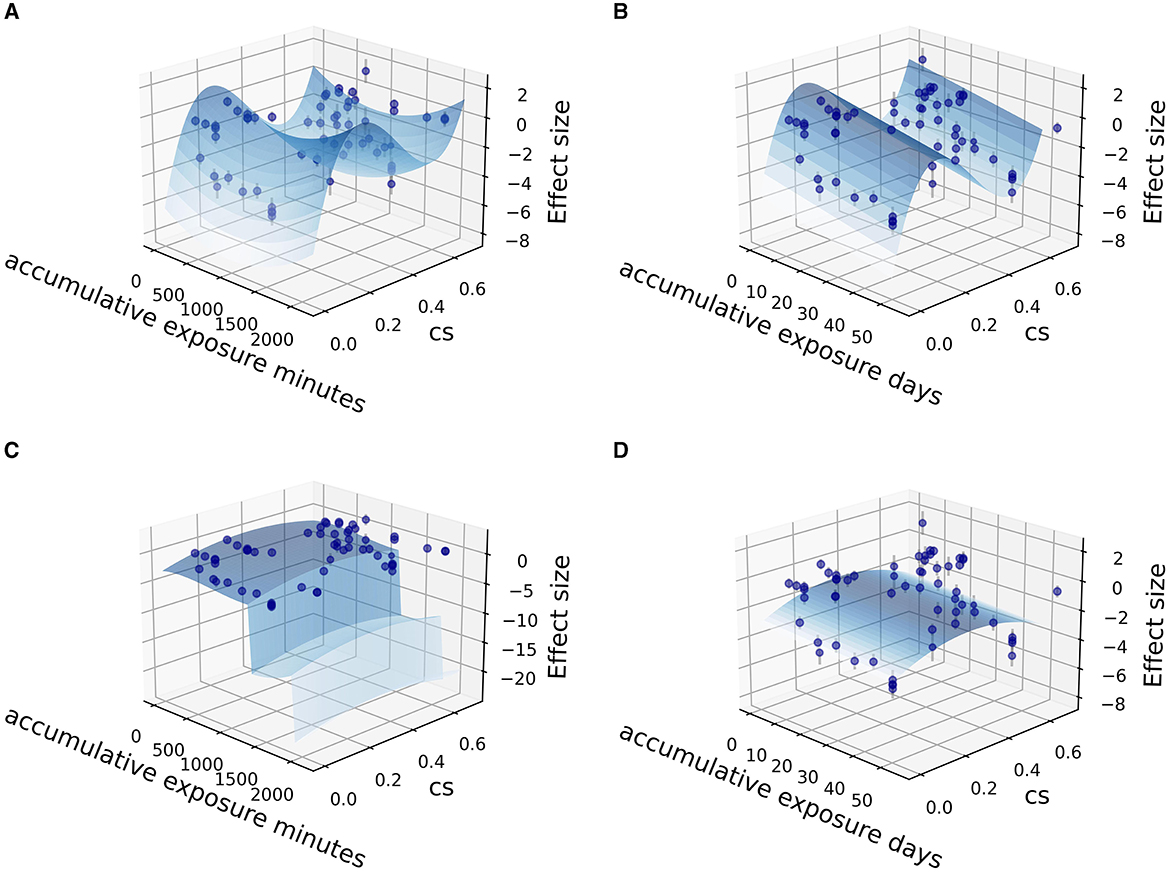
Figure 6. Fitting for data points clusters of 18 co-medication studies on polynormal 2D fitting models. (A) Polynormal 2D model fitted with accumulative exposure minutes, z = 6.211–0.0037x + 0.000975x2 + 80.506y + 0.00000188x2 – 265.733y2 + 236.39y3 (R2 = 15.62%). (B) Polynormal 2D model fitted with accumulative exposure days, z = −5.349 – 0.1556x + 0.002x2 + 75.99y + 0.00000188x2 – 258.30y2 + 233.96y3 (R2 = 28.0%). (C) Polynormal 2D model fitted with accumulative exposure minutes (–). (D) Polynormal 2D model fitted with accumulative exposure days, z = 2.933 + 0.306x – 0.0185x2 + 0.000243x3 + 8.649y – 11.993y2 (R2 = 33.76%).
The overall dose-response relationship among depressed, co-medicated patients (18 studies) implied saturation would reach at about 36 days (1,000 min) modeled on both poly 2D parabora2D equations and 34.4 days modeled on polynormal 2D models.
Figures 7, 8 show that the dose-response relationship between therapeutic effect size (SMD value) and its standard error with accumulative exposure time as an independent variable in various CSt,f ranges fit well with the exponent function or polynomial function. Among medicated individuals, The effect size would reach saturation in about 1,000 min/36–38 days in CSt,f < 0.1–0.4 range (n = 7 studies, 26 items), 900–1,000 min/32–33 days in 0.6–0.665 range (n = 7 studies, 20 items), and 40 days in 0.665–0.7 (n = 7 studies, 13 items) ranges (780–850 min/37–38 days in 0.6–0.7 range, 970–1,000 min/41–42 days in 0.2–0.7 range). On the whole, in most “circadian” conditions (0.2 < CSt,f < 0.7), polynomial models implied the saturation would reach 900–1,000 min (32–42 days) as temporal saturation for medicated AYAs.
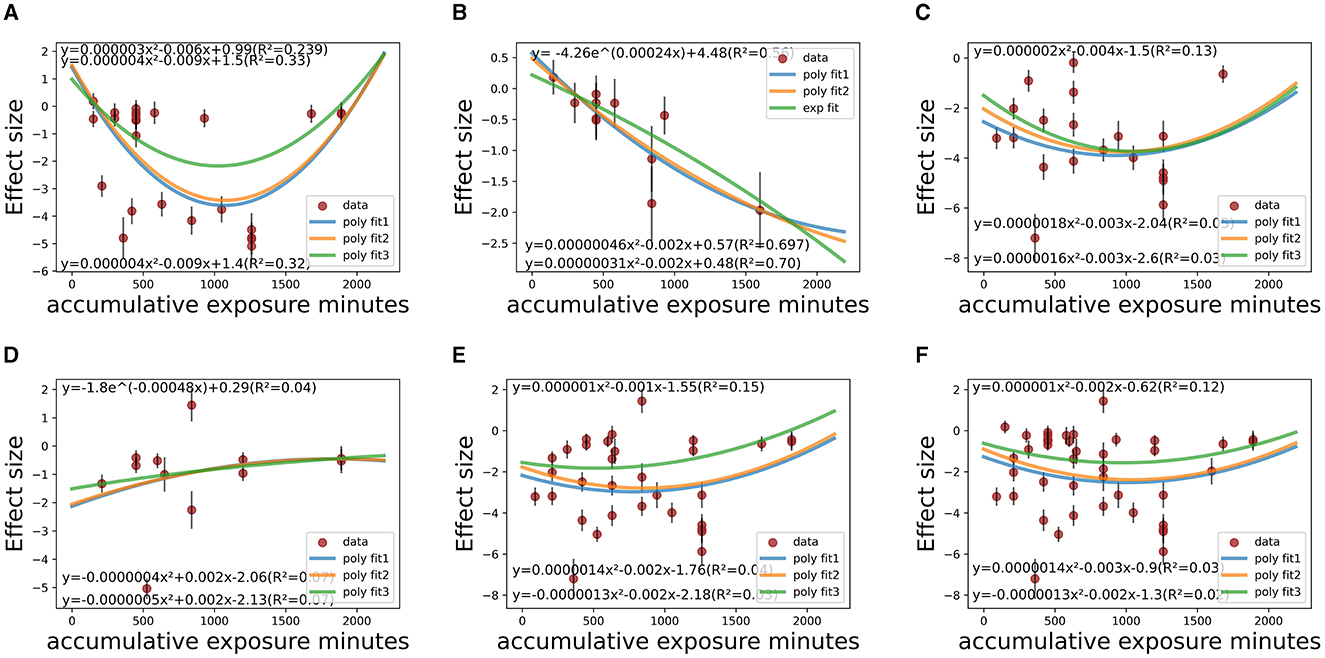
Figure 7. Temporal fitting (accumulative exposure minutes) among co-medicated studies within various CSt,f ranges. (A) CSt,f range < 0.1–0.4 (R2 = 23.9–33.0%). (B) CSt,f range 0.40–0.60 (R2 = 28.2–30.8%). (C) CSt,f range 0.60–0.665 (R2 = 1.0–13%). (D) CSt,f range 0.665–0.7 (R2 = 4.0–7.0%). (E) CSt,f range 0.60–0.70 (R2 = 3.0–15.0%). (F) CSt,f range 0.20–0.70 (R2 = 2.0–12.0%).
