- 1Department of Medicine, Faculty of Medicine, Autonomous University of Madrid, Madrid, Spain
- 2Intensive Care Unit, La Paz University Hospital, Madrid, Spain
- 3Institute for Health Research (idiPAZ), La Paz University Hospital, Madrid, Spain
- 4Department of Neurosurgery, Ospedale Policlinico San Martino, Istituto di Ricovero eCura a Carattere Scientifico (IRCCS) for Oncology and Neuroscience, Genoa, Italy
- 5Department of Nursing, University of La Rioja, Logroño, Spain
- 6Health and Healthcare Research Group (GRUPAC), Faculty of Health Sciences, University of La Rioja, Logroño, Spain
- 7Department of Nursing and Physiatry, Faculty of Health Sciences, University of Zaragoza, Zaragoza, Spain
- 8Aragon Healthcare Service, Aragon, Zaragoza, Spain
- 9Faculty of Medicine, University of Salamanca, Salamanca, Spain
- 10Intensive Care Unit, Valencia University Clinical Hospital, Valencia, Spain
- 11Intensive Care Unit, Germans Trias i Pujol University Hospital, Badalona, Spain
- 12Intensive Care Unit, Burgos University Hospital, Burgos, Spain
- 13Emergency Department, University Hospital of Salamanca, Salamanca, Spain
- 14Critical Care Department, Neurointensive Care Unit, Sanatorio Pasteur, Catamarca, Argentina
Introduction: Coagulopathy associated with isolated traumatic brain injury (C-iTBI) is a frequent complication associated with poor outcomes, primarily due to its role in the development or progression of haemorrhagic brain lesions. The independent risk factors for its onset are age, severity of traumatic brain injury (TBI), volume of fluids administered during resuscitation, and pre-injury use of antithrombotic drugs. Although the pathophysiology of C-iTBI has not been fully elucidated, two distinct stages have been identified: an initial hypocoagulable phase that begins within the first 24 h, dominated by platelet dysfunction and hyperfibrinolysis, followed by a hypercoagulable state that generally starts 72 h after the trauma. The aim of this study was to design an acronym as a mnemonic device to provide clinicians with an auxiliary tool in the treatment of this complication.
Methods: A narrative analysis was performed in which intensive care physicians were asked to list the key factors related to C-iTBI. The initial sample was comprised of 33 respondents. Respondents who were not physicians, not currently working in or with experience in coagulopathy were excluded. Interviews were conducted for a month until the sample was saturated. Each participant was asked a single question: Can you identify a factor associated with coagulopathy in patients with TBI? Factors identified by respondents were then submitted to a quality check based on published studies and proven evidence. Because all the factors identified had strong support in the literature, none was eliminated. An acronym was then developed to create the mnemonic device.
Results and conclusion: Eleven factors were identified: cerebral computed tomography, oral anticoagulant & antiplatelet use, arterial blood pressure (Hypotension), goal-directed haemostatic therapy, use fluids cautiously, low calcium levels, anaemia-transfusion, temperature, international normalised ratio (INR), oral antithrombotic reversal, normal acid–base status, forming the acronym “Coagulation.” This acronym is a simple mnemonic device, easy to apply for anyone facing the challenge of treating patients of moderate or severe TBI on a daily basis.
1 Specific aims and theoretical grounds of the study
A narrative approach involves pluralism, relativism, and subjectivity. Despite advances in the care of neurocritically ill patients from the prehospital stage through rehabilitation, traumatic brain injury (TBI) continues to be a pathological entity associated with significant rates of functional disability and mortality (1). Narratives have classically been a mode of transmitting culture on macro-societal levels, and a way to create evidence. Irrespective of the mechanism of injury, TBI is understood to consist of two clearly defined processes (1–4). The primary injury depends on the type, location, and amount of energy absorbed by the structures that comprise the cranial cavity and the results of focal (intra or extra-axial haemorrhages) or diffuse (axonal damage) lesions. These lesions, in turn, trigger different and multiple neurotoxic cascades, most notably, the inflammatory and coagulation cascades that negatively influence haemodynamics, oxygenation, and cerebral energy metabolism (1–4). Secondary injury involves a series of phenomena that can originate within the skull (intracranial hypertension, cerebral hypoxia) or systemically (hypotension, hypoxemia, sodium, and glycaemic alterations) which combine to perpetuate or exacerbate the primary injury (1–4). While primary injuries are irreversible, secondary injuries can be prevented and corrected, and this is precisely one of the pillars upon which the modern management of severe TBI is based (1–4).
Normal haemostasis involves a delicate balance between mechanisms that promote bleeding and those that try to prevent it, a balance that can be disrupted following TBI. Coagulation disorders in severe isolated TBI (C-iTBI) are common and contribute to secondary damage, mainly due to their role in facilitating the development or progression of both ischemic and haemorrhagic lesions (5–10). TBI has increasingly come to affect older patients who often present with polypharmacy including antithrombotics and anticoagulants due to multiple comorbidities (11). The incidence of C-iTBI as reported in the literature averages 33%, with figures ranging from 7 to 90%. The disparities in results may be due to differences in study design, lack of uniform definition, varied cohorts, points of the parameters used to define it, concomitant presence of polytrauma, or the time it was analysed (5–10, 12). Nonetheless, based on these figures, we can posit that approximately one of three patients will develop C-iTBI, strongly suggesting its origin in the brain itself (7–10, 12).
The pathophysiology of C-iTBI has not yet been fully and accurately elucidated; however, current evidence indicates that it is a primary haemostasis disorder broadly characterised by an early hypocoagulable period, where platelet dysfunction and hyperfibrinolysis predominate, and a later prothrombotic stage, where the described mechanisms reverse their role (12). By platelet dysfunction, we understand the generic and non-specific term conventionally used to denote alterations in platelet physiology. In the context of trauma, this may either involve a decrease in number (thrombocytopenia) or function, i.e., alteration of one of the steps in which platelets are actively involved (adhesion, aggregation, and secretion). Such alterations may occur directly secondary to traumatic injury or as a consequence of pre-existing disease or prior use of antithrombotic medications. The incidence of platelet dysfunction ranges from less than 1% in mild TBI to >60% in severe TBI.
Predisposing factors identified for the development of C-iTBI include the severity of the trauma (GCS <9), age (> 75 years), previous fluid therapy (> 2 L), base excess (< −6), hypothermia, arterial hypotension, and prior use of anticoagulant or antiplatelet agents (12, 13). The presence of C-iTBI adds predictive power to validated prognostic scales (14, 15). C-iTBI is associated with poor outcomes in terms of functionality and mortality, which explains why this factor is a key therapeutic target to consider (7–10, 12–16). The real implications that early detection and correction of coagulation disorders have in the context of severe TBI remain unknown, however, they open up a wide range of future research possibilities.
Healthcare providers communicate the events of treating a difficult case through narratives. Narratives are also used to concretize a body of knowledge in specific contexts, as in the case of intensive care medicine. Stories encapsulate professional experiences to be shared with students in practise, or advanced professionals. In this study, we used the narrative experience of intensive care physicians to design a mnemonic device we called “Coagulation” to provide clinicians with an auxiliary tool in the treatment of C-iTBI.
2 Methods
To carry out this study based on narrative, the authors of the manuscript have selected the best possible evidence on the topic under study. Once selected, it was debated until the variables that were considered most important were obtained. Three rounds were carried out until the selection of variables began to be repeated and we considered that the selection was saturated, something that in qualitative methodology indicates the end of the selection process. The initial sample was comprised of 33 respondents. Respondents who were not physicians, not currently working in or with experience in coagulopathy were excluded. The process and the meeting to select variables were conducted for a month until the sample was saturated. In this meeting, all participants have been arranged to make the mnemonic rule more easily understandable.
