- 1Department of Food Science, College of Food Science and Technology, Sichuan Tourism University, Chengdu, China
- 2Industrial College of Prepared Dishes, Sichuan Tourism University, Chengdu, China
- 3Department of Food Science, University of Massachusetts, Amherst, MA, United States
The ultrasound-assisted extraction technique was used for extracting polysaccharides of Tricholoma matsutake (PTM) in this work. The effects of different parameters, i.e., solid-to-liquid ratio, ultrasonic power, extraction time, and extraction temperature on the PTM yield were optimized using a rotatable, orthogonal central composite design. In the derived optimal conditions, the PTM yield was 16.97 ± 0.06%, which corresponded well with the regression model predicted value of 16.85%. The extracted PTM was purified, and the structural characteristics and antioxidant activities were investigated. The PTM was found to be composed mainly of fructose, mannose, glucose, and galactose with a molar molecular ratio of 1:2.25:9.35:5, and an average molecular weight of 90,100 Da. High antioxidant effects of PTM were seen in the tests of DPPH, hydroxyl, and ABTS radical scavenging, along with a moderate superoxide radical scavenging rate.
1 Introduction
Tricholoma matsutake, also known as the Matsutake, Synth, or Taiwan Mushroom, belongs to the Basidiomycotina subdivision within the Basidiomycota phylum. It is an ectomycorrhizal fungus that grows on pine and oak trees. Since ancient times, it has been used extensively in conventional foods and folk medicines (Li et al., 2021). It has increasingly attracted research on its nutritional values and biological applications as an anticancer and antioxidant agent in recent years (You et al., 2013; Ren et al., 2014). Polysaccharides as one of its key compounds, have been long used as a natural food additive as a stabilizer, thickener, water retention agent, and so on (Yin et al., 2011). Moreover, they also exhibit numerous pharmacological advantages, such as improved steroid-induced avascular necrosis of the femoral head in rats (Cui et al., 2019); increased immune response and expression of interleukin in lymphocytes (Hu H. et al., 2018; Hu J. L. et al., 2018); and inhibition of cervical cancer cell proliferation and an increased rate of apoptosis (Yang et al., 2018). Therefore, these polysaccharides can be regarded as natural and safe compounds for applications in foods and medicine. However, investigation of the optimal conditions for extraction of the polysaccharides of Tricholoma matsutake is still quite limited.
There are several conventional methods (Zheng et al., 2016) for the extraction of polysaccharides, such as maceration (Medlej et al., 2020), hot water extraction (Zhao et al., 2019), cold water extraction (Zhou et al., 2020), microwave-assisted extraction (Yuan et al., 2019), and ultrasound-assisted extraction (UAE) (Hu H. et al., 2018; Hu J. L. et al., 2018). Among these techniques, UAE has the advantages of shorter extraction times, low energy requirement, simplified manipulation, and high extraction yield. Therefore, it is an effective and sensible method for further improving PTM extraction. A rotatable, orthogonal central composite design (CCD) is suitable for multi-factor and multi-level experiments; it can obtain a regression model with the same number of items with fewer processing combinations (Quinlan et al., 2015). Compared with the Box–Behnken experiment, the rotatable, orthogonal CCD can better fit the required surface with a simpler calculation process and higher efficiency (Zheng et al., 2016; Quinlan et al., 2015). To the best of our knowledge, there have been no reports on the optimal UAE conditions for PTM with a CCD.
In light of this, during the extraction processes, the effects of multiple variables should be considered. Furthermore, although there are numerous studies on PTM, they are relatively scattered, and little is known about the ultrasonic-assisted extraction process, monosaccharide composition, molecular weight, structural characteristics, and bioactive properties of PTM. Therefore, this work aimed to study the ultrasonic extraction of polysaccharides from Tricholoma matsutake. Based on single-factor experiments and the response surface methodology, the extraction method was optimized. Structural characterization and antioxidant activities were also studied. It focuses on the analysis of the structure and antioxidant activity of PTM.
2 Materials and methods
2.1 Materials
Tricholoma matsutake was purchased from Xiaojin County (Ngawa Tibetan and Qiang Au-tonomous Prefecture Sichuan, China). DEAE-52 cellulose, Sephadex G-100, and dextran standards were from Sigma-Aldrich (United States). All chemicals and reagents used in this work were of analytical grade.
2.2 PTM preparation
The T. matsutake samples were dried at 50°C for to constant weight and ground with a multi-function crusher (HK-043, Anhui Xuzhong Intelligent Technology Co., Ltd., China) to obtain fine powders sieved through a size 40 mesh. The powder (20 g) was mixed with purified water in a 500 mL beaker. The PTM was extracted by using an ultrasonic cell disruptor (VCX500, 20 Hz, Sonics, United States) with adjustable power, time, and temperature. The extraction was performed for a variety of liquid-to-solid ratios (1:10, 1:15, 1:20, 1:25, and 1:30 w/v), extraction times (20, 30, 40, 50 and 60 min), ultrasonic power (80, 100, 125, 150, and 175 W), and extraction temperatures (40°C, 50°C, 60°C, 70°C, and 80°C). Each group of experiments was repeated thrice, and the polysaccharide content of the solution was determined after filtering. The solutions were then transferred to a rotary evaporator (SY5000, Shanghai Yarong Biochemical Instrument Factory, China) and their volumes were reduced to one-third of the original volume at 0.08 Pa. The solutions were mixed with 4 times the volume of anhydrous ethanol and kept overnight at 4°C. The precipitate was obtained through centrifugation (6,000 RPM, 10 min) and dissolved into a 5% aqueous solution. The protein was then separated five times using the Sevag method (Zeng et al., 2019). The supernatant was dialyzed with dialysis bags (cut-off MW 14000 Da) in deionized water for 3 days to remove molecular impurities from the solution, followed by freeze-drying to obtain PTM.
2.3 PTM extraction yield determination
The PTM content was determined by the DNS (dinitrosalicylic acid) method (Saqib and Whitney, 2011), with glucose as the standard, using the enzyme mark instrument (ELx808, Molecular Devices, United States). Using a test tube with only purified water as a blank control, the absorbance values of each test tube were measured at a wavelength of 470 nm. A standard curve was drawn for the glucose content (transverse coordinate) vs. absorbance values (longitudinal coordinate). The PTM concentration was calculated from the standard curve. Finally, the percentage of PTM yield was computed from Equation 1.
2.4 Single-factor experiments
The T. matsutake solutions were treated with ultrasonic waves, and the effect of the solid-to-liquid ratio, extraction time, ultrasonic power, and extraction temperature, on the PTM yield were evaluated separately by changing only one factor at a time while keeping the other three constant.