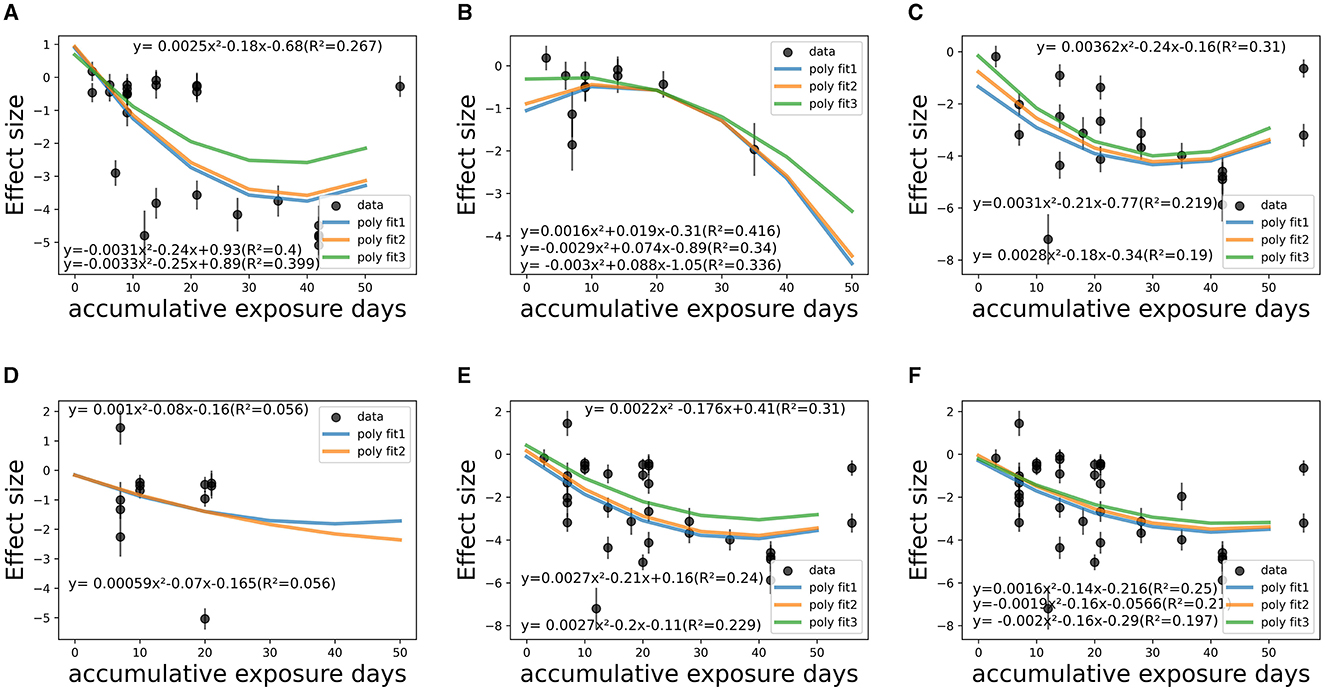
Figure 8. Temporal fitting (accumulative exposure days) among co-medicated studies within various CSt,f ranges. (A) CSt,f range < 0.1–0.40 (R2 = 26.7–40.0%). (B) CSt,f range 0.40–0.60 (R2 = 33.6–41.6%). (C) CSt,f range 0.60–0.665 (R2 = 19.0–31.0%). (D) CSt,f range 0.665–0.70 (R2 = 5.6%). (E) CSt,f range 0.60–0.70 (R2 = 22.9–31.0%). (F) CSt,f range 0.20–0.70 (R2 = 19.7–25.0%).
Similar quantification outcomes were checked in non-medicated and non-depressed people (Supplementary Figures 5, 6) and severity × co-medication interaction tests (Supplementary Figures 7, 8). For non-medicated, depressed people (six studies), it was found that saturation would reach in ~1,350 min (accumulative) fitted by the poly 2D model and 1,450 min (58.9 days) by the parabora2D equations. The polynomial models implied 1,100–1,500 min as temporal saturation for non-medicated AYAs. Similar outcomes were verified among those who suffered at least moderate disease severity, where 700–1,000 min (22–41 days) saturation was implied with co-medication and 700–1,500 min for the non-medicated subgroup, indicating the dominant synergistic effect of medication. In contrast, among those with mainly mild depression (seven studies) or non-depressed (seven studies), the saturation seemed indistinct. The confounding effect of disease severity was not qualified due to limited samples.
For circadian RCT studies (CSt,f > 0.1), the implied saturation reached about 1,000 min (46 days) based on the poly2D model, 1,145 min (44 days) on the parabora2D model, and 1,230 min (44 days) modeled on the polynormal 2D equation. No quantitative conclusions were derived among quasi-experimental studies. Whether the study design was influencing remains ambiguous (Supplementary Figure 9).
On the whole, CSt,f, duration, and co-medication proved to be dose-response influencing factors, whereas the potential confounding effects of disease severity, study design, and lighting administration are yet to be fully explicated (Supplementary Figures 4, 10). Overall, 1,000–1,500 min (~30–60 days) of accumulative duration can be inferred as saturation, as fully discussed with certain factors. Meanwhile, it is concluded that accumulative duration T(u) suitably fitted into the polynomial model within various CSt,f intervals, and the relationship did not change significantly after adjusting for various confounding factors (equation 6), that:
4.4 Saturation of light therapy
Since continuous dose-response analysis showed a non-linear relationship between temporal pattern and depression reduction, subgroup meta-analysis of temporal pattern within CSt,f variation was further specified, considering co-medication as a covariate. Depression reduction showed in pooled estimates of SMD was associated with cumulative duration intervals (subdivided by 5, 35, 65, 95%, i.e., dose division). The categorical dose-response analysis was undertaken by comparing 0–300 min/300–500 min/500–1,000 min/1,000–1,500 min/>1,500 min subgroups with each other, using random-effects modeling techniques, and p-values < 0.05 were considered statistically significant with all double-sided testing (Table 3).