Each participant was asked a single question: Can you identify a factor associated with coagulopathy in patients with TBI? The respondents identified specific concepts during the interviews which were then collected and mapped. Following the initial reading and validation, we consulted the literature to justify the factors identified.
Ultimately, we identified 11 factors.
The factors identified by the respondents were submitted to a quality check, based on the published literature and proven evidence. Because all the factors identified had strong support in the literature, none was eliminated.
3 Results
3.1 Factor 1: cerebral computed tomography was identified by participants as “Essential to the classification of severity and monitoring”
Cerebral computed tomography (CT) is the neuroimaging of choice during the acute phase of severe TBI (17–19). In addition to being widely available, it is cost-effective, has a short radiation exposure time and can even be performed on patients with prostheses or on mechanical ventilation (17–19). The CT scan allows for the simultaneous evaluation of other body regions and is an essential complementary tool to categorize TBI (17–19). It provides an objective assessment of the type and extension of lesions, their volume, location, and impact on intracranial anatomical structures (18–20). It is highly sensitive for the detection of blood collections (17, 18, 21). Finally, it yields pathophysiological information and has a proven predictive capacity for both intracranial hypertension and outcome (20, 22, 23).
Different CT scales have been developed and validated in the clinical field (20, 23–26). Its serial determination allows clinicians to monitor the progression of the broad spectrum of lesions following TBI (17–20, 22–26). Therefore, CT plays an important role in diagnosis, evaluation of lesion progression, and therapeutic decision-making in the context of C-iTBI (Figure 1).
3.2 Factor 2: oral anticoagulant and antiplatelet use was identified by participants as “High Alert”
The increase in life expectancy over the last decades has led to an increasingly older adult population with associated pathologies, mainly cardiac and cerebrovascular, that require the use of oral anticoagulants (OAC) or antiplatelet agents (APA). The global incidence of cerebral bleeding secondary to OAC/APA utilisation is 20% (12). Specifically, clinical studies have shown that the use of warfarin before iTBI doubles the possibility of poor outcomes (27), while pre-injury use of APAs increases the risk of presenting post-traumatic cerebral haemorrhage (28). This risk is increased even further with the use of second-generation APAs (clopidogrel) in individuals with mild TBI (29). Regarding the new oral anticoagulants (NOAC), the few prospective clinical studies conducted on iTBI have had conflicting results, making it difficult to draw valid conclusions (30, 31), however a retrospective study found increased hematoma expansion in individuals with pre-injury use of NOACs compared to warfarin (32). Recently published data from the Collaborative European NeuroTrauma Effectiveness Research in Traumatic Brain Injury (CENTER-TBI) corroborate the aforementioned epidemiological data, indicating that prior use of OACs/APAs increases the risk of development or progression of haemorrhagic lesions, with a 3-fold higher mortality rate and greater frequency of poor outcomes at 6 months after iTBI, especially in individuals taking vitamin K antagonists (12, 13). Mathieu et al. (33) serially and comparatively analysed CT scans in iTBI patients who used antithrombotic agents (APAs or OACs) pre-injury vs. those who did not. In the group with prior antithrombotic use, an increase in the volume of extra-axial hematomas was observed, as well as an increase in the rate of haematoma expansion and the risk of developing delayed traumatic intracerebral haemorrhages (33). A Swedish study evaluating the risk of bleeding in individuals with prior use of OACs vs. APAs and iTBI secondary to ground-level falls found an increased risk of bleeding in the group that had used APAs (34). Finally, a recent meta-analysis (low quality of the included studies) that sought to establish the adverse effects of pre-iTBI APA use, found associations between dual therapy and the progression of lesions (OR 2.81; 95% CI 1.19–6.65; p = 002) and need for neurosurgery (OR 1.61; 95% CI 1.15–2.28) but no related impact on hospital mortality (35).
3.3 Factor 3: arterial blood pressure (hypotension) was defined by participants as “the most dreaded latent threat for the traumatised brain”
Maintaining cerebral blood flow (CBF), one of the primary goals in the management of TBI, is usually associated with the metabolic rate of oxygen (CMRO2) (1, 4, 17). Its key determinants are cerebral perfusion pressure (CPP) and the diameter of resistance arterioles (50–150 μm) (1, 4). CPP is the result of the difference between mean arterial pressure (MAP) and intracranial pressure (ICP) (1, 4).
The CBF remains normal and stable through the intrinsic capacity of its resistance vessels to modify their diameter, a phenomenon called “cerebral autoregulation” (CAR). The change in diameter is due to different stimuli, including MAP, arterial partial pressure of oxygen (PaO2), arterial partial pressure of carbon dioxide (PaCO2), and others of neural origin (1). CAR is a natural survival mechanism that is not infinite; on the contrary, it typically functions within certain CPP levels ranging from 50 to 150 mmHg. Above or below these limits, the CBF passively follows changes in MAP (1, 17).
During TBI, however, this mechanism is altered, and the limits narrow and shift to the right. Therefore, during injury, higher levels of CPP are necessary to maintain adequate levels of CBF (17, 21). When CAR is disrupted or completely lost, even temporarily, arterial hypotension is deleterious as it causes a decrease in CBF and ischemic hypoxia with irreversible and devastating consequences (1, 17).
The traumatised brain does not cause arterial hypotension per se and it should not always be attributed to hypovolaemia, which is why its cause should be thoroughly investigated (36). Arterial hypotension independently increases the possibility of poor outcomes (37, 38) and is a risk factor for the development of C-iTBI (12, 13).
3.4 Factor 4: goal-directed haemostatic therapy was defined by participants as a factor in which “Knowledge of pathophysiology is essential”
Goal-directed haemostatic therapy (GDHT) is an element of personalised precision medicine. This approach focuses on the specific point problem a given pathology can cause, considering the overall context at all times. In order to do so, monitoring systems capable of promptly alerting healthcare professionals to any alterations that threaten physiological homeostasis are essential. At the same time, in-depth knowledge of the pathophysiology of the disorder is necessary for the correct interpretation, analysis, and decision-making based on the information such monitoring provides.
The coagulation system is finely tuned process, continuously balancing the mechanisms that promote and prevent clot formation. C-iTBI, a multifactorial entity different from coagulopathy secondary to multisystem trauma without TBI, occurs when these mechanisms are disrupted (6–10, 39). While its pathophysiology has yet to be accurately defined, C-iTBI generally begins within 24 h of admission; the more severe the TBI, the earlier it manifests (40). Its duration is variable, averaging 72 h, although this period is sometimes prolonged (40). C-iTBI is characterised by an initial hypocoagulable state consisting of platelet dysfunction, increased consumption of platelets and coagulation factors, disseminated intravascular coagulation (DIC), and hyperfibrinolysis, followed by a hypercoagulable, prothrombotic state, both local (cerebral microcirculation-ischemic lesions) and systemic (deep vein thrombosis). However, these states are linked and often it is difficult to differentiate one from the other (6–10, 39).
Although discussion of these mechanisms is beyond the scope of this manuscript, it is worth noting that multiple mediators and cascades are involved in the genesis of C-iTBI, including protein C, protein S, thromboxane, prostaglandins, adenosine, and brain tissue factor (6, 10, 39).