2.5 Orthogonal CCD
To optimize the UAE conditions for PTM, based on the single-factor experiments, a five-level orthogonal CCD was constructed with four factors, i.e., (A) solid-to-liquid ratio, (B) extraction time, (C) ultrasonic power, and (D) extraction temperature (Table 1; each condition assigned a value between −2 and 2). The PTM extraction yield served as the dependent variable. The orthogonal CCD consisted of 30 experimental points and was carried out three times in parallel in a random order (Table 2). The prediction model was obtained by stepwise regression. The effects of the four factors on the PTM yield and their interactions were analyzed by the response surface (Design Expert 8.06).
2.6 Molecular weight detection of PTM
The concentration of the dextran solution was 3 mg∙mL−1, the pump pressure was 3.5 bar, and the flow rate was 2.5 mL∙min−1. The elution volume of each glucan solution was recorded as Ve. dextran 2000 was treated in the same way, and the volume of blue glucan 2000 eluent was recorded as V0. Vt was the volume of Sephadex G-100. The K values of the different glucan solutions were then calculated from Equation 2.
2.7 HPLC analysis for PTM monosaccharides composition
Five mg of polysaccharide was dissolved in 2 mL of 2 M TFA and incubated at 100°C under sealed conditions for 2 h. The 5 mL methanol was added to the hydrolysate and concentrated by a rotary evaporator, and the processes were repeated three times to remove the excess TFA. Finally, the mixture was centrifuged at 15,000 rpm for 10 min and the supernatant was obtained.
The monosaccharide hydrolysate was analyzed by HPLC with an Agilent Amino column (4.6 × 25 cm) and a refractive index detector (RID) detector. Mobile phases A and B (v/v, 17:83) consisted of acetonitrile and phosphate solution, respectively. The flow rate was 1 mL/min, the temperature of the column was 35°C and the injection volume was 20 μL.
2.8 Fourier-transform infrared spectroscopy of PTM
Dried PTM was ground with KBr powder and then pressed into 1 mm pellets for spectroscopy measurements (Qian et al., 2009). The crude PTM was verified by the KBr pressed-disk method with an FT-IR spectrometer (IRPrestige−21, Shimadzu, Japan) in the wave number range 4,000–400 cm−1 (An et al., 2020). Each sample was measured thrice.
2.9 SEM analysis of PTM
For SEM analysis, purified PTM was placed overnight in a solution containing 3% glutaraldehyde (C5H8O2), 0.1 M sodium cacodylate [(CH3)2AsO2Na] at a pH of 7.4, and 5 mM calcium chloride (CaCl2), at 23°C. The sample was stained for 1 h at 23°C in 1% osmium tetroxide (OsO4) and 1% K3Fe(CN)6 in a cacodylate buffer and washed with double distilled water. The sample was dehydrated through 10 min incubations in a graded series: two incubations each in 30, 50, and 70% acetone, and four incubations in 90 and 100% acetone. The coverslips were dried to the critical point and sputter-coated with 4 nm of gold particles. Images were collected using a LEO1550 scanning electron microscope at 2.5 kV using an in-lens detector (Qian et al., 2009).
2.10 PTM antioxidant activity assay
The DPPH radical scavenging activity of PTM was measured according to a previously reported method (Xu et al., 2019). Two mL of PTM solution was mixed with 2.5 mL of DPPH. The mixture was incubated for 30 min at 25°C and the absorbance of the solution at 517 nm was recorded and compared with that of ascorbic acid (vitamin C) and double distilled water.
The superoxide anion radical (O2•−) scavenging activity of PTM was determined by using a previously reported method (Chen et al., 2021). PTM solutions (0.5 mL of different concentrations) were mixed with 0.5 mL of 25 mmol/L pyrogallol and 2 mL of 50 mmol/L Tris–HCl at 25°C for 5 min. The reaction was stopped by the addition of hydrochloric acid. The absorbance was recorded at 560 nm and vitamin C was used as a positive control.
The hydroxyl radical (OH•) scavenging activity of PTM was determined by a previously reported method (Devanthéry et al., 2020). A PTM solution of 1 mL was mixed with 1 mL of hydrogen peroxide (H2O2; 9 mmol/L), 1 mL of salicylic acid-ethanol (C7H6O3 + C2H5OH; 9 mmol/L), and 1 mL of ferrous sulfate (FeSO4; 9 mmol/L) solutions. The mixture was incubated for 6 min at 25°C, and the absorbance of the solution at 510 nm was recorded and compared with that of vitamin C and double distilled water.
The ABTS radical scavenging activity of PTM was determined by a previously reported method (Wu et al., 2020). Different concentrations of PTM were mixed with 3 mL ABTS solution. The mixture was incubated for 6 min at 25°C, and the absorbance of the solution at 510 nm was recorded and compared with that of vitamin C and double distilled water.
The scavenging activities above were determined from Equation 3.
The K value was set as the ordinate and log[M] was plotted on the abscissa as a standard curve, where M is the standard molecular weight of glucan. The molecular weight of PTM was determined in the same way.
2.11 Statistical analysis
The experimental design and RSM analysis were performed by Design Expert 8.06. All experiments were carried out thrice. The results were analyzed by SPSS19.0 software and reported as mean ± standard deviation of the three replicates. Models with p < 0.05 were considered to be statistically significant.
3 Results and discussion
3.1 Single-factor experiments
For an extraction time of 40 min, extraction power of 80 W, and extraction temperature of 40°C, the effect of the solid-to-liquid ratio on the PTM yield was studied (Figure 1A). When the ratio was increased from 1:10 to 1:15, the PTM yield increased from 16.32 to 16.64% and gradually decreased to 15.49% with a further increase in the ratio. At an appropriate solid-to-liquid ratio, polysaccharides are known to dissolve quickly under the action of ultrasonic waves (Ferarsa et al., 2018; Pandey et al., 2018). However, with a decrease in this ratio, the viscosity of the solution increased, making the dissolution of PTM slightly slower and more difficult. At the same time, for a relatively large ratio, the PTM in the solution would be diluted, reducing the extraction yield. To improve the PTM yield and reduce the solvent and ex-traction time simultaneously, the optimal solid-to-liquid ratio was chosen as 1:15.