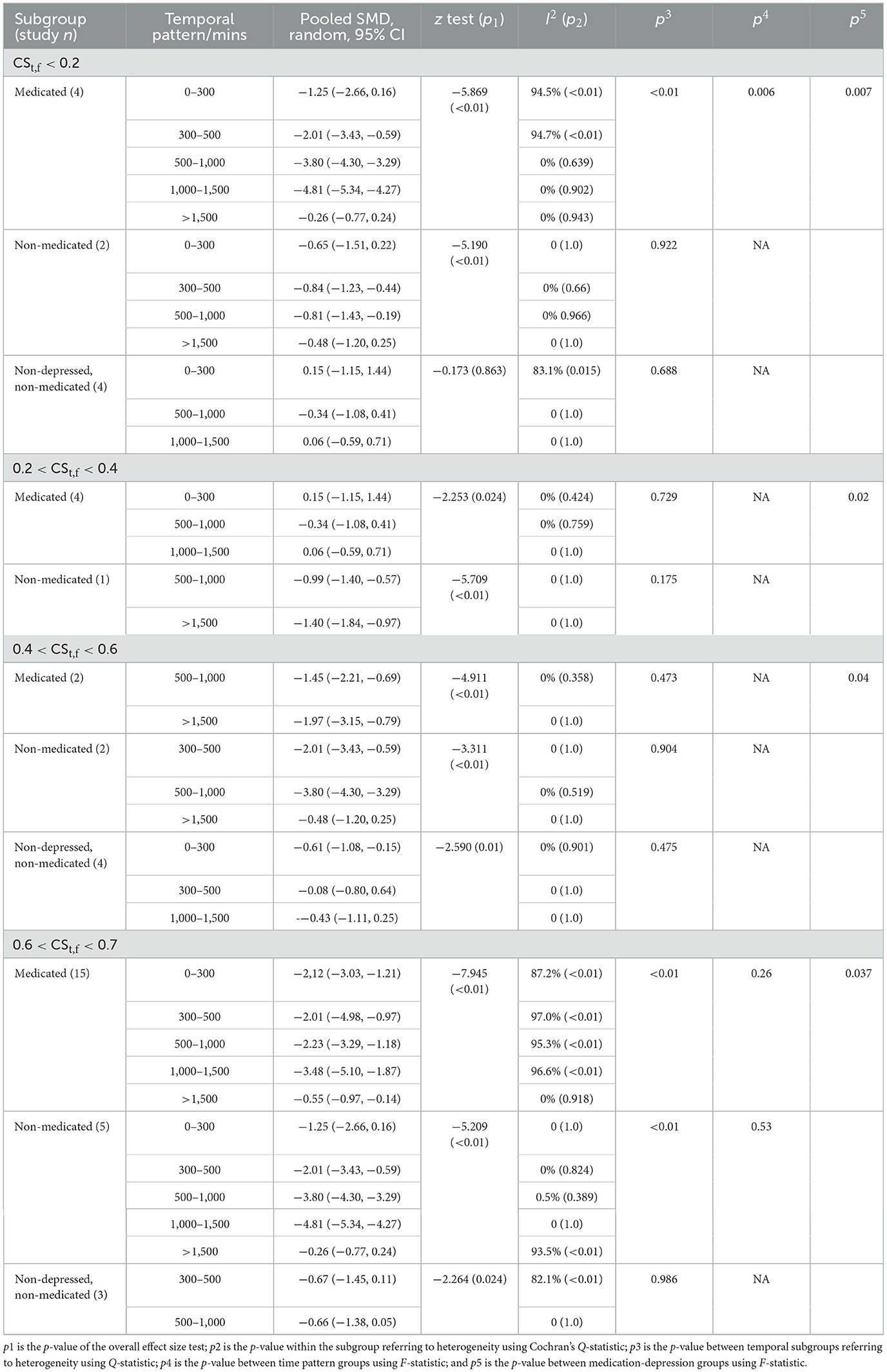
Table 3. Significant between-group differences are sub grouped by main heterogeneity sources (CSt,f, co-medication, and temporal pattern).
In the CSt,f < 0.1–0.2 range that is barely considered as “circadian” condition, there was significant pre- to post-treatment difference between 1,000–1,500 min/>1,500 min (p = 0.0018), 1,000–1,500 min/0–300 min (p = 0.004), 1,000–1,500 min/300–500 min (p = 0.008), and 500–1,000 min/0–300 min (p = 0.03), 500–1,000 min/>1,500 min (p = 0.01) subgroups among depressed and medicated AYAs, indicating time as a confounding factor [F(4,12) = 6.06, p = 0.006]. There was no significant between-group heterogeneity among depressed but non-medicated persons (p = 0.922), nor among non-depressed, non-medicated people (p = 0.688). In contrast, there was a significant difference between the three subgroups [F(2,24) = 5.99, p = 0.007].
In the CSt,f 0.2–0.4 range, there was no significant between-group heterogeneity of time pattern among depressed and medicated patients (p = 0.729) or non-medicated people (p = 0.175). No significant pre- to post-treatment difference was observed between various duration subgroups [F(2,5) = 0.33, p = 0.73]. In contrast, a significant difference existed between with/without co-medication subgroups [F(2,8) = 15.01, p = 0.002].
In the CSt,f 0.4–0.6 range, there was no significant between-group heterogeneity of time pattern among medicated patients (p = 0.473), depressed but non-medicated patients (p = 0.904), and non-depressed, non-medicated people (p = 0.475). In contrast, a significant difference existed between these three groups [F(2,8) = 4.55, p = 0.04].
In the CSt,f 0.6–0.7 range among the depressed but non-medicated people, there was significant between-group heterogeneity (p = 0.004 < 0.5), but it could not be explained by between-group differentiation due to high heterogeneity within subgroups. No significant difference was found between temporal pattern groups [F(4,7) = 0.87, p = 0.53]. In the CSt,f 0.6–0.7 range among depressed, medicated people, no significant pre to post-treatment difference was found between time pattern differentiation [F(4,29) = 1.39, p = 0.26]. However, there was a significant difference between 1,000 and 1,500 min/>1,500 min subgroups (p = 0.03 < 0.05). Similarly, a significant difference was observed between the three groups [F(2,45) = 3.52, p = 0.037].
In conclusion, 1,000–1,500 min of accumulative exposure duration is suggested as a threshold, especially on a higher CSt,f basis, and co-medication was verified as the main heterogeneity source, corresponding with previous outcomes.
4.5 Publication bias
Using Egger's linear regression test, we found there existed publication bias and small study effects (Supplementary Figure 11) with all outcomes (97 items, intercept = −6.25, 95% CI = −8.52 to −3.99, t = 5.48, p = 0.000 < 0.05), only “circadian” studies (73 items, intercept = −4.82, 95% CI = −7.23 to −2.41, t = 3.98, p = 0.000 < 0.05), only “depression” studies (85 items, intercept = −7.27, 95% CI = −9.69 to −4.86, t = 5.99, p = 0.000 < 0.05), only co-medicated studies (61 items, intercept = −10.75, 95% CI = −13.62 to −7.88, t = 7.49, p = 0.000 < 0.05), as well as only RCT studies (85 items, intercept = −7.20, 95% CI = −9.61 to −4.78, t = 5.94, p = 0.000 < 0.05), respectively. However, for the purpose of dose-response quantification, the more data was included, the better.
5 Discussion
5.1 Efficacy of specified, quantified circadian light therapy
To our knowledge, the study may not be the first systematic review of BLT on youth, but it is the first meta-analysis of lighting therapy focusing on circadian stimulus and its accumulative dose-response on depression-related illnesses for AYAs. On the whole, bright light therapy for depressed AYA with higher CSt,f cannot be proved significantly efficacious over lower CSt,f light interventions since symptom reduction was seen in both groups. It was largely influenced by both circadian ways and visual ways, accompanied by the fact that strong circadian evidence has not been found in young individuals with severe visual impairment or blindness (capacity for photoentrainment may be sustained) since empirical evidence was only derived from certain adults (98). However, measuring circadian timing in future trials would allow for a more rigorous examination of mechanisms (and possibly different pathways) linking circadian misfunction with depressive symptomology.
To explore circadian stimulus connection with therapeutic efficacy, CSt,f can indeed be used as a metric quantification method for its accuracy in circadian phototransduction process in AYA depression-oriented clinical trials and theoretical studies. The conclusion was supported and validates previous conclusions quantitatively in the following ways. (1) It can be conservatively concluded that when exerting light exposure with certain circadian stimulus (CSt,f = < 0.1–0.7), 30–2,100 min of accumulative time (roughly within 8 weeks) is efficacious for disease amelioration. Despite the value of I2 (I2 = 92.8%) indicating a high degree of heterogeneity, the pooled SMD values of the vast majority of studies indicated at least small (>0.2) to large (>2.0) change of effect size. The result has supported a broader range of CSt,f of light therapy compared to previous conclusions where CSt,f ranged from 0.57 to 0.7 (1).
(2) The therapeutic effect has shown a positive relationship with increasing light dose in both within-group and crossover changes. For young people primarily aged <32 (approximate mean age 22.3 ± 7.4), temporal duration of exposure contributed up to about 20–30% (or much higher) to within-group effect size variation (fitted by various models), while the therapeutic effect size was less be explained by CSt,f (R2 = 5.71%), co-medication (R2 = 6.94%) or hardly by other confounding factors, indicating that overall temporal pattern was the most crucial. These quantified conclusions have been drawn from regression models performed by Statas 17.0, CMA 3.0, and Python 3.9 that polynomial 2D models can better illustrate quantification correlation between therapeutic effect and accumulative circadian stimulus, despite fitting models showing imperfection statistically (much-oscillated R2).