3.5 Factor 5: use fluids cautiously was defined by participants as a factor in which “Type and volume matter”
Fluid administration is one of the key elements in the initial resuscitation of TBI, especially when necessary to correct arterial hypotension and maintain adequate CPP values (41). Recent guidelines recommend 0.9% normal saline as the fluid of choice (41). Some considerations are necessary in this context such as avoiding hypotonic solutions (dextrose 5%, lactated ringer, albumin 4%) and the use of colloids (41). In terms of the implications that fluids have on coagulation, both isotonic crystalloids and colloid solutions provoke haemodilution, causing a decrease in the function and number of platelets and plasma factors involved in coagulation (42–44). This effect is directly proportional to the volume infused (42–44). Available evidence indicates that 0.9% saline at low doses does not affect coagulation (45) or has a minimal hypercoagulable effect, while at higher doses the effect is the opposite (hypocoagulable), associated with increased bleeding (42–44, 46–49). Infusions of more than 2,000 mL of fluids have been identified as an independent risk factor for the development of C-iTBI (51). Because the osmotically active solutions used for the control of intracranial hypertension such as hypertonic saline or mannitol are crystalloids, they retain their dose-dependent haemodilution capacity and cause hypocoagulable states (42–44). However, clinical studies have shown that neither 20% mannitol nor 3.5% saline affect coagulation at equimolar doses and limited infusion volumes during neurosurgery or in moderate TBI (50, 51). On the other hand, all colloids (dextran, gelatins, and hydroxyethyl starches) invariably affect coagulation, compromising platelet function and fibrin formation, decreasing the activity of coagulation factors, and increasing fibrinolysis (42–44).
3.6 Factor 6: low calcium levels was defined by participants as a “necessary and essential co-factor”
Calcium (Ca++) is an essential co-factor for the enzymatic activation of the coagulation cascade, specifically K- Vitamin dependent factors (II, VII, IX, and X) and factor XIII, which is involved in the structure and strength of the clot (52–54). Additionally, it plays a central role in platelet activation (52–54). Eleven percent of individuals suffering from spontaneous intracerebral haemorrhage in the acute phase present hypocalcaemia (serum Ca++ < 8.4 mg/dL), which is associated with the development of coagulopathy, higher haematoma volume, and increased risk of haematoma expansion (55). Shock associated with polytrauma (without TBI), causes a decrease in ionised Ca++ (<1.1 mmol/L) in 50% of individuals, a condition associated with the development of coagulopathy, increased transfusion requirements, and death (55), findings corroborated in a recent systematic review (56). In patients with moderate and severe TBI, decreases in ionic Ca++ (<1.1 mmol/L) on the third day postadmission were found to be predictive of mortality and poor functional outcomes (57). The main pathophysiological reasons for the decrease in calcium are chelation phenomena, primarily through the action of inflammatory mediators, lactate, and intracellular proteins released by astrocytes or damaged neurons (58). Additional mechanisms of hypocalcaemia include hypoalbuminemia secondary to increased capillary permeability post-shock resuscitation and citrate chelation after transfusion of storage blood (56, 57, 59).
3.7 Factor 7: anaemia-transfusion was defined by participants as “Sometimes beneficial, sometimes dangerous”
Traumatic brain injury itself rarely causes blood loss requiring transfusion (60, 61). Most TBI patients are not severely anaemic at the time they are admitted to the ICU but develop anaemia during their stay (60, 61). Post-ICU anaemia is a secondary complication but its effect on outcome remains controversial (60–62). Since the landmark work of Hébert et al. (63) more than 20 years ago, the trend has been towards restrictive transfusion therapy (64, 65). There is no cutoff point to consider a haemoglobin (Hgb) level as optimal (61, 66). Inasmuch as red blood transfusion has been associated with poor outcomes after TBI (65–70), transfusion should be guided by clinical goals rather than a “magic” number (61, 64, 65).
Indications for transfusion in the context of iTBI should therefore follow the hemodynamic status, cerebral tissue oxygenation, and premorbid state (cardiopathic patients are less tolerant to anaemia) (61, 64, 65). Hgb is responsible for nearly all the O2 transported by the blood, provided it is of good quality and in sufficient quantity. When these conditions are compromised, cerebral tissue hypoxia (anaemic hypoxia) may develop (71, 72). Physiologically, below 7 g/dL, O2 transport capacity drops by half, while above 12 g/dL, O2 availability provides no beneficial changes; on the contrary, O2 transport may decrease due to increased blood viscosity and decreased CBF (61, 71). Transfusion does not ensure correction of cerebral tissue hypoxia, which is multifactorial and requires exhaustive analysis (71, 73). Blood stored for long periods decreases its 2,3-diphosphoglycerate component, which increases the affinity of Hgb for O2, restricting the availability of O2 to the cell (65–69, 74). Transfusions can generate acute lung injury (TRALI), multiorgan dysfunction, and C-iTBI depending mainly on the volume (> risk if massive) and storage time, the so-called “blood bank coagulopathy,” which causes a series of physiological alterations such as tissue hypoxia, hypocalcaemia (citrate intoxication), hypothermia, acidosis, hyperglycaemia and hyperkalaemia, generating a vicious circle that perpetuates and exacerbates C-iTBI. In sum, balancing the risks and benefits of transfusion is paramount (65, 67–69, 74). Available evidence suggests that it is reasonable to maintain Hgb values between 7 and 9 g/dL (61, 65, 66).
3.8 Factor 8: temperature was defined by participants as a factor where “Too low or too high, both are detrimental”
Post-trauma and shock hypothermia affect the coagulation system, resulting in an independent risk factor for mortality and poor outcomes (75–77). Hypothermia increases the affinity of Hgb for O2 (shift to the left), making it more difficult to transfer the necessary O2 to the cell (high-affinity hypoxia) (72, 78). Hypothermia generates a hypocoagulable state, facilitating bleeding by blocking coagulation enzymatic cascades, prolonging the initiation phase, altering platelet aggregability, and decreasing fibrinogen synthesis (79–82). These mechanisms are mediated by thrombin (79, 80). Hyperthermia is prevalent after TBI (83–85). During the first hours of evolution, this constitutes a secondary insult, associated with the severity of the injury and with poor outcomes (83–85). Hyperthermia increases ICP, exacerbates oedema and inflammation, causes rupture of the blood–brain barrier (BBB), and can trigger cerebral hypoxia (83–85). Temperatures between 38 and 39 degrees Celsius generally either do not modify coagulation or cause a prothrombotic state without major consequences (86), but in cases of extreme elevation (heatstroke), the fibrinolytic mechanism initially ceases to function to then consume coagulation factors and platelets, leading to DIC and multiple organ dysfunction (87).
3.9 Factor 9: international normalised ratio-monitoring was defined by participants as a factor in which “Interpreting information is key”
For years and through today, in ICUs worldwide, coagulation monitoring has been based on the determination of conventional coagulation assays (CCA) such as International Normalised Ratio (INR), platelet count, activated partial thromboplastin time (aPPT), and D-dimer, which have also been used to define C-iTBI (Table 1) (6–10, 12, 88).
Two issues are important to highlight. On the one hand, the methods of analysis and cohort points from which to define alterations have been variable and heterogeneous. On the other, multiple limitations emerge in assessing the role of the components during the different phases of coagulation, among which we found (6–10, 12, 88):
• Non-standardised parameters and cutoff points
• Do not assess the onset of clot formation
• Do not assess clot strength and integrity
• Low sensitivity especially in trauma
• Do not assess platelet function
• Non-specific and inaccurate assessment of the fibrinolytic system
• Do not detect the broad spectrum of clotting alterations when antithrombotic drugs were used pre-injury
As a point of comparison, viscoelastic tests (VET) such as TEG thromboelastography or ROTEM thromboelastometry and their variations allow for a more detailed and rapid evaluation of the full spectrum of coagulation physiology at the bedside using whole blood (6–10, 12, 88). Clot formation kinetics, fibrin-platelet interactions, platelet functionality, and fibrinolysis can be graphically monitored (6–10, 12, 88). The parameters evaluated with VETs and their cohort points reported in the literature are outlined in Figure 2.
Viscoelastic tests are more sensitive than CCAs for the full evaluation of the coagulation system (6–10, 12, 87) and their use is recommended in current guidelines for the management of bleeding secondary to trauma (89); however, these modalities also have limitations, including the following:
• Not widely available
• Longer learning curve
• They are in vitro tests (like CCA) that do not allow for assessment of endothelial contribution or von Willebrand factor deficiency.