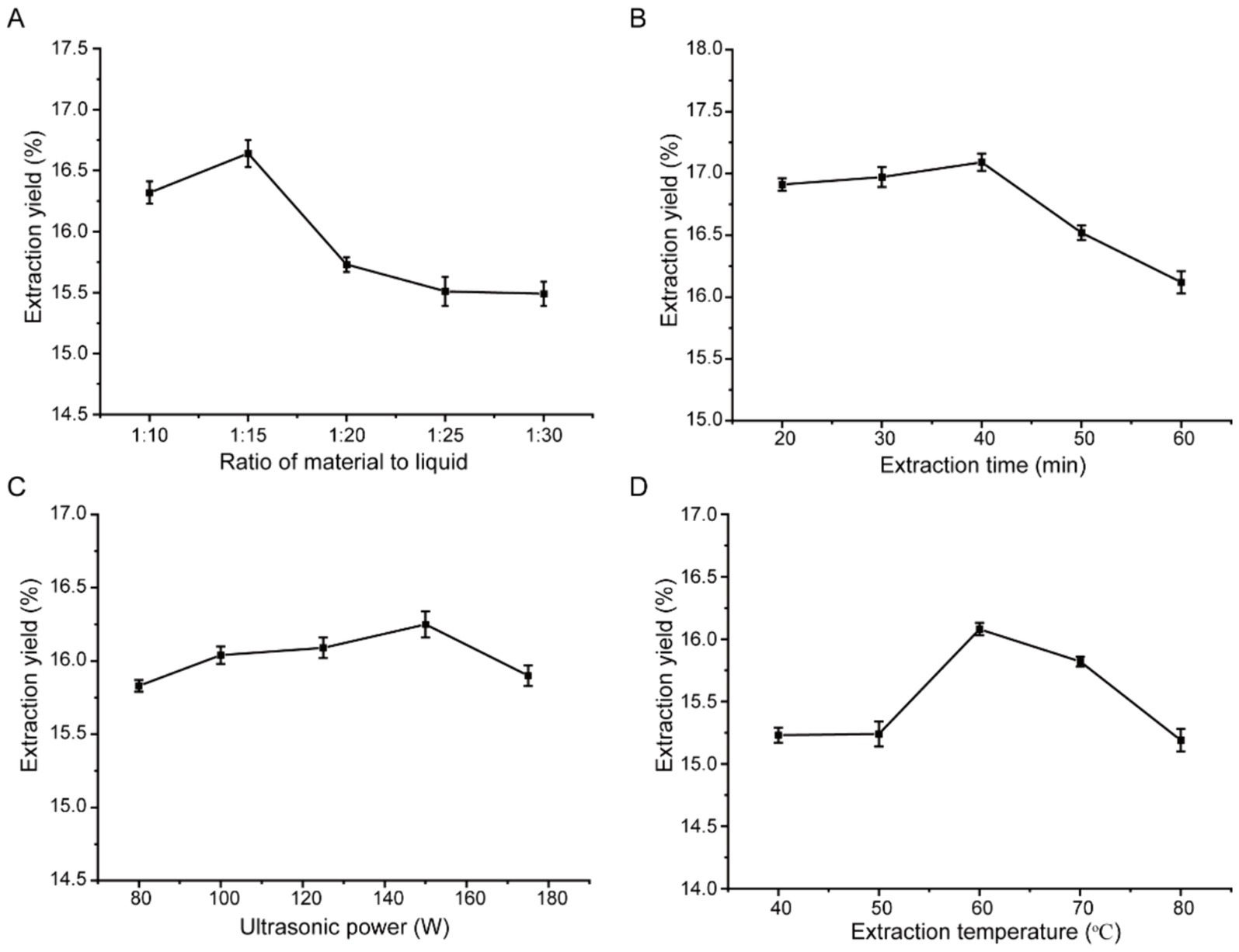
Figure 1. The influences of the solid-liquid ratio (A), extraction time (B), ultrasonic power (C), and temperature (D) on the yield of PTM.
For a solid-to-liquid ratio of 1:15, ultrasonic extraction power of 80 W, and extraction temperature of 40°C, the effect of extraction time on the PTM yield was studied (Figure 1B). When the extraction time was increased from 20 to 40 min, the PTM yield increased from 15.12 to 16.79%. However, the yield of polysaccharides decreases with a further increase of the ultrasound treatment time. Excessive ultrasound treatment May lead to the destruction of the PTM. Therefore, the optimal extraction time was chosen as 40 min.
For a solid-to-liquid ratio of 1:15, extraction time of 40 min, and extraction temperature of 40°C, the effect of the ultrasonic power on the PTM yield was studied (Figure 1C). When the ultrasonic power was increased from 80 to 150 W, the PTM yield increased from 15.23 to 16.31%; it decreased to 15.60% upon further increase of ultrasonic power. This effect occurred due to the strong ultrasonic cavitation, rapid breaking of smaller bubbles, and accelerated collision and diffusion of the reactants and products under high ultrasonic power (Ferreira et al., 2015). When the PTM concentration reached a certain threshold, a further increase in the ultrasonic power led to PTM degradation and a decrease in solution viscosity. This caused a reduction in the PTM yield. Therefore, the optimal ultrasonic power was chosen as 150 W.
For a solid-to-liquid ratio of 1:15, ultrasonic power of 150 W, and extraction time of 40 min, the effect of the extraction temperature on the PTM yield was studied (Figure 1D). When the extraction temperature was increased from 40°C to 60°C, the PTM yield increased from 15.32 to 16.54% and then decreased with further increase of the temperature. The increase in temperature will lead to the destruction and decomposition of polysaccharide structures, resulting in a decrease in their molecular weight and thus affecting the yield of polysaccharides. Therefore, the optimal extraction temperature was chosen as 60°C.
The extraction rate of polysaccharides is affected by extraction method, temperature, time, and solid–liquid ratio. Ultrasonic-assisted extraction can improve the yield of PTM compared with traditional hot-water extraction and cold-water extraction (Yin et al., 2014). In addition, excessive extraction temperature and ultrasonic power will affect the molecular weight of polysaccharides, that is, the detailed structure of polysaccharides, thus affecting the biological activity of polysaccharides, so it is necessary to optimize the extraction conditions of polysaccharides.
3.2 Response surface analysis
The testing conditions for the response surface design are listed in Table 1, and the different condition combinations and their PTM extraction yields are listed in Table 2. By multiple regression fitting and stepwise regression analysis of the data in Table 3, the regression model was defined as.
where A, B, C, D, and Y are the coded variables for the solid-to-liquid ratio, extraction time, ultrasonic power, extraction temperature, and PTM yield, respectively. The correlation coefficient reflected the real relationship between the factors and their response values. The R2 value of 0.9757 for this model showed that the experimental value of the PTM extraction yield was in good agreement with the predicted value. The p-value of the model was <0.001, implying the statistical significance of the multivariate quadratic model (regression) equation (Equation 4) and the reliability of the test. The p-value of the lack of fit term was 0.5025, indicating only a small proportion of abnormal error between the equation and the actual fit. Therefore, the model equation reflected the real experimental process. The model was then used to analyze and predict the changes in the PTM yield under different extraction conditions. The primary factors A, B, C, and D had significant effects on the PTM yield (p < 0.01). The quadratic terms AB, BC, and CD were eliminated through the stepwise analysis process as the interactions of AB (Figure 2A), BC (Figure 2D), and CD (Figure 2F) were small and had negligible effects on the PTM yield. In contrast, AC (Figure 2B), AD (Figure 2C), and BD (Figure 2E) had significant effects on the PTM yield. The trend of the response values was used to determine the significance of the interaction between every two variables; an ellipse and steep slope indicated significant interactions unlike a circle and gentle slope. Through the response surface diagram, it could be seen that the interaction between AC and AD was stronger than that of BD.