(3) Dose-response saturation. From the dose-response fitting and subgroup meta-analysis of temporal patterns, accumulative 900–1,000 min (32–42 days) of duration may be the saturation for depressed and medicated AYAs and 1,100–1,500 min (58–59 days) for non-medicated patients. Albeit, 1,000–1,500 min (5–7 weeks) of accumulative exposure duration showed more efficacy in symptom reduction than <1,000 min (3–4 weeks) or >1,500 min (7+ weeks) subgroups within high circadian stimulus (0.6 < CSt,f < 0.7). For CSt,f < 0.2 intervals that are barely considered as “circadian” conditions, accumulative 500–1,000 min duration may be the most efficacious among depressed and medicated AYAs. Meanwhile, for non-depressed individuals, the temporal pattern could not be verified due to limited samples. The results suggest that for common LT devices (LT-box, lamps, glasses), 1,000–1,500 min (5–7 weeks) of the threshold may be saturation combined with medication, regardless of their lighting features (e.g., light levels, spectra, light distribution). This conclusion endorses and expands previous conclusions, suggesting 2–5 weeks of exposure (16).
(4) Possible polynomial models on accumulative circadian stimulus and therapeutic effect have been quantified beyond consecutive light dose (CSt,f value), which has not been illustrated in previous studies.
5.2 Heterogeneity and clinical efficacy discussion
Discussion on clinical efficacy with regard to PICO principles: (1) Participants. Although the subjects discussed were all adolescents and youth, their depression episodes, phenotype, severity, light exposure history, and co-medication status may have caused differentiation and heterogeneity. From another perspective, there is preliminary evidence with regard to various circadian-related illnesses where light therapy has shown improvement [e.g., bipolar depression (99), atypical depression (100), melancholic depression (100), unipolar depression (101), light therapy with more accumulated circadian stimulus may be an efficacious treatment for “circadian” depression (102)], where conventional pharmacological intervention had poor responses. Indeed, AYAs have benefited from light therapy as an adjunctive, additive, and non-invasive treatment to their continued treatment modalities despite uncertainties and difficulties. In this study, the discrepancy may partially be explained by demonstrated resistance to pharmacotherapy (83) or depression severity [mild depression might coexist (62, 67, 76, 81, 82, 92)], whilst compliance and adverse side effect did not appear to be the confounding factors.
As indicated, bipolar depression, major depressive disorder, postpartum depression, subthreshold depression, and dysthymia may share and respond to similar lighting therapeutic mechanisms. However, as not yet extensively investigated, the presence of certain comorbid disorders may compromise treatment efficacy, e.g., whether seasonality or comorbid SAD increases the likelihood of positive response to light (103) as reported included (22, 71, 72, 74, 88, 97), or Axis I anxiety disorders (90) and Axis II personality disorders vice versa (72). For most non-comorbid cases, a reduction in disease severity had been observed (30, 31, 70, 78). On one hand, it is necessary to identify homogenous patient groups. On the other hand, we still emphasize the vital role of dosing. As had been implied, emerging hypomanic symptoms may be relieved after a small increment in exposure duration (22), but qualitative discussion on comorbidity alone may be far from sufficient.
(2) Intervention perspective. Only three studies excluded any form of intervention (medication, psychotherapy, etc.) within at least the past 6 months (30, 31, 75). Three studies reported no medication (22, 82, 87). A few studies reported no recently initiated antidepressants or the use of psychotropic medication had remained stable (68, 70–72, 74, 88). Several studies excluded light-sensitizing medication that may act as photosensitizers and increase the risk of eye/skin damage (62, 74). Additional interventions were generally balanced between experiment vs. control groups, and participants from both groups had received identical medication/psychotherapy, if applicable (64). A few studies reported medication had little or no effect on the overall result (66, 97). However, it is scarcely possible that the evaluation of light therapy on mood eliminated a potentially confounding variable of medication. Light therapy has been reported with a clear synergistic effect when combined with SSRIs (selective serotonin reuptake inhibitors) during a moderate to severe major depressive episode (4). Though medication components were barely reported by included studies, significant differences between with and without co-medication were seen not only in between-group comparison but also in pre-to-post-effect size and dose-response relationship, although it was impossible to completely separate the effects of co-medication from depression severity. Additionally, it is less possible that medication masks the effects of light therapy since the outcomes of LT monotherapy were found to be equivalent or superior to that of the medicated group on a lower CSt,f basis (Table 3).
(3) Experimental design. In this study, both RCTs and non-randomized experimental studies were included for therapeutic efficacy evaluation, while few previous studies have discussed the aspect by merely including RCT studies (3) since total blindness for RCTs is quite hard to achieve, as mentioned. Since intuitive, neurophysiological, and chronobiological light therapies are distinct from pharmaceutical interventions, we deduced less differentiation caused by design methodology and combined overall outcomes by fully discussing between-group differences and comparing dose-response saturation. Moreover, the heterogeneity could be influenced by in-, out- patients or whether they adopted home-based protocol, since those administered in laboratory or hospital treatment rooms where the protocol may largely be correctly followed had implied more eligibility than those less-supervised home-based evidence.
(4) Statistics. Although SMD effect size and random-effect model were applied for collected data, depression measurement outcomes with different scales may have led to certain heterogeneity.
6 Limitation
The study has several limitations. On the whole, there were limited samples since only a few studies focused on AYA-oriented depression light therapy with mainly small samples. In order to elaborate on accumulative light stimulus and reductions in relevant maladies, 31 articles (N = 1,031) included not only diagnosed depressed individuals but also healthy participants for circadian improvement intentions. Since the adopted outcomes were SMD mean values rather than individual results, the meta-regression may result in aggregation bias. The outcomes of fitting accumulative exposure time and CSt,f as two independent variables indicated imperfection in mathematics. However, the results had been adjusted and intercalibrated with subgroup meta-analysis and have implied trend and saturation of accumulative light dose.
The limitations based on CSt,f model, are as follows: (1) Despite that CSt,f values ranged from <0.1–0.7, it is scarcely possible that continuous CSt,f values can be acquired. Subgroup meta-analysis and meta-regression have been carried out on this basis, by which the accuracy of the fitting models was also influenced by restricted CSt,f values. (2) Spatial distribution. The circadian light spatial distribution factor f and intensity have not been totally validated. Parameters like the distance, the angle between the lighting device and human eyes, light source positions (104), and background reflection factors were not provided. Therefore, CSt,f factor was not well-discussed without details. (3) Intervention moment factor may also be influential. Some studies (105, 106) have quantified light moments in circadian phase shift and DLMO calculation. However, since only one included study (83) carried out morning and night BLT, four studies carried out nocturnal BLT (63, 71, 80, 81). In some studies, subjects were relatively flexible in receiving BLT at home; further validation is needed for the quantification. The quantified model could be explored in future studies with larger samples and specific individual results.
At present, there is only a CSt,f model in discussion; other light dose-related responses through light-sensitive circuits have not yet been explored. More targeted phototherapy studies on depression-related light-sensitive circuits on patients with different depression phenotypes, severity, light exposure history, physiological characteristics, gender as well and exposure duration are necessary for therapeutic efficacy validation. Moreover, light therapies and correspondent circadian stimulus for combined treatment (e.g., antidepressants, chronotherapy) should be explored with consistent clinical trials and follow-ups. In addition to larger, all-around samples and precise experimental design for heterogeneity reduction, more objective parameters and indicators are necessary for efficacy evaluation beyond standardized depression measurement outcomes. Objective evaluation methods and approaches like neuron-related blood inflammatory markers (107), cerebrospinal fluid (CSF) analysis (108), or brain physiological examinations electroencephalogram/EEG (109) can also be adopted as evaluation tools when possible.