• Low sensitivity for detecting abnormalities when using low molecular weight heparins or the new oral anticoagulants
• High negative predictive power, but low positive predictive power
• Quality control and maintenance of difficult systems
Coagulation disorder therapy guided by VETs following craniotomy has shown promising results (90). A recent clinical study comparing the management of coagulation disorders during trauma resuscitation demonstrated improved survival and less use of plasma and platelets in patients managed by GDHT directed by VETs vs. GHDT guided by CCA, although an analysis of the subgroup of individuals with TBI found no difference (91). Similar results were observed in the ITACTIC trial, specifically in terms of mortality (92). In the pre-defined TBI group, 64% of individuals managed and guided by VETs did not undergo massive transfusion protocols during the first day of trauma compared to 40% of patients in the group whose therapy was CCA-guided (OR 2.12, 95% CI 0.84–5.34) (91). Irrespective of the monitoring methodology available, we can direct therapy more precisely based on the results obtained. An example found in the evidence is outlined in Figure 3.
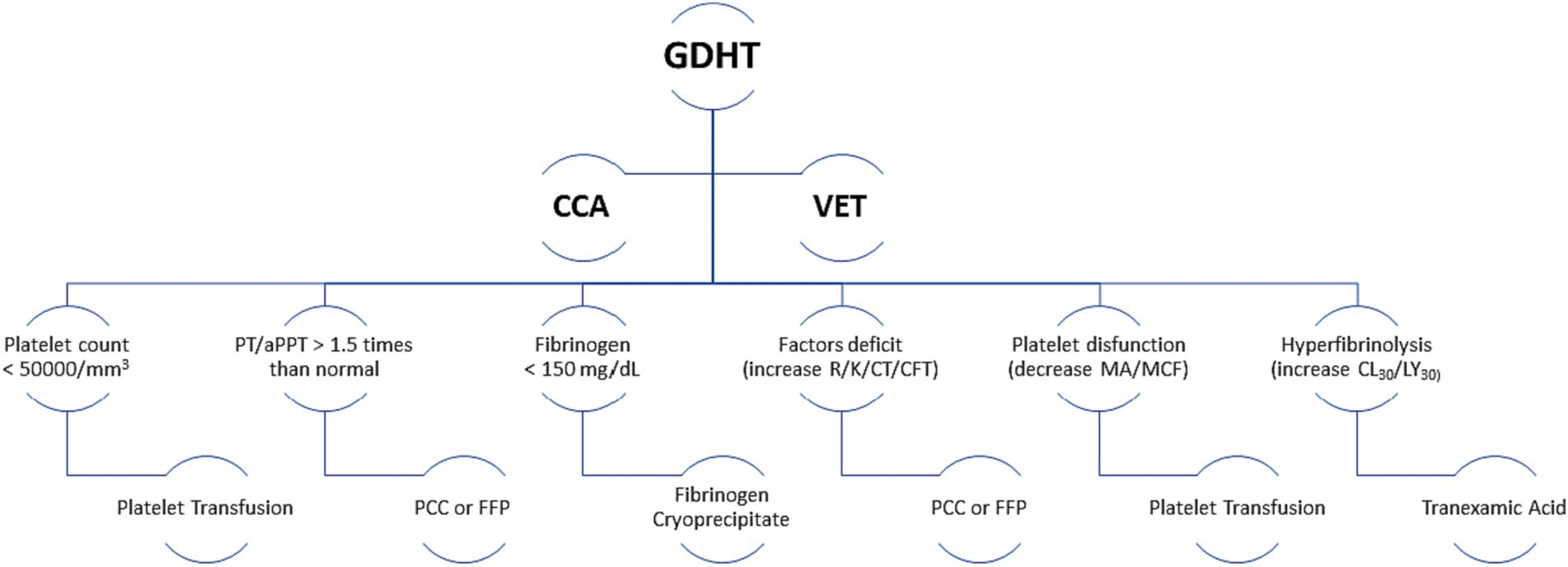
Figure 3. Monitoring methodology. PCC, Prothrombin complex concentrate; FFP, Fresh frozen plasma; GDHT, Goal-directed haemostatic therapy; CCA, Conventional coagulation assays; VET, Viscoelastic tests.
3.10 Factor 10: oral antithrombotic reversal was defined by participants as “Urgent, emergent, or both?”
We have previously explained why clinicians must be on “high alert” when a patient with TBI has a history of pre-injury antithrombotic drugs (12, 28–35). Due to the risk of lesion progression, and that of haemorrhagic lesions in particular, and given its close association with poor outcomes, this is a situation that requires prompt resolution (12, 28–35). Once the administration of the causative agent has been suspended, this next step must emphasise the assessment and clinical-tomographic categorisation of the TBI, establishing the presence of bleeding (volume, location, extension, anatomical distortions or displacements, and associated lesions); its severity and therapeutic possibilities, including the need for surgical intervention (89, 93–95). Coagulation monitoring (CCA or VET) is essential to establish the starting point, follow-up, and above all to avoid the undesirable effects of “overtreatment” (89). Undoubtedly, severe life-threatening bleeding associated with pre-injury use of antithrombotic agents requires immediate reversal (89, 94). In other situations, the need for reversal must be balanced against the risks involved (thrombosis) (89, 94). A detailed analysis of how and with what agents this reversal should be implemented is beyond the scope of this manuscript; but generally the strategy depends fundamentally on the antithrombotic agent in question (89, 93–97). In Table 2, we summarise the agents used in accordance with current guidelines (89).
Although they are not oral agents, we have included unfractionated heparins (UFH) and low molecular weight heparins (LWMH) here due to their frequent pre-injury use, particularly in older adult with significant prothrombotic comorbidities or undergoing prolonged postoperative periods, especially orthopaedic surgery (94).
3.11 Factor 11: normal acid–base status was defined by participants as “Neither acid nor alkaline; neutral”
During acute TBI it is essential to achieve a balanced physiological microenvironment to prevent secondary insults (1, 4, 36, 71). Acidosis causes cerebral vasodilation, increased cerebral blood volume, and consequent increase in ICP; while alkalosis increases the affinity of Hgb for O2 without the possibility of ceding it to the cells, causing cerebral tissue hypoxia of low extraction (1, 4, 36, 71, 72). Coagulation is not exempt from modifications if the acid–base state is modified (75, 76, 80). Although alkalosis does not modify coagulation parameters (86); acidosis compromises both platelet aggregation and clot formation, increases the prolongation phase due to alterations in thrombin, and accelerates the degradation of fibrinogen (75–77).
4 Summary of the design and implications of the mnemonic device “Coagulation”
In this study, we complemented our narrative analysis with evidence-based studies. The methodological premise was that analytic actions should coincide with both evidence-based studies and the characteristics of the factor identified by study participants.
In the Figure 4, we summarise the algorithm based on the comprehensive analysis of the acronym “Coagulation,” we designed as a mnemonic device encompassing the primary factors involved in the management of C-iTBI in the intensive care unit. In the process, we have suggested a comprehensive clinical approach to the variables and situations that may predispose patients to developing this condition, as well as the management of risk factors and complications requiring prompt resolution.
5 Conclusion
Narrative analysis is an interdisciplinary tool that may be applied as a qualitative approach to research using temporally situated data from a wide range of discourses. In this study, we conducted a narrative analysis based on the responses of intensive care physicians regarding the key factors associated with C-iTBI.
Knowledge and detection of the risk factors, pathophysiology, and kinetics of the coagulation system and its alterations allow for the prompt recognition and management of traumatic injury to the brain. The essential information obtained from the serial monitoring of coagulation in conjunction with clinical judgement allows for a personalised and goal-directed therapy that can help prevent or minimise the adverse effects of conventional therapies and reduce the negative consequences of coagulopathy.
Given the time-sensitive nature of such decisions, in this study, we aimed to design an acronym as a mnemonic device to provide clinicians with a simple auxiliary tool in the treatment of this critical complication. We believe that the acronym “Coagulation” meets the stated objectives. It is a simple and easy to apply tool for anyone facing the challenge of treating victims of moderate or severe TBI on a daily basis.