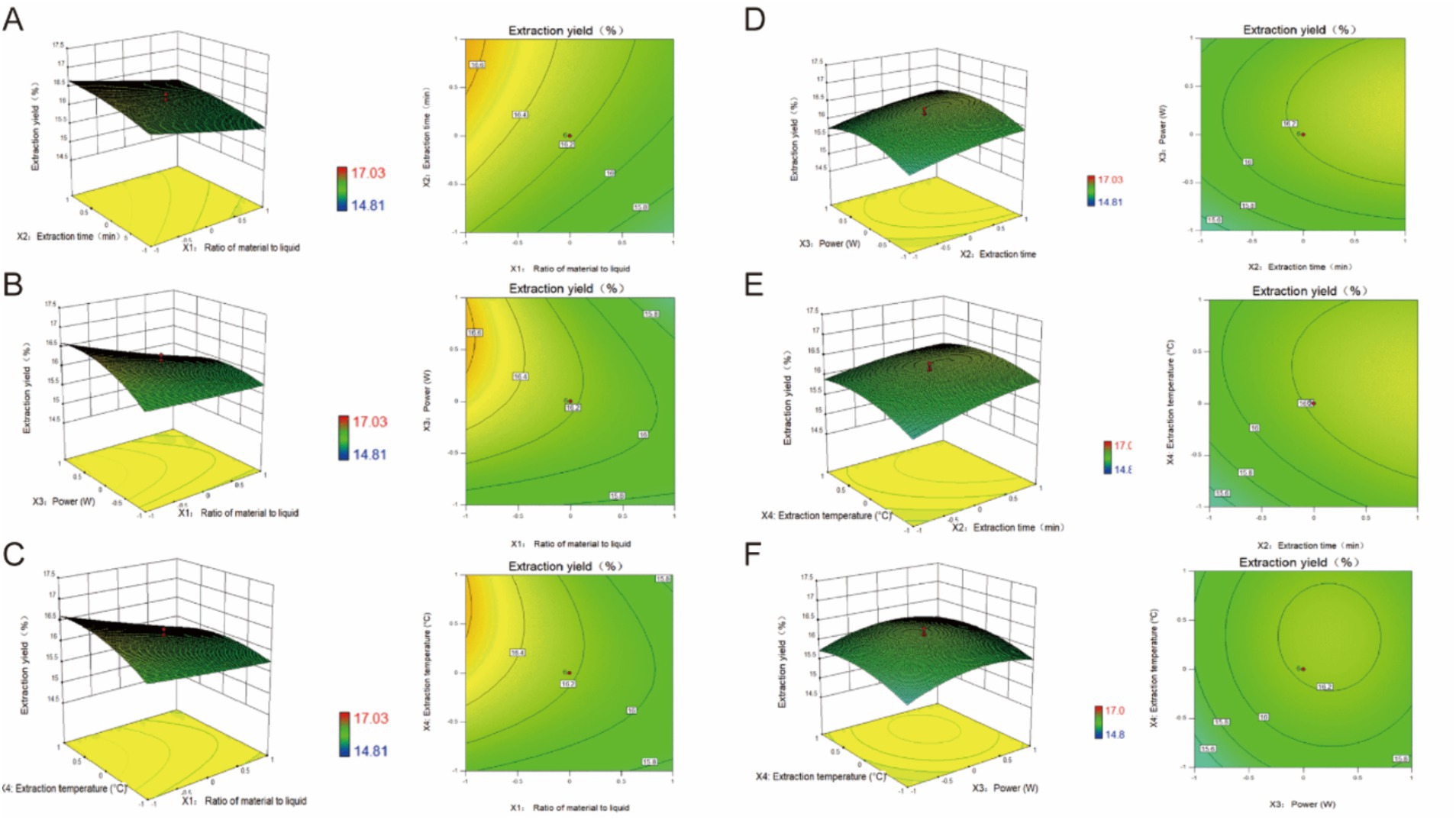
Figure 2. Response surface and contour plots. (A) Extraction time and solid-liquid ratio, (B) ultrasonic power and solid-liquid ratio, (C) extraction temperature and solid-liquid ratio, (D) ultrasonic power and extraction time, (E) extraction temperature and extraction time, (F) extraction temperature and ultrasonic power.
Through the model analysis, the optimum extraction conditions were obtained as follows: solid-to-liquid ratio of 1:15, extraction time of 50 min, ultrasonic power of 135.75 W, and extraction temperature of 65.5°C. In these conditions, the PTM yield reached 16.85%. Considering the feasibility of the operation, the ultrasonic power and extraction temperature were adjusted to 135 W and 65°C, respectively. In these conditions, three parallel experiments were carried out to verify the prediction model, and the PTM yield was found to be 16.97 ± 0.006%. The results showed that the model could predict the PTM yield well.
3.3 Molecular weight of the PTM
The standard curve equation was Y = 6.0117–5.7109X (R2 = 0.9901). The relative molecular weight of the PTM was 90,100 Da. The size of molecular weight is related to the activity of polysaccharides; however, this does not imply that the activity is proportional to the molecular weight (Ferreira et al., 2015). It is also necessary to analyze the biological activity of polysaccharides by combining the characteristics of monosaccharide composition and glycoside linkage. The molecular weight is closely related to the solubility and bioactivity of polysaccharides.
3.4 HPLC analysis for PTM monosaccharides composition
The commonly found monosaccharides and polysaccharides are shown in Figure 3A. The PTM were heteropolysaccharides composed of fructose, mannose, glucose, and galactose with a molar ratio of 1:2.25:9.35:5 (Figure 3B). The main chain of PTM May be composed of glucose and galactose, while fructose and mannose May be the constituent units of PTM branch chains. A similar result was obtained by Chen et al. (2017) and Wang et al. (2022) on similar polysaccharides. Some scholars have argued that the activity of polysaccharides depends on their composition. Polysaccharides with high glucose content have been reported to have strong immune activity, whereas those with a greater variety of monosaccharides have been shown to have high antioxidant activity (Li et al., 2019). However, these trends are not common to all studies and the specific relationships for PTM remain to be determined. PTM contains glucose and galactose, and the two monosaccharides can be separated by HPLC compared to GC. However, the overlapping spectra of glucose, galactose, mannose, and arabinose in 1H NMR quantification of monosaccharides affect the integrated quantitative analysis. Therefore, the monosaccharides composition in PTM was simply and effectively analyzed by HPLC using an amino column.