7 Conclusion
The significant efficacy of a higher circadian stimulus of light therapy over a lower circadian stimulus of light intervention among AYAs remains unproven. Yet, factors such as co-medication, disease severity, time pattern, visual characteristics, etc., are considered sources of heterogeneity that affect the response potential. Conservatively, light therapy with certain circadian stimuli has indicated significant reductions in relevant maladies both among medicated (pooled SMD = −2.1, 95% CI = −2.51 to −1.68; z = −9.979, p = 0.000; I2 = 94.8%) as well as non-medicated persons (pooled SMD = −1.03, 95% CI = −1.27 to −0.78; z = −8.283, p = 0.000; I2 = 64.5%), with enhanced response superiority through co-medication. The dose-response relationship between accumulative circadian stimulus (considered as light dose for the circadian system) and disease reduction has been specified by meta-regression and dose-response quantification based on CLA and CSt,f models, indicating accumulative 32–58 days (1,000–1,500 min) as saturation, considering co-medication, severity, study design, etc., are all dose-response influencing factors. It is advised that for the treatment of depression in adolescents and young adults, using current common light therapy devices for “circadian” light therapy (0.1 < CSt,f < 0.7), an accumulative duration of 1,000–1,500 min (5–7 weeks/32–58 days) may be effective. However, for co-medicated patients, the effect size may reach saturation in about 900–1,000 min (32–42 days), while for non-medicated, depressed individuals, it may take 1,100–1,500 min (48–58 days) to reach saturation. It is also possible that an accumulative duration of more than 1,500 min may not be as efficacious on a high CSt,f basis. Overall, the study has provided quantified references for light patterns and neural responses that are vital in the neuropsychological mechanism of light intervention, as well as guidance for clinical application.
Author contributions
RC: Conceptualization, Data curation, Methodology, Software, Writing – original draft, Writing – review & editing. YY: Conceptualization, Supervision, Writing – original draft, Writing – review & editing. XC: Data curation, Writing – original draft, Writing – review & editing.
Funding
The author(s) declare financial support was received for the research, authorship, and/or publication of this article. This research was supported by the program for the National Natural Science Foundation of China (Grant Nos. 51978097 and 51778081).
Acknowledgments
The authors would like to thank Baojie He from Chongqing University for advice on this review.
Conflict of interest
The authors declare that the research was conducted in the absence of any commercial or financial relationships that could be construed as a potential conflict of interest.
Publisher's note
All claims expressed in this article are solely those of the authors and do not necessarily represent those of their affiliated organizations, or those of the publisher, the editors and the reviewers. Any product that may be evaluated in this article, or claim that may be made by its manufacturer, is not guaranteed or endorsed by the publisher.
Supplementary material
The Supplementary Material for this article can be found online at: https://www.frontiersin.org/articles/10.3389/fpubh.2023.1257093/full#supplementary-material
References
1. Zhou L, Hou D, Wang Y, Zhou S, Lin Y. High circadian stimulus lighting therapy for depression: Meta-analysis of clinical trials. Front Neurosci. (2022) 16:975576. doi: 10.3389/fnins.2022.975576
2. Rea MS. A guide for characterizing and prescribing light therapy devices. In: Auger, editor. Circadian Rhythm Sleep-Wake Disorders: An Evidence-Based Guide for Clinicians and Investigators. Cham: Springer (2020). p. 207–19.
3. Do A, Li VW, Huang S, Michalak EE, Tam EM, Chakrabarty T, et al. Blue-light therapy for seasonal and non-seasonal depression: a systematic review and meta-analysis of randomized controlled trials. Can J Psychiatry. (2022) 67:745–54. doi: 10.1177/07067437221097903
4. Geoffroy PA, Schroder CM, Reynaud E, Bourgin P. Efficacy of light therapy versus antidepressant drugs, and of the combination versus monotherapy, in major depressive episodes: a systematic review and meta-analysis. Sleep Med Rev. (2019) 48:101213. doi: 10.1016/j.smrv.2019.101213
5. Nguyen C, Murray G, Anderson S, Filipowicz A, Ingram KK. In vivo molecular chronotyping, circadian misalignment, and high rates of depression in young adults. J Affect Disord. (2019) 250:425–31. doi: 10.1016/j.jad.2019.03.050
6. Haraden DA, Mullin BC, Hankin BL. Internalizing symptoms and chronotype in youth: a longitudinal assessment of anxiety, depression, and tripartite model. Psychiat Res. (2019) 272:797–805. doi: 10.1016/j.psychres.2018.12.117
7. Faria AD, de Azevedo Cardoso T, Mondin TC, de Mattos Souza LD, da Silva Magalhaes PV, Zeni CP, et al. Biological rhythms in bipolar and depressive disorders: a community study with drug-na ive young adults. J Affect Disord. (2015) 186:145–8. doi: 10.1016/j.jad.2015.07.004
8. Mondin TC, de Azevedo Cardoso T, de Mattos Souza LD, Jansen K, da Silva Magalh aes PV, Kapczinski F, et al. Mood disorders and biological rhythms in young adults: a large population-based study. J Psychiatr Res. (2017) 84:98–104. doi: 10.1016/j.jpsychires.2016.09.030
9. Robillard R, Carpenter JS, Rogers NL, Fares S, Grierson AB, Hermens DF, et al. Circadian rhythms and psychiatric profiles in young adults with unipolar depressive disorders. Ttansl Psychiat. (2018) 8:213. doi: 10.1038/s41398-018-0255-y
10. Sivertsen B, Harvey AG, Pallesen S, Hysing M. Mental health problems in adolescents with delayed sleep phase: results from a large population-based study in Norway. J Sleep Res. (2015) 24:11–8. doi: 10.1111/jsr.12254
11. Crouse JJ, Carpenter JS, Song YJC, Hockey SJ, Naismith SL, Grunstein RR, et al. Circadian rhythm sleep–wake disturbances and depression in young people: implications for prevention and early intervention. Lancet Psychiatry. (2021) 8:813–23. doi: 10.1016/S2215-0366(21)00034-1
12. Burish MJ, Chen Z, Yoo SH. Emerging relevance of circadian rhythms in headaches and neuropathic pain. Acta Physiologica. (2019) 225:e13161. doi: 10.1111/apha.13161
13. Parry BL. Optimal management of perimenopausal depression. Int J Womens Health. (2010) 143–51. doi: 10.2147/IJWH.S7155
14. Lam RW, Levitan RD. Pathophysiology of seasonal affective disorder: a review. J Psychiatry Neurosci. (2000) 25:469–80.