Numerous challenges remain to prevent or minimise secondary damage and ensure improved patient outcomes in C-iTBI. Issues such as the harmonisation of definitions and validation of timely, appropriate, personalised, goal-based management require the prompt design and execution of large-scale clinical studies. The dissemination of knowledge as well as technological advances in the monitoring systems of the coagulation system offer a unique opportunity to unify criteria aimed at achieving increasingly precise medicine in this critical field.
6 Limitations and bias
This study is presented as a narrative method supported by scientific evidence and must take into account the limitations that such an approach entails. It is also specifically focused on TBI.
7 Clinical implications
In this article, we provide a mnemonic device for critical situations that will improve clinical care in the context of intensive care units.
Data availability statement
The raw data supporting the conclusions of this article will be made available upon request to the last author.
Author contributions
MQ-D: Conceptualization, Data curation, Formal Analysis, Funding acquisition, Investigation, Methodology, Project administration, Resources, Writing – original draft, Writing – review & editing, Supervision, Validation. PA: Investigation, Methodology, Project administration, Writing – original draft. RJ-V: Methodology, Project administration, Resources, Writing – original draft. EE-S: Software, Validation, Writing – original draft. CT-G: Data curation, Formal Analysis, Investigation, Writing – review & editing. KN-N: Software, Validation, Writing – original draft. AS-L: Methodology, Project administration, Writing – original draft. PM-N: Validation, Visualization, Writing – original draft. MG-E: Resources, Validation, Visualization, Writing – review & editing. JG-C: Methodology, Project administration, Validation, Visualization, Writing – review & editing. DG: Supervision, Writing – original draft, Writing – review & editing. PS-C: Software, Writing – original draft.
Funding
The author(s) declare financial support was received for the research, authorship, and/or publication of this article. This article has been funded by the scientific research grants of Mutua Madrileña.
Conflict of interest
The authors declare that the research was conducted in the absence of any commercial or financial relationships that could be construed as a potential conflict of interest.
Publisher’s note
All claims expressed in this article are solely those of the authors and do not necessarily represent those of their affiliated organizations, or those of the publisher, the editors and the reviewers. Any product that may be evaluated in this article, or claim that may be made by its manufacturer, is not guaranteed or endorsed by the publisher.
References
1. Stocchetti, N, Carbonara, M, Citerio, G, Ercole, A, Skrifvars, MB, Smielewski, P, et al. Severe traumatic brain injury: targeted management in the intensive care unit. Lancet Neurol. (2017) 16:452–64. doi: 10.1016/S1474-4422(17)30118-7
2. Godoy, DA, and Murillo-Cabezas, F. Evolutionary conceptualization of the injury mechanisms in traumatic brain injury. Med Intens. (2020) 46:90–3. doi: 10.1016/j.medin.2020.02.003
4. Bouzat, P, Sala, N, Payen, JF, and Oddo, M. Beyond intracranial pressure: optimization of cerebral blood flow, oxygen, and substrate delivery after traumatic brain injury. Ann Intensive Care. (2013) 3:23. doi: 10.1186/2110-5820-3-23
5. Yuan, Q, Sun, YR, Wu, X, Yu, J, Li, ZQ, du, ZY, et al. Coagulopathy in traumatic brain injury and its correlation with progressive hemorrhagic injury: a systematic review and meta-analysis. J Neurotrauma. (2016) 33:1279–91. doi: 10.1089/neu.2015.4205
6. Maegele, M. Coagulopathy and progression of intracranial hemorrhage in traumatic brain injury: mechanisms, impact, and therapeutic considerations. Neurosurgery. (2021) 89:954–66. doi: 10.1093/neuros/nyab358
7. Laroche, M, Kutcher, ME, Huang, MC, Cohen, MJ, and Manley, GT. Coagulopathy after traumatic brain injury. Neurosurgery. (2012) 70:1334–45. doi: 10.1227/NEU.0b013e31824d179b
8. Zhang, J, Zhang, F, and Dong, JF. Coagulopathy induced by traumatic brain injury: the systemic manifestation of a localized injury. Blood. (2018) 131:2001–6. doi: 10.1182/blood-2017-11-784108
9. Mathur, R, and Suarez, JI. Coagulopathy in isolated traumatic brain injury: myth or reality. Neurocrit Care. (2022) 38:429–38. doi: 10.1007/s12028-022-01647-4
10. Maegele, M, Schöchl, H, Menovsky, T, Maréchal, H, Marklund, N, Buki, A, et al. Coagulopathy and hemorrhagic progression in traumatic brain injury: advances in mechanisms, diagnosis, and management. Lancet Neurol. (2017) 16:630–47. doi: 10.1016/S1474-4422(17)30197-7
11. Roozenbeek, B, Maas, AI, and Menon, DK. Changing patterns in the epidemiology of traumatic brain injury. Nat Rev Neurol. (2013) 9:231–6. doi: 10.1038/nrneurol.2013.22
12. Böhm, JK, Güting, H, Thorn, S, Schäfer, N, Rambach, V, Schöchl, H, et al. CENTER-TBI participants and investigators. Global characterization of coagulopathy in isolated traumatic brain injury (iTBI): a CENTER-TBI analysis. Neurocrit Care. (2021) 35:184–96. doi: 10.1007/s12028-020-01151-7
13. Wafaisade, A, Lefering, R, Tjardes, T, Wutzler, S, Simanski, C, Paffrath, T, et al. Acute coagulopathy in isolated blunt traumatic brain injury. Neurocrit Care. (2010) 12:211–9. doi: 10.1007/s12028-009-9281-1
14. Solla, DJF, de Amorim, RLO, Kolias, AG, Hutchinson, PJ, de Andrade, AF, Teixeira, MJ, et al. Incremental prognostic value of coagulopathy in addition to the crash score in traumatic brain injury patients. Neurocrit Care. (2021) 34:130–8. doi: 10.1007/s12028-020-00991-7
15. Murray, GD, Butcher, I, McHugh, GS, Lu, J, Mushkudiani, NA, Maas, AI, et al. Multivariable prognostic analysis in traumatic brain injury: results from the IMPACT study. J Neurotrauma. (2007) 24:329–37. doi: 10.1089/neu.2006.0035
16. Greuters, S, van den Berg, A, Franschman, G, Viersen, VA, Beishuizen, A, Peerdeman, SM, et al. Acute and delayed mild coagulopathy are related to outcome in patients with isolated traumatic brain injury. Crit Care. (2011) 15:R2. doi: 10.1186/cc9399
17. Brain Trauma Foundation. American association of neurological surgeons, congress of neurological surgeons. Guidelines for the management of severe traumatic brain injury. J Neurotrauma. (2007) 24:i–vi. doi: 10.1089/neu.2007.9999
18. Lolli, V, Pezzullo, M, Delpierre, I, and Sadeghi, N. MDCT imaging of traumatic brain injury. Br J Radiol. (2016) 89:20150849. doi: 10.1259/bjr.20150849
19. Mutch, CA, Talbott, JF, and Gean, A. Imaging evaluation of acute traumatic brain injury. Neurosurg Clin N Am. (2016) 27:409–39. doi: 10.1016/j.nec.2016.05.011
20. Marshall, LF, Marshall, SB, Klauber, MR, Clark, MB, Eisenberg, HM, Jane, JA, et al. A new classification of head injury based on computerized tomography. J Neurosurg. (1991) 75:S14–20. doi: 10.3171/SUP.1991.75.1S.0S14
21. Daiute, C, and Lightfoot, C. Theory and craft in narrative inquiry In: C Daiute and C Lightfoot, editors. Narrative Analysis: Studying the Development of Individuals in Society. Thousand Oaks, CA: Sage (2004). vii–xviii.