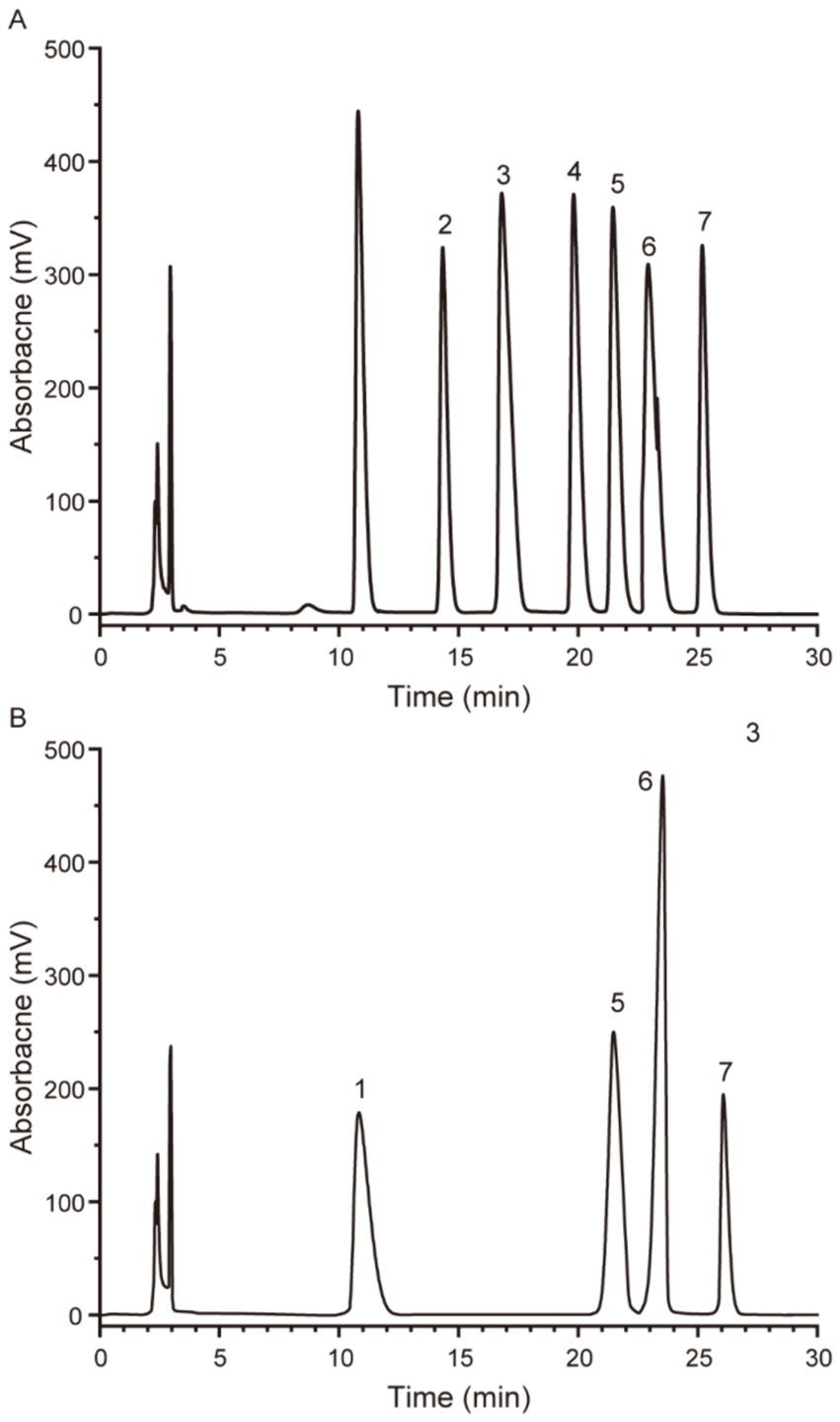
Figure 3. HPLC of monosaccharide standard (A) and PTM (B). 1: fructose; 2: rhamnose; 3: arabinose; 4: xylose; 5: mannose; 6: glucose; 7: galactose.
3.5 FT-IR analysis of PTM
The structural features of the PTM were further analyzed by FT-IR spectra (Figure 4). The polysaccharides had typical absorption peaks in the range of 4,000 cm−1 to 400 cm−1. A strong absorption peak appeared at around 3,400 cm−1, which was the stretching vibration peak of O–H in the sugar molecule. The peak at 2,939 cm−1 belonged to C–H stretching of CH2 and CH3 groups. Bands at both 1,740 cm−1 and 1,500 cm−1 were observed, corresponding to symmetric and asymmetric stretching, respectively, of C=O (Chen et al., 2017). The absorption peak near 1,200 cm−1 to 800 cm−1 was the characteristic absorption peak of the carbohydrate (Xie et al., 2020).
3.6 SEM analysis of PTM
SEM images of PTM (Figure 5) showed flake-like, irregular structures. Under 5,000× magnification, we observed large spacings irregular shapes, and various forms of fragmented stacking. The particle size was ~10 μm, with rod-shaped, flake-shaped, and spherical components, with a smooth surface and a small internal gap. The surface of the PTM was smooth with an intermolecular gap, possibly due to molecular repulsion.
3.7 In vitro antioxidant activities of PTM
Antioxidants scavenge DPPH radicals by donating electrons or hydrogen atoms to the DPPH to form stable DPPH-H compounds. This results in a noticeable change in the color of the mixture from purple to yellow, along with a decrease in absorbance (Ullah et al., 2017). For PTM, a maximum scavenging rate of 64.91% was achieved for a concentration of 1.8 mg/mL, compared with 99.6% for the vitamin C control (Figure 6A). Although the scavenging activity of PTM was slightly weaker than that of vitamin C, it works well in high concentrations. Thus, PTM has an appreciable scavenging capacity for DPPH radicals.