15. Meyerhoff J, Young MA, Rohan KJ. Patterns of depressive symptom remission during the treatment of seasonal affective disorder with cognitive-behavioral therapy or light therapy. Depress Anxiety. (2018) 35:457–67. doi: 10.1002/da.22739
16. Al-Karawi D, Jubair L. Bright light therapy for nonseasonal depression: meta-analysis of clinical trials. J Affect Disord. (2016) 198:64–71. doi: 10.1016/j.jad.2016.03.016
17. Wang S, Zhang Z, Yao L, Ding N, Jiang L, Wu Y. Bright light therapy in the treatment of patients with bipolar disorder: a systematic review and meta-analysis. PLoS ONE. (2020) 15:e0232798. doi: 10.1371/journal.pone.0232798
18. Lam RW, Levitt AJ, Levitan RD, Michalak EE, Cheung AH, Morehouse R, et al. Efficacy of bright light treatment, fluoxetine, and the combination in patients with nonseasonal major depressive disorder: a randomized clinical trial. JAMA Psychiatry. (2016) 73:56–63. doi: 10.1001/jamapsychiatry.2015.2235
19. Niederhofer H. Stabilization of circadian rhythm, its augmentation by bright light treatment and its importance for ADHD and depression of adolescents. Neurosci Med. (2013) 4:150–4. doi: 10.4236/nm.2013.43024
20. Lin F, Su Y, Weng Y, Lin X, Weng H, Cai G, et al. The effects of bright light therapy on depression and sleep disturbances in patients with Parkinson's disease: a systematic review and meta-analysis of randomized controlled trials. Sleep Med. (2021) 83:280–9. doi: 10.1016/j.sleep.2021.03.035
21. Roccaro I, Smirni D. Fiat lux: the light became therapy. An overview on the bright light therapy in Alzheimer's disease sleep disorders. J Alzheimers Dis. (2020) 77:113–25. doi: 10.3233/JAD-200478
22. Epperson CN, Terman M, Terman JS, Hanusa BH, Oren DA, Peindl KS, et al. Randomized clinical trial of bright light therapy for antepartum depression: preliminary findings. J Clin Psychiatry. (2004) 65:421–5. doi: 10.4088/JCP.v65n0319
23. Auger RR, Burgess HJ, Emens JS, Deriy LV, Thomas SM, Sharkey KM. Clinical practice guideline for the treatment of intrinsic circadian rhythm sleep-wake disorders: advanced sleep-wake phase disorder (ASWPD), delayed sleep-wake phase disorder (DSWPD), non-24-hour sleep-wake rhythm disorder (N24SWD), and irregular sleep-wake rhythm disorder (ISWRD). An update for (2015: an American Academy of Sleep Medicine clinical practice guideline. J Clin Sleep Med. (2015) 11:1199–236. doi: 10.5664/jcsm.5100
24. Hurd D, Herrera M, Brant JM, Coombs NC, Arzubi E. Prospective, open trial of adjunctive triple chronotherapy for the acute treatment of depression in adolescent inpatients. J Child Adolesc Psychopharmacol. (2019) 29:20–7. doi: 10.1089/cap.2018.0063
25. Even C, Schr oder CM, Friedman S, Rouillon F. Efficacy of light therapy in nonseasonal depression: a systematic review. J Affect Disord. (2008) 108:11–23. doi: 10.1016/j.jad.2007.09.008
26. Terman M, Terman JS. Controlled trial of naturalistic dawn simulation and negative air ionization for seasonal affective disorder. Am J Psychiatry. (2006) 163:2126–33. doi: 10.1176/ajp.2006.163.12.2126
27. Alotaibi MA, Halaki M, Chow CM. A systematic review of light therapy on mood scores in major depressive disorder: light specification, dose, timing and delivery. Int J Basic Appl Sci. (2016) 5:30–7. doi: 10.14419/ijbas.v5i1.5456
28. Geoffroy PA, Maruani J, Etain B, Lejoyeux M, Amad A, Courtet P, et al. Bright light therapy in the morning or at mid-day in the treatment of non-seasonal bipolar depressive episodes (LuBi): study protocol for a dose research phase I/II trial. Psychiatry Investig. (2018) 15:1188. doi: 10.30773/pi.2018.09.27.1
29. Janas-Kozik M, Krzystanek M, Stachowicz M, Krupka-Matuszczyk I, Janas A, Rybakowski JK. Bright light treatment of depressive symptoms in patients with restrictive type of anorexia nervosa. J Affect Disord. (2011) 130:462–5. doi: 10.1016/j.jad.2010.10.014
30. Donmez M, Yorguner N, Kora K, Topcuoglu V. Efficacy of bright light therapy in perinatal depression: a randomized, double-blind, placebo-controlled study. J Psychiatr Res. (2022) 149:315–22. doi: 10.1016/j.jpsychires.2022.02.027
31. Jiang L, Zhang S, Wang Y, So KF, Ren C, Tao Q. Efficacy of light therapy for a college student sample with non-seasonal subthreshold depression: an RCT study. J Affect Disord. (2020) 277:443–9. doi: 10.1016/j.jad.2020.08.055
32. Rea MS, Nagare R, Figueiro MG. Predictions of melatonin suppression during the early biological night and their implications for residential light exposures prior to sleeping. Sci Rep. (2020) 10:14114. doi: 10.1038/s41598-020-70619-5
33. Daneault V, Dumont M, Masse E, Vandewalle G, Carrier J. Light-sensitive brain pathways and aging. J Physiol Anthropol. (2016) 35:1–12. doi: 10.1186/s40101-016-0091-9
34. Stephenson KM, Schroder CM, Bertschy G, Bourgin P. Complex interaction of circadian and non-circadian effects of light on mood: shedding new light on an old story. Sleep Med Rev. (2012) 16:445–54. doi: 10.1016/j.smrv.2011.09.002
35. Boivin DB, Czeisler CA, Dijk DJ, Duffy JF, Folkard S, Minors DS, et al. Complex interaction of the sleep-wake cycle and circadian phase modulates mood in healthy subjects. Arch Gen Psychiatry. (1997) 54:145–52. doi: 10.1001/archpsyc.1997.01830140055010
36. Oldham MA, Ciraulo DA. Bright light therapy for depression: a review of its effects on chronobiology and the autonomic nervous system. Chronobiol Int. (2014) 31:305–19. doi: 10.3109/07420528.2013.833935
37. Lucas RJ, Peirson SN, Berson DM, Brown TM, Cooper HM, Czeisler CA, et al. Measuring and using light in the melanopsin age. Trends Neurosci. (2014) 37:1–9. doi: 10.1016/j.tins.2013.10.004
38. Cie S. 026/E: 2018 CIE system for metrology of optical radiation for ipRGC-influenced responses to light. Color Res Appl. (2018) 44:316–316. doi: 10.1002/col.22350
39. Brown TM, Thapan K, Arendt J, Revell VL, Skene DJ. S-cone contribution to the acute melatonin suppression response in humans. J Pineal Res. (2021) 71:e12719. doi: 10.1111/jpi.12719
40. CIE. Fundamental chromaticity diagram with physiological axes – Part 1. Technical Report 170-1. Vienna: Central Bureau of the Commission Internationale de l' Éclairage (2006).
41. Anderson JL, Glod CA Dai J, Cao Y, Lockley SW. Lux vs. wavelength in light treatment of seasonal affective disorder. Acta Psychiatr Scand. (2009) 120:203–12. doi: 10.1111/j.1600-0447.2009.01345.x
42. Rea MS, Nagare R, Figueiro MG. Modeling circadian phototransduction: Quantitative predictions of psychophysical data. Front Neurosci. (2021) 15:615322. doi: 10.3389/fnins.2021.615322
43. Rea MS, Nagare R, Figueiro MG. Modeling circadian phototransduction: retinal neurophysiology and neuroanatomy. Front Neurosci. (2021) 14:615305. doi: 10.3389/fnins.2020.615305
44. Tsai JW, Hannibal J, Hagiwara G, Colas D, Ruppert E, Ruby NF, et al. Melanopsin as a sleep modulator: circadian gating of the direct effects of light on sleep and altered sleep homeostasis in Opn4-/- mice. PLoS Biol. (2009) 7:e1000125. doi: 10.1371/journal.pbio.1000125
45. Maruani J, Geoffroy PA. Multi-Level processes and retina–brain pathways of photic regulation of mood. J Clin Med. (2022) 11:448. doi: 10.3390/jcm11020448
46. Chen Y, Chen T, Cai X. Light-sensitive circuits related to emotional processing underlie the antidepressant neural targets of light therapy. Behav Brain Res. (2021) 396:112862. doi: 10.1016/j.bbr.2020.112862
47. Dong Y, Hu H. Taming the “Black Dog” by light: a retina-habenula circuit mechanism unveiled. Neuron. (2019) 102:3–5. doi: 10.1016/j.neuron.2019.02.033
48. Huang L, Xi Y, Peng Y, Yang Y, Huang X, Fu Y, et al. A visual circuit related to habenula underlies the antidepressive effects of light therapy. Neuron. (2019) 102:128–42. doi: 10.1016/j.neuron.2019.01.037