22. Narayan, RK, Greenberg, RP, Miller, JD, Enas, GG, Choi, SC, Kishore, PRS, et al. Improved confidence of outcome prediction in severe head injury: a comparative analysis of the clinical examination, multimodality evoked potentials, CT scanning, and intracranial pressure. J Neurosurg. (1981) 54:751–62. doi: 10.3171/jns.1981.54.6.0751
23. Servadei, FD, Murray, GD, Key, P, Teasdale, GM, Dearden, M, Iannotti, F, et al. The value of the “worst” computed tomographic scan in clinical studies of moderate and severe head injury. Neurosurgery. (2000) 46:70–7. doi: 10.1097/00006123-200001000-00014
24. Maas, AI, Hukkelhoven, CW, Marshall, LF, and Steyerberg, EW. Prediction of outcome in traumatic brain injury with computed tomographic characteristics: a comparison between the computed tomographic classification and combinations of computed tomographic predictors. Neurosurgery. (2005) 57:1173. doi: 10.1227/01.neu.0000186013.63046.6b
25. Mishra, R, Vasquez Ucros, HE, Florez-Perdomo, WA, Suarez, JR, Moscote-Salazar, LR, Rahman, MM, et al. Predictive value of Rotterdam score and Marshall score in traumatic brain injury: a contemporary review. Ind J Neurotrauma. (2021) 19:069–77. doi: 10.1055/s-0041-1727404
26. Raj, R, Siironen, J, Skrifvars, MB, Hernesniemi, J, and Kivisaari, R. Predicting outcome in traumatic brain injury: development of a novel computerized tomography classification system (Helsinki computerized tomography score). Neurosurgery. (2014) 75:632–47. doi: 10.1227/NEU.0000000000000533
27. Batchelor, JS, and Grayson, A. A meta-analysis to determine the effect of anticoagulation on mortality in patients with blunt head trauma. Br J Neurosurg. (2012) 26:525–30. doi: 10.3109/02688697.2011.650736
28. Fabbri, A, Servadei, F, Marchesini, G, Bronzoni, C, Montesi, D, and Arietta, L. et al., Antiplatelet therapy and the outcome of subjects with intracranial injury: the Italian SIMEU study. Crit Care. (2013) 17:R53. doi: 10.1186/cc12575
29. Uccella, L, Zoia, C, Bongetta, D, Gaetani, P, Martig, F, Candrian, C, et al. Are antiplatelet and anticoagulants drugs a risk factor for bleeding in mild traumatic brain injury? World Neurosurg. (2018) 110:e339–45. doi: 10.1016/j.wneu.2017.10.173
30. Kobayashi, L, Barmparas, G, Bosarge, P, Brown, CV, Bukur, M, Carrick, MM, et al. Novel oral anticoagulants and trauma: the results of a prospective American Association for the Surgery of Trauma multi-institutional trial. J Trauma Acute Care Surg. (2017) 82:827–35. doi: 10.1097/TA.0000000000001414
31. Zeeshan, M, Jehan, F, O’Keeffe, T, Khan, M, Zakaria, ER, Hamidi, M, et al. The novel oral anticoagulants (NOACs) have worse outcomes compared to warfarin in patients with intracranial hemorrhage after TBI. J Trauma Acute Care Surg. (2018) 85:915–20. doi: 10.1097/TA.0000000000001995
32. Markou, M, Pleger, B, Grötzlinger, M, Pintea, B, Hamsen, U, Könen, S, et al. Intake of NOAC is associated with hematoma expansion of intracerebral hematomas after traumatic brain injury. Eur J Trauma Emerg Surg. (2021) 47:565–71. doi: 10.1007/s00068-019-01228-9
33. Mathieu, F, Güting, H, Gravesteijn, B, Monteiro, M, Glocker, B, Kornaropoulos, EN, et al. Collaborative European NeuroTrauma effectiveness research in traumatic brain injury (CENTER-TBI) investigators and participants. Impact of antithrombotic agents on radiological lesion progression in acute traumatic brain injury: a CENTER-TBI propensity-matched cohort analysis. J Neurotrauma. (2020) 37:2069–80. doi: 10.1227/NEU.0000000000000575
34. Vedin, T, Lundager Forberg, J, Anefjäll, E, Lehtinen, R, Faisal, M, and Edelhamre, M. Antiplatelet therapy contributes to a higher risk of traumatic intracranial hemorrhage compared to anticoagulation therapy in ground-level falls: a single-center retrospective study. Eur J Trauma Emerg Surg. (2022) 48:4909–17. doi: 10.1007/s00068-022-02016-8
35. Mathieu, F, Malhotra, AK, Ku, JC, Zeiler, FA, Wilson, JR, Pirouzmand, F, et al. Pre-injury antiplatelet therapy and risk of adverse outcomes after traumatic brain injury: a systematic review and meta-analysis. Neurotrauma Rep. (2022) 3:308–20. doi: 10.1089/neur.2022.0042
36. Godoy, DA, Videtta, W, Santa Cruz, R, Silva, X, Aguilera-Rodríguez, S, Carreño-Rodríguez, JN, et al. General care in the management of severe traumatic brain injury: Latin american consensus [published online ahead of print, 2020 may 3]. Med Int. (2020) 44:500–8. doi: 10.1016/j.medin.2020.01.014
37. Chesnut, RM, Marshall, LF, Klauber, MR, Blunt, BA, Baldwin, N, Eisenberg, HM, et al. The role of secondary brain injury in determining outcome from severe head injury. J Trauma. (1993) 34:216–22. doi: 10.1097/00005373-199302000-00006
38. Manley, G, Knudson, MM, Morabito, D, Damron, S, Erickson, V, and Pitts, L. Hypotension, hypoxia, and head injury: frequency, duration, and consequences. Arch Surg. (2001) 136:1118. doi: 10.1001/archsurg.136.10.1118
39. Maegele, M. Coagulopathy after traumatic brain injury: incidence, pathogenesis, and treatment options. Transfusion. (2013) 53:28S–37S. doi: 10.1111/trf.12033
40. Lustenberger, T, Talvig, P, Kobayashi, L, Inaba, K, Lam, L, Plurad, D, et al. The time course of coagulopathy is isolated severe traumatic brain injury. Injury. (2010) 41:924–8. doi: 10.1016/j.injury.2010.04.019
41. Oddo, M, Poole, D, Helbok, R, Meyfroidt, G, Stocchetti, N, Bouzat, P, et al. Fluid therapy in neurointensive care patients: ESICM consensus and clinical practice recommendations. Intensive Care Med. (2018) 44:449–63. doi: 10.1007/s00134-018-5086-z
42. Coats, TJ, Brazil, E, and Heron, M. The effects of commonly used resuscitation fluids on whole blood coagulation. Emerg Med J. (2006) 23:546–9. doi: 10.1136/emj.2005.032334
43. Kozek-Langenecker, SA. Fluids and coagulation. Curr Opin Crit Care. (2015) 21:285–91. doi: 10.1097/MCC.0000000000000219
44. Kozek-Langenecker, SA. Influence of fluid therapy on the hemostatic system of intensive care patients. Best Pract Res Clin Anaesthesiol. (2009) 23:225–36. doi: 10.1016/j.bpa.2008.11.002
45. Konrad, C, Markl, T, Schuepfer, G, Schmeck, J, and Gerber, HR. In vitro effects of different medium molecular hydroxyethyl starch solutions and lactated Ringer’s solution on coagulation using SONOCLOT. Anesth Analg. (2000) 90:274–9. doi: 10.1097/00000539-200002000-00007
46. Tocantins, LM. The clot accelerating effect of dilution on blood and plasma. Relation to the mechanism of coagulation of normal and haemophiliac blood. Blood. (1951) 6:720–39. doi: 10.1182/blood.V6.8.720.720