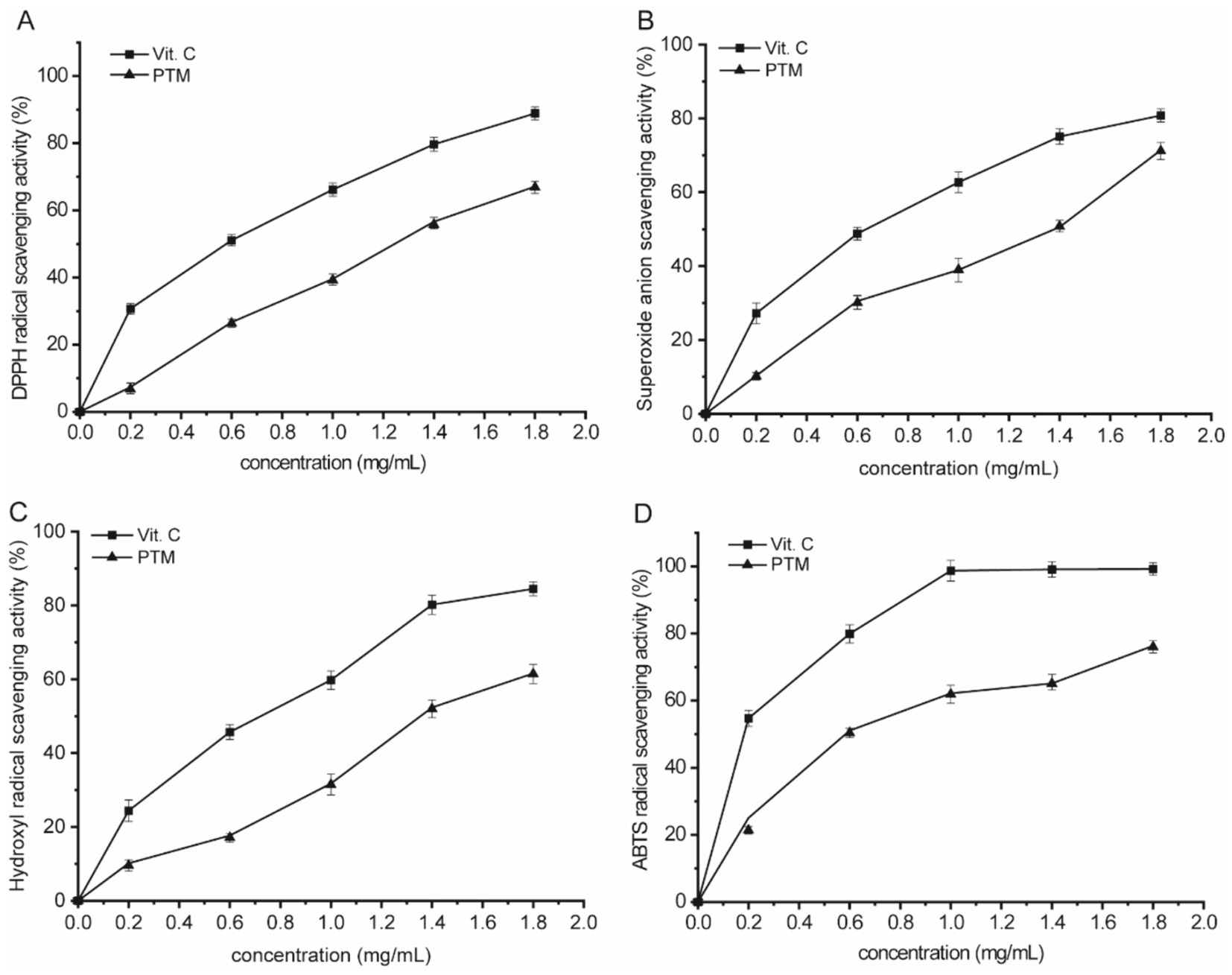
Figure 6. Antioxidant activities of PTM. (A) DPPH radical scavenging activity, (B) superoxide anion scavenging activity; (C) hydroxyl radical scavenging activity; and (D) ABTS radical scavenging activity.
There is a certain quantity of superoxide radicals, which are relatively weak oxidizers in the human body. These radicals are harmless before any chemical change, but superoxide radicals can interact with other molecules to generate secondary ROS such as reactive oxygen species (ROS) H2O2 and OH radicals, resulting in oxidation damage to lipids, proteins, and DNA (Dondurmacıoğlu et al., 2017). PTM was found to exhibit superoxide radical scavenging in a concentration-dependent manner (Figure 6B). The scavenging effect increased to 71.33% when the PTM concentration was increased to 1.8 mg/mL. Thus, PTM has a significant capacity to scavenge superoxide radicals.
Hydroxyl radicals are another representative ROS and are found in a variety of cell species. Because these radicals can easily inflict severe damage to cells or tissues (Chimi et al., 1991), their removal from the body is extremely important. Hydroxyl radical scavenging by PTM was measured based on the principle at Fenton reaction (Figure 6C). The scavenging rate increased with PTM concentration, reaching 60.99% at a concentration of 1.8 mg/mL. The potential antioxidant mechanism might be due to the capture of essential radical ions by PTM and termination of the radical chain reaction.
ABTS radical scavenging assay is commonly used to determine the total antioxidant activity of polysaccharides in natural products (Zhang et al., 2017). ABTS radical scavenging by PTM enhanced with the increase of PTM concentration (Figure 6D), reaching 76.79% at the concentration 1.8 mg/mL. The PTM antioxidant effects determined from the ABTS assay were positively correlated with those obtained from the DPPH radical assay, although the scavenging power of PTM was higher in the former assay. One can speculate that the ABTS assay might be more suitable than the DPPH assay for evaluating highly water-soluble polysaccharides.
4 Conclusion
In this study, we investigated a UAE technique for polysaccharide extraction from T. matsutake. Firstly, we assessed the four main factors significantly affecting PTM extraction yield—solid-to-liquid ratio, ultrasonic power, extraction time, and extraction temperature—through single-factor experiments. Then, an orthogonal, rotatable CCD was used to optimize the PTM extraction. The second-order regression equation provides a satisfactory prediction of the experimental data. The optimal conditions were chosen as follows: solid-to-liquid ratio of 1:15, extraction time 50 min, ultrasonic power of 135 W, and extraction temperature 65°C. In these conditions, the PTM extraction yield was 16.97%, which corresponded well with the predicted yield value of 16.85%. The extracted PTM showed high hydroxyl radical, ABTS and DPPH scavenging activity in vitro and PTM could be applied as a novel natural antioxidant drug. Further work is being conducted on the physicochemical characteristics, structural characteristics, and biological functions of PTM.
Data availability statement
The raw data supporting the conclusions of this article will be made available by the authors, without undue reservation.
Ethics statement
The manuscript presents research on animals that do not require ethical approval for their study.
Author contributions
C-JX: Funding acquisition, Methodology, Project administration, Visualization, Writing – original draft. Y-WY: Formal analysis, Investigation, Writing – original draft. YL: Investigation, Methodology, Supervision, Writing – original draft. YZ: Formal analysis, Software, Writing – original draft. DL: Data curation, Resources, Writing – original draft. Z-YZ: Conceptualization, Formal analysis, Writing – review & editing. NZ: Investigation, Resources, Writing – original draft. HX: Conceptualization, Supervision, Writing – original draft.