49. Fiori LM, Kos A, Lin R, Th eroux JF, Lopez JP, K uhne C, et al. miR-323a regulates ERBB4 and is involved in depression. Mol Psychiatry. (2021) 26:4191–4204. doi: 10.1038/s41380-020-00953-7
50. Kennard BD, Silva SG, Tonev S, Rohde P, Hughes JL, Vitiello B, et al. Remission and recovery in the Treatment for Adolescents with Depression Study (TADS): acute and long-term outcomes. J Am Acad Child Adolesc Psychiatry. (2009) 48:186–95. doi: 10.1097/CHI.0b013e31819176f9
51. Mayer JS, Hees K, Medda J, Grimm O, Asherson P, Bellina M, et al. Bright light therapy versus physical exercise to prevent co-morbid depression and obesity in adolescents and young adults with attention-deficit/hyperactivity disorder: study protocol for a randomized controlled trial. Trials. (2018) 19:1–19. doi: 10.1186/s13063-017-2426-1
52. Curry J, Silva S, Rohde P, Ginsburg G, Kratochvil C, Simons A, et al. Recovery and recurrence following treatment for adolescent major depression. Arch Gen Psychiatry. (2011) 68:263–9. doi: 10.1001/archgenpsychiatry.2010.150
53. Robillard R, Hermens DF, Naismith SL, White D, Rogers NL, Ip TK, et al. Ambulatory sleep-wake patterns and variability in young people with emerging mental disorders. J Psychiatry Neurosci. (2015) 40:28–37. doi: 10.1503/jpn.130247
54. Ballard R, Parkhurst J, Julian K, Pasetes LN, Fawcett A, Li A, et al. Light Therapy for Adolescent Depression: A Scoping Review. Curr Psychiatry Rep. (2023) 25:373–86. doi: 10.1007/s11920-023-01437-5
55. Norren DV, Vos JJ. Spectral transmission of the human ocular media. Vision Res. (1974) 14:1237–44. doi: 10.1016/0042-6989(74)90222-3
56. Nagare R, Rea MS, Plitnick B, Figueiro MG. Nocturnal melatonin suppression by adolescents and adults for different levels, spectra, and durations of light exposure. J Biol Rhythms. (2019) 34:178–94. doi: 10.1177/0748730419828056
57. Eto T, Ohashi M, Nagata K, Shin N, Motomura Y, Higuchi S. Crystalline lens transmittance spectra and pupil sizes as factors affecting light-induced melatonin suppression in children and adults. Ophthalmic Physiol Opt. (2021) 41:900–10. doi: 10.1111/opo.12809
58. Gibson EM, Williams WP III, Kriegsfeld LJ. Aging in the circadian system: considerations for health, disease prevention and longevity. Exp Gerontol. (2009) 44:51–6. doi: 10.1016/j.exger.2008.05.007
59. Kessler BA, Stanley EM, Frederick-Duus D, Fadel J. Age-related loss of orexin/hypocretin neurons. Neuroscience. (2011) 178:82–8. doi: 10.1016/j.neuroscience.2011.01.031
60. Liberati A, Altman DG, Tetzlaff J, Mulrow C, Gøtzsche PC, Ioannidis JP, et al. The PRISMA statement for reporting systematic reviews and meta-analyses of studies that evaluate health care interventions: explanation and elaboration. Ann Intern Med. (2009) 151:65. doi: 10.7326/0003-4819-151-4-200908180-00136
61. Smarr KL, Keefer AL. Measures of depression and depressive symptoms: Beck depression Inventory-II (BDI-II), center for epidemiologic studies depression scale (CES-D), geriatric depression scale (GDS), hospital anxiety and depression scale (HADS), and patient health Questionnaire-9 (PHQ-9). Arthritis Care Res. (2011) 63:S454–66. doi: 10.1002/acr.20556
62. House LA, Walton B. The effectiveness of light therapy for college student depression. J College Stud Psychother. (2018) 32:42–52. doi: 10.1080/87568225.2017.1321975
63. Huang LB, Tsai MC, Chen CY, Hsu SC. The effectiveness of light/dark exposure to treat insomnia in female nurses undertaking shift work during the evening/night shift. J Clin Sleep Med. (2013) 9:641–6. doi: 10.5664/jcsm.2824
64. Kirschbaum-Lesch I, Gest S, Legenbauer T, Holtmann M. Feasibility and efficacy of bright light therapy in depressed adolescent inpatients. Zeitschrift Kinder Jugendpsychiatrie Psychotherapie. (2018) 46:423–9. doi: 10.1024/1422-4917/a000603
65. Danielsson K, Jansson-Fr ojmark M, Broman JE, Markstrom A. Cognitive behavioral therapy as an adjunct treatment to light therapy for delayed sleep phase disorder in young adults: a randomized controlled feasibility study. Behav Sleep Med. (2016) 14:212–32. doi: 10.1080/15402002.2014.981817
66. Gest S, Holtmann M, Bogen S, Schulz C, Pniewski B, Legenbauer T. Chronotherapeutic treatments for depression in youth. Eur Child Adolesc Psychiatry. (2016) 25:151–61. doi: 10.1007/s00787-015-0720-6
67. Kopp BT, Hayes Jr D, Ghera P, Patel A, Kirkby S, Kowatch RA, et al. Pilot trial of light therapy for depression in hospitalized patients with cystic fibrosis. J Affect Disord. (2016) 189:164–8. doi: 10.1016/j.jad.2015.08.056
68. LaRosa KN, MacArthur E, Wang F, Zhang H, Pan H, Brigden J, et al. Light therapy for QoL/Depression in AYA with cancer: a randomized trial. J Pediatr Psychol. (2022) 47:306–17. doi: 10.1093/jpepsy/jsab098
69. Ricketts EJ, Burgess HJ, Montalbano GE, Coles ME, McGuire JF, Thamrin H, et al. Morning light therapy in adults with Tourette's disorder. J Neurol. (2022) 269:1–12. doi: 10.1007/s00415-021-10645-z
70. Braun DL, Sunday SR, Fornari VM, Halmi KA. Bright light therapy decreases winter binge frequency in women with bulimia nervosa: a double-blind, placebo-controlled study. Compr Psychiatry. (1999) 40:442–8. doi: 10.1016/S0010-440X(99)90088-3
71. Blouin AG, Blouin JH, Iversen H, Carter J, Goldstein C, Goldfield G, et al. Light therapy in bulimia nervosa: a double-blind, placebo-controlled study. Psychiatry Res. (1996) 60:1–9. doi: 10.1016/0165-1781(95)02532-4
72. Bromundt V, Wirz-Justice A, Kyburz S, Opwis K, Dammann G, Cajochen C. Circadian sleep-wake cycles, well-being, and light therapy in borderline personality disorder. J Pers Disord. (2013) 27:680–96. doi: 10.1521/pedi_2012_26_057
73. Bais B, Kamperman AM, Bijma HH, Hoogendijk WJ, Souman JL, Knijff E, et al. Effects of bright light therapy for depression during pregnancy: a randomised, double-blind controlled trial. BMJ Open. (2020) 10:e038030. doi: 10.1136/bmjopen-2020-038030
74. Swanson LM, Burgess HJ, Zollars J, Todd Arnedt J. An open-label pilot study of a home wearable light therapy device for postpartum depression. Arch Womens Ment Health. (2018) 21:583–6. doi: 10.1007/s00737-018-0836-z
75. Li D, Fang P, Liu H, Chen L, Fu Y, Liu J, et al. The clinical effect of blue light therapy on patients with delayed sleep-wake phase disorder. Nat Sci Sleep. (2022) 14:75. doi: 10.2147/NSS.S344616
76. Richardson C, Gradisar M. Depressed mood and repetitive negative thinking in delayed sleep–wake phase disorder: treatment effects and a comparison with good sleepers. J Sleep Res. (2022) 31:e13452. doi: 10.1111/jsr.13452
77. van Kol M. A MOMENT OF BRIGHTNESS: The effects of brief morning Bright Light Therapy on Burnout Related (master's thesis). Eindhoven: Eindhoven University of Technology (2015).
78. Raikes AC, Dailey NS, Shane BR, Forbeck B, Alkozei A, Killgore WD. Daily morning blue light therapy improves daytime sleepiness, sleep quality, and quality of life following a mild traumatic brain injury. J Head Trauma Rehabil. (2020) 35:E405–21. doi: 10.1097/HTR.0000000000000579
79. Lee SY, Aycock DM, Moloney MF. Bright light therapy to promote sleep in mothers of low-birth-weight infants: a pilot study. Biol Res Nurs. (2013) 15:398–406. doi: 10.1177/1099800412445612
80. Sasseville A, Martin JS, Houle J, H ebert M. Investigating the contribution of short wavelengths in the alerting effect of bright light. Physiol Behav. (2015) 151:81–7. doi: 10.1016/j.physbeh.2015.06.028
81. Goel N, Etwaroo GR. Bright light, negative air ions and auditory stimuli produce rapid mood changes in a student population: a placebo-controlled study. Psychol Med. (2006) 36:1253–63. doi: 10.1017/S0033291706008002
82. Grandner MA. Sleep, Mood, and Circadian Responses to Bright Green Light During Sleep (dissertation/Doctor's thesis). San Diego, CA: University of California, and San Diego State University (2007).