47. Ruttmann, TG, James, MF, and Viljoen, JF. Haemodilution induces a hypercoagulable state. Br J Anaesth. (1996) 76:412–4. doi: 10.1093/bja/76.3.412
48. Jamnicki, M, Zollinger, A, Seifert, B, Popovic, D, Pasch, T, and Spahn, DR. The effect of potato starch derived and corn starch derived hydroxyethyl starch on in vitro blood coagulation. Anaesthesia. (1998) 53:638–44. doi: 10.1046/j.1365-2044.1998.489-az0565.x
49. Roche, AM, James, MFM, Grocott, MPW, and Mythen, MG. Coagulation effects of in vitro haemodilution with a balanced electrolyte hetastarch solution and lactated Ringer’s solution. Anaesthesia. (2002) 57:950–5. doi: 10.1046/j.1365-2044.2002.02707.x
50. Wang, H, Cao, H, Zhang, X, Ge, L, and Bie, L. The effect of hypertonic saline and mannitol on coagulation in moderate traumatic brain injury patients. Am J Emerg Med. (2017) 35:1404–7. doi: 10.1016/j.ajem.2017.04.020
51. Hernández-Palazón, J, Fuentes-García, D, Doménech-Asensi, P, Piqueras-Pérez, C, Falcón-Araña, L, and Burguillos-López, S. Equiosmolar solutions of hypertonic saline and mannitol do not impair blood coagulation during elective intracranial surgery. J Neurosurg Anesthesiol. (2017) 29:8–13. doi: 10.1097/ANA.0000000000000255
52. Palta, S, Saroa, R, and Palta, A. Overview of the coagulation system. Ind J Anaesth. (2014) 58:515–23. doi: 10.4103/0019-5049.144643
53. O'Donnell, JS, O'Sullivan, JM, and Preston, RJS. Advances in understanding the molecular mechanisms that maintain normal haemostasis. Br J Haematol. (2019) 186:24–36. doi: 10.1111/bjh.15872
54. Singh, S, Dodt, J, Volkers, P, Hethershaw, E, Philippou, H, Ivaskevicius, V, et al. Structure functional insights into calcium binding during the activation of coagulation factor XIII a. Sci Rep. (2019) 9:11324. doi: 10.1038/s41598-019-47815-z
55. Morotti, A, Charidimou, A, Phuah, CL, Jessel, MJ, Schwab, K, Ayres, AM, et al. Calcium level and extent of bleeding in patients with intracerebral hemorrhage. JAMA Neurol. (2016) 73:1285–90. doi: 10.1001/jamaneurol.2016.2252
56. Vasudeva, M, Mathew, JK, Groombridge, C, Tee, JW, Johnny, CS, Maini, A, et al. Hypocalcemia in trauma patients: a systematic review. J Trauma Acute Care Surg. (2021) 90:396–402. doi: 10.1097/TA.0000000000003027
57. Manuel, VR, Martin, SA, Juan, SR, Fernando, MA, Frerk, M, Thomas, K, et al. Hypocalcemia as a prognostic factor in mortality and morbidity in moderate and severe traumatic brain injury. Asian J Neurosurg. (2015) 10:190–4. doi: 10.4103/1793-5482.161171
58. DeBot, M, Sauaia, A, Schaid, T, and Moore, EE. Trauma-induced hypocalcemia. Transfusion. (2022) 62:S274–80. doi: 10.1111/trf.16959
59. Vasudeva, M, Mathew, JK, Fitzgerald, MC, Cheung, Z, and Mitra, B. Hypocalcaemia and traumatic coagulopathy: an observational analysis. Vox Sang. (2020) 115:189–95. doi: 10.1111/vox.12875
60. Vanhala, H, Junttila, E, Kataja, A, Huhtala, H, Luostarinen, T, and Luoto, T. Incidence and associated factors of Anemia in patients with acute moderate and severe traumatic brain injury. Neurocrit Care. (2022) 37:629–37. doi: 10.1007/s12028-022-01561-9
61. Lelubre, C, Bouzat, P, Crippa, IA, and Taccone, FS. Anemia management after acute brain injury. Crit Care. (2016) 20:152. doi: 10.1186/s13054-016-1321-6
62. Salim, A, Hadjizacharia, P, DuBose, J, Brown, C, Inaba, K, Chan, L, et al. Role of anemia in traumatic brain injury. J Am Coll Surg. (2008) 207:398–406. doi: 10.1016/j.jamcollsurg.2008.03.013
63. Hébert, PC, Wells, G, Blajchman, MA, Marshall, J, Martin, C, Pagliarello, G, et al. A multicenter, randomized, controlled clinical trial of transfusion requirements in critical care. Transfusion requirements in critical care investigators, Canadian critical care trials group. N Engl J Med. (1999) 340:409–17. doi: 10.1056/NEJM199902113400601
64. Egea-Guerrero, JJ, García-Sáez, I, and Quintana-Díaz, M. Trigger transfusion in severe traumatic brain injury. Med Intens. (2021) S0210-5691:00071. doi: 10.1016/j.medin.2021.03.012
65. Montgomery, EY, Barrie, U, Kenfack, YJ, Edukugho, D, Caruso, JP, Rail, B, et al. Transfusion guidelines in traumatic brain injury: a systematic review and Meta-analysis of the currently available evidence. Neurotrauma Rep. (2022) 3:554–68. doi: 10.1089/neur.2022.0056
66. Florez-Perdomo, WA, García-Ballestas, E, Martinez-Perez, R, Agrawal, A, Deora, H, Joaquim, AF, et al. Hemoglobin levels as a transfusion criterion in moderate to severe traumatic brain injury: a systematic review and meta-analysis. Br J Neurosurg. (2021) 37:1473–9. doi: 10.1080/02688697.2021.1940850
67. Boutin, A, Moore, L, Lauzier, F, Chassé, M, English, S, Zarychanski, R, et al. Transfusion of red blood cells in patients with traumatic brain injuries admitted to Canadian trauma health centres: a multicentre cohort study. BMJ Open. (2017) 7:e014472. doi: 10.1136/bmjopen-2016-014472
68. Spahn, DR, and Rossaint, R. Coagulopathy and blood component transfusion in trauma. Br J Anaesth. (2005) 95:130–9. doi: 10.1093/bja/aei169
69. Vernon, T, Morgan, M, and Morrison, C. Bad blood: a coagulopathy associated with trauma and massive transfusion review. Acute Med Surg. (2019) 6:215–22. doi: 10.1002/ams2.402
70. Zhang, W, Du, K, and Chen, X. Benefits of red blood cell transfusion in patients with traumatic brain injury. Crit Care. (2019) 23:218. doi: 10.1186/s13054-019-2498-2
71. Godoy, DA, Lubillo, S, and Rabinstein, AA. Pathophysiology and management of intracranial hypertension and tissular brain hypoxia after severe traumatic brain injury: an integrative approach. Neurosurg Clin N Am. (2018) 29:195–212. doi: 10.1016/j.nec.2017.12.001
72. Siggaard-Andersen, O, Gothgen, IH, Fogh-Andersen, N, and Larsen, VH. Oxygen status of arterial and mixed venous blood. Crit Care Med. (1995) 23:1284–93. doi: 10.1097/00003246-199507000-00020
73. Oddo, M, Levine, JM, Mackenzie, L, Frangos, S, Feihl, F, Kasner, SE, et al. Brain hypoxia is associated with short-term outcome after severe traumatic brain injury independently of intracranial hypertension and low cerebral perfusion pressure. Neurosurgery. (2011) 69:1037–45. doi: 10.1227/NEU.0b013e3182287ca7
74. MacLeod, JB. Trauma and coagulopathy: a new paradigm to consider. Arch Surg. (2008) 143:797–801. doi: 10.1001/archsurg.143.8.797
75. Eddy, VA, Morris, JA Jr, and Cullinane, DC. Hypothermia, coagulopathy, and acidosis. Surg Clin North Am. (2000) 80:845–54. doi: 10.1016/s0039-6109(05)70099-2
76. Gerecht, R. The lethal triad. Hypothermia, acidosis & coagulopathy create a deadly cycle for trauma patients. JEMS. (2014) 39:56–61.