Funding
The author(s) declare that financial support was received for the research, authorship, and/or publication of this article. This work was supported by Sichuan Science and Technology Department Regional Innovation Cooperation Project [Grant No. 24QYCX0265], Sichuan Tourism University Annual High level Research Project Cultivation Special Project [Grant No. 2023PY02], the Science and Technology Planning Project of Corps-Scientific and Technological Research in Key Areas of Corps [Grant No. 2018AB014], Xinjiang Academy of Agricultural and Reclamation Science Oil Deep Processing and Nutritional Safety Innovation Team [Grant No. NCG202301], and the Development and Utilization of Characteristic Horticultural Biological Resources (Open Project of Key Laboratories in Sichuan Provincial Universities) [Grant No. 2023TSYY-08].
Acknowledgments
The authors are grateful to Sichuan Tourism University, Industrial College of Prepared Dishes, Sichuan Tourism University, and Department of Food Science, University of Massachusetts for providing materials, equipment, and support.
Conflict of interest
The authors declare that the research was conducted in the absence of any commercial or financial relationships that could be construed as a potential conflict of interest.
Publisher’s note
All claims expressed in this article are solely those of the authors and do not necessarily represent those of their affiliated organizations, or those of the publisher, the editors and the reviewers. Any product that may be evaluated in this article, or claim that may be made by its manufacturer, is not guaranteed or endorsed by the publisher.
References
An, Q., Ye, X., Han, Y., Zhao, M., Chen, S., Liu, X., et al. (2020). Structure analysis of polysaccharides purified from Cyclocarya paliurus with DEAE-cellulose and its antioxidant activity in RAW264. 7 cells. Int. J. Biol. Macromol. 157, 604–615. doi: 10.1016/j.ijbiomac.2019.11.212
Chen, Y., Du, X. J., Zhang, Y., Liu, X. H., and Wang, X. D. (2017). Ultrasound extraction optimization, structural features, and antioxidant activity of polysaccharides from tricholoma matsutake. J. Zhejiang Univ. Sci. B (Biomed. Biotechnol.) 18, 674–684. doi: 10.1631/jzus.B1600239
Chen, X., Sun-Waterhouse, D., Yao, W., Li, X., Zhao, M., and You, L. (2021). Free radical-mediated degradation of polysaccharides: mechanism of free radical formation and degradation, influence factors and product properties. Food Chem. 365:130524. doi: 10.1016/j.foodchem.2021.130524
Chimi, H., Cillard, J., Cillard, P., and Rahmani, M. (1991). Peroxyl and hydroxyl radical scavenging activity of some natural phenolic antioxidants. J. Am. Oil Chem. Soc. 68, 307–312. doi: 10.1007/BF02657682
Cui, D., Zhao, D., and Huang, S. (2019). Beneficial contribution of a safflower (Carthamus tinctorius L.) polysaccharide on steroid-induced avascular necrosis of the femoral head in rats. Int. J. Biol. Macromol. 123, 581–586. doi: 10.1016/j.ijbiomac.2018.11.046
Devanthéry, T., Nakamura, K., Hashiguchi, S., and Hashimoto, M. (2020). Structure of a heptose-containing polysaccharide derived from Komagataeibacter europaeus NBRC 3261. Carbohydr. Res. 492:107989. doi: 10.1016/j.carres.2020.107989
Dondurmacıoğlu, F., Avan, A. N., and Apak, R. (2017). Simultaneous detection of superoxide anion radicals and determination of the superoxide scavenging activity of antioxidants using a N, N-dimethyl-p-phenylene diamine/Nafion colorimetric sensor. Anal. Methods 9, 6202–6212. doi: 10.1039/C7AY02132A
Ferarsa, S., Zhang, W., Moulai-Mostefa, N., Ding, L., Jaffrin, M. Y., and Grimi, N. (2018). Recovery of anthocyanins and other phenolic compounds from purple eggplant peels and pulps using ultrasonic-assisted extraction. Food Bioprod. Process. 109, 19–28. doi: 10.1016/j.fbp.2018.02.006
Ferreira, S. S., Passos, C. P., Madureira, P., Vilanova, M., and Coimbra, M. A. (2015). Structure–function relationships of immunostimulatory polysaccharides: a review. Carbohydr. Polym. 132, 378–396. doi: 10.1016/j.carbpol.2015.05.079
Hu, J. L., Nie, S. P., and Xie, M. Y. (2018). Antidiabetic mechanism of dietary polysaccharides based on their gastrointestinal functions. J. Agric. Food Chem. 66, 4781–4786. doi: 10.1021/acs.jafc.7b05410
Hu, H., Zhao, Q., Pang, Z., Xie, J., Lin, L., and Yao, Q. (2018). Optimization extraction, characterization and anticancer activities of polysaccharides from mango pomace. Int. J. Biol. Macromol. 117, 1314–1325. doi: 10.1016/j.ijbiomac.2018.05.225
Li, M., Dong, L., Du, H., Bao, Z., and Lin, S. (2021). Potential mechanisms underlying the protective effects of Tricholoma matsutake singer peptides against LPS-induced inflammation in RAW264. 7 macrophages. Food Chem. 353:129452. doi: 10.1016/j.foodchem.2021.129452
Li, J., Wang, D., Xing, X., Cheng, T. J. R., Liang, P. H., Bulone, V., et al. (2019). Structural analysis and biological activity of cell wall polysaccharides extracted from Panax ginseng marc. Int. J. Biol. Macromol. 135, 29–37. doi: 10.1016/j.ijbiomac.2019.05.077
Medlej, M. K., Cherri, B., Nasser, G., Zaviska, F., Hijazi, A., Li, S., et al. (2020). Optimization of polysaccharides extraction from a wild species of Ornithogalum combining ultrasound and maceration and their anti-oxidant properties. Int. J. Biol. Macromol. 161, 958–968. doi: 10.1016/j.ijbiomac.2020.06.021
Pandey, A., Belwal, T., Sekar, K. C., Bhatt, I. D., and Rawal, R. S. (2018). Optimization of ultrasonic-assisted extraction (UAE) of phenolics and antioxidant compounds from rhizomes of Rheum moorcroftianum using response surface methodology (RSM). Ind. Crop. Prod. 119, 218–225. doi: 10.1016/j.indcrop.2018.04.019