83. Papatheodorou G, Kutcher S. The effect of adjunctive light therapy on ameliorating breakthrough depressive symptoms in adolescent-onset bipolar disorder. J Psychiatry Neurosci. (1995) 20:226.
84. Figueiro MG, Rea MS. Office lighting and personal light exposures in two seasons: impact on sleep and mood. Lighting Res Technol. (2016) 48:352–64. doi: 10.1177/1477153514564098
85. Figueiro MG, Steverson B, Heerwagen J, Kampschroer K, Hunter CM, Gonzales K, et al. The impact of daytime light exposures on sleep and mood in office workers. Sleep Health. (2017) 3:204–15. doi: 10.1016/j.sleh.2017.03.005
86. Higgins JP, Savovi c J, Page MJ, Elbers RG, Sterne JA. Assessing Risk of Bias in a Randomized Trial. Cochrane Handbook for Systematic Reviews of Interventions. Wiley (2019). p. 205–28.
87. Bogen S, Legenbauer T, Gest S, Holtmann M. Lighting the mood of depressed youth: Feasibility and efficacy of a (2 week-placebo controlled bright light treatment for juvenile inpatients. J Affect Disord. (2016) 190:450–6. doi: 10.1016/j.jad.2015.09.026
88. Spezzano MA. The Efficacy of Bright Light Treatment on the Symptoms of Seasonal Affective Disorder on a College-Aged Population (doctor's thesis). Prescott, AR: Northcentral University (2006).
89. Sterne JA, Savovi c J, Page MJ, Elbers RG, Blencowe NS, Boutron I, et al. RoB 2: a revised tool for assessing risk of bias in randomised trials. BMJ. (2019) 366. doi: 10.1136/bmj.l4898
90. Youngstedt SD, Kline CE, Ginsberg JP, Zielinski MR, Hardin JW. Bright light treatment for high-anxious young adults: a randomized controlled pilot study. Depress Anxiety. (2011) 28:324–32. doi: 10.1002/da.20784
91. Smith PF. A note on the advantages of using linear mixed model analysis with maximal likelihood estimation over repeated measures ANOVAs in psychopharmacology: comment on Clark et al. (2012). J Psychopharmacol. (2012) 26:1605–7. doi: 10.1177/0269881112463471
92. Nixon A, Strike MK, Feilds KL, Glozier N, Thatte S, Hickie IB, et al. Temporal dynamics of subjective sleep profiles predicting mood improvements during adjunctive light therapy combined with sleep rescheduling. J Affect Disord Rep. (2021) 4:100106. doi: 10.1016/j.jadr.2021.100106
93. Wyszecki G, Stiles WS. Color Science: Concepts and Methods, Quantitative Data and Formulae. 2nd Edn. New York, NY: John Wiley & Sons (2000).
94. Smith VC, Pokorny J. Spectral sensitivity of the foveal cone photopigments between 400 and 500 nm. Vision Res. (1975) 15:161–71. doi: 10.1016/0042-6989(75)90203-5
95. Snodderly D, Brown P, Delori F, Auran J. The macular pigment. I Absorbance spectra, localization, and discrimination from other yellow pigments in primate retinas. Invest Ophthalmol Vis Sci. (1984) 25:660–73.
96. Roufs JAJ. Light as a True Visual Quantity: Principles of Measurement. Paris: CIE Publication (1978).
97. Flory R, Ametepe J, Bowers B. A randomized, placebo-controlled trial of bright light and high-density negative air ions for treatment of Seasonal Affective Disorder. Psychiatry Res. (2010) 177:101–8. doi: 10.1016/j.psychres.2008.08.011
98. Madsen HØ, Ba-Ali S, Hageman I, Lund-Andersen H, Martiny K. Light therapy for seasonal affective disorder in visual impairment and blindness–a pilot study. Acta Neuropsychiatr. (2021) 33:191–9. doi: 10.1017/neu.2021.6
99. Lam RW, Teng MY, Jung YE, Evans VC, Gottlieb JF, Chakrabarty T, et al. Light therapy for patients with bipolar depression: systematic review and meta-analysis of randomized controlled trials. Can J Psychiatry. (2020) 65:290–300. doi: 10.1177/0706743719892471
100. Naus T, Burger A, Malkoc A, Molendijk M, Haffmans J. Is there a difference in clinical efficacy of bright light therapy for different types of depression? A pilot study. J Affect Disord. (2013) 151:1135–7. doi: 10.1016/j.jad.2013.07.017
101. Chan JW, Lam S, Li SX, Chau SW, Chan S, Chan N, et al. Adjunctive bright light treatment with gradual advance in unipolar major depressive disorder with evening chronotype–A randomized controlled trial. Psychol Med. (2022) 52:1448–57. doi: 10.1017/S0033291720003232
102. Carpenter JS, Crouse JJ, Scott EM, Naismith SL, Wilson C, Scott J, et al. Circadian depression: a mood disorder phenotype. Neurosci Biobehav Rev. (2021) 126:79–101. doi: 10.1016/j.neubiorev.2021.02.045
103. Terman M, Terman JS. Light therapy for seasonal and nonseasonal depression: efficacy, protocol, safety, and side effects. CNS Spectr. (2005) 10:647–63. doi: 10.1017/S1092852900019611
104. Diakoumis AA, ALP I, Aarts MM, Khademagha PP. Characterization of Light Therapy Devices (master's thesis). Eindhoven: Eindhoven University of Technology (2016).
105. Wirz-Justice A. From the basic neuroscience of circadian clock function to light therapy for depression: on the emergence of chronotherapeutics. J Affect Disord. (2009) 116:159. doi: 10.1016/j.jad.2009.04.024
106. Hou D, Lin C, Lin Y. Diurnal circadian lighting accumulation model: a predictor of the human circadian phase shift phenotype. Phenomics. (2022) 2:50–63. doi: 10.1007/s43657-021-00039-6
107. Kuwano N, Kato TA, Mitsuhashi M, Sato-Kasai M, Shimokawa N, Hayakawa K, et al. Neuron-related blood inflammatory markers as an objective evaluation tool for major depressive disorder: an exploratory pilot case-control study. J Affect Disord. (2018) 240:88–98. doi: 10.1016/j.jad.2018.07.040
108. Gadad BS, Jha MK, Czysz A, Furman JL, Mayes TL, Emslie MP, et al. Peripheral biomarkers of major depression and antidepressant treatment response: current knowledge and future outlooks. J Affect Disord. (2018) 233:3–14. doi: 10.1016/j.jad.2017.07.001
Keywords: light therapy, circadian light, circadian stimulus, youth, meta-analysis, dose response
Citation: Chen R, Yan Y and Cheng X (2024) Circadian light therapy and light dose for depressed young people: a systematic review and meta-analysis. Front. Public Health 11:1257093. doi: 10.3389/fpubh.2023.1257093
Received: 12 July 2023; Accepted: 06 December 2023;
Published: 08 January 2024.
Edited by:
Gabriele Nibbio, University of Brescia, ItalyReviewed by:
Antje Buettner-Teleaga, Woosuk University, Republic of KoreaLi Zhou, Fudan University, China
Copyright © 2024 Chen, Yan and Cheng. This is an open-access article distributed under the terms of the Creative Commons Attribution License (CC BY). The use, distribution or reproduction in other forums is permitted, provided the original author(s) and the copyright owner(s) are credited and that the original publication in this journal is cited, in accordance with accepted academic practice. No use, distribution or reproduction is permitted which does not comply with these terms.
*Correspondence: Yonghong Yan, eWFueW9uZ2hvbmdAY3F1LmVkdS5jbg==