77. Vardon, F, Mrozek, S, Geeraerts, T, and Fourcade, O. Accidental hypothermia in severe trauma. Anaesth Crit Care Pain Med. (2016) 35:355–61. doi: 10.1016/j.accpm.2016.05.001
78. Zauner, A, Daugherty, WP, Bullock, MR, and Warner, DS. Brain oxygenation and energy metabolism: part I-biological function and pathophysiology. Neurosurgery. (2002) 51:289–301. doi: 10.1097/00006123-200208000-00003
79. Wallner, B, Schenk, B, Hermann, M, Paal, P, Falk, M, Strapazzon, G, et al. Hypothermia-associated coagulopathy: a comparison of viscoelastic monitoring, platelet function, and real time live confocal microscopy at low blood temperatures, an in vitro experimental study. Front Physiol. (2020) 11:843. doi: 10.3389/fphys.2020.00843
80. De Robertis, E, Kozek-Langenecker, SA, Tufano, R, Romano, GM, Piazza, O, and Zito Marinosci, G. Coagulopathy induced by acidosis, hypothermia and hypocalcaemia in severe bleeding. Minerva Anestesiol. (2015) 81:65–75.
81. Mitrophanov, AY, Szlam, F, Sniecinski, RM, Levy, JH, and Reifman, J. Controlled multifactorial coagulopathy: effects of dilution, hypothermia, and acidosis on thrombin generation in vitro. Anesth Analg. (2020) 130:1063–76. doi: 10.1213/ANE.0000000000004479
82. Levi, M. Hemostasis and thrombosis in extreme temperatures (hypo and hyperthermia). Semin Thromb Hemost. (2018) 44:651–5. doi: 10.1055/s-0038-1648231
83. Badjatia, N. Hyperthermia and fever control in brain injury. Crit Care Med. (2009) 37:S250–7. doi: 10.1097/CCM.0b013e3181aa5e8d
84. Bao, L, Chen, D, Ding, L, Ling, W, and Xu, F. Fever burden is an independent predictor for prognosis of traumatic brain injury. PLoS One. (2014) 9:e90956. doi: 10.1371/journal.pone.0090956
85. Puccio, AM, Fischer, MR, Jankowitz, BT, Yonas, H, Darby, JM, and Okonkwo, DO. Induced normothermia attenuates intracranial hypertension and reduces fever burden after severe traumatic brain injury. Neurocrit Care. (2009) 11:82–7. doi: 10.1007/s12028-009-9213-0
86. Ramaker, AJ, Meyer, P, van der Meer, J, Struys, MM, Lisman, T, van Oeveren, W, et al. Effects of acidosis, alkalosis, hyperthermia and hypothermia on haemostasis: results of point-of-care testing with the thromboelastography analyser. Blood Coagul Fibrinolysis. (2009) 20:436–9. doi: 10.1097/MBC.0b013e32832dc327
87. Iba, T, Connors, JM, Levi, M, and Levy, JH. Heatstroke-induced coagulopathy: biomarkers, mechanistic insights, and patient management. EClinicalMedicine. (2022) 44:101276. doi: 10.1016/j.eclinm.2022.101276
88. Bradbury, JL, Thomas, SG, Sorg, NR, Mjaess, N, Berquist, MR, Brenner, TJ, et al. Viscoelastic testing and coagulopathy of traumatic brain injury. J Clin Med. (2021) 10:5039. doi: 10.3390/jcm10215039
89. Spahn, DR, Bouillon, B, Cerny, V, Duranteau, J, Filipescu, D, Hunt, BJ, et al. The European guideline on management of major bleeding and coagulopathy following trauma. Crit Care. (2019) 23:98. doi: 10.1186/s13054-019-2347-3
90. Rimaitis, M, Bilskienė, D, Tamošuitis, T, Vilcinis, R, Rimaitis, K, and Macas, A. Implementation of thromboelastometry for coagulation management in isolated traumatic brain injury patients undergoing craniotomy. Med Sci Monit. (2020) 26:e922879. doi: 10.12659/MSM.922879
91. Gonzalez, E, Moore, EE, Moore, HB, Chapman, MP, Chin, TL, Ghasabyan, A, et al. Goal-directed hemostatic resuscitation of trauma-induced coagulopathy: a pragmatic randomized clinical trial comparing a viscoelastic assay to conventional coagulation assays. Ann Surg. (2016) 263:1051–9. doi: 10.1097/SLA.0000000000001608
92. Baksaas-Aasen, K, Gall, LS, Stensballe, J, Juffermans, NP, Curry, N, Maegele, M, et al. Viscoelastic haemostatic assay augmented protocols for major trauma haemorrhage (ITACTIC): a randomized, controlled trial. Intensive Care Med. (2021) 47:49–59. doi: 10.1007/s00134-020-06266-1
93. Sperry, JD, Rose, AE, Williams, E, Dierks, MR, and Medow, JE. Emergent reversal of antithrombotics and treatment of life-threatening bleeding from coagulopathies: a clinical review. J Emerg Med. (2022) 63:17–48. doi: 10.1016/j.jemermed.2022.05.011
94. Frontera, JA, Lewin III, JJ, Rabinstein, AA, Aisiku, IP, Alexandrov, AW, Cook, AM, et al. Guideline for reversal of Antithrombotics in intracranial hemorrhage. A statement for healthcare professionals from the neurocritical care society and society of critical care medicine. Neurocrit Care. (2016) 24:6–46. doi: 10.1007/s12028-015-0222-x
95. Ammar, AA, Ammar, MA, Owusu, KA, Brown, SC, Kaddouh, F, Elsamadicy, AA, et al. Andexanet alfa versus 4-factor prothrombin complex concentrate for reversal of factor Xa inhibitors in intracranial hemorrhage. Neurocrit Care. (2021) 35:255–61. doi: 10.1007/s12028-020-01161-5
96. Badiwhala, JH, Wilson, JR, and Fehlings, MG. Global burden of traumatic brain and spinal cord injury. Lancet Neurol. (2019) 18:24–5. doi: 10.1016/S1474-4422(18)30444-7
Keywords: coagulopathy, diagnosis, traumatic brain injury, haemostasis, blood coagulation, anticoagulants, antithrombotic drugs
Citation: Quintana-Diaz M, Anania P, Juárez-Vela R, Echaniz-Serrano E, Tejada-Garrido CI, Sanchez-Conde P, Nanwani-Nanwani K, Serrano-Lázaro A, Marcos-Neira P, Gero-Escapa M, García-Criado J and Godoy DA (2023) “COAGULATION”: a mnemonic device for treating coagulation disorders following traumatic brain injury—a narrative-based method in the intensive care unit. Front. Public Health. 11:1309094. doi: 10.3389/fpubh.2023.1309094
Edited by:
Felix Carl Fabian Schmitt, Heidelberg University Hospital, GermanyReviewed by:
Laura Uccella, Lugano Regional Hospital, SwitzerlandAman Mamo, Madda Walabu University, Ethiopia
Copyright © 2023 Quintana-Diaz, Anania, Juárez-Vela, Echaniz-Serrano, Tejada-Garrido, Sanchez-Conde, Nanwani-Nanwani, Serrano-Lázaro, Marcos-Neira, Gero-Escapa, García-Criado and Godoy. This is an open-access article distributed under the terms of the Creative Commons Attribution License (CC BY). The use, distribution or reproduction in other forums is permitted, provided the original author(s) and the copyright owner(s) are credited and that the original publication in this journal is cited, in accordance with accepted academic practice. No use, distribution or reproduction is permitted which does not comply with these terms.
*Correspondence: Raúl Juárez-Vela, cmF1bC5qdWFyZXpAdW5pcmlvamEuZXM=