Qian, J. Y., Chen, W., Zhang, W. M., and Zhang, H. (2009). Adulteration identification of some fungal polysaccharides with SEM, XRD, IR and optical rotation: a primary approach. Carbohydr. Polym. 78, 620–625. doi: 10.1016/j.carbpol.2009.05.025
Quinlan, P. J., Tanvir, A., and Tam, K. C. (2015). Application of the central composite design to study the flocculation of an anionic azo dye using quaternized cellulose nanofibrils. Carbohydr. Polym. 133, 80–89. doi: 10.1016/j.carbpol.2015.06.095
Ren, M., Ye, L., Hao, X., Ren, Z., Ren, S., Xu, K., et al. (2014). Polysaccharides from Tricholoma matsutake and Lentinus edodes enhance 5-fluorouracil-mediated H22 cell growth inhibition. J. Tradit. Chin. Med. 34, 309–316. doi: 10.1016/S0254-6272(14)60095-9
Saqib, A. A. N., and Whitney, P. J. (2011). Differential behaviour of the dinitrosalicylic acid (DNS) reagent towards mono-and di-saccharide sugars. Biomass Bioenergy 35, 4748–4750. doi: 10.1016/j.biombioe.2011.09.013
Ullah, F., Iqbal, N., Ayaz, M., Sadiq, A., Ullah, I., Ahmad, S., et al. (2017). DPPH, ABTS free radical scavenging, antibacterial and phytochemical evaluation of crude methanolic extract and subsequent fractions of Chenopodium botrys aerial parts. Pak. J. Pharm. Sci. 30, 761–766
Wang, G. L., Li, J. Y., Wang, Y., Chen, Y., and Wen, Q. L. (2022). Extraction, structure and bioactivity of polysaccharides from Tricholoma matsutake (S. Ito et Imai) singer. Appl. Biochem. Microbiol. 58, 375–381. doi: 10.1134/S0003683822040184
Wu, Q., Luo, M., Yao, X., and Yu, L. (2020). Purification, structural characterization, and antioxidant activity of the COP-W1 polysaccharide from Codonopsis tangshen Oliv. Carbohydr. Polym. 236:116020. doi: 10.1016/j.carbpol.2020.116020
Xie, L., Shen, M., Wen, P., Hong, Y., and Xie, J. (2020). Preparation, characterization, antioxidant activity and protective effect against cellular oxidative stress of phosphorylated polysaccharide from cyclocarya paliurus. Food Chem. Toxicol. 145:111754. doi: 10.1016/j.fct.2020.111754
Xu, G. Y., Liao, A. M., Huang, J. H., Zhang, J. G., Thakur, K., and Wei, Z. J. (2019). Evaluation of structural, functional, and anti-oxidant potential of differentially extracted polysaccharides from potatoes peels. Int. J. Biol. Macromol. 129, 778–785. doi: 10.1016/j.ijbiomac.2019.02.074
Yang, X., Ji, H., Feng, Y., Yu, J., and Liu, A. (2018). Structural characterization and antitumor activity of polysaccharides from Kaempferia galanga L. Oxidative Med. Cell. Longev. 2018:9579262. doi: 10.1155/2018/9579262
Yin, X., You, Q., and Jiang, Z. (2011). Optimization of enzyme assisted extraction of polysaccharides from Tricholoma matsutake by response surface methodology. Carbohydr. Polym. 86, 1358–1364. doi: 10.1016/j.carbpol.2011.06.053
Yin, X., You, Q., and Su, X. (2014). A comparison study on extraction of polysaccharides from tricholoma matsutake by response surface methodology. Carbohydr. Polym. 102, 419–422. doi: 10.1016/j.carbpol.2013.11.072
You, L., Gao, Q., Feng, M., Yang, B., Ren, J., Gu, L., et al. (2013). Structural characterisation of polysaccharides from Tricholoma matsutake and their antioxidant and antitumour activities. Food Chem. 138, 2242–2249. doi: 10.1016/j.foodchem.2012.11.140
Yuan, Q., Lin, S., Fu, Y., Nie, X. R., Liu, W., Su, Y., et al. (2019). Effects of extraction methods on the physicochemical characteristics and biological activities of polysaccharides from okra (Abelmoschus esculentus). Int. J. Biol. Macromol. 127, 178–186. doi: 10.1016/j.ijbiomac.2019.01.042
Zeng, X., Li, P., Chen, X., Kang, Y., Xie, Y., Li, X., et al. (2019). Effects of deproteinization methods on primary structure and antioxidant activity of Ganoderma lucidum polysaccharides. Int. J. Biol. Macromol. 126, 867–876. doi: 10.1016/j.ijbiomac.2018.12.222
Zhang, N., Yin, H., Liu, R., Yu, M., Li, T., and Wang, Z. (2017). Forest, U.N. ABTS radical scavenging activity of Theaflavins combined with fungal polysaccharides. Mod. Food Technol. 33, 21–28. doi: 10.13982/j.mfst.1673-9078.2017.2.004
Zhao, P., Zhou, H., Zhao, C., Li, X., Wang, Y., Huang, L., et al. (2019). Purification, characterization and immunomodulatory activity of fructans from Polygonatum odoratum and P. cyrtonema. Carbohydr. Polym. 214, 44–52. doi: 10.1016/j.carbpol.2019.03.014
Zheng, Q., Ren, D., Yang, N., and Yang, X. (2016). Optimization for ultrasound-assisted extraction of polysaccharides with chemical composition and antioxidant activity from the Artemisia sphaerocephala Krasch seeds. Int. J. Biol. Macromol. 91, 856–866. doi: 10.1016/j.ijbiomac.2016.06.042
Keywords: Tricholoma matsutake , polysaccharides, ultrasound-assisted extraction, structural characteristics, antioxidant activity
Citation: Xu C-J, Yi Y-W, Liu Y, Zhou Y, Lin D, Zhu Z-Y, Zhu N and Xiao H (2024) Ultrasound-assisted optimized extraction and analysis of polysaccharides of Tricholoma matsutake. Front. Sustain. Food Syst. 8:1433565. doi: 10.3389/fsufs.2024.1433565
Edited by:
Dibya Jyoti Hazarika, AAU-Zonal Research Station, Gossaigaon, IndiaReviewed by:
Nishant Rachayya Swami Hulle, Tezpur University, IndiaVasiliki Kachrimanidou, Ionian University, Greece
Copyright © 2024 Xu, Yi, Liu, Zhou, Lin, Zhu, Zhu and Xiao. This is an open-access article distributed under the terms of the Creative Commons Attribution License (CC BY). The use, distribution or reproduction in other forums is permitted, provided the original author(s) and the copyright owner(s) are credited and that the original publication in this journal is cited, in accordance with accepted academic practice. No use, distribution or reproduction is permitted which does not comply with these terms.
*Correspondence: Zhong-Yan Zhu, emh1emhvbmd5YW4xMjNAMTYzLmNvbQ==
†These authors have contributed equally to